- 1Laboratorio de neuro-inmuno-endocrinología testicular, Instituto de Biología y Medicina Experimental (IBYME), Fundación IBYME, Consejo Nacional de Investigaciones Científicas y Técnicas (CONICET), Ciudad de Buenos Aires, Argentina
- 2Biomedical Center Munich (BMC), Cell Biology, Anatomy III, Faculty of Medicine, Ludwig Maximilian University of Munich, Planegg-Martinsried, Germany
Biogenic amines are signaling molecules with multiple roles in the central nervous system and in peripheral organs, including the gonads. A series of studies indicated that these molecules, their biosynthetic enzymes and their receptors are present in the testis and that they are involved in the regulation of male reproductive physiology and/or pathology. This mini-review aims to summarize the current knowledge in this field and to pinpoint existing research gaps. We suggest that the widespread clinical use of pharmacological agonists/antagonists of these signaling molecules, calls for new investigations in this area. They are necessary to evaluate the relevance of biogenic amines for human male fertility and infertility, as well as the potential value of at least one of them as an anti-aging compound in the testis.
1 Introduction
Biogenic amines are best known for their roles as neurotransmitters, namely three catecholamines (dopamine –DA–, norepinephrine –NE–, and epinephrine –Epi–), serotonin (5-HT) and histamine (HA) (1). Furthermore, the biogenic amine melatonin is a neurotransmitter-like compound (2).
Catecholamines in the brain play an important role in the secretion of GnRH and the regulation of the pituitary–testicular axis mainly via α1-adrenergic receptors (3–5). The existence of a descending multi-synaptic, pituitary-independent neural pathway linking the hypothalamus and the testis, which involves, at least in part, activation of β-adrenergic receptors, was reported in the male rat (6). D1- and/or D2-like DA receptors are expressed in GnRH neurons and thereby participate in the regulation of the endocrine function of the testis (3, 7). GnRH neurons also express the HA receptors H1 and H2 (5, 8), and synaptic contacts between the serotoninergic system and GnRH neurons were described (9). Pineal-derived melatonin, through its binding to specific sites in the suprachiasmatic nucleus and the pars tuberalis, and via modulation of the kisspeptin (kiss) signaling, influences GnRH and the adenohypophyseal gonadotropins (10–12).
Besides central actions, biogenic amines play roles in peripheral organs including the male gonad. This short review attempts to summarize available insights into sources, receptors, signaling mechanisms, local actions and the clinical relevance of biogenic amines in the testis. We also pinpoint research gaps, concerning their role in male reproduction. We want to apologize to authors, whose work we do not mention here, due the short format of this mini-review.
2 Biogenic amines in the testis
2.1 Sources of biogenic amines
The main common sources of biogenic amines in peripheral organs are innervation, bloodstream and local production. The testicular sources of the three catecholamines (DA, NE and Epi), 5-HT and HA are described in detail below.
2.1.1 Testicular innervation
Studies revealing the existence of a catecholaminergic and a serotonergic innervation of the testis are summarized in Table 1. In human and non-human primates, adrenergic sympathetic innervation of the testis is documented. In addition, intrinsic neuron-like cell bodies with a bipolar or a multipolar elongated phenotype (19–21) were reported, based on the immunodetection of typical markers such as tyrosine hydroxylase (TH). Age-related differences in the number of neuronal elements were found in the testes of the Rhesus monkey. Neuron-like cell bodies were only detected in immature animals. Nerve fibers were observed at all ages but they were more prominent in prepubertal and pubertal monkeys (19, 20). A significant increase in the number of catecholaminergic fibers and neuron-like cells was described in testicular biopsies of patients with idiopathic infertility (21). These changes were accompanied by increased local immune cells (mast cells and macrophages), possibly hinting to mutual interactions (20, 22, 31, 32). Because in the human testis these immune cells may modulate Sertoli cell activity, androgen production and fibrotic events in the tubular wall (31–35), an interactive network between the testicular catecholaminergic input and the local immune system may exist.
Of note, the mentioned studies focus on the catecholaminergic nature of testicular nerve fibers. Yet, in catecholaminergic nerve fibers of the human testis several co-transmitters exist, including peptides such as the neuropeptide Y (NPY), ATP, as well as others (36–38). Once released, these may have important actions, as documented recently for ATP (39, 40). Yet their specific and potentially species-dependent presence in the testis and the interaction with catecholamines are not well known and remain to be fully explored.
2.1.2 Blood circulatory system as a source of testicular biogenic amines
Catecholamines are typical adrenal hormones. In human plasma, resting catecholamine levels range from 30 to 70 pg/ml for Epi, 200 to 300 pg/ml for NE and 25 to 100 pg/ml for DA. Levels increase within minutes upon the onset of stress (41, 42). Specifically, during stress, one can assume that catecholamines can reach the testis in concentrations, which allow interaction with their receptors that were described in different human and rodent somatic cells (22, 43–45).
