- 1The Seventh Clinical Medical College of Guangzhou University of Chinese Medicine, Shenzhen, Guangdong, China
- 2Shenzhen Bao’an Chinese Medicine Hospital, Guangzhou University of Chinese Medicine, Shenzhen, Guangdong, China
Background: The oxidative balance score (OBS) is a composite indicator that evaluates the balance between pro-oxidants and antioxidants in one’s diet and lifestyle. However, the relationship between OBS and circadian syndrome (CircS) has remained unexplored. This investigation aimed to determine a correlation between OBS and CircS.
Methods: This population-based study examined 7,202 participants from the 2005 to 2018 National Health and Nutrition Examination Survey (NHANES), 1,433 of whom had CircS. We utilized weighted multivariate logistic regression, trend tests, subgroup analysis, and interaction tests to evaluate the correlation between OBS (total OBS, dietary OBS, and lifestyle OBS) and CircS. Restricted cubic splines (RCS) models and threshold effect analysis were used to explore nonlinear relationships.
Results: Multivariate logistic regression analysis indicated that the protective factor for CircS was a high OBS level (total OBS: Odds ratio (OR) = 0.95, 95% Confidence interval (CI): 0.93-0.97; dietary OBS: OR = 0.98, 95% CI: 0.96-1.00; lifestyle OBS: OR = 0.65, 95% CI: 0.61-0.69). Compared to the quartile 1 group, OBS (total OBS, dietary OBS, and lifestyle OBS) was negatively and statistically significantly associated with the risk of developing Circs in the quartile 4 group (total OBS: OR = 0.47, 95% CI: 0.32-0.70; dietary OBS: OR = 0.69, 95% CI: 0.48-0.99; lifestyle OBS: OR = 0.07, 95% CI: 0.04-0.11). According to subgroup analysis and interaction tests, there was an interaction effect between the association of lifestyle OBS and CircS in terms of education level (p for interaction = 0.01). Furthermore, we observed a nonlinear negative relationship between lifestyle OBS and CircS prevalence, with inflection points at 6 (p for nonlinearity = 0.002).
Conclusion: The results showed a substantial negative connection between OBS and CircS. Encouraging foods filled with antioxidants and antioxidant-rich lifestyles may reduce the risk of CircS.
Introduction
Metabolic syndrome (MetS) is a set of cardiometabolic risk elements and comorbidities, including hypertension, abdominal obesity, insulin resistance, and dyslipidemia (1). It represents a substantial risk factor in the progression of atherosclerotic cardiovascular disease (2). MetS is becoming a global epidemic, affecting more than one in every five adults (3). Nevertheless, the common cause of MetS is still not fully comprehended (4). Circadian rhythm disturbance has become more prevalent in recent years due to factors like night lighting, social jet lag, meal timing, and shift or night shift work (5–8). An increasing amount of evidence supports that circadian rhythm disruption is strongly associated with MetS (9–11). In this situation, a new concept closely related to circadian rhythms, called circadian syndrome, has emerged (12).
The primary diagnostic criteria for CircS include elevated blood pressure, dyslipidemia, abdominal obesity, elevated blood glucose, short sleep, and depressive state (12). All of these symptoms are influenced by the circadian clock, implying that circadian rhythm disturbances could be a common cause (13–17). CircS affects around 40.8% of individuals in the United States (18). A comparable incidence of around 39% has been discovered among Chinese adults (19). Furthermore, studies have found that CircS is linked to a higher risk of stroke, kidney stones, overactive bladder syndrome, and chronic renal disease (20–23). The increased prevalence of CircS and its negative consequences emphasize the importance of early prevention and evaluation.
Oxidative stress is regarded as a predominant etiological factor in the occurrence of many diseases, and it causes an unbalanced between oxidant and antioxidant defenses in the body through the generation of reactive substances and redox signaling (24–26). Moderate antioxidant consumption has been demonstrated to modulate oxidative homeostasis and the levels of oxidative stress in the body (27, 28). In addition, Unhealthy lifestyles (e.g., smoking, alcohol misuse, and insufficient physical activity) have been recognized to disrupt redox homeostasis (29–31). OBS is a comprehensive metric that considers both pro-oxidants and antioxidants in one’s diet and lifestyle (32). Higher OBS reflects a lower level of oxidative stress (33). Some epidemiologic studies have identified a link between OBS and an increased rate of developing diabetes, cardiovascular disease, and stroke (34–36). However, no studies have examined whether OBS has affects CircS. As a result, We conducted a population-based study to assess the connection between OBS and CircS.
Data and participants
NHANES is a program that conducts surveys every two years to estimate the nutritional status and health of children and adults in the United States. All participants grant their consent after being fully informed. Our analysis included data from seven successive NHANES cycles from 2005 to 2018, with a total of 70,190 people. We applied some exclusion standards: 1) Participants under the age of 20 (n = 30441); 2) missing CircS component data: waist circumference (WC, n = 3860), blood pressure (n = 1043), blood glucose (n = 18615), high-density lipoprotein cholesterol (HDL-C, n = 152), triglycerides (TG, n = 155), sleep (n = 34), and depression (n = 1046); 3) Missing OBS component data: inconsistent or missing dietary data (n=394), body mass index (BMI, n=35), serum cotinine (n = 7), and physical activity (n = 5734); 4) Covariates: serological markers (n = 47), low-density lipoprotein cholesterol (LDL-C, n = 146), education level (n = 5), PIR (n = 640), and marital status (n = 1); 5) Pregnancy (n = 153); 6) Unreliable energy intake (defined as men consuming < 800 kcal per day and > 4,200 kcal per day, and women consuming < 500 kcal per day and > 3,500 kcal per day) (n = 480). Our final study population consisted of 7,202 people (Figure 1).
