- 1Reproductive Medical Center, Affiliated Hospital of Guangdong Medical University, Zhanjiang, Guangdong, China
- 2Department of Gynaecology, The Second Affiliated Hospital of Guangdong Medical University, Zhanjiang, Guangdong, China
- 3Department of Obstetrics and Gynecology, Affiliated Hospital of Guangdong Medical University, Zhanjiang, Guangdong, China
- 4Graduate School of Guangdong Medical University, Zhanjiang, Guangdong, China
- 5Department of Hematology, Affiliated Hospital of Guangdong Medical University, Zhanjiang, Guangdong, China
Objective: To investigate the causal effect of immune cells on endometriosis (EMS), we performed a Mendelian randomization analysis.
Methods: Mendelian randomization (MR) uses genetic variants as instrumental variables to investigate the causal effects of exposures on outcomes in observational data. In this study, we conducted a thorough two-sample MR analysis to investigate the causal relationship between 731 immune cells and endometriosis. We used complementary Mendelian randomization (MR) methods, including weighted median estimator (WME) and inverse variance weighted (IVW), and performed sensitivity analyses to assess the robustness of our results.
Results: Four immune phenotypes have been found to be significantly associated with the risk of developing EMS: B cell %lymphocyte (WME: OR: 1.074, p = 0.027 and IVW: OR: 1.058, p = 0.008), CD14 on Mo MDSC (WME: OR: 1.056, p =0.021 and IVW: OR: 1.047, p = 0.021), CD14+ CD16− monocyte %monocyte (WME: OR: 0.947, p = 0.024 and IVW: OR: 0.958, p = 0.011), CD25 on unsw mem (WME: OR: 1.055, p = 0.030 and IVW: OR: 1.048, p = 0.003). Sensitivity analyses confirmed the main findings, demonstrating consistency across analyses.
Conclusions: Our MR analysis provides compelling evidence for a direct causal link between immune cells and EMS, thereby advancing our understanding of the disease. It also provides new avenues and opportunities for the development of immunomodulatory therapeutic strategies in the future.
1 Introduction
Endometriosis (EMS) is a chronic inflammatory condition characterized by the presence of endometrium-like tissue outside the uterus (1). Patients with endometriosis have 25–40% concurrent infertility, while 40–87% experience chronic pelvic pain (2). Genetic predisposition (3), hormonal irregularities (4), environmental influences (5), immune dysregulation (6) and unique anatomical configurations (7) are recognized as potential risk factors associated with the onset of endometriosis. The available evidence suggests that immune dysfunction plays an important role in both the pathogenesis of endometriosis and the manifestation of its clinical symptoms (8).
Disturbances in immune homeostasis can create a favorable environment for implantation, proliferation and angiogenesis of ectopic endometrial tissue (9). The abnormal activation of immune cells such as B cells (10), T cells (11), natural killer (NK) cells (12), Dendritic cells (DC) (13), Monocytes (14) and macrophages (15) leads to increased levels of various inflammatory factors, autoantibodies and cytokines. However, findings on the relationship between immune cells and EMS have been inconsistent.
A systematic review that synthesized the results of 22 selected trials found that the majority of trials reported an increased number and/or activation of B cells in endometriosis. However, seven trials did not find a significant difference, while two trials showed a reduced number of B cells (16). These discrepancies may be due to a limited sample size, to flaws in the design of the studies, and to confounding factors that are beyond the scope of the existing studies.
While quantitative changes in CD4+ T cells have been investigated in numerous studies, there have been conflicting results regarding the frequency of CD4+ T cells in both peripheral blood (PB) and/or peritoneal fluid (PF) when comparing individuals with endometriosis (EM) to healthy women (17).
NK cells have been proposed to have a significant influence on the pathogenesis of the disease, potentially either promoting tolerance or inhibiting the survival, implantation and proliferation of endometrial cells (18). Several studies have reported a significant reduction in the levels of CD56+ NK cells in peritoneal fluid samples from women diagnosed with endometriosis (19, 20). However, alternative research perspectives posit that there are no discernible distinctions in CD56+ NK cell levels within the peritoneal fluid between patients and control groups (21, 22).
The growth and vascularization of endometriosis is dependent on the presence of endogenous dendritic cells, which infiltrate endometriotic lesions and promote endothelial cell migration through the secretion of proangiogenic factors (23). Hey-Cunningham et al. identified variations in dendritic cell populations both locally in endometrial tissue and systemically in the circulation in women with endometriosis, with stage-specific associations within the endometrium (24). However, the impact of dendritic cells on the development of endometriosis lesions in mouse models has been inconsistent (25–27).
Monocytes, macrophages and dendritic cells (DCs) act as antigen-presenting cells (APCs), stimulate T cells and secrete a variety of inflammatory mediators that can modulate immune responses (28).The phagocytic function of peripheral monocytes is reduced in patients with endometriosis compared to healthy women, which may contribute to the observed immunological changes in the disease, as well as being influenced by the presence of ectopic endometrial lesions (29).
Macrophages are classified according to their activation pathway as either ‘classically activated’ (M1) or ‘alternatively activated’ (M2) macrophages. While it is widely accepted that M2 macrophages promote the progression of endometriosis, with M1 macrophages playing a lesser role (30, 31), Takebayashi et al. (32) and Vallvé-Juanico et al. (33) present a contrasting perspective, suggesting that M1 macrophages are the predominant macrophage population in the endometrium of patients with endometriosis. The temporal distribution of macrophages in ectopic endometrial tissue remains uncertain. Braun et al. (34) found a decrease in macrophage numbers only during the early proliferative phase in patients with endometriosis, whereas Khan et al. (35) found higher macrophage numbers in patients with endometriosis than in non-endometriosis patients during all phases. In addition, Berbic et al. (36) reported an increase in macrophage numbers throughout the proliferative phase in patients with endometriosis.
Mendelian randomization (MR) is a well-established epidemiological method that uses genetic studies to elucidate causality (37). MR uses single nucleotide polymorphisms (SNPs) identified through genome-wide association studies (GWAS) as instrumental variables, effectively simulating randomized controlled trials (RCTs) and mitigating biases associated with confounding and reverse causation (38). Previous observational studies have suggested numerous associations between immune cell characteristics and endometriosis. In this study, we further elucidate the causal relationship between immune cell characteristics and endometriosis from a genetic perspective using MR analysis, thereby providing more targeted strategies for future interventions and treatments. This has significant implications for the prevention, diagnosis and treatment of endometriosis (Figure 1).
2 Materials and methods
2.1 Study design
In this study, we first assessed 731 immune cell types as potential exposure factors, using single nucleotide polymorphisms (SNPs) significantly correlated with these cells as instrumental variables. Endometriosis was then considered as the outcome variable in our analysis. The analysis was performed using a two-sample Mendelian randomization (MR) approach. To ensure the reliability of the results, we tested for heterogeneity, pleiotropy and sensitivity analysis.
2.2 GWAS data sources
We downloaded data on 731 immune cells from the GWAS Catalogue website (https://www.ebi.ac.uk/gwas/home) with PubMed ID 32929287. This dataset has accession numbers from GCST0001391 to GCST0002121 and includes data from 563,085 European samples, covering approximately 22 million SNPs (39).
We obtained genetic data on endometriosis from the MRC-IEU OpenGWAS platform (https://gwas.mrcieu.ac.uk/). Specifically, we retrieved GWAS data on endometriosis patients with dataset ID ebi-a-GCST90018839. This dataset includes 4,511 cases and 227,260 controls from European samples. The data allowed the estimation of genetic associations between 24089752 SNPs and endometriosis.
