- 1School of Nursing, Shaoyang University, Shaoyang, Hunan, China
- 2Department of Endocrinology, The Second Affiliated Hospital of Shaoyang University, Shaoyang, Hunan, China
- 3Department of Anesthesiology, Central Hospital of Shaoyang, Shaoyang, Hunan, China
Introduction: To explore whether blood flow-restrictive resistance exercise (BFRE) can be used as an alternative strategy to moderate-intensity resistance training (RT) to improve metabolic disorder and body composition in older adults with type 2 diabetes (T2DM).
Methods: This is a single-blind, randomized, controlled trial. Ninety-eight older adults with T2DM were randomly divided into three groups: BFRE group (n = 34), RT group (n = 31) and control group (n = 33). Two exercise groups received supervised collective training for a period of six months, each lasting 50 min, three times a week. The primary outcomes included fasting plasma glucose (FPG), Glycosylated hemoglobin (HbA1c), blood lipids, blood pressure, and body composition. The secondary outcome was muscle performance.
Results: After six months of intervention, the FPG, HbA1c, blood lipids, diastolic blood pressure, body composition, and muscle performance of the two exercise groups were significantly improved relative to the control group and baseline measurements (P < 0.05). There was no significant increase in lean mass between the two exercise groups compared to the control group and baseline (p > 0.05). There was no significant decrease in systolic blood pressure between the two exercise groups compared to the control group (p > 0.05), but it was significantly lower than their baseline (P < 0.05). There was no significant difference in all indicators between the two exercise groups at the baseline, third and sixth months of intervention (p > 0.05).
Discussion: BFRE can safely and effectively improve the metabolic disorder and body composition of older adults with T2DM. For elderly exercise beginners, BFRE can be used as an alternative strategy to moderate-intensity resistance training.
Clinical trial registration: https://www.chictr.org.cn/showproj.html?proj=178886, identifier ChiCTR2300074357.
1 Introduction
The number of older adults with diabetes is increasing with the aging of society (1, 2). The aging process leads to changes in human body composition. One of the characteristics is the continuous and inevitable decline of muscle mass (3). The loss of muscle mass reduces the quality of available insulin-responsive target tissues, thus promoting insulin resistance and leading to the occurrence of diabetes (4). Increasing skeletal muscle mass helps regulate glucose use, lipid oxidation, and resting metabolic rate.
On the basis of a healthy diet, ensuring sufficient exercise is the most effective way to enhance skeletal muscle. Therefore, many guidelines (2) recommend that diabetic patients should perform a total of 150 min of moderate- to high-intensity exercise at least three days a week, including two-three days of resistance training (RT). Classic resistance training [60–80% of an individual’s one-repetition maximum (1-RM)] has proven to be effective in improving the mass and strength of skeletal muscle and controlling blood glucose (5). Unfortunately, due to a sedentary lifestyle, fatigue, pain, cardiovascular and cerebrovascular diseases, as well as concerns about safety and lack of professional guidance, so that elderly diabetes patients find it difficult to tolerate and adhere to high-intensity exercise training (6). Older people are more inclined to choose exercise methods with high safety factors and low intensity. However, low-intensity exercise not only requires athletes to achieve exhaustion, but also consumes more time and has significantly poorer effects compared to high-intensity training (7). Therefore, there is an urgent need to find a relatively low-intensity exercise that is easy to accomplish, safe, and effective as an alternative strategy that can match the beneficial effects of moderate- to high-intensity exercise.
A promising exercise method to achieve this goal is blood flow-restrictive resistance exercise (BFRE), which is a new exercise method that combines distal ischemic preconditioning and low-intensity resistance training (8). The main difference from classical resistance exercise is reduced blood flow to the moving limbs (9). Numerous studies (10, 11) have shown that the skeletal muscle hypertrophy and strength enhancement effects observed in BFRE using only 20–30% 1-RM are comparable to those observed in moderate- to high-intensity resistance training (≥ 70% 1-RM) (12). Wang et al. (13) concluded that after six weeks of training with BFRE in healthy older adults, BFRE was more effective in stimulating skeletal muscle growth and improving muscle function in the elderly compared to the non-exercise control group. Therefore, they advocated the use of BFRE as a strategy to prevent age-related deterioration of skeletal muscle mass and function. Christiansen et al. (14) conducted a six-week study of exercise training in healthy men, in which one leg was trained with BFRE and the other leg without BFRE. The results indicated that the skeletal muscles of the leg trained with BFRE significantly increased glucose intake. This may be due to BFRE promoting a significant increase in muscle antioxidant function, GLUT4 abundance, and/or nitric oxide availability.
Compared to people of the same age and with normal blood glucose, older adults with T2DM experienced accelerated loss of muscle mass and strength (15), and had a higher risk of hypoglycemia, thrombosis, and cardiovascular and cerebrovascular diseases (16). The existing BFRE research is mainly aimed at people who are non-diabetic. Whether BFRE can safely and effectively control abnormal glucose and dyslipidemia by improving the body composition of older adults with T2DM remains unknown.
To address this problem, the effect of low-intensity BFRE on the abnormal metabolism of glucose and lipids was evaluated, along with improvements on human body composition in older adults with T2DM during a six-month, supervised exercise intervention. The results from BFRE and moderate-intensity resistance exercise were compared to explore whether BFRE can become an alternative strategy. The aim of this study is to provide more extensive exercise choices for older adults with T2DM, improve their life years, and provide a reference for exercise-based prevention and treatment of diabetes.
2 Materials and methods
2.1 Study design and participants
Participants were recruited from March to May 2023 at the three community health service centers in Daxiang District, Shaoyang City, Hunan Province, through medical staff referrals, physical examinations, lectures, broadcasts, and advertisements, etc. in the streets under their jurisdiction. The main inclusion criteria to meet eligibility for this study were: 60–80 years old, diagnosed with T2DM according to WHO diagnostic criteria (17), sedentary (exercise< 1 hour per week), not using an insulin pump, HbA1c levels of 6.5–11.0%, stable weight (weight loss or increase of no more than 2 kg), stable medication within three months before registration, and those who signed informed consent and volunteered to participate. The exclusion criteria mainly included guidelines (2, 18–20) and research (16) that indicated that individuals with contraindications to exercise, cognitive impairment, use of drugs that affect body composition, or inability to complete predetermined exercise programs were not suitable for inclusion in this study. Before randomization, participants were guided by exercise rehabilitation therapists to perform an incremental load test using a power vehicle for a duration of 20 minutes. The exercise test comprehensively evaluated cardiopulmonary reserve function, reserve oxygen uptake, and metabolic equivalents to assess the safety of the participants during exercise. Personal baseline data was recorded to develop personalized exercise programs (21). After obtaining medical approval from a physical therapist and endocrinologist, all participants were assigned to different exercise groups and completed corresponding exercise programs as required, with a total intervention period of six months (24 weeks).
2.2 Ethical considerations
This study protocol was reviewed and approved for implementation by the Medical Ethics Committee of Shaoyang University in accordance with the International Ethical Guidelines for Biomedical Research Involving Human Subjects and the Declaration of Helsinki. In addition, this study is registered on the Chinese Clinical Trials Registry (Registration No.: ChiCTR2300074357). All participants voluntarily participated in the study and signed informed consent forms. Participants had the right to withdraw from the study at any time for any reason without any consequences for further treatment, and the data set is kept confidential.
2.3 Randomization and blinding
This study was a single-blind, randomized, controlled trial, in which an independent chief researcher assigned unique codes to anonymize participant information according to inclusion order. Then, participants were randomly assigned to three parallel groups based on a computer-generated numerical sequence: the control group, the resistance training group (RT), and the blood flow restrictive resistance exercise group (BFRE). The researchers responsible for each group intervention and evaluation were independent of each other, and the evaluators were blinded to each participant’s group assignment.
2.4 Sample size
The necessary sample size was estimated as follows: . In our pilot study, the mean and standard deviation (mean ± SD) of fasting plasma glucose (FPG) for the three groups were 8.64 ± 1.25 for the control group, 7.51 ± 1.31 for the RT group, and 8.01 ± 1.18 for the BFRE group. One – way ANOVA of α = 0.05 (bilateral), β = 0.10, Ψ=2.55 were taken. To test whether there are significant differences among the three groups in the final data analysis, at least 31 participants were needed in each group. Therefore, the total sample size was calculated as 93. Based on a dropout rate of 20%, this required at least 38 participants per group, or a total of 114 participants.
2.5 Intervention measures
2.5.1 Diabetes education
Before the exercise intervention, all participants were invited to join different WeChat groups according to their grouping. We sent notifications and diabetes education materials to them through WeChat, and arranged for them to participate in diabetes health education classes at different times and stages, including diabetes healthy diet, exercise regularly, medication, blood glucose monitoring and regular follow-up appointments. The teaching format was online and offline blended teaching over a total of 13 hours (4 hours before the intervention, 1.5 hours per month of the intervention). All courses were administered by a diabetes-specialist nurse with 12 years of work experience. Before the end of each course, all participants were scheduled to take a test to ensure that they mastered the relevant knowledge.
