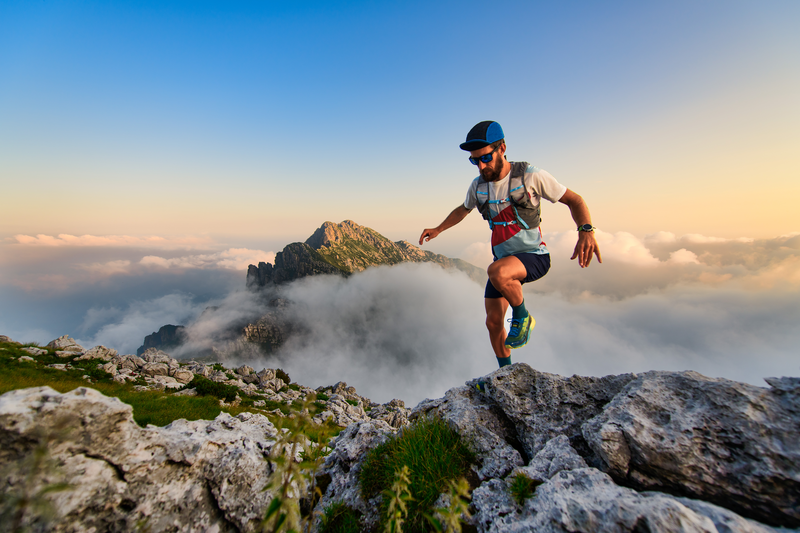
94% of researchers rate our articles as excellent or good
Learn more about the work of our research integrity team to safeguard the quality of each article we publish.
Find out more
SYSTEMATIC REVIEW article
Front. Endocrinol. , 27 August 2024
Sec. Bone Research
Volume 15 - 2024 | https://doi.org/10.3389/fendo.2024.1426490
Background and aims: Reduced bone mineral density (BMD) and microarchitectural deterioration contribute to increased fracture risk. Although the effects of anti-fracture medications (AFMs) on BMD are well-documented, their impact on bone material properties (BMPs) remains poorly characterized. Accordingly, we conducted a systematic review and meta-analysis to evaluate the effects of AFMs on BMPs. Based on data availability, we further categorized AFMs into anti-resorptives, bisphosphonates alone, and strontium ranelate subgroups to perform additional analyses of BMPs in osteoporotic patients.
Methods: We did a comprehensive search of three databases, namely, PubMed, Web of Science, and Google Scholar, using various permutation combinations, and used Comprehensive Meta-Analysis software to analyze the extracted data.
Results: The 15 eligible studies (randomized and non-randomized) compared the following: (1) 301 AFM-treated patients with 225 on placebo; (2) 191 patients treated with anti-resorptives with 131 on placebo; (3) 86 bisphosphonate-treated patients with 66 on placebo; and (4) 84 strontium ranelate-treated patients with 70 on placebo. Pooled analysis showed that AFMs significantly decreased cortical bone crystallinity [standardized difference in means (SDM) −1.394] and collagen maturity [SDM −0.855], and collagen maturity in cancellous bone [SDM −0.631]. Additionally, anti-resorptives (bisphosphonates and denosumab) significantly increased crystallinity [SDM 0.387], mineral–matrix ratio [SDM 0.771], microhardness [SDM 0.858], and contact hardness [SDM 0.952] of cortical bone. Anti-resorptives increased mineral–matrix ratio [SDM 0.543] and microhardness [SDM 0.864] and decreased collagen maturity [SDM −0.539] in cancellous bone. Restricted analysis of only bisphosphonate-treated studies showed a significant decrease in collagen maturity [SDM −0.650] in cancellous bone and an increase in true hardness [SDM 1.277] in cortical bone. In strontium ranelate-treated patients, there was no difference in BMPs compared to placebo.
Conclusion: Collectively, our study suggests that AFMs improve bone quality, which explains their anti-fracture ability that is not fully accounted for by increased BMD in osteoporosis patients.
Osteoporosis, primarily age- and menopause-related, leads to declining bone strength in older individuals. Areal bone mineral density (aBMD) is a standard measure for diagnosis, but it only accounts for 30%–40% of bone strength variation (1, 2). Other factors like bone geometry, microarchitecture, mineralization, collagen integrity, and biomaterial composition also impact bone quality (2–4). Assessing these parameters aids in fracture risk identification and developing better bone health strategies.
Bone health in adults is maintained by a continuous process of bone remodeling, in which old damaged bone is resorbed by osteoclasts and subsequently replaced by new bone by osteoblasts. In healthy individuals, this remodeling process is balanced, with the amount of bone resorbed is replaced by almost an equal amount of new bone, ensuring the maintenance of bone strength and integrity. In osteoporosis, the bone remodeling rate is increased. Since the bone resorption phase that precedes the bone formation phase has a much shorter duration, increases in the remodeling rate lead to net bone loss (5, 6). In this regard, anti-resorptives, the drugs that suppress bone remodeling including bisphosphonates (BPs), neutralizing antibody against RANKL [denosumab (DMab)], and raloxifene (Ral), a selective estrogen receptor modulator (SERM), prevent bone loss over time and increase BMD (7, 8). As bone remodeling is a physiological process, inhibiting it could potentially affect the quality of the bone matrix.
Besides increases in bone resorption, osteoporosis is also marked by an impairment in new bone formation (9). In this context, osteo-anabolic drugs, including teriparatide (TPTD) and abaloparatide, signaling through the type 1 PTH receptor, increase BMD by stimulating osteoblast number and function without affecting the function of osteoclasts (9, 10). TPTD and abaloparatide maintain a higher rate of bone remodeling in which there is a time window when formation exceeds resorption, lasting approximately 2 years, often referred to as “anabolic window” (11). Romosozumab, a neutralizing antibody against sclerostin, although believed to have a dual action of promoting bone formation and inhibiting resorption, displayed a limited period when bone formation exceeds resorption (12, 13). Strontium ranelate (SR) showed dual action in preclinical studies, but it did not show significant osteoanabolic effect in clinical trials (14). Considering that remodeling–suppressing drugs and osteoanabolic drugs have two distinct mechanisms of action and target different aspects of bone remodeling, they can lead to variations in bone material composition, matrix properties, and strength.
To understand the effects of anti-fracture medications (AFMs) on bone material and strength, we conducted a systematic review and meta-analyses by comparing outcomes in osteoporosis patients on medication with those on a placebo. We then classified the AFM groups according to their modes of action (anti-resorptive and anabolic) and investigated their effects on bone material and strength measures. Various measurement techniques, including Raman spectroscopy (RS), Fourier transform infrared imaging (FTIRI), quantitative back-scattered electron imaging (qBEI), quantitative micro-radiography (QMR), x-ray diffraction (XRD), and nanoindentation, were employed to assess bone composition and mechanical properties. RS and FTIRI determine the chemical composition of bone, including collagen maturity, the mean degree of mineralization, and carbonate/phosphate and carbonate/amide-I ratios (15, 16). qBEI and QMR quantified mineral content and distribution within bone tissue, revealing mineralization state and density. XRD examined bone mineral crystal structure, assessing crystallinity and orientation (17). Nanoindentation and microindentation (Vickers indentation), which measure dynamic and static mechanical properties, such as hardness and elasticity, offer insights into bone strength and resilience (18). By analyzing this evidence, we sought to determine the effectiveness of various AFMs in improving bone quality and strength properties in osteoporosis patients.