2.3 Selection of instrumental variables
SNPs serve as instrumental variables (IVs) in this Mendelian randomization (MR) analysis aimed at assessing the causal relationship between exposure and outcome (40). The selection of IVs in this study must meet three key assumptions: (1) IVs have a strong correlation with exposure (e.g. immune cell characteristics); (2) IVs are not directly associated with outcome (e.g. endometriosis); (3) IVs are independent of confounding variables.
Following current research standards, and allowing for the possibility of missing some immune cells due to overly high thresholds, SNPs significantly associated with immune cells (P < 1×10^-5) were selected from the GWAS summary data for further analysis (41–44). Parameters such as r^2 = 0.001 and kb=10000 were set to eliminate the influence of linkage disequilibrium in the analysis. To reduce bias and eliminate weak instrumental variables, only SNPs with an F-statistic greater than 10 were retained for subsequent analysis. SNPs potentially associated with confounding factors were identified and excluded using PhenoScanner V2 (http://www.phenoscanner.medschl.cam.ac.uk/).
2.4 Statistical analysis
The Inverse Variance Weighted (IVW) method is widely regarded as the standard approach for performing MR analysis. The IVW method assumes that all instrumental variables are valid. Its principle is to aggregate the Wald ratio estimates for each instrumental variable to estimate causality. If heterogeneity is detected, a random effects model is used, otherwise a fixed effects model is used (45). The weighted median method (WME) requires that more than 50% of the instrumental variables correspond to true SNPs. Compared to other methods, the weighted median method requires a smaller sample size and guarantees less bias and a lower type I error rate (46).
To estimate causal effects in MR analysis, this study used both the WME and IVW methods. If both methods give results of P < 0.05, this is considered to indicate a direct causal relationship, thus ensuring a high level of confidence in the results. All analyses were performed using the TwoSampleMR package within R version 4.3.1.
2.5 Sensitivity analysis
Sensitivity analysis is of paramount importance in Mendelian randomization studies to identify and address potential pleiotropy. In this study, sensitivity analyses were performed using the Cochran Q test, MR-PRESSO, MR-Egger and leave-one-out methods. The presence of heterogeneity in the instrumental variables was assessed using the Cochran Q test and the MR-PRESSO global heterogeneity test, with P > 0.05 indicating no heterogeneity. The multi-effect test was performed using the MR-Egger regression method, and the intercept term P<0.05 indicates horizontal pleiotropy. The leave-one-out method involved systematically removing each SNP in turn and then recalculating the results using the remaining SNPs. This procedure was used to assess whether the effect of individual SNPs disproportionately influenced the association.
2.6 Reverse MR analysis
Research on endometriosis as an Exposure Factor and the immune cells as the Outcome. According to the screening criteria (p = 5e-08, r2 = 0.001, kb = 10000), SNPs significantly associated with endometriosis were selected. The reverse causal relationship between endometriosis and immune cells was then analyzed using the IVW, MR-Egger, WME, WM and Simple Mode methods.
3 Results
3.1 Exploration of the causal effect of immunophenotypes on EMS
To investigate the causal effects of 731 immune cells on endometriosis, a two-sample MR analysis was performed. The IVW method identified a total of 19 immune cells associated with the onset of endometriosis (Figure 2). After further screening, only four immune cells remained that met the criteria of P < 0.05 in both the WME and IVW methods (Figure 3).
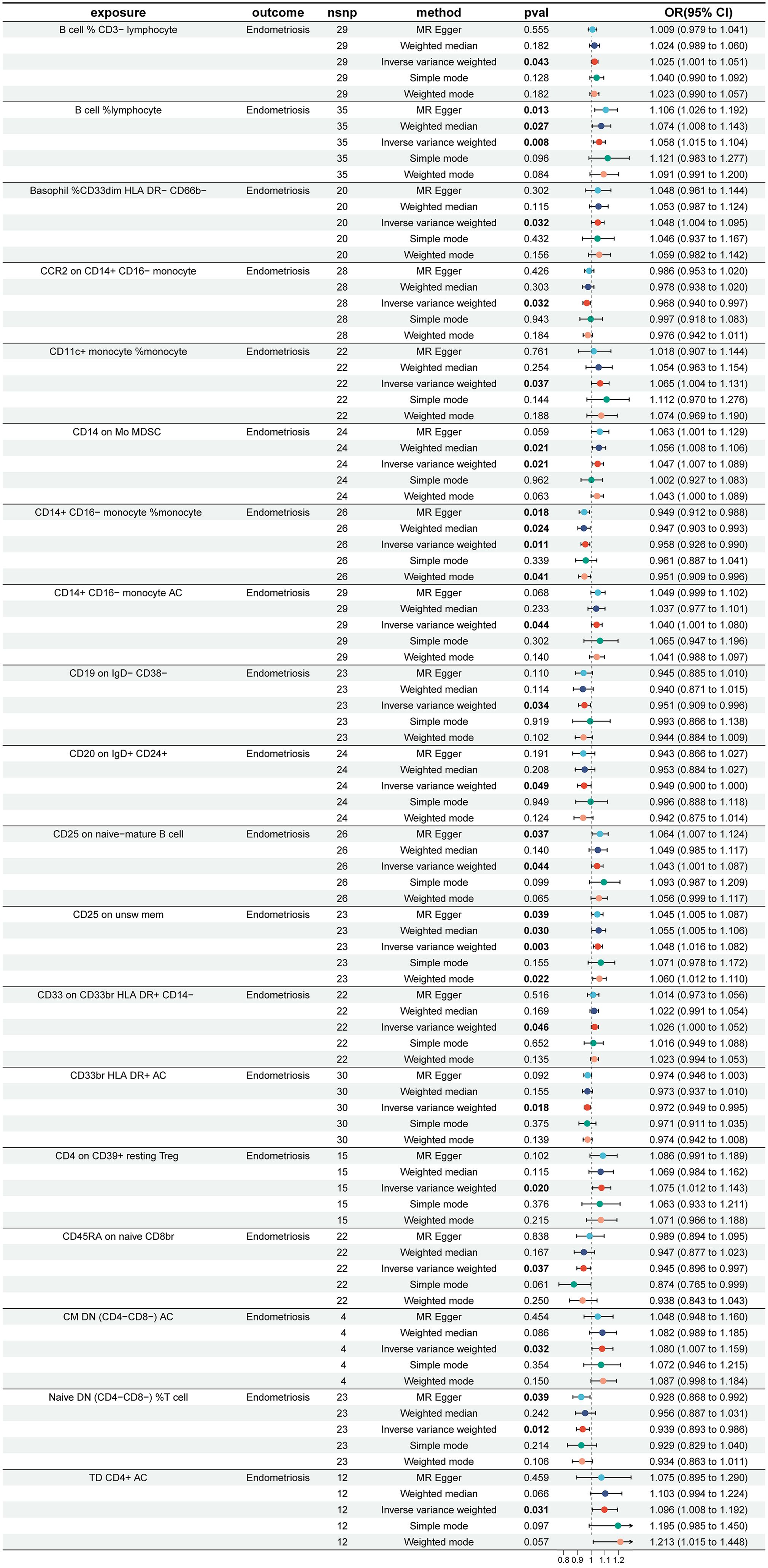
Figure 2 Impact of 19 Immune Cells on EMS (IVW: p < 0.05). nsnp, number of single nucleotide polymorphisms; OR, odds ratio; CI, confidence interval.
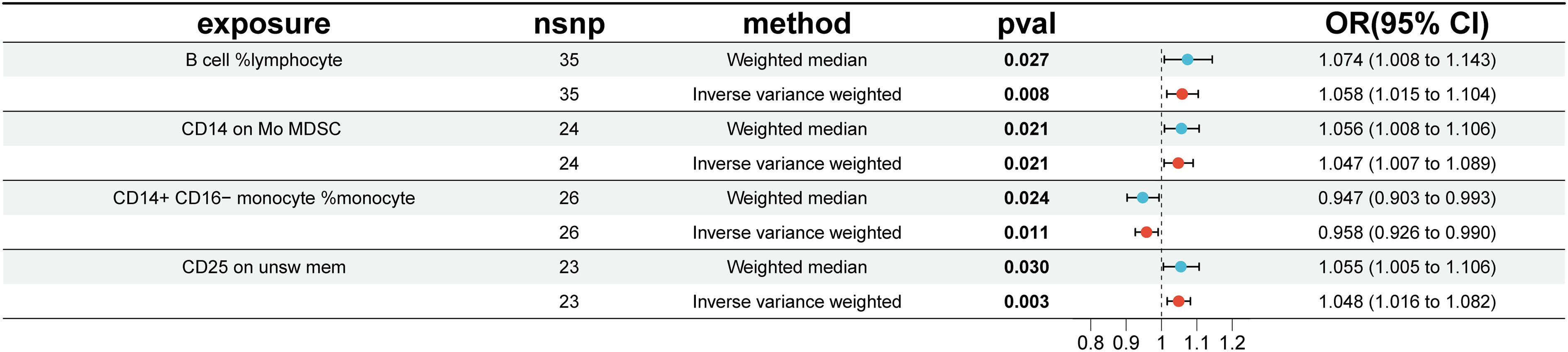
Figure 3 Impact of 4 Immune Cells on EMS (WME and IVW: p < 0.05). nsnp, number of single nucleotide polymorphisms; OR, odds ratio; CI, confidence interval.
The WME and IVW MR analyses identified four immunological features significantly associated with the presence of endometriosis: B cell %lymphocyte (WME: OR: 1.074, p = 0.027 and IVW: OR: 1.058, p = 0.008), CD14 on Mo MDSC (WME: OR: 1.056, p =0.021 and IVW: OR: 1.047, p = 0.021), CD14+ CD16− monocyte %monocyte (WME: OR: 0.947, p = 0.024 and IVW: OR: 0.958, p = 0.011), CD25 on unsw mem (WME: OR: 1.055, p = 0.030 and IVW: OR: 1.048, p = 0.003). As shown in the scatter plot, the characteristics (e.g. B cell %lymphocyte, CD14 on Mo MDSC and CD25 on unsw mem) were positively associated with EMS(Figures 4A, B, D), whereas the characteristics (i.e. CD14+ CD16- monocyte %monocyte) was negatively associated with EMS (Figures 4C).
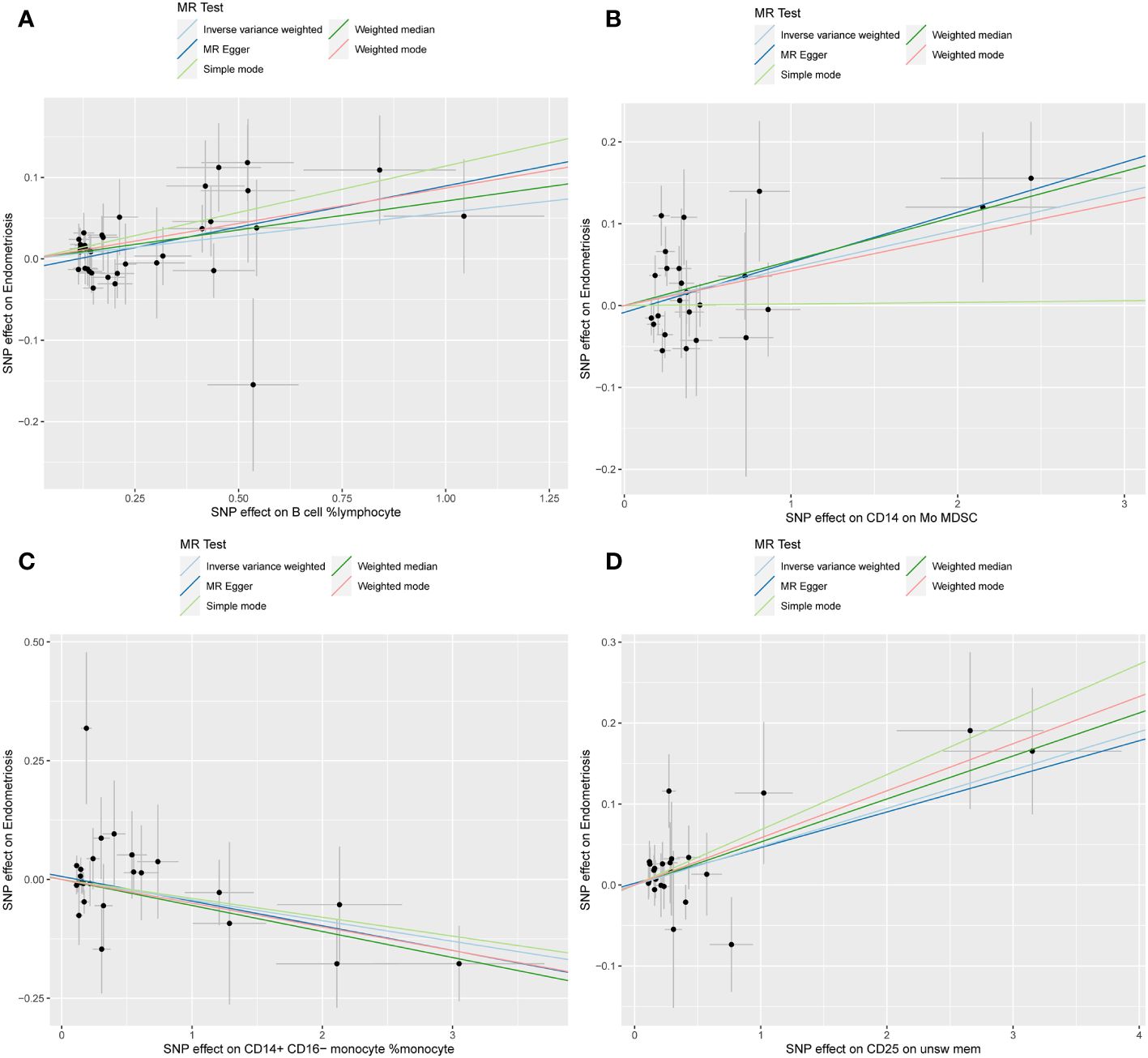
Figure 4 Scatter plot illustrating the relationship between the SNP effect size of causal immune traits (x-axis) and the corresponding effect size estimates of EMSs (y-axis). (A) B cell %lymphocyte, (B) CD14 on Mo MDSC, (C) CD14+ CD16− monocyte %monocyte, (D) CD25 on unsw mem.
3.2 Sensitivity analysis
Cochran Q test results indicated no heterogeneity between SNPs (Supplementary Table 1). The result of the MR-Egger intercept test was that horizontal pleiotropy had no effect on the MR analysis results (Supplementary Table 2). The funnel plot shows a symmetrical distribution on both sides. This indicates that there is no bias in the results of the MR analysis (Figure 5). There were no outlier SNPs in the MR-PRESSO global test results (Supplementary Table 3). Sensitivity analysis using the leave-one-out method showed that the results of the MR analysis were not influenced by individual SNPs (Figure 6).
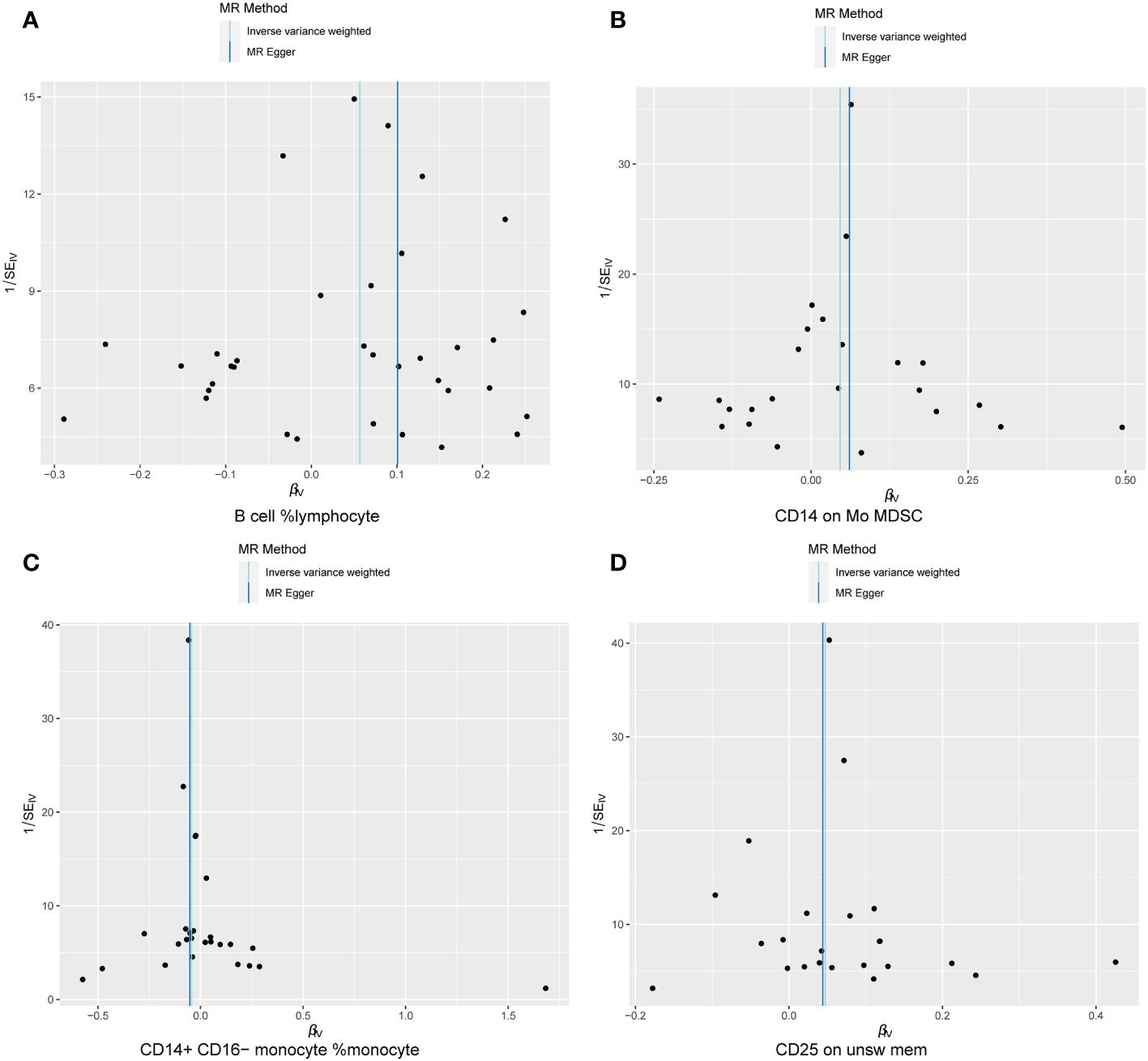
Figure 5 Sensitivity results Funnel plot. (A) B cell %lymphocyte, (B) CD14 on Mo MDSC, (C) CD14+ CD16− monocyte %monocyte, (D) CD25 on unsw mem.
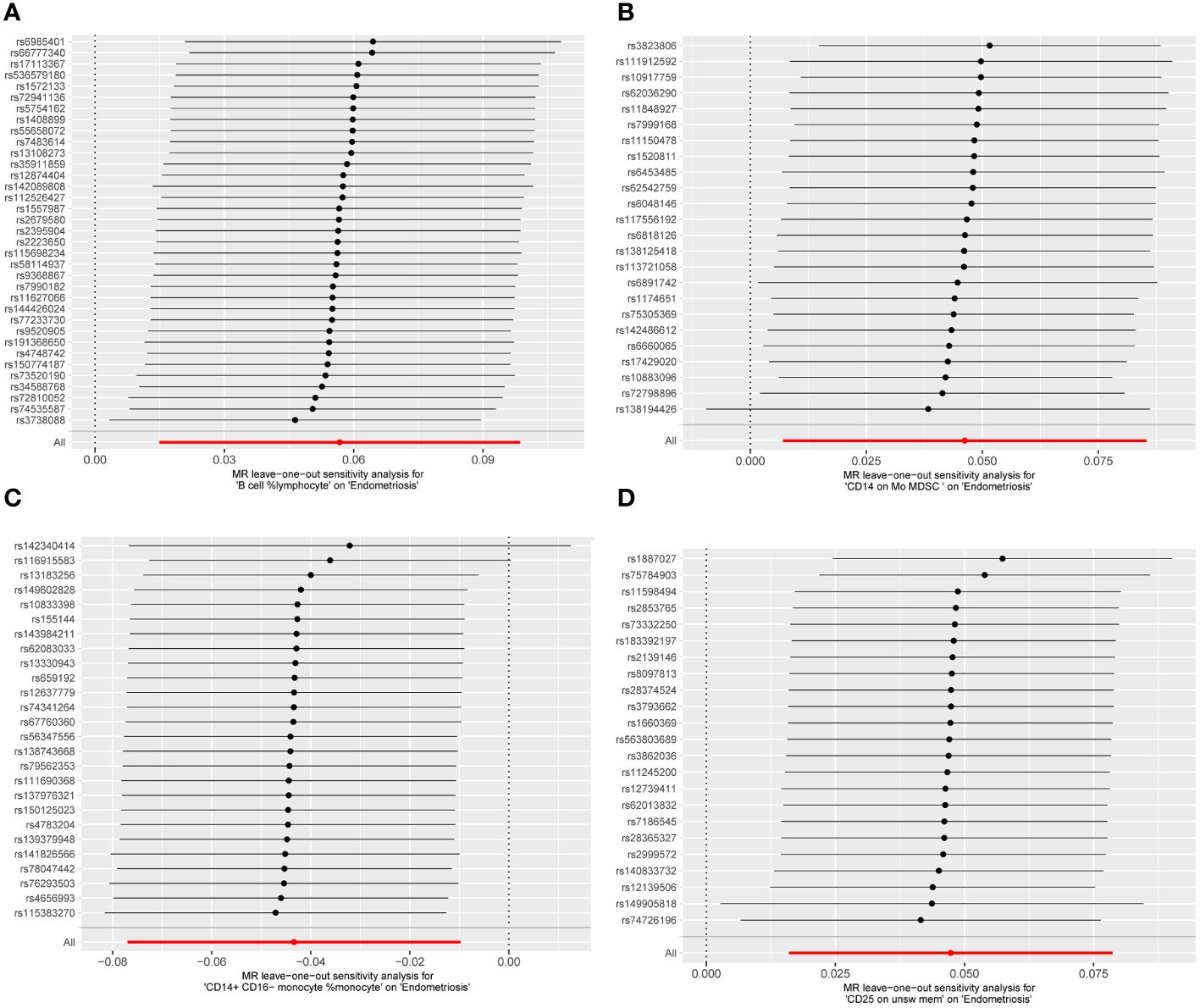
Figure 6 MR leave−one−out sensitivity analysis. (A) B cell %lymphocyte, (B) CD14 on Mo MDSC, (C) CD14+ CD16− monocyte %monocyte, (D) CD25 on unsw mem.
3.3 Causal Effects of EMS on the four immune cells
In the reverse causal analysis, none of the five MR analysis methods suggested a significant causal relationship between EMS and the four immune cells (P>0.05) (Figure 7). The remaining 15 immune cells identified by the IVW method showed only one with a reverse causal relationship with endometriosis: CD11c+ monocyte %monocyte (IVW: OR: 1.271, p = 0.004) (Supplementary PDF1).
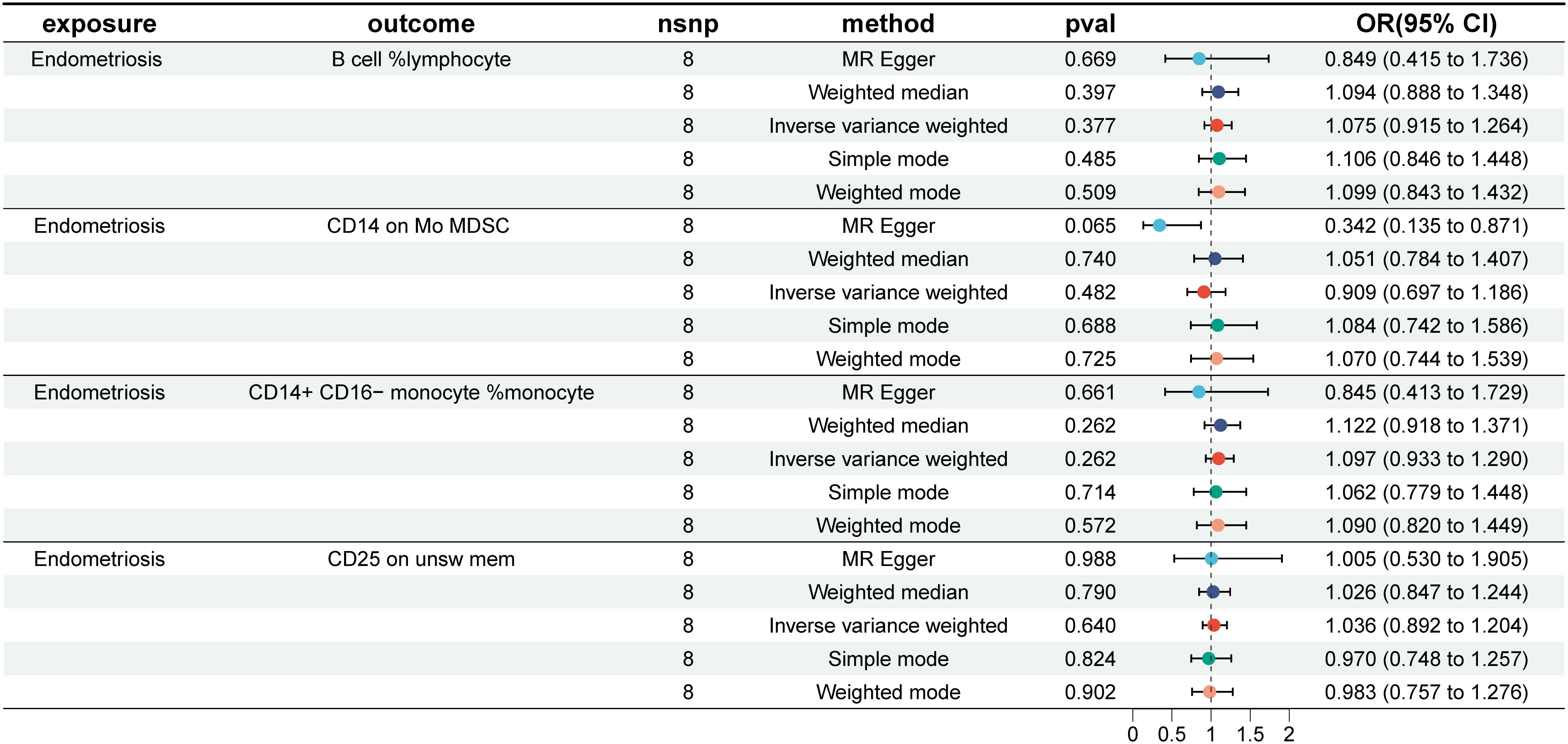
Figure 7 EMS and the four immune cells. nsnp, number of single nucleotide polymorphisms; OR, odds ratio; CI, confidence interval.
4 Discussion
We investigated the causal relationships between 731 immune cell traits and endometriosis using large, publicly available genetic datasets. In this study, the IVW method identified 19 immune cells, while the combined WME method identified four immune phenotypes that were significantly associated with EMS causality: ‘B cell %lymphocyte’, ‘CD14 on Mo MDSC’, ‘CD14+ CD16- monocyte %monocyte’, and ‘CD25 on unsw mem’. However, there is no reverse causal relationship between endometriosis and these four immune cells.
Our results suggest a significant correlation between two types of B cells, ‘B cell %lymphocyte’ and ‘CD25 on unsw mem’, and an increased risk of endometriosis.
‘B cell %lymphocyte’ is the proportion of B cells in the total number of lymphocytes. Lymphocytic immune cells are essential for endometrial cells to survive and proliferate (47). A systematic review shows that the majority of studies have documented an increase in both the number and activity of B cells in the peripheral blood, endometrial tissue or peritoneal fluid of people with endometriosis (16). In addition, Andrew J. Shih et al. performed single-cell RNA sequencing (scRNA-Seq) analysis comparing endometrial tissue obtained from freshly collected menstrual fluid (MF) samples from 33 subjects. They found a significant increase in B cells in the shed endometrium of individuals diagnosed with endometriosis (p = 5.8 × 10–6) (48). Studies by Nothnick et al. have shown elevated serum levels of autoantibodies of varying specificity, including anti-endometrial and antisperm antibodies, in women diagnosed with endometriosis (49). The dysfunctional behavior of B lymphocytes in endometriosis is characterized by an increase in the production and quantity of antibodies, particularly autoantibodies, which can lead to immune evasion by endometrial cells, thereby accelerating disease progression (50). Antsiferova et al. used peripheral blood or uterine endometrial lymphocytes from healthy women as controls and observed a significant increase in the amount of pan-B cells, especially B lymphocytes of the B-1 subset, and the level of activated B lymphocytes in the ectopic endometrium (50). B-1 cells, derived from fetal B lymphocytes, have unique developmental and functional characteristics. They demonstrate the ability to generate natural, polyreactive antibodies that are critical for maintaining tissue homeostasis and enhancing immune defense (51). The increased production of autoantibodies resulting from B-1 cell activation may serve as a mechanism to facilitate evasion of immune surveillance by endometrial cells. A key feature of B-1 cells is their synthesis of low-affinity poly-reactive immunoglobulins, which have the ability to recognize a wide range of autoantigens and show cross-reactivity with many bacterial antigens, including polysaccharides and lipopolysaccharides (52). Lebovic et al. suggested that anti-endometrial autoantibodies may mask the antigenic determinants of endometrial cells, potentially shielding them from immune cell attack (53).
‘CD25 on unsw mem’ means that CD25 is expressed on non-switched memory B cells. Switched memory B cells originate from the germinal center and consist of isotype-switched IgG, IgA, IgE and pre-switched IgM+ only cells. Conversely, non-switched memory B cells are antigen-experienced B cells expressing either IgM+IgD+ or the smaller subset expressing only IgD+ (IgM-) (54). B cells expressing CD25 spontaneously secrete immunoglobulins of the IgA, IgG and IgM subclasses and have an enhanced migratory capacity compared to CD25(-) B cells (55). The increased migratory capacity of endometrial stromal cells (ESCs) is a fundamental determinant in the development of functional endometrium-like tissue outside the uterine cavity in EMS (56).
In an experimental rat model of endometriosis, Dogan et al. observed a significant reduction in the volume of endometriotic implants following treatment with Rituximab, a B-cell antibody (57). Although several studies have demonstrated aberrant production of endometrial autoantibodies in endometriosis, there is no consensus about the concentration of B cells (in eutopic and ectopic endometrium, circulating blood and/or peritoneal fluid) and their roles in this disorder (58). Future studies of ‘B cell %lymphocytes’ and ‘CD25 on unsw mem’ may provide additional insights into the pathogenesis of endometriosis and facilitate consensus in this field.
Our results also showed a positive correlation between increased levels of ‘CD14 on Mo MDSC’ and increased risk of EMS, while an increase in ‘CD14+ CD16- monocyte %monocyte’ was inversely associated with EMS risk.
Zhang et al. found that the number of myeloid-derived suppressor cells (MDSCs) decreases in human patients after laparoscopic surgery, while depletion of MDSCs in mouse models significantly reduces endometriotic lesions and adoptive transfer of MDSCs restores lesion growth. This suggests a proactive recruitment process of MDSCs during endometriosis, which may promote lesion survival and progression (59). Researchers identify the lack of definitive markers for human MDSCs as a major hurdle, contributing to delays in characterizing and conducting in situ studies of this complex immunosuppressive population (60). The ‘CD14 on Mo MDSC’ subset of MDSCs may serve as a focal point for future research efforts, facilitating a deeper understanding of the pathogenic mechanisms underlying endometriosis.
‘CD14+ CD16- monocyte %monocyte’ indicates the proportion of CD14+ CD16- monocytes in the total monocyte population. In healthy people, about 90% of monocytes are characterized as being CD14 positive and CD16 negative, known as CD14+CD16- classical monocytes (61). In inflammatory contexts, classical monocytes migrate into tissues where they differentiate into either macrophages or dendritic cells (62). In this capacity, they perform various functions, including the removal of apoptotic bodies, the promotion of angiogenesis and the restoration of tissue integrity, thereby contributing to the reduction of lesions (63). Research by Hogg et al. shows that endometriosis triggers a sustained recruitment of monocytes into the peritoneal cavity and an increased influx of monocytes into the large peritoneal macrophage (LpM) reservoir. In this context, monocyte-derived LpMs have been observed to exert a protective influence against the progression of endometriosis lesions (64). Although ‘CD14+ CD16- monocyte %monocyte’ has rarely been reported in endometriosis, our study results provide new insights into the differentiation of monocytes into macrophages and the activation of monocytes in endometriosis, thus providing valuable guidance for future research efforts.
Our study used two-sample MR analysis, drawing on extensive GWAS datasets of approximately 231771 individuals, ensuring robust statistical power. The study’s conclusions relied on genetic instrumental variables with a predefined threshold of P<0.05 for both WME and IVW MR analysis methods, aiming to enhance result robustness against potential issues like horizontal pleiotropy and other confounding factors. The identification of four immune cell types (‘B cell %lymphocyte’, ‘CD14 on Mo MDSC’, ‘CD14+ CD16- monocyte %monocyte’, and ‘CD25 on unsw mem’) elucidated the interaction patterns between the immune system and endometriosis and provided additional valuable data on the immune environment surrounding the complex pathogenic molecular mechanisms of endometriosis. Our findings suggest the potential for integrating checkpoint inhibitors with strategies targeting B cells and MDSC in future immunotherapy studies for endometriosis. However, this study also has several limitations. First, the two-sample MR analysis was based on summary data from the GWAS, which lacked detailed demographic information and clinical characteristics of the participants, precluding subsequent subgroup analyses. As a result, we were unable to explore potential variations in the causal relationship between immune cells and endometriosis across different phases of the menstrual cycle. Secondly, the predominantly European origin of the study sample limits the generalizability of the findings to other populations. Future research could include clinical trials in different countries to achieve more precise immunotherapy interventions.
5 Conclusions
In summary, our MR analysis provides robust evidence for a causal link between immune cells and susceptibility to EMS. This finding holds great promise for informing clinical decisions regarding disease prognosis and treatment modalities, while also paving the way for novel drug development efforts.
Data availability statement
The datasets presented in this study can be found in online repositories. The names of the repository/repositories and accession number(s) can be found in the article/Supplementary Material.
Author contributions
XF: Conceptualization, Validation, Writing – original draft. QD: Conceptualization, Writing – original draft. HY: Conceptualization, Writing – original draft. ZY: Data curation, Writing – original draft. ZP: Formal Analysis, Writing – original draft. YZ: Investigation, Writing – original draft. TL: Methodology, Writing – original draft. ZT: Project administration, Writing – original draft. JL: Software, Writing – original draft. LL: Software, Writing – review & editing. HH: Resources, Writing – review & editing. LZ: Funding acquisition, Writing – review & editing.
Funding
The author(s) declare financial support was received for the research, authorship, and/or publication of this article. The research was supported by the Special Funds for Public Welfare Research and Capacity Building of Guangdong Province, China (No. 2015A080803009).
Conflict of interest
The authors declare that the research was conducted in the absence of any commercial or financial relationships that could be construed as a potential conflict of interest.
Publisher’s note
All claims expressed in this article are solely those of the authors and do not necessarily represent those of their affiliated organizations, or those of the publisher, the editors and the reviewers. Any product that may be evaluated in this article, or claim that may be made by its manufacturer, is not guaranteed or endorsed by the publisher.
Supplementary material
The Supplementary Material for this article can be found online at: https://www.frontiersin.org/articles/10.3389/fendo.2024.1397670/full#supplementary-material
References
1. Kennedy S, Bergqvist A, Chapron C, Hooghe T, Dunselman G, Greb R, et al. ESHRE guideline for the diagnosis and treatment of endometriosis. Hum Reprod. (2005) 20:2698–704. doi: 10.1093/humrep/dei135
2. Santanam N, Kavtaradze N, Murphy A, Dominguez C, Parthasarathy S. Antioxidant supplementation reduces endometriosis-related pelvic pain in humans. Trans Res. (2013) 161:189–95. doi: 10.1016/j.trsl.2012.05.001
3. Guo SW. Epigenetics of endometriosis. Mol Hum Reprod. (2009) 15:587–607. doi: 10.1093/molehr/gap064
4. Barbosa CP, De Souza AMB, Bianco B, Christofolini DM. The effect of hormones on endometriosis development. Minerva Ginecol. (2011) 63:375–86.
5. Carmona F, Martínez-Zamora A, Bassols ML, Balasch J. Environmental influences on the development of endometriosis. J Endometriosis Pelvic Pain Disord. (2013) 5:49–61. doi: 10.5301/je.5000153
6. Jiang Y, Chai X, Chen S, Chen Z, Tian H, Liu M, et al. Exosomes from the uterine cavity mediate immune dysregulation via inhibiting the JNK signal pathway in endometriosis. Biomedicines. (2022) 10:3110. doi: 10.3390/biomedicines10123110
7. Manieri Rocha R, Leonardi M, Eathorne A, Eathorne A, Armour M, Condous G. Anatomical distribution of endometriosis: A cross-sectional analysis of transvaginal ultrasound in symptomatic patients. Australas J ultrasound Med. (2023) 26:131–41. doi: 10.1002/ajum.12327
8. Carli C, Metz CN, Al-Abed Y, Naccache PH, Akoum A. Up-regulation of cyclooxygenase-2 expression and prostaglandin E2 production in human endometriotic cells by macrophage migration inhibitory factor: involvement of novel kinase signaling pathways. Endocrinology. (2009) 150:3128–37. doi: 10.1210/en.2008-1088
9. Matarese G, De Placido G, Nikas Y, Alviggi C. Pathogenesis of endometriosis: natural immunity dysfunction or autoimmune disease? Trends Mol Med. (2003) 9:223–8. doi: 10.1016/S1471-4914(03)00051-0
10. Badawy SZA, Cuenca V, Kaufman L, Stitzel A, Thompson M. The regulation of immunoglobulin production by B cells in patients with endometriosis. Fertil steril. (1989) 51:770–3. doi: 10.1016/S0015-0282(16)60664-7
11. Szukiewicz D. Epigenetic regulation and T-cell responses in endometriosis–something other than autoimmunity. Front Immunol. (2022) 13:943839. doi: 10.3389/fimmu.2022.943839
12. He J, Xu Y, Yi M, Gu C, Zhu Y, Hu G. Involvement of natural killer cells in the pathogenesis of endometriosis in patients with pelvic pain. J Int Med Res. (2020) 48:0300060519871407. doi: 10.1177/0300060519871407
13. Qiaomei Z, Ping W, Yanjing Z, Jinhua W, Shaozhan C, Lihong C. Features of peritoneal dendritic cells in the development of endometriosis. Reprod Biol Endocrinol. (2023) 21:4. doi: 10.1186/s12958-023-01058-w
14. Sari FM, Mirkalantari S, Nikoo S, Sepahvand F, Allahqoli L, Asadi A, et al. Potential of Lactobacillus acidophilus to modulate cytokine production by peripheral blood monocytes in patients with endometriosis. Iran J Microbiol. (2022) 14:698. doi: 10.18502/ijm.v14i5.10965
15. Hogg C, Horne AW, Greaves E. Endometriosis-associated macrophages: origin, phenotype, and function. Front Endocrinol. (2020) 11:507486. doi: 10.3389/fendo.2020.00007
16. Riccio LGC, Baracat EC, Chapron C, Batteux F, Abrão MS. The role of the B lymphocytes in endometriosis: a systematic review. J Reprod Immunol. (2017) 123:29–34. doi: 10.1016/j.jri.2017.09.001
17. Yang H, Zhuang Y. The deviations of CD4+ T cells during peripheral blood and peritoneal fluid of endometriosis: a systematic review and meta-analysis. Arch Gynecol Obstet. (2023) 308:1431–46. doi: 10.1007/s00404-023-06964-3
18. Thiruchelvam U, Wingfield M, O’Farrelly C. Natural killer cells: key players in endometriosis. Am J Reprod Immunol. (2015) 74:291–301. doi: 10.1111/aji.12408
19. Funamizu A, Fukui A, Kamoi M, Fuchinoue K, Yokota M, Fukuhara R, et al. Expression of natural cytotoxicity receptors on peritoneal fluid natural killer cell and cytokine production by peritoneal fluid natural killer cell in women with endometriosis. Am J Reprod Immunol. (2014) 71:359–67. doi: 10.1111/aji.12206
20. Jeung IC, Cheon K, Kim MR. Decreased cytotoxicity of peripheral and peritoneal natural killer cell in endometriosis. BioMed Res Int. (2016) 2016:2916070. doi: 10.1155/2016/2916070
21. Ho HN, Chao KH, Chen HF, Wu MY, Yang YS, Lee TY, et al. Peritoneal natural killer cytotoxicity and CD25+ CD3+ lymphocyte subpopulation are decreased in women with stage III–IV endometriosis. Hum Reprod. (1995) 10:2671–5. doi: 10.1093/oxfordjournals.humrep.a135765
22. Oosterlynck DJ, Meuleman C, Lacquet FA, Waer M, Koninckx PR. Flow cytometry analysis of lymphocyte subpopulations in peritoneal fluid of women with endometriosis. Am J Reprod Immunol. (1994) 31:25–31. doi: 10.1111/j.1600-0897.1994.tb00843.x
23. Laginha PA, Arcoverde FVL, Riccio LGC, Andres MP, Abrao MS. The role of dendritic cells in endometriosis: A systematic review. J Reprod Immunol. (2022) 149:103462. doi: 10.1016/j.jri.2021.103462
24. Hey-Cunningham AJ, Wong C, Hsu J, Fromm PD, Clark GJ, Kupresanin F, et al. Comprehensive analysis utilizing flow cytometry and immunohistochemistry reveals inflammatory changes in local endometrial and systemic dendritic cell populations in endometriosis. Hum Reprod. (2021) 36:415–28. doi: 10.1093/humrep/deaa318
25. Stanic AK, Kim M, Styer AK, Rueda BR. Dendritic cells attenuate the early establishment of endometriosis-like lesions in a murine model. Reprod Sci. (2014) 21:1228–36. doi: 10.1177/1933719114525267
26. Fainaru O, Adini A, Benny O, Adini I, Short S, Bazinet L, et al. Dendritic cells support angiogenesis and promote lesion growth in a murine model of endometriosis. FASEB J. (2008) 22:522–9. doi: 10.1096/fj.07-9034com
27. Pencovich N, Luk J, Hantisteanu S, Hornstein MD, Fainaru O. The development of endometriosis in a murine model is dependent on the presence of dendritic cells. Reprod BioMed Online. (2014) 28:515–21. doi: 10.1016/j.rbmo.2013.12.011
28. Na YJ, Jin JO, Lee MS, Song MG, Lee KS, Kwak JY. Peritoneal fluid from endometriosis patients switches differentiation of monocytes from dendritic cells to macrophages. J Reprod Immunol. (2008) 77:63–74. doi: 10.1016/j.jri.2007.03.013
29. Lukács L, Kovács AR, Pál L, Szűcs S, Kövér Á, Lampé R. Phagocyte function of peripheral neutrophil granulocytes and monocytes in endometriosis before and after surgery. J Of Gynecol Obstet And Hum Reprod. (2021) 50:101796. doi: 10.1016/j.jogoh.2020.101796
30. Bacci M, Capobianco A, Monno A, Cottone L, Di Puppo F, Camisa B, et al. Macrophages are alternatively activated in patients with endometriosis and required for growth and vascularization of lesions in a mouse model of disease. Am J Pathol. (2009) 175:547–56. doi: 10.2353/ajpath.2009.081011
31. Wang Y, Fu Y, Xue S, Ai A, Chen H, Lyu Q, et al. The M2 polarization of macrophage induced by fractalkine in the endometriotic milieu enhances invasiveness of endometrial stromal cells. Int J Clin Exp Pathol. (2014) 7:194.
32. Takebayashi A, Kimura F, Kishi Y, Ishida M, Takahashi A, Yamanaka A, et al. Subpopulations of macrophages within eutopic endometrium of endometriosis patients. Am J Reprod Immunol. (2015) 73:221–31. doi: 10.1111/aji.12331
33. Vallvé-Juanico J, Santamaria X, Vo KC, Houshdaran S, Giudice LC. Macrophages display proinflammatory phenotypes in the eutopic endometrium of women with endometriosis with relevance to an infectious etiology of the disease. Fertil steril. (2019) 112:1118–28. doi: 10.1016/j.fertnstert.2019.08.060
34. Braun DP, Ding J, Shen J, Rana N, Fernandez BB, Dmowski WP. Relationship between apoptosis and the number of macrophages in eutopic endometrium from women with and without endometriosis. Fertil steril. (2002) 78:830–5. doi: 10.1016/S0015-0282(02)03334-4
35. Khan KN, Masuzaki H, Fujishita A, Kitajima M, Sekine I, Ishimaru T. Differential macrophage infiltration in early and advanced endometriosis and adjacent peritoneum. Fertil steril. (2004) 81:652–61. doi: 10.1016/j.fertnstert.2003.07.037
36. Berbic M, Schulke L, Markham R, Tokushige N, Russell P, Fraser IS. Macrophage expression in endometrium of women with and without endometriosis. Hum Reprod. (2009) 24:325–32. doi: 10.1093/humrep/den393
37. Davies NM, Holmes MV, Smith GD. Reading Mendelian randomization studies: a guide, glossary, and checklist for clinicians. BMJ. (2018) 362:k601. doi: 10.1136/bmj.k601
38. Hemani G, Bowden J, Davey Smith G. Evaluating the potential role of pleiotropy in Mendelian randomization studies. Hum Mol Genet. (2018) 27:R195–208. doi: 10.1093/hmg/ddy163
39. Orrù V, Steri M, Sidore C, Marongiu M, Serra V, Olla S, et al. Complex genetic signatures in immune cells underlie autoimmunity and inform therapy. Nat Genet. (2020) 52:1036–45. doi: 10.1038/s41588-020-0684-4
40. Burgess S, Foley CN, Allara E, Staley JR, Howson JMM. A robust and efficient method for Mendelian randomization with hundreds of genetic variants. Nat Commun. (2020) 11:376. doi: 10.1038/s41467-019-14156-4
41. Wang A, Zhang J. Causal role of immune cells in psoriasis: a Mendelian randomization analysis. Front Immunol. (2024) 15:1326717. doi: 10.3389/fimmu.2024.1326717
42. Wang Y, Zhou C, Wang D, Ma J, Sun XH, Wang Y, et al. Unraveling the causal role of immune cells in gastrointestinal tract cancers: insights from a Mendelian randomization study. Front Immunol. (2024) 15:1343512. doi: 10.3389/fimmu.2024.1343512
43. Gu J, Qiao Y, Cong S. Causal role of immune cells on risk of Parkinson’s disease: a Mendelian randomization study. Front Aging Neurosci. (2024) 16:1368374. doi: 10.3389/fnagi.2024.1368374
44. Wang C, Zhu D, Zhang D, Zuo X, Yao L, Liu T, et al. Causal role of immune cells in schizophrenia: Mendelian randomization (MR) study. BMC Psychiatry. (2023) 23:590. doi: 10.1186/s12888-023-05081-4
45. Burgess S, Butterworth A, Thompson SG. Mendelian randomization analysis with multiple genetic variants using summarized data. Genet Epidemiol. (2013) 37:658–65. doi: 10.1002/gepi.21758
46. Bowden J, Davey Smith G, Haycock PC, Burgess S. Consistent estimation in Mendelian randomization with some invalid instruments using a weighted median estimator. Genet Epidemiol. (2016) 40:304–14. doi: 10.1002/gepi.21965
47. Klein NA, Montoya IA, Schenken RS. Characterization of the resident lymphoid cell population in endometriosis. Soc Gynecol Invest Abst. (1992) 518.
48. Shih AJ, Adelson RP, Vashistha H, Khalili H, Nayyar A, Puran R, et al. Single-cell analysis of menstrual endometrial tissues defines phenotypes associated with endometriosis. BMC Med. (2022) 20:315. doi: 10.1186/s12916-022-02500-3
49. Nothnick WB. Treating endometriosis as an autoimmune disease. Fertil steril. (2001) 76:223–31. doi: 10.1016/S0015-0282(01)01878-7
50. Antsiferova YS, Sotnikova NY, Posiseeva LV, Shor AL. Changes in the T-helper cytokine profile and in lymphocyte activation at the systemic and local levels in women with endometriosis. Fertil steril. (2005) 84:1705–11. doi: 10.1016/j.fertnstert.2005.05.066
51. Baumgarth N. The double life of a B-1 cell: self-reactivity selects for protective effector functions. Nat Rev Immunol. (2011) 11:34–46. doi: 10.1038/nri2901
52. Murakami M, Honjo T. Involvement of B-1 cells in mucosal immunity and autoimmunity. Immunol Today. (1995) 16:534–9. doi: 10.1016/0167-5699(95)80047-6
53. Lebovic DI, Mueller MD, Taylor RN. Immunobiology of endometriosis. Fertil steril. (2001) 75:1–10. doi: 10.1016/S0015-0282(00)01630-7
54. Castleman MJ, Santos AL, Lesteberg KE, Maloney JP, Janssen WJ, Mould KJ, et al. Activation and pro-inflammatory cytokine production by unswitched memory B cells during SARS-CoV-2 infection. Front Immunol. (2023) 14:1213344. doi: 10.3389/fimmu.2023.1213344
55. Amu S, Gjertsson I, Brisslert M. Functional characterization of murine CD25 expressing B cells. Scandinavian J Immunol. (2010) 71:275–82. doi: 10.1111/sji.2010.71.issue-4
56. Zhang D, Wang L, Guo HL, Zhang ZW, Wang C, Chian RC, et al. MicroRNA−202 inhibits endometrial stromal cell migration and invasion by suppressing the K−Ras/Raf1/MEK/ERK signaling pathway. Int J Mol Med. (2020) 46:2078–88. doi: 10.3892/ijmm
57. Dogan AC, Dogan M, Togrul C, Ozkan NT. The effects of Rituximab on experimental endometriosis model in rats. J Reprod Immunol. (2023) 156:103814. doi: 10.1016/j.jri.2023.103814
58. Vallvé-Juanico J, Houshdaran S, Giudice LC. The endometrial immune environment of women with endometriosis. Hum Reprod Update. (2019) 25:565–92. doi: 10.1093/humupd/dmz018
59. Zhang T, Zhou J, Man GCW, Leung KT, Liang B, Xiao B, et al. MDSCs drive the process of endometriosis by enhancing angiogenesis and are a new potential therapeutic target. Eur J Immunol. (2018) 48:1059–73. doi: 10.1002/eji.201747417
60. Gabrilovich DI, Bronte V, Chen SH, Colombo MP, Ochoa A, Ostrand-Rosenberg S, et al. The terminology issue for myeloid-derived suppressor cells. Cancer Res. (2007) 67:425–5. doi: 10.1158/0008-5472.CAN-06-3037
61. Calderon TM, Williams DW, Lopez L, Eugenin EA, Cheney L, Gaskill PJ, et al. Dopamine increases CD14+ CD16+ monocyte transmigration across the blood brain barrier: implications for substance abuse and HIV neuropathogenesis. J Neuroimmune Pharmacol. (2017) 12:353–70. doi: 10.1007/s11481-017-9726-9
62. Ginhoux F, Jung S. Monocytes and macrophages: developmental pathways and tissue homeostasis. Nat Rev Immunol. (2014) 14:392–404. doi: 10.1038/nri3671
63. Geissmann F, Manz MG, Jung S, Sieweke MH, Merad M, Ley K. Development of monocytes, macrophages, and dendritic cells. Science. (2010) 327:656–61. doi: 10.1126/science.1178331
Keywords: immune cells, endometriosis, MR analysis, SNP, sensitivity analyses
Citation: Fang X, Deng Q, Yang H, Yan Z, Peng Z, Zhao Y, Liao T, Tu Z, Liu J, Liu L, Zou L and He H (2024) Causal association of immune cells and endometriosis: a Mendelian randomization study. Front. Endocrinol. 15:1397670. doi: 10.3389/fendo.2024.1397670
Received: 08 March 2024; Accepted: 09 May 2024;
Published: 29 May 2024.
Edited by:
Francesca de Michele, Chirec Delta Hospital, BelgiumReviewed by:
Chi Chiu Wang, The Chinese University of Hong Kong, ChinaHao Wang, Shenzhen University General Hospital, China
Copyright © 2024 Fang, Deng, Yang, Yan, Peng, Zhao, Liao, Tu, Liu, Liu, Zou and He. This is an open-access article distributed under the terms of the Creative Commons Attribution License (CC BY). The use, distribution or reproduction in other forums is permitted, provided the original author(s) and the copyright owner(s) are credited and that the original publication in this journal is cited, in accordance with accepted academic practice. No use, distribution or reproduction is permitted which does not comply with these terms.
*Correspondence: Lin Zou, emp4anNAMTYzLmNvbQ==; Honghua He, aGVob25naHVhQGVkbXUuZWR1LmNu
†These authors have contributed equally to this work