2.5.2 Control group
During the study, participants were recommended to change their lifestyle according to our diabetes education content. However, supervised exercise interventions and detailed resistance training programs were not provided; participants could adhere to the advice to exercise and adjust their diet on their own or maintain their original lifestyle habits. A researcher recorded the daily exercise habits of participants through a fitness tracker or WeChat exercise mini-program, mobile health management software. Participants in the control group were invited to participate in physical examinations and face-to-face interviews before the intervention and at the third and sixth months of follow up appointments.
2.5.3 exercise intervention
The exercise intervention was conducted at the sports fields near the three community health service centers. The baseline values of the participants were measured before the exercise programs began. The first two weeks of the formal exercise intervention were for adaptation training to help participants develop exercise programs based on their individual baseline levels and familiarize themselves with the training process. Two professionally trained researchers supervised and led participants in training at each sports field to ensure standardized movements, achieve predetermined intensity, and ensure safety. After conducting a motivation survey, the exercise time was set between 7:30–8:30 in the evening. Thirty minutes before each exercise, the researchers asked the participants about their diet and physical condition, and measured their blood glucose, blood pressure, and heart rate (HR). Both exercise groups warmed up for ten minutes before the main training session, which lasted for 30 minutes. A heart rate monitoring bracelet was used to dynamically monitor the HR of participants during exercise to maintain a moderate-intensity of exercise (40–59% HR reserve). Afterwards, they stretched and relaxed for ten minutes, for a total of 50 minutes. The exercise sessions were provided three times a week, with 24–48 hours’ interval between each session, over a total of six months (24 weeks). If participants participated a total of 15 times a month, their attendance rate was considered to be 100%; if their attendance was ≥ 10 times per month (≥ 70% attendance rate), they were considered to have met the standard (22); if their attendance was less than seven times per month (< 50% attendance rate) (5), they were excluded if their attendance did not improve after encouragement and communication.
2.5.3.1 RT group
Resistance training was performed using small equipment such as barbells, dumbbells, elastic bands/ropes, and kettle bells because participants were expected to be able to learn to use small equipment at home after the study was over. Upper body exercises (shoulder press and pull down, elbow extension and flexion), hip and leg exercises (leg press, extension and flexion), and core muscle group exercises (flat-ground support, glute bridge, push-ups, sit-ups) were mainly selected for training. The initial intensity of the strength training program was low (40%–50% 1-RM) to reduce muscle soreness, avoid Valsalva movements and tendon injuries, and ensure proper weight lifting form. If the participant could fully adapt, that was, repeat 10–15 times/set with the same resistance, complete 2–4 sets, and self-rate the degree of fatigue during and after exercise to achieve 12–13 points of the Borg ratings of perceived exertion (RPE) scale, resistance can be gradually increased 5%–10% 1-RM until they can complete the moderate-intensity resistance training at a personal 60%-70% 1-RM, repeating 10–15 times/set, 60 seconds interval between sets (19, 23). Table 1 shows the detailed resistance training scheme.
2.5.3.2 BFRE group
The KAATSU Air Band (Product type: C3, USA) was tied to the most proximal end of the subject’s upper or lower limb, with a tightness that could accommodate one finger. The KAATSU host was used to control the bandage to gradually apply pressure to the limb, while the laser Doppler flowmeter quantitative analyzer (SONIMAGE, product type: HS1, China) was used to test the minimum pressure required for the subject’s limb to be blocked by arterial blood flow. The pressure displayed on the KAATSU host at this time was referred to as the total limb occlusion pressure (LOP). During each training session, the cuffs were tied to the most proximal ends of the subject’s upper or lower limbs based on the main muscle groups trained, and blood flow was limited to 50% LOP of the individual’s limbs (24). At the same time, resistance training intensity was 20–30% 1-RM. The training equipment and movements were the same as those in the ST group. The volume followed a scheme from 30 repetitions in the first set and 15 repetitions in set three. The rest periods between the sets were 30 s with the cuffs remaining inflated during rest. The blood flow restriction lasted for a total of 6.5 minutes. Then, the cuffs were completely relaxed to allow blood reperfusion, during which time two sets of core muscle group trainings were continued. There was a rest period of 30 s between sets. The total time for blood flow restriction + reperfusion training was ten minutes for each round, with three rounds in total for a total of 30 minutes. It is important to note that the applied cuff pressure should not cause pain or any obvious discomfort in the subject during training, and can be adjusted to 40–50% LOP based on the comfort of the subject (25). The goal in this study was to use pressure sufficient to induce muscle adaptation while minimizing discomfort to avoid interruptions in exercise due to subcutaneous bleeding, thrombosis, limb soreness, and other problems in the subject. Information on this regimen is shown in Table 2.
2.6 Outcome measures
The measurements were conducted at three time points: baseline (0 month), and in the third and sixth months of the intervention. The non-blood-test results were available at the end of the test, and the blood test results were reported the day after the blood collection. Researchers entered the three groups’ measurement data into Excel spreadsheets separately and conducted double checks to ensure the accuracy of the data. The data entry personnel and data analysts were independent of each other. Identity information of the subjects was replaced with corresponding numbers, and all qualified data were scanned and encrypted for storage. The original report forms were distributed to the subjects themselves, and no one was allowed to disclose patient privacy information.
2.6.1 Baseline data
Before intervention, demographic and clinical characteristics of the subjects were investigated with structured questionnaires.
2.6.2 Primary outcomes
2.6.2.1 Measurement of FPG, HbA1c, blood lipids, and blood pressure
At three time points during the measurement, three groups of participants went to designated community health service centers for physical examination between 7:00 and 9:00 am. Venous blood samples were collected from the subjects after fasting for more than eight hours, then frozen at -80 °C. After centrifugation at 3000 rpm for 15 minutes, the serum was separated and analyzed. (1) HbA1c levels were measured using ion-exchange resin high-performance liquid chromatography by a Variant II HbA1c instrument (Bio-Rad, product type: 270–2001, USA). (2) A fully automatic biochemical analyzer instrument (Hitachi, product type: 7600, Japan) was used to measure levels of fasting plasma glucose (FPG), total cholesterol (TC), triglyceride (TG), low density lipoprotein cholesterol (LDL-C), and high density lipoprotein cholesterol (HDL-C). (3) After the subject rested for 15 minutes, an electronic blood pressure monitor (OmRon, product type: U730, China) was used to measure the blood pressure (BP), included the systolic blood pressure (SBP) and diastolic blood pressure (DBP). A medical professional instructed the subjects to straighten their arms and tie cuffs two centimeters above their elbow sockets. The tightness of the cuffs can accommodate one finger. The subject was seated with the brachial artery measurement point, blood pressure monitor, and heart position at the same level. They were kept quiet until the end of the blood pressure measurement, accurate to 1 mmHg.
2.6.2.2 Body composition
A direct, segmented, multi-frequencies bioelectrical impedance analyzer (DSM-BIA) (InBody, product type: 770, Korea) was used to estimate body composition. The instrument was equipped with eight electrode touch points on both sides of the thumb, palm, sole, and heel. Five segments of the limbs and trunk were subjected to multi-frequency current through six different impedance frequencies (1, 5, 50, 250, 500, and 1000 kHz) and three different reactance frequencies (5, 50, and 250 kHz) to estimate body composition. Before the test, the subjects were required to fast and empty their urine, not exercise, or drink excessively for 30 minutes. They were asked to remove their metal jewelry, shoes, and socks; clean their hands and feet with a cloth; stand barefoot in the center of the instrument chassis; and slightly separate their legs. The forefoot and heel were placed on the front and rear foot electrodes on the instrument chassis, respectively. The weight was automatically measured to an accuracy of 0.01 kg. After entering the correct height of the subject into the system, the subject was prompted to place their thumbs and palms on the handle electrodes, stand straight, stretch their arms away from both sides of their body, remain quiet, and maintain their posture until the end of the test. Upon completion of the test, a test report was available for the researcher to enter the subject’s skeletal muscle mass (SMM), appendicular skeletal muscle mass (ASM), fat mass (FM), lean mass (LM), weight, and body mass index (BMI) into an Excel table and calculate the following indicators: fat mass index (FMI) = FM/Height2 (kg/m2), skeletal muscle mass index (SMI) = SMM/Height2 (kg/m2), appendicular skeletal muscle mass index (ASMI) = ASM/Height2 (kg/m2). Waist circumference and height were measured with a tape measure, accurate to 0.1 cm. Waist-to-height ratio (WHtR) was calculated based on waist circumference (cm)/height (cm); a WHtR > 0.5 indicates abdominal obesity. Obesity was evaluated based on BMI and divided into normal (18–23.9 kg/m2), overweight (24–27.9 kg/m2), and obese (≥ 28 kg/m2) (2).
2.6.3 Secondary outcome
2.6.3.1 Muscle performance
(1) Grip strength test: An electronic grip strength meter (CAMRY, product type: EH101, China) was used to estimate grip strength. During the test, the patient was required to stand straight, fully extend their arms, and squeeze the handle of the dynamometer with maximum strength for three seconds. The measurement was taken twice with both hands alternately. The maximum grip strength value was taken, accurate to 0.1 kg. (2) Five times-sit-to-stand test: The subject was instructed to always keep their arms crossed in front of their chest, stand up as quickly as possible from a 40 cm-high chair, and sit down again. The action was repeated five times and the time used was recorded, accurate to 0.01 seconds. (3) The 6-m walking speed test: The patient was instructed to walk straight at the fastest speed for a distance of 6 m, twice in total. The shortest time of the two records was taken, then the walking speed of the examinee was calculated, accurate to 0.01 m/s.
2.6.4 Quality control
All exercise interventions were carried out under the supervision of the researchers. During this period, if the researcher discovered events such as hypoglycemia, high blood pressure, illness, injury, etc., the participant would be required to immediately suspend or terminate the experiment, and given treatment measures within their ability. Afterwards, the treatment process should have been supplemented and recorded. The researchers needed to truthfully report the handling of adverse events to the chief researcher to arrange subsequent treatment and necessary compensation. If it was not suitable to go out for training in extreme weather, online live streaming, video playback, and other methods to guide participants to complete training at home was provided. All other training was conducted in a collective form. To reduce the dropout rate, the research group also took measures, such as regularly holding diabetes-control themed activities, providing free physical examinations and medical consultations, and issuing incentive gifts and bonuses.
2.7 Statistical analysis
Statistical analysis was performed using SPSS 23.0 (SPSS Inc., Chicago, IL, USA). For the comparison of baseline demographic characteristics between groups, the analysis of variance (ANOVA) or Kruskal-Wallis test was used for quantitative data, and the chi-square test was used for count data. The independent sample t-test was used to compare the attendance rates of the two control groups. The two-factor repeated measures ANOVA was used to analyze the changes in each dependent variable over time (from baseline to six months), and to analyze the interaction between time and population. In the case of non-compliance with the Mauchly’s test of sphericity hypothesis, the results were analyzed using Greenhouse-Geisser correction. The Bonferroni correction was used for post-hoc multiple comparisons. The mean difference [95% Confidence Interval (CI)] within the group from post-measurement to baseline was represented by the mean difference. The effect sizes of repeated measurement ANOVA was expressed by partial eta-squared (η2p, small ≥ 0.01; medium, ≥ 0.06; large ≥ 0.14). The effect sizes of mean differences between groups were expressed by Cohen’s d (d; small, ≥ 0.2; medium, ≥ 0.5; large ≥ 0.8). The level of statistical significance was defined as 0.05.
3 Results
3.1 Participant demographics and clinical characteristics
As shown in Figure 1, in June 2023, 139 older adults with T2DM who received a qualification assessment were selected to meet the inclusion criteria. After six months of intervention (June 2023-December 2023), 41 participants were excluded due to non-compliance, disease, relocation, and other reasons. Therefore, a total of 98 participants completed this study, including 33 in the control group (dropout rate of 28.26%), 31 in the RT group (dropout rate of 34.04%), and 34 in the BFRE group (dropout rate of 26.09%). Their average attendance rate was ≥ 70%, with 82.37 ± 3.55% in the RT group and 81.74 ± 2.59% in the BFRE group. There was not significant difference in attendance rates between the two exercise groups (t = 0.818, p = 0.416, df = 63).
3.2 Baseline data
As shown in Table 3, the baseline data for each dependent variable among the three groups were consistent (p > 0.05). The average age of the participants was 66.19 ± 4.75 years old, with 39 males and 59 females, and an average height of 161.43 ± 8.80 cm. Among all participants, 91 (92.9%) had abdominal obesity (WHtR > 0.5), 88 (89.8%) were overweight/obese (BMI ≥ 24 kg/m2), 69 (70.41%) used antihypertensive drugs, and 32 (32.7%) used medicine to regulate dyslipidemia. All participants had diabetes for more than two years, used hypoglycemic drugs to control blood glucose, and used them steadily for at least three months. During the intervention period, no patients changed the way they used hypoglycemic drugs, but some patients had to adjust their drug dosage due to changes in their condition. Four patients in the control group increased their dosage, while two patients in the RT group and two patients in the BFRE group needed to lower their dosage. There was no significant difference in drug adjustment among the three groups of participants (χ2 = 1.047, p = 0.593, df = 2).
3.3 Results of repeated measures analysis of variance
Tables 4–7 report the results of two-factor repeated measures of ANOVA used to compare and evaluate differences in FPG, HbA1C, BP, lipid profile, body composition, and muscle performance of the three groups. According to the Shapiro-Wilk test, all dependent variables basically obeyed normal distribution (p > 0.05). According to the Mauchly’s sphericity test, except for HbA1c, DBP, LDL-C, and ASMI, all other dependent variables did not meet the sphericity hypothesis (p< 0.05). The dependent variables that did not meet the sphericity hypothesis were subject to the results of Greenhouse-Geisser correction. The Levene test showed that the variance of the dependent variables was homogeneous (p > 0.05). The results of repeated measures ANOVA showed that except for the (group × time) interaction effect and the main effects of group and time on lean mass, which were not significant (p > 0.05), as well as the main effect of group on SBP, which was not significant (p > 0.05), the remaining dependent variables were significantly affected by the (group × time) interaction effect, and the main effects of group and time (p< 0.05).
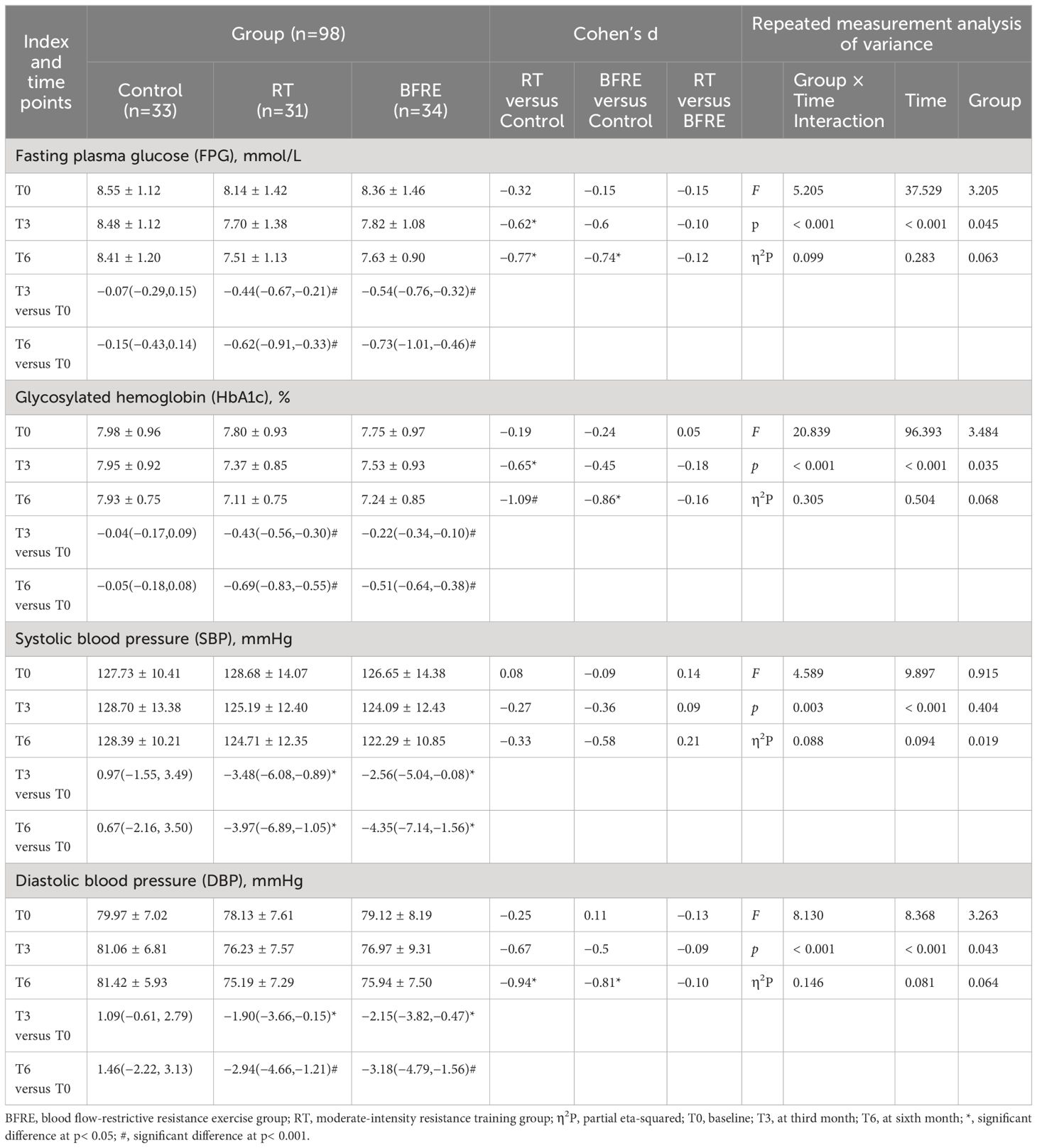
Table 4 Effects of interventions on FPG, HbA1c, and BP among the three groups (mean ± SD or mean difference [95% CI]).
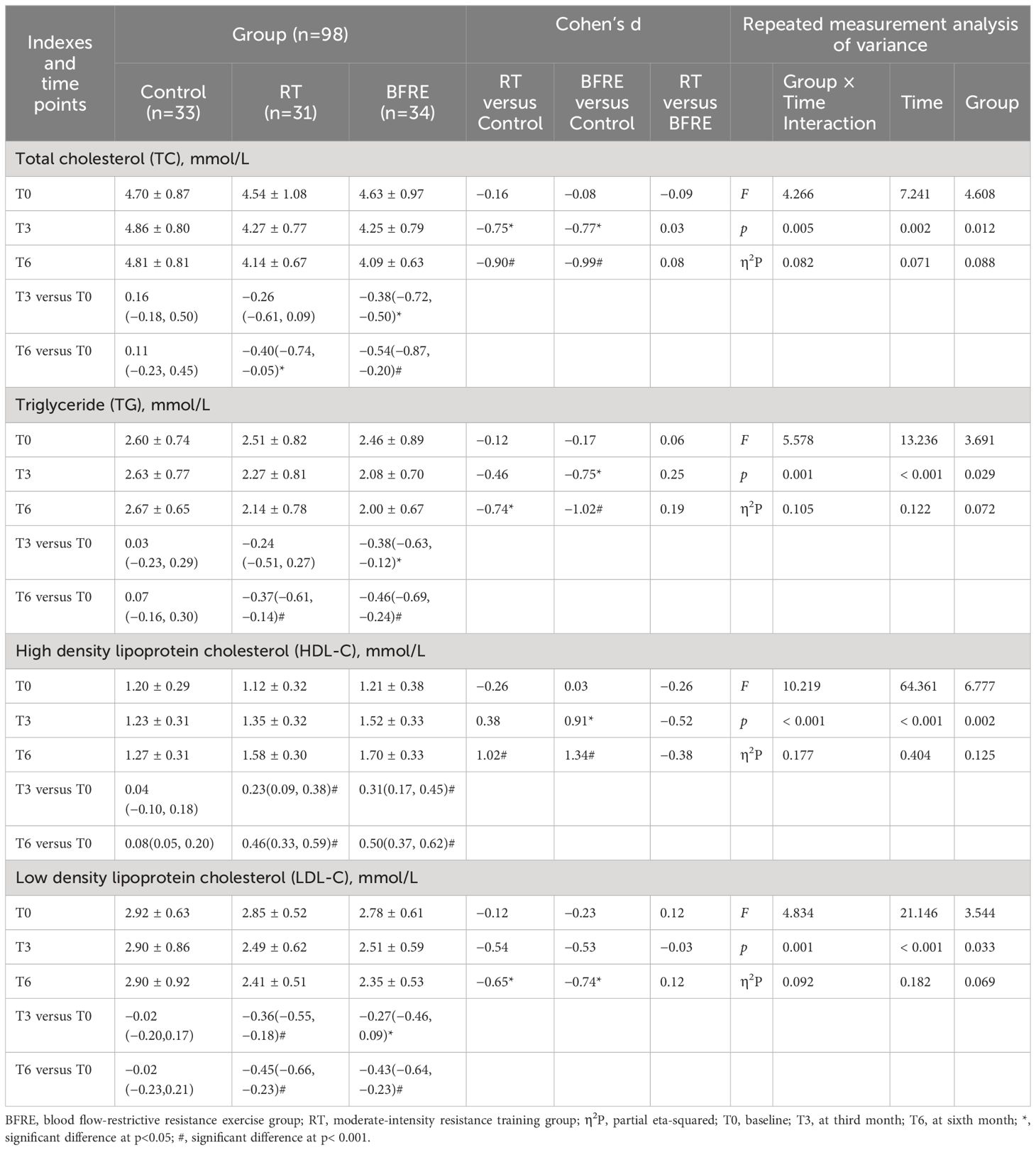
Table 5 Effects of interventions on blood lipids among the three groups [mean ± SD or mean difference (95% CI)].
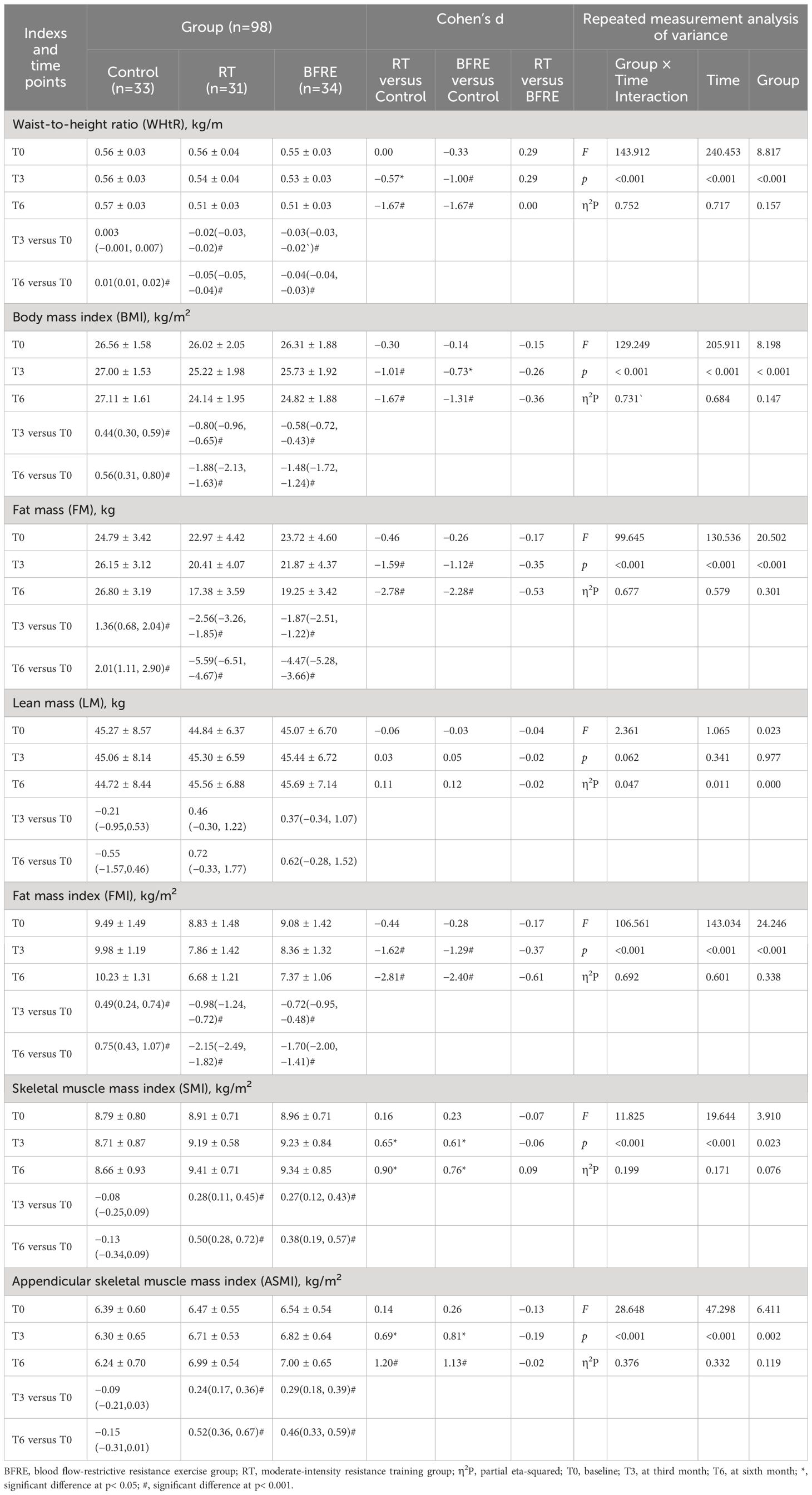
Table 6 Effects of interventions on body composition among the three groups [mean ± SD or mean difference (95% CI)].
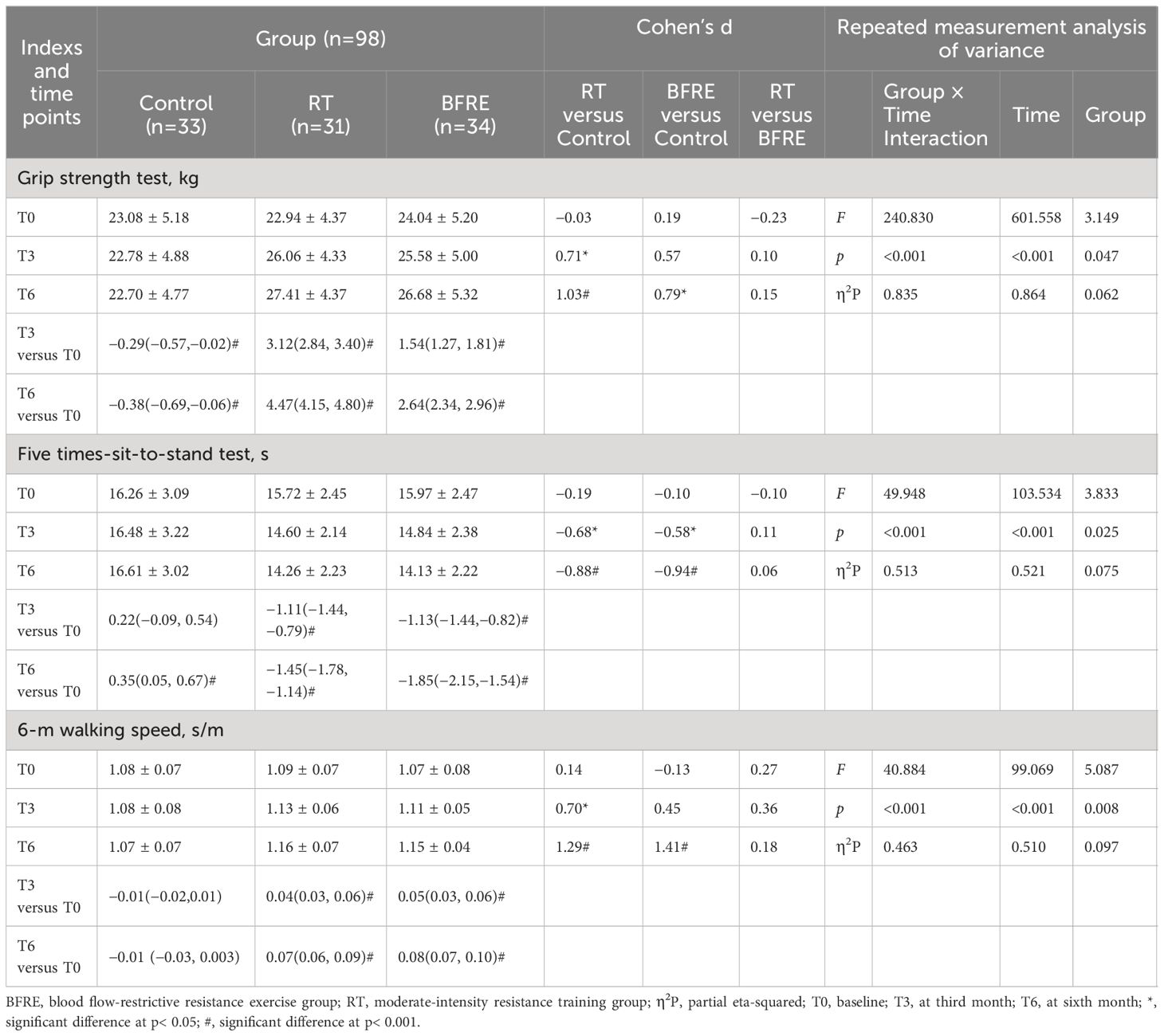
Table 7 Effects of interventions on muscle performance among three groups [mean ± SD or mean difference (95% CI)].
3.4 Primary outcomes
Table 4 shows the changes in FPG, HbA1c, and BP for the three groups. The results of repeated measures ANOVA indicate that the main effect of time in FPG, HbA1c, SBP and DBP were significant (p< 0.001). Compared to baseline, from the third month of intervention, the FPG, HbA1c, SBP and DBP of the two exercise groups decreased significantly from baseline (p< 0.05), and the improvement effect became more significant over time. Compared to the control group, the FPG (d = -0.62, p = 0.032) and HbA1c (d = -0.65, p = 0.038) in the RT group began to significantly decrease at the third month of intervention, while the same effect occurred in the BFRE group at the sixth month of intervention. At the sixth month of intervention, there was no significant difference in SBP between the three groups (F = 0.915, p = 0.404, η2P = 0.019), while the FPG, HbA1c, and DBP in RT and BFRE were significantly decreased compared to the control group (p< 0.05), with no significant difference between the two exercise groups (p > 0.05).
Table 5 shows the changes in blood lipids. Compared with baseline, at the third month of intervention, TC [with a mean (95% CI) change of -0.38 (-0.72, -0.50), p = 0.018] and TG [-0.38, (-0.63, -0.12), p = 0.002] in the BFRE group decreased significantly, while HDL-C increased significantly and LDL-C decreased significantly in both exercise groups (p< 0.05). At the sixth month of intervention, TC, TG, and LDL-C decreased significantly and HDL-C increased significantly in the two exercise groups (p< 0.05), while the improvement of blood lipids in the control group were not significant (p > 0.05). Compared with the control group, at the third month of intervention, TC in both exercise groups decreased significantly [(RT, d = -0.75, p = 0.011), (BFRE, d = -0.77, p = 0.006)], and TG decreased (d = -0.75, p = 0.012) and HDL-C increased (d = 0.91, p = 0.002) significantly in the BFRE group. At the sixth month of intervention, dyslipidemia in both exercise groups improved significantly compared with the control group (p< 0.05), with no significant difference in blood lipids between the two exercise groups (p > 0.05).
Table 6 shows the changes in body composition. The results of repeated measures ANOVA indicate that the main effect of time and the interaction effect of (group × time) on WHtR, BMI, FM, FMI, SMI, ASMI were significant (p< 0.001), indicating that the improvement effect of exercise intervention on these indicators became more pronounced over time. Compared with baseline, from the third month of intervention, WHtR, BMI, FM, FMI, SMI, ASMI showed significant improvements in both exercise groups (p< 0.05). At the third month of intervention, increases in SMI [RT, 0.28 (0.11, 0.45); BFRE, 0.27 (0.12, 0.43)] and ASMI [RT, 0.24 (0.17, 0.36); BFRE, 0.29 (0.18, 0.39)] in both exercise groups was similar, while at the sixth month of intervention, the SMI and ASMI increase in the BFRE group [SMI, 0.38 (0.19, 0.57); ASMI, 0.46 (0.33, 0.59)] was lower than that of the RT group [SMI, 0.50 (0.28, 0.72), ASMI, 0.52 (0.36, 0.67)]. At the sixth month follow-up, WHtR, BMI, FM, FMI in the control group were significantly higher than baseline (p< 0.05). Compared with the control group, WHtR, BMI, FM, FMI, SMI, ASMI in two exercise groups were significantly improved since third month of intervention (p< 0.05). There were no significant differences in body composition between the two exercise groups (p > 0.05).
3.5 Secondary outcomes
Table 7 shows the muscle performance data. Compared with the baseline, the muscle performance of both exercise groups significantly increased from the third month of intervention (p< 0.05), with the grip strength of the RT group increasing by 1.7–2 times that of the BFRE group. At the sixth month of follow-up, the performance of the control group in the grip strength and five times-sit-to-stand tests significantly decreased than baseline (p< 0.05). Compared with the control group, the two exercise groups showed significantly better performance in five times-sit-to-stand test at the third month of intervention (p< 0.05). At the same time, the grip strength (d = 0.71, p = 0.021) and 6-m walking speed (d = 0.70, p = 0.005) of the RT group significantly increased compared with the control group, while the same improvement effect was observed in the BFRE group at the sixth month of intervention. There was no significant difference in muscle performance between the two exercise groups (p > 0.05).
3.6 Safety outcomes
Adverse events in this study were defined as any adverse symptoms or events related to the study measures or exercise intervention that occurred during the study. Serious adverse events were defined as those that were life-threatening, fatal, or resulted in permanent disability. During the research period, four events were defined as adverse events, with similar incidence rates in each group (RT, n = 2; BFRE, n = 1; control group, n = 1). One person in the RT group developed lateral epicondylitis of the humerus, which may be related to inappropriate force during resistance training and housework. After rest and treatment, her symptoms had been resolved. One participant in each of the RT and BFRE groups experienced similar symptoms of hypoglycemia at home, but their self-measured blood glucose levels were 4.1 mmol/L and 4.3 mmol/L, respectively, at the time. The symptoms resolved after eating, which was considered to be related to increased physical activity but not timely eating. One person in the control group experienced dizziness during a blood test taken while fasting, which may be due to excessive tension. In addition, the safety testing and two-week of adaptation training were completed before the exercise intervention. Those who could not continue due to illness or other reasons withdrew. No abnormal fluctuations in blood pressure or blood glucose occurred during exercise, and no serious adverse events, such as syncope, thrombosis, subcutaneous bleeding, falls, cardiovascular and cerebrovascular accidents occurred.
4 Discussion
The preliminary finding of this randomized controlled study was that for older adults with T2DM, BFRE was not only safe and easy to implement, but also achieved similar effects to moderate-intensity resistance training in improving patients’ FPG, HbA1c, dyslipidemia, blood pressure, body composition, and muscle performance. Although there was not significant difference between the BFRE and RT in improving metabolic disorder and body composition in older adults with T2DM, from the trend of data changes, The BFRE had a faster effect in improving dyslipidemia, and the magnitude of increase in muscle mass and strength of the RT was greater.
Our results showed that moderate-intensity resistance exercise could significantly improve the FPG and HbA1c in older adults with T2DM. This was consistent with many previous research findings (26, 27). However, it is worth noting that the dropout rate of the RT group reached 34.04% in this study, and the main reason for withdrawal was feeling fatigued and sore in the early stages of exercise, which made them worried about worsening joint and muscle damage. In the cognition of older adults, resistance training is more suitable for those who are muscular, and participation in resistance training may increases the risk of having a heart attack, stroke or death in the elderly (6). Although the likelihood of these occurrences is small, it hinders their participation in resistance training (28).
Our research designed a BFRE program for older adults with T2DM. The main purpose of this design was to lower the load of resistance training, reduce the initial intensity and fatigue, and quickly obtain the protective effect of resistance training on blood glucose control. The results of this study show that six months of BFRE could effectively improve the FPG and HbA1c in older adults with T2DM. The effect was similar to that of moderate-intensity resistance exercise. Previous studies (14) have confirmed that BFRE can restrict blood flow by continuously pressurizing the limbs, causing severe fluctuations in the redox state of muscles due to ischemia and hypoxia, and increasing the high accumulation of reactive oxygen species (ROS). ROS activates 5’-AMP-activated protein kinase, enhancing GLUT4 mRNA and protein expression, increasing the abundance of GLUT4 in human skeletal muscle, and thus increasing glucose uptake. Therefore, most studies on BFRE use continuous pressure on the limbs during training to stimulate skeletal muscle hypertrophy and increase glucose uptake, but this can significantly increase exercise fatigue in subjects (13, 29, 30). Wang et al. (31) found that continuous compression during BFRE can improve muscle functional capacity more than intermittent compression, but higher fatigue phenomena also occur. In contrast, although intermittent BFRE can also bring fatigue, the recovery speed is faster. It is recommended that beginners adopt intermittent BFRE. Husmann et al. (32) pointed out that BFRE exacerbated the accumulation of exercise-induced, fatigue-related metabolites and prevented the recovery of contractile function during rest intervals. However, after two minutes of reperfusion, muscle contraction function recovered substantially, diminishing the impact of blood flow restriction on muscle fatigue. Therefore, in our BFRE program, there were 6.5 minutes of continuous compression on both the upper or lower limbs to stimulate skeletal muscle hypertrophy and increase skeletal muscle glucose uptake. Additionally, there were two minutes of blood flow reperfusion to reduce fatigue, allowing subjects to complete longer single-training sessions (30 minutes), fully exercise the muscles and joints of the body, and enhance exercise endurance.
In our study, although there was no significant difference in SBP between the two exercise groups and the control group, there was a significant decrease in both systolic and diastolic blood pressure compared to baseline. This shows that BFRE can steadily reduce the blood pressure of the older adults with T2DM. The impact of BFRE on blood pressure is still controversial. The experimental results of Rossow et al. (33) showed that only high-intensity resistance exercise showed a good antihypertensive effect on young men, while the effect of BFRE was not significant. The research results of Crisafulli et al. (34) and Maior et al. (35) indicate that young men only need to use relatively small amounts of muscles (such as grasping, biceps curling, or single-joint exercise) for low-load BFRE (≤ 40% 1-RM) to reduce post-exercise blood pressure. The meta-analysis results of Domingos et al. (36) suggested that although BFRE lead to greater post-exercise hypotension compared to traditional exercise, higher SBP and/or DBP were observed during BFRE, especially in hypertensive patients. Therefore, caution should be exercised when using BFRE. Another study suggests (37) that for older adults, the acute hemodynamic response caused by low-intensity BFRE is similar to that caused by high-load training, and can return to normal levels within 30 minutes after training with a more significant decrease in SBP. However, these studies were aimed at young adults and the non-diabetes population. The effect of BFRE on blood pressure in older adults with T2DM is rarely reported. Given previous studies suggesting that BFRE may cause fluctuations in blood pressure during exercise, in this study, we monitored the blood pressure of participants before exercise and found that abnormally elevated blood pressure may be related to factors such as climate change, poor sleep, and underlying disease changes. We tracked and treated patients with high blood pressure as necessary, so there were no significant fluctuations in blood pressure during exercise. After the six-month intervention, the blood pressure of participants in the BFRE group slowly declined with the extension of exercise time, but it did not cause a sudden drop in blood pressure, which has a protective effect on the cardiovascular health of the elderly.
In our research results, after exercise intervention, there was no significant change in lean mass, but WHtR, BMI, FM, and FMI were significantly reduced, and dyslipidemia was significantly improved in the RT and BFRE groups. This indicated that the two types of exercise could effectively reduce fat, especially abdominal fat, to achieve the goal of optimizing body shape and blood lipids. This is consistent with the research findings of Sun et al. (38). Abdominal obesity in elderly T2DM patients is often accompanied by more serious dyslipidemia (39, 40). The increased LDL-C has a lower affinity for vascular endothelial tissue and arterial wall proteoglycans, and is more prone to oxidation, leading to the formation of atherosclerotic plaques in the arteries (41). HDL-C dysfunction leads to the reduction of its anti-atherosclerosis, antioxidant, and anti-inflammatory properties, and accelerates the process of atherosclerosis (42). Elderly T2DM patients should be encouraged to exercise regularly in various ways to change abdominal obesity and dyslipidemia, in order to reduce the risk of cardiovascular complications. Compared to moderate-intensity resistance training, BFRE has a lower load during training, allowing athletes to use portable and lightweight exercise equipment to complete a variety of movements. This makes exercise more enjoyable and interesting without being limited by venue and time, making it more conducive to long-term persistence in elderly T2DM patients.
In this study, the SMI and ASMI of the two exercise groups were significantly improved compared to the baseline and control group, and the effects of the two exercise groups were similar. This may be because the hypoxic environment created by BFRE increases blood lactate concentration, accumulates metabolites, and promotes increased secretion of muscle hypertrophy hormones (such as growth hormone), synergistically promoting muscle protein synthesis (43). In addition, BFRE may further promote muscle hypertrophy by affecting the generation of nitric oxide or the activation of specific heat shock proteins (44). Therefore, even if low-intensity exercise were used, it could also achieve the muscle strengthening effect of moderate-intensity resistance training, which is of great significance to older adults with T2DM to prevent and treat sarcopenia. However, Lixandrão et al. (45) pointed out that muscle hypertrophy does not necessarily mean an increase in muscle strength. Even if both exercises can induce similar muscle mass increases, high-intensity resistance training (> 65% 1-RM) significantly improves muscle strength growth compared to low-intensity BFRE. This may be because low-intensity BFRE has a relatively low promoting effect on neuromuscular driving ability. The ability to recruit muscle fibers cannot achieve the same effect as high-intensity training. In our study, although the increase in grip strength in the RT group was 1.7–2 times that of the BFRE group, there was no significant difference in grip strength, sit-to-stand and walking speed between the two exercise groups. This may be because our research mainly focused on older adults with T2DM. The strength and speed improvement of elderly people with sedentary habits was relatively limited, and diabetes patients tend to be weaker and more prone to fatigue. BFRE puts skeletal muscles in a hypoxic environment, leading to premature fatigue of type I muscle fibers. To resist external stress, more type II muscle fibers are activated to participate in work, and type II muscle fibers are the key to muscle hypertrophy and muscle strength growth. BFRE can improve the muscle mass and strength of older adults with T2DM with less effort, which is essential to preventing falls during exercise. After older adults improve their physical strength, balance, and stability through BFRE to a certain extent, their acceptance of exercise will be greatly enhanced, laying a good foundation for further acceptance of moderate-to-high-intensity resistance exercise or the other exercises.
5 Limitations of the study
This study also has some limitations, such as the intake of protein, carbohydrates, fat, and water in food affecting changes in lean mass and muscle mass. However, we did not provide a detailed evaluation of the participants’ dietary behaviors or nutritional content of the food consumed, as recording was difficult for older adults. This makes it difficult to determine why six months of training did not significantly increase the lean mass of participants. A possible reason is that to control blood glucose, the participants mainly consumed light vegetables in their diet, with a reduced proportion of fruits, staple foods, meat, and fats. In addition, age-related muscle loss slowed down the growth of lean mass after exercise to a certain extent. In the future, the impact of combining dietary control with BFRE on T2DM will be explored further, in hopes of better promoting this exercise.
6 Conclusion
In this study, a BFRE program was designed combining intermittent restriction of limbs blood flow and low-intensity resistance training. The results showed that BFRE could effectively improve the metabolic disorder of blood glucose, dyslipidemia, and blood pressure in older adults with T2DM, and could also enhance the muscle function of patients by controlling abdominal obesity and reducing muscle loss. The effects of BFRE were similar to moderate-intensity resistance training. However, from the perspective of long-term data trends, BFRE may not be as effective as moderate-intensity resistance exercise in balancing muscle mass and strength in patients. Therefore, it is suggested that the older adults with T2DM should use BFRE for training under the guidance of professional medical staff at the beginning of exercise to obtain exercise adaptation in this relatively simple and easy way, then consider carrying out moderate- intensity resistance training, and choosing proper exercise methods according to their own conditions to better control diabetes.
Data availability statement
The original contributions presented in the study are included in the article/supplementary material. Further inquiries can be directed to the corresponding authors.
Ethics statement
This study protocol has been reviewed and approved for implementation by the Medical Ethics Committee of Shaoyang University and registered on the Chinese Clinical Trials Registry (Registration No.: ChiCTR2300074357). The studies were conducted in accordance with the local legislation and institutional requirements. The participants provided their written informed consent to participate in this study. Written informed consent was obtained from the individual(s) for the publication of any potentially identifiable images or data included in this article.
Author contributions
XM: Writing – review & editing, Writing – original draft, Visualization, Validation, Supervision, Software, Resources, Project administration, Methodology, Investigation, Funding acquisition, Formal analysis, Data curation, Conceptualization. YA: Writing – review & editing, Writing – original draft, Methodology. FL: Writing – review & editing, Writing – original draft, Investigation. XT: Writing – review & editing, Writing – original draft, Investigation. QL: Writing – review & editing, Writing – original draft, Investigation. YH: Writing – review & editing, Writing – original draft, Investigation. YZ: Writing – review & editing, Writing – original draft, Investigation. QM: Writing – review & editing, Writing – original draft, Investigation. LW: Writing – review & editing, Writing – original draft, Supervision, Methodology. FL: Writing – review & editing, Writing – original draft, Supervision, Methodology. QY: Writing – review & editing, Writing – original draft, Supervision, Methodology. FY: Writing – review & editing, Writing – original draft, Data curation. XY: Writing – review & editing, Writing – original draft, Software, Data curation. BH: Writing – review & editing, Writing – original draft, Supervision, Resources, Funding acquisition. LZ: Writing – review & editing, Writing – original draft, Software, Data curation. SR: Writing – review & editing, Writing – original draft, Supervision, Resources, Funding acquisition.
Funding
The author(s) declare financial support was received for the research, authorship, and/or publication of this article. This research was supported by the Natural Science Foundation of Hunan Province (2021JJ70046, 2022JJ50027, 2023JJ50250), the Hunan Province College Student Innovation and Entrepreneurship Training Program Project (S202310547069), and the Shaoyang City-level Science and Technology Plan Project (2023ZD0058, 2023GZ4042).
Acknowledgments
We are sincerely grateful for the patients and workers who participated in this study.
Conflict of interest
The authors declare that the research was conducted in the absence of any commercial or financial relationships that could be construed as a potential conflict of interest.
Publisher’s note
All claims expressed in this article are solely those of the authors and do not necessarily represent those of their affiliated organizations, or those of the publisher, the editors and the reviewers. Any product that may be evaluated in this article, or claim that may be made by its manufacturer, is not guaranteed or endorsed by the publisher.
References
1. Li Y, Teng D, Shi X, Qin G, Qin Y, Quan H, et al. Prevalence of diabetes recorded in mainland China using 2018 diagnostic criteria from the American Diabetes Association: National cross sectional study. BMJ. (2020) 369:m997. doi: 10.1136/bmj.m997
2. China. Clinical guidelines for prevention and treatment of type 2 diabetes mellitus in the elderly in China (2022 edition). Chin J Intern Med. (2022) 30:2–51.
3. Izzo A, Massimino E, Riccardi G, Della Pepa G. A narrative review on sarcopenia in type 2 diabetes mellitus: prevalence and associated factors. Nutrients. (2021) 13:183. doi: 10.3390/nu13010183
4. Li Y, Lin S, Xu X, Jin W, Su Y, Yuan F, et al. Skeletal muscle HSF1 prevents insulin resistance by improving glucose utilization. FASEB J. (2022) 36:e22667. doi: 10.1096/fj.202201160RR
5. Kobayashi Y, Long J, Dan S, Johannsen NM, Talamoa R, Raghuram S, et al. Strength training is more effective than aerobic exercise for improving glycaemic control and body composition in people with normal-weight type 2 diabetes: a randomised controlled trial. Diabetologia. (2023) 66:1897–907. doi: 10.1007/s00125–023-05958–9
6. Burton E, Farrier K, Lewin G, Pettigrew S, Hill A-M, Airey P, et al. Motivators and barriers for older people participating in resistance training: A systematic review. J Aging Phys Act. (2017) 25:311–24. doi: 10.1123/japa.2015–0289
7. Lee J, Kim D, Kim C. Resistance training for glycemic control, muscular strength, and lean body mass in old Type 2 diabetic patients: A meta-analysis. Diabetes Ther. (2017) 8:459–73. doi: 10.1007/s13300–017-0258–3
8. Saatmann N, Zaharia O-P, Loenneke JP, Roden M, Pesta DH. Effects of blood flow restriction exercise and possible applications in type 2 diabetes. Trends Endocrinol Metab. (2021) 32:106–17. doi: 10.1016/j.tem.2020.11.010
9. Cook SB, Laroche DP, Villa MR, Barile H, Manini TM. Blood flow restricted resistance training in older adults at risk of mobility limitations. Exp Gerontol. (2017) 99:138–45. doi: 10.1016/j.exger.2017.10.004
10. Hughes L, Paton B, Rosenblatt B, Gissane C, Patterson SD. Blood flow restriction training in clinical musculoskeletal rehabilitation: a systematic review and meta-analysis. Br J Sports Med. (2017) 51:1003–11. doi: 10.1136/bjsports-2016–097071
11. Hwang PS, Willoughby DS. Mechanisms behind blood flow–restricted training and its effect toward muscle growth. J Strength Cond Res. (2019) 33:S167–79. doi: 10.1519/JSC.0000000000002384
12. Hughes L, Rosenblatt B, Haddad F, Gissane C, Mccarthy D, Clarke T, et al. Comparing the effectiveness of blood flow restriction and traditional heavy load resistance training in the post-surgery rehabilitation of anterior cruciate ligament reconstruction patients: A UK National Health Service randomised controlled trial. Sports Med. (2019) 49:1787–805. doi: 10.1007/s40279–019-01137–2
13. Wang J, Mogensen AG, Thybo F, Brandbyge M, Brorson J, Van Hall G, et al. Low-load blood flow-restricted resistance exercise produces fiber type-independent hypertrophy and improves muscle functional capacity in older individuals. J Appl Physiol (1985). (2023) 134:1047–62. doi: 10.1152/japplphysiol.00789.2022
14. Christiansen D, Eibye KH, Hostrup M, Bangsbo J. Blood flow-restricted training enhances thigh glucose uptake during exercise and muscle antioxidant function in humans. Metabolism. (2019) 98:1–15. doi: 10.1016/j.metabol.2019.06.003
15. Miller EG, Nowson CA, Dunstan DW, Kerr DA, Menzies D, Daly RM. Effects of whey protein plus vitamin D supplementation combined with progressive resistance training on glycaemic control, body composition, muscle function and cardiometabolic risk factors in middle-aged and older overweight/obese adults with type 2 diabetes: A 24-week randomized controlled trial. Diabetes Obes Metab. (2021) 23:938–49. doi: 10.1111/dom.14299
16. Nascimento DDC, Rolnick N, Neto IVS, Severin R, Beal FLR. A useful blood flow restriction training risk stratification for exercise and rehabilitation. Front Physiol. (2022) 13:808622. doi: 10.3389/fphys.2022.808622
17. Federation. Definition and diagnosis of diabetes mellitus and intermediate hyperglycaemia. Report of a WHO/IDF consultation (2016). Available online at: https://www.who.int/diabetes/publications/diagnosis_diabetes2006/en/.
18. Colberg SR, Sigal RJ, Fernhall B, Regensteiner JG, Blissmer BJ, Rubin RR, et al. Exercise and type 2 diabetes: The American College of Sports Medicine and the American Diabetes Association: Joint position statement. Diabetes Care. (2010) 33:e147–67. doi: 10.2337/dc10–9990
19. Colberg SR, Sigal RJ, Yardley JE, Riddell MC, Dunstan DW, Dempsey PC, et al. Physical activity/exercise and diabetes: A position statement of the American Diabetes Association. Diabetes Care. (2016) 39:2065–79. doi: 10.2337/dc16–1728
20. Elsayed NA, Aleppo G, Aroda VR, Bannuru RR, Brown FM, Bruemmer D, et al. 13. Older adults: standards of care in diabetes—2023. Diabetes Care. (2023) 46:S216–29. doi: 10.2337/dc23-S013
21. Hansen D, Niebauer J, Cornelissen V, Barna O, Neunhäuserer D, Stettler C, et al. Exercise prescription in patients with different combinations of cardiovascular disease risk factors: a consensus Statement from the EXPERT Working Group. Sports Med. (2018) 48:1781–97. doi: 10.1007/s40279-018-0930-4
22. Dai X, Zhai L, Chen Q, Miller JD, Lu L, Hsue C, et al. Two-year-supervised resistance training prevented diabetes incidence in people with prediabetes: A randomised control trial. Diabetes Metab Res Rev. (2019) 35:e3143. doi: 10.1002/dmrr.3143
23. Garber CE, Blissmer B, Deschenes MR, Franklin BA, Lamonte MJ, Lee IM, et al. Quantity and quality of exercise for developing and maintaining cardiorespiratory, musculoskeletal, and neuromotor fitness in apparently healthy adults. Med Sci Sports Exerc. (2011) 43:1334–59. doi: 10.1249/MSS.0b013e318213fefb
24. Zhuang M, Shi J, Liu J, He X, Chen N. Comparing the efficacy of low-load resistance exercise combined with blood flow restriction versus conventional-load resistance exercise in Chinese community-dwelling older people with sarcopenic obesity: a study protocol for a randomised controlled trial. BMC Geriatr. (2023) 23:874. doi: 10.1186/s12877–023-04592–9
25. Sieljacks P, Degn R, Hollaender K, Wernbom M, Vissing K. Non-failure blood flow restricted exercise induces similar muscle adaptations and less discomfort than failure protocols. Scand J Med Sci Sports. (2019) 29(3):336–47. doi: 10.1111/sms.13346
26. Ciumărnean L, Milaciu MV, Negrean V, Orășan OH, Vesa SC, Sălăgean O, et al. Cardiovascular risk factors and physical activity for the prevention of cardiovascular diseases in the elderly. Int J Environ Res Public Health. (2021) 19:207. doi: 10.3390/ijerph19010207
27. Jansson AK, Chan LX, Lubans DR, Duncan MJ, Plotnikoff RC. Effect of resistance training on HbA1c in adults with type 2 diabetes mellitus and the moderating effect of changes in muscular strength: a systematic review and meta-analysis. BMJ Open Diabetes Res Care. (2022) 10:e002595. doi: 10.1136/bmjdrc-2021–002595
28. Fragala MS, Cadore EL, Dorgo S, Izquierdo M, Kraemer WJ, Peterson MD, et al. Resistance training for older adults: position statement from the National Strength and Conditioning Association. J Strength Cond Res. (2019) 33:2019–52. doi: 10.1519/JSC.0000000000003230
29. Karabulut M, Cramer JT, Abe T, Sato Y, Bemben MG. Neuromuscular fatigue following low-intensity dynamic exercise with externally applied vascular restriction. J Electromyogr Kinesiol. (2010) 20:440–7. doi: 10.1016/j.jelekin.2009.06.005
30. Chen N, He X, Zhao G, Lu L, Ainsworth BE, Liu Y, et al. Efficacy of low-load resistance training combined with blood flow restriction vs. high-load resistance training on sarcopenia among community-dwelling older Chinese people: study protocol for a 3-arm randomized controlled trial. Trials. (2021) 22:518. doi: 10.1186/s13063-021-05495-z
31. Wang Y, Li Z, Tongtong C, Zhang W, Li X. Effect of continuous and intermittent blood flow restriction deep-squat training on thigh muscle activation and fatigue levels in male handball players. Sci Rep. (2023) 13:19152. doi: 10.1038/s41598–023-44523–7
32. Husmann F, Mittlmeier T, Bruhn S, Zschorlich V, Behrens M. Impact of blood flow restriction exercise on muscle fatigue development and recovery. Med Sci Sports Exerc. (2018) 50:436–46. doi: 10.1249/MSS.0000000000001475
33. Rossow LM, Fahs CA, Sherk VD, Seo D-I, Bemben DA, Bemben MG. The effect of acute blood-flow-restricted resistance exercise on postexercise blood pressure. Clin Physiol Funct Imaging. (2011) 31:429–34. doi: 10.1111/cpf.2011.31.issue-6
34. Crisafulli A, De Farias RR, Farinatti P, Lopes KG, Milia R, Sainas G, et al. Blood flow restriction training reduces blood pressure during exercise without affecting Metaboreflex activity. Front Physiol. (2018) 9:1736. doi: 10.3389/fphys.2018.01736
35. Maior AS, Simão R, Martins MSR, de Salles BF, Willardson JM. Influence of blood flow restriction during low-intensity resistance exercise on the postexercise hypotensive response. J Strength Cond Res. (2015) 29:2894–9. doi: 10.1519/JSC.0000000000000930
36. Domingos E, Polito MD. Blood pressure response between resistance exercise with and without blood flow restriction: A systematic review and meta-analysis. Life Sci. (2018) 209:122–31. doi: 10.1016/j.lfs.2018.08.006
37. Centner C, Wiegel P, Gollhofer A, König D. Effects of blood flow restriction training on muscular strength and hypertrophy in older individuals: A systematic review and meta-analysis. Sports Med. (2018) 49:95–108. doi: 10.1007/s40279-018-0994-1
38. Sun L. Effects of blood flow restriction training on anthropometric and blood lipids in overweight/obese adults: meta-analysis. Front Physiol. (2022) 13:1039591. doi: 10.3389/fphys.2022.1039591
39. Lee JS, Chang P-Y, Zhang Y, Kizer JR, Best LG, Howard BV. Triglyceride and HDL-C dyslipidemia and risks of coronary heart disease and ischemic stroke by glycemic dysregulation status: The strong heart study. Diabetes Care. (2017) 40:529–37. doi: 10.2337/dc16–1958
40. Bhatt DL, Steg PG, Miller M, Brinton EA, Jacobson TA, Ketchum SB, et al. Cardiovascular risk reduction with icosapent ethyl for hypertriglyceridemia. N Engl J Med. (2019) 380:11–22. doi: 10.1056/NEJMoa1812792
41. Banach M, Surma S, Reiner Z, Katsiki N, Penson PE, Fras Z, et al. Personalized management of dyslipidemias in patients with diabetes-it is time for a new approach, (2022). Cardiovasc Diabetol. (2022) 21:263. doi: 10.1186/s12933–022-01684–5
42. Kaze AD, Santhanam P, Musani SK, Ahima R, Echouffo-Tcheugui JB. Metabolic dyslipidemia and cardiovascular outcomes in type 2 diabetes mellitus: findings from the look AHEAD study. J Am Heart Assoc. (2021) 10:e016947. doi: 10.1161/JAHA.120.016947
43. Franz A, Ji S, Bittersohl B, Zilkens C, Behringer M. Impact of a six-week prehabilitation with blood-flow restriction training on pre- and postoperative skeletal muscle mass and strength in patients receiving primary total knee arthroplasty. Front Physiol. (2022) 13:881484. doi: 10.3389/fphys.2022.881484
44. Nielsen JL, Aagaard P, Prokhorova TA, Nygaard T, Bech RD, Suetta C, et al. Blood flow restricted training leads to myocellular macrophage infiltration and upregulation of heat shock proteins, but no apparent muscle damage. J Physiol. (2017) 595:4857–73. doi: 10.1113/JP273907
45. Lixandrão ME, Ugrinowitsch C, Berton R, Vechin FC, Conceição MS, Damas F, et al. Magnitude of muscle strength and mass adaptations between high-load resistance training versus low-load resistance training associated with blood-flow restriction: A systematic review and meta-analysis. Sports Med. (2017) 48:361–78.
Keywords: blood flow-restrictive resistance exercise, type 2 diabetes, metabolic disorders, body composition, older adults
Citation: Ma X, Ai Y, Lei F, Tang X, Li Q, Huang Y, Zhan Y, Mao Q, Wang L, Lei F, Yi Q, Yang F, Yin X, He B, Zhou L and Ruan S (2024) Effect of blood flow-restrictive resistance training on metabolic disorder and body composition in older adults with type 2 diabetes: a randomized controlled study. Front. Endocrinol. 15:1409267. doi: 10.3389/fendo.2024.1409267
Received: 29 March 2024; Accepted: 20 May 2024;
Published: 06 June 2024.
Edited by:
Roberto Codella, University of Milan, ItalyReviewed by:
Emiliano Cè, University of Milan, ItalyEmanuela Faelli, University of Genoa, Italy
Simone Di Gennaro, University of Genoa, Italy, in collaboration with reviewer EF
Copyright © 2024 Ma, Ai, Lei, Tang, Li, Huang, Zhan, Mao, Wang, Lei, Yi, Yang, Yin, He, Zhou and Ruan. This is an open-access article distributed under the terms of the Creative Commons Attribution License (CC BY). The use, distribution or reproduction in other forums is permitted, provided the original author(s) and the copyright owner(s) are credited and that the original publication in this journal is cited, in accordance with accepted academic practice. No use, distribution or reproduction is permitted which does not comply with these terms.
*Correspondence: Sijie Ruan, 2623398873@qq.com; Lei Zhou, 1056555372@qq.com
†These authors share first authorship
‡These authors share last authorship