OBS and CircS definitions
The OBS is composed of 20 components, including 16 dietary and 4 lifestyle elements. The OBS was divided into tertiles according to gender differences, using previous studies’ allocation methodologies (37). Antioxidants were ranked from lowest to highest tertile, with 0, 1, and 2 scores. In contrast, ratings of 2 and 0 represent the lowest and highest pro-oxidant tertiles, respectively. Physical activity and body measures were allocated based on the metabolic equivalent (MET) score and BMI. Serum cotinine, a nicotine metabolite, was utilized as a biomarker to assess smoking. In terms of alcohol consumption, no alcohol intake was considered a nondrinker. Alcohol intake exceeds 30 g per day for males and 15 g per day for females were considered heavy drinkers. Alcohol consumption was categorized as nondrinkers, moderate drinkers, or heavy drinkers, with 0, 1, and 2 scores. Table 1 shows the scheme for assigning OBS scores.
CircS comprises elements of metabolic syndrome, short sleep, and depressive state. The diagnosis relies on the subsequent standards: (1) WC equal to or exceeding 102 cm in males and 88 cm in females, (2) TG equal to or exceeding 1.7 mmol/L or previous recommendation by a physician to take lipid-lowering medications, (3) HDL-C levels below 1.0 mmol/L in males and below 1.3 mmol/L in females or previous recommendation by a physician to take lipid-lowering medications, (4) Systolic blood pressure (SBP) equal to or greater than 135 mmHg or diastolic blood pressure (DBP) equal to or greater than 85 mmHg, or use of antihypertensive drugs, (5) Fasting blood glucose (FBG) equal to or exceeding 100 mg/dL or use of hypoglycemic drugs and insulin, (6) sleeping less than 6 hours, and (7) Depression scores equal to or greater than 9 on the Health Questionnaire. Participants diagnosed with CircS exhibited four or more of these seven components.
Covariates
Based on previous research (38, 39), we included a range of covariates that may be associated with CircS. Covariates consisted of age (20–39, 40–65, or ≥65 years), gender (male or female), race (Mexican American, Non-Hispanic White, Non-Hispanic Black others), marital status (married or partnered, never married, widowed or divorced, separated), education attainment (below high school, high school, above high school), and PIR (≤1.3, 1.3–3.5, or >3.5). Energy intake, LDL–C, serum cholesterol, uric acid (UA), creatinine (Cr), aspartate aminotransferase (AST), alanine aminotransferase (ALT), and gamma-glutamyl transferase (GGT).
Statistical analyses
All data were analyzed with the NHANES complex weighted sampling design. The OBS quartile stratified the study population’s baseline characteristics (Q1: < 25th percentile; Q2: 25–50th percentile; Q3: 50–75th percentile; Q4: ≥ 75th percentile). Weighted means and standard deviations are utilized to calculate constant variables, whereas weighted percentages are employed to calculate categorical variables. We utilized chi-square and t-tests to analyze differences within the OBS quartile classifications. The correlation between OBS and CircS was investigated in four distinct models using multivariable logistic regression. Model 1 was free of any covariate components. Model 2 included modifications for age, gender, and race. Model 3 included modifications for age, gender, race, marital status, education attainment, PIR, and energy intake. Model 4 was additionally adjusted for serum cholesterol, LDL-C, ALT, AST, Cr, GGT, and UA based on Model 3. Dietary OBS and lifestyle OBS were calculated according to gender differences to examine the robustness of the correlation between OBS and CircS. Then, stratified analyses and interaction tests were performed based on age, gender, race, marital status, education attainment, and PIR. In the end, RCS analysis and threshold effect analysis were applied to investigate the non-linear relationship between OBS and the risk of CircS. All the analyses were conducted employing R (version 4.3.2), with a statistically significant p-value < 0.05.
Results
General characteristics of the participants
7702 individuals were enrolled in our study, with a mean age of 46.31 ± 16.65 years. Among these, 50.12% were males, and the prevalence of CircS was found to be 26.92%. OBS was classified into four categories based on gender differences: Q1, Q2, Q3, and Q4. The corresponding sample sizes were as follows: 2016, 1865, 1593, and 1728. The range of scores for the following groups: Q1 had a range of <15 points, Q2 had a range of 15-20 points, Q3 had a range of 21-25 points, and Q4 had a range of >25 points. The proportion of people diagnosed with CircS declined as the quartiles increased. People in the top quartile of OBS were inclined to be 40-65 years old, female, non-Hispanic white, have an education level above high school, have a PIR greater than 3.5, be married or live with a partner, consume more energy, and have lower levels of GGT, AST, ALT, and UA. Furthermore, in the top quartile of OBS, the CircS seven diagnostic components were the least prevalent. Table 2 depicts the population’s baseline characteristics and variables categorized by the OBS quartile.
Relationship between total OBS and CircS
As shown in Table 3, weighted logistic regression models were employed to investigate the correlation between total OBS and CircS. When total OBS was measured as a continuous variable, there was a significant correlation between higher OBS and lower prevalence of CircS. The negative correlation between total OBS and CircS was also present even after adjustment for covariates (model 4), implying a 5% decrease in the prevalence of CircS for each unit increase in total OBS. (OR = 0.95, 95% CI: 0.93-0.97, p < 0.001). We further converted OBS into a quartile variable for sensitivity analysis. With individuals in the Q1 group as a reference, the reduction in the risk of developing CircS was statistically significant for those in groups Q3 and Q4 (p < 0.05). In the fully adjusted model (model 4), the ratio of individuals in groups Q3 and Q4 developing CircS was 0.61 (95% CI: 0.44- 0.84) and 0.47 (95% CI: 0.32 - 0.70), respectively.