Blood levels of gastrointestinal tract-derived 5-HT in man range between 200 and 300 ng/ml (42). Via interaction with the serotonergic transporter (SERT) and/or specific testicular receptors described in rodent Leydig, Sertoli and germ cells (spermatogonia and sperm) (43, 46–48), blood-derived 5-HT might also influence testicular processes.
Melatonin, once released from the pineal, is taken up by the testis and modulates testicular function (49–55). In humans, physiological levels of melatonin in the blood range from several pg/ml during the day to more than 50 pg/ml at its nighttime peak (56).
Basal plasma HA levels in humans are normally very low (less than 1 ng/ml) (57) but they significantly rise in anaphylaxis (58). Although the potential impact of blood HA on testicular activity is still controversial, HA receptors and regulatory actions exerted by this biogenic amine were described in the testis (59–61).
2.1.3 Other testicular sources of biogenic amines
Enzymes involved in the biosynthesis of catecholamines and histamine were reported to be expressed in germ cells. Leydig cells express the biosynthetic enzymes of the biogenic amines namely catecholamines, 5-HT, HA and melatonin. Melatonin is also locally produced in peritubular cells of the seminiferous tubules, while testicular immune cells synthesize 5-HT, histamine and melatonin. Table 2 summaries the pertinent reports.
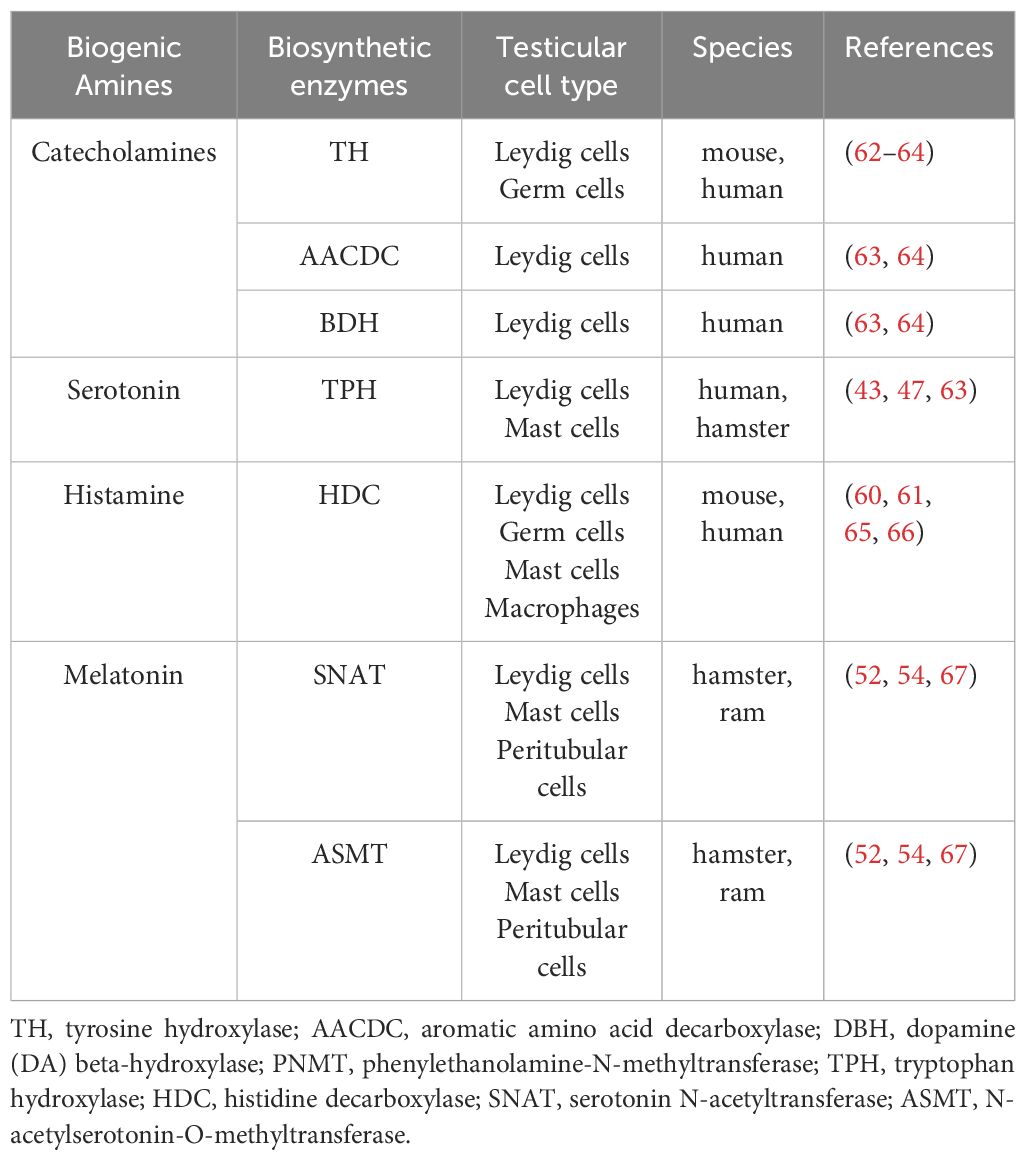
Table 2 Expression of catecholamines, serotonin, histamine and melatonin biosynthetic enzymes in the testis.
Mast cell store HA and 5-HT in secretory granules, and macrophages express the SERT protein and they have been proposed as an alternative source of HA and 5-HT (68, 69).
Testicular mast cell and macrophage numbers change during sexual development and in idiopathic infertility in monkeys and humans, respectively (19–21). Moreover, alterations in the testicular population of immune cells were observed during sexual maturation, aging as well as in the seasonally breeding Syrian hamster (47, 70). Particularly, the increase in mast cells during sexual development was accompanied by higher local levels of 5-HT and its metabolite 5-hydroxyindoleacetic acid in hamster testes (47).
3 Receptors, signaling mechanisms and local actions of biogenic amines in the testis
DA receptors D1 and D2 were reported in mouse and rat Leydig cells and germ cells (62, 71), and DA was implicated in the regulation of size and proliferation of rat Leydig cells (72). While D1 receptors participate in the epigenetic reprogramming, namely histone modifications triggered by cocaine in mice germ cells (73), D2 receptors, via decreased cAMP levels, may regulate spermatogenesis and spermiogenesis in rats (74). In addition, DA presumably via D2 receptors and AKT/PKB phosphorylation, can modulate sperm capacitation, motility and acrosomal integrity in several species (i.e. rat, stallion, boar) (74–76).
In hamsters, Epi and NE stimulate testicular steroidogenesis via α1- and β1-adrenergic receptors (43, 77–79). It was reported that α1/β1-adrenergic receptors interact with local 5-HT2 receptors mediating the response of the testicular corticotropin releasing hormone (CRH) system on cAMP and testosterone production (43). Interestingly, the impact of catecholaminergic stimuli on testicular testosterone production in hamsters exposed to a short-day photoperiod is greater than in gonads of animals kept under a long day photoperiod (77, 79).
In α1b-adrenergic receptor knockout male mice, testicular steroidogenic capacity is affected, Sertoli cell/Leydig cell communication is altered, and disruption of spermatogenesis was reported (80). Stojkov et al. (81) described that oral administration of an α1-adrenergic receptor blocker, mitigated stress induced disturbance of cAMP/cGMP signaling in testosterone-producing rat Leydig cells. An early study from Cohen et al. (82) reported that chronic exposure of the testicular vasculature to NE may cause spermatogenic dysfunction in subfertile men with varicocele. On the contrary, Nagao (83) proposed that Epi and NE may facilitate the in vitro viability of meiotic prophase spermatocytes in rats.
In hamster and human testicular macrophages, β1- and β2-adrenergic receptors were described and Epi and NE participated in the up-regulation of cyclooxygenase 2 (COX2) expression, prostaglandin production and the generation of local inflammatory processes (22). Laser microdissection followed by RT-PCR studies suggested that human testicular peritubular cells (HTPCs) of the wall of the seminiferous tubules express α1B-, α1D-, β1- and β2-adrenergic receptors. Phenylephrine, an α1-adrenergic receptor agonist, increased intracellular Ca2+-levels and induced inflammatory processes in cultured HTPCs (45). Therefore, elevation of catecholamines, for instance during stress, may also be able to promote inflammatory events by targeting these testicular cells.
It was further reported that stress increases testicular synthesis of HA in rats (84, 85). HA receptors H1 and H2 were found in germ cells, Leydig cells, Sertoli cells and peritubular cells of the human testis (60, 61). Human, mouse and rat Leydig cells also express H4 receptors (86–88).
HA regulates testosterone production in the MA-10 mouse Leydig tumor cell line, in primary culture of rat and wall lizard Leydig cells, and in testicular fragments of gonadally regressed hamsters kept in a short photoperiod (59, 87, 89). In histidine decarboxylase (HDC) knockout mice, the steroidogenic capacity of Leydig cells is significantly lower than in wild type mice (89). HA seems to exert a biphasic effect on testicular steroidogenesis in rodents (89). HA-induced stimulation of steroidogenesis seems primarily be mediated via H2 receptors and an increment of cAMP, HA-induced reduction of steroidogenesis may involve H1 receptors, stimulation of the PLC/IP3 pathway and increased nitric oxide synthase (NOS) activity (90). Via H1 and H2 receptors, HA also modulates the diameter of seminiferous tubules, testicular peritubular cells activity as well as sperm count and quality in different species (i.e. mouse, rat, bull, human) (61, 91–96). In testicular macrophages, HA inhibits phagocytosis and superoxide production, at least in the wall lizards (87).
In rodent Leydig cells, 5-HT1A, 5-HT1B and 5-HT2A receptors were found (43, 48, 97), and 5-HT and agonists of the 5-HT1A and 5-HT2A receptor subtypes inhibited cAMP and testosterone production, acting through the local CRH system and testicular α1- and β1-adrenergic receptors (43, 47, 97). 5-HT3A receptors were reported in rat Sertoli cells, spermatogonia and spermatocytes, while 5-HT1B and 5-HT2A receptors were described in spermatogonia and sperm (48). Furthermore, 5-HT seems to be involved in the development of normal spermatogenesis and sperm quality in rats (98–100), as well as in the regulation of testicular blood flow (101).
MT1 and MT2 receptors were reported in mouse, rat, hamster and bovine Sertoli cells (55, 102, 103), where this indoleamine stimulates lactate dehydrogenase (LDH) expression and activity and, consequently, increases intracellular lactate production (55, 102). As recently described, daily oral melatonin supplementation positively regulates testicular expression of LDH (55) in men with idiopathic infertility. Melatonin also increased the levels of the glucose transporter 1 (GLUT1), glucose consumption and acetate production in rat Sertoli cells (102). Moreover, it upregulated pyruvate dehydrogenase E1 alpha subunit (PDHA1) phosphorylation in mouse and hamster Sertoli cells (55), inhibited the pyruvate dehydrogenase complex and increased the levels of pyruvate, which can be used to generate lactate via LDH activity (55).
Of note, melatonin abolishes the insulin-dependent increment of intracellular lactate and alanine, and the decrease of the lactate/alanine ratio in rat Sertoli cells controlling, in consequence, the intracellular redox state (102). In agreement with these results, Rossi et al. (55) reported that melatonin prevents the increase of lipid peroxidation and the decrease of the expression of antioxidant enzymes in hamster Sertoli cells.
Melatonin receptors are detectable in human and rodent testicular mast cells and macrophages (51). While melatonin inhibits proliferation and the expression of pro-inflammatory cytokines and COX2 in testicular macrophages, it increases the expression levels of enzymes of the anti-oxidant system and decreases the generation of reactive oxygen species (ROS) in testicular mast cells. Therefore, melatonin exerts anti-proliferative and anti-inflammatory effects on testicular macrophages and protective effects against oxidative stress on testicular mast cells (51).
In addition, MT1 receptors were identified in Leydig cells (49, 50). In MA-10 mouse Leydig tumor cells, as well as in primary cultures of rat and hamster Leydig cells, melatonin inhibited the production of sex steroid hormones (49, 104, 105). At least in the Syrian hamster, melatonin, through specific MT1 receptors of Leydig cells stimulated CRH production. CRH, via CRH-R1 receptors, activated tyrosine phosphatases leading to reduced phosphorylation of erk 44/42 and jnk 54/46, down-regulation of c-jun and c-fos, inhibition of transcription factors phosphorylation, decreased expression of StAR and finally inhibition of androgen production (43, 49, 50, 52). Melatonin can also regulate the expression of steroidogenic genes by binding to its nuclear receptors RORα in mammalian Leydig cells (106).
Circannual rhythms of circulating and testicular melatonin occur in response to changes of the daily photoperiod. This regulates steroidogenesis and the overall reproductive status of male Syrian hamsters, which are seasonal breeders (49, 107). In this species, the aging-related decrease of the testicular melatonin concentration was related to a diminished androgen production and an increment in inflammatory markers and indicators of oxidative stress (70, 108). Interestingly, daily i.p. injections of melatonin in old hamsters, as well as the transfer of aged animals from a long day to a short-day photoperiod, increased testicular melatonin levels and significantly improved the local inflammatory-oxidant status (70, 108). Particularly, hamster peritubular myoid cells of the tubular wall express MT1 and MT2 receptors, and melatonin regulates immune and inflammatory functions, as well as their contractile capacity (54).
In infertile men with unexplained azoospermia, lower testicular melatonin concentrations were found if testicular morphological abnormalities were present, together with increased macrophage numbers, elevated lipid peroxidation, expression of inflammation-related markers and antioxidant enzymes, as well as tubular wall collagen fibers disorganization and thickening (53). Consequently, testicular melatonin concentrations in biopsies of infertile men were negatively correlated with the gonadal levels of inflammatory markers (51, 53). In these patients, a daily oral supplementation with a 3 mg dose of melatonin, which is currently used to treat sleep disorders, not only increased the testicular levels of this indoleamine but also improved testicular inflammatory-oxidative status, as well as the tubular wall architecture (53). Therefore, melatonin appears to have a positive impact on testicular steroidogenesis and inflammatory-oxidative events.
4 Discussion
The studies mentioned clearly indicate that modulation of testicular functions can result upon interaction of biogenic amines with their target cells, Supplementary graphic summarizes the current knowledge about receptors and functions of biogenic amines in the different cell populations of the testis, However, this narrative also reveals that we only have a rudimentary knowledge of the actions of these signaling molecules in the testis. This has several reasons. For example, most of the investigations stem from earlier years and used traditional methods, including immunohistochemistry, RT-PCR or pharmacological tools (natural biogenic amines, agonists, antagonists). These approaches have obvious limitation as, for example, antibodies in some cases may lack specificity. Lack of specificity is also an issue for pharmacological agonist/antagonists. Furthermore, often isolated cells were examined and systemic studies were rarely performed. The fact that several species and different states of gonadal development or functionality were studied, may explain the sometimes contradictory results. Therefore, the currently limited knowledge about the role of biogenic amines in peripheral tissues calls for focused investigations in (systemic and cellular) models that resemble closely the human. They are required to answer the question, how important biogenic amines are for the male gonad especially in the human.
We believe that new studies trying to answer this question are worthwhile being performed, as biogenic amines, and drugs targeting their biosynthetic pathways and/or receptors are in daily clinical use or are being developed for a variety of conditions, yet their potential testicular consequences are not well known.
Looking at the widespread use of biogenic amines and/or their agonists/antagonists, in man, the importance of better insights becomes clear. In brief, a DA-precursor is used to treat Parkinson’s disease, DA-agonists are used in human prolactinomas, acromegaly, and in type 2 diabetes (109). Epi, NE and agonists of adrenergic receptors are used as vasopressors in acute hypotensive states, as vasoconstrictors to reduce bleeding, and as nasal decongestants, but also in the treatment of anaphylaxis and bronchospasms. Adrenergic receptors antagonists are employed to treat hypertension, congestive heart failure, and some cardiovascular diseases. Inhibitors of catecholamine reuptake are part of several psychiatric treatments and used against musculoskeletal pain (110). 5-HT reuptake inhibitors are prescribed to treat depression (111, 112). Melatonin is prescribed to improve sleep in men suffering from insomnia and jet lag (113). As a consequence of its strong antioxidant action, the therapeutic potential of melatonin was suggested to be extended to Parkinson’s disease, cancer and male infertility (53, 114, 115). Finally, anti-HA medications and mast cell stabilizers are frequently used in allergic diseases (116).
Of note, there is vast body of evidence showing that catecholamines interact with cells of the immune system (117–120). Immune cells and innervation density and pattern in the male gonad change during development and infertility (20–22, 31, 32). Yet, the clinical implications of the interplay of adrenergic compounds and local immune cells of the testis, have not yet been investigated.
Furthermore, adrenergic nerves are important players in the aging of hematopoietic stem cell niche in bone marrow of mice (121). The testis also harbors a stem cell niche, yet the possibility of an influence of testicular nerves and biogenic amines on the spermatogonial stem cell niche is not known, neither in young adult nor in middle-aged adult and aged men. With exception of melatonin, for which current findings in rodents indicate a potential beneficial role on the steroidogenic activity and the local inflammatory-oxidative status in aged testes (70, 108, 122), biogenic amines and testicular aging represent almost unexplored field of research.
We believe that the time for new studies of the old topic “biogenic amines and their roles in the testis”, yet with a focus on the clinical, i.e. human-specific aspects, may have come. Modern techniques will provide unprecedented novel insights and may identify adequate models. In particular, (spatial) multiomics approaches (123, 124) will be instrumental, i.e. the integration of molecular (DNA, RNA, protein) data and lineage data, possibly with three-dimensional spatial resolution (or even four-dimensional, temporal resolution). Indeed, more and more single cell sequencing studies are already being published (125, 126) and together with other approaches (127, 128), they eventually will provide a clear and precise picture of the sources of biogenic amines, and their testicular receptors, as well as their changes during development, aging and diseases.
Author contributions
MF: Conceptualization, Writing – original draft, Writing – review & editing. AM: Conceptualization, Writing – original draft, Writing – review & editing.
Funding
The author(s) declare financial support was received for the research, authorship, and/or publication of this article. This study was supported by grants from the Agencia Nacional de Promoción Científica y Tecnológica (ANPCyT, project numbers BID PICT 2017 1327, BID PICT 2018 01221 and BID PICT 2021 0277), and the Fundación Alberto J. Roemmers, Fundación René Barón, and Fundación Williams of Argentina and, in part, by grants from Deutsche Forschungsgemeinschaft (DFG), project # 245169951 and project # 427588170.
Conflict of interest
The authors declare that the research was conducted in the absence of any commercial or financial relationships that could be construed as a potential conflict of interest.
The author(s) declared that they were an editorial board member of Frontiers, at the time of submission. This had no impact on the peer review process and the final decision.
Publisher’s note
All claims expressed in this article are solely those of the authors and do not necessarily represent those of their affiliated organizations, or those of the publisher, the editors and the reviewers. Any product that may be evaluated in this article, or claim that may be made by its manufacturer, is not guaranteed or endorsed by the publisher.
Supplementary material
The Supplementary Material for this article can be found online at: https://www.frontiersin.org/articles/10.3389/fendo.2024.1392917/full#supplementary-material
References
1. Purves D, Williams SM. The biogenic amines. In: Purves D, Augustine GJ, Fitzpatrick D, Hall WC, LaMantia A-S, McNamara JO, Williams SM, editors. Neuroscience, 3rd edn. U.S.A. Sinauer Associates Inc, Sunderland, MA (2004). p. 147–51. Chapter 6.
2. Lee JG, Woo YS, Park SW, Seog D-H, Seo MK, Bahk W-M. The neuroprotective effects of melatonin: possible role in the pathophysiology of neuropsychiatric disease. Brain Sci. (2019) 9:285. doi: 10.3390/brainsci9100285
3. Naumenko EV. Role of brain catecholamines (CA) in the control of hypothalamic-pituitary-testicular complex. In: Sark E, Makara GB, Halász B, Rappay Gy, editors. Endocrinology, Neuroendocrinology, neuropeptides, vol. 14 . Pergamon Press, Oxford, United Kingdom (1981). p. 149–60.
4. McCann SM, Karanth S, Mastronardi CA, Dees WL, Childs G, Miller B, et al. Hypothalamic control of gonadotropin secretion. In: Parhar IS, editor. Progress in Brain Research, vol. 141 . Elsevier Science B.V, Amsterdam, The Netherlands (2002). p. 151–64.
5. Campbell RE, Herbison AE. Definition of brainstem afferents to gonadotropin-releasing hormone neurons in the mouse using conditional viral tract tracing. Endocrinology. (2007) 148:5884–90. doi: 10.1210/en.2007–0854
6. Selvage DJ, Lee SY, Parsons LH, Seo DO, Rivier CL. A hypothalamic-testicular neural pathway is influenced by brain catecholamines, but not testicular blood flow. Endocrinology. (2004) 145:1750–9. doi: 10.1210/en.2003–1441
7. Spergel DJ. Modulation of gonadotropin-releasing hormone neuron activity and secretion in mice by non-peptide neurotransmitters, gasotransmitters, and gliotransmitters. Front Endocrinol (Lausanne). (2019) 10:329. doi: 10.3389/fendo.2019.00329
8. Noris G, Hol D, Clapp C, Martínez de la Escalera G. Histamine directly stimulates gonadotropin-releasing hormone secretion from GT1–1 cells via H1 receptors coupled to phosphoinositide hydrolysis. Endocrinology. (1995) 136:2967–74. doi: 10.1210/endo.136.7.7789322
9. Kim HS, Yumkham S, Choi JH, Son GH, Kim K, Ryu SH, et al. Serotonin stimulates GnRH secretion through the c-Src-PLC γ1 pathway in GT1–7 hypothalamic cells. J Endocrinol. (2006) 190:581–91. doi: 10.1677/joe.1.0672
10. Reiter RJ. Pineal melatonin: cell biology of its synthesis and of its physiological interactions. Endocrine Rev. (1991) 12:151–80. doi: 10.1210/edrv-12–2-151
11. Irwig MS, Fraley GS, Smith JT, Acohido BV, Popa SM, Cunningham MJ, et al. Kisspeptin activation of gonadotropin releasing hormone neurons and regulation of KiSS-1 mRNA in the male rat. Neuroendocrinology. (2004) 80:264–72. doi: 10.1159/000083140
12. Revel FG, Saboureau M, Masson-Pévet M, Pévet P, Mikkelsen JD, Simonneaux V. Kisspeptin mediates the photoperiodic control of reproduction in hamsters. Curr Biol. (2006) 16:1730–5. doi: 10.1016/j.cub.2006.07.025
13. Dayan AD. Variation between species in the innervation of intra-testicular blood vessels. Experientia. (1970) 26:1359–60. doi: 10.1007/BF02113031
14. Bell A, Mc Lean JR. The autonomic innervation of the rat testicular capsule. J Reprod Fertil. (1973) 32:253–8. doi: 10.1530/jrf.0.0320253
15. Koji M, Yamamoto M, Mitsuya H. Pharmacological and histological evidence for adrenergic innervation of the myoid cells in the rat seminiferous tubule. Tohoku J Exp Med. (1986) 149:79–87. doi: 10.1620/tjem.149.79
16. Campos MB, Vitale ML, Ritta MN, Chiocchio SR, Calandra RS. Catecholamine distribution in adult rat testis. Andrologia. (1990) 22:247–50. doi: 10.1111/j.1439-0272.1990.tb01974.x
17. Mayerhofer A, Amador AG, Steger RW, Bartke A. Testicular function after local injection of 6-hydroxydopamine or norepinephrine in the golden hamster (Mesocricetus auratus). J Androl. (1990) 11:301–11. doi: 10.1002/j.1939-4640.1990.tb03244.x
18. Zhu BC, Chiocchio SR, Suburo AM, Tramezzani JH. Monoaminergic and peptidergic contributions of the superior and inferior spermatic nerves to the innervation of the testis in the rat. J Androl. (1995) 16:248–58. doi: 10.1002/j.1939-4640.1995.tb00521.x
19. Mayerhofer A, Danilchik M, Pau KY, Lara HE, Russell LD, Ojeda SR. Testis of prepubertal rhesus monkeys receives a dual catecholaminergic input provided by the extrinsic innervation and an intragonadal source of catecholamines. Biol Reprod. (1996) 55:509–18. doi: 10.1095/biolreprod55.3.509
20. Frungieri MB, Urbanski HF, Höhne-Zell B, Mayerhofer A. Neuronal elements in the testis of the rhesus monkey: ontogeny, characterization and relationship to testicular cells. Neuroendocrinology. (2000) 71:43–50. doi: 10.1159/000054519
21. Mayerhofer A, Frungieri MB, Fritz S, Bulling A, Jessberger B, Vogt HJ, et al. Evidence for catecholaminergic, neuronlike cells in the adult human testis: changes associated with testicular pathologies. J Androl. (1999) 20:341–7. doi: 10.1002/j.1939-4640.1999.tb02527.x
22. Matzkin ME, Riviere E, Rossi SP, Ponzio R, Puigdomenech E, Levalle O, et al. β-adrenergic receptors in the up-regulation of COX2 expression and prostaglandin production in testicular macrophages: Possible relevance to male idiopathic infertility. Mol Cell Endocrinol. (2019) 498:110545. doi: 10.1016/j.mce.2019.110545
23. Okkels H, Sand K. Morphological relationship between testicular nerves and Leydig cells in man. J Endocrinol. (1940) 2:38–46. doi: 10.1677/joe.0.0020038
24. Campenhout Ev. Les relation nerveuses de la glande interstitielle des glandes genitales des mammiferes. Rev Can Biol. (1949) 8:374–429.
25. Baumgarten HG, Falck B, Holstein AF, Owman CH, Owman T. Adrenergic innervation of the human testis, epididymis, ductus deferens and prostate: a fluorescence microscopic and fluorimetric study. Z Zellforsch Mikroskop Anat. (1968) 90:81–95. doi: 10.1007/BF00496704
26. Nistal M, Paniagua R, Asunción-Abaurrea M. Varicose axons bearing “synaptic” vesicles on the basal lamina of the human seminiferous tubules. Cell Tissue Res. (1982) 226:75–82. doi: 10.1007/BF00217083
27. Yamamoto M, Takaba H, Hashimoto J, Koji M. Evidence for innervation of the myoid cell in the human seminiferous tubule. Urol Int. (1987) 42:137–9. doi: 10.1159/000281872
28. Campos MB, Vitale ML, Calandra RS, Chiocchio SR. Serotonergic innervation of the rat testis. J Reprod Fert. (1990) 88:475–9. doi: 10.1530/jrf.0.0880475
29. Csaba Zs, Csernus V, Gerendai I. Intratesticular serotonin affects steroidogenesis in the rat testis. J Neuroendocrinol. (1998) 10:371–6. doi: 10.1046/j.1365-2826.1998.00217.x
30. Gerendai I, Banczerowski P, Csernus V, Halász B. Innervation and serotoninergic receptors of the testis interact with local action of interleukin-1beta on steroidogenesis. Auton Neurosci. (2007) 131:21–7. doi: 10.1016/j.autneu.2006.06.002
31. Meineke V, Frungieri MB, Jessberger B, Vogt HJ, Mayerhofer A. Human testicular mast cells contain tryptase: increased mast cell number and altered distribution in the testes of infertile men. Fertil Steril. (2000) 74:239–44. doi: 10.1016/s0015–0282(00)00626–9
32. Frungieri MB, Calandra RS, Lustig L, Meineke V, Köhn FM, Vogt HJ, et al. Number, distribution pattern, and identification of macrophages in the testes of infertile men. Fertil Steril. (2002) 78:298–306. doi: 10.1016/s0015–0282(02)03206–5
33. Jezek D, Banek L, Hittmair A, Pezerovic-Panijan R, Goluza T, Schulze W. Mast cells in testicular biopsies of infertile men with ‘mixed atrophy’ of seminiferous tubules. Andrologia. (1999) 31:203–10. doi: 10.1046/j.1439-0272.1999.00287.x
34. Apa DD, Cayan S, Polat A, Akbay E. Mast cells and fibrosis on testicular biopsies in male infertility. Arch Androl. (2002) 48:337–44. doi: 10.1080/01485010290099183
35. Mechlin CW, Levesque J, Feustel P, Kogan BA. Mast cell numbers negatively correlate with fibrosis in cryptorchid testes. J Pediatr Urol. (2014) 10:527–31. doi: 10.1016/j.jpurol.2013.11.014
36. Tainio H. Peptidergic innervation of the human testis and epididymis. Acta Histochem. (1994) 96:415–20. doi: 10.1016/S0065–1281(11)80028–0
37. Gong YG, Feng MM, Hu XN, Wang YQ, Gu M, Zhang W, et al. Peptidergic not monoaminergic fibers profusely innervate the young adult human testis. J Anat. (2009) 214:330–8. doi: 10.1111/j.1469-7580.2008.01038.x
38. Omirinde JO, Azeez IA. Neuropeptide profiles of mammalian male genital tract: distribution and functional relevance in reproduction. Front Vet Sci. (2022) 9:842515. doi: 10.3389/fvets.2022.842515
39. Walenta L, Fleck D, Fröhlich T, von Eysmondt H, Arnold GJ, Spehr J, et al. ATP-mediated events in peritubular cells contribute to sterile testicular inflammation. Sci Rep. (2018) 8:1431. doi: 10.1038/s41598–018-19624–3
40. Fleck D, Kenzler L, Mundt N, Strauch M, Uesaka N, Moosmann R, et al. ATP activation of peritubular cells drives testicular sperm transport. Elife. (2021) 10:e62885. doi: 10.7554/eLife.62885
41. Tank AW, Lee Wong D. Peripheral and central effects of circulating catecholamines. Compr Physiol. (2015) 5:1–15. doi: 10.1002/cphy.c140007
42. Arazi H, Rahim Mollazadeh R, Dadvand SS, Davaran M. The blood levels of serotonin and dopamine and physical fitness factors in active and inactive men addicted to opium during rehabilitation. Phys Act Rev. (2016) 4:1–8. doi: 10.16926/par.2016.04.01
43. Frungieri MB, Zitta K, Pignataro OP, Gonzalez-Calvar SI, Calandra RS. Interactions between testicular serotoninergic, catecholaminergic, and corticotropin-releasing hormone systems modulating cAMP and testosterone production in the golden hamster. Neuroendocrinology. (2002) 76:35–46. doi: 10.1159/000063682
44. Mayerhofer A, Steger RW, Gow G, Bartke A. Catecholamines stimulate testicular testosterone release of the immature golden hamster via interaction with alpha- and beta-adrenergic receptors. Acta Endocrinol (Copenh). (1992) 127:526–30. doi: 10.1530/acta.0.1270526
45. Rossi SP, Walenta L, Rey-Ares V, Köhn FM, Schwarzer JU, Welter H, et al. Alpha 1 adrenergic receptor-mediated inflammatory responses in human testicular peritubular cells. Mol Cell Endocrinol. (2018) 474:1–9. doi: 10.1016/j.mce.2018.01.027
46. Tinajero JC, Fabbri A, Ciocca DR, Dufau ML. Serotonin secretion from rat Leydig cells. Endocrinology. (1993) 133:3026–9. doi: 10.1210/endo.133.6.8243331
47. Frungieri MB, Gonzalez-Calvar SI, Rubio M, Ozu M, Lustig L, Calandra RS. Serotonin in golden hamster testes: testicular levels, immunolocalization and role during sexual development and photoperiodic regression-recrudescence transition. Neuroendocrinology. (1999) 69:299–308. doi: 10.1159/000054431
48. Jiménez-Trejo F, Coronado-Mares I, Arriaga-Canon C, Herrera LA, Roque-Ramírez B, Chávez-Saldaña M, et al. Indolaminergic system in adult rat testes: evidence for a local serotonin system. Front Neuroanat. (2021) 14:570058. doi: 10.3389/fnana.2020.570058
49. Frungieri MB, Mayerhofer A, Zitta K, Pignataro OP, Calandra RS, Gonzalez-Calvar SI. Direct effect of melatonin on Syrian hamster testes: melatonin subtype 1a receptors, inhibition of androgen production, and interaction with the local corticotropin-releasing hormone system. Endocrinology. (2005) 146:1541–52. doi: 10.1210/en.2004–0990
50. Rossi SP, Matzkin ME, Terradas C, Ponzio R, Puigdomenech E, Levalle O, et al. New insights into melatonin/CRH signaling in hamster Leydig cells. Gen Comp Endocrinol. (2012) 178:153–63. doi: 10.1016/j.ygcen.2012.04.031
51. Rossi SP, Windschuettl S, Matzkin ME, Terradas C, Ponzio R, Puigdomenech E, et al. Melatonin in testes of infertile men: evidence for anti-proliferative and anti-oxidant effects on local macrophage and mast cell populations. Andrology. (2014) 2:436–49. doi: 10.1111/j.2047-2927.2014.00207.x
52. Frungieri MB, Calandra RS, Rossi SP. Local actions of melatonin in somatic cells of the testis. Int J Mol Sci. (2017) 18:e1170. doi: 10.3390/ijms18061170
53. Riviere E, Rossi SP, Tavalieri YE, Muñoz de Toro MM, Ponzio R, Puigdomenech E, et al. Melatonin daily oral supplementation attenuates inflammation and oxidative stress in testes of men with altered spermatogenesis of unknown aetiology. Mol Cell Endocrinol. (2020) 515:110889. doi: 10.1016/j.mce.2020.110889
54. Riviere E, Rossi SP, Tavalieri YE, Muñoz de Toro MM, Calandra RS, Mayerhofer A, et al. Pleiotropic actions of melatonin in testicular peritubular myoid cells of immature Syrian hamsters. Biochim Biophys Acta Gen Subj. (2022) 1866:130187. doi: 10.1016/j.bbagen.2022.130187
55. Rossi SP, Matzkin ME, Riviere E, Martinez G, Ponzio R, Levalle O, et al. Melatonin improves oxidative state and lactate metabolism in rodent Sertoli cells. Mol Cell Endocrinol. (2023) 576:112034. doi: 10.1016/j.mce.2023.112034
56. Tan D-X, Manchester LC, Sanchez-Barcelo E, Mediavilla MD, Reiter RJ. Significance of high levels of endogenous melatonin in mammalian cerebrospinal fluid and in the central nervous system. Curr Neuropharmacol. (2010) 8:162–7. doi: 10.2174/157015910792246182
57. Kaliner M, