- 1Dongzhimen Hospital, Beijing University of Chinese Medicine, Beijing, China
- 2School of Traditional Chinese Medicine, Beijing University of Chinese Medicine, Beijing, China
- 3School of Traditional Chinese Medicine, Hubei University of Chinese Medicine, Wuhan, Hubei, China
Objective: Emerging evidence suggests alterations in gut microbiota (GM) composition following thyroid nodules (TNs) development, yet the causal relationship remains unclear. Utilizing Mendelian Randomization (MR), this study aims to elucidate the causal dynamics between GM and TNs.
Methods: Employing summary statistics from the MiBioGen consortium (n=18,340) and FinnGen consortium (1,634 TNs cases, 263,704 controls), we conducted univariable and multivariable MR analyses to explore the GM-TNs association. Techniques including inverse variance weighted, MR-Egger regression, weighted median, and MR-PRESSO were utilized for causal inference. Instrumental variable heterogeneity was assessed through Cochran’s Q statistic and leave-one-out analysis. Reverse MR was applied for taxa showing significant forward MR associations, with multivariate adjustments for confounders.
Results: Our findings suggest that certain microbiota, identified as Ruminococcaceae_NK4A214_group (OR, 1.89; 95%CI, 0.47-7.64; p = 0.040), Senegalimassilia (OR, 1.72; 95%CI, 1.03-2.87; p =0.037), Lachnospiraceae (OR,0.64; 95%CI,0.41-0.99; p =0.045), exhibit a protective influence against TNs’ development, indicated by negative causal associations. In contrast, microbiota categorized as Desulfovibrionales (OR, 0.63; 95%CI, 0.41-0.95; p =0.028), Prevotella_7 (OR, 0.79; 95%CI, 0.63-1.00; p =0.049), Faecalibacterium (OR, 0.66; 95%CI, 0.44-1.00; p =0.050), Desulfovibrionaceae (OR, 0.55; 95%CI, 0.35-0.86; p =0.008), Deltaproteobacteria (OR, 0.65; 95%CI, 0.43-0.97; p =0.036) are have a positive correlation with with TNs, suggesting they may serve as risk factors. Reverse MR analyses did not establish significant causal links. After comprehensive adjustment for confounders, taxa Desulfovibrionales (Order), Desulfovibrionaceae (Family), Deltaproteobacteria (Class) remain implicated as potential contributors to TNs’ risk.
Discussion: This study substantiates a significant causal link between GM composition and TNs development, underscoring the thyroid-gut axis’s relevance. The findings advocate for the integration of GM profiles in TNs’ prevention and management, offering a foundation for future research in this domain.
1 Introduction
Thyroid nodules (TNs), considered localized enlargements of the thyroid gland, represent a prevalent category of endocrine disorders. The annual incidence of TNs is escalating, with the prevalence in females being approximately triple that observed in males (1, 2). During long-term follow-up, an increase in nodule grading is observed in a subset of patients with TNs. Among these patients, the proportion diagnosed with thyroid cancer (TC) via fine-needle aspiration biopsy ranges from 4.0% to 6.5% (3). TNs poses a substantial burden on global health (4) and exerts significant psychological stress on patients. Research has identified several risk factors for TNs, including exposure to radiation, genetic predispositions, estrogen levels, Body Mass Index (BMI), smoking habits, and metabolic syndrome (5, 6). Recently, the correlation between the gut microbiota (GM) and thyroid health has attracted considerable attention. However, the specific pathogenesis of TNs remains unclear, and effective clinical treatment strategies are lacking.
The GM, also known as gut flora, comprises a complex ecosystem of bacteria, viruses, fungi, and other microorganisms inhabiting the human gastrointestinal tract. This ecosystem, predominantly bacterial in healthy adults, includes a mix of anaerobic and aerobic organisms, with a notable presence of bifidobacteria and lactobacilli (7). The GM plays an essential role in health, supporting digestion, nutrient absorption, metabolism, and immune function. However, alterations in diet, medication use, and stress can disrupt the microbial balance, potentially favoring pathogenic bacteria and triggering or exacerbating diseases (8).
In the past decade, a large number of studies have been devoted to exploring the impact of microbial communities on various diseases, affirming their critical role. A causal link has been established between GM disturbances and diseases affecting the nervous, cardiovascular, and respiratory systems, among others (9–11), including disorders of the thyroid, a key organ within the endocrine system. The thyroid and gastrointestinal epithelium, both of endodermal origin, exhibit functional and morphological similarities that contribute to mutual regulation, maintaining both thyroid and gastrointestinal homeostasis. The high co-occurrence of thyroid and gastrointestinal diseases underlines a potential thyroid-gut axis (12). Thyroid hormones are known to enhance gastrointestinal motility, with thyroid dysfunction often manifesting as gastrointestinal complaints such as dyspepsia, diarrhea, constipation, and microbiota dysbiosis (13). The GM influences various thyroid conditions, including Hashimoto’s thyroiditis, TC, hyperthyroidism, and hypothyroidism (14–17). The GM possesses a variety of degradative enzymes that are involved in the metabolism of various nutrients, including iodine, selenium, zinc, and iron. It also plays a crucial role in the synthesis and regulation of minerals and vitamins. The mechanisms by which the GM affects the thyroid may involve influencing deiodinase activity and inhibiting TSH, directly impacting thyroid hormone levels and potentially leading to thyroid dysfunction (18, 19). Additionally, the GM may promote inflammation and reduce immune tolerance, thereby altering immune responses (20). This can disrupt the intestinal mechanical and immune barriers, increase intestinal permeability, promote bacterial translocation, and ultimately trigger local and systemic inflammatory responses. Studies have linked GM composition and its metabolic activities, such as butyrate production, to the presence of TNs and their TI-RADS grading (21), suggesting a reciprocal impact on thyroid function (22). Early identification of TN risk factors is imperative for the development of diagnostic and therapeutic strategies. Currently, the primary methods for intervening in GM include the administration of probiotics and fecal microbiota transplantation.
Mendelian Randomization (MR), using Single Nucleotide Polymorphisms (SNPs) as instrumental variables (IVs) for genome-wide association studies (GWAS), is utilized to infer causal relationships between exposures and outcomes (23). Emulating the random allocation mechanism of randomized controlled trials (RCTs) through the distribution of alleles at conception, MR offers a powerful tool to circumvent the limitations and biases inherent in observational studies (24). The application of MR in delineating causal pathways among complex diseases has been extended to explore the connections between GM and a range of conditions, including autoimmune, cardiovascular, and psychiatric disorders.
However, the causal relationship between GM and TNs remains undefined. As a critical environmental factor in disease pathogenesis, GM warrants focused investigation. Systematic reviews indicate a lack of studies on the causal association between GM and TNs. Therefore, this study aims to employ MR analysis, utilizing GWAS summary data from the MiBioGen and FinnGen consortia, to elucidate the bidirectional causal relationship between GM and TNs. This research aspires to provide novel insights for the prevention and management of TNs.
2 Materials and methods
2.1 Ethics statement and study design
This investigation utilizes publicly available data of international acclaim, compliant with the Declaration of Helsinki and sanctioned by pertinent ethical bodies (the MiBioGen Consortium and the FinnGen research consortium). Such foundational datasets have garnered ethics and institutional review board endorsements, obviating the necessity for further ethical scrutiny. Selection of SNPs significantly linked with exposures as IVs was executed adhering to rigorous selection paradigms, incorporating these IVs into MR and sensitivity analyses based on the hypothetical constructs of MR.
The focal point of this research is the examination of the GM and TNs as exposures to discern their implications on specific outcomes. The MR analytical framework employed herein is anchored in three cardinal assumptions (25). Firstly, it is verified that the genetic variations possess independent and significant correlations with the exposures, with the instrumental variable SNPs demonstrating substantial linkage to GM and TNs, as ascertained through F-statistics and p-values within correlation analyses. In the second place, it is validated that the genetic variations pertinent to the exposures are not directly correlated with the outcomes, with SNPs influencing TNs solely via GM. Lastly, it is presupposed that these variables are exempt from any association with confounding factors (Figure 1).
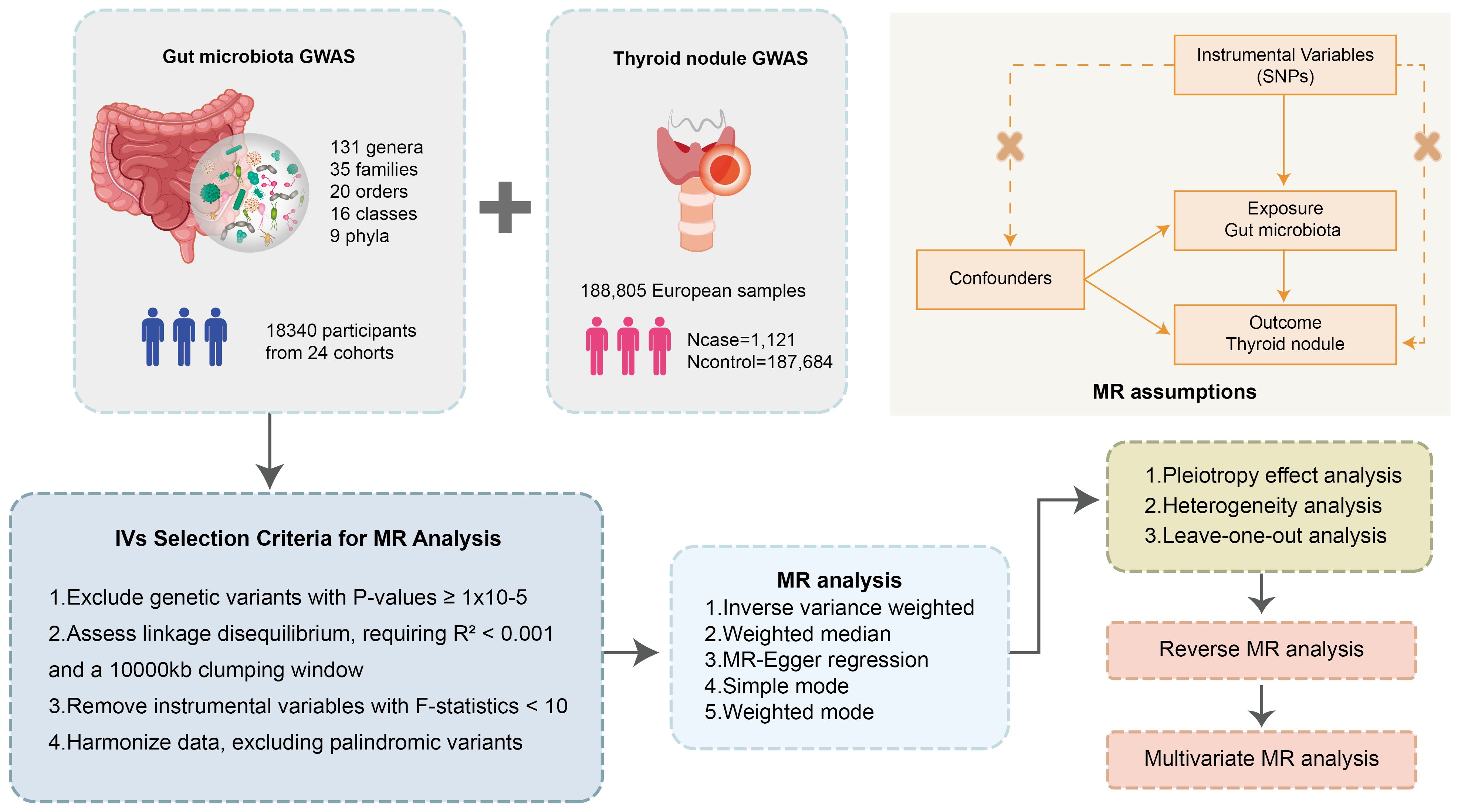
Figure 1 Flowchart of the MR randomization study and key assumptions. MR, Mendelian randomization; SNPs, single nucleotide polymorphisms; IVW, inverse-variance weighted; MR-PRESSO, MR pleiotropy residual sum and outlier.
The study’s reporting of this study is in strict alignment with adheres closely to the Guidelines on Strengthening the Reporting of Observational Studies in Epidemiology using Mendelian Randomization (STROBE-MR) (26), guaranteeing thorough and transparent disclosure of methodological and analytical details (Supplementary Table S1).
2.2 Data sources
The data on the human GM is derived from the largest GWAS published to date, representing the most extensive multi-ethnic, whole-genome analysis of human autosomal genetic variations and the GM (27). The research includes 18,340 participants from various nations/areas, primarily representing the European population. The research successfully identified 211 bacterial taxa, including 9 phyla, 16 classes, 20 orders, 35 families (3 of which are unknown), and 131 genera (12 unknown). After excluding 15 taxa lacking family and genus information, the study conducted a preliminary analysis on the remaining 196 bacteria (details shown in Supplementary Table S2).
Moreover, summary data for TNs’ GWAS were obtained from the FinnGen consortium, which includes 1,634 cases and 263,704 controls, all of European descent (details shown in Supplementary Table S2).
Summary data for GWAS on risk factors for TNs (including high blood pressure, obesity class 3, alcohol consumption, type 2 diabetes, and ever smoked) were sourced from the corresponding consortium within the Integrative Epidemiology Unit (IEU) Open GWAS project (Supplementary Table S2).
2.3 Selection of instrumental variables
In this investigation, SNPs manifesting a p-value below 1×10-5 were harnessed as preliminary genetic instrumental variables for the MR analysis. This p-value threshold has been identified as optimally conducive across numerous MR studies investigating the GM, aiming to enhance the genetic variance explained by genetic predictors and to expand the array of SNPs available for sensitivity analysis (28). Furthermore, the F-statistic is employed as a robust measure to access the stability of the instrumental variables.
It is typically derived using a formula where R2 denotes the variance elucidated by the instrumental variable, n symbolizes the sample size, and k denotes the number of selected IVs. The F-statistic is calculated to ascertain the strength of the chosen instrumental variables, with an F-value exceeding 10 signaling that the instruments are robust against the weak instrument bias (29); SNPs exhibiting an F< 10 were disqualified.
To mitigate the impact of linkage disequilibrium (LD) on the results, the included genetic variants were clustered using an R2 threshold greater than 0.001 and a clustering window of less than 10,000 kb (30), to sift through the genetic variations. Subsequent to this, SNPs associated with TNs were disqualified based on the criterion: Poutcome< Pexposure. Lastly, SNPs featuring palindromic structures were eliminated to guarantee that the filtered SNPs were congruently oriented in terms of alleles for both exposure and outcome, thus refining the integrity of the analytical framework.
2.4 Statistical analysis of mendelian randomization
For the univariate Mendelian randomization (UVMR) analysis, the inverse variance weighted (IVW) method, the main tool for exploring the relationship between GM and TNs. This approach synthesizes Wald estimators across SNPs to deduce the causal impact of exposure on the result, amalgamating ratio estimates from each genetic variant (31), and provides the most precise estimations when the genetic variants are valid IVs (32). Additionally, adjunctive analytical techniques such as MR-Egger regression, weighted median, simple mode, and weighted mode are utilized.
Primarily, MR-Egger regression addresses pleiotropy concerns (33, 34), with significance in the MR-PRESSO (p< 0.05) bolstering the validity of positive outcomes. A suite of sensitivity analyses ensures the robustness of results. Cochran’s Q test within the IVW framework and via MR-Egger regression (considering p< 0.05 as indicative of potential heterogeneity) assesses IV heterogeneity. Leave-one-out sensitivity analysis evaluates outliers and the stability of results (33). MR-Egger and MR-PRESSO tests identify pleiotropy and outliers, with the MR-Egger intercept specifically testing for horizontal pleiotropy. The absence of significant horizontal pleiotropy is suggested by p > 0.05. MR-PRESSO, compared to MR-Egger, more accurately detects horizontal pleiotropy and outliers (35).
To fully understand the connection between GM and TNs, reverse MR analysis was also performed on GM causally associated to TNs in the forward MR assessment, using the same settings and methodologies. Moreover, potential confounders such as alcohol consumption, high blood pressure, obesity class 3, smoking status, and type 2 diabetes could influence the causal link between GM and TNs. To account for these confounders, the study incorporated these factors into the forward MR analysis via multivariable Mendelian randomization (MVMR). IVW, MR-Egger regression, and weighted median methods were applied in MVMR analyses, with Cochran’s Q tests evaluating the consistency of outcomes.
Statistical analyses were performed using R software (version 4.3.1), employing the TwoSampleMR, MR-PRESSO, and Mendelian Randomization R packages for MR investigations.
3 Results
3.1 Selection of instrumental variables
In this study, adhering to the instrumental variable selection criteria with an F-statistic greater than 10 to mitigate the risk of weak instrumental bias, 196 GM were associated with 2, 077 SNPs in total. Among these, 102 SNPs were related to 9 phyla, 178 SNPs to 16 classes, 216 SNPs to 20 orders, 351 SNPs to 32 families, and 1, 230 SNPs to 119 genera. The analysis utilized the IVW method as the primary reference, with p< 0.05 as the threshold, to conduct MR analysis on 196 bacterial taxa. A total of 163 bacterial groups from 16 groups were found to be associated with TNs. These groups include 1 class (14 SNPs), 1 order (13 SNPs), 2 families (29 SNPs), and 5 genera (60 SNPs) (Supplementary Table S3).
3.2 Causal effects of gut microbiota on thyroid nodules by UVMR
The results of MR Analysis are shown in Figure 2, where the beta values for all MR analysis methods and the p-values for IVW methods are recorded. Positive results from the MR analysis were identified based on significant p-values from the IVW. The IVW revealed that 9 taxa of GM are related to TNs. However, the beta results from the five methods for Eisenbergiella (Genus) showed inconsistent directions, and to ensure the study outcomes were as free from confounding factors and horizontal pleiotropy as possible, they were excluded. The direction of the beta results from all five methods for the remaining taxa was consistent, enhancing the credibility of a true causal relationship (Supplementary Table S4; Figure 3). The F-statistics of the IVs included in the analysis that were significantly associated with the GM were all greater than 10, suggesting that the estimates are less susceptible to weak instrumental bias (Supplementary Table S1).
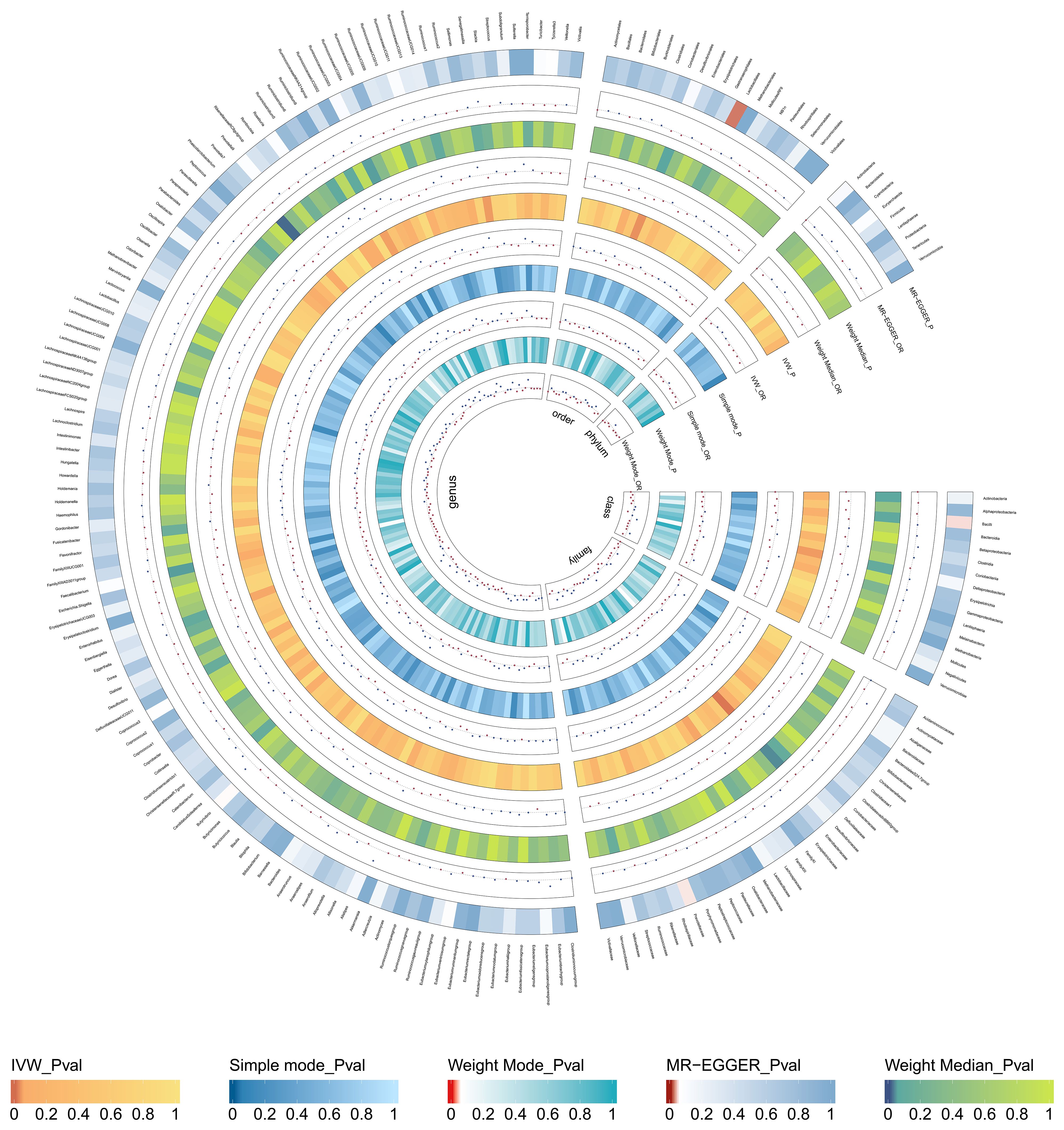
Figure 2 Causal effect of the gut microbiota on thyroid nodules based on MR analyses. From outside to inside, the p-values and OR of MR-Egger, weighted median, inverse variance weighted(IVW), simple mode, and weighted mode are represented, respectively.
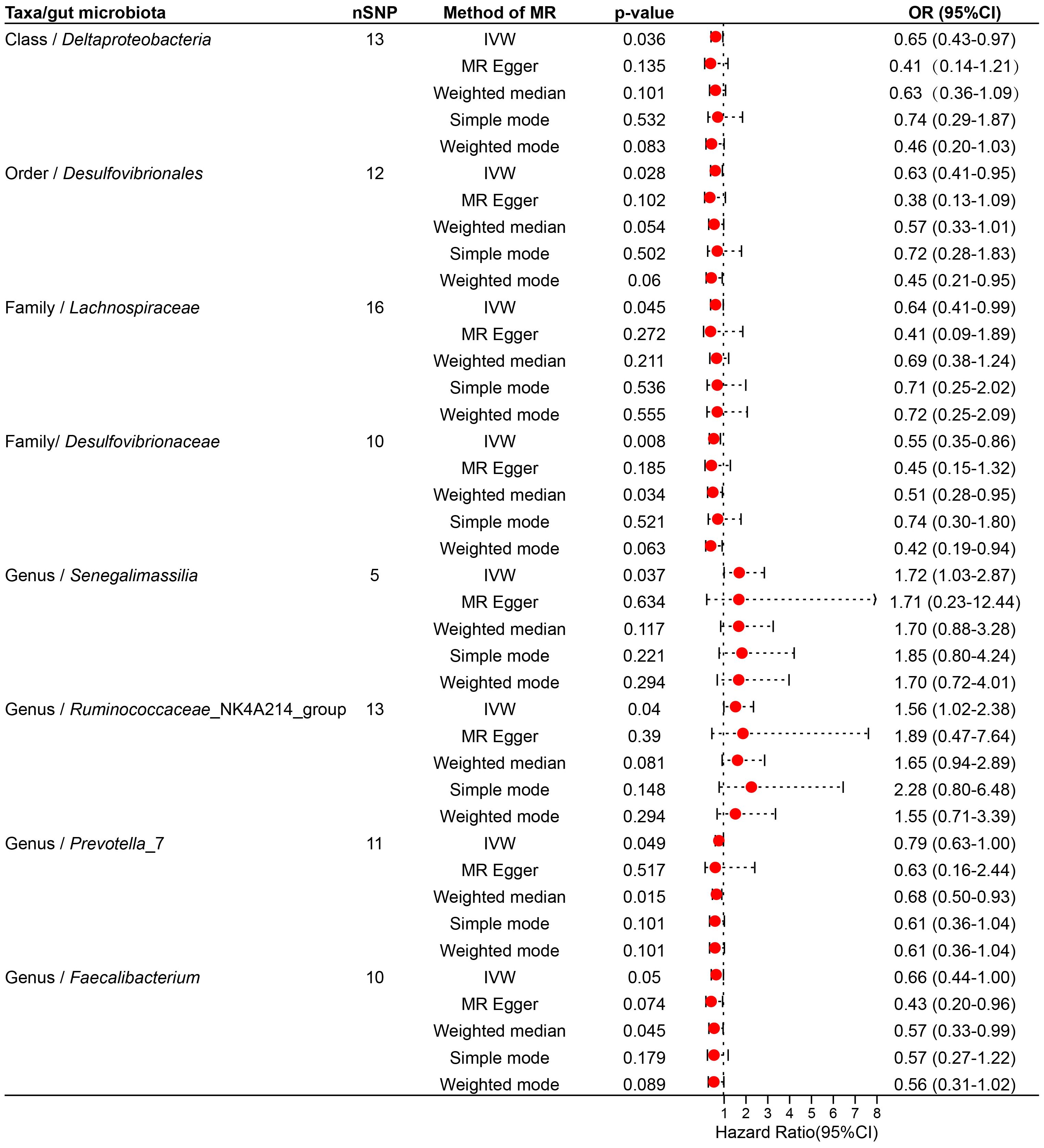
Figure 3 Forest plot illustrating the causal effect of the gut microbiota on thyroid nodules using five methods of MR analyses.
