- 1Department of Nephrology, First Teaching Hospital of Tianjin University of Traditional Chinese Medicine, Tianjin, China
- 2Department of Nephrology, National Clinical Research Center for Chinese Medicine Acupuncture and Moxibustion, Tianjin, China
- 3Graduate School, Tianjin University of Traditional Chinese Medicine, Tianjin, China
Objectives: To evaluate the efficacy and safety of non-steroid mineralocorticoid receptor antagonists (ns-MRAs) and sodium-glucose cotransporter 2 inhibitors (SGLT2is) in patients with diabetic kidney disease (DKD).
Methods: Systematic literature searches were performed using PubMed, Embase and Web of Science encompassing inception until January 20, 2024. Randomized control trials (RCTs) comparing ns-MRAs and SGLT2is in DKD were selected. The efficacy outcomes of interest included kidney-specific composite outcome, cardiovascular (CV)-specific composite outcome, end-stage kidney disease (ESKD), and overall mortality. We also investigated safety outcomes, including acute kidney injury (AKI) and hyperkalemia.
Results: A total of 10 randomized clinical trials with 35,786 patients applying various treatments were included. SGLT2is (SUCRA 99.84%) have potential superiority in kidney protection. SGLT2is (RR 1.41, 95%CI 1.26 to 1.57) and ns-MRAs (RR 1.17, 95% CI 1.08 to 1.27) were associated with significantly lower kidney-specific composite outcome than the placebo. Regarding the reduction in CV-specific composite outcome and ESKD, SGLT2is (SUCRA 91.61%; 91.38%) have potential superiority in playing cardiorenal protection. Concerning the CV-specific composite outcome (RR 1.27, 95%CI 1.09 to 1.43) and ESKD (RR 1.43, 95%CI 1.20 to 1.72), SGLT2is significantly reduced the risks compared to placebo. Regarding the reduction in overall mortality, SGLT2is (SUCRA 83.03%) have potential superiority in postponing mortality. Concerning the overall mortality, SGLT2is have comparable effects (RR 1.27, 95%CI 1.09 to 1.43) with placebo to reduce the risk of overall mortality compared to placebo. For AKI reduction, ns-MRAs (SUCRA 63.58%) have potential superiority. SGLT2is have comparable effects (RR 1.24, 95%CI 1.05 to 1.46) with placebo to reduce the risk of AKI. For hyperkalemia reduction, SGLT2is (SUCRA 93.12%) have potential superiority. SGLT2is have comparable effects (RR 1.24, 95%CI 1.05 to 1.46) with placebo to reduce the risk of AKI. Concerning hyperkalemia reduction, nsMRAs (RR 1.24 95%CI 0.39 to 3.72) and SGLT2is (RR 1.01 95%CI 0.40 to 3.02) did not show significant benefit compared to placebo.
Conclusion: Concerning the efficacy and safety outcomes, SGLT2is may be recommended as a treatment regimen for maximizing kidney and cardiovascular protection, with a minimal risk of hyperkalemia in DKD.
Systematic review registration: https://www.crd.york.ac.uk/prospero/, identifier CRD42023458613.
1 Introduction
Diabetes mellitus (DM) constitutes a significant threat to public health and presents a major healthcare burden in most countries. Preventive measures and appropriate management are indispensable to preventing long-term complications (1). As reported in the 10th Edition of the Diabetes Atlas by the International Diabetes Federation (IDF), the total number of DM patients (aged 20 to 79) worldwide has reached 537 million as of 2021, with an anticipated increase to 783 million by 2045 (2).
Sustained chronic hyperglycemia leads to vital damage both to macrovascular and microvascular systems, which are the main causes of cardiovascular and cerebrovascular diseases, peripheral artery disease, retinopathy, and nephropathy (3). Approximately 35% of patients with type 2 diabetes (T2D) are projected to develop chronic kidney disease (CKD). Diabetic kidney disease (DKD) is a critical complication of DM, and it is identified as a major cause of end-stage kidney disease (ESKD) (4). The characteristic of DKD is progressive proteinuria. Initially, the glomerular filtration rate (GFR) will gradually decline, resulting in failure eventually. The process is accompanied by capillary injury, mesangial cell proliferation, accumulation of extracellular matrix, thickening of the glomerular basement membrane, loss of podocytes, glomerulosclerosis, and ultimately renal tubulointerstitial fibrosis (5). DKD is a significant cause of ESKD and also serves as a critical risk factor for cardiovascular events and mortality (6). With the rapid rise in the prevalence of T2D worldwide and the significant number of patients developing DKD, there is a considerable economic burden (7). Therefore, it is crucial to slow down the progression through effective medical interventions, considering both a healthcare system and societal perspectives.
At present, the main therapies aimed at slowing down the progression of DKD involve blood pressure lowering, intensive glucose control, and blood lipid regulation, with angiotensin-converting enzyme inhibitors (ACEI) or angiotensin II receptor blockers (ARB) serving as the cornerstone of DKD management (8). The medications assist in mitigating the effects of the renin-angiotensin system, a key role in the onset and progression of DKD. The renoprotective effect of RAS blockade with ACEIs or ARBs is well established (9, 10). DKD patients have predominantly received treatment with RAS inhibitors, despite their limited efficacy, possibly as a result of angiotensin escape and/or aldosterone escape, which leads to an escalation in renin activity subsequent to the prolonged inhibition of renin-angiotensin-aldosterone system (11).
With the emergence of the new class of anti-hyperglycemia medications, ground breaking impulses were given to the treatment of DKD. Increasing evidence supports that employing new classes of medication, such as sodium-glucose cotransporter 2 (SGLT2is) and non-steroid mineralocorticoid receptor antagonist (ns-MRA) could potentially reduce the predefined composite renal outcomes and cardiovascular (CV) outcomes in patients with DKD. SGLT2is act by blocking the carrier protein SGLT2, which leads to glycosuria and subsequently initiates a cascade of positive effects on both the kidneys and cardiovascular system (12). ns-MRAs exhibit a high degree of specificity for mineralocorticoid receptor (MR). Consequently, this selective binding enables the nsMRAs to effectively counteract the effects of aldosterone, a steroid hormone that is believed to play a pivotal role in the progression of DKD (13).
Since there have been few trials directly comparing the efficacy and safety of SGLT2is and ns-MRAs, the debate continues regarding which interventions demonstrate the most significant renal and cardiovascular protective benefits. Network meta-analysis (NMA) serves as an extension of conventional meta-analysis. NMA enables the simultaneous comparison of multiple drug treatments for a disease, allowing for the decision of the most effective treatment option, in the absence of direct head-to-head evidence. Our study aims to identify the optimal treatment regimen, in conjunction with ACEI or ARB for patients with DKD. The study mainly centers around the following questions:
(1) Which medication should be administered to a patient with DKD to effectively prevent the progression of renal and cardiovascular composite outcomes?
(2) Which medication effectively decreases the risk of overall mortality, the development of end-stage kidney disease, acute kidney injury, and hyperkalemia?
To answer the questions, we performed a systemic review and NMA, to evaluate the efficacy and safety of SGLT2is and ns-MRAs for the treatment of DKD, and to provide an evidence-based basis for future clinical practice, medical decision-making, and research direction. Thus, the study provides an overview of the current state of DKD treatment and offers evidence-based guidance.
2 Methods
The study was carried out in line with the Preferred Reporting Items for Systematic Review and Meta-Analysis (PRISMA) 2020 statement (14) and was registered with PROSPERO (CRD 42023458613) https://www.crd.york.ac.uk/prospero/.
2.1 Data sources and search strategies
Database search was conducted using PubMed, Embase and Web of Science without any limitations on language from inception until January 20, 2024. The major search headings included “diabetic kidney disease”, “sodium glucose cotransporter 2 inhibitors” and “nonsteroidal mineralocorticoid receptor antagonists”. Mesh terms and free terms were searched (Supplementary Table S1).
2.2 Study selection and data extraction
Two reviewers (YSQ and WYH) independently screened the article titles, abstracts, and full texts to identify potential trials according to the following inclusion criteria:
(1) randomized controlled trials (RCTs) that compared SGLT2is or ns-MRAs with placebo or conventional treatment.
(2) DKD was defined as the presence of CKD in individuals with T2D. Adults (aged>18 years) with T2D and CKD. The clinical diagnosis of CKD is based on either of the following criteria:
-Persistent high albuminuria defined as UACR≥30 mg/g but <300 mg/g and eGFR≥25 but <60 mL/min/1.73 m2 (Chronic Kidney Disease Epidemiology Collaboration);
-Persistent very high albuminuria defined as UACR≥300 mg/g and eGFR≥25 but <75 mL/min/1.73 m2 (ChronicA Kidney Disease Epidemiology Collaboration).
(3) at least one of these outcomes must be included in the RCT: kidney-specific composite outcome; cardiovascular (CV)-specific composite outcome; ESKD; overall mortality.
Data extraction was conducted by two reviewers (YSQ and WYH), subsequently the members of the study (ZX, ZJ, LH) checked and eventually discussed the extracted data. The extracted information included trial name, registration number, study period, type of study, study drug, mean age, No. of male patients, sample size, outcome data.
2.3 Risk of bias and quality assessment
The Risk-of-Bias 2 tool (The Cochrane Collaboration’s tool for assessing risk of bias) will be used to assess and report the bias associated with the individual studies (15).
2.4 Outcomes
The efficacy outcomes assessed in the NMA include:
(1) kidney-specific composite outcome;
(2) CV-specific composite outcome;
(3) ESKD;
(4) overall mortality.
The kidney-specific composite outcome definitions differed among trials (Supplementary Table S2) but generally involved a combination of worsening eGFR or serum creatinine, ESKD with or without renal replacement therapy or transplant, or death from renal causes. The CV-specific composite outcome definitions varied across trials (Supplementary Table S3) but generally included a combination of CV death, nonfatal myocardial infarction (MI), nonfatal stroke or hospitalization for heart failure (HHF).
The safety outcomes assessed in the NMA include: hyperkalemia and acute kidney injury (AKI).
2.5 Statistical analysis calculated for each study to assess the effect sizes
The BUGSnet package in R (version 4.3.3) was utilized for statistical analysis of Bayesian network meta-analysis. Fixed- or random-effects models were selected for each outcome based on the deviance information criterion (DIC), using the model with the smallest value. Notably, a lower DIC value denotes a superior model fit in relation to the sample size. Risk ratios (RRs) was calculated to assess the effect sizes for dichotomous variables. All results are reported with 95% confidence intervals (CIs). The surface under the cumulative ranking curve (SUCRA) and mean ranks were used to rank the treatments for each outcome. A higher SUCRA value signifies that the treatment is more effective with respect to that outcome. As there were no closed loops, direct estimates could not be obtained in this review. Additionally, a P-value of less than 0.05 is indicative of statistical significance.
3 Results
3.1 Characteristics of included studies
The selection process was illustrated in a flowchart based on the PRISMA guidelines. Figure 1 depicts the selection process. Our literature research initially yielded 1526 publications, out of which 10 RCTs (16–32) were found fully eligible, in total including 35,786 patients. The detailed characteristics of included studies are presented in Table 1. The main baseline information of included studies is summarized in Table 2. All RCTs had placebo as the comparator. The leverage plot was drawn for the option of fixed- or random-effects models (Supplementary Figure S1). The forest plot of NMA was drawn for the comparison for the efficacy and safety outcomes (Supplementary Figure S2).
3.2 Risk of bias in included studies
Six RCTs had an overall low risk of bias. Four RCTs had some concerns. Two RCTs had some concerns for deviations from intended interventions (17, 21), two for missing outcome data (17, 26), one for randomization process (26), one for measurement of the outcome (21), one for selection of the reported result (24). The risk of bias assessments of included studies are shown in Figure 2.
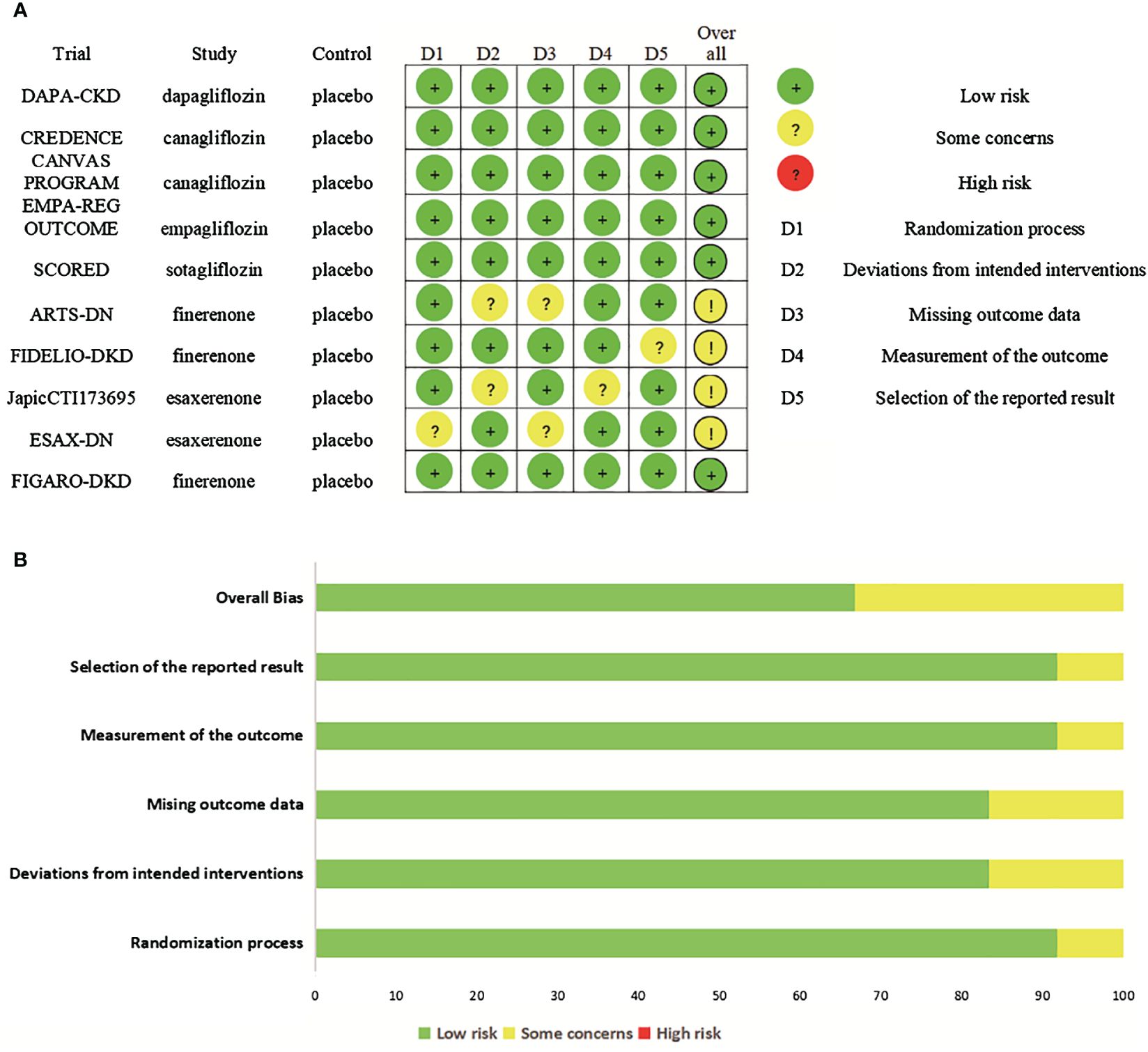
Figure 2 Risk of bias. (A) Risk of bias summary for included studies, showing each risk of bias item for every included study. (B) Risk of bias graph presenting each risk of bias item as percentages across all included studies.
3.3 Efficacy outcomes
Figure 3 shows the network of treatment comparisons in available trials. In this network meta-analysis, none of the six outcomes had a closed loop. This implies that neither outcomes from direct nor indirect comparisons are considered, which renders the need for assessing coherence redundant.
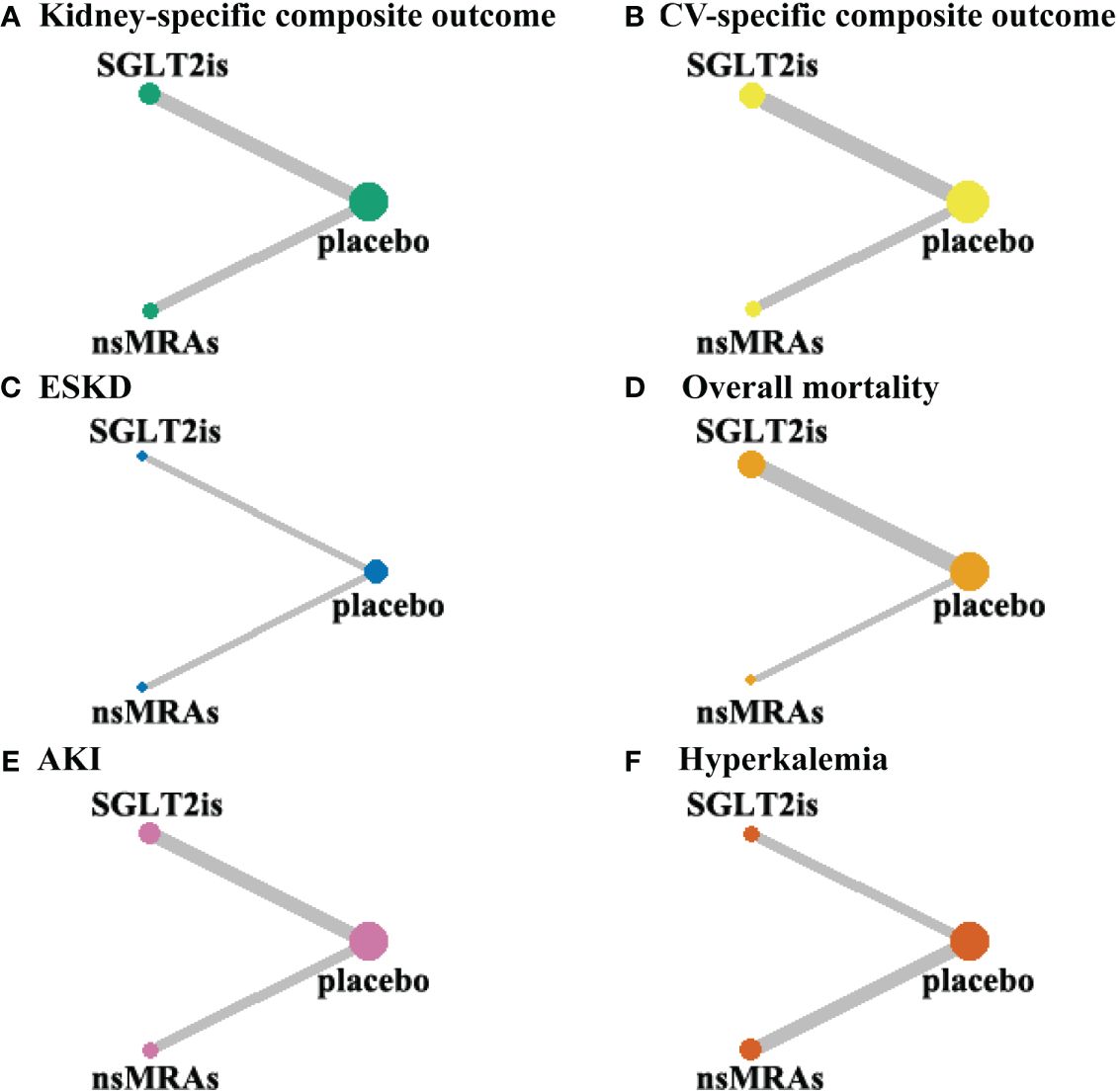
Figure 3 Evidence network maps. (A-F) were drawn evidence network plots of different treatments for DKD with kidney-specific composite outcome, CV-specific composite outcome, ESKD, overall mortality, AKI, and hyperkalemia as outcomes, respectively. The thickness of line segments in the figure represented the number of studies, that could be directly compared between the two connected interventions, and the size of the points represented the total sample size of the intervention included in the study. SGLT2is, sodium glucose cotransporter 2 inhibitors; nsMRAs, non steroid mineralocorticoid receptor antagonists; CV, cardiovascular; ESKD, end-stage kidney disease; AKI, acute kidney disease.
3.3.1 Kidney-specific composite outcome
The analyses of kidney-specific composite outcome included six studies (four with SGLT2is, two with ns-MRAs), that had enrolled a total of 33,183 participants.
The rankogram (Figure 4A) showed that SGLT2is had the superior probability of reducing the risk of kidney-specific composite outcome (99.84%), followed by ns-MRAs (50.15%). For kidney-specific composite outcome reduction, SGLT2is were most likely to rank best. When compared with placebo, both SGLT2is (RR 1.41, 95% CI 1.26 to 1.57) and ns-MRAs (RR 1.17, 95% CI 1.08 to 1.27) were associated with reductions in kidney-specific composite outcome (Figure 5A). SGLT2is were associated with a lower risk of kidney-specific composite outcome compared with ns-MRAs (RR 1.20, 95% CI 1.05 to 1.38).
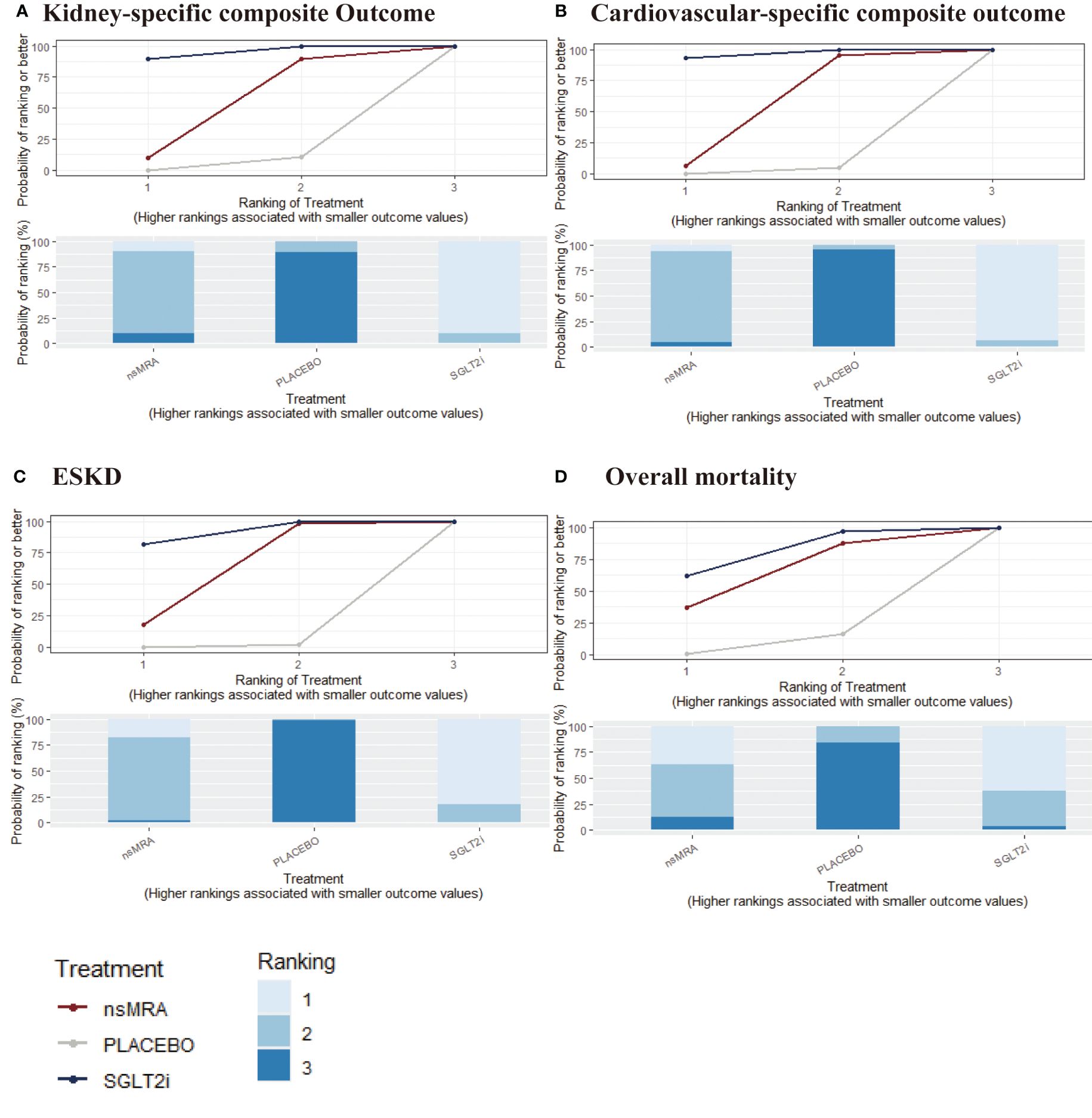
Figure 4 SUCRA probabilities for the effectiveness outcomes of interventions. (A-D) were drawn SUCRA probabilities plots for the effectiveness outcomes of interventions. (A) kidney-specific composite outcome; (B) CV-specific composite outcome; (C) ESKD; (D) overall mortality. A higher SUCRA indicates a lower probability that the drug can reach the endpoint. SGLT2i, sodium glucose cotransporter 2 inhibitor; nsMRA, non steroid mineralocorticoid receptor antagonist; ESKD, end-stage kidney disease.
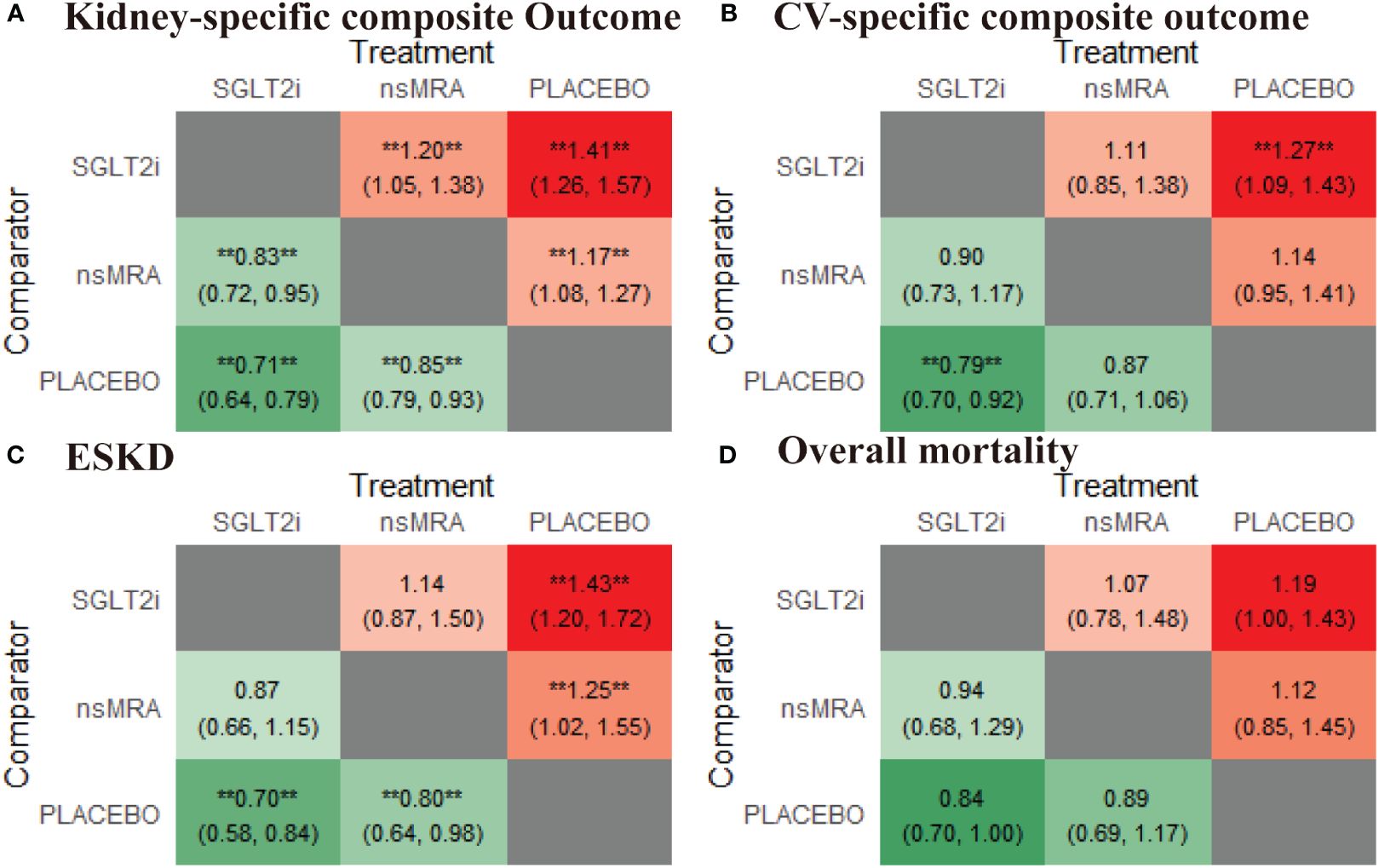
Figure 5 Comparative heatmap for effectiveness outcomes of interventions. (A-D) drawn comparative heat map of interventions with the kidney-specific composite outcome, CV-specific composite outcome, ESKD, overall mortality. Comparisons between treatments should be read from left to right. The effect size (RR) and 95% confidence interval are in the cell in common between the column-defining treatment and the row-defining treatment. RR>1 favors the row-defining treatment. SGLT2i, sodium glucose cotransporter 2 inhibitor; nsMRA, non steroid mineralocorticoid receptor antagonist; CV, Cardiovascular; ESKD, end-stage kidney disease; RR, Risk ratio. **represents statistical significance.
3.3.2 CV-specific composite outcome
The analyses of CV-specific composite outcome included eight studies (five with SGLT2is, three with ns-MRAs), that had enrolled a total of 35,194 participants.
The rankogram (Figure 4B) showed that SGLT2is had the superior probability of reducing the risk of CV-specific composite outcome (91.61%), followed by ns-MRAs (55.17%). For CV-specific composite outcome reduction, SGLT2is were most likely to rank best. Compared with the placebo, SGLT2is (RR 1.27, 95% CI 1.09 to 1.43) were associated with reductions in CV-specific composite outcome significantly (Figure 5B). And ns-MRAs (RR 1.14, 95% CI 0.95 to 1.41) were similar in CV-specific composite outcome reduction compared with placebo.
3.3.3 ESKD
The analyses of ESKD included four studies (two with SGLT2is, two with ns-MRAs), that had enrolled a total of 20,333 participants.
The rankogram (Figure 4C) showed that SGLT2is had the superior probability of reducing the risk of ESKD (91.38%), followed by ns-MRAs (57.81%). For ESKD, SGLT2is were most likely to rank best. When compared with placebo, both SGLT2is (RR 1.43, 95% CI 1.20 to 1.72) and ns-MRAs (RR 1.25, 95% CI 1.02 to 1.55) were associated with reductions in ESKD (Figure 5C).
3.3.4 Overall mortality
The analyses of overall mortality included nine studies (five with SGLT2is, four with ns-MRAs), that had enrolled a total of 35,594 participants.
The rankogram (Figure 4D) showed that SGLT2is had the superior probability of reducing the risk of overall mortality (83.03%), followed by ns-MRAs (57.5%). For overall mortality, SGLT2is were most likely to rank best. We found that treatments were similar in overall mortality reduction since compared with placebo, neither of the drugs showed significant changes [SGLT2is (RR 1.19, 95% CI 1.00 to 1.43), ns-MRAs (RR 1.12, 95% CI 0.85 to 1.45)] (Figure 5D).
3.4 Safety outcomes
3.4.1 AKI
The analyses of hyperkalemia included seven studies (four with SGLT2is, three with ns-MRAs), that had enrolled a total of 24,610 participants.
The rankogram (Figure 6A) showed that ns-MRAs had the superior probability of reducing the risk of AKI (63.58%), followed by SGLT2is (44.76%). For AKI reduction, ns-MRAs were most likely to rank best. Compared with the placebo, SGLT2is (RR 1.24, 95% CI 1.05 to 1.46) were associated with reductions in AKI significantly (Figure 7A). And ns-MRAs (RR 1.08, 95% CI 0.90 to 1.28) were similar in AKI reduction compared with placebo.
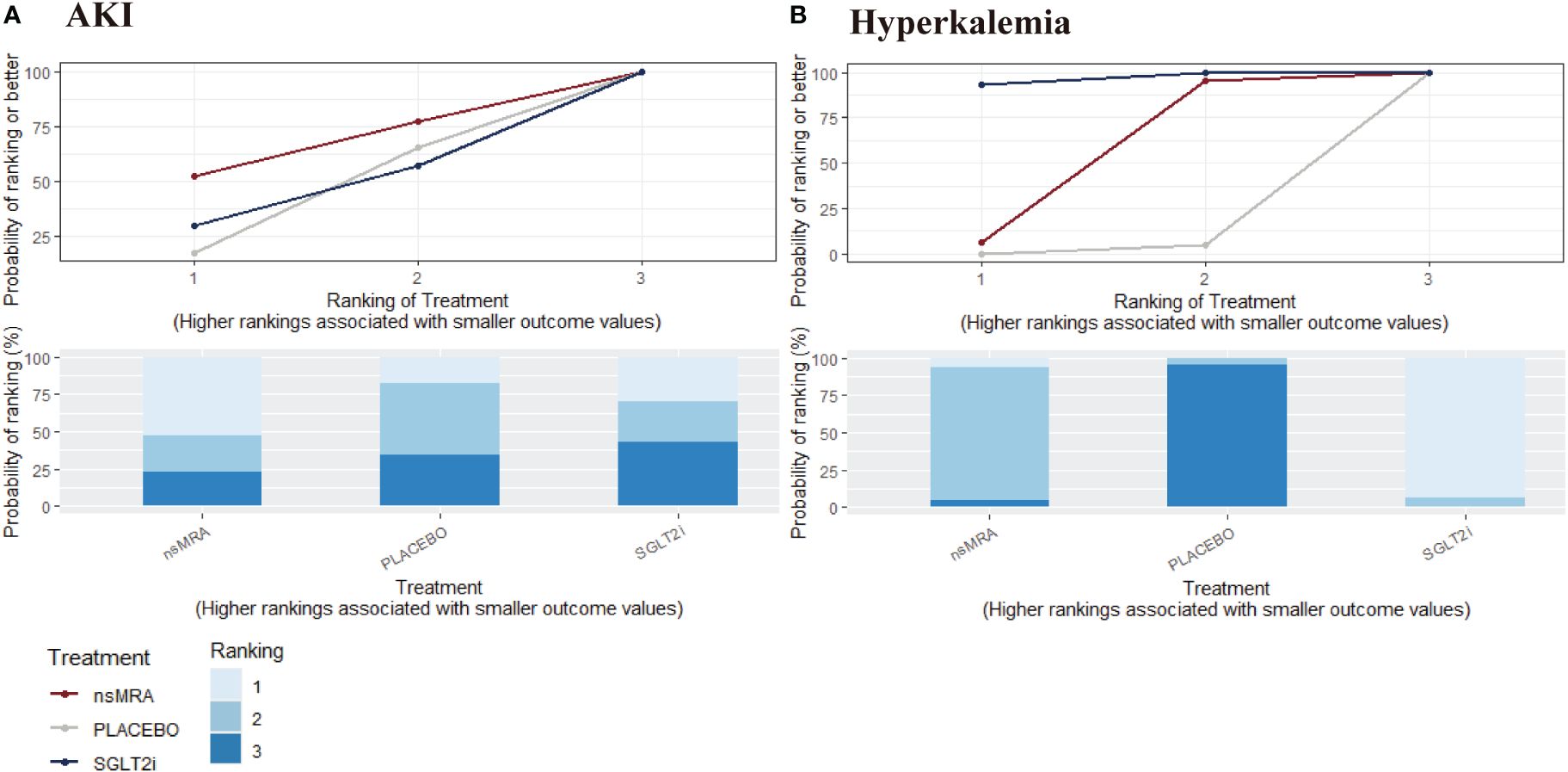
Figure 6 SUCRA probabilities for safety outcomes of interventions. (A, B) were drawn SUCRA probabilities plots for the safety outcomes of interventions. (A) AKI; (B) Hyperkalemia. A higher SUCRA indicates a lower probability that the drug can reach the endpoint. SGLT2i, sodium glucose cotransporter 2 inhibitor; nsMRA, non steroid mineralocorticoid receptor antagonist; AKI, acute kidney disease.
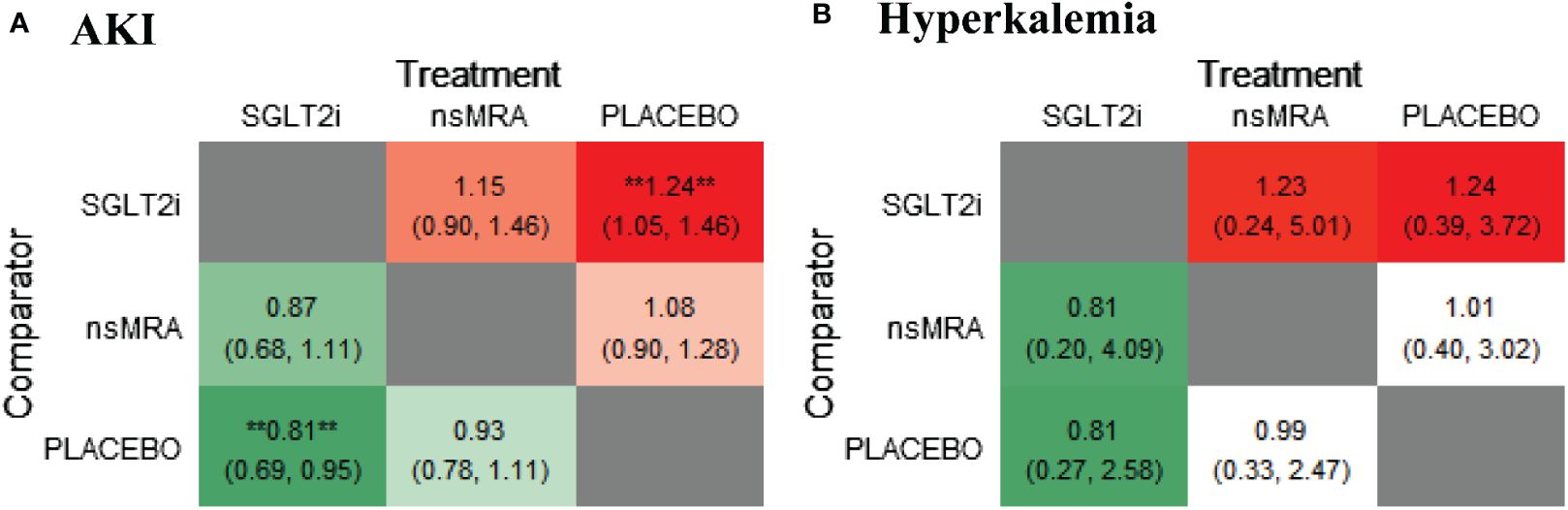
Figure 7 Comparative heatmap for safety outcomes of interventions. (A, B) drawn comparative heat map of interventions with AKI and hyperkalemia. Comparisons between treatments should be read from left to right. The effect size (RR) and 95% confidence interval are in the cell in common between the column-defining treatment and the row-defining treatment. RR>1 favors the row-defining treatment. SGLT2i, sodium glucose cotransporter 2 inhibitor; nsMRA, non steroid mineralocorticoid receptor antagonist; AKI, Acute kidney injury; RR, Risk ratio. **represents statistical significance.
3.4.2 Hyperkalemia
The analyses of hyperkalemia included nine studies (four with SGLT2is, five with ns-MRAs), that had enrolled a total of 32, 880 participants.
The rankogram (Figure 6B) showed that SGLT2is had the superior probability of reducing the risk of hyperkalemia (93.12%), followed by ns-MRAs (46.18%). For hyperkalemia reduction, SGLT2is were most likely to rank best. We found that treatments were similar in hyperkalemia reduction since compared with placebo, neither of the drugs showed significant changes [SGLT2is (RR 1.24, 95% CI 0.39 to 3.72), ns-MRAs (RR 1.01, 95% CI 0.40 to 3.02)] (Figure 7B).
4 Discussion
This Bayesian NMA included 10 RCTs, encompassing 35,786 participants. The efficacy and safety outcomes in the study were mainly derived from the DAPA-CKD, CREDENCE, CANVAS Program, EMPA-REG OUTCOME, SCORED, ARTS-DN, FIDELIO-DKD, JapicCTI173695, ESAX-DN, and FIGARO-DKD trials. The main comparison subjects were ns-MRAs (finerenone or esaxerenone) and SGLT2is (canagliflozin, dapagliflozin, empagliflozin or sotagliflozin.
The study compared SGLT2is and ns-MRAs to provide comprehensive evidence of various drug regimens in the DKD population for improving kidney and cardiovascular prognosis. The primary strength of the NMA lies in its targeted focus on DKD patients. This specialized emphasis has facilitated, for the first time, a comparison of the efficacy and safety of innovative antidiabetic drug classes in relation to their impacts on cardiorenal outcomes, specifically within DKD patients.
The findings of the study revealed that, when compared to ns-MRAs, the utilization of SGLT2is resulted in the superior favorable outcomes. SGLT2is have been demonstrated a significant reduction in the risks associated with kidney-specific composite outcome, CV-specific composite outcome, ESKD, overall mortality by 41%, 27%, 43% and 19%, respectively, compared with placebo. While ns-MRAs lowered the risks of kidney-specific composite outcome and ESKD by 17% and 25%, respectively, there was no benefit with regard to the risk of CV-specific composite outcome and overall mortality compared with placebo. As for safety outcomes, the NMA findings suggest that the use of SGLT2is was linked to reduced hyperkalemia; rather, the use of ns-MRAs was associated with reduced AKI. Overall, SGLT2is may be favored over ns-MRAs based on their significant correlation with lower mortality and their additional cardiorenal benefits. SGLT2is ought to be considered an essential addition to the treatment regimen for each patient diagnosed with DKD.
4.1 The potential organ-protective effects of SGLT2is and MRAs
Clinical studies have demonstrated that SGLT2is not only lower blood glucose levels but also have the ability to decrease blood pressure and postpone the onset and/or progression of kidney dysfunction (23, 25, 33). The Kidney Disease: Improving Global Outcomes (KDIGO) diabetes workgroup recommends SGLT2is as a first-line treatment for patients with T2DM who also have CKD (34). The SGLT2is exerts its therapeutic effects by blocking the reabsorption of filtered sodium ions and glucose within the proximal tubule (35). This inhibition increases sodium ion transportation, and the subsequent rise in sodium concentration prompts activation of the tubuloglomerular feedback at the macula densa, which triggers constriction of the afferent arteriole (36). This constriction induces a decline in plasma flow and intra-glomerular capillary pressure, thereby culminating in the reduction of glomerular filtration rate (GFR) observed following the intake of SGLT2is (37).
Patients with T2D are exposed to substantial risks of mortality and the probability of developing complications due to unresolved metabolic, cellular, and inflammatory events (38). Upregulation of the MR has been detected in an assortment of clinical conditions, including hyperglycemia, obesity, insulin resistance, high salt intake, and CKD (39–43). Under pathological conditions, the overactivation of the MR prompts the increase of diverse proinflammatory and profibrotic cytokines (e.g., tumor necrosis factor receptor-α, interleukin-1, fibronectin, and transforming growth factor-α) within the cardiovascular and renal system, ultimately resulting in chronic inflammation and fibrosis (6, 44). Accordingly, MR antagonists have certain benefits in lowering urinary albumin, inhibiting tissue fibrosis, and offering cardiorenal protective effect (44, 45). Traditional MR antagonists, including spironolactone and eplerenone, are not widely used clinically owing to the high incidence of hyperkalemia and gynecomastia (46). Traditional MR antagonists are generally used as diuretics in the management of hypertension, primary aldosteronism, and heart failure (47). Over the past decade, novel and first-in-class ns-MRAs (finerenone, esaxerenone) have been developed and differ significantly with traditional MR antagonists due to their higher selective MRA activity, devoid of androgen antagonism and progesterone agonism (48). The U.S. Food and Drug Administration has granted approval for finerenone to reduce the risks of decline in kidney function, kidney failure, cardiovascular death, nonfatal myocardial infarctions, and hospitalization for heart failure in adults with CKD associated with T2D (49).
4.2 Advantage of the use of SGLT2is in DKD patients
Based on the available evidence of our study, SGLT2is had the highest SUCRA rank for all efficacy outcomes, indicating that they possess a greater probability than ns-MRAs of reducing risks of both CV and renal outcomes. Obesity is considered to be closely related to insulin resistance. The weight-reducing effect of SGLT2is could improve insulin resistance, regulate energy homeostasis, and thereby exert renal protective effects that go beyond glucose-lowering (50). It has been proposed that SGLT2is maintain the balance of sodium-potassium ion exchange (natriuretic and diuretic effects) and energy metabolism, as well as reduce oxidative stress in cells to provide favorable effects on the risk of renal protection (51, 52). A study by Ravindran et al. (53) suggested that SGLT2is are capable of inhibiting the RAAS activation, playing a role in lowering blood pressure and improving renal hyperfiltration.
4.3 Risks associated with the use of ns-MRAs
Adverse events associated with ns-MRAs are a common concern in clinical practice. For ns-MRAs, some common issues include hyperkalemia, upper respiratory tract infections, pharyngitis, abdominal discomfort, constipation, dizziness, and renal impairment. Among various adverse events, hyperkalemia has always been the major concerning, as the use of ns-MRAs could lead to decreased potassium excretion due to the blockade of aldosterone receptors, resulting in an elevated level of serum potassium (54). Although the incidence of hyperkalemia has increased compared to the placebo, there is study showing that the risk of ns-MRAs is lower than that of traditional steroidal MRA (55). In addition, as some trials included a combination of RAS inhibitors, there is a possibility of increased blood potassium changes. Studies have found that serum potassium elevation most commonly occurs in subsets with low baseline eGFR or high baseline potassium levels. It has been observed that there tends to be a generally stable return to baseline blood potassium levels by the final stages of treatment, or upon treatment completion (56, 57).
4.4 The related meta-analysis
In a comprehensive meta-analysis (58), it was demonstrated that the utilization of SGLT2is can lead to a significant reduction in glycated hemoglobin, blood pressure, body weight, and proteinuria levels. Furthermore, these outcomes have been shown to postpone the decline in the eGFR (with a significant difference when compared to placebo: 1.35 95% CI: 0.78-1.93). Additionally, the study revealed that the risks of renal composite outcome, encompassing the doubling of serum creatinine levels, ESKD, or renal death, were considerably reduced in patients treated with SGLT2is (RR 0.71, 95% CI: 0.53-0.95). Compared to patients who did not use SGLT2is, those who did see a delayed decline in GFR slope. This conclusion also effectively explains the renal protection of SGLT2is.
A NMA (59) based on 18 large trials comparing SGLT2i, finerenone, and glucagonlike peptide-1 receptor agonists (GLP-1 RA) found finerenone can decrease the risk of major adverse cardiovascular events, renal outcome, and HHF, along with the tendency to reduce all-cause death in patients with T2DM and CKD. GLP-1 RA showed a marked impact on reducing cardiovascular events when juxtaposed with exendin-4 analogues. The study further elucidated that finerenone boasts the same potency as SGLT2is in lowering MACE risk. The inconsistency observed may be credited to the different definition of CV- specific composite outcome, specifically the inclusion of unstable angina, or without trials of ESAX-DN.
A comprehensive NMA (60) comprising data from 42346 patients with DKD, had compared all treatments for DKD that have been used by nephrologists over the last two decades. The study found SGLT2is should be a crucial part of the treatment of DKD patients alongside either ACEI or ARB, concerning mortality and ESKD. The finding aligns with the results observed in our study.
4.5 Limitations
Our study has certain limitations that warrant further discussion. First, our search was confined to placebo-controlled trials only. To date, there have been no RCTs that focus on kidney or cardiovascular outcomes to directly compare the efficacy of SGLT2is and ns-MRAs in patients diagnosed with DKD. Additionally, the absence of closed-loop meta-analyses resulted in findings from our network meta-analysis producing limited insights.
Second, it is of note that FIDELIO-DKD and FIGARO-DKD trials permitted the concurrent administration of finerenone in conjunction with SGLT2is (with 6.4% of patients receiving SGLT2is at baseline), whereas combination therapy with SGLT2is and ns-MRA was limited in CREDENCE and DAPA-CKD trials (as baseline ns-MRA use was prohibited). This could potentially influence the difference in renal and cardiac outcomes between the two agents.
Third, the follow-up period for the trials included varied, ranging from 12 to 104 weeks. Therefore, there may be some differences in kidney-specific composite outcome in patients with DKD in this study.
Finally, there were notable differences in baseline levels of HbA1c and eGFR between patients receiving SGLT2is and ns-MRA treatments. The absence of pooled individual patient data and the small number of eligible trials restricted our capacity to conduct substantial subgroup analyses based on factors like the stage of DKD, the presence of albuminuria. Additionally, since most trials included in this meta-analysis excluded participants with eGFR<25 ml/min/1.73 m2, our findings may not be applicable to patients with severe renal insufficiency. Future studies are required to clarify the effectiveness of ns-MRAs and SGLT2is in individuals with diverse clinical profiles.
5 Conclusion
The latest Standards of Medical Care in Diabetes guidelines from the American Diabetes Association (ADA) recommend the use of SGLT2is in patients with stage 3 CKD or higher and T2DM regardless of glycemic control, to slow the progression of CKD and reduce CV and renal risks. Our data lend further support to ADA and KDIGO guidelines, which suggest that SGLT2is are needed in DKD.
Data availability statement
The original contributions presented in the study are included in the article/Supplementary Material. Further inquiries can be directed to the corresponding authors.
Author contributions
S-QY: Data curation, Methodology, Visualization, Writing – original draft. XZ: Conceptualization, Funding acquisition, Supervision, Writing – review & editing. JZ: Data curation, Writing – review & editing. HL: Data curation, Writing – review & editing. Y-HW: Data curation, Methodology, Writing – review & editing. Y-GW: Funding acquisition, Writing – review & editing.
Funding
The author(s) declare financial support was received for the research, authorship, and/or publication of this article. This work was supported by National Natural Science Foundation of China (grant numbers 81603544, 81573888), National Famous Traditional Chinese Medicine Expert Inheritance Studio (grant numbers 978022) and Inheritance and Development of Traditional Chinese Medicine: Famous Traditional Chinese Medicine Expert Inheritance Studio of Tianjin (grant numbers 883022).
Acknowledgments
We would like to sincerely express our gratitude for the support of all participants in this study.
Conflict of interest
The authors declare that the research was conducted in the absence of any commercial or financial relationships that could be construed as a potential conflict of interest.
Publisher’s note
All claims expressed in this article are solely those of the authors and do not necessarily represent those of their affiliated organizations, or those of the publisher, the editors and the reviewers. Any product that may be evaluated in this article, or claim that may be made by its manufacturer, is not guaranteed or endorsed by the publisher.
Supplementary material
The Supplementary Material for this article can be found online at: https://www.frontiersin.org/articles/10.3389/fendo.2024.1429261/full#supplementary-material
Abbreviations
ns-MRAs, Non-steroid mineralocorticoid receptor antagonists; SGLT2is, Sodium-glucose cotransporter 2 inhibitors; DKD, Diabetic kidney disease; RCTs, Randomized control trials; CV, Cardiovascular; ESKD, End-stage kidney disease; AKI, Acute kidney injury; DM, Diabetes mellitus; IDF, International Diabetes Federation; T2D, Type 2 diabetes; CKD, Chronic kidney disease; GFR, Glomerular filtration rate; ACEI, Angiotensin-converting enzyme inhibitors; ARB, Angiotensin receptor blockers; MR, Mineralocorticoid receptor; NMA, Network meta-analysis; MI, Myocardial infarction; HHF, Hospitalization for heart failure; DIC, Deviance information criterion; SUCRA, Surface under the cumulative ranking curve; RR, Risk ratio; CI, Confidence interval; KDIGO, Kidney Disease: Improving Global Outcomes; GLP-1 RA, Glucagonlike peptide-1 receptor agonists; ADA, American Diabetes Association.
References
1. ElSayed NA, Aleppo G, Aroda VR, Bannuru RR, Brown FM, Bruemmer D, et al. Summary of revisions: standards of care in diabetes—2023. Diabetes Care. (2022) 46:S5–9. doi: 10.2337/dc23-Srev
2. Ogurtsova K, Guariguata L, Barengo NC, Ruiz PL-D, Sacre JW, Karuranga S, et al. IDF diabetes Atlas: Global estimates of undiagnosed diabetes in adults for 2021. Diabetes Res Clin Pract. (2022) 183:109118. doi: 10.1016/j.diabres.2021.109118
3. Elbatreek MH, PaChado MP, Cuadrado A, Jandeleit-Dahm K, Schmidt HHHW. Reactive oxygen comes of age: mechanism-based therapy of diabetic end-organ damage. Trends Endocrinol Metab TEM. (2019) 30:312–27. doi: 10.1016/j.tem.2019.02.006
4. Lim AK. Diabetic nephropathy - complications and treatment. Int J Nephrol. Renov. Dis. (2014) 7:361–81. doi: 10.2147/IJNRD.S40172
5. Barrera-Chimal J, Jaisser F. Pathophysiologic mechanisms in diabetic kidney disease: A focus on current and future therapeutic targets. Diabetes Obes Metab. (2020) 22 Suppl 1:16–31. doi: 10.1111/dom.13969
6. Alicic RZ, Rooney MT, Tuttle KR. Diabetic kidney disease: challenges, progress, and possibilities. Clin J Am Soc Nephrol. CJASN. (2017) 12:2032–45. doi: 10.2215/CJN.11491116
7. Vupputuri S, Kimes TM, Calloway MO, Christian JB, Bruhn D, Martin AA, et al. The economic burden of progressive chronic kidney disease among patients with type 2 diabetes. J Diabetes Complications. (2014) 28:10–6. doi: 10.1016/j.jdiacomp.2013.09.014
8. Barrera-Chimal J, Prince S, Fadel F, El Moghrabi S, Warnock DG, Kolkhof P, et al. Sulfenic acid modification of endothelin B receptor is responsible for the benefit of a nonsteroidal mineralocorticoid receptor antagonist in renal ischemia. J Am Soc Nephrol. JASN. (2016) 27:398–404. doi: 10.1681/ASN.2014121216
9. Ruggenenti P, Cravedi P, Remuzzi G. The RAAS in the pathogenesis and treatment of diabetic nephropathy. Nat Rev Nephrol. (2010) 6:319–30. doi: 10.1038/nrneph.2010.58
10. Warren AM, Knudsen ST, Cooper ME. Diabetic nephropathy: an insight into molecular mechanisms and emerging therapies. Expert Opin Ther Targets. (2019) 23:579–91. doi: 10.1080/14728222.2019.1624721
11. Bell DSH, Jerkins T. The potential for improved outcomes in the prevention and therapy of diabetic kidney disease through ‘stacking’ of drugs from different classes. Diabetes Obes Metab. (2024) 26:2046–53. doi: 10.1111/dom.15559
12. Maltese G, Koufakis T, Kotsa K, Karalliedde J. Can sodium-glucose cotransporter 2 inhibitors “spin the thread of life”? Trends Endocrinol Metab TEM. (2023) 34:1–4. doi: 10.1016/j.tem.2022.10.002
13. Grune J, Beyhoff N, Smeir E, Chudek R, Blumrich A, Ban Z, et al. Selective mineralocorticoid receptor cofactor modulation as molecular basis for Finerenone’s antifibrotic activity. Hypertens Dallas Tex 1979. (2018) 71:599–608. doi: 10.1161/HYPERTENSIONAHA.117.10360
14. Page MJ, McKenzie JE, Bossuyt PM, Boutron I, Hoffmann TC, Mulrow CD, et al. The PRISMA 2020 statement: an updated guideline for reporting systematic reviews. BMJ. (2021) 372:n71. doi: 10.1136/bmj.n71
15. Higgins JPT, TThomas J, Chandler J, Cumpston M, Li T, Page MJ, et al. Cochrane Handbook for Systematic Reviews of Interventions Version 6.2 (2021). Available online at: www.training.cochrane.org/handbook (Accessed February, 2021).
16. Pitt B, Kober L, Ponikowski P, Gheorghiade M, Filippatos G, Krum H, et al. Safety and tolerability of the novel non-steroidal mineralocorticoid receptor antagonist BAY 94-8862 in patients with chronic heart failure and mild or moderate chronic kidney disease: a randomized, double-blind trial. Eur Heart J. (2013) 34:2453–63. doi: 10.1093/eurheartj/eht187
17. Bakris GL, Agarwal R, Chan JC, Cooper ME, Gansevoort RT, Haller H, et al. Effect of finerenone on albuminuria in patients with diabetic nephropathy: A randomized clinical trial. JAMA. (2015) 314:884–94. doi: 10.1001/jama.2015.10081
18. Katayama S, Yamada D, Nakayama M, Yamada T, Myoishi M, Kato M, et al. A randomized controlled study of finerenone versus placebo in Japanese patients with type 2 diabetes mellitus and diabetic nephropathy. J Diabetes Complications. (2017) 31:758–65. doi: 10.1016/j.jdiacomp.2016.11.021
19. Neuen BL, Ohkuma T, Neal B, Matthews DR, de Zeeuw D, Mahaffey KW, et al. Cardiovascular and renal outcomes with Canagliflozin according to baseline kidney function. Circulation. (2018) 138:1537–50. doi: 10.1161/CIRCULATIONAHA.118.035901
20. Bakris GL, Agarwal R, Anker SD, Pitt B, Ruilope LM, Nowack C, et al. Design and baseline characteristics of the finerenone in reducing kidney failure and disease progression in diabetic kidney disease trial. Am J Nephrol. (2019) 50:333–44. doi: 10.1159/000503713
21. Ito S, Shikata K, Nangaku M, Okuda Y, Sawanobori T. Efficacy and safety of esaxerenone (CS-3150) for the treatment of type 2 diabetes with microalbuminuria: A randomized, double-blind, placebo-controlled, phase II trial. Clin J Am Soc Nephrol. CJASN. (2019) 14:1161–72. doi: 10.2215/CJN.14751218
22. Neuen BL, Ohkuma T, Neal B, Matthews DR, de Zeeuw D, Mahaffey KW, et al. Effect of canagliflozin on renal and cardiovascular outcomes across different levels of albuminuria: data from the CANVAS program. J Am Soc Nephrol. JASN. (2019) 30:2229–42. doi: 10.1681/ASN.2019010064
23. Perkovic V, Jardine MJ, Neal B, Bompoint S, Heerspink HJL, Charytan DM, et al. Canagliflozin and renal outcomes in type 2 diabetes and nephropathy. N Engl J Med. (2019) 380:2295–306. doi: 10.1056/NEJMoa1811744
24. Bakris GL, Agarwal R, Anker SD, Pitt B, Ruilope LM, Rossing P, et al. Effect of finerenone on chronic kidney disease outcomes in type 2 diabetes. N Engl J Med. (2020) 383:2219–29. doi: 10.1056/NEJMoa2025845
25. Heerspink HJL, Stefánsson BV, Correa-Rotter R, Chertow GM, Greene T, Hou F-F, et al. Dapagliflozin in patients with chronic kidney disease. N Engl J Med. (2020) 383:1436–46. doi: 10.1056/NEJMoa2024816
26. Ito S, Kashihara N, Shikata K, Nangaku M, Wada T, Okuda Y, et al. Esaxerenone (CS-3150) in patients with type 2 diabetes and microalbuminuria (ESAX-DN). Clin J Am Soc Nephrol. CJASN. (2020) 15:1715–27. doi: 10.2215/CJN.06870520
27. Jardine MJ, Zhou Z, Mahaffey KW, Oshima M, Agarwal R, Bakris G, et al. Renal, cardiovascular, and safety outcomes of canagliflozin by baseline kidney function: A secondary analysis of the CREDENCE randomized trial. J Am Soc Nephrol. JASN. (2020) 31:1128–39. doi: 10.1681/ASN.2019111168
28. Bhatt DL, Szarek M, Pitt B, Cannon CP, Leiter LA, McGuire DK, et al. Sotagliflozin in patients with diabetes and chronic kidney disease. N Engl J Med. (2021) 384:129–39. doi: 10.1056/NEJMoa2030186
29. Pitt B, Filippatos G, Agarwal R, Anker SD, Bakris GL, Rossing P, et al. Cardiovascular events with finerenone in kidney disease and type 2 diabetes. N Engl J Med. (2021) 385:2252–63. doi: 10.1056/NEJMoa2110956
30. Wanner C, Inzucchi SE, Lachin JM, Fitchett D, von Eynatten M, Mattheus M, et al. Empagliflozin and progression of kidney disease in type 2 diabetes. N Engl J Med. (2016) 375:323–34. doi: 10.1056/NEJMoa1515920
31. Wanner C, Inzucchi SE, Zinman B, Koitka-Weber A, Mattheus M, George JT, et al. Consistent effects of empagliflozin on cardiovascular and kidney outcomes irrespective of diabetic kidney disease categories: Insights from the EMPA-REG OUTCOME trial. Diabetes Obes Metab. (2020) 22:2335–47. doi: 10.1111/dom.14158
32. Wheeler DC, Stefánsson BV, Jongs N, Chertow GM, Greene T, Hou FF, et al. Effects of dapagliflozin on major adverse kidney and cardiovascular events in patients with diabetic and non-diabetic chronic kidney disease: a prespecified analysis from the DAPA-CKD trial. Lancet Diabetes Endocrinol. (2021) 9:22–31. doi: 10.1016/S2213-8587(20)30369-7
33. Zinman B, Lachin JM, Inzucchi SE. Empagliflozin, cardiovascular outcomes, and mortality in type 2 diabetes. N Engl J Med. (2016) 374:1094. doi: 10.1056/NEJMc1600827
34. Kidney Disease: Improving Global Outcomes (KDIGO) Diabetes Work Group. KDIGO 2020 clinical practice guideline for diabetes management in chronic kidney disease. Kidney Int. (2020) 98:S1–S115. doi: 10.1016/j.kint.2020.06.019
35. DeFronzo RA, Reeves WB, Awad AS. Pathophysiology of diabetic kidney disease: impact of SGLT2 inhibitors. Nat Rev Nephrol. (2021) 17:319–34. doi: 10.1038/s41581-021-00393-8
36. Vallon V, Thomson SC. Renal function in diabetic disease models: the tubular system in the pathophysiology of the diabetic kidney. Annu Rev Physiol. (2012) 74:351–75. doi: 10.1146/annurev-physiol-020911-153333
37. Ruggenenti P, Porrini EL, Gaspari F, Motterlini N, Cannata A, Carrara F, et al. Glomerular hyperfiltration and renal disease progression in type 2 diabetes. Diabetes Care. (2012) 35:2061–8. doi: 10.2337/dc11-2189
38. Babel RA, Dandekar MP. A review on cellular and molecular mechanisms linked to the development of diabetes complications. Curr Diabetes Rev. (2021) 17:457–73. doi: 10.2174/1573399816666201103143818
39. Shibata S, Nagase M, Yoshida S, Kawarazaki W, Kurihara H, Tanaka H, et al. Modification of mineralocorticoid receptor function by Rac1 GTPase: implication in proteinuric kidney disease. Nat Med. (2008) 14:1370–6. doi: 10.1038/nm.1879
40. Garg R, Hurwitz S, Williams GH, Hopkins PN, Adler GK. Aldosterone production and insulin resistance in healthy adults. J Clin Endocrinol Metab. (2010) 95:1986–90. doi: 10.1210/jc.2009-2521
41. Bădilă E. The expanding class of mineralocorticoid receptor modulators: New ligands for kidney, cardiac, vascular, systemic and behavioral selective actions. Acta Endocrinol Buchar. Rom. (2020) 16:487–96. doi: 10.4183/aeb.2020.487
42. Griesler B, Schuelke C, Uhlig C, Gadasheva Y, Grossmann C. Importance of micromilieu for pathophysiologic mineralocorticoid receptor activity-when the mineralocorticoid receptor resides in the wrong neighborhood. Int J Mol Sci. (2022) 23:12592. doi: 10.3390/ijms232012592
43. Patera F, Gatticchi L, Cellini B, Chiasserini D, Reboldi G. Kidney fibrosis and oxidative stress: from molecular pathways to new pharmacological opportunities. Biomolecules. (2024) 14:137. doi: 10.3390/biom14010137
44. Mende CW, Samarakoon R, Higgins PJ. Mineralocorticoid receptor-associated mechanisms in diabetic kidney disease and clinical significance of mineralocorticoid receptor antagonists. Am J Nephrol. (2023) 54:50–61. doi: 10.1159/000528783
45. Lo KB, Rangaswami J, Vaduganathan M. Non-steroidal mineralocorticoid receptor antagonists and cardiorenal outcomes in chronic kidney disease. Nephrol. Dial. Transplant. Off Publ. Eur Dial. Transpl. Assoc - Eur Ren. Assoc. (2023) 38:845–54. doi: 10.1093/ndt/gfac322
46. Chung EY, Ruospo M, Natale P, Bolignano D, Navaneethan SD, Palmer SC, et al. Aldosterone antagonists in addition to renin angiotensin system antagonists for preventing the progression of chronic kidney disease. Cochrane Database Syst Rev. (2020) 10:CD007004. doi: 10.1002/14651858.CD007004.pub4
47. Agarwal R, Kolkhof P, Bakris G, Bauersachs J, Haller H, Wada T, et al. Steroidal and non-steroidal mineralocorticoid receptor antagonists in cardiorenal medicine. Eur Heart J. (2021) 42:152–61. doi: 10.1093/eurheartj/ehaa736
48. Verma A, Patel AB. Finerenone: A non-steroidal mineralocorticoid receptor blocker for diabetic kidney disease. Trends Endocrinol Metab TEM. (2021) 32:261–3. doi: 10.1016/j.tem.2021.02.002
49. Research, C. for D.E. FDA approves drug to reduce risk of serious kidney and heart complications in adults with chronic kidney disease associated with type 2 diabetes. FDA (2021). Available online at: https://www.fda.gov/drugs/news-events-human-drugs/fda-approves-drug-reduce-risk-serious-kidney-and-heart-complications-adults-chronic-kidney-disease.
50. Kabir M, Bergman RN, Porter J, Stefanovski D, Paszkiewicz RL, Piccinini F, et al. Dapagliflozin prevents abdominal visceral and subcutaneous adipose tissue dysfunction in the insulin-resistant canine model. Obes Silver Spring Md. (2023) 31:1798–811. doi: 10.1002/oby.23771
51. Griffin M, Rao VS, Ivey-Miranda J, Fleming J, Mahoney D, Maulion C, et al. Empagliflozin in heart failure: diuretic and cardiorenal effects. Circulation. (2020) 142:1028–39. doi: 10.1161/CIRCULATIONAHA.120.045691
52. Petrie MC, Verma S, Docherty KF, Inzucchi SE, Anand I, Belohlávek J, et al. Effect of dapagliflozin on worsening heart failure and cardiovascular death in patients with heart failure with and without diabetes. JAMA. (2020) 323:1353–68. doi: 10.1001/jama.2020.1906
53. Ravindran S, Munusamy S. Renoprotective mechanisms of sodium-glucose co-transporter 2 (SGLT2) inhibitors against the progression of diabetic kidney disease. J Cell Physiol. (2022) 237:1182–205. doi: 10.1002/jcp.30621
54. Cohen S, Sternlicht H, Bakris GL. Mineralocorticoid receptor antagonists in the treatment of diabetic kidney disease: their application in the era of SGLT2 inhibitors and GLP-1 receptor agonists. Curr Diab Rep. (2022) 22:213–8. doi: 10.1007/s11892-022-01461-4
55. Patel V, Joharapurkar A, Jain M. Role of mineralocorticoid receptor antagonists in kidney diseases. Drug Dev Res. (2021) 82:341–63. doi: 10.1002/ddr.21760
56. Barrera-Chimal J, Kolkhof P, Lima-Posada I, Joachim A, Rossignol P, Jaisser F. Differentiation between emerging non-steroidal and established steroidal mineralocorticoid receptor antagonists: head-to-head comparisons of pharmacological and clinical characteristics. Expert Opin Investig Drugs. (2021) 30:1141–57. doi: 10.1080/13543784.2021.2002844
57. Grossmann C, Almeida-Prieto B, Nolze A, Alvarez de la Rosa D. Structural and molecular determinants of mineralocorticoid receptor signalling. Br J Pharmacol. (2022) 179:3103–18. doi: 10.1111/bph.15746
58. Toyama T, Neuen BL, Jun M, Ohkuma T, Neal B, Jardine MJ, et al. Effect of SGLT2 inhibitors on cardiovascular, renal and safety outcomes in patients with type 2 diabetes mellitus and chronic kidney disease: A systematic review and meta-analysis. Diabetes Obes Metab. (2019) 21:1237–50. doi: 10.1111/dom.13648
59. Zhang Y, Jiang L, Wang J, Wang T, Chien C, Huang W, et al. Network meta-analysis on the effects of finerenone versus SGLT2 inhibitors and GLP-1 receptor agonists on cardiovascular and renal outcomes in patients with type 2 diabetes mellitus and chronic kidney disease. Cardiovasc Diabetol. (2022) 21:232. doi: 10.1186/s12933-022-01676-5
Keywords: diabetic kidney disease, sodium-glucose cotransporter-2 inhibitor, non-steroid mineralocorticoid receptor antagonists, network meta-analysis, cardiorenal
Citation: Yang S-Q, Zhao X, Zhang J, Liu H, Wang Y-H and Wang Y-G (2024) Comparative efficacy and safety of SGLT2is and ns-MRAs in patients with diabetic kidney disease: a systematic review and network meta-analysis. Front. Endocrinol. 15:1429261. doi: 10.3389/fendo.2024.1429261
Received: 07 May 2024; Accepted: 17 June 2024;
Published: 04 July 2024.
Edited by:
Divya Bhatia, NewYork-Presbyterian, United StatesReviewed by:
Aditya Yashwant Sarode, Columbia University, United StatesMineaki Kitamura, Nagasaki University, Japan
Copyright © 2024 Yang, Zhao, Zhang, Liu, Wang and Wang. This is an open-access article distributed under the terms of the Creative Commons Attribution License (CC BY). The use, distribution or reproduction in other forums is permitted, provided the original author(s) and the copyright owner(s) are credited and that the original publication in this journal is cited, in accordance with accepted academic practice. No use, distribution or reproduction is permitted which does not comply with these terms.
*Correspondence: Yao-Guang Wang, Yaoguangwa@163.com; Xi Zhao, zhaoxi614@163.com