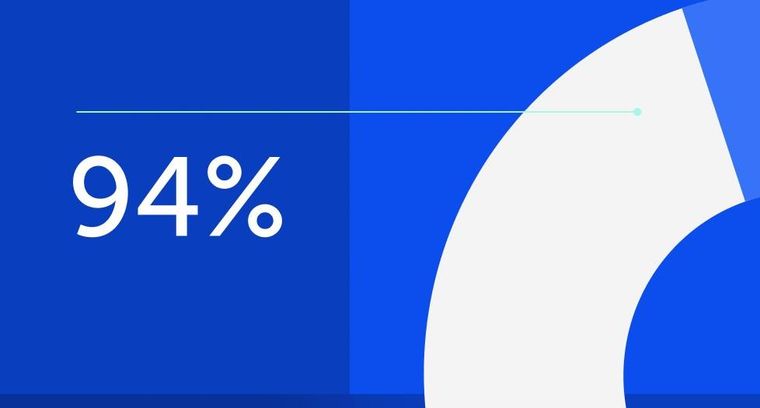
94% of researchers rate our articles as excellent or good
Learn more about the work of our research integrity team to safeguard the quality of each article we publish.
Find out more
ORIGINAL RESEARCH article
Front. Endocrinol., 02 October 2024
Sec. Bone Research
Volume 15 - 2024 | https://doi.org/10.3389/fendo.2024.1412872
This article is part of the Research TopicThe Endocrine Role of the Musculoskeletal SystemView all 11 articles
Introduction: Previous studies have indicated that exposure to heavy metals related to bone health is primarily limited to some common harmful metals, and the impact of lead has not been fully understood. This study aims to explore the relationship between urine lead exposure and bone density.
Methods: 1,310 adults were included from the NHANES database (2015-2018), and through generalized linear regression analysis and constrained cubic spline models, the association between lead levels and total bone density as well as lumbar spine bone density was explored. The study also examined the impact of combined exposure to lead and cadmium on bone density.
Results and conclusions: Urinary lead levels were significantly negatively correlated with total bone mineral density (β: −0.015; 95%CI: −0.024, −0.007) and lumbar spine bone mineral density (β: −0.019; 95%CI: −0.031, −0.006). Compared to the lowest three quartiles of lead levels, the adjusted odds ratios for T3 changes in total bone mineral density and lumbar spine bone mineral density were 0.974 (95%CI: 0.959, 0.990) and 0.967 (95%CI: 0.943, 0.991), indicating a significant negative trend. Further analysis with constrained cubic spline models revealed a non-linear decreasing relationship between urinary lead and total bone mineral density as well as lumbar spine bone mineral density. Stratified analyses suggested that the relationship between urinary lead levels and bone mineral density might be significantly influenced by age, while gender showed no significant impact on the relationship. Moreover, combined exposure to lead and cadmium was found to be associated with decreased bone mineral density, emphasizing the potential synergistic effects between lead and cadmium on bone health. However, the specific mechanisms of lead and its effects on different populations require further comprehensive research. This study provides valuable insights for further exploration and development of relevant public health policies.
Osteoporosis is a metabolic bone disease characterized by changes in bone microstructure and decreased bone mineral density (BMD) (1). Bone density is an important indicator for evaluating bone health, with the two most common sites for measuring bone density being the whole body and the lumbar spine (2). Factors leading to decreased bone density include genetics (3), metabolism (4), and nutrition (5). According to recent evidence, decreased bone density may be related to environmental toxin exposure (6, 7), and heavy metals such as lead (Pb) may be associated with osteoporosis and related fractures (8, 9).
As a typical heavy metal, lead has received much attention due to its harmful effects on human health. The role of lead exposure in human biology is intricate and far-reaching, impacting not only the endocrine system by disrupting thyroid and sex hormone levels but also exerting significant effects on the nervous, immune systems, and reproductive health. As an endocrine-disrupting compound (EDC), lead binds to estrogen and androgen receptors, mimicking estrogenic effects and obstructing androgen actions, thus disturbing hormonal balance (10). This action is not only linked to thyroid dysfunction but also to reproductive health issues, particularly in males, where the association between lead exposure and infertility is increasingly evident. Lead exposure further damages reproductive capabilities through the induction of reactive oxygen species (ROS), cell apoptosis, local necrosis, immunosuppression, and mutagenic stimulation, negatively impacting the male reproductive system and potentially leading to azoospermia (11).
Moreover, lead exposure significantly impairs cognitive and behavioral development in children, correlating with decreased IQ, alterations in neurotransmitter levels, and reduced cognitive and behavioral scores (12–15). In terms of immune function, lead exposure is associated with altered levels of pro-inflammatory cytokines in children, potentially triggering a cascade of health issues spanning neurological, respiratory, cardiovascular, reproductive, and renal systems (16). More alarmingly, lead and its compounds are classified by the International Agency for Research on Cancer (IARC) as probable human carcinogens, indicating a potential cancer risk associated with long-term lead exposure (17). Although the organ toxicity of lead has been widely studied, research on the impact of lead on human bone health is limited.
More than 90% of lead in the human body is found in the bones (18). Lead has strong cytotoxicity, affecting osteoblasts, osteoclasts, and chondrocytes (19). An vitro studies have shown that lead can replace calcium in hydroxyapatite crystals and has a higher affinity for bone sialoprotein than calcium (20). Many animal studies have reported that lead exposure is associated with pathological processes in bone, resulting in decreased bone density and strength (21). In the United States, studies on elderly individuals have shown a significant negative correlation between blood lead levels and osteoporosis, particularly among Caucasian subjects (22). Furthermore, a study conducted in Taiwan found that adults, especially females, with higher urinary lead levels may have an increased risk of osteopenia and osteoporosis (23). Other studies have indicated that lead exposure is associated with femoral and spinal bone density in premenopausal women in the United States (24, 25), and lead and manganese exposure have been found to have a synergistic effect on bone density (8). The toxic effects of lead on bone density in different bone sites vary among children and adolescents, and there are differences in various age groups, genders, and levels of exposure (26). Overall, the research on the effects of normal lead exposure on bone density in adults is still limited, and further systematic studies are needed to obtain accurate conclusions.Therefore, we utilized data from the 2015-2018 National Health and Nutrition Examination Survey (NHANES) database to investigate the correlation between urinary lead levels and bone density in a representative sample of adults aged 20 and above in the United States.
The research data is derived from the NHANES database and is a cross-sectional study. All analyses were performed under logarithmic transformation and statistical analysis was conducted using multiplicative interaction models and generalized linear regression models. For other continuous variables, differences between groups were calculated using generalized linear regression models. Weighted chi-square tests were used for categorical variables.
The study selected information from the US NHANES database from January 2015 to December 2018, and the samples were taken from the general population of the United States.
The data for the study was derived from the US NHANES database. The NHANES database collects nutritional and health information from the general population of the United States and is a cross-sectional study. The NHANES database uses a large-scale, multi-stage complex sampling method, with non-repetitive sampling population, abundant sample size, and good representativeness. The study was approved by the Ethics Review Committee of the National Center for Health Statistics (NCHS), with ethics protocol numbers Continuation of Protocol #2011-17 (2013-2016) and Protocol #2018-01 (2017-2020), and written informed consent was obtained from each participant. The study subjects were selected from data spanning four years, from 2015 to 2018. Among the participants who underwent urinary metal testing from 2015 to 2018, a total of 6102 individuals were included. After excluding individuals with missing data on urine lead, bone density, renal insufficiency, or age less than 20 years (n=4565), 1537 participants were selected. Further exclusions were made for individuals with missing information on basic covariates including poverty-income ratio, body mass index, serum cotinine, and serum 25(OH)D (n=227), resulting in a final analytical sample of 1310 participants, as shown in Figure 1.
The selection of covariates is based on previous literature (27). The final covariates include age, gender (male and female), race/ethnicity (Mexican American, Other Hispanic, Non-Hispanic White, Non-Hispanic Black, Other), body mass index (kg/m2), poverty income ratio (<1, ≥1), education level (less than high school, high school or equivalent, higher than high school), serum cotinine (≥10ng/mL, 1-9.9ng/mL, and <1ng/mL), physical activity (<10 minutes/week and ≥10 minutes/week), serum 25(OH)D, thyroid disease (yes or no), diabetes (yes or no), and hypertension (yes or no).
Urine metal determination: Urine samples were collected and stored at -70°C, then transported to the National Center for Environmental Health for testing. Urine lead concentration was measured using inductively coupled plasma mass spectrometry (ICPMS). The laboratory procedures are described in detail on the NHANES website. The metals included in this study were detectable in over 99% of the participants. The metal levels in urine were calibrated with urine creatinine and expressed as μg/g creatinine.At a dedicated mobile examination center, total bone density and lumbar spine bone density were measured using the Hologic QDR 4500A fan-beam dual-energy X-ray absorptiometry (Hologic, Inc., Bedford, Massachusetts). For more detailed information on bone density assessment, please visit the NHANES website.
Due to skewed distributions, both bone density and urine lead concentration underwent natural logarithm (ln) transformation. Descriptive analysis was conducted on the participants’ basic demographic characteristics and bone density. Continuous variables were expressed as means and standard deviations (± s) or percentiles, while categorical variables were expressed as frequencies (proportions).
Generalized linear regression was used to evaluate the correlation between individual urine lead and bone density, treating each metal as a continuous exposure variable. The transformed regression coefficient represents the percentage change in bone density with a doubling of urine metal levels, using the following formula: (e(ln2 × β) - 1) × 100%. To further explore the relationship between urine lead and bone density, the generalized linear regression model treated urine lead concentration as tertiles. The percentage change in bone density associated with urine metal tertiles was estimated as (eOR - 1) × 100%. Restricted cubic splines (RCS) were used to assess the dose-response relationship between urine lead and bone density. The RCS model included three knots: the 25th, 50th, and 75th percentiles of the transformed metal concentration.
Stratified analysis by gender and age was conducted, followed by multiplicative interaction analysis. Generalized linear regression was used to further evaluate the combined effect of urine lead and urine cadmium exposure. Participants were divided into low exposure and high exposure groups based on the median levels of the metals. The group with low exposure to both metals was considered as the reference group. The percentage change in bone density for the exposed group was estimated as (eOR - 1) × 100%.
All analyses were performed using R (version 4.2.3), with statistical significance set at P<0.05 (two-tailed). Generalized linear regression analysis and RCS models were implemented using the “ggplot2” and “rms” packages, respectively.
The study included a total of 1310 subjects, with mean ages and body mass indexes of 39.5 ± 11.2 years and 29.0 ± 6.9 kg/m2 , respectively. Among the study population, 642 individuals (49.0%) were male, 777 individuals (59.3%) had received higher education (beyond high school), 388 individuals (29.6%) were non-Hispanic white, 1069 individuals (81.6%) were at or above the poverty line, 915 individuals (69.8%) were non-smokers, 86 individuals (6.6%) were informed of thyroid issues, 101 individuals (7.7%) had diabetes, and 275 individuals (21.0%) had hypertension. The median serum 25(OH)D, total bone density, and lumbar spine bone density were 56.7 (42.0, 73.7) nmol/L, 1.11 (1.04, 1.18) g/cm2, and 1.02 (0.93, 1.12) g/cm2, respectively. See Table 1 for details.
The geometric mean of urine lead concentration corrected for urinary creatinine was 3.918 μg/g creatinine. The median urine lead concentration was 2.826 μg/g creatinine, with an interquartile range (IQR) of 1.785-4.580 μg/g creatinine, and the standard deviation of urine lead was 4.0927 μg/g creatinine.
In the fully adjusted model, a significant negative correlation was observed between urinary lead levels and total bone density (β: -0.015; 95%CI: -0.024, -0.007) as well as lumbar spine bone density (β: -0.019; 95%CI: -0.031, -0.006). Furthermore, in the multivariable adjusted model, compared with the lowest tertile of lead level, the odds ratios (ORs) (95%CI) of total bone density at T2 and T3 levels were 0.997 (0.982, 1.011) and 0.974 (0.959, 0.990), respectively, when introducing the tertiles of urinary lead concentration. Similarly, compared with the lowest tertile of lead level, the ORs (95%CI) of lumbar spine bone density at T2 and T3 levels were 0.999 (0.978, 1.021) and 0.967 (0.943, 0.991) (Table 2), respectively. This indicates a significant negative correlation between total bone density and lumbar spine bone density at the highest tertile of urinary lead concentration. It is worth noting that the relationship between these bone density indicators and moderate levels of urinary lead concentration was not significant. This result emphasizes the different effects of urinary lead concentrations on bone density at different levels. A restricted cubic spline showed a nonlinear relationship between urinary lead and total bone density as well as lumbar spine bone density (Figure 2).
Figure 2. Restricted cubic spline regression of urinary lead with total bone density and lumbar spine bone density.
In a multivariable adjusted model, gender-stratified analysis revealed that the β values (95% CI) of urinary lead levels and total bone density in men and women were −0.012 (−0.025, 0.0004) and −0.021 (−0.033, −0.010), with corresponding P values of 0.059 and <0.001, respectively. For women, there was a significant negative correlation between urinary lead levels and total bone density, with a β value of −0.021, a 95% CI not including zero, and P value <0.001. However, in men, although the β value was negative, the P value did not reach significance. As for the relationship between urinary lead levels and lumbar bone density, the β values (95% CI) in men and women were −0.021 (−0.033, −0.010) and −0.022 (−0.040, −0.004), with P values of 0.089 and 0.015, respectively. Urinary lead levels were significantly negatively correlated with lumbar bone density in both men and women. However, in men, the P value was 0.089, which did not reach significance, while in women, the P value was 0.015, indicating a higher level of significance (Table 3).
Table 3. Interaction of sex and age on the relationship between urinary lead levels and total BMD and lumbar BMD.
Overall, the relationship between urinary lead levels and total bone density as well as lumbar bone density was significant in women and tended towards a negative correlation in men but was not significant. However, the overall bone Pint for gender stratification was 0.241, and the lumbar bone density Pint was 0.380, indicating no significant interactive effects, showing that gender had no significant impact on the relationship between urinary lead levels and bone density.
Similarly, in a multivariable adjusted model, stratified analysis based on the median age (39 years) revealed that the β values (95% CI) of urinary lead levels and total bone density in the low-age and high-age groups were −0.009 (−0.021, 0.003) and −0.023 (−0.035, −0.010), with corresponding P values of 0.138 and <0.001, respectively. Regarding total bone density, the relationship between urinary lead levels and bone density was not significant in the low-age group (P value = 0.138), while in the high-age group, there was a significant negative correlation between urinary lead levels and total bone density (P value <0.001). As for the relationship between urinary lead levels and lumbar bone density, the β values (95% CI) in the low-age and high-age groups were −0.006 (−0.022, 0.011) and −0.031 (−0.051, −0.012), with P values of 0.509 and 0.001, respectively. In terms of lumbar bone density, the relationship between urinary lead levels and bone density was similarly not significant in the low-age group (P value = 0.509), whereas there was a significant negative correlation between urinary lead levels and lumbar bone density in the high-age group (P value = 0.001).
Furthermore, the overall bone Pint for age stratification was 0.034, and the lumbar bone density Pint was 0.045. These data suggest that the relationship between urinary lead levels and bone density may be significantly influenced by age, and in the high-age group, there is a stronger correlation between increasing urinary lead levels and decreasing bone density.
Further evaluates the combined effects of lead and cadmium exposure on total bone density and lumbar spine bone density. The groups with low exposure levels to both metals were considered as the reference group. The odds ratios (OR) for total bone density in the low-cadmium high-lead exposure group, high-cadmium low-lead exposure group, and high-cadmium high-lead exposure group were 0.991 (0.976, 1.008), 1.002 (0.985, 1.020), and 0.984 (0.968, 1.001) respectively, with p-values of 0.337, 0.806, and 0.071. The odds ratios (OR) for lumbar spine bone density in the low-cadmium high-lead exposure group, high-cadmium low-lead exposure group, and high-cadmium high-lead exposure group were 0.989 (0.966, 1.014), 1.000 (0.975, 1.026), and 0.968 (0.945, 0.993) respectively, with p-values of 0.387, 0.999, and 0.012 (Table 4). Through the high-cadmium high-lead exposure group, it is demonstrated that the combined exposure to cadmium and lead has a negative impact on bone density, and this effect is statistically significant.
In this study, we investigated the relationship between urinary lead exposure and bone density during the NHANES survey period from 2015 to 2018. Overall, our study results showed that urinary lead exposure was associated with reduced total bone density and lumbar spine bone density. In stratified analysis, it was also found that urinary lead levels were correlated with decreased bone density, and the relationship between urinary lead levels and bone density may be significantly influenced by age with no significant gender effect observed. The combined effect of lead and cadmium was found to be related to decreased bone density.
The results of this study showed a negative correlation between urinary lead exposure levels and bone density. To our knowledge, this is the largest epidemiological research report on lead exposure and bone density across a wide age range. Lead is highly toxic and lead poisoning can cause damage to the nervous system and brain function. There have been few studies on the impact of lead on bone health. A report from Sweden found no association between adult lead exposure and bone density (28). The potential mechanism by which elevated lead levels cause bone disease is not clear. In vitro studies have shown that lead can exchange calcium in hydroxyapatite crystals, with higher affinity for osteocalcin than calcium (20), and inhibit the activation of vitamin D and dietary calcium absorption (29). However, more investigations are needed to validate our research results and elucidate the specific mechanisms behind the reduction of bone density with lead exposure.
Plasma lead concentration is difficult to accurately measure because of its low concentration and susceptibility to contamination (30). Whole blood lead levels are usually used as a biological marker of lead exposure because over 99% of lead is bound to red blood cells. However, due to the saturation effect of lead-binding sites in red blood cells, male blood lead levels are higher than female levels, while urinary lead levels show no significant difference between genders (24). Urinary lead is considered an alternative indicator reflecting plasma lead levels, as lead is mainly filtered through the glomerulus and excreted in urine. To accurately reflect urinary lead excretion, adjustments for urine dilution need to be considered. This study used urinary lead as a biological marker of lead exposure.
One finding in our study is the non-linear negative correlation between urinary lead levels in adults and total bone density and lumbar bone density. We found a stronger association between increasing urinary lead levels and decreasing bone density in the older age group (≥39 years old). This differs from previous studies in adults (8), but the reasons are still unclear. It could be due to variations in hormone levels at different ages. Further research is needed to investigate the differences in bone remodeling, absorption, and formation capabilities in different bone sites under various levels of lead exposure and in different age groups. We found that urinary lead concentration has different effects on bone density at different levels, and as urinary lead concentration increases, the decrease in bone density shows an initial rapid decline followed by a slower decline. The differences in lead’s impact on bone density may be attributed to the complex mechanisms of lead metabolism in bone at different levels. Previous studies have found that long-term lead exposure at low levels (blood levels <10μg/dL) inhibits the Wnt signaling pathway and leads to decreased bone density in adult rats, while increased bone mass has been observed in mice exposed to high levels of lead, which inhibits the ability of osteoclasts to reabsorb bone mass (31). This may suggest the need for greater attention to the effects of lead exposure on bone health in older age groups.
In our study, there was a significant relationship between urinary lead levels and total bone density and lumbar bone density in females, while in males, the correlation tended to be negative but not significant. In gender-stratified analyses, the P interaction values were 0.241 for total bone density and 0.380 for lumbar bone density, indicating that gender does not significantly affect the relationship between urinary lead levels and bone density. The negative association between lead exposure levels and bone density in adult women is consistent with previous studies, which found an association between lead exposure and decreased bone density in premenopausal women (32). However, another study of 50-70-year-old women also found a non-significant correlation (33). Even at low levels, lead can affect follicles in mice (34), which are the primary source of endogenous estrogen. Due to the decrease in estrogen, women experience rapid bone loss in the first 5-10 years after menopause (35). Therefore, we speculate that lead exposure induces a decrease in bone density by suddenly lowering estrogen levels. No association between lead concentration and bone density was observed in postmenopausal women because their ovaries no longer produce endogenous estrogen. The lack of significant correlation in males may be due to the fact that males have higher peak bone mass than females in early adulthood.
In this study, the combined exposure of cadmium and lead has a negative impact on bone density, and this impact is statistically significant, emphasizing a potential synergistic effect of these two metals on bone density. The production of reactive oxygen species induced oxidative stress is an important mechanism of lead and cadmium toxicity, which may be crucial for bone metabolism (36). In the real world, humans are simultaneously exposed to multiple heavy metals, which interact with each other. Further research is needed to validate this finding and explore its potential mechanisms in the future.
The relationship between urinary lead exposure and bone density is intricate, encompassing interactions with bone metabolism, endocrine regulation, oxidative stress, and interplays with age, gender, and metabolic status. When evaluating bone density risk, a holistic consideration of multiple risk factors, including urinary lead levels, elements of metabolic syndrome (e.g., abdominal obesity, hypertension, glucose abnormalities), and demographic factors, is essential. The potential interplay between urinary lead exposure and components of metabolic syndrome deserves attention (37). For example, certain aspects of metabolic syndrome may influence the body’s lead handling, affecting lead’s absorption, distribution, and excretion, and thus altering the lead burden in the body, which can have implications for bone density. Concurrently, lead exposure could exacerbate metabolic syndrome risks through its impacts on metabolic functions, such as insulin resistance and lipid metabolism, indirectly affecting bone health (38).
This study has several key strengths. First, it reports an epidemiological study of the maximum range of age groups with a negative correlation between lead exposure and bone density. Additionally, objectively measured urinary lead levels were used as a biomarker reflecting lead status. Furthermore, this study also conducted stratified analyses, yielding more stable results. However, the study also has some limitations. First, our study is a cross-sectional study, and further longitudinal research is needed to investigate more accurate causal relationships. Second, potential residual confounding factors such as genetics, diet, and other environmental chemicals were not fully considered. Lastly, during the continuous physiological process of bone remodeling, nearly 10% of bone is rebuilt each year (39), which this study cannot reflect in long-term bone health.
Our study has unveiled a nonlinear negative correlation between urinary lead exposure and bone density, along with variations in this association across different age and gender groups. This finding holds significant implications for clinical practice and public health interventions. At the clinical level, healthcare professionals should recognize lead exposure as a potential risk factor for osteoporosis, particularly in older women. Public health strategies should encompass educating the public on measures to reduce lead exposure, such as abstaining from lead-containing products, ensuring the safety of drinking water, and enforcing stricter regulations on industrial emissions, all aimed at safeguarding public bone health.
The original contributions presented in the study are included in the article/supplementary material. Further inquiries can be directed to the corresponding author.
All NHANES protocols were approved by the NCHS Ethics Review Board. The studies were conducted in accordance with the local legislation and institutional requirements. Written informed consent for participation was not required from the participants or the participants’ legal guardians/next of kin in accordance with the national legislation and institutional requirements.
SW: Writing – original draft. XZ: Writing – review & editing. RZ: Writing – review & editing. YJ: Writing – review & editing. XW: Writing – review & editing. XM: Writing – review & editing. XL: Writing – review & editing.
The author(s) declare financial support was received for the research, authorship, and/or publication of this article. The current research was funded by Shanxi Provincial Basic Research Program Plan Project (202203021221274).
The authors declare that the research was conducted in the absence of any commercial or financial relationships that could be construed as a potential conflict of interest.
All claims expressed in this article are solely those of the authors and do not necessarily represent those of their affiliated organizations, or those of the publisher, the editors and the reviewers. Any product that may be evaluated in this article, or claim that may be made by its manufacturer, is not guaranteed or endorsed by the publisher.
1. Hipps D, Dobson PF, Warren C, McDonald D, Fuller A, Filby A, et al. Detecting respiratory chain defects in osteoblasts from osteoarthritic patients using imaging mass cytometry. Bone. (2022) 158:116371. doi: 10.1016/j.bone.2022.116371
2. Medina-Gomez C, Kemp JP, Trajanoska K, Luan J, Chesi A, Ahluwalia TS, et al. Life-course genome-wide association study meta-analysis of total body BMD and assessment of age-specific effects. Am J Hum Genet. (2018) 102(1):88–102. doi: 10.1016/j.ajhg.2017.12.005
3. Hou R, Cole SA, Graff M, Haack K, Laston S, Comuzzie AG, et al. Genetic variants affecting bone mineral density and bone mineral content at multiple skeletal sites in Hispanic children. Bone. (2020) 132:115175. doi: 10.1016/j.bone.2019.115175
4. Lupsa BC, Insogna K. Bone health and osteoporosis. Endocrinol Metab Clin North Am. (2015) 44(3):517–30. doi: 10.1016/j.ecl.2015.05.002
5. Rizzoli R, Biver E, Brennan-Speranza TC. Nutritional intake and bone health. Lancet Diabetes Endocrinol. (2021) 9(9):606–21. doi: 10.1016/S2213-8587(21)00119-4
6. Gu L, Wang Z, Pan Y, Wang H, Sun L, Liu L, et al. Associations between mixed urinary phenols and parabens metabolites and bone mineral density: Four statistical models. Chemosphere. (2023) 311(Pt 2):137065. doi: 10.1016/j.chemosphere.2022.137065
7. Zhao X, Lin JY, Dong WW, Tang ML, Yan SG. Per- and polyfluoroalkyl substances exposure and bone mineral density in the U.S. population from NHANES 2005-2014. J Expo Sci Environ Epidemiol. (2023) 33(1):69–75. doi: 10.1038/s41370-022-00452-7
8. Wei MH, Cui Y, Zhou HL, Song WJ, Di DS, Zhang RY, et al. Associations of multiple metals with bone mineral density: A population-based study in US adults. Chemosphere. (2021) 282:131150. doi: 10.1016/j.chemosphere.2021.131150
9. Scimeca M, Feola M, Romano L, Rao C, Gasbarra E, Bonanno E, et al. Heavy metals accumulation affects bone microarchitecture in osteoporotic patients. Environ Toxicol. (2017) 32(4):1333–42. doi: 10.1002/tox.22327
10. Liu D, Shi Q, Liu C, Sun Q, Zeng X. Effects of endocrine-disrupting heavy metals on human health. Toxics. (2023) 11(4):322. doi: 10.3390/toxics11040322
11. Crocetto F, Risolo R, Colapietro R, Bellavita R, Barone B, Ballini A, et al. Heavy metal pollution and male fertility: an overview on adverse biological effects and socio-economic implications. Endocr Metab Immune Disord Drug Targets. (2023) 23(2):129–46. doi: 10.2174/1871530322666220627141651
12. Liu L, Zhang B, Lin K, Zhang Y, Xu X, Huo X. Thyroid disruption and reduced mental development in children from an informal e-waste recycling area: A mediation analysis. Chemosphere. (2018) 193:498–505. doi: 10.1016/j.chemosphere.2017.11.059
13. Zeng X, Xu C, Xu X, Zhang Y, Huang Y, Huo X. Elevated lead levels in relation to low serum neuropeptide Y and adverse behavioral effects in preschool children with e-waste exposure. Chemosphere. (2021) 269:129380. doi: 10.1016/j.chemosphere.2020.129380
14. Axelrad DA, Coffman E, Kirrane EF, Klemick H. The relationship between childhood blood lead levels below 5 µg/dL and childhood intelligence quotient (IQ): Protocol for a systematic review and meta-analysis. Environ Int. (2022) 169:107475. doi: 10.1016/j.envint.2022.107475
15. Santa Maria MP, Hill BD, Kline J. Lead (Pb) neurotoxicology and cognition. Appl Neuropsychol Child. (2019) 8(3):272–93. doi: 10.1080/21622965.2018.1428803
16. Zhang Y, Huo X, Lu X, Zeng Z, Faas MM, Xu X. Exposure to multiple heavy metals associate with aberrant immune homeostasis and inflammatory activation in preschool children. Chemosphere. (2020) 257:127257. doi: 10.1016/j.chemosphere.2020.127257
17. Pearce N, Blair A, Vineis P, Ahrens W, Andersen A, Anto JM, et al. IARC monographs: 40 years of evaluating carcinogenic hazards to humans. Environ Health Perspect. (2015) 123(6):507–14. doi: 10.1289/ehp.1409149
18. Li X, Li R, Yan J, Song Y, Huo J, Lan Z, et al. Co-exposure of cadmium and lead on bone health in a southwestern Chinese population aged 40-75 years. J Appl Toxicol. (2020) 40(3):352–62. doi: 10.1002/jat.3908
19. Liu XB, Gong ZL, Zhang Y, Zhang HD, Wang J, Tan HX, et al. A comparative study of blood lead levels in urban children in China: the China nutrition and health survey (CNHS) 2002 and 2012. BioMed Environ Sci. (2023) 36(4):376–80. doi: 10.3967/bes2023.044
20. Dowd TL, Rosen JF, Mints L, Gundberg CM. The effect of Pb(2+) on the structure and hydroxyapatite binding properties of osteocalcin. Biochim Biophys Acta. (2001) 1535(2):153–63. doi: 10.1016/s0925-4439(00)00094-6
21. Ronis MJ, Aronson J, Gao GG, Hogue W, Skinner RA, Badger TM, et al. Skeletal effects of developmental lead exposure in rats. Toxicol Sci. (2001) 62(2):321–9. doi: 10.1093/toxsci/62.2.321
22. Campbell JR, Auinger P. The association between blood lead levels and osteoporosis among adults–results from the third national health and nutrition examination survey (NHANES III). Environ Health Perspect. (2007) 115(7):1018–22. doi: 10.1289/ehp.9716
23. Tsai TL, Pan WH, Chung YT, Wu TN, Tseng YC, Liou SH, et al. Association between urinary lead and bone health in a general population from Taiwan. J Expo Sci Environ Epidemiol. (2016) 26(5):481–7. doi: 10.1038/jes.2015.30
24. Wang WJ, Wu CC, Jung WT, Lin CY. The associations among lead exposure, bone mineral density, and FRAX score: NHANES, 2013 to 2014. Bone. (2019) 128:115045. doi: 10.1016/j.bone.2019.115045
25. Lu J, Lan J, Li X, Zhu Z. Blood lead and cadmium levels are negatively associated with bone mineral density in young female adults. Arch Public Health. (2021) 79(1):116. doi: 10.1186/s13690-021-00636-x
26. Li T, Xie Y, Wang L, Huang G, Cheng Y, Hou D, et al. The association between lead exposure and bone mineral density in childhood and adolescence: results from NHANES 1999-2006 and 2011-2018. Nutrients. (2022) 14(7):1523. doi: 10.3390/nu14071523
27. Tang P, Liao Q, Huang H, Chen Q, Liang J, Tang Y, et al. Effects of urinary barium exposure on bone mineral density in general population. Environ Sci pollut Res Int. (2023) 30(48):106038–46. doi: 10.1007/s11356-023-29791-0
28. Alfvén T, Järup L, Elinder CG. Cadmium and lead in blood in relation to low bone mineral density and tubular proteinuria. Environ Health Perspect. (2002) 110(7):699–702. doi: 10.1289/ehp.110-1240916
29. Silbergeld EK, Schwartz J, Mahaffey K. Lead and osteoporosis: mobilization of lead from bone in postmenopausal women. Environ Res. (1988) 47(1):79–94. doi: 10.1016/s0013-9351(88)80023-9
30. Tsaih SW, Schwartz J, Lee ML, Amarasiriwardena C, Aro A, Sparrow D, et al. The independent contribution of bone and erythrocyte lead to urinary lead among middle-aged and elderly men: the normative aging study. Environ Health Perspect. (1999) 107(5):391–6. doi: 10.1289/ehp.99107391
31. Beier EE, Holz JD, Sheu TJ, Puzas JE. Elevated lifetime lead exposure impedes osteoclast activity and produces an increase in bone mass in adolescent mice. Toxicol Sci. (2016) 149(2):277–88. doi: 10.1093/toxsci/kfv234
32. Lim HS, Lee HH, Kim TH, Lee BR. Relationship between heavy metal exposure and bone mineral density in korean adult. J Bone Metab. (2016) 23(4):223–31. doi: 10.11005/jbm.2016.23.4.223
33. Theppeang K, Glass TA, Bandeen-Roche K, Todd AC, Rohde CA, Links JM, et al. Associations of bone mineral density and lead levels in blood, tibia, and patella in urban-dwelling women. Environ Health Perspect. (2008) 116(6):784–90. doi: 10.1289/ehp.10977
34. Junaid M, Chowdhuri DK, Narayan R, Shanker R, Saxena DK. Lead-induced changes in ovarian follicular development and maturation in mice. J Toxicol Environ Health. (1997) 50(1):31–40. doi: 10.1080/009841097160582
35. Wang X, Ding N, Harlow SD, Randolph JF, Mukherjee B, Gold EB, et al. Exposure to heavy metals and hormone levels in midlife women: The Study of Women’s Health Across the Nation (SWAN). Environ pollut. (2023) 317:120740. doi: 10.1016/j.envpol.2022.120740
36. Lopes ACBA, Peixe TS, Mesas AE, Paoliello MMB. Lead exposure and oxidative stress: A systematic review. Rev Environ Contam Toxicol. (2016) 236:193–238. doi: 10.1007/978-3-319-20013-2_3
37. Rendina D, D’Elia L, Evangelista M, De Filippo G, Giaquinto A, Abate V, et al. Metabolic syndrome is associated to an increased risk of low bone mineral density in free-living women with suspected osteoporosis. J Endocrinol Invest. (2021) 44(6):1321–6. doi: 10.1007/s40618-020-01428-w
38. Rendina D, D’Elia L, De Filippo G, Abate V, Evangelista M, Giaquinto A, et al. Metabolic syndrome is not associated to an increased risk of low bone mineral density in men at risk for osteoporosis. J Endocrinol Invest. (2022) 45(2):309–15. doi: 10.1007/s40618-021-01638-w
Keywords: bone mineral density, urinary lead, national health and nutrition examination survey (NHANES), lead exposure, combined exposure
Citation: Wang S, Zhao X, Zhou R, Jin Y, Wang X, Ma X and Lu X (2024) The influence of adult urine lead exposure on bone mineral densit: NHANES 2015-2018. Front. Endocrinol. 15:1412872. doi: 10.3389/fendo.2024.1412872
Received: 05 April 2024; Accepted: 16 September 2024;
Published: 02 October 2024.
Edited by:
Giuseppina Storlino, University of Foggia, ItalyReviewed by:
Biagio Barone, ASL Napoli 1 Centro, ItalyCopyright © 2024 Wang, Zhao, Zhou, Jin, Wang, Ma and Lu. This is an open-access article distributed under the terms of the Creative Commons Attribution License (CC BY). The use, distribution or reproduction in other forums is permitted, provided the original author(s) and the copyright owner(s) are credited and that the original publication in this journal is cited, in accordance with accepted academic practice. No use, distribution or reproduction is permitted which does not comply with these terms.
*Correspondence: Xiangdong Lu, d2FsbGZvcmV2ZXJAc29odS5jb20=
Disclaimer: All claims expressed in this article are solely those of the authors and do not necessarily represent those of their affiliated organizations, or those of the publisher, the editors and the reviewers. Any product that may be evaluated in this article or claim that may be made by its manufacturer is not guaranteed or endorsed by the publisher.
Research integrity at Frontiers
Learn more about the work of our research integrity team to safeguard the quality of each article we publish.