We searched three electronic databases, namely, PubMed, Web of Science, and Google Scholar, until 20 July 2024, to identify the studies that assessed the effect of AFM on bone quality, and articles were exported to Endnote. The search strategy was a combination of keywords and Boolean operators and was limited to original articles published in the English language (Supplementary Table 1). The search terms included: (“bisphosphonates” OR “alendronate” OR “residronate” OR “zoledronic acid” OR “ibandronate” OR “etidronate” OR “denosumab” OR “teriparatide” OR “abaloparatide” OR “raloxifene” OR “romosozumab” OR “strontium ranelate”), (“human” OR “men” OR “women”), (“bone quality” OR “mineral matrix ratio” OR “cross-linking” OR “carbonate phosphate ratio” OR “crystallinity” OR “water content” OR “heterogeneity index” OR “heterogeneity” OR “microdamage” OR “microdamage accumulation” OR “microdamage propagation”), (“Raman spectroscopy” OR “Fourier transform infrared imaging” OR “quantitative back-scattered electron imaging” OR “quantitative micro-radiography” OR “X-ray diffraction, OR “nanoindentation”), (“osteoporosis” OR “post-menopausal osteoporosis” OR “age-related Osteoporosis” OR “chronic kidney disease” OR “glucocorticoid-induced osteoporosis” OR “hypertension” OR “inflammatory bowel disease” OR “diabetes” OR “arthritis”). The PRISMA flowchart depicts the findings of the literature search (Figure 1).
Figure 1. The flow of information is shown in a PRISMA diagram. n = the number of studies; black arrows indicate studies that were included in the meta-analyses; red arrows indicate excluded studies.
The literature search results were screened based on inclusion/exclusion criteria. Inclusion criteria encompassed original full-text articles related to osteoporosis from various causes [postmenopausal, aging, chronic kidney disease (CKD), glucocorticoid-induced osteoporosis (GIO), hypertension, inflammatory bowel disease (IBD), diabetes, and arthritis]. Exclusion criteria included review articles, case reports, book chapters, letters, editorials, and conference proceedings. No restrictions were imposed on age, gender, or treatment duration.
Three authors (S.S., V.S., and N.C.) independently screened titles, abstracts, and full texts to confirm the eligibility of each study and compiled an Excel sheet (Windows 10 edition; Microsoft Corporation, Lisbon, Portugal) depicting the following parameters: author name, year of publication, techniques used, gender, age, and bone-related parameters. Data from each article were retrieved in numeric form from the tables and bar graphs using the WebPlotDigitilizer program.
Study quality was assessed through a checklist, including criteria such as the inclusion of osteoporosis patients, specific treatments, trial type, randomization, informed consent, ethics approval, and conflict of interest statements. We considered bone material and strength as primary outcome measures in response to AFM.
All statistical analyses in this study were conducted using Comprehensive Meta-Analysis Software (CMA) Version 2. Cochran’s Q-test and heterogeneity index (I2) were used to determine the degree of heterogeneity across the studies. The I2 value ranges from 0% to 100%. High heterogeneity is present when I2 value is >75%, moderate heterogeneity is present when I2 value is 50%, and low heterogeneity is present when I2 value is <25%. We appropriately applied the fixed- and random-effect models to calculate the pooled effect size, as previously described (19). The fixed-effect model assumes that different studies estimate the same effect, whereas the random-effect model assumes that different studies estimate different effects (19).
The level of sensitivity for each bone quality parameter was determined by a single study exclusion statistic using the CMA software as described previously (19). We examined publication bias qualitatively using the funnel plot and quantitatively using Egger’s regression intercept test (20), Begg and Mazumdar rank correlation test (21), and Duval and Tweedie’s trim-and-fill method (22).
Data from each article were extracted and manually entered in numeric form. The data were presented in a Microsoft Excel spreadsheet (Windows 10 edition; Microsoft Corporation, Lisbon, Portugal) to record treatment, dose, treatment duration, study design, gender, disease, sample size, number of patients, age, and techniques used for quantifying bone quality. For the current study, references were managed using Endnote X7 (Thomson Reuters, Toronto, Canada), and data were analyzed using Comprehensive Meta-Analysis software.
A literature search for the effect of AFM identified 44,626 articles: PubMed and Web of Science (n = 9,726), and Google Scholar (n = 34,900). Of these, 44,533 studies were excluded for being either reviews or not relevant and the remaining 93 were eligible after screening for the titles and abstracts. Of these, 35 studies were duplicates and the data were unextractable in 25 studies, leaving 33 studies that underwent full-text assessment. Of the 33 studies, 18 were excluded due to the following reasons: (a) patients who received a prior AFM different from the one being studied for BMPs, to avoid confounding effects and ensure accurate interpretation of outcomes; (b) no control group; and (c) data were not presented as mean ± SD/SEM. The remaining 15 studies of AFM were eligible for meta-analyses. Of these studies, six used BPs, two used DMab, five used SR, one used TPTD, and one used Ral (Figure 1); all studies were published from 2003 to 2022 (16, 23–36). Patient details are provided in Table 1.
We first pooled data across all AFMs to study their combined effects on BMPs. We then categorized AFMs by their mode of action (anti-resorptive vs. anabolic) and investigated their effects on BMPs. Adequate data on anti-resorptive drugs (BPs, DMab, and Ral) allowed us to form this category and analyze their impact on bone material and strength. Subgroup analyses of BPs and SR were possible due to sufficient datasets, comparing their effects to placebo in osteoporotic patients. However, insufficient data for DMab, Ral, and the anabolic agent teriparatide prevented individual assessments of these treatments.
QMR and XRD measured degree of mineralization of bone (DMB), representing the amount of mineral in a unit volume of tissue matrix (37, 38). Pooled data from three studies (nine datasets) for a total skeleton, standardized difference in means [(SDM) = 0.230, 95% CI = −0.038 to 0.498, p = 0.093, I2 = 0.000; Q = 3.326, p = 0.912], four studies (11 datasets) for cortical bones (SDM = 0.206, 95% CI = −0.033 to 0.445, p = 0.091, I2 = 0.000; Q = 3.033, p = 0.981), and three studies (10 datasets) for cancellous bones (SDM = 0.196, 95% CI = −0.049 to 0.441, p = 0.117, I2 = 0.000; Q = 7.172, p = 0.619) (Supplementary Figures 1A–C) revealed that AFM did not affect DMB (Table 2).
Heterogeneity index (HI) is the heterogeneity of the distribution of the DMB, calculated as the width at half-maximum of the curve quantified by QMR (32, 34). Pooled data from three studies (nine datasets) for cortical bones (SDM = 0.020, 95% CI = −0.274 to 0.313, p = 0.896, I2 = 28.920; Q = 11.255, p = 0.188) and two studies (eight datasets) for cancellous bones (SDM = 0.434, 95% CI = −0.163 to 1.030, p = 0.154, I2 = 70.914; Q = 24.067, p = 0.001) (Supplementary Figures 2A, B) revealed that AFM had no significant impact on HI (Table 2).
FTIR-based measurement of crystallinity reflects the size and shape of hydroxyapatite crystals. Appropriate packing of thin nanocrystals of carbonated apatite and calcium phosphate within the organic matrix is an important determinant of bone strength with smaller-sized crystals improving mechanical behavior (39). Pooled data were available from six studies (10 datasets) for cortical bones and five studies (7 datasets) for cancellous bones, which revealed that AFM significantly reduced mineral crystallinity (XST) in cortical (SDM = −1.394, 95% CI = −2.525 to −0.263, p = 0.016, I2 = 92.550; Q = 120.808, p = 0.000) (Figure 2A) and cancellous bones (SDM = −0.902, 95% CI = −1.837 to 0.034, p = 0.059, I2 = 87.134; Q = 46.634, p = 0.000) (Supplementary Figure 3A) compared with placebo (Table 2).
Figure 2. Forest plot showing the impact of AFM on (A) XST and (B, C) XLR at the indicated sites. The Z-value denotes the strength and degree of the relationship, and the p-value indicates the significance of outcomes. A fixed- or random-effect model was used, as indicated in the red box.
FTIR-based measurement of mineral-to-matrix ratio (MMTR) represents the amount of mineral ion substitution in the collagenous matrix in the bone tissue and is a determinant of bone fragility and strength (40). Data from four studies provided a total of seven datasets at the cortical site and five datasets at the cancellous site. Analysis indicated no significant difference in MMTR between patients who received AFM and those on a placebo, in both cortical (SDM = −1.304, 95% CI = −2.815 to 0.207 and, p = 0.091, I2 = 95.207; Q = 125.177, p = 0.000) (Supplementary Figure 3B) and cancellous bones (SDM = −0.668, 95% CI = −1.931 to 0.596, p = 0.300, I2 = 92.545; Q = 53.656, p = 0.000) (Supplementary Figure 3C; Table 2).
FTIR-based measurement of carbonate/phosphate ratio (C/P) represents how much PO43− ion in the organic matrix is exchanged by a CO32− ion (41). Data from three studies provided as many datasets for cortical (SDM = −0.093, 95% CI = −0.443 to 0.257, p = 0.603, I2 = 0.000; Q = 1.935, p = 0.380) (Supplementary Figure 4A) and cancellous bones (SDM = −0.255, 95% CI = −0.998 to 0.487, p = 0.500, I2 = 72.428; Q = 7.254, p = 0.027) (Supplementary Figure 4B), revealing no effect of AFM on the C/P ratio (Table 2).
FTIR and RS techniques were used to evaluate collagen maturity ratio (XLR), which indicates the proportion of mature to immature cross-links and is influenced by both age and bone turnover (42). Eight datasets from five studies and seven datasets from six studies were available for pooled analysis for cortical (Figure 2B) and cancellous bones (Figure 2C), respectively. AFM reduced XLR in cortical (SDM = −0.855, 95% CI = −1.481 to −0.229, p = 0.007, I2 = 78.942; Q = 33.241, p = 0.000) and cancellous bones (SDM = −0.631, 95% CI = −0.913 to −0.348, p = 0.000, I2 = 24.815; Q = 7.980, p = 0.240) compared with placebo (Table 2).
Pooled data from three studies (seven datasets) for cortical bones (SDM = 0.208, 95% CI = −0.066 to 0.482, p = 0.136, I2 = 0.000; Q = 1.055, p = 0.983) (Supplementary Figure 5A) and two studies (six datasets) for cancellous bones (SDM = 0.165, 95% CI = −0.117 to 0.448, p = 0.252, I2 = 0.000; Q = 4.806, p = 0.440) (Supplementary Figure 5B) revealed that anti-resorptive drugs (BPs and Ral) did not affect DMB (Table 3).
Pooled data from four studies (four datasets) for cortical bones and three studies (three datasets) for cancellous bones revealed that compared with the placebo group, the anti-resorptives (BPs and DMAb) significantly increased XST in the cortical (SDM = 0.387, 95% CI = 0.048 to 0.726, p = 0.025, I2 = 0.000; Q = 2.220, p = 0.528) (Figure 3A) but not in the cancellous bone (SDM = −0.140, 95% CI = −0.698 to 0.417, p = 0.622, I2 = 53.275; Q = 4.280, p = 0.118) (Supplementary Figure 6A) (Table 3).
Figure 3. Forest plot showing the impact of anti-resorptive drugs on (A) XST, (B) XLR, and (C, D) MMTR at the indicated sites, compared with the placebo group. A fixed-effect model was used.
Pooled data from four studies (four datasets) were available for cortical (Supplementary Figure 6B) and cancellous bones (Figure 3B). Anti-resorptive drugs (BPs and DMAb) decreased XLR in the cancellous bone (SDM = −0.539, 95% CI = −0.872 to −0.206, p = 0.002, I2 = 0.000; Q = 1.535, p = 0.674) compared with placebo (Figure 3B), but had no effect in cortical bones (SDM = − 0.122, 95% CI = −0.457 to 0.214, p = 0.477, I2 = 0.000; Q = 1.287, p = 0.732) (Supplementary Figure 6B) (Table 3).
Pooled data from three studies (three datasets) were available for cortical (SDM = 0.771, 95% CI = 0.407 to 1.134, p = 0.000, I2 = 48.245; Q = 3.864, p = 0.145) (Figure 3C) and cancellous (SDM = 0.543, 95% CI = 0.188 to 0.899, p = 0.003, I2 = 0.000; Q = 0.477, p = 0.788) bones (Figure 3D). BPs and DMAb increased MMTR at both sites compared with the placebo group (Table 3).
Micro-hardness (MH) obtained by the microindentation technique (Vickers hardness test) is a measure of bone’s mechanical properties during deformation at the levels of the bone structural unit (43, 44). Pooled data from two studies (five datasets) were available for cortical (SDM = 0.858, 95% CI = 0.551 to 1.166, p = 0.000, I2 = 0.000; Q = 2.652, p = 0.618) (Figure 4A) and cancellous bones (SDM = 0.864, 95% CI = 0.230 to 1.498, p = 0.008, I2 = 73.858; Q = 15.301, p = 0.004) (Figure 4B). Anti-resorptive drugs (BPs and DMAb) significantly increased MH at both sites compared with placebo (Table 3).
Figure 4. Forest plot showing the effect of anti-resorptive drugs on (A, B) MH and (C) Hc at indicated sites. A fixed- or random-effect model was used, as indicated in the red box.