Association of dietary OBS and lifestyle OBS with CircS
As shown in Table 4, dietary OBS and lifestyle OBS were both negatively associated with CircS in Model 4. For each unit increase in dietary OBS, the risk of CircS would be reduced by 2% (OR = 0.98, 95% CI: 0.96-1.00, p = 0.02). Lifestyle OBS seemed more closely related to the risk of CircS than dietary OBS. For each unit increase in lifestyle OBS, the risk of CircS would be reduced by 35% (OR = 0.65, 95% CI: 0.61-0.69, p < 0.001). We transformed dietary OBS and lifestyle OBS into categorical variables. In all four models, there was a statistically significant negative correlation between lifestyle OBS and CircS in the other three groups, using the Q1 group as a reference (p < 0.001). There was also a negative correlation observed between dietary OBS and CircS. This relationship was statistically significant in the Q4 group (model 1: OR = 0.56, 95% CI: 0.42-0.74, p < 0.001; model 2: OR = 0.57, 95% CI: 0.43-0.77, p < 0.001; model 3: OR = 0.56, 95% CI: 0.40-0.79, p = 0.001; model 4: OR = 0.69, 95% CI: 0.48-0.99, p = 0.046). According to interaction tests, no interaction effect was identified between dietary OBS and lifestyle OBS (p for interaction = 0.133).
Subgroup analysis and interaction tests for total OBS and CircS
We applied subgroup analysis and interaction tests according to age, gender, race, education attainment, and marital status. The outcomes of subgroup analyses revealed that the association between total OBS and CircS varied among groups. Participants exhibited substantial differences in subgroups divided by age, gender, education attainment, PIR, and marital status (P < 0.05). A negative relationship was also observed among the Mexican American population in the ethnicity stratification, but this relationship was not statistically significant (p = 0.4). Interaction tests found that total OBS did not correlate with age, gender, race, education attainment, PIR, and marital status. Figure 2 displays the association between CircS and total OBS in various subgroups in model 4.
Subgroup analysis and interaction tests of dietary OBS and lifestyle OBS with CircS
Subgroup analysis exploring the connection between dietary OBS and CircS identified differences in the following categories: females, aged 20-39, below high school, individuals of other ethnic backgrounds, married or cohabiting individuals, and those with a PIR of less than or equal to. Subgroup analysis exploring the correlation between lifestyle OBS and CircS revealed that all subgroups observed differences that are statistically significant (p < 0.001). The interaction test proved an interaction effect between lifestyle OBS and education attainment (p for interaction = 0.01). Higher levels of education were associated with a more significant correlation between lifestyle OBS and CircS (OR = 0.61, 95% CI: 0.56-0.66, p < 0.001). The subgroup analysis and interaction tests for OBS (total OBS, dietary OBS, and lifestyle OBS) and CircS are described in detail in Supplementary Tables 1-3.
Restricted cubic spline analysis
Adjusting for all confounders, RCS curves were utilized to further explore the connection between OBS (total OBS, dietary OBS, and lifestyle OBS) and CircS. We found a linear correlation between OBS(total OBS and dietary OBS) and CircS (total OBS: p = 0.117; dietary OBS: p = 0.486). A nonlinear negative connection was identified between lifestyle OBS and CircS (p = 0.002). Figure 3 displays the results of the analysis of RCS.
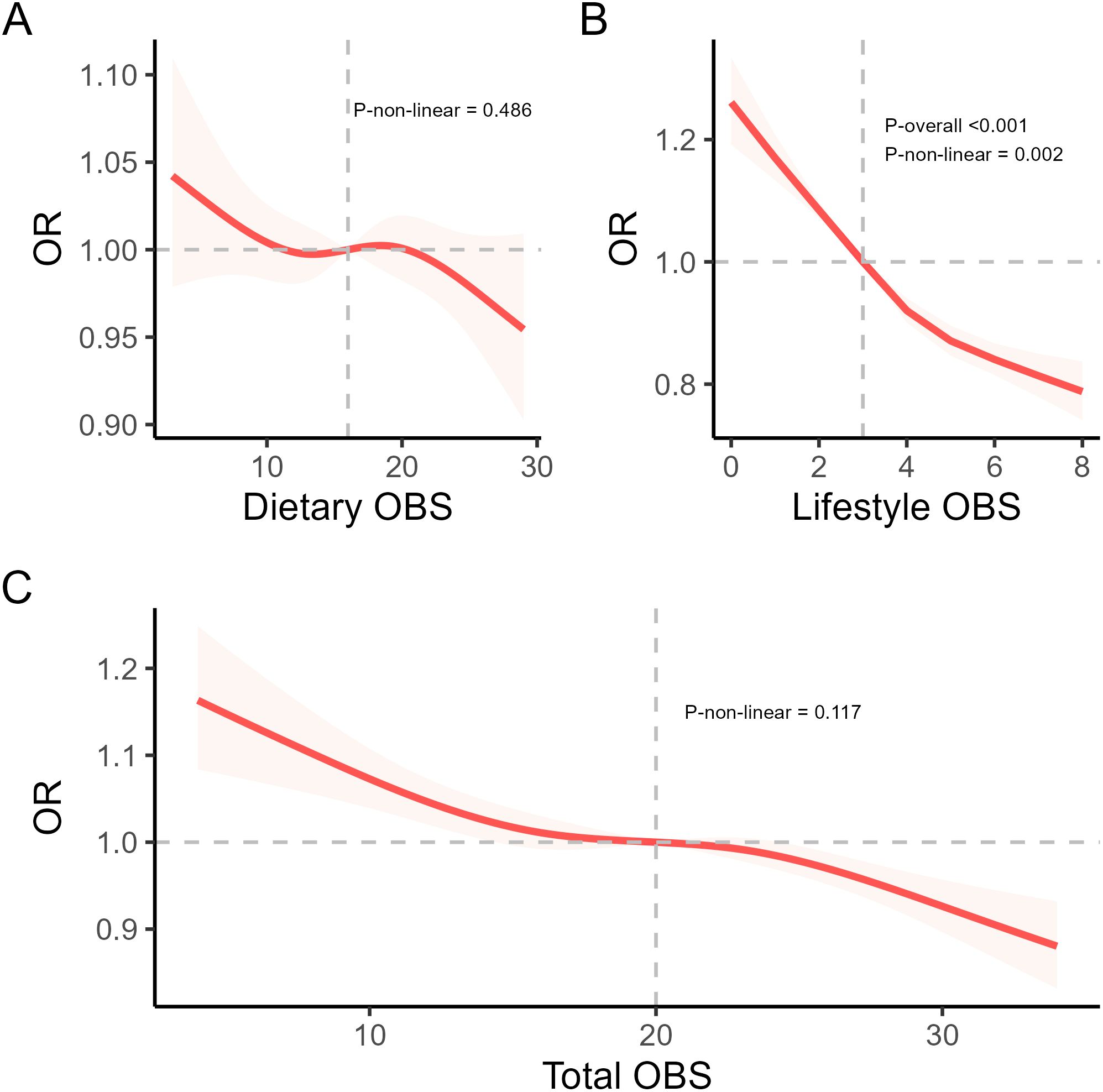
Figure 3. The RCS analysis of OBS and CircS. (A) a linear negative correlation between dietary OBS and CircS. (B) a non-linear negative correlation between lifestyle OBS and CircS. (C) a linear negative correlation between total OBS and CircS.