Specifically, Ruminococcaceae_NK4A214_group (Genus) (OR, 1.89; 95%CI, 0.47-7.64; p = 0.040), Senegalimassilia (Genus) (OR, 1.72; 95%CI, 1.03-2.87; p =0.037), Lachnospiraceae (Family) (OR,0.64; 95%CI,0.41-0.99; p =0.045) presented a negative causal association with TNs, potentially acting as a protective factor against the onset of TNs. Conversely, an increase in the abundance of Desulfovibrionales (Order) (OR, 0.63; 95%CI, 0.41-0.95; p =0.028), Prevotella_7 (Genus) (OR, 0.79; 95%CI, 0.63-1.00; p =0.049), Faecalibacterium (Genus) (OR, 0.66; 95%CI, 0.44-1.00; p =0.050), Desulfovibrionaceae (Family) (OR, 0.55; 95%CI, 0.35-0.86; p =0.008), Deltaproteobacteria (Class) (OR, 0.65; 95%CI, 0.43-0.97; p =0.036) may induce the occurrence of TNs, with higher abundance of these taxa associated with an increased potential risk of TNs (Supplementary Table S4; Figure 3).
3.3 Sensitivity analysis
We performed pleiotropy, heterogeneity test, and leave-one-out analysis in sensitivity analyses. The regression intercepts of all MR-Egger regressions were not significantly deviated from zero (all intercepts p > 0.05). Cochran’s Q test and MR-PRESSO p-values were greater than 0.05; all of these conditions indicated the absence of significant pleiotropy and heterogeneity (as shown in Supplementary Tables S5, S6). In addition, leave-one-out analysis revealed no specific SNP driving the link between GM and TNs (as shown in Supplementary Table S7).
3.4 Causal effects of thyroid nodules on gut microbiota
Further, a reverse MR analysis was specifically conducted on GM with positive results to explore whether there is a causal relationship between TNs and GM. With TNs as the exposure and the GM (Ruminococcaceae_NK4A214_group, Senegalimassilia, Lachnospiraceae, Desulfovibrionales, Prevotella_7, Faecalibacterium, Desulfovibrionaceae, Deltaproteobacteria) with a definitive causal relationship with bacterial pneumonia as the outcome, reverse MR was conducted (shown in Supplementary Tables S8, S9). No heterogeneity or pleiotropy was found in the reverse MR analysis, and leave-one-out analysis indicated that no SNP were significantly linked with the outcome (Supplementary Tables S10, S11).
A total of 40 instrumental variables were included under the screening conditions of p<1×10-5, r2 = 0.001, and kb=10,000, but no significant reverse causal relationships were detected in subsequent MR analyses.
3.5 Causal effects of thyroid nodules on gut microbiota
To determine whether the aforementioned microbiota directly or indirectly impact the risk of TNs through common confounding factors, further MVMR analyses were performed. In MR analyses with TNs as the outcome, confounders such as high blood pressure, obesity class 3, alcohol consumption, type 2 diabetes, and ever smoked, were considered, with the final results shown in Figure 4, Supplementary Table S12. After simultaneously considering all confounders, the results were shown in Figure 5, Supplementary Table S13. To be specific, Desulfovibrionales (Order), Desulfovibrionaceae (Family), Deltaproteobacteria (Class) remained a potential risk factor for TNs when TNs was the outcome. The consistent direction across different MR models further supports the causal inference. Moreover, Cochran’s Q from multivariable IVW and multivariable MR-Egger tests and intercepts detected no potential pleiotropy and heterogeneity (Supplementary Table S14).
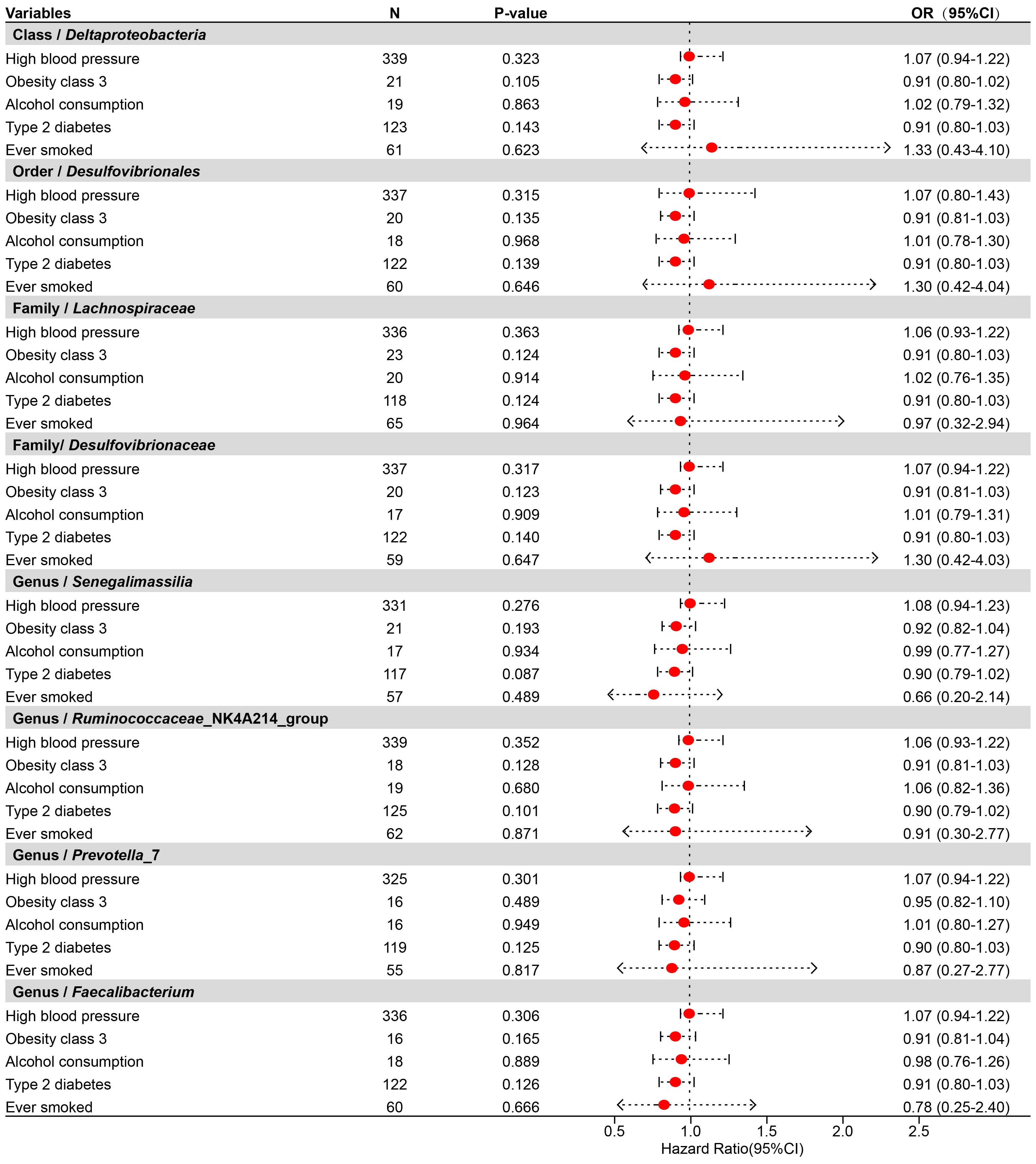
Figure 4 Forest plot illustrating the causal effect of gut microbiota on thyroid nodules using the IVW method to adjust for each of the five confounders (including high blood pressure, obesity class 3, alcohol consumption, type 2 diabetes, and ever smoked).
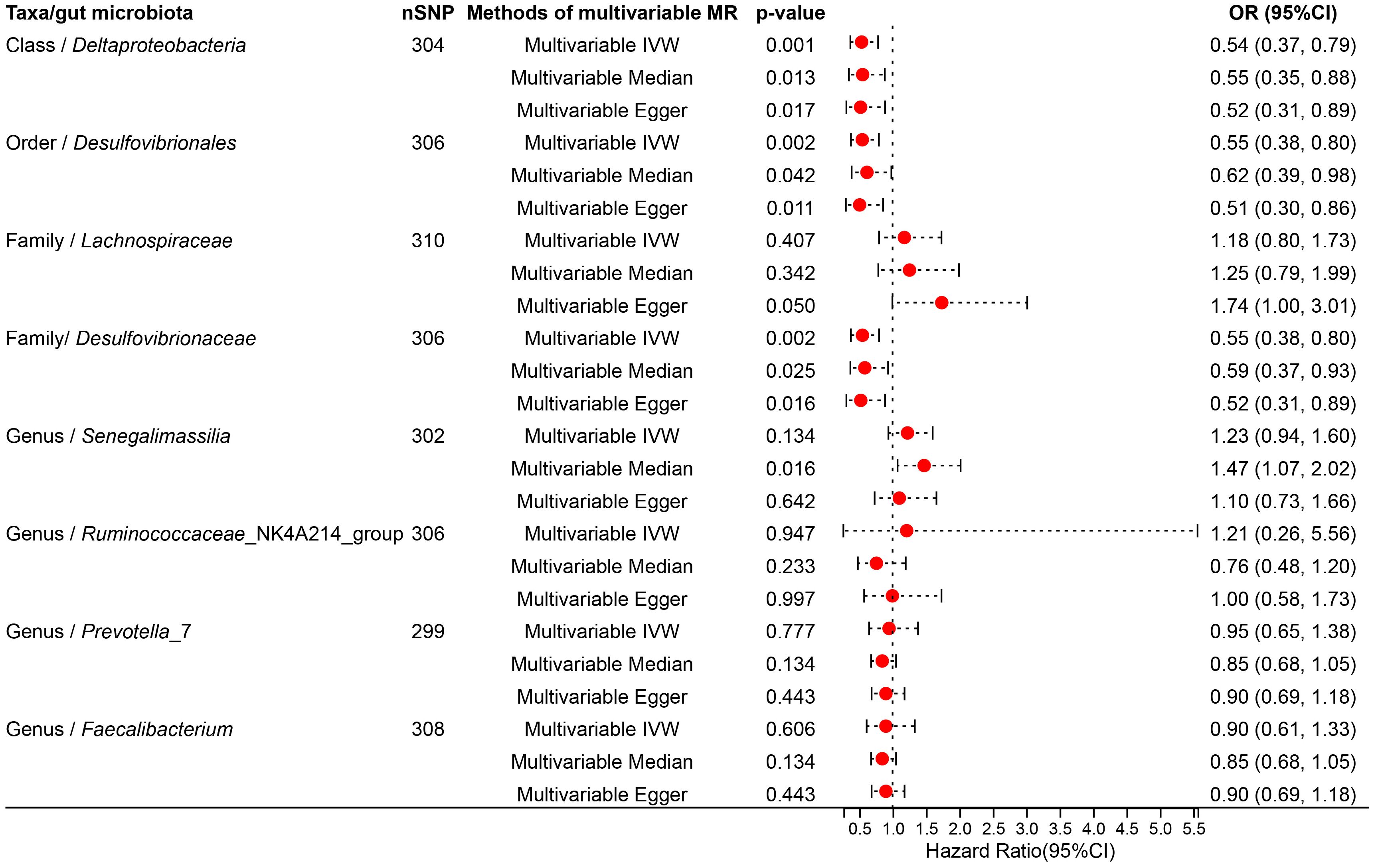
Figure 5 Forest plot illustrating the causal effect of gut microbiota on thyroid nodules using IVW methods with simultaneous adjustment for five confounders.
4 Discussion
In the context of escalating global health consciousness, the detection rates of TNs have experienced a marked increase, metaphorically likened to a “time bomb” profoundly impacting patient emotional well-being. Clinically, individuals diagnosed with TNs frequently exhibit symptoms of depression and anxiety, conditions that are closely linked with gastrointestinal disorders. Recent epidemiological studies have noted a significant escalation in TNs prevalence among individuals with persistent Helicobacter pylori infection (36). The pathogenesis of TNs remains complex and multifaceted, influenced by environmental, genetic, and autoimmune factors, underscoring the critical need for further research and exploration. Although a substantial body of evidence underscores the association between thyroid pathologies and the composition of the GM, research on the role of GM in patients with TNs remains scant. This investigation delves into the bidirectional causal relationship between the GM and TNs, providing new perspectives.
This study reveals a negative causal association between GM, considered as an exposure factor, and TNs as an outcome, identifying specific microbial taxa (Ruminococcaceae_NK4A214_group, Senegalimassilia, Lachnospiraceae) as potential protective factors against TNs development. In contrast, other microbial taxa (Desulfovibrionales, Prevotella_7, Faecalibacterium, Desulfovibrionaceae, Deltaproteobacteria) exhibit a positive causal relationship with TNs, potentially serving as biomarkers of the disease. Upon adjusting for confounding variables, certain taxonomic classifications—Desulfovibrionales (Order), Desulfovibrionaceae (Family), Deltaproteobacteria (Class)—persist as potential risk factors for TNs development.
The Ruminococcaceae_NK4A214_group, a member of the Ruminococcaceae family, is related in the gut’s energy metabolism and fatty acid synthesis, particularly in the fermentation of complex carbohydrates to produce butyrate, noted for its anti-inflammatory properties (37). The association between butyrate production and thyroid disorders, including TNs, is well established. A clinical study by Ang Li et al. involving 196 TNs patients and a cohort of healthy controls demonstrated a reduction in the diversity and number of gene families within the GM of TNs patients, alongside a decreased relative abundance of butyrate-producing microbes, a finding especially pronounced in patients with advanced TNs (21). The diminished expression of NIS mRNA characterizes benign nodules, suggesting an epigenetic pattern intermediate between normal thyroid tissue and malignant TNs (38). The administration of sodium butyrate, a byproduct of butyrate, induces demethylation, facilitating the restoration of normal epigenetic expression, and highlights the critical role of butyrate fluctuations in TNs severity. An increased presence of the genus Ruminococcaceae_NK4A214_group enhances butyrate production, potentially slowing the progression to advanced TNs stages. This study identified a negative causal relationship between Ruminococcaceae_NK4A214_group and TNs, suggesting the Ruminococcaceae family may mitigate TNs progression through regulatory mechanisms of butyrate production, exerting anti-inflammatory effects, modulating immune responses, and restoring epigenetic expressions. Conversely, this investigation identifies a negative causal relationship between the Lachnospiraceae and TNs risk. Bacteria within the Lachnospiraceae family are essential for maintaining gut health and the balance of the host immune system by fermenting dietary fibers to produce short-chain fatty acids (SCFAs), including butyrate, affecting the host’s digestion and metabolism (39). SCFAs not only serve as an energy source but also regulate hormone secretion, such as insulin. Alterations in Lachnospiraceae have been associated to the development of obesity and type 2 diabetes—both recognized risk factors for TNs (40, 41). Hence, a reduction in Lachnospiraceae bacteria leads to GM imbalance, notably a reduction in butyrate-producing bacteria, impacting the metabolic and immune regulation of the thyroid-gut axis, corroborating the findings of Ang Li et al. (21).
Senegalimassilia, characterized as a class of anaerobic probiotics involved in cellulose breakdown, has a significant impact on maintaining gut health and immune system balance. The prevalence of Senegalimassilia in the gut has been associated with the progression of various conditions, including non-alcoholic fatty liver disease, bladder cancer, pancreatic cancer, and polycystic ovary syndrome (42–45). Research indicates that metformin treatment enhances the abundance of Senegalimassilia in the guts of young, healthy males, correlating with decreased thyroid volumes and reduced risks of thyroid enlargement, TNs, and cancer in treated patients (46). The increase in Senegalimassilia may confer a protective effect on the thyroid via the thyroid-intestine axis, warranting further investigation into this intricate relationship.
Desulfovibrionales, classified within the δ-Proteobacteria class, are implicated in an array of conditions, including psychiatric and gastrointestinal disorders. Research has demonstrated that Desulfovibrionales foster cholesterol gallstone formation by augmenting secondary bile acid production in the cecum, which increases the hydrophobicity of bile acids and regulating the absorption and secretion of intestinal cholesterol (47). The interconnection between thyroid disorders, including thyroid nodules (TNs), and cholelithiasis is established; thyroid pathologies are significant risk factors for gallstones (48), with the influence of thyroid hormones on nuclear factors and cholesterol transport proteins playing a critical role in cholesterol homeostasis and the progression of cholelithiasis (49). Intestinal epithelial cells, capable of responding to signals from pathogenic and commensal bacteria such as secondary bile acids, relay these signals to gut-resident innate immune cells (50). This process promotes the translocation of bacteria and their metabolites through the intestinal barrier into the bloodstream, triggering persistent inflammatory stimulation and initiating immune responses. Chronic inflammation plays a crucial role in TNs, with elevated leukocyte counts serving as independent predictors for TNs. It is proposed that the type 2 immune response, marked by an increase in eosinophil percentages, is associated with TNs presence (51). Our results indicate a positive causal relationship between Desulfovibrionales and TNs, suggesting that enrichment of Desulfovibrionales may provoke inflammatory responses by altering nutrient metabolism, thus intensifying immune reactions and increasing TNs risk.
Prevotella, an anaerobic Gram-negative genus within the order Bacteroidales and family Prevotellaceae, encompasses Prevotella_7, a dominant intestinal bacterium widespread in the human gut. Evidence indicates that a low proportion of Prevotella may beneficially affect gut microbial biodiversity balance (52). Prevotella enrichment has been observed to disrupt the Th1/Th2 cytokine equilibrium, elevating cytokine levels such as TNF-α and IFN-γ, potentially causing thyroid damage (53). Additionally, there is considerable evidence of parallels between oral microbiota and GM (54, 55). Investigations into oral microbiota have shown that unclassified Prevotellaceae may accelerate TNs progression, with increased Alloprevotella abundance in saliva linked to thyroid cancer; Alloprevotella belongs to the Prevotella genus (56). Analyses of GM in TC patients also identified significant dysbiosis in Prevotellaceae and Prevotella_9 among others (57). Our study posits a positive causal association between Prevotella_7 and TNs, potentially due to Prevotella_7 enrichment compromising intestinal barrier integrity, exacerbating intestinal inflammation, and affecting signaling pathways involved in systemic immune responses, thereby influencing thyroid health (58–60).
Deltaproteobacteria are a group of Gram-negative Proteobacteria consisting of obligate anaerobes, including several sulfate-reducing and sulfur-reducing bacteria (61). Although generally present in low abundance in healthy individuals, they may play significant roles in specific pathological conditions. A strong causal relationship between Deltaproteobacteria and thyroid function, particularly hyperthyroidism, has been established, with studies identifying Deltaproteobacteria as a risk factor for Graves’ disease (62); Cao J et al. highlighted Deltaproteobacteria as a potential risk factor (63). Desulfovibrionaceae, within the Deltaproteobacteria class, decomposes sulfur-containing compounds in the gastrointestinal tract into hydrogen sulfide. At elevated concentrations, hydrogen sulfide can harm intestinal epithelial cells, induce DNA damage, and exert pro-inflammatory effects, correlating with various diseases (64). Overgrowth of Desulfovibrionaceae has been associated with colitis and colorectal cancer (65). Furthermore, Desulfovibrionaceae impact hormone metabolism, including sex and thyroid hormones. Shen H et al. observed that iodine supplementation resulted in decreased serum thyroid hormone levels in female mice, with significant GM composition shifts, notably an increase in Desulfovibrionaceae, suggesting a higher thyroid disease risk in females associated with Desulfovibrionaceae proliferation (66). The absorption of essential thyroid minerals, such as iodine and selenium, is closely linked to Deltaproteobacteria and Desulfovibrionaceae, influencing TNs development. Our research indicates a positive causal link between Deltaproteobacteria, Desulfovibrionaceae, and TNs, suggesting their enrichment may elevate TNs risk, potentially alongside thyroid function disturbances.
Faecalibacterium, recognized for its probiotic properties, plays a important ecological role in the human gut. Its functions include the synthesis of anti-inflammatory metabolites such as butyrate and salicylate, safeguarding the digestive tract from pathogenic threats (67). In patients with Hashimoto’s thyroiditis, a decline in Faecalibacterium prausnitzii abundance has been noted with an inverse correlation between Faecalibacterium’s relative abundance and thyroid autoantibodies or the FT3 to FT4 ratio, underscoring a close association with thyroid hormones and their functionality (21, 68, 69). Nonetheless, our findings suggest a positive correlation between Faecalibacterium and TNs incidence, raising queries about whether Faecalibacterium overproliferation leading to gut microbiota dysbiosis is implicated, necessitating further investigation.
In essence, the prevalence of various GM in patients with TNs alters as the disease progresses, and reciprocally, the GM can influence the onset and development of TNs through mechanisms such as regulation of glycolipid metabolism, mitigation of intestinal inflammation, participation in immune responses, modulation of the intestinal barrier, and provision of gut nutrition.
However, this study is not devoid of limitations. Primarily, the GWAS statistical data were derived solely from European populations, potentially limiting the generalizability of our findings. Additionally, the application of stringent p-value thresholds (p< 1×10-5) might have introduced potential false-positive associations. Moreover, the lack of individual-level data from the GWAS prevented detailed patient stratification analysis, restricting the exploration of interactions between the GM and various TNs patient stratifications. Despite these limitations, this study, through comprehensive MR analyses, provides supportive evidence for a causal relationship between the GM and TNs, emphasizing the importance of the thyroid-gut axis. These insights pave the way for future studies in broader populations, potentially heralding novel diagnostic and therapeutic interventions.
5 Conclusion
In this study, the causal relationship between the GM and TNs were evaluated using univariate and multivariate MR analyses. Our findings suggest that certain microbiota, identified as Ruminococcaceae_NK4A214_group, Senegalimassilia, Lachnospiraceae, exhibit a protective influence against TNs’ development, indicated by negative causal associations. In contrast, microbiota categorized as Desulfovibrionales, Prevotella_7, Faecalibacterium, Desulfovibrionaceae, Deltaproteobacteria are positively associated with TNs, suggesting they may serve as risk factors. Reverse MR analyses did not establish significant causal links. After comprehensive adjustment for confounders, taxa Desulfovibrionales (Order), Desulfovibrionaceae (Family), Deltaproteobacteria (Class) remain implicated as potential contributors to TNs’ risk. Future studies are warranted to further substantiate the GM-TNs causal relationship, elucidate underlying mechanisms, and enrich preventive and therapeutic strategies in clinical settings.
Data availability statement
The original contributions presented in the study are included in the article/Supplementary Material. Further inquiries can be directed to the corresponding authors.
Author contributions
SY: Writing – original draft, Writing – review & editing. JH: Writing – original draft, Writing – review & editing. XY: Writing – original draft, Writing – review & editing. JS: Writing – original draft, Writing – review & editing. YZ: Writing – original draft, Writing – review & editing. HB: Writing – original draft, Writing – review & editing. XZ: Writing – original draft, Writing – review & editing. XX: Writing – original draft, Writing – review & editing. LL: Writing – original draft, Writing – review & editing.
Funding
The author(s) declare financial support was received for the research, authorship, and/or publication of this article. This study received funding from Beijing Traditional Chinese Medicine “Torch Inheritance 3 + 3 Project”-the Wang Pei Famous Doctor Inheritance Workstation - Dongzhimen Hospital Branch (NO.4051206-02).
Acknowledgments
We extend our appreciation to the researchers who graciously supplied the GWAS data.
Conflict of interest
The authors declare that the research was conducted in the absence of any commercial or financial relationships that could be construed as a potential conflict of interest.
Publisher’s note
All claims expressed in this article are solely those of the authors and do not necessarily represent those of their affiliated organizations, or those of the publisher, the editors and the reviewers. Any product that may be evaluated in this article, or claim that may be made by its manufacturer, is not guaranteed or endorsed by the publisher.
Supplementary material
The Supplementary Material for this article can be found online at: https://www.frontiersin.org/articles/10.3389/fendo.2024.1417009/full#supplementary-material
Abbreviations
GM, gut microbiota; TNs, thyroid nodules; TC, thyroid cancer; MR, Mendelian randomization; SNP, Single-nucleotide polymorphisms; IVs, Instrumental variables; IVW, Inverse variance weighted; MR-PRESSO, MR pleiotropy residual um and outlier; OR, Odds ratio; GWAS, Genome-wide association study.
References
1. Sung H, Ferlay J, Siegel RL, Laversanne M, Soerjomataram I, Jemal A, et al. Global cancer statistics 2020: GLOBOCAN estimates of incidence and mortality worldwide for 36 cancers in 185 countries. CA Cancer J Clin. (2021) 71:209–49. doi: 10.3322/caac.21660
2. Jiang H, Tian Y, Yan W, Kong Y, Wang H, Wang A, et al. The prevalence of thyroid nodules and an analysis of related lifestyle factors in Beijing communities. Int J Environ Res Public Health. (2016) 13:442. doi: 10.3390/ijerph13040442
3. Popoveniuc G, Jonklaas J. Thyroid nodules. Med Clin North Am. (2012) 96:329–49. doi: 10.1016/j.mcna.2012.02.002
4. Uppal N, Collins R, James B. Thyroid nodules: Global, economic, and personal burdens. Front Endocrinol (Lausanne). (2023) 14:1113977. doi: 10.3389/fendo.2023.1113977
5. Yan Y, Dong J, Li S, Yang G, Huang K, Tian W, et al. Risk factors associated with the prevalence of thyroid nodules in adults in Northeast China: a cross-sectional population-based study. BMJ Open. (2023) 13:e069390. doi: 10.1136/bmjopen-2022-069390
6. Ahmadi S, Pappa T, Kang AS, Coleman AK, Landa I, Marqusee E, et al. Point of care measurement of body mass index and thyroid nodule Malignancy risk assessment. Front Endocrinol (Lausanne). (2022) 13:824226. doi: 10.3389/fendo.2022.824226
7. Lozupone CA, Stombaugh JI, Gordon JI, Jansson JK, Knight R. Diversity, stability and resilience of the human gut microbiota. Nature. (2012) 489:220–30. doi: 10.1038/nature11550
8. Adak A, Khan MR. An insight into gut microbiota and its functionalities. Cell Mol Life Sci. (2019) 76:473–93. doi: 10.1007/s00018-018-2943-4
9. Fung TC, Olson CA, Hsiao EY. Interactions between the microbiota, immune and nervous systems in health and disease. Nat Neurosci. (2017) 20:145–55. doi: 10.1038/nn.4476
10. Tang WH, Kitai T, Hazen SL. Gut microbiota in cardiovascular health and disease. Circ Res. (2017) 120:1183–96. doi: 10.1161/CIRCRESAHA.117.309715
11. Budden KF, Gellatly SL, Wood DL, Cooper MA, Morrison M, Hugenholtz P, et al. Emerging pathogenic links between microbiota and the gut-lung axis. Nat Rev Microbiol. (2017) 15:55–63. doi: 10.1038/nrmicro.2016.142
12. Knezevic J, Starchl C, Tmava Berisha A, Amrein K. Thyroid-gut-axis: how does the microbiota influence thyroid function? Nutrients. (2020) 12:1769. doi: 10.3390/nu12061769
13. Ishaq HM, Mohammad IS, Guo H, Shahzad M, Hou YJ, Ma C, et al. Molecular estimation of alteration in intestinal microbial composition in Hashimoto's thyroiditis patients. BioMed Pharmacother. (2017) 95:865–74. doi: 10.1016/j.biopha.2017.08.101
14. Virili C, Fallahi P, Antonelli A, Benvenga S, Centanni M. Gut microbiota and Hashimoto's thyroiditis. Rev Endocr Metab Disord. (2018) 19:293–300. doi: 10.1007/s11154-018-9467-y
15. Yu X, Jiang W, Kosik RO, Song Y, Luo Q, Qiao T, et al. Gut microbiota changes and its potential relations with thyroid carcinoma. J Adv Res. (2021) 35:61–70. doi: 10.1016/j.jare.2021.04.001
16. Hou J, Tang Y, Chen Y, Chen D. The role of the microbiota in graves' Disease and graves' Orbitopathy. Front Cell Infect Microbiol. (2021) 11:739707. doi: 10.3389/fcimb.2021.739707
17. Su X, Zhao Y, Li Y, Ma S, Wang Z. Gut dysbiosis is associated with primary hypothyroidism with interaction on gut-thyroid axis. Clin Sci (Lond). (2020) 134:1521–35. doi: 10.1042/CS20200475
18. Fröhlich E, Wahl R. Microbiota and thyroid interaction in health and disease. Trends Endocrinol Metab. (2019) 30:479–90. doi: 10.1016/j.tem.2019.05.008
19. Shen H, Han J, Li Y, Lu C, Zhou J, Li Y, et al. Different host-specific responses in thyroid function and gut microbiota modulation between diet-induced obese and normal mice given the same dose of iodine. Appl Microbiol Biotechnol. (2019) 103:3537–47. doi: 10.1007/s00253-019-09687-1
20. Shi N, Li N, Duan X, Niu H. Interaction between the gut microbiome and mucosal immune system. Mil Med Res. (2017) 4:14. doi: 10.1186/s40779-017-0122-9
21. Li A, Li T, Gao X, Yan H, Chen J, Huang M, et al. Gut microbiome alterations inpatients with thyroid nodules. Front Cell Infect Microbiol. (2021) 11:643968. doi: 10.3389/fcimb.2021.643968
22. Greenberg VL, Williams JM, Boghaert E, Mendenhall M, Ain KB, Zimmer SG. Butyrate alters the expression and activity of cell cycle components in anaplastic thyroid carcinoma cells. Thyroid. (2001) 11:21–9. doi: 10.1089/10507250150500621
23. Sanderson E, Glymour MM, Holmes MV, Kang H, Morrison J, Munafò MR, et al. Mendelian randomization. Nat Rev Methods Primers. (2022) 2:6. doi: 10.1038/s43586-021-00092-5
24. Emdin CA, Khera AV, Kathiresan S. Mendelian randomization. JAMA. (2017) 318:1925–6. doi: 10.1001/jama.2017.17219
25. Davies NM, Holmes MV, Davey Smith G. Reading Mendelian randomisation studies: a guide, glossary, and checklist for clinicians. BMJ. (2018) 362:k601. doi: 10.1136/bmj.k601
26. Skrivankova VW, Richmond RC, Woolf BAR, Yarmolinsky J, Davies NM, Swanson SA, et al. Strengthening the reporting of observational studies in epidemiology using mendelian randomization: The STROBE-MR statement. JAMA. (2021) 326:1614–21. doi: 10.1001/jama.2021.18236
27. Kurilshikov A, Medina-Gomez C, Bacigalupe R, Radjabzadeh D, Wang J, Demirkan A, et al. Large-scale association analyses identify host factors influencing human gut microbiome composition. Nat Genet. (2021) 53:156–65. doi: 10.1038/s41588-020-00763-1
28. Liu X, Qi X, Han R, Mao T, Tian Z. Gut microbiota causally affects cholelithiasis: a two-sample Mendelian randomization study. Front Cell Infect Microbiol. (2023) 13:1253447. doi: 10.3389/fcimb.2023.1253447
29. Sanna S, van Zuydam NR, Mahajan A, Kurilshikov A, Vich Vila A, Võsa U, et al. Causal relationships among the gut microbiome, short-chain fatty acids and metabolic diseases. Nat Genet. (2019) 51:600–5. doi: 10.1038/s41588-019-0350-x
30. Li P, Wang H, Guo L, Gou X, Chen G, Lin D, et al. Association between gut microbiota and preeclampsia-eclampsia: a two-sample Mendelian randomization study. BMC Med. (2022) 20:443. doi: 10.1186/s12916-022-02657-x
31. Choi KW, Chen CY, Stein MB, Klimentidis YC, Wang MJ, Koenen KC, et al. Assessment of Bidirectional Relationships Between Physical Activity and Depression Among Adults: A 2-Sample Mendelian Randomization Study (published correction appears in JAMA Psychiatry. 2023 Oct 1;80(10):1078). JAMA Psychiatry. (2019) 76:399–408. doi: 10.1001/jamapsychiatry.2018.4175
32. Yin KJ, Huang JX, Wang P, Yang XK, Tao SS, Li HM, et al. No genetic causal association between periodontitis and arthritis: A bidirectional two-sample mendelian randomization analysis. Front Immunol. (2022) 13:808832. doi: 10.3389/fimmu.2022.808832
33. Boehm FJ, Zhou X. Statistical methods for Mendelian randomization in genome-wide association studies: A review. Comput Struct Biotechnol J. (2022) 20:2338–51. doi: 10.1016/j.csbj.2022.05.015
34. Chen M, Peng WY, Tang TC, Zheng H. Differential sleep traits have no causal effect on inflammatory bowel diseases: A mendelian randomization study. Front Pharmacol. (2021) 12:763649. doi: 10.3389/fphar.2021.763649
35. Gao Y, Fan ZR, Shi FY. Hypothyroidism and rheumatoid arthritis: a two-sample Mendelian randomization study. Front Endocrinol (Lausanne). (2023) 14:1179656. doi: 10.3389/fendo.2023.1179656
36. Di J, Ge Z, Xie Q, Kong D, Liu S, Wang P, et al. Helicobacter pylori infection increases the risk of thyroid nodules in adults of Northwest China. Front Cell Infect Microbiol. (2023) 13:1134520. doi: 10.3389/fcimb.2023.1134520
37. Wang L, Liao Y, Yang R, Zhu Z, Zhang L, Wu Z, et al. An engineered probiotic secreting Sj16 ameliorates colitis via Ruminococcaceae/butyrate/retinoic acid axis. Bioeng Transl Med. (2021) 6:e10219. doi: 10.1002/btm2.10219
38. Yim JH, Choi AH, Li AX, Qin H, Chang S, Tong ST, et al. Identification of tissue-specific DNA methylation signatures for thyroid nodule diagnostics. Clin Cancer Res. (2019) 25:544–51. doi: 10.1158/1078-0432.CCR-18-0841
39. Yang YH, Chen C, Zheng Y, Wu ZJ, Zhou MQ, Liu XY, et al. Fucoxanthin alleviates dextran sulfate sodium-induced colitis and gut microbiota dysbiosis in mice. J Agric Food Chem. (2024) 72:4142–54. doi: 10.1021/acs.jafc.3c08811