Bone hardness, encompassing both elastic and plastic deformation, was assessed using nanoindentation. Contact hardness (Hc) was calculated from both elastic and plastic properties using the Oliver–Pharr method (35, 36). For the cortical bones, pooled data from three studies (four datasets) for Hc (SDM = 0.952, 95% CI = 0.092 to 1.811, p = 0.030, I2 = 66.684; Q = 9.005, p = 0.029) (Figure 4C) were available. Anti-resorptive drugs (BPs and DMAb) significantly increased Hc compared with placebo (Table 3).
Elastic modulus (EM) is the measure of elastic properties of bone material. EM is a function of the unloading stiffness of the force–displacement curve, as measured by the nanoindentation method (36). Pooled data from three studies (4 datasets) were available from cortical bone (SDM = 0.190, 95% CI = −0.258 to 0.637, p = 0.406, I2 = 26.708; Q = 4.093, p = 0.252) that showed no significant effect of anti-resorptive drugs (BPs and DMAb) on EM (Supplementary Figure 6C; Table 3).
Pooled analysis from two studies (five datasets) for cortical DMB (SDM = 0.205, 95% CI = −0.155 to 0.566, p = 0.265, I2 = 0.000; Q= 0.806, p= 0.938) (Supplementary Figure 7A; Table 4) and three studies (three datasets) for cortical XST (SDM = 0.252, 95% CI = −0.230 to 0.734, p = 0.306, I2 = 0.000; Q = 1.624, p = 0.444) (Supplementary Figure 7B; Table 4) showed no significant difference between the BP-treated and placebo groups.
Three datasets from as many studies were analyzed for cancellous and cortical bones. Pooled analysis showed that cancellous XLR was significantly reduced (SDM = −0.650, 95% CI = −1.118 to −0.181, p = 0.007, I2 = 0.000; Q = 3.697, p = 0.448) in BP-treated patients compared with the placebo (Figure 5; Table 4). However, there was no change at the cortical site (SDM = −0.112, 95% CI = −0.593 to 0.368, p = 0.646, I2 = 0.000; Q = 1.284, p = 0.526) (Supplementary Figure 7C; Table 4).
Figure 5. Forest plot showing the effect of BPs on (A) XLR and (B) H compared to placebo. A fixed- or random-effect model was used, as indicated by a red box.
True hardness (H) represents resistance to purely plastic deformation relative to the EM (35, 36). For the cortical bones, pooled data from two studies (three datasets) for H (SDM = 1.277, 95% CI = 0.040 to 2.514 and p = 0.043, I2 = 72.684; Q = 7.322, p = 0.026) (Figure 5B) were available. BPs significantly increased H compared with placebo (Table 4).
At the cortical site, two studies (three datasets) were analyzed for Hc (SDM = 0.944, 95% CI = −0.399 to 2.286, p = 0.168, I2 = 77.769; Q = 8.997, p = 0.011) (Supplementary Figure 8A; Table 4) and EM data (SDM = −0.043, 95% CI = −0.609 to 0.522, p = 0.881, I2 = 14.890; Q = 2.350, p = 0.309) (Supplementary Figure 8B; Table 4), which showed no difference between the BP-treated and placebo groups.
Since a sufficient number of studies were available for examining the effect of SR on bone quality and strength, we went ahead with conducting a meta-analysis. SR is used as a treatment for postmenopausal osteoporosis as it has a dual action on bone remodeling by increasing both bone formation and decreasing bone resorption, resulting in the prevention of bone loss and an increase in bone mass and strength (32, 34). Therefore, in this present study, SR could not be included in either the category of anti-resorptive or anabolic.
Pooled analysis of two studies (seven datasets) for total bones DMB (SDM = 0.270, 95% CI = −0.077 to 0.618, p = 0.127, I2 = 0.000; Q = 3.128, p = 0.793) (Supplementary Figure 9A) and HI (SDM = 0.588, 95% CI = −0.014 to 1.190, p = 0.055, I2 = 60.335; Q = 15.127, p = 0.019) (Supplementary Figure 9B) showed no differences between the SR-treated and placebo groups (Table 5).
The funnel plot showed an asymmetric distribution in cortical XST (Egger’s regression intercept = 0.51077, p = 0.76503) and cancellous MMTR (Egger’s regression intercept = −1.121539, p = 0.40103) and MH (Egger’s regression intercept = 6.97054, p = 0.00079), suggesting the presence of publication bias in anti-resorptive (BPs and DMab)-treated patients compared to placebo. Thus, we used trim-and-fill analysis to compute unbiased estimates and adjusted the values under the random-effect model. The imputed point estimate for XST was 0.36090 (95% CI = 0.72583 –0.68741), MMTR was 0.54344 (95% CI = 0.18831 – 0.89857), and MH was 0.26402 (95% CI = −0.37207 – 0.90011). Based on Egger’s regression, Duval and Tweedie’s trim and fill, and Begg and Mazumdar’s rank correlation test, we conclude that for the majority of measurements, the computed effects are free of publication bias (Table 6).
The present study revealed modest changes; within 10% of the pooled estimates and the 95% CIs. No single study was sufficiently sensitive to change the results.
In the pooled analysis, we observed that in comparison to placebo, AFM decreased XST (cortical) and XLR (at both compartments). Subgroup analyses revealed that (a) anti-resorptive drugs (BPs and DMab) increased cortical XST, Hc, MMTR, and MH (at both compartments), and decreased cortical XLR; (b) BPs reduced XLR at the cancellous site and increased H at cortical site compared to placebo; and (c) SR did not affect either the bone material or strength. Assessments of study quality, heterogeneity, publication biases, and sensitivity analysis verified the validity of the findings, suggesting that AFM favorably altered bone material and mechanical behavior.
Anti-resorptive medication, by increasing bone mass, may have led to an enhanced MMTR by inhibiting osteoclast activity, reducing the bone remodeling rate, and refiling the remodeling area (45, 46). Despite the rise in MMTR, DMB remained unchanged, suggesting that anti-resorptive medications maintain the mineral–organic matrix balance, enhancing stiffness and hardness, and thereby increasing resistance to fracture. Since all studies were from BPs and Ral treatments, the effect of other classes of drugs on DMB needs to be studied.
The C/P ratio is an essential indicator of the chemical composition of the mineral component of bone and an increased C substitution of P in the hydroxyapatite lattice has a negative impact on bone mechanical strength (47, 48). The lack of effect of AFM on the C/P ratio suggests that the bone’s mineralization pattern and maturity level remained relatively stable during the treatment.
AFM in a pooled analysis showed decreased XST, suggesting that hydroxyapatite crystals in bone are less perfect and have smaller crystal sizes that could enhance the energy-absorbing capacity of bone, making it less prone to fractures. Less perfect crystals enhance heterogeneity in bone microarchitecture, composition, and density and are less likely to propagate microfractures through the bone structure. This may contribute to enhanced bone strength and decreased fragility by making it more difficult for microfractures to propagate within the bone. The decrease in XST could be attributable to TPTD, which is known to lower it, resulting in reduced matrix mineralization (29, 49) similar to that seen in young bones. In contrast, a pooled analysis of anti-resorptive medications revealed that DMab appeared to be the main contributor to increased XST through lowering the rate of bone remodeling, which further supports the transition of newly deposited crystals to more mature crystals in size/perfection and accelerates the rate of secondary matrix mineralization (45, 46).
Collagen properties including composition and cross-links (XLR) contribute to bone’s elastic property and only the data on XLR were available in the selected studies. The primary determinant of XLR is the ratio between mature and immature cross-links within the collagen matrix. Higher collagen maturity, which represents mature collagen, typically implies a greater degree of organization and cross-linking of collagen fibers, resulting in a stronger and more stable collagen network within a tissue. Lysyl oxidase (LOX) drives the formation of enzymatic cross-links between and within the collagen fibrils to impart stability to the bone matrix and provide mechanical strength. LOX first catalyzes the formation of divalent reducible collagen cross-links, dehydro-hydoxylysino-norleucine and hydroxylysino-norleucine, which are then matured to become trivalent pyridinium cross-links such as pyrdinoline (PYD), deoxypyridinoline (DPD), and pyrrolic analogs by interacting with aldehyde groups (42, 50). The contribution of enzymatic cross-linking in bone strength has been demonstrated in animal studies, where LOX inhibition by beta aminoproprionitrile resulted in a 50% decrease in pyridinium cross-links leading to reductions in bending strength and modulus of cortical bone, and compressive energy of cancellous bone without affecting stiffness (51, 52). In the vertebrae of human cadaveric specimens, compressive strength correlated with the PYD/DPD ratio but not their concentrations or BMD (53). However, in another study, the degree of anisotropy (orientation and connectivity of trabeculae with the bone matrix) of vertebrae was correlated with vertebral compression strength but not PYD, DPD, and pentosidine (54). Thus, it appears that trabecular microarchitecture contributes to vertebral strength and is notably unaffected by matrix-level composition such as cross-link profile (55). In another study, Patrick Garnero et al. used an in vitro model of fetal bovine cortical bone specimens, incubated them at 37°C for 60 days, and showed an increased level of PYD and DPD that were associated with a 30% decrease in bending and compressive yield stress and a 2.5-fold increase in compressive post-yield energy absorption, with no significant change in bone stiffness (56). Thus, an inconclusive relationship was noticed across the studies among cross-linking and strength parameters. Therefore, the extent of enzymatic cross-links that contribute to bone strength at physiological levels remains unclear. AFM and anti-resorptive drugs in pooled analysis lowered XLR, suggesting weaker cross-links. Because the anti-resorptive drugs suppress bone remodeling, the normal turnover of collagen may be disrupted, potentially leading to the accumulation of older collagen and a decrease in collagen maturity. Indeed, subgroup analysis, which was possible only with BPs, showed a significant decrease in XLR compared with placebo. The combined effects of increased BMD and improved mineral properties by the AFM/anti-resorptive drugs including BPs could outweigh the potential negative impact of decreased XLR. Moreover, decreased XLR could reduce the stress concentrations that lead to fractures. However, there are no studies on the types of collagen present, their relative proportions, and their organization within the bone, which could have shed more light on the mechanical behavior of bone.
Nanoindentation measurements provide an understanding of bone mechanics at the microscale, including MH indices, Hc, H, and EM. These measurements specifically determine bone’s resilience to microdamage and its overall material behavior in response to small loads at a very localized level, dependent on hardness and EM (18, 35, 36). While hardness measurements were increased by BPs alone and anti-resorptive drugs (BPs and DMab), EM remained unchanged by these treatments. Furthermore, increased MMTR likely contributed to an increase in the hardness parameters, which could enhance resistance against micro-fractures and their accumulation (57). Thus, it appears that the reduction in fracture risk by AFM goes beyond merely increasing BMD, as it maintains a healthy mineral composition and promotes proper mineralization and deposition within the bone matrix. These effects could contribute to bone’s improved structural integrity and ability to absorb and distribute mechanical forces, reducing the risk of fractures.
The major limitation of this meta-analysis is the inclusion of both randomized control trials (RCTs) and non-RCT studies because the number of RCT-designed studies was insufficient for conducting a meta-analysis. Second, of the various types of osteoporosis, we were able to obtain sufficient data concerning the impacts of AFM on post-menopausal and age-related osteoporosis. The number of studies on the effects of such medications on osteoporosis caused by CKD, GIO, hypertension, IBD, diabetes, and arthritis was insufficient to allow for a meta-analysis. Third, owing to the absence of a placebo group and small sample size, we could not conduct a subgroup analysis to assess the impact of TPTD, DMab, and Ral treatments on bone quality and strength in osteoporosis patients. In addition, in a few studies involving TPTD treatment, patients had previously undergone treatment with BPs (49, 58, 59). Consequently, the specific effects attributed to each drug could not be delineated, leading to the exclusion of these studies from the present meta-analysis. Fourth, High-Resolution Peripheral Quantitative Computed Tomography (HR-pQCT) provides 3D reconstituted data on bone microarchitecture at peripheral sites that go beyond BMD and reveals how bone structures are arranged, interconnected, and oriented, which is crucial for bone strength. There are no studies assessing the effect of anti-osteoporosis drugs on HR-pQCT measures. Fifth, Finite Element Analysis (FEA) is based on simulated bone mechanical testing that is employed to predict how bones respond to forces, stresses, and strains. There were insufficient studies evaluating the response of bones to AFM to different simulated loads and predicting areas of high stress or potential failure by the FEA-based technique (60). Sixth, like BMD, all BMPs are presumed to be negatively influenced by age and estrogen deficiency. However, normative data for BMPs are lacking. The included studies vary in recruitment age and treatment duration, leading to insufficient data for consistent age correction of BMPs. Finally, there are concerns regarding the accumulation of microdamage in response to long-term use of BPs potentially affecting bone strength. There are insufficient studies that assess the extent of microdamage accumulation in the bones of BP-treated patients towards the goal of determining the duration of BP treatment that is safe (61, 62).
AFM significantly influences bone quality by decreasing mineral crystallinity and XLR. Anti-resorptive (BPs and DMab) drugs promote mineral maturation, improving hardness and deformation resistance. Long-term BP treatment does not compromise bone strength despite reducing collagen maturity. Further research is needed to investigate the effects of BP therapy duration beyond 8 years. Taken together, these findings underscore the multi-scale impact of AFM on bone quality that encompasses changes in both mineralization and matrix properties.
The original contributions presented in the study are included in the article/Supplementary Material. Further inquiries can be directed to the corresponding authors.
SS: Conceptualization, Data curation, Formal analysis, Investigation, Methodology, Resources, Software, Supervision, Validation, Visualization, Writing – original draft, Writing – review & editing. VS: Methodology, Validation, Writing – review & editing, Formal analysis, Investigation. SR: Conceptualization, Data curation, Formal analysis, Investigation, Methodology, Software, Validation, Visualization, Writing – original draft, Writing – review & editing. AM: Conceptualization, Data curation, Formal analysis, Investigation, Methodology, Supervision, Validation, Visualization, Writing – original draft, Writing – review & editing. SDR: Conceptualization, Data curation, Formal analysis, Funding acquisition, Investigation, Methodology, Project administration, Resources, Software, Supervision, Validation, Visualization, Writing – original draft, Writing – review & editing. NC: Conceptualization, Data curation, Formal analysis, Funding acquisition, Investigation, Methodology, Project administration, Resources, Software, Supervision, Validation, Visualization, Writing – original draft, Writing – review & editing.
The author(s) declare financial support was received for the research, authorship, and/or publication of this article. NC acknowledges a grant from the Council of Scientific and Industrial Research, Government of India (MLP-2035). SDR was partially supported by the National Institute of Arthritis and Musculoskeletal and Skin Diseases (NIAMS) under Award Number AR062103.
The authors declare that the research was conducted in the absence of any commercial or financial relationships that could be construed as a potential conflict of interest.
The author(s) declared that they were an editorial board member of Frontiers, at the time of submission. This had no impact on the peer review process and the final decision.
All claims expressed in this article are solely those of the authors and do not necessarily represent those of their affiliated organizations, or those of the publisher, the editors and the reviewers. Any product that may be evaluated in this article, or claim that may be made by its manufacturer, is not guaranteed or endorsed by the publisher.
The Supplementary Material for this article can be found online at: https://www.frontiersin.org/articles/10.3389/fendo.2024.1426490/full#supplementary-material
Supplementary Figure 1 | Impact of pooled AFM on (A, B) DMB at the indicated- effect sites (a fixed model was used).
Supplementary Figure 2 | Impact of pooled AFM on (A, B) HI at the indicated sites (a fixed- or random-effect model was used, as designated by a red box).
Supplementary Figure 3 | Impact of pooled AFM compared with placebo on (A) XST, and (B, C) MMTR at the indicated sites (a random-effect model was used).
Supplementary Figure 4 | Impact of pooled AFM compared with placebo on (A, B) C/P at the indicated sites. A fixed- or random-effect model was used, as designated by a red box.
Supplementary Figure 5 | Impact of pooled anti-resorptive treatments compared with placebo on (A, B) DMB at the indicated sites. A fixed-effect model was used.
Supplementary Figure 6 | Impact of pooled anti-resorptive treatments compared with placebo on (A) XST, (B) XLR, and (C) EM at the indicated sites. A fixed- or random-effect model was used, as designated by a red box.
Supplementary Figure 7 | Impact of BPs on (A) DMB, (B) XST, and (C) XLR at the indicated sites. A fixed- effect model was used.
Supplementary Figure 8 | Impact of BPs on (A) Hc and (B) EM compared with placebo at the indicated sites. A fixed- or random-effect model was used, as designated by a red box.
Supplementary Figure 9 | Impact of SR on (A) DMB and (B) HI compared with placebo at the indicated sites. A fixed- or random- effect model was used, as designated by a red box.
1. Osterhoff G, Morgan EF, Shefelbine SJ, Karim L, McNamara LM, Augat P. Bone mechanical properties and changes with osteoporosis. Injury. (2016) 47 Suppl 2:S11–20. doi: 10.1016/S0020-1383(16)47003-8
2. Hart NH, Nimphius S, Rantalainen T, Ireland A, Siafarikas A, Newton RU. Mechanical basis of bone strength: Influence of bone material, bone structure and muscle action. J Musculoskelet Neuronal Interact. (2017) 17:114–39.
3. Boskey AL. Erratum: Bone composition: relationship to bone fragility and antiosteoporotic drug effects. Bonekey Rep. (2015) 4:710. doi: 10.1038/BONEKEY.2015.79
4. Oei L, Koromani F, Rivadeneira F, Zillikens MC, Oei EHG. Quantitative imaging methods in osteoporosis. Quant Imaging Med Surg. (2016) 6:680–98. doi: 10.21037/QIMS.2016.12.13
5. Ukon Y, Makino T, Kodama J, Tsukazaki H, Tateiwa D, Yoshikawa H, et al. Molecular-based treatment strategies for osteoporosis: A literature review. Int J Mol Sci. (2019) 20(10):2557. doi: 10.3390/IJMS20102557
6. Feng X, McDonald JM. Disorders of bone remodeling. Annu Rev Pathol. (2011) 6:121–45. doi: 10.1146/ANNUREV-PATHOL-011110-130203
7. Langdahl B, Ferrari S, Dempster DW. Bone modeling and remodeling: potential as therapeutic targets for the treatment of osteoporosis. Ther Adv Musculoskelet Dis. (2016) 8:225–35. doi: 10.1177/1759720X16670154
8. Föger-Samwald U, Dovjak P, Azizi-Semrad U, Kerschan-Schindl K, Pietschmann P. Osteoporosis: Pathophysiology and therapeutic options. EXCLI J. (2020) 19:1017–37. doi: 10.17179/EXCLI2020-2591
9. Macías I, Alcorta-Sevillano N, Rodríguez CI, Infante A. Osteoporosis and the potential of cell-based therapeutic strategies. Int J Mol Sci. (2020) 21(5):1653. doi: 10.3390/IJMS21051653
10. Tabacco G, Bilezikian JP. Osteoanabolic and dual action drugs. Br J Clin Pharmacol. (2019) 85:1084–94. doi: 10.1111/BCP.13766
11. Bandeira L, Michael Lewiecki E. Anabolic therapy for osteoporosis: update on efficacy and safety. Arch Endocrinol Metab. (2022) 66:707–16. doi: 10.20945/2359-3997000000566
12. Kerschan-Schindl K. Romosozumab: a novel bone anabolic treatment option for osteoporosis? Wien Med Wochenschr. (2020) 170:124–31. doi: 10.1007/S10354-019-00721-5
13. Shah AD, Shoback D, Michael Lewiecki E. Sclerostin inhibition: a novel therapeutic approach in the treatment of osteoporosis. Int J Womens Health. (2015) 7:565–80. doi: 10.2147/IJWH.S73244
14. Stepan JJ. Strontium ranelate: In search for the mechanism of action. J Bone Miner Metab. (2013) 31:606–12. doi: 10.1007/S00774-013-0494-1/FIGURES/2
15. Gourion-Arsiquaud S, Faibish D, Myers E, Spevak L, Compston J, Hodsman A, et al. Use of FTIR spectroscopic imaging to identify parameters associated with fragility fracture. J Bone Miner Res. (2009) 24:1565–71. doi: 10.1359/JBMR.090414
16. Boskey AL, Spevak L, Weinstein RS. Spectroscopic markers of bone quality in alendronate-treated postmenopausal women. Osteoporos Int. (2009) 20:793–800. doi: 10.1007/S00198-008-0725-9
17. Tadano S, Giri B. X-ray diffraction as a promising tool to characterize bone nanocomposites. Sci Technol Adv Mater. (2012) 12:64708. doi: 10.1088/1468-6996/12/6/064708
18. Edward Hoffler C, Edward Guo X, Zysset PK, Goldstein SA. An application of nanoindentation technique to measure bone tissue Lamellae properties. J Biomech Eng. (2005) 127:1046–53. doi: 10.1115/1.2073671
19. Sharma S, Mehta P, Patil A, Gupta SK, Rajender S, Chattopadhyay N. Meta-analyses of the quantitative computed tomography data in dialysis patients show differential impacts of renal failure on the trabecular and cortical bones. Osteoporos Int. (2022) 33:1521–33. doi: 10.1007/S00198-022-06366-2
20. Egger M, Smith GD, Schneider M, Minder C. Bias in meta-analysis detected by a simple, graphical test. BMJ. (1997) 315:629–34. doi: 10.1136/BMJ.315.7109.629
21. Operating characteristics of a rank correlation test for publication bias . Available online at: https://pubmed.ncbi.nlm.nih.gov/7786990/ (Accessed December 23, 2021).
22. Duval S, Tweedie R. Trim and fill: A simple funnel-plot-based method of testing and adjusting for publication bias in meta-analysis. Biometrics. (2000) 56:455–63. doi: 10.1111/J.0006-341X.2000.00455.X
23. Boivin G, Lips P, Ott SM, Harper KD, Sarkar S, Pinette KV, et al. Contribution of raloxifene and calcium and vitamin D3 supplementation to the increase of the degree of mineralization of bone in postmenopausal women. J Clin Endocrinol Metab. (2003) 88:4199–205. doi: 10.1210/JC.2002-022020
24. Donnelly E, Meredith DS, Nguyen JT, Gladnick BP, Rebolledo BJ, Shaffer AD, et al. Reduced cortical bone compositional heterogeneity with bisphosphonate treatment in postmenopausal women with intertrochanteric and subtrochanteric fractures. J Bone Miner Res. (2012) 27:672–8. doi: 10.1002/JBMR.560
25. Bala Y, Kohles J, Recker RR, Boivin G. Oral ibandronate in postmenopausal osteoporotic women alters micromechanical properties independently of changes in mineralization. Calcif Tissue Int. (2013) 92:6–14. doi: 10.1007/S00223-012-9658-6
26. Jähn-Rickert K, Wölfel EM, Jobke B, Riedel C, Hellmich M, Werner M, et al. Elevated bone hardness under denosumab treatment, with persisting lower osteocyte viability during discontinuation. Front Endocrinol (Lausanne). (2020) 11:250. doi: 10.3389/FENDO.2020.00250
27. Farlay D, Rizzo S, Dempster DW, Huang S, Chines A, Brown JP, et al. Bone mineral and organic properties in postmenopausal women treated with denosumab for up to 10 years. J Bone Miner Res. (2022) 37:856–64. doi: 10.1002/JBMR.4538
28. Doublier A, Farlay D, Jaurand X, Vera R, Boivin G. Effects of strontium on the quality of bone apatite crystals: A paired biopsy study in postmenopausal osteoporotic women. Osteoporos Int. (2013) 24:1079–87. doi: 10.1007/s00198-012-2181-9
29. Paschalis EP, Glass EV, Donley DW, Eriksen EF. Bone mineral and collagen quality in iliac crest biopsies of patients given teriparatide: new results from the fracture prevention trial. J Clin Endocrinol Metab. (2005) 90:4644–9. doi: 10.1210/JC.2004-2489
30. Durchschlag E, Paschalis EP, Zoehrer R, Roschger P, Fratzl P, Recker R, et al. Bone material properties in trabecular bone from human iliac crest biopsies after 3- and 5-year treatment with risedronate. J Bone Miner Res. (2006) 21:1581–90. doi: 10.1359/JBMR.060701
31. Li C, Paris O, Siegel S, Roschger P, Paschalis EP, Klaushofer K, et al. Strontium is incorporated into mineral crystals only in newly formed bone during strontium ranelate treatment. J Bone Miner Res. (2010) 25:968–75. doi: 10.1359/JBMR.091038
32. Boivin G, Farlay D, Khebbab MT, Jaurand X, Delmas PD, Meunier PJ. In osteoporotic women treated with strontium ranelate, strontium is located in bone formed during treatment with a maintained degree of mineralization. Osteoporos Int. (2010) 21:667–77. doi: 10.1007/S00198-009-1005-Z
33. Roschger P, Manjubala I, Zoeger N, Meirer F, Simon R, Li C, et al. Bone material quality in transiliac bone biopsies of postmenopausal osteoporotic women after 3 years of strontium ranelate treatment. J Bone Miner Res. (2010) 25:891–900. doi: 10.1359/JBMR.091028
34. Doublier A, Farlay D, Khebbab MT, Jaurand X, Meunier PJ, Boivin G. Distribution of strontium and mineralization in iliac bone biopsies from osteoporotic women treated long-term with strontium ranelate. Eur J Endocrinol. (2011) 165:469–76. doi: 10.1530/EJE-11-0415
35. Tjhia CK, Odvina CV, Rao DS, Stover SM, Wang X, Fyhrie DP. Mechanical property and tissue mineral density differences among severely suppressed bone turnover (SSBT) patients, osteoporotic patients, and normal subjects. Bone. (2011) 49:1279–89. doi: 10.1016/J.BONE.2011.09.042
36. Bala Y, Depalle B, Farlay D, Douillard T, Meille S, Follet H, et al. Bone micromechanical properties are compromised during long-term alendronate therapy independently of mineralization. J Bone Miner Res. (2012) 27:825–34. doi: 10.1002/JBMR.1501
37. Boivin G, Meunier PJ. The degree of mineralization of bone tissue measured by computerized quantitative contact microradiography. Calcif Tissue Int. (2002) 70:503–11. doi: 10.1007/S00223-001-2048-0
38. Follet H, Boivin G, Rumelhart C, Meunier PJ. The degree of mineralization is a determinant of bone strength: A study on human calcanei. Bone. (2004) 34:783–9. doi: 10.1016/j.bone.2003.12.012
39. Hu YY, Rawal A, Schmidt-Rohr K. Strongly bound citrate stabilizes the apatite nanocrystals in bone. Proc Natl Acad Sci U.S.A. (2010) 107:22425–9. doi: 10.1073/PNAS.1009219107/-/DCSUPPLEMENTAL/PNAS.1009219107_SI.PDF
40. Ahn T, Jueckstock M, Mandair GS, Henderson J, Sinder BP, Kozloff KM, et al. Matrix/mineral ratio and domain size variation with bone tissue age: A photothermal infrared study. J Struct Biol. (2022) 214(3):107878. doi: 10.1016/J.JSB.2022.107878
41. Ou-Yang H, Paschalis EP, Mayo WE, Boskey AL, Mendelsohn R. Infrared microscopic imaging of bone: spatial distribution of CO3(2-). J Bone Miner Res. (2001) 16:893–900. doi: 10.1359/JBMR.2001.16.5.893
42. Garnero P. The contribution of collagen crosslinks to bone strength. Bonekey Rep. (2012) 1:182. doi: 10.1038/BONEKEY.2012.182
43. Li S, Wang Jz, Yin B, Hu Zs, Zhang Xj, Wu W, et al. Atlas of human skeleton hardness obtained using the micro-indentation technique. Orthop Surg. (2021) 13:1417–22. doi: 10.1111/OS.12841
44. Boivin G, Bala Y, Doublier A, Farlay D, Ste-Marie LG, Meunier PJ, et al. The role of mineralization and organic matrix in the microhardness of bone tissue from controls and osteoporotic patients. Bone. (2008) 43:532–8. doi: 10.1016/J.BONE.2008.05.024
45. Zebaze RM, Libanati C, Austin M, Ghasem-Zadeh A, Hanley DA, Zanchetta JR, et al. Differing effects of denosumab and alendronate on cortical and trabecular bone. Bone. (2014) 59:173–9. doi: 10.1016/J.BONE.2013.11.016
46. Zebaze R, Libanati C, McClung MR, Zanchetta JR, Kendler DL, Høiseth A, et al. Denosumab reduces cortical porosity of the proximal femoral shaft in postmenopausal women with osteoporosis. J Bone Miner Res. (2016) 31:1827–34. doi: 10.1002/JBMR.2855
47. Wehrle-Martinez A, Waterland MR, Naffa R, Lawrence K, Back PJ, Rogers CW, et al. Bone quality changes as measured by Raman and FTIR spectroscopy in primiparous cows with humeral fracture from New Zealand. Front Vet Sci. (2023) 10:1063427. doi: 10.3389/FVETS.2023.1063427
48. Akkus O, Adar F, Schaffler MB. Age-related changes in physicochemical properties of mineral crystals are related to impaired mechanical function of cortical bone. Bone. (2004) 34:443–53. doi: 10.1016/j.bone.2003.11.003
49. Hofstetter B, Gamsjaeger S, Varga F, Dobnig H, Stepan JJ, Petto H, et al. Bone quality of the newest bone formed after two years of teriparatide therapy in patients who were previously treatment-naïve or on long-term alendronate therapy. Osteoporos Int. (2014) 25:2709–19. doi: 10.1007/S00198-014-2814-2
50. Bala Y, Seeman E. Bone’s material constituents and their contribution to bone strength in health, disease, and treatment. Calcif Tissue Int. (2015) 97:308–26. doi: 10.1007/S00223-015-9971-Y
51. Paschalis EP, Tatakis DN, Robins S, Fratzl P, Manjubala I, Zoehrer R, et al. Lathyrism-induced alterations in collagen cross-links influence the mechanical properties of bone material without affecting the mineral. Bone. (2011) 49:1232–41. doi: 10.1016/J.BONE.2011.08.027
52. Oxlund H, Barckman M, Ørtoft G, Andreassen TT. Reduced concentrations of collagen cross-links are associated with reduced strength of bone. Bone. (1995) 17(4 Suppl):365S–371S. doi: 10.1016/8756-3282(95)00328-B
53. Banse X, Sims TJ, Bailey AJ. Mechanical properties of adult vertebral cancellous bone: correlation with collagen intermolecular cross-links. J Bone Miner Res. (2002) 17:1621–8. doi: 10.1359/JBMR.2002.17.9.1621
54. Follet H, Viguet-Carrin S, Burt-Pichat B, Dépalle B, Bala Y, Gineyts E, et al. Effects of preexisting microdamage, collagen cross-links, degree of mineralization, age, and architecture on compressive mechanical properties of elderly human vertebral trabecular bone. J Orthop Res. (2011) 29:481–8. doi: 10.1002/JOR.21275
55. Fields AJ, Eswaran SK, Jekir MG, Keaveny TM. Role of trabecular microarchitecture in whole-vertebral body biomechanical behavior. J Bone Miner Res. (2009) 24:1523–30. doi: 10.1359/JBMR.090317
56. Garnero P, Borel O, Gineyts E, Duboeuf F, Solberg H, Bouxsein ML, et al. Extracellular post-translational modifications of collagen are major determinants of biomechanical properties of fetal bovine cortical bone. Bone. (2006) 38:300–9. doi: 10.1016/J.BONE.2005.09.014
57. Bala Y, Farlay D, Delmas PD, Meunier PJ, Boivin G. Time sequence of secondary mineralization and microhardness in cortical and cancellous bone from ewes. Bone. (2010) 46:1204–12. doi: 10.1016/J.BONE.2009.11.032
58. Paschalis EP, Krege JH, Gamsjaeger S, Eriksen EF, Burr DB, Disch DP, et al. Teriparatide treatment increases mineral content and volume in cortical and trabecular bone of iliac crest: A comparison of infrared imaging with X-ray-based bone assessment techniques. J Bone Miner Res. (2018) 33:2230–5. doi: 10.1002/JBMR.3565
59. Misof BM, Roschger P, Zhou H, Nieves JW, Bostrom M, Cosman F, et al. No evidence for alteration in early secondary mineralization by either alendronate, teriparatide or combination of both in transiliac bone biopsy samples from postmenopausal osteoporotic patients. Bone Rep. (2020) 12:100253. doi: 10.1016/J.BONR.2020.100253
60. Nishiyama KK, Cohen A, Young P, Wang J, Lappe JM, Guo XE, et al. Teriparatide increases strength of the peripheral skeleton in premenopausal women with idiopathic osteoporosis: a pilot HR-pQCT study. J Clin Endocrinol Metab. (2014) 99:2418–25. doi: 10.1210/JC.2014-1041
61. Stepan JJ, Burr DB, Pavo I, Sipos A, Michalska D, Li J, et al. Low bone mineral density is associated with bone microdamage accumulation in postmenopausal women with osteoporosis. Bone. (2007) 41:378–85. doi: 10.1016/J.BONE.2007.04.198
Keywords: bone-quality, bone-strength, osteoporosis, bisphosphonate, denosumab, raloxifene, teriparatide, strontium-ranelate
Citation: Sharma S, Shankar V, Rajender S, Mithal A, Rao SD and Chattopadhyay N (2024) Impact of anti-fracture medications on bone material and strength properties: a systematic review and meta-analysis. Front. Endocrinol. 15:1426490. doi: 10.3389/fendo.2024.1426490
Received: 01 May 2024; Accepted: 31 July 2024;
Published: 27 August 2024.
Edited by:
J. Patrick O’Connor, The State University of New Jersey, United StatesReviewed by:
Shuting Sun, BioVinc LLC, United StatesCopyright © 2024 Sharma, Shankar, Rajender, Mithal, Rao and Chattopadhyay. This is an open-access article distributed under the terms of the Creative Commons Attribution License (CC BY). The use, distribution or reproduction in other forums is permitted, provided the original author(s) and the copyright owner(s) are credited and that the original publication in this journal is cited, in accordance with accepted academic practice. No use, distribution or reproduction is permitted which does not comply with these terms.
*Correspondence: Sudhaker D. Rao, c3JhbzFAaGZocy5vcmc=
Disclaimer: All claims expressed in this article are solely those of the authors and do not necessarily represent those of their affiliated organizations, or those of the publisher, the editors and the reviewers. Any product that may be evaluated in this article or claim that may be made by its manufacturer is not guaranteed or endorsed by the publisher.
Research integrity at Frontiers
Learn more about the work of our research integrity team to safeguard the quality of each article we publish.