Furthermore, the inflection point for this nonlinear relationship between lifestyle OBS and CircS was found to be at point 6. Following this, we conducted a threshold effect analysis centered on this inflection point. On the left side of the turning point, the effect size was 0.341 (95% CI: 0.148 -0.653), and on the right side of the turning point, the effect size was 0.684 (95% CI: 0.651- 0.717). Table 5 describes the results of the threshold effect analysis.
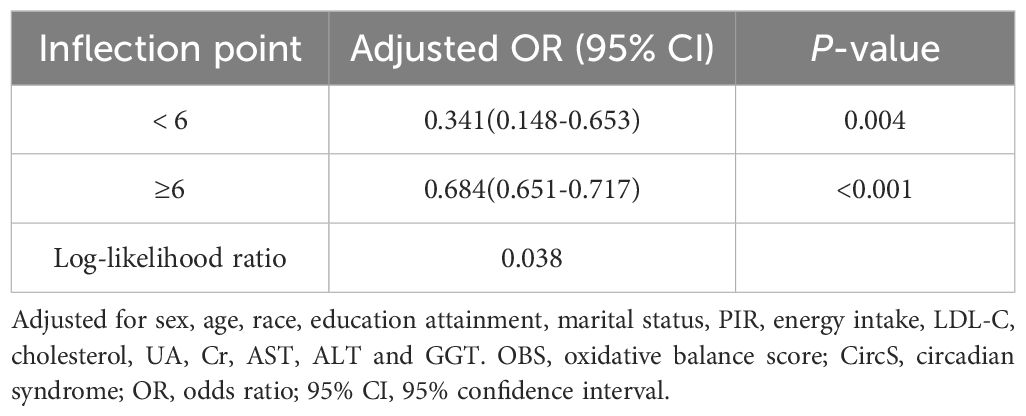
Table 5. Threshold effect analysis of lifestyle OBS on CircS by the two-piecewise linear regression.
Discussion
As circadian rhythm disruption becomes more common with changing lifestyles in our society, early intervention for CircS is necessary (40). This study eventually involved 7202 patients from the NHANES 2005-2018 cohort, with 1433 having CircS. Patients with CircS have lower OBS (total OBS, dietary OBS, and lifestyle OBS) than normal individuals. This negative connection persisted even after controlling for all variables. Although the effect estimates for the connection between OBS and CircS varied by subgroup, their correlation remained negative across all categories. The interaction test results revealed a pronounced connection between lifestyle OBS and CircS in people with education attainment levels above high school. In the RCS, we discovered that total OBS and dietary OBS were linearly and adversely linked to CircS, while lifestyle OBS was nonlinearly and negatively associated. In addition, lifestyle OBS was significantly negatively associated with CircS risk in an overall trend both before and after the inflection point. We identified the inflection point at 6.
To our knowledge, this study is the first to evaluate the correlation between OBS and CircS. CircS is composed of MetS, short sleep duration, and depressive state. Numerous studies have revealed a substantial link between oxidative stress and these factors. A population-based study by Zhixiao Xu et al. exploring the correlation between OBS and MetS among adult Americans showed that higher OBS was related to a lower incidence of MetS and all-cause mortality (38). Another cross-sectional study found a significant correlation between OBS and MetS along with its components (41). In addition to cardiometabolic risk profiles, oxidative stress was similarly associated with sleep quality and depression. Populations with higher OBS have fewer sleep disturbances and have longer sleep duration (42). A randomized controlled design suggests that lowering anti-oxidative stress improves depression scores in patients with major depressive disorder (43). Overall, these epidemiologic studies imply that elevated levels of oxidative stress may increase the likelihood of developing CircS. As expected, we discovered that higher OBS was negatively linked with the development of CircS.
Dietary and lifestyle changes raise the risk of circadian disturbances. Many studies have found significant correlations between the components of OBS and CircS. A cross-sectional study found that consuming vegetables, whole grains, oils, nuts, and seeds helped to reduce the incidence of CircS (44). Keizo Kaneko and his colleagues demonstrated that obesity interferes with the circadian expression of crucial clock genes in the central nervous system (45). Alcohol misuse disrupts circadian rhythms (46). Grigsby, K et al. reported that chronic alcoholism affected circadian gene expression (47). Research has demonstrated that nicotine can induce phase alterations in circadian rhythms in rats (48). Fuentes-Cano, Martin A. et al. discovered that the circadian locomotion and learning efficiency rhythms of juvenile rodents are influenced by perinatal nicotine exposure (49). In addition, Camerbiru et al. found that physical exercise synchronized the circadian system and prevented the development of CircS in indoor fat sand rats (50).
Given that excellent eating habits and lifestyle contribute to regulating the circadian rhythm, our work suggests non-pharmacological prophylactic treatment for the affected population. We can help patients regulate their biological clocks by promoting healthy eating habits and lifestyles, such as increasing physical activity, adhering to regular schedules, limiting high-calorie foods, and reducing sedentary time. Furthermore, our findings alert medical professionals to the lifestyle behaviors of patients with circadian abnormalities.
Interestingly, interaction tests indicated that the negative correlation between lifestyle OBS and CircS was more significant among participants with high levels of schooling. Some studies have revealed that people with less education are more prone to lead unhealthy lifestyles (51, 52). Individuals with higher levels of education have higher levels of self-perceived health and a greater ability to engage in healthier behaviors (53). Additionally, people with higher levels of education engage in physical activity for longer periods and have access to better sports facilities (54). These all contribute to health-promoting behaviors.
The relationship between OBS and CircS is uncertain. Metabolic alterations in CircS are strongly associated with disruption of circadian rhythms, and we thus hypothesize that oxidative stress may have an impact on the regulatory control of circadian rhythms. Oxidative stress is triggered by the hyperproduction of damaging reactive oxygen species (ROS) and reactive nitrogen species (RNS) (55). Oxidative stress arises as the balance of ROS, nitric oxide, and antioxidants is interrupted, causing DNA, lipids, proteins, and cellular structural damage (56). Cellular redox status regulates the biological clock, and rhythmic ROS generates redox oscillations that give feedback to the cellular clock machinery (57). Schmalen, Ira, et al. discovered that maintaining adequate oxidative homeostasis governs the complexes generated by cyclins and cryptochromes, which are key components of circadian regulation (58). Second, melatonin, a neurobiological hormone generated by the pineal gland, regulates circadian rhythms (59). Melatonin deficiency can lead to prolonged wakefulness, brain injury, and metabolic disturbance (60–64). In an animal model, the researchers discovered that persistent stress causes decreased levels of pineal melatonin (65). It was found that myocardial infarction-induced oxidative stress caused an increase in free radicals, which in turn decreased plasma melatonin levels (66). In addition, Koh, Kyunghee, et al. found that increased oxidative stress disrupted the sleep-wake cycle (66). Such research is currently limited, but preliminary findings warrant further investigation.
This study emphasizes the significance of oxidative stress levels for the development of CircS and enriches existing studies. However, there are certain constraints to this study. First, the design of our research was cross-sectional, which may be subject to other confounding factors and the inability to determine causality. Second, our study population was primarily from the United States. Therefore, we were unable to generalize the results to different populations. Finally, it has been demonstrated that shift work, exposure to light, and noise all produce circadian disruptions (67). We cannot rule out all of these confounding factors. Further comprehensive studies are needed to elucidate the causal relationship between OBS and CircS prevalence.
Conclusion
The results showed a substantial negative connection between OBS and CircS. Encouraging foods filled with antioxidants and antioxidant-rich lifestyles may decrease the risk of CircS. Nevertheless, additional studies are required to investigate the potential mechanisms between OBS and CircS.
Data availability statement
The original contributions presented in the study are included in the article/Supplementary Material. Further inquiries can be directed to the corresponding author.
Ethics statement
The studies involving humans were approved by the National Health and Nutrition Examination Survey. The studies were conducted in accordance with the local legislation and institutional requirements. The participants provided their written informed consent to participate in this study.
Author contributions
LX: Writing – original draft, Formal analysis, Methodology. JL: Methodology, Visualization, Writing – original draft. MX: Formal analysis, Visualization, Writing – original draft. YL: Methodology, Visualization, Writing – original draft. XC: Supervision, Visualization, Writing – review & editing. JX: Supervision, Writing – review & editing.
Funding
The author(s) declare financial support was received for the research, authorship, and/or publication of this article. This study was supported by the Sanming Project of Medicine in Shenzhen(No.SZZYSM202206014).
Acknowledgments
The authors would like to thank all the staff and participants of the National Health and Nutrition Examination Survey.
Conflict of interest
The authors declare that the research was conducted in the absence of any commercial or financial relationships that could be construed as a potential conflict of interest.
Publisher’s note
All claims expressed in this article are solely those of the authors and do not necessarily represent those of their affiliated organizations, or those of the publisher, the editors and the reviewers. Any product that may be evaluated in this article, or claim that may be made by its manufacturer, is not guaranteed or endorsed by the publisher.
Supplementary material
The Supplementary Material for this article can be found online at: https://www.frontiersin.org/articles/10.3389/fendo.2024.1431223/full#supplementary-material
Abbreviations
OBS, Oxidative balance score; CircS, circadian syndrome; NHANES, National Health and Nutrition Examination Survey; MetS, Metabolic syndrome; WC, Waist circumference; HDL-C, High-density lipoprotein cholesterol; TG, Triglycerides; BMI, Body mass index; PIR, poverty income ratio; MET, metabolic equivalent; SBP, Systolic blood pressure; DBP, Diastolic blood pressure; FBG, Fasting blood glucose; UA, Uric acid; Cr, Creatinine; AST, Aspartate aminotransferase; ALT, Alanine aminotransferase; GGT, Gamma-glutamyl transferase; RCS, Restricted cubic spline; OR; Odds ratio; CI; Confidence interval; ROS, Reactive oxygen species; RNS, Reactive nitrogen species.
References
1. Rochlani Y, Pothineni NV, Kovelamudi S, Mehta JL. Metabolic syndrome: pathophysiology, management, and modulation by natural compounds. Ther Adv Cardiovasc Dis. (2017) 11:215–25. doi: 10.1177/1753944717711379
2. Wang Y, Yu Q, Chen Y, Cao F. Pathophysiology and therapeutics of cardiovascular disease in metabolic syndrome. Curr Pharm Des. (2013) 19:4799–805. doi: 10.2174/1381612811319270002
3. Grundy SM. Metabolic syndrome pandemic. Arterioscler Thromb Vasc Biol. (2008) 28:629–36. doi: 10.1161/atvbaha.107.151092
4. Cornier MA, Dabelea D, Hernandez TL, Lindstrom RC, Steig AJ, Stob NR, et al. The metabolic syndrome. Endocr Rev. (2008) 29:777–822. doi: 10.1210/er.2008-0024
5. Dominoni DM, Borniger JC, Nelson RJ. Light at night, clocks and health: from humans to wild organisms. Biol Lett. (2016) 12:20160015. doi: 10.1098/rsbl.2016.0015
6. Parsons MJ, Moffitt TE, Gregory AM, Goldman-Mellor S, Nolan PM, Poulton R, et al. Social jetlag, obesity and metabolic disorder: investigation in a cohort study. Int J Obes (Lond). (2015) 39:842–8. doi: 10.1038/ijo.2014.201
7. Boege HL, Bhatti MZ, St-Onge MP. Circadian rhythms and meal timing: impact on energy balance and body weight. Curr Opin Biotechnol. (2021) 70:1–6. doi: 10.1016/j.copbio.2020.08.009
8. Voigt RM, Forsyth CB, Keshavarzian A. Circadian rhythms: a regulator of gastrointestinal health and dysfunction. Expert Rev Gastroenterol Hepatol. (2019) 13:411–24. doi: 10.1080/17474124.2019.1595588
9. Świątkiewicz I, Woźniak A, Taub PR. Time-restricted eating and metabolic syndrome: current status and future perspectives. Nutrients. (2021) 13:221. doi: 10.3390/nu13010221
10. Bilu C, Einat H, Barak O, Zimmet P, Vishnevskia-Dai V, Govrin A, et al. Linking type 2 diabetes mellitus, cardiac hypertrophy and depression in a diurnal animal model. Sci Rep. (2019) 9:11865. doi: 10.1038/s41598-019-48326-7
11. Kumar Jha P, Challet E, Kalsbeek A. Circadian rhythms in glucose and lipid metabolism in nocturnal and diurnal mammals. Mol Cell Endocrinol. (2015) 418 Pt 1:74–88. doi: 10.1016/j.mce.2015.01.024
12. Zimmet P, Alberti K, Stern N, Bilu C, El-Osta A, Einat H, et al. The Circadian Syndrome: is the Metabolic Syndrome and much more! J Intern Med. (2019) 286:181–91. doi: 10.1111/joim.12924
13. Gnocchi D, Pedrelli M, Hurt-Camejo E, Parini P. Lipids around the clock: focus on circadian rhythms and lipid metabolism. Biol (Basel). (2015) 4:104–32. doi: 10.3390/biology4010104
14. Froy O. Metabolism and circadian rhythms–implications for obesity. Endocr Rev. (2010) 31:1–24. doi: 10.1210/er.2009-0014
15. Kalsbeek A, la Fleur S, Fliers E. Circadian control of glucose metabolism. Mol Metab. (2014) 3:372–83. doi: 10.1016/j.molmet.2014.03.002
16. McClung CA. How might circadian rhythms control mood? Let me count the ways. Biol Psychiatry. (2013) 74:242–9. doi: 10.1016/j.biopsych.2013.02.019
17. Buxton OM, Cain SW, O'Connor SP, Porter JH, Duffy JF, Wang W, et al. Adverse metabolic consequences in humans of prolonged sleep restriction combined with circadian disruption. Sci Transl Med. (2012) 4:129ra143. doi: 10.1126/scitranslmed.3003200
18. Shi Z, Tuomilehto J, Kronfeld-Schor N, Alberti G, Stern N, El-Osta A, et al. The Circadian Syndrome Is a Significant and Stronger Predictor for Cardiovascular Disease than the Metabolic Syndrome-The NHANES Survey during 2005-2016. Nutrients. (2022) 14:5317. doi: 10.3390/nu14245317
19. Shi Z, Tuomilehto J, Kronfeld-Schor N, Alberti GK, Stern N, El-Osta A, et al. The circadian syndrome predicts cardiovascular disease better than metabolic syndrome in Chinese adults. J Intern Med. (2021) 289:851–60. doi: 10.1111/joim.13204
20. Wang Y, Yang L, Zhang Y, Liu J. Relationship between circadian syndrome and stroke: A cross-sectional study of the national health and nutrition examination survey. Front Neurol. (2022) 13:946172. doi: 10.3389/fneur.2022.946172
21. Ran J, Tao C, Zhang S, Chen Q, Yang P, Hu Y, et al. Circadian syndrome is associated with the development of chronic kidney disease and rapid decline in kidney function in middle-aged and elder adults: a China nationwide cohort study. J Nutr Health Aging. (2024) 28:100011. doi: 10.1016/j.jnha.2023.100011
22. Xiao Y, Yin S, Bai Y, Yang Z, Wang J, Cui J, et al. Correction: Association between circadian syndrome and the prevalence of kidney stones in overweight adults: a cross-sectional analysis of NHANES 2007-2018. BMC Public Health. (2023) 23:1105. doi: 10.1186/s12889-023-16029-4
23. Xiao Y, Yin S, Wang J, Cui J, Yang Z, Wang J, et al. A positive association between the prevalence of circadian syndrome and overactive bladder in United States adults. Front Public Health. (2023) 11:1137191. doi: 10.3389/fpubh.2023.1137191
24. Forman HJ, Zhang H. Targeting oxidative stress in disease: promise and limitations of antioxidant therapy. Nat Rev Drug Discovery. (2021) 20:689–709. doi: 10.1038/s41573-021-00233-1
25. Bhat AH, Dar KB, Anees S, Zargar MA, Masood A, Sofi MA, et al. Oxidative stress, mitochondrial dysfunction and neurodegenerative diseases; a mechanistic insight. BioMed Pharmacother. (2015) 74:101–10. doi: 10.1016/j.biopha.2015.07.025
26. Valko M, Leibfritz D, Moncol J, Cronin MT, Mazur M, Telser J. Free radicals and antioxidants in normal physiological functions and human disease. Int J Biochem Cell Biol. (2007) 39:44–84. doi: 10.1016/j.biocel.2006.07.001
27. Ma E, Iso H, Yamagishi K, Ando M, Wakai K, Tamakoshi A. Dietary antioxidant micronutrients and all-cause mortality: the Japan collaborative cohort study for evaluation of cancer risk. J Epidemiol. (2018) 28:388–96. doi: 10.2188/jea.JE20170023
28. Chen J, Jiang W, Shao L, Zhong D, Wu Y, Cai J. Association between intake of antioxidants and pancreatic cancer risk: a meta-analysis. Int J Food Sci Nutr. (2016) 67:744–53. doi: 10.1080/09637486.2016.1197892
29. Van Hoydonck PG, Temme EH, Schouten EG. A dietary oxidative balance score of vitamin C, beta-carotene and iron intakes and mortality risk in male smoking Belgians. J Nutr. (2002) 132:756–61. doi: 10.1093/jn/132.4.756
30. Nogales F, Rua RM, Ojeda ML, Murillo ML, Carreras O. Oral or intraperitoneal binge drinking and oxidative balance in adolescent rats. Chem Res Toxicol. (2014) 27:1926–33. doi: 10.1021/tx5002628
31. Fisher-Wellman KH, Neufer PD. Linking mitochondrial bioenergetics to insulin resistance via redox biology. Trends Endocrinol Metab. (2012) 23:142–53. doi: 10.1016/j.tem.2011.12.008
32. Chen K, Yin Q, Guan J, Yang J, Ma Y, Hu Y, et al. Association between the oxidative balance score and low muscle mass in middle-aged US adults. Front Nutr. (2024) 11:1358231. doi: 10.3389/fnut.2024.1358231
33. Lakkur S, Bostick RM, Roblin D, Ndirangu M, Okosun I, Annor F, et al. Oxidative balance score and oxidative stress biomarkers in a study of Whites, African Americans, and African immigrants. Biomarkers. (2014) 19:471–80. doi: 10.3109/1354750x.2014.937361
34. Wu C, Ren C, Song Y, Gao H, Pang X, Zhang L. Gender-specific effects of oxidative balance score on the prevalence of diabetes in the US population from NHANES. Front Endocrinol (Lausanne). (2023) 14:1148417. doi: 10.3389/fendo.2023.1148417
35. Li Y, Liu Y. Adherence to an antioxidant diet and lifestyle is associated with reduced risk of cardiovascular disease and mortality among adults with nonalcoholic fatty liver disease: evidence from NHANES 1999-2018. Front Nutr. (2024) 11:1361567. doi: 10.3389/fnut.2024.1361567
36. Chen J, Liu J, Gu Z, Fan J, Lei S, Zhang Q, et al. Adherence to oxidative balance score is inversely associated with the prevalence of stroke: results from National Health and Nutrition Examination Survey 1999-2018. Front Neurol. (2024) 15:1348011. doi: 10.3389/fneur.2024.1348011
37. Zhang W, Peng SF, Chen L, Chen HM, Cheng XE, Tang YH. Association between the oxidative balance score and telomere length from the national health and nutrition examination survey 1999-2002. Oxid Med Cell Longev. (2022) 2022:1345071. doi: 10.1155/2022/1345071
38. Xu Z, Lei X, Chu W, Weng L, Chen C, Ye R. Oxidative balance score was negatively associated with the risk of metabolic syndrome, metabolic syndrome severity, and all-cause mortality of patients with metabolic syndrome. Front Endocrinol (Lausanne). (2023) 14:1233145. doi: 10.3389/fendo.2023.1233145
39. Asher G, Zhu B. Beyond circadian rhythms: emerging roles of ultradian rhythms in control of liver functions. Hepatology. (2023) 77:1022–35. doi: 10.1002/hep.32580
40. van Kerkhof LW, Van Dycke KC, Jansen EH, Beekhof PK, van Oostrom CT, Ruskovska T, et al. Diurnal variation of hormonal and lipid biomarkers in a molecular epidemiology-like setting. PloS One. (2015) 10:e0135652. doi: 10.1371/journal.pone.0135652
41. Lu Y, Wang M, Bao J, Chen D, Jiang H. Association between oxidative balance score and metabolic syndrome and its components in US adults: a cross-sectional study from NHANES 2011-2018. Front Nutr. (2024) 11:1375060. doi: 10.3389/fnut.2024.1375060
42. Lei X, Xu Z, Chen W. Association of oxidative balance score with sleep quality: NHANES 2007-2014. J Affect Disord. (2023) 339:435–42. doi: 10.1016/j.jad.2023.07.040
43. Khanzode SD, Dakhale GN, Khanzode SS, Saoji A, Palasodkar R. Oxidative damage and major depression: the potential antioxidant action of selective serotonin re-uptake inhibitors. Redox Rep. (2003) 8:365–70. doi: 10.1179/135100003225003393
44. Akbar Z, Shi Z. Dietary patterns and circadian syndrome among adults attending NHANES 2005-2016. Nutrients. (2023) 15:3396. doi: 10.3390/nu15153396
45. Kaneko K, Yamada T, Tsukita S, Takahashi K, Ishigaki Y, Oka Y, et al. Obesity alters circadian expressions of molecular clock genes in the brainstem. Brain Res. (2009) 1263:58–68. doi: 10.1016/j.brainres.2008.12.071
46. Spanagel R, Rosenwasser AM, Schumann G, Sarkar DK. Alcohol consumption and the body's biological clock. Alcohol Clin Exp Res. (2005) 29:1550–7. doi: 10.1097/01.alc.0000175074.70807.fd
47. Grigsby K, Ledford C, Batish T, Kanadibhotla S, Smith D, Firsick E, et al. Targeting the maladaptive effects of binge drinking on circadian gene expression. Int J Mol Sci. (2022) 23:11084. doi: 10.3390/ijms231911084
48. O'Hara BF, Edgar DM, Cao VH, Wiler SW, Heller HC, Kilduff TS, et al. Nicotine and nicotinic receptors in the circadian system. Psychoneuroendocrinology. (1998) 23:161–73. doi: 10.1016/s0306-4530(97)00077-2
49. Fuentes-Cano MA, Bustamante-Valdez DJ, Durán P. Perinatal exposure to nicotine disrupts circadian locomotor and learning efficiency rhythms in juvenile mice. Brain Struct Funct. (2020) 225:2287–97. doi: 10.1007/s00429-020-02126-2
50. Bilu C, Einat H, Zimmet P, Vishnevskia-Dai V, Schwartz WJ, Kronfeld-Schor N. Beneficial effects of voluntary wheel running on activity rhythms, metabolic state, and affect in a diurnal model of circadian disruption. Sci Rep. (2022) 12:2434. doi: 10.1038/s41598-022-06408-z
51. Shaikh R, Khan J. Clustering of lifestyle risk factors among adult population in India: A cross-sectional analysis from 2005 to 2016. PloS One. (2021) 16:e0244559. doi: 10.1371/journal.pone.0244559
52. Gelaw YA, Koye DN, Alene KA, Ahmed KY, Assefa Y, Erku DA, et al. Socio-demographic correlates of unhealthy lifestyle in Ethiopia: a secondary analysis of a national survey. BMC Public Health. (2023) 23:1528. doi: 10.1186/s12889-023-16436-7
53. Sandri E, Pardo J, Cantín Larumbe E, Cerdá Olmedo G, Falcó A. Analysis of the influence of educational level on the nutritional status and lifestyle habits of the young Spanish population. Front Public Health. (2024) 12:1341420. doi: 10.3389/fpubh.2024.1341420
54. Saint Onge JM, Krueger PM. Education and racial-ethnic differences in types of exercise in the United States. J Health Soc Behav. (2011) 52:197–211. doi: 10.1177/0022146510394862
55. Schieber M, Chandel NS. ROS function in redox signaling and oxidative stress. Curr Biol. (2014) 24:R453–462. doi: 10.1016/j.cub.2014.03.034
56. Sies H. Oxidative stress: a concept in redox biology and medicine. Redox Biol. (2015) 4:180–3. doi: 10.1016/j.redox.2015.01.002
57. Putker M, O'Neill JS. Reciprocal control of the circadian clock and cellular redox state - a critical appraisal. Mol Cells. (2016) 39:6–19. doi: 10.14348/molcells.2016.2323
58. Schmalen I, Reischl S, Wallach T, Klemz R, Grudziecki A, Prabu JR, et al. Interaction of circadian clock proteins CRY1 and PER2 is modulated by zinc binding and disulfide bond formation. Cell. (2014) 157:1203–15. doi: 10.1016/j.cell.2014.03.057
59. Ahmad SB, Ali A, Bilal M, Rashid SM, Wani AB, Bhat RR, et al. Melatonin and health: insights of melatonin action, biological functions, and associated disorders. Cell Mol Neurobiol. (2023) 43:2437–58. doi: 10.1007/s10571-023-01324-w
60. Vasey C, McBride J, Penta K. Circadian rhythm dysregulation and restoration: the role of melatonin. Nutrients. (2021) 13:3480. doi: 10.3390/nu13103480
61. Grima NA, Rajaratnam SMW, Mansfield D, Sletten TL, Spitz G, Ponsford JL. Efficacy of melatonin for sleep disturbance following traumatic brain injury: a randomized controlled trial. BMC Med. (2018) 16:8. doi: 10.1186/s12916-017-0995-1
62. Feybesse C, Chokron S, Tordjman S. Melatonin in neurodevelopmental disorders: A critical literature review. Antioxidants (Basel). (2023) 12:2017. doi: 10.3390/antiox12112017
63. Hohor S, Mandanach C, Maftei A, Zugravu CA, Oțelea MR. Impaired melatonin secretion, oxidative stress and metabolic syndrome in night shift work. Antioxidants (Basel). (2023) 12:959. doi: 10.3390/antiox12040959
64. Corbalán-Tutau D, Madrid JA, Nicolás F, Garaulet M. Daily profile in two circadian markers "melatonin and cortisol" and associations with metabolic syndrome components. Physiol Behav. (2014) 123:231–5. doi: 10.1016/j.physbeh.2012.06.005
65. Yocca FD, Friedman E. Effect of immobilization stress on rat pineal beta-adrenergic receptor-mediated function. J Neurochem. (1984) 42:1427–32. doi: 10.1111/j.1471-4159.1984.tb02804.x
66. Domínguez-Rodríguez A, Abreu-González P, García MJ, Sanchez J, Marrero F, de Armas-Trujillo D. Decreased nocturnal melatonin levels during acute myocardial infarction. J Pineal Res. (2002) 33:248–52. doi: 10.1034/j.1600-079x.2002.02938.x
Keywords: oxidative balance score, circadian syndrome, NHANES, oxidative stress, circadian rhythm
Citation: Xie L, Li J, Xu M, Lei Y, Chen X and Xie J (2024) The relationship between oxidative balance score and circadian syndrome: evidence from the NHANES 2005-2018. Front. Endocrinol. 15:1431223. doi: 10.3389/fendo.2024.1431223
Received: 11 May 2024; Accepted: 24 September 2024;
Published: 11 October 2024.
Edited by:
Sardar Sindhu, Dasman Diabetes Institute, KuwaitReviewed by:
Munkhtuya Tumurkhuu, Wake Forest Baptist Medical Center, United StatesYunfei Xiao, Sichuan University, China
Mamunur Rashid, University of Nebraska Medical Center, United States
Copyright © 2024 Xie, Li, Xu, Lei, Chen and Xie. This is an open-access article distributed under the terms of the Creative Commons Attribution License (CC BY). The use, distribution or reproduction in other forums is permitted, provided the original author(s) and the copyright owner(s) are credited and that the original publication in this journal is cited, in accordance with accepted academic practice. No use, distribution or reproduction is permitted which does not comply with these terms.
*Correspondence: Jiajia Xie, eGllamlhamlhYmF6eXlAMTYzLmNvbQ==