- 1Department of Clinical and Experimental Medicine, University of Catania, Catania, Italy
- 2Department of Obstetrics and Gynecology, Azienda Ospedaliera Nazionale SS. Antonio e Biagio e Cesare Arrigo, Alessandria, Italy
- 3Department of Clinical, Surgical, Diagnostic and Pediatric Sciences, University of Pavia, Pavia, Italy
- 4Research Center for Reproductive Medicine, Gynecological Endocrinology and Menopause, IRCCS San Matteo Foundation, Pavia, Italy
- 5Glickman Urological and Kidney Institute, Cleveland Clinic, Cleveland, OH, United States
Functional hypothalamic amenorrhea (FHA) is a common cause of amenorrhea and chronic anovulation in adolescent girls and young women, diagnosed after excluding other organic causes. It is commonly associated with calorie restriction, excessive physical exercise, and psychosocial stress. These stressors alter the pulsatile secretion of gonadotropin-releasing hormone, leading to a chronic condition of hypoestrogenism and significant health consequences. Recent evidence has highlighted a genetic predisposition to FHA that could explain interindividual variability in stress response. Indeed, not all women experience FHA in response to stress. Rare variants in genes associated with idiopathic hypogonadotropic hypogonadism have been identified in women with FHA, suggesting that these mutations may contribute to an increased susceptibility of women to the trigger of stress exposure. FHA appears today as a complex disease resulting from the combination of genetic predisposition, environmental factors, and epigenetic changes. Furthermore, the genetic background of FHA allows for the hypothesis of a male counterpart. Despite the paucity of data, preliminary findings indicate that an equivalent condition of FHA exists in men, warranting further investigation. This narrative review aims to summarize the recent genetic evidence contributing to the pathophysiology of FHA and to raise awareness on a possible male counterpart.
1 Introduction
Functional hypothalamic amenorrhea (FHA) is a common cause of amenorrhea and chronic anovulation in adolescent girls and young women (1). FHA is responsible for approximately 20-35% of secondary amenorrhea in women of reproductive age and approximately 3% of primary amenorrhea (2), which is estimated to affect 17.4 million women worldwide (3).
FHA is defined as the absence of menses for at least 3 to 6 months, low or normal gonadotropin levels, and hypoestrogenism without organic causes. The term FHA is used to describe the lack of menstruation resulting from exposure to various types of stress, after excluding other etiologies of amenorrhea. It is commonly associated with three main stressful conditions: caloric restriction and weight loss, strenous physical exercise, and psychosocial stress, which may occur alone or, more often, in combination (1).
These stressor triggers reduce the pulsatile secretion of hypothalamic gonadotropin-releasing hormone (GnRH) (4), which impair the pulsatile release of luteinizing hormone (LH) resulting in decreased production of 17ß-estradiol in the ovary. Pulsatile GnRH secretion is influenced by a multitude of factors, hormonal and non-hormonal, which contribute to the complex pathophysiology of FHA, that is not yet fully understood (5).
Hypoestrogenism resulting from suppression of the hypothalamic-pituitary-ovarian (HPO) axis can impact the function of multiple systems including reproduction, bone, emotional, cognitive, and cardiovascular health (3, 6). Therefore, timely diagnosis and management of this condition are essential to prevent long-term consequences.
Recent evidence suggests a genetic predisposition to FHA (7–9). Rare variants of genes linked to the development and function of GnRH neurons have been identified in women with FHA (7, 8) and could explain interindividual variability in response to stressful events. Indeed, not all women experience FHA during stress exposure. Epigenetic changes could also compromise several pathways involved in the HPO axis, contributing to the onset of FHA (10). Furthermore, although little studied, a corresponding condition has also been described in men (11).
On this basis, this narrative review aims to summarize the recent genetic evidence contributing to the pathophysiology of FHA, while also raising awareness about the existence of a possible male counterpart.
2 Stressor triggers and their impact on the hypothalamic-pituitary-gonadal axis
In 1974, Frisch & McArthur proposed that a minimum level of body fat was necessary for ovulation and menstrual cycles in women (12). Early studies suggested that body fat percentage had to reach the “critical threshold” of 17% for menarche to occur and that secondary amenorrhea could be triggered if body fat percentage fell below 22% (12). However, subsequent studies have contradicted this hypothesis by showing that female athletes with low body fat have regular menses (13). Indeed, it is difficult to establish a body weight or fat cuf-off for having a regular menstrual cycle due to individual differences, and the change in physiological state appears to be more important than the absolute value (14). A condition of low energy availability can result from insufficient energy intake, as seen in eating disorders, or excessive energy expenditure, as seen with strenous physical exercise.
In 1992, the American College of Sports Medicine first coined the term “female athlete triad”, referring to a constellation of 3 clinical entities: menstrual dysfunction, low energy availability (with or without an eating disorder), and decreased bone mineral density (15). The prevalence of FHA in sportswomen is variable depending on the type of activity, duration, and intensity, with the highest prevalences reported in esthetic (such as cheerleading, diving, and gymnastics), endurance, and weight-class sports (16). When energy expenditure exceeds dietary energy intake due to intense training, the resulting macro- and micronutrients imbalance can alter the HPO axis function, contributing to the onset of FHA (17). This alteration in body composition translates into reduced adipokines production and an alteration in the levels of hormones that regulate appetite. Among these, leptin has a central role in the regulation of the HPO axis.
Leptin is a 16-kDa adipokine produced by adipose tissue. In addition to its well-known role in the regulation of food intake where it acts as a potent satiety factor, leptin may also have other functions. These include the regulation of reproduction, acting as both a central and peripheral factor. Low leptin levels are associated with GnRH suppression and leptin treatment has been shown to increase LH pulsatility in women with FHA (18). The effects of leptin on hypothalamic GnRH release are not direct since GnRH-secreting neurons do not express receptors for this adipokine. The central role of leptin in reproduction appears to be mediated by other peptides, including kisspeptin. Indeed, kisspeptin/neurokinin B/dynorphin neurons of the hypothalamic arcuate nucleus secrete kisspeptin, which acts on GnRH-secreting neurons by stimulating the release of GnRH (19). Several other factors, including neuropeptide Y, oxytocin, ghrelin, thyroid hormones, and insulin, interact with each other and play an important role in the pathophysiology of energy imbalance-related amenorrhea (14). Low levels of insulin (20) and triiodothyronine (21) characterize a state of negative energy balance and are often described in patients with FHA.
Psychological disorders are common in FHA patients and may themselves be a cause of FHA or synergize with metabolic stress (22). Psychosocial stressors are variable and depend on the individual’s perception (23). Patients with FHA have been demonstrated to cope less well with stress in their autonomic responses, with significantly higher increases in heart rate, systolic and diastolic blood pressure, and serum cortisol levels, in response to exposure to neuropsychological stress, compared to controls (24). Recent data indicate that FHA may contribute to preclinical cardiovascular dysfunction, not explained by hypoestrogenemia alone (25).
Stress, regardless of its type, activates the hypothalamic-pituitary-adrenal (HPA) axis and the autonomic nervous system, causing a constellation of neuroendocrine alterations (26). Both chronic and severe acute stressors can impact the function of the HPG axis. Stress and the resulting activation of the HPA axis impair HPG axis function, as reproduction is not a priority in the “fight or flight” response during stressful situations (27). Activation of the HPA axis is due to increased secretion of corticotropin-releasing hormone (CRH), adrenocorticotrophin (ACTH), cortisol, and endogenous opioids. Glucocorticoids (GLCs) interfere with reproductive function at both central and peripheral levels. Studies have shown that corticosterone, the main GLC in rodents, and dexamethasone can suppress GnRH release in vitro, confirming the central effect of GLCs (28). Furthermore, endogenous opioids also play a relevant role in inhibiting the pulsatile release of both GnRH and gonadotropins during stressful events (1) along with other neurotransmitters, including serotonin (29).
3 Inter-individual variability in the response to stress
Stressors are condition that threatens to alter or alters the homeostasis of an organism. As a result, a complex array of responses is activated to counterpoise the imbalance caused by stressful forces. Stress response mechanisms involve the endocrine, nervous, and immune systems, collectively referred as stress response effectors (30). FHA can be considered a natural protective mechanism in women against stressful events, which lead to the temporary suppression of gonadal function when physical conditions are not suitable to support a pregnancy (10). The onset times of menstrual irregularities following exposure to a stressful factor are variable; generally, the alterations begin with oligomenorrhea and then evolve into amenorrhea (1). Although the stress triggers leading to FHA are well documented, there is limited understanding of individual differences in how people respond to stress and the resulting impact on the HPO axis (10). Notably, not all women develop FHA under stressful circumstances (10). At the same time, the timing of the return of menses also varies greatly and to date we cannot predict whether the menstrual cycle resumes after the restoration of normal weight and energy balance in individual women (31). Recovery times appear to be influenced by the initial cause of FHA, with female athletes having a quicker recovery period than women with eating disorders (32, 33). As we will discuss in the following paragraphs, a genetic vulnerability, arising from rare or polymorphic variants of genes that control the development, homing, and function of GnRH neurons, could explain the variable impact of stress on FHA in individual women (7–10). Epigenetic modifications could also play a role and could potentially influence individual susceptibility to amenorrhea following stressful events or recovery times after experiencing FHA.
4 Genetics of functional hypothalamic amenorrhea
4.1 Idiopathic hypogonadotropic hypogonadism: genotype vs. phenotype
Idiopathic hypogonadotropic hypogonadism (IHH) is caused by defects in hypothalamic GnRH secretion or by defects in the action of GnRH on the pituitary gland, failing puberty onset or hypogonadism that occurs after the onset of puberty (34). IHH is a rare, genetically heterogenous disorder, with a prevalence of 1:125,000 in females and 1:30,000 in males (35). This form of hypogonadism is classified into IHH associated with hyposmia/anosmia, also known as Kallmann syndrome (KS) and normosmic IHH (nIHH). The reduction of smell in KS can be explained by the common embryogenic origin of GnRH-secreting neurons and olfactory neurons. Indeed, during embryogenesis, GnRH neurons migrate from the olfactory epithelium to their final position in the hypothalamus. With puberty, these neurons begin to secrete GnRH in a pulsatile pattern and this is the key stimulator of LH and FSH secretion. IHH is caused by numerous and rare sequence variants in several genes that encode proteins essential for the development, differentiation, and homing of GnRH-secreting neurons and for the secretion and action of GnRH (36).
To date, approximately 40% of patients with IHH have an identifiable genetic mutation (37). The first gene believed to be responsible for KS is the KAL1 gene, mapping on the distal portion of the X chromosome (Xp22.3). KAL1 encodes for the protein anosmin 1, which drives neural cell adhesion and axonal migration. Its mutation causes a migratory defect and therefore a failed or incorrect homing of GnRH-secreting neurons (38). Since then, understanding of the genetic basis of IHH has expanded significantly. This progress has been aided by notable technological advances, particularly the introduction of next-generation sequencing (NGS) methods. Numerous other genes have been identified, some primarily cause KS, others cause nIHH, while others cause either one or the other form (39). In addition to KAL1, other genes can disrupt the development and migration of GnRH neurons. These include fibroblast growth factor receptor 1 (FGFR1), fibroblast growth factor 8, prokineticin 2 (PROK2), and PROK receptor 2 (PROKR2) genes. Mutations on GnRH receptor (GnRHR) gene have also been identified. Other genes that interfere with the neuroendocrine physiology of normal GnRH secretion include kisspeptin 1 (KISS1), Kiss1 receptor, leptin (LEP), LEP receptor (LEPR), tachykinin 3 (TAC3), and TAC3 receptor 3, which encode for neurokinin B and its receptor, respectively (39). Many of these genes have been shown to interact oligogenically, and most of them act in both neurodevelopmental and neuroendocrine pathways. A high genetic variability can be explained by an incomplete penetrance in variable expressivity that characterizes this disease (39).
Previous studies have also investigated the phenotype-genotype correlation of GnRHR gene mutations in male and female patients with different forms of GnRH deficiency (40–42). GnRHR mutations were first described by de Roux and colleagues in 1997 (43). Since then, more than 20 mutations in the coding sequence of the GnRHR gene have been reported in patients with sporadic or familial forms of IHH.
In 2012, Gianetti and coworkers screened a cohort of patients with different forms of GnRH deficiency, including adult-onset IHH (280 males and 95 females), KS (272 males and 88 females), FHA (77 females), and constitutional delay of puberty (29 males and 22 females) for GnRHR gene mutations (40). Among the 863 probands, the 70 mutation-carrying patients were divided into 4 groups (G1-G4) based on the severity of the mutations (complete or partial loss of function) and the number of allelic mutations (monoallelic or biallelic).
Then, the authors correlated the identified genotypic classes (G1-G4) to patient clinical phenotypes (40). Interestingly, in groups G1-G3, the patient phenotypes were consistent with severe GnRH deficiency. In contrast, G4 patients, with only monoallelic mutations, demonstrated a greater diversity of clinical phenotypes, ranging from severe GnRH deficiency such as KS and IHH to mild GnRH deficiency such as FHA, adult-onset IHH, or constitutional delay of puberty, to normal GnRH function. Therefore, these data (40) indicate that in patients with biallelic mutations of the GnRHR gene it is possible to identify a correlation between genotype and phenotype severity. In contrast, for patients with monoallelic GnRHR mutations, this correlation was not observed. Therefore, it can be hypothesized that other genetic or non-genetic factors (such as environmental or psychosocial stress) in combination with the mutated GnRHR allele contribute to decreased HPO axis function. This hypothesis cannot be excluded for biallelic mutations even if there seems to be a clear correlation between genotype and phenotype, as stated. Similarly, Beneduzzi and colleagues reported a relatively good genotype-phenotype correlation in male patients with IHH and GnRHR mutations (41).
4.2 Genetic overlap between functional hypothalamic amenorrhea and idiopathic hypogonadotropic hypogonadism
For the first time, in 2011, Caronia and colleagues showed that rare variants of IHH-associated genes can be found in patients with FHA, suggesting that these mutations may contribute to an increase in women’s susceptibility to stressors (7). In their study, Caronia and colleagues included 55 patients with FHA and 422 controls (7). They found six heterozygous variants in the FGFR1, PROKR2, KAL1, and GnRHR genes in 7 patients with FHA (7/55; 12.7%). The affected genes play fundamental roles in the ontogeny and function of the GnRH-secreting neuron. In particular, the FGFR1 gene controls the migration and survival of GnRH neurons (44), PROKR2 and KAL1 genes are critical for their migration (45, 46), and GnRHR encodes the unique receptor that is activated by GnRH at the pituitary level (47). As reported in the previous paragraph, mutations in these genes have been associated with IHH in humans (36). All identified variants were considered pathogenic as they all were found in conserved amino acid residues and induced significant loss of function. None of these mutations were observed in the control group. All seven patients with gene mutations had secondary amenorrhea for at least 6 months and at least one factor for FHA (7). Four of these patients also reported a family history of FHA or delayed puberty, but the genetic analysis was not extended to other affected relatives to confirm the association. Two of the seven patients continued to receive long-term hormone replacement therapy (HRT), whereas the other five discontinued HRT and had their menstrual cycle resumed (7).
However, the study by Caronia and colleagues examined only a limited number of genes associated with IHH and the genetic investigation of controls was limited to the search of variants identified in patients with FHA (7). To overcome this problem, in 2020, the same group expanded the analysis to include 53 genes associated with IHH and sequenced all coding regions in 106 women with FHA and 468 controls (8).
According to the hypothesis of Caronia and colleagues (7) and with the results of their first study, Delaney and coworkers found a high frequency of heterozygous rare sequence variants associated with IHH in patients with FHA. In detail, they identified 78 heterozygous variants in 58 out of 106 patients with FHA. Two hundred control women also harbored 255 rare sequence variants. However, the frequency of these mutations was significantly higher in FHA women compared to controls. Additionally, patients with FHA were more likely to have a greater number (3 or more) of rare sequence variants compared to controls. It is also important to highlight that a greater number of mutations previously identified in patients with severe IHH phenotypes were present in FHA patients compared to controls. Furthermore, all mutations identified in women with FHA were present in heterozygosity (8). This is in line with other evidence suggesting a genotype-phenotype relationship (40–42). Therefore, patients with biallelic or di/oligogenic mutations show severe forms of GnRH deficiency resulting in IHH, while it can be hypothesized that these heterozygous mutations are not sufficient to cause IHH, but they could lower the threshold for functional inhibition of the HPO axis caused by exposure to stressful events leading to FHA. This is supported by the identification of some rare variants even in controls with regular menstrual cycles. The results of the studies conducted by Caronia and colleagues (7) and Delaney and colleagues (8) allow us to hypothesize that FHA is part of a spectrum of diseases with a genetic base (10). Indeed, as mentioned previously, Gianetti and coworkers studied the correlation between the severity of the phenotype of the HPO axis dysfunction and the severity of rare sequence variants of the GnRHR gene. They also included 77 patients with FHA and, among these, 3 patients presented monoallelic alterations of GnRHR (40). Table 1 summarizes the rare variants of genes related to GnRH-secreting neuron function identified in patients with FHA.
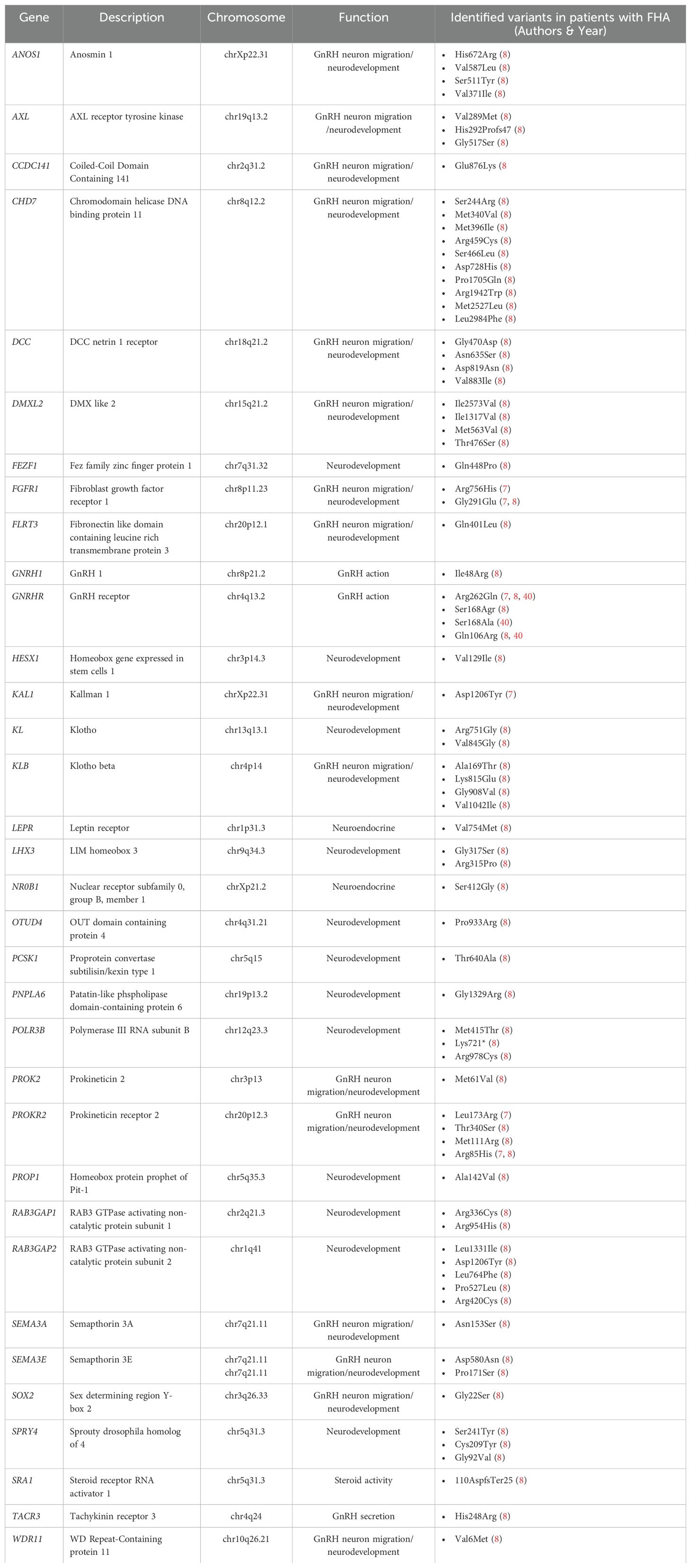
Table 1. Rare variants of genes related to GnRH-secreting neuron function identified in patients with functional hypothalamic amenorrhea (FHA).
5 Epigenetic modifications
Epigenetics is a field of study that explains how environmental factors can modify gene expression without changing the DNA sequence. It involves processes that regulate gene expression without altering the structure of chromatin (48). The three main epigenetic mechanisms that regulate gene activity regulation are DNA methylation, histone modifications, and non-coding RNAs. Recent research has highlighted the significance of epigenetic modifications in the pathogenesis of various human diseases. For instance, epigenetic changes could potentially represent the mechanism by which stressful events can triggers FHA in women with a susceptibility genotype (10).
Over the last decade, the significance of epigenetic factors in the expression of genes involved in GnRH-neuron function has increased. In vitro studies have shown that the GnRH gene responds to external stimuli by modifying chromatin in mature GnRH neurons as well (49). Studies on animal models have demonstrated that nutritional changes can affect the expression of KISS1 (the gene encoding kisspeptin) during puberty through epigenetic mechanisms and is regulated by sirtuin 1 (SIRT1) (50). When undenutrition occurs, the activity of SIRT1 increases, leading to prolonged KISS1 suppression and delayed onset of puberty in negative energy balance conditions (50).
Altered patterns of DNA methylation have been found in patients with anorexia nervosa (AN) in relation to several genes, including synuclein alpha, dopamine transporter, dopamine receptor D2, oxytocin receptor, and LEP (51–53). Women with AN have been found to exhibit lower LEP and LEPR methylation compared to controls. Interestingly, women with lower DNA methylation of the LEP gene have shown full recovery after psychotherapeutic treatment, and a significant hypermethylation of the LEP gene during treatment (52). These results suggest that LEP methylation could serve as a predictor to identify patients with a higher chance of recovery after treatment (52).
Ghrelin, along with leptin, plays an important role in regulating appetite and energy expenditure. By activating its receptor (growth hormone secretagogue receptor, GHS-R1a), ghrelin induces an orexigenic state, which facilitates food intake (54). GHS-R1a promoter methylation was found to be increased in patients with AN compared to healthy controls (53). Hypermethylation of the GHS-R1a promoter might be a consequence of acute undernutrition, inhibiting the expression of the GHS-R1a gene. This is consistent with several studies that have reported a dysregulation of ghrelin signaling pathway in patients with AN, which have increased ghrelin levels, suggesting a condition of ghrelin resistance (55).
Recent studies have also revealed that microRNAs (miRNAs) may play a significant role in regulating GnRH secretion and may be implicated in the pathophysiology of FHA (10, 56). miRNAs are small non-coding RNA molecules, which play a critical role in regulating gene expression by binding to messenger RNAs (mRNAs) and inhibiting their translation or promoting their degradation. Some miRNAs (miR- 132, miR-212, miR-361-3p) have been reported to be induced by GnRH (57, 58) and play a role in gonadotropin pathways. In addition, specific miRNAs, such as miR-34c, have been linked to the regulation of stress-related pathways (59), which are critical in FHA. Although microRNAs are not yet in clinical use, these findings open new research horizons to identify the involvement of miRNAs in FHA and their possible use as peripheral biomarkers to monitor effects of treatment in FHA (10).
6 Is functional hypogonadotropic hypogonadism the male equivalent of functional hypothalamic amenorrhea?
There have been only a few studies that have examined the male phenotypic counterpart of FHA. Previous research has found that men who engage in strenuous physical exercise (60–68) or undergo periods of caloric restriction (69–71) may experience dysregulation of their hypothalamic-pituitary-testicular (HPT) axis.
In 1986, MacConnie and colleagues conducted one of the first studies investigating the integrity of hypothalamic-pituitary-gonadal (HPG) axis in athletes. The authors found that six highly trained marathon runners (who were running 125 to 200 km per week) had decreased LH pulse frequency and amplitude (every-20-minute sampling for 8 hours) compared to healthy controls (62). Several studies have shown that soldiers who are exposed to prolonged caloric restriction, high energy expenditure, and disrupted sleep may experience a reduction in their testosterone levels (72, 73). In 2019, Dwyer and coworkers conducted a study on 18 young men who presented for evaluation of hypogonadism secondary to excessive exercise or weight loss and investigated their biochemical and genetic profiles (74). The results showed patients had significantly lower body mass index (BMI), testosterone, and LH compared to controls. Men with functional HH had a lower LH pulse amplitude then controls. So far, no studies have investigated the impact of excessive physical exercise or caloric restriction on spermatogenesis. Only 3 of the 10 patients who participated in the study underwent a semen analysis that showed a normal sperm count. However, serum inhibin B levels were significantly lower in patients compared to controls (74). Inhibin B is a glycoprotein hormone produced by Sertoli cells that inhibits FSH secretion and stimulates testosterone secretion by Leydig cells (75). It is, therefore, an essential marker of spermatogenesis.
Previous studies have not provided clear information on whether the decrease in testosterone levels in men due to excessive strenous exercise or caloric restriction was associated with clinical symptoms of hypogonadism. However, a study conducted by Dwyer and colleagues found that 9 out of the 10 patients examined presented low libido, which was associated with erectile dysfunctions in two patients and with reduction of potency/training performance in six patients (74). It is important to note that two of the 10 patients included had a family history of FHA and two patients had two rare genetic variants in genes related to GnRH deficiency, Chromodomain Helicase DNA-binding Protein 7 (CHD7) and NMDA Receptor Synaptonuclear Signaling and Neuronal Migration Factor (NSMF) (74). As reported in paragraph 4.1, in both men and women, mutations in genes related to GnRH can lead to a wide spectrum of reproductive phenotypes. Men with biallelic partial loss-of-function mutations in GnRHR may experience delayed puberty, congenital HH (CHH), and reversal and relapse of CHH (40, 76, 77). Hietamaki and colleagues described the phenotypic and genetic characteristics of two monozygotic twin brothers with stalled puberty. They had heterozygous mutations in the GnRHR gene and, interestingly, the one with higher BMI had lower testosterone levels. This finding suggests that excess adipose tissue may suppress the HPT axis in patients with a partial form of gonadotropin deficiency (42). Metabolic hypogonadism is a well-characterized condition in men (78, 79), although a similar condition has recently been proposed in women, as “female obesity-related secondary hypogonadism” (80). In men, obesity can significantly compromise the gonadal function through different mechanisms, including disruption of the hormonal milieu, induction of systemic low-grade inflammation, and increased oxidative stress with consequent alterations of conventional and biofunctional sperm parameters (78). Obesity also causes epigenetic changes that can be transferred to offspring (78). In men, changes in the normal methylation pattern of the genes H19/insulin-like growth factor 2 and other imprinted genes have been associated with infertility and the risk of transmitting epigenetic abnormalities to offspring (81).
Reproduction is a complex process influenced by environmental, social, neuronal, endocrine, and metabolic factors. Unlike women, men appear to be more resistant to stress-induced alterations in gonadal function. In women, FHA is considered an adaptive response of the female body to stress. This adaptation is thought to be the body’s ability to priorize energy allocation to vital physiological functions over reproduction and pregnancy when energy intake is insufficient (82). It is less clear whether functional HH may also represent a similar adaptive response to conserve energy in men when energy is limited. However, because reproductive function is not essential for survival and requires a large amount of energy, it is biologically plausible that stressors may interfere in both sexes. Certainly, in women, FHA provides a clear model for understanding how stress reduces the function of the HPG axis. Unlike women who experience the evident symptom of amenorrhea, men show less obvious clinical manifestations, although similar mechanisms cannot be ruled out. For this reason, further studies are needed to examine the hormonal and genetic profile of men undergoing strenuous physical exercise, weight loss, or prolonged psychological stress, to identify a potential role in male sexual and reproductive health.
7 Final remarks
Recent evidence has highlighted a genetic predisposition to FHA that could explain inter-individual variability in response to stress. Indeed, rare variants in genes associated with IHH have been identified in women with FHA, suggesting that these mutations may contribute to increasing women’s susceptibility to stressor triggers. FHA appears today as a complex disease resulting from the combination of genetic predisposition, environmental factors, and epigenetic changes (Figure 1). The identification of rare genetic variants associated with FHA holds promise for advancing our understanding of the condition and developing personalized approaches to this disease, ultimately improving both short-term management and long-term health consequences for affected women.
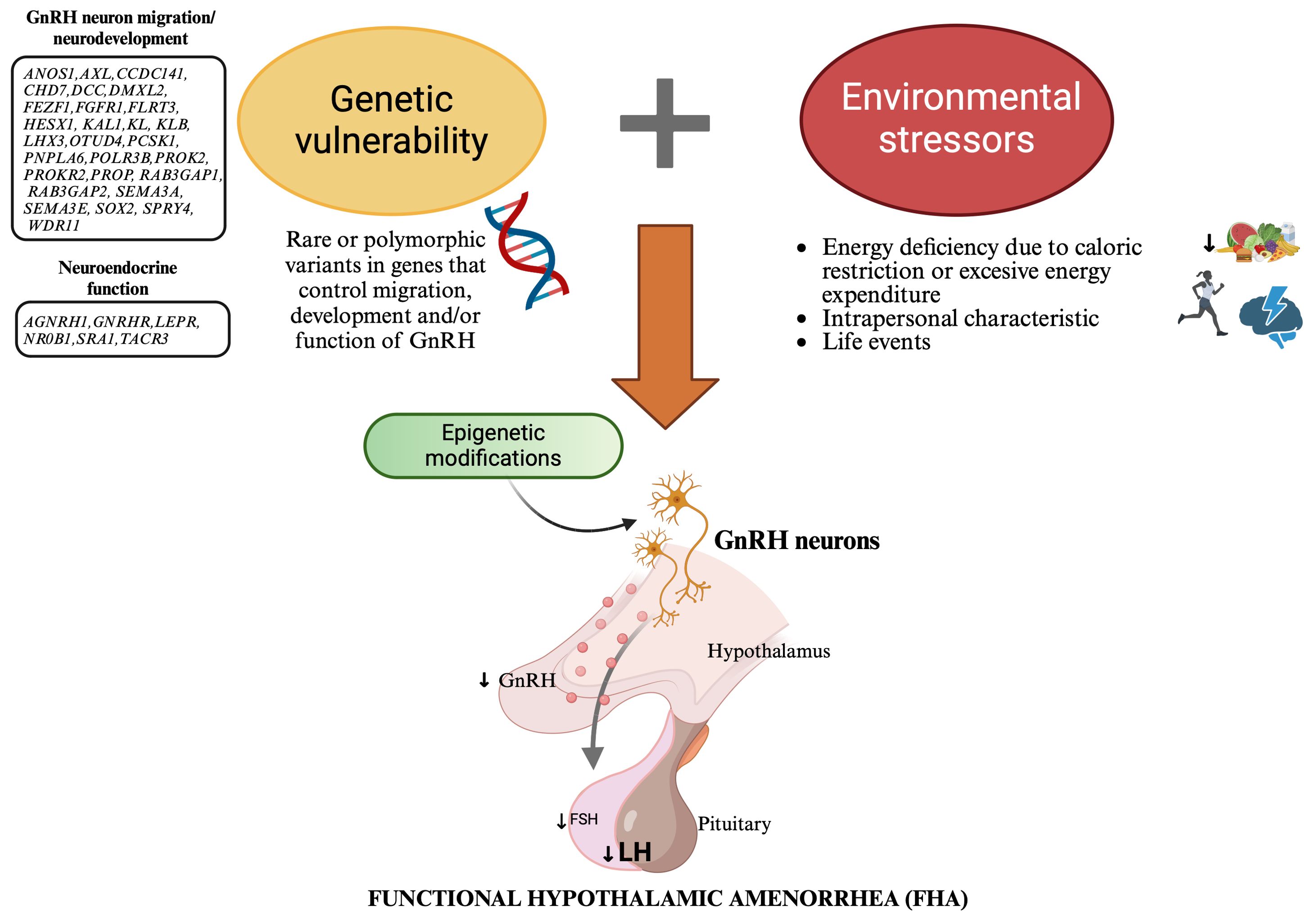
Figure 1. New model explaining the etiopathogenesis of functional hypothalamic amenorrhea: a combination of genetic predisposition, environmental factors, and epigenetic changes. This figure illustrates a new model for the etiopathogenesis of functional hypothalamic amenorrhea (FHA), highlighting the interplay between genetic predisposition, environmental factors, and epigenetic changes. Genetic vulnerability: Rare genetic variants in genes associated with idiopathic hypogonadotropic hypogonadism (IHH), which control the migration, development and/or function of GnRH neurons, predispose some women to FHA by impairing GnRH neuron function. Environmental factors: Energy deficiency due to caloric restriction or excessive energy expenditure, intrapersonal characteristics, and life events act as stressors that disrupt normal GnRH secretion. Stressors have a particularly significant impact in genetically predisposed people, leading to decreased LH levels and subsequent menstrual irregularities. Epigenetic changes: Environmental stressors induce epigenetic modifications, such as DNA methylation, histone modification, and miRNA regulation. These changes alter gene expression without changing the DNA sequence, resulting in long-lasting hypothalamic dysfunction. This model highlihghts the importance of personalized approaches in the diagnosis, treatment, and prevention of FHA. GnRH, Gonadotropin Releasing Hormone; FSH, follicle stimulating hormone (FSH); LH, luteinizing hormone.
Further studies are needed to examine better rare variants in genes associated with IHH in larger cohorts of women with FHA possibly in multicenter studies. When designing new research, it may be interesting to match healthy controls to cases based on exposure to risk factors. Genetic investigation could be very useful for patients experiencing amenorrhea, where stress, as a trigger, may not be strong enough to justify FHA, or even in cases where the menstrual cycle fails to resume despite stress factors being resolved. In such situations, there may be an underlying genetic predisposition that needs to be identified.
This emerging genetic predisposition to FHA may be even more complex. Indeed, previous studies have shown genetic overlap not only with genes linked to IHH but also with other psychological disorders, such as anxiety and mood disorders (10). Therefore, exploring rare genetic variants of genes involved in appetite control, energy homeostasis, and stress response pathways could be highly interesting.
Finally, the genetic background of FHA suggests the existence of a male equivalent. To date, little is known about the existence of a “functional hypogonadotropic hypogonadism” and its possible short- and long-term consequences in men. Indeed, there have been few studies on the clinical presentation and hormonal imbalance in men who have undergone caloric restriction, strenuous physical activity, and psychological stress. To date, only one small sized study has examined the genetic profile of these patients. Despite the paucity of data, preliminary findings suggest the existence of a condition parallel to FHA in men, thus warranting further investigation.
Author contributions
FB: Data curation, Investigation, Methodology, Writing – original draft, Writing – review & editing. DB: Investigation, Writing – review & editing. VP: Investigation, Writing – review & editing. LC: Investigation, Writing – review & editing, Data curation. DD: Writing – review & editing, Supervision. RC: Writing – review & editing, Data curation. AC: Supervision, Writing – review & editing. RN: Conceptualization, Supervision, Writing – original draft, Writing – review & editing.
Funding
The author(s) declare that no financial support was received for the research, authorship, and/or publication of this article.
Conflict of interest
The authors declare that the research was conducted in the absence of any commercial or financial relationships that could be construed as a potential conflict of interest.
The author(s) declared that they were an editorial board member of Frontiers, at the time of submission. This had no impact on the peer review process and the final decision.
Publisher’s note
All claims expressed in this article are solely those of the authors and do not necessarily represent those of their affiliated organizations, or those of the publisher, the editors and the reviewers. Any product that may be evaluated in this article, or claim that may be made by its manufacturer, is not guaranteed or endorsed by the publisher.
References
1. Gordon CM, Ackerman KE, Berga SL, Kaplan JR, Mastorakos G, Misra M, et al. Functional hypothalamic amenorrhea: an endocrine society clinical practice guideline. J Clin Endocrinol Metab. (2017) 102:1413–39. doi: 10.1210/jc.2017-00131
2. Practice Committee of the American Society for Reproductive Medicine. Current evaluation of amenorrhea. Fertil Steril. (2006) 86:S148–55. doi: 10.1016/j.fertnstert.2006.08.013
3. Saadedine M, Kapoor E, Shufelt C. Functional hypothalamic amenorrhea: recognition and management of a challenging diagnosis. Mayo Clin Proc. (2023) 98:1376–85. doi: 10.1016/j.mayocp.2023.05.027
4. Gordon CM. Clinical practice. Functional hypothalamic amenorrhea. N Engl J Med. (2010) 363(4):365–71. doi: 10.1056/NEJMcp0912024
5. Męczekalski B, Niwczyk O, Battipaglia C, Troia L, Kostrzak A, Bala G, et al. Neuroendocrine disturbances in women with functional hypothalamic amenorrhea: an update and future directions. Endocrine. (2024) 84(3):769–85. doi: 10.1007/s12020-023-03619-w
6. Battipaglia C, Petrillo T, Semprini E, Ricciardiello F, Rusce ML, Prampolini G, et al. Low-dose estrogens as neuroendocrine modulators in functional hypothalamic amenorrhea (fha): the putative triggering of the positive feedback mechanism(s). Biomedicines. (2023) 11(6):1763. doi: 10.3390/biomedicines11061763
7. Caronia LM, Martin C, Welt CK, Sykiotis GP, Quinton R, Thambundit A, et al. A genetic basis for functional hypothalamic amenorrhea. N Engl J Med. (2011) 364:215–25. doi: 10.1056/NEJMoa0911064
8. Delaney A, Burkholder AB, Lavender CA, Plummer L, Mericq V, Merino PM, et al. Increased burden of rare sequence variants in gnRH-associated genes in women with hypothalamic amenorrhea. J Clin Endocrinol Metab. (2021) 106:e1441–52. doi: 10.1210/clinem/dgaa609
9. Young J. Does genetic susceptibility of the gonadotropic axis explain the variable impact of stressors causing functional hypothalamic amenorrhea? J Clin Endocrinol Metab. (2021) 106:e1473–5. doi: 10.1210/clinem/dgaa677
10. Fontana L, Garzia E, Marfia G, Galiano V, Miozzo M. Epigenetics of functional hypothalamic amenorrhea. Front Endocrinol (Lausanne). (2022) 13:953431. doi: 10.3389/fendo.2022.953431
11. Dwyer AA, Pitteloud N. Transition of care from childhood to adulthood: congenital hypogonadotropic hypogonadism. Endocr Dev. (2018) 33:82–98. doi: 10.1159/000487527
12. Frisch RE, McArthur JW. Menstrual cycles: fatness as a determinant of minimum weight for height necessary for their maintenance or onset. Science. (1974) 185:949–51. doi: 10.1126/science.185.4155.949
13. Sanborn CF, Albrecht BH, Wagner WW Jr. Athletic amenorrhea: lack of association with body fat. Med Sci Sports Exerc. (1987) 19:207–12. doi: 10.1249/00005768-198706000-00004
14. Morrison AE, Fleming S, Levy MJ. A review of the pathophysiology of functional hypothalamic amenorrhoea in women subject to psychological stress, disordered eating, excessive exercise or a combination of these factors. Clin Endocrinol (Oxf). (2021) 95:229–38. doi: 10.1111/cen.14399
15. Nazem TG, Ackerman KE. The female athlete triad. Sports Health. (2012) 4:302–11. doi: 10.1177/1941738112439685
16. Redman LM, Loucks AB. Menstrual disorders in athletes. Sports Med. (2005) 35:747–55. doi: 10.2165/00007256-200535090-00002
17. Chen L, Lu Y, Zhou YF, Wang Y, Zhan HF, Zhao YT, et al. The effects of weight loss-related amenorrhea on women’s health and the therapeutic approaches: a narrative review. Ann Transl Med. (2023) 11:132. doi: 10.21037/atm-22-6366
18. Welt CK, Chan JL, Bullen J, Murphy R, Smith P, DePaoli AM, et al. Recombinant human leptin in women with hypothalamic amenorrhea. New Engl J Med. (2004) 351:987–97. doi: 10.1056/NEJMoa040388
19. Xie Q, Kang Y, Zhang C, Xie Y, Wang C, Liu J, et al. The role of kisspeptin in the control of the hypothalamic-pituitary-gonadal axis and reproduction. Front Endocrinol (Lausanne). (2022) 13:925206. doi: 10.3389/fendo.2022.925206
20. Laughlin GA, Dominguez CE, Yen SS. Nutritional and endocrine-metabolic aberrations in women with functional hypothalamic amenorrhea. J Clin Endocrinol Metab. (1998) 83:25–32. doi: 10.1210/jcem.83.1.4502
21. Dominguez CE, Laughlin GA, Nelson JC, Yen SS. Altered binding of serum thyroid hormone to thyroxine-binding globulin in women with functional hypothalamic amenorrhea. Fertil Steril. (1997) 68:992–6. doi: 10.1016/s0015-0282(97)00393-2
22. Williams NI, Berga SL, Cameron JL. Synergism between psychosocial and metabolic stressors: impact on reproductive function in cynomolgus monkeys. Am J Physiol Endocrinol Metab. (2007) 293:E270–6. doi: 10.1152/ajpendo.00108.2007
23. Nappi RE, Facchinetti F. Psychoneuroendocrine correlates of secondary amenorrhea. Arch Womens Ment Health. (2003) 6:83–9. doi: 10.1007/s00737-002-0152-4
24. Gallinelli A, Matteo ML, Volpe A, Facchinetti F. Autonomic and neuroendocrine responses to stress in patients with functional hypothalamic secondary amenorrhea. Fertil Steril. (2000) 73(4): 812–6. doi: 10.1016/s0015-0282(99)00601-9
25. Shufelt CL, Saadedine M, Cook-Wiens G, Pisarska MD, Manson JE, Berga SL, et al. Functional hypothalamic amenorrhea and preclinical cardiovascular disease. J Clin Endocrinol Metab. (2023) 109:e51–7. doi: 10.1210/clinem/dgad498
26. Ruiz-Zambrana A, Berga SL. A clinician’s guide to functional hypothalamic amenorrhea. Clin Obstet Gynecol. (2020) 63:706–19. doi: 10.1097/GRF.0000000000000573
27. Geraghty AC, Kaufer D. Glucocorticoid regulation of reproduction. Adv Exp Med Biol. (2015) 872:253–78. doi: 10.1007/978-1-4939-2895-8_11
28. Calogero AE, Burrello N, Bosboom AM, Garofalo MR, Weber RF, D’Agata R. Glucocorticoids inhibit gonadotropin-releasing hormone by acting directly at the hypothalamic level. J Endocrinol Invest. (1999) 22:666–70. doi: 10.1007/BF03343627
29. Nappi RE, Petraglia F, Genazzani AD, D’Ambrogio G, Zara C, Genazzani AR. Hypothalamic amenorrhea: evidence for a central derangement of hypothalamic-pituitary-adrenal cortex axis activity. Fertil Steril. (1993) 59:571–6. doi: 10.1016/S0015-0282(16)55802-6
30. Valsamakis G, Chrousos G, Mastorakos G. Stress, female reproduction and pregnancy. Psychoneuroendocrinology. (2019) 100:48–57. doi: 10.1016/j.psyneuen.2018.09.031
31. Pape J, Herbison AE, Leeners B. Recovery of menses after functional hypothalamic amenorrhoea: if, when and why. Hum Reprod Update. (2021) 27:130–53. doi: 10.1093/humupd/dmaa032
32. Cialdella-Kam L, Guebels CP, Maddalozzo GF, Manore MM. Dietary intervention restored menses in female athletes with exercise-associated menstrual dysfunction with limited impact on bone and muscle health. Nutrients. (2014) 6:3018–39. doi: 10.3390/nu6083018
33. Karountzos V, Lambrinoudaki I, Tsitsika A, Deligeoroglou E. The role of total body fat mass and trunk fat mass, combined with other endocrine factors, in menstrual recovery and psychopathology of adolescents with Anorexia Nervosa. Gynecol Endocrinol. (2017) 33:757–62. doi: 10.1080/09513590.2017.1334199
34. Seminara SB, Hayes FJ, Crowley WF Jr. Gonadotropin-releasing hormone deficiency in the human (idiopathic hypogonadotropic hypogonadism and Kallmann’s syndrome): pathophysiological and genetic considerations. Endocr Rev. (1998) 19:521–39. doi: 10.1210/edrv.19.5.0344
35. Laitinen EM, Vaaralahti K, Tommiska J, Eklund E, Tervaniemi M, Valanne L, et al. Incidence, phenotypic features and molecular genetics of Kallmann syndrome in Finland. Orphanet J Rare Dis. (2011) 6:41. doi: 10.1186/1750-1172-6-41
36. Bianco SD, Kaiser UB. The genetic and molecular basis of idiopathic hypogonadotropic hypogonadism. Nat Rev Endocrinol. (2009) 5:569–76. doi: 10.1038/nrendo.2009.177
37. Stamou MI, Cox KH, Crowley WF Jr. Discovering genes essential to the hypothalamic regulation of human reproduction using a human disease model: adjusting to life in the “-omics” Era. Endocr Rev. (2015) 36:603–21. doi: 10.1210/er.2015-1045
38. Franco B, Guioli S, Pragliola A, Incerti B, Bardoni B, Tonlorenzi R, et al. A gene deleted in Kallmann’s syndrome shares homology with neural cell adhesion and axonal path-finding molecules. Nature. (1991) 353:529–36. doi: 10.1038/353529a0
39. Stamou MI, Georgopoulos NA. Kallmann syndrome: phenotype and genotype of hypogonadotropic hypogonadism. Metabolism. (2018) 86:124–34. doi: 10.1016/j.metabol.2017.10.012
40. Gianetti E, Hall JE, Au MG, Kaiser UB, Quinton R, Stewart JA, et al. When genetic load does not correlate with phenotypic spectrum: lessons from the GnRH receptor (GNRHR). J Clin Endocrinol Metab. (2012) 97:E1798–807. doi: 10.1210/jc.2012-1264
41. Beneduzzi D, Trarbach EB, Min L, Jorge AA, Garmes HM, Renk AC, et al. Role of gonadotropin-releasing hormone receptor mutations in patients with a wide spectrum of pubertal delay. Fertil Steril. (2014) 102:838–846.e2. doi: 10.1016/j.fertnstert.2014.05.044
42. Hietamäki J, Hero M, Holopainen E, Känsäkoski J, Vaaralahti K, Iivonen AP, et al. GnRH receptor gene mutations in adolescents and young adults presenting with signs of partial gonadotropin deficiency. PloS One. (2017) 12:e0188750. doi: 10.1371/journal.pone.0188750
43. de Roux N, Young J, Misrahi M, Genet R, Chanson P, Schaison G, et al. A family with hypogonadotropic hypogonadism and mutations in the gonadotropin-releasing hormone receptor. N Engl J Med. (1997) 337:1597–602. doi: 10.1056/NEJM199711273372205
44. Kim SH, Hu Y, Cadman S, Bouloux P. Diversity in fibroblast growth factor receptor 1 regulation: learning from the investigation of Kallmann syndrome. J Neuroendocrinol. (2008) 20:141–63. doi: 10.1111/j.1365-2826.2007.01627.x
45. Schwanzel-Fukuda M, Bick D, Pfaff DW. Luteinizing hormone-releasing hormone (LHRH)-expressing cells do not migrate normally in an inherited hypogonadal (Kallmann) syndrome. Brain Res Mol Brain Res. (1989) 6:311–26. doi: 10.1016/0169-328x(89)90076-4
46. Matsumoto S, Yamazaki C, Masumoto KH, Nagano M, Naito M, Soga T, et al. Abnormal development of the olfactory bulb and reproductive system in mice lacking prokineticin receptor PKR2. Proc Natl Acad Sci USA. (2006) 103:4140–5. doi: 10.1073/pnas.0508881103
47. Cheng CK, Leung PC. Molecular biology of gonadotropin-releasing hormone (GnRH)-I, GnRH-II, and their receptors in humans. Endocr Rev. (2005) 26:283–306. doi: 10.1210/er.2003-0039
48. Dupont C, Armant DR, Brenner CA. Epigenetics: definition, mechanisms and clinical perspective. Semin Reprod Med. (2009) 27:351–7. doi: 10.1055/s-0029-1237423
49. Iyer AK, Brayman MJ, Mellon PL. Dynamic chromatin modifications control GnRH gene expression during neuronal differentiation and protein kinase C signal transduction. Mol Endocrinol. (2011) 25:460–73. doi: 10.1210/me.2010-0403
50. Vazquez MJ, Toro CA, Castellano JM, Ruiz-Pino F, Roa J, Beiroa D, et al. SIRT1 mediates obesity- and nutrient-dependent perturbation of pubertal timing by epigenetically controlling Kiss1 expression. Nat Commun. (2018) 9:4194. doi: 10.1038/s41467-018-06459-9
51. Frieling H, Gozner A, Römer KD, Lenz B, Bönsch D, Wilhelm J, et al. Global DNA hypomethylation and DNA hypermethylation of the alpha synuclein promoter in females with anorexia nervosa. Mol Psychiatry. (2007) 12:229–30. doi: 10.1038/sj.mp.4001931
52. Neyazi A, Buchholz V, Burkert A, Hillemacher T, de Zwaan M, Herzog W, et al. Association of leptin gene DNA methylation with diagnosis and treatment outcome of anorexia nervosa. Front Psychiatry. (2019) 10:197. doi: 10.3389/fpsyt.2019.00197
53. Batury VL, Walton E, Tam F, Wronski ML, Buchholz V, Frieling H, et al. DNA methylation of ghrelin and leptin receptors in underweight and recovered patients with anorexia nervosa. J Psychiatr Res. (2020) 131:271–8. doi: 10.1016/j.jpsychires.2020.08.026
54. Nakazato M, Murakami N, Date Y, Kojima M, Matsuo H, Kangawa K, et al. A role for ghrelin in the central regulation of feeding. Nature. (2001) 409:194–8. doi: 10.1038/35051587
55. Bernardoni F, Bernhardt N, Pooseh S, King JA, Geisler D, Ritschel F, et al. Metabolic state and value-based decision-making in acute and recovered female patients with anorexia nervosa. J Psychiatry Neurosci. (2020) 45:253–61. doi: 10.1503/jpn.190031
56. Cao C, Ding Y, Kong X, Feng G, Xiang W, Chen L, et al. Reproductive role of miRNA in the hypothalamic-pituitary axis. Mol Cell Neurosci. (2018) 88:130–7. doi: 10.1016/j.mcn.2018.01.008
57. Lannes J, L’Hôte D, Garrel G, Laverrière JN, Cohen-Tannoudji J, Quérat B. Rapid communication: A microRNA-132/212 pathway mediates GnRH activation of FSH expression. Mol Endocrinol. (2015) 29:364–72. doi: 10.1210/me.2014-1390
58. Ye RS, Li M, Li CY, Qi QE, Chen T, Cheng X, et al. miR-361-3p regulates FSH by targeting FSHB in a porcine anterior pituitary cell model. Reproduction. (2017) 153:341–9. doi: 10.1530/REP-16-0373
59. Higuchi Y, Soga T, Parhar IS. Potential roles of microRNAs in the regulation of monoamine oxidase A in the brain. Front Mol Neurosci. (2018) 11:339. doi: 10.3389/fnmol.2018.00339
60. Wheeler GD, Wall SR, Belcastro AN, Cumming DC. Reduced serum testosterone and prolactin levels in male distance runners. JAMA. (1984) 252:514–6. doi: 10.1001/jama.1984.03350040044020
61. Ayers JW, Komesu Y, Romani T, Ansbacher R. Anthropomorphic, hormonal, and psychologic correlates of semen quality in endurance-trained male athletes. Fertil Steril. (1985) 43:917–21. doi: 10.1016/s0015-0282(16)48622-x
62. MacConnie SE, Barkan A, Lampman RM, Schork MA, Beitins IZ. Decreased hypothalamic gonadotropin-releasing hormone secretion in male marathon runners. N Engl J Med. (1986) 315:411–7. doi: 10.1056/NEJM198608143150702
63. Cumming DC, Wheeler GD, McColl EM. The effects of exercise on reproductive function in men. Sports Med. (1989) 1):1–17. doi: 10.2165/00007256-198907010-00001
64. McColl EM, Wheeler GD, Gomes P, Bhambhani Y, Cumming DC. The effects of acute exercise on pulsatile LH release in high-mileage male runners. Clin Endocrinol (Oxf). (1989) 31:617–21. doi: 10.1111/j.1365-2265.1989.tb01286.x
65. Hackney AC, Sinning WE, Bruot BC. Hypothalamic-pituitary-testicular axis function in endurance-trained males. Int J Sports Med. (1990) 11:298–303. doi: 10.1055/s-2007-1024811
66. Burge MR, Lanzi RA, Skarda ST, Eaton RP. Idiopathic hypogonadotropic hypogonadism in a male runner is reversed by clomiphene citrate. Fertil Steril. (1997) 67:783–5. doi: 10.1016/s0015-0282(97)81384-2
67. Fahrner CL, Hackney AC. Effects of endurance exercise on free testosterone concentration and the binding affinity of sex hormone binding globulin (SHBG). Int J Sports Med. (1998) 19:12–5. doi: 10.1055/s-2007-971872
68. Hackney AC, Fahrner CL, Gulledge TP. Basal reproductive hormonal profiles are altered in endurance trained men. J Sports Med Phys Fitness. (1998) 38:138–41.
69. Klibanski A, Beitins IZ, Badger T, Little R, McArthur JW. Reproductive function during fasting in men. J Clin Endocrinol Metab. (1981) 53:258–63. doi: 10.1210/jcem-53-2-258
70. Strauss RH, Lanese RR, Malarkey WB. Weight loss in amateur wrestlers and its effect on serum testosterone levels. JAMA. (1985) 254:3337–8. doi: 10.1001/jama.1985.03360230069025
71. Shemesh A, Endevelt R, Levy Y. Reversible nutritional hypogonadism in a 22-year-old man. Am J Med. (2011) 124:e1–2. doi: 10.1016/j.amjmed.2011.04.021
72. Opstad PK, Aakvaag A. Decreased serum levels of oestradiol, testosterone and prolactin during prolonged physical strain and sleep deprivation, and the influence of a high calorie diet. Eur J Appl Physiol Occup Physiol. (1982) 49:343–8. doi: 10.1007/BF00441295
73. Nindl BC, Barnes BR, Alemany JA, Frykman PN, Shippee RL, Friedl KE. Physiological consequences of U.S. Army Ranger training. Med Sci Sports Exerc. (2007) 39:1380–7. doi: 10.1249/MSS.0b013e318067e2f7
74. Dwyer AA, Chavan NR, Lewkowitz-Shpuntoff H, Plummer L, Hayes FJ, Seminara SB, et al. Functional hypogonadotropic hypogonadism in men: underlying neuroendocrine mechanisms and natural history. J Clin Endocrinol Metab. (2019) 104:3403–14. doi: 10.1210/jc.2018-02697
75. Jensen TK, Andersson AM, Hjollund NH, Scheike T, Kolstad H, Giwercman A, et al. Inhibin B as a serum marker of spermatogenesis: correlation to differences in sperm concentration and follicle-stimulating hormone levels. A study of 349 Danish men. J Clin Endocrinol Metab. (1997) 82:4059–63. doi: 10.1210/jcem.82.12.4456
76. Chevrier L, Guimiot F, de Roux N. GnRH receptor mutations in isolated gonadotropic deficiency. Mol Cell Endocrinol. (2011) 346:21–8. doi: 10.1016/j.mce.2011.04.018
77. Tommiska J, Jørgensen N, Christiansen P, Juul A, Raivio T. A homozygous R262Q mutation in the gonadotropin-releasing hormone receptor presenting as reversal of hypogonadotropic hypogonadism and late-onset hypogonadism. Clin Endocrinol (Oxf). (2013) 78:316–7. doi: 10.1111/j.1365-2265.2012.04493.x
78. Barbagallo F, Condorelli RA, Mongioì LM, Cannarella R, Cimino L, Magagnini MC, et al. Molecular mechanisms underlying the relationship between obesity and male infertility. Metabolites. (2021) 11:840. doi: 10.3390/metabo11120840
79. Barbagallo F, La Vignera S, Cannarella R, Mongioì LM, Garofalo V, Leanza. C, et al. Obesity and male reproduction: do sirtuins play a role? Int J Mol Sci. (2022) 23:973. doi: 10.3390/ijms23020973
80. Eng PC, Phylactou M, Qayum A, Woods C, Lee H, Aziz S, et al. Obesity-related hypogonadism in women. Endocr Rev. (2024) 45:171–89. doi: 10.1210/endrev/bnad027
81. Cannarella R, Crafa A, Barbagallo F, Lundy SD, La Vignera S, Condorelli RA, et al. H19 sperm methylation in male infertility: A systematic review and meta-analysis. Int J Mol Sci. (2023) 24:7224. doi: 10.3390/ijms24087224
Keywords: functional hypothalamic amenorrhea (FHA), idiopathic hypogonadotropic hypogonadism, genetic susceptibility, epigenetic, stress, male equivalent of FHA
Citation: Barbagallo F, Bosoni D, Perone V, Cucinella L, Dealberti D, Cannarella R, Calogero AE and Nappi RE (2024) Gene-environment interaction in functional hypothalamic amenorrhea. Front. Endocrinol. 15:1423898. doi: 10.3389/fendo.2024.1423898
Received: 26 April 2024; Accepted: 12 August 2024;
Published: 29 August 2024.
Edited by:
Naguib Bin Salleh, University of Malaya, MalaysiaReviewed by:
Dana Manuela Savulescu, Bioforum, IsraelJames William Crane, University of Tasmania, Australia
Copyright © 2024 Barbagallo, Bosoni, Perone, Cucinella, Dealberti, Cannarella, Calogero and Nappi. This is an open-access article distributed under the terms of the Creative Commons Attribution License (CC BY). The use, distribution or reproduction in other forums is permitted, provided the original author(s) and the copyright owner(s) are credited and that the original publication in this journal is cited, in accordance with accepted academic practice. No use, distribution or reproduction is permitted which does not comply with these terms.
*Correspondence: Rossella E. Nappi, nappi@rossellanappi.com