40. Zeng L, Ma J, Wei T, Wang H, Yang G, Han C, et al. The effect of canagliflozin on gut microbiota and metabolites in type 2 diabetic mice. Genes Genomics. (2024) 46(5):541–55. doi: 10.1007/s13258-024-01491-0
41. Yamamoto M, Ogura H, Kuda T, Xia Y, Nakamura A, Takahashi H, et al. Detection of typical indigenous gut bacteria related to kanpyo Lagenaria siceraria var. hispida powder in murine caecum and human faecal cultures. 3 Biotech. (2024) 14:118. doi: 10.1007/s13205-024-03960-5
42. Dai W, Cai D, Zhou S, Li A, Xie J, Zhang J. Uncovering a causal connection between the Lachnoclostridium genus in fecal microbiota and non-alcoholic fatty liver disease: a two-sample Mendelian randomization analysis. Front Microbiol. (2023) 14:1276790. doi: 10.3389/fmicb.2023.1276790
43. Yin Z, Liu B, Feng S, He Y, Tang C, Chen P, et al. A large genetic causal analysis of the gut microbiota and urological cancers: A bidirectional mendelian randomization study. Nutrients. (2023) 15:4086. doi: 10.3390/nu15184086
44. Jiang Z, Mou Y, Wang H, Li L, Jin T, Wang H, et al. Causal effect between gut microbiota and pancreatic cancer: a two-sample Mendelian randomization study. BMC Cancer. (2023) 23:1091. doi: 10.1186/s12885-023-11493-y
45. Garcia-Beltran C, Malpique R, Carbonetto B, González-Torres P, Henares D, Brotons P, et al. Gut microbiota in adolescent girls with polycystic ovary syndrome: Effects of randomized treatments. Pediatr Obes. (2021) 16:e12734. doi: 10.1111/ijpo.12734
46. Meng X, Xu S, Chen G, Derwahl M, Liu C. Metformin and thyroid disease. J Endocrinol. (2017) 233:R43–51. doi: 10.1530/JOE-16-0450
47. Hu H, Shao W, Liu Q, Liu N, Wang Q, Xu J, et al. Gut microbiota promotes cholesterol gallstone formation by modulating bile acid composition and biliary cholesterol secretion. Nat Commun. (2022) 13:252. doi: 10.1038/s41467-021-27758-8
48. Song ST, Shi J, Wang XH, Guo YB, Hu PF, Zhu F, et al. Prevalence and risk factors for gallstone disease: A population-based cross-sectional study. J Dig Dis. (2020) 21:237–45. doi: 10.1111/1751-2980.12857
49. Ravi PC, Thugu TR, Singh J, Dasireddy RR, Kumar SA, Isaac NV, et al. Gallstone disease and its correlation with thyroid disorders: A narrative review. Cureus. (2023) 15:e45116. doi: 10.7759/cureus.45116
50. Kayama H, Okumura R, Takeda K. Interaction between the microbiota, epithelia, and immune cells in the intestine. Annu Rev Immunol. (2020) 26:23–48. doi: 10.1146/annurev-immunol-070119-115104
51. Wang S, Wang X, Hua X, Jiang S, Xie Y, Liu H. Adjusted association between type 2 immunity and low risk thyroid nodules: a retrospective cohort study. BMC Endocr Disord. (2022) 22:2. doi: 10.1186/s12902-021-00917-0
52. Liang Y, Yu W, Wang H, Yao L, He Z, Sun M, et al. Flash extraction of ulvan polysaccharides from marine green macroalga Ulva linza and evaluation of its antioxidant and gut microbiota modulation activities. Int J Biol Macromol. (2024) 262:130174. doi: 10.1016/j.ijbiomac.2024.130174
53. Wu B, Xu Y, Ban Y, Zhang M, Sun Z, Cai Y, et al. Correlation between the intestinal microflora and peripheral blood Th1/Th2 balance in hypothyroidism during the first half of pregnancy. Front Cell Infect Microbiol. (2023) 13:1159238. doi: 10.3389/fcimb.2023.1159238
54. Maki KA, Kazmi N, Barb JJ, Ames N. The oral and gut bacterial microbiomes: Similarities, differences, and connections. Biol Res Nurs. (2021) 23:7–20. doi: 10.1177/1099800420941606
55. Campisciano G, Zanotta N, Quadrifoglio M, Careri A, Torresani A, Cason C, et al. The bacterial DNA profiling of chorionic villi and amniotic fluids reveals overlaps with maternal oral, vaginal, and gut microbiomes. Int J Mol Sci. (2023) 24:2873. doi: 10.3390/ijms24032873
56. Jiao J, Zheng Y, Zhang Q, Xia D, Zhang L, Ma N. Saliva microbiome changes in thyroid cancer and thyroid nodules patients. Front Cell Infect Microbiol. (2022) 12:989188. doi: 10.3389/fcimb.2022.989188
57. Ishaq HM, Mohammad IS, Hussain R, Parveen R, Shirazi JH, Fan Y, et al. Gut-Thyroid axis: How gut microbial dysbiosis associated with euthyroid thyroid cancer. J Cancer. (2022) 13:2014–28. doi: 10.7150/jca.66816
58. Sun L, Jia H, Li J, Yu M, Yang Y, Tian D, et al. Cecal gut microbiota and metabolites might contribute to the severity of acute myocardial ischemia by impacting the intestinal permeability, oxidative stress, and energy metabolism. Front Microbiol. (2019) 10:1745. doi: 10.3389/fmicb.2019.01745
59. Feng W, Yang Z, Liu Y, Chen R, Song Z, Pan G, et al. Gut microbiota: A new target of traditional Chinese medicine for insomnia. BioMed Pharmacother. (2023) 160:114344. doi: 10.1016/j.biopha.2023.114344
60. Chen Y, Liu Y, Wang Y, Chen X, Wang C, Chen X, et al. Prevotellaceae produces butyrate to alleviate PD-1/PD-L1 inhibitor-related cardiotoxicity via PPARα-CYP4X1 axis in colonic macrophages. J Exp Clin Cancer Res. (2022) 41:1. doi: 10.1186/s13046-021-02201-4
61. Totzeck AA-O, Ramakrishnan E, Schlag M, Stolte B, Kizina K, Bolz S, et al. Gut bacterial microbiota in patients with myasthenia gravis: results from the MYBIOM study. Ther Adv Neurol Disord. (2021) 14:17562864211035657. doi: 10.1177/17562864211035657
62. Xie L, Zhao H, Chen W. Relationship between gut microbiota and thyroid function: a two-sample Mendelian randomization study. Front Endocrinol (Lausanne). (2023) 14:1240752. doi: 10.3389/fendo.2023.1240752
63. Cao J, Wang N, Luo Y, Ma C, Chen Z, Chenzhao C, et al. A cause-effect relationship between Graves' disease and the gut microbiome contributes to the thyroid-gut axis: A bidirectional two-sample Mendelian randomization study. Front Immunol. (2023) 14:977587. doi: 10.3389/fimmu.2023.977587
64. Ma F, Sun M, Song Y, Wang A, Jiang S, Qian F, et al. Lactiplantibacillus plantarum-12 Alleviates Inflammation and Colon Cancer Symptoms in AOM/DSS-Treated Mice through Modulating the Intestinal Microbiome and Metabolome. Nutrients. (2022) 14:1916. doi: 10.3390/nu14091916
65. Millien V, Rosen D, Hou J, Shah R. Proinflammatory sulfur-reducing bacteria are more abundant in colonic biopsies of patients with microscopic colitis compared to healthy controls. Dig. Dis Sci. (2019) 64:432–8. doi: 10.1007/s10620-018-5313-z
66. Shen H, Xu J, Lu C, Han J, Zhou J, Ming T, et al. Effects of the sex factor on mouse iodine intake: Interactions between the gut microbiota composition and metabolic syndromes. ACS Omega. (2021) 6:28569–78. doi: 10.1021/acsomega.1c02697
67. Martín R, Rios-Covian D, Huillet E, Auger S, Khazaal S, Bermúdez-Humarán LG, et al. Faecalibacterium: a bacterial genus with promising human health applications. FEMS Microbiol Rev. (2023) 47:fuad039. doi: 10.1093/femsre/fuad039
68. Gong B, Wang C, Meng F, Wang H, Song B, Yang Y, et al. Association between gut microbiota and autoimmune thyroid disease: A systematic review and meta-analysis. Front Endocrinol (Lausanne). (2021) 12:774362. doi: 10.3389/fendo.2021.774362
Keywords: gut microbiota, thyroid nodules, Mendelian randomization, causal association, thyroid-gut axis
Citation: Yan S, He J, Yu X, Shang J, Zhang Y, Bai H, Zhu X, Xie X and Lee L (2024) Causal relationship between gut microbiota and thyroid nodules: a bidirectional two-sample Mendelian randomization study. Front. Endocrinol. 15:1417009. doi: 10.3389/fendo.2024.1417009
Received: 13 April 2024; Accepted: 10 July 2024;
Published: 08 August 2024.
Edited by:
Cristina Alina Silaghi, University of Medicine and Pharmacy Iuliu Hatieganu, RomaniaCopyright © 2024 Yan, He, Yu, Shang, Zhang, Bai, Zhu, Xie and Lee. This is an open-access article distributed under the terms of the Creative Commons Attribution License (CC BY). The use, distribution or reproduction in other forums is permitted, provided the original author(s) and the copyright owner(s) are credited and that the original publication in this journal is cited, in accordance with accepted academic practice. No use, distribution or reproduction is permitted which does not comply with these terms.
*Correspondence: Jianwei Shang, c2p3MzEyNEAxNjMuY29t; Yaosheng Zhang, emhhbmd5YW9zaGVuZ0BidWNtLmVkdS5jbg==
†These authors share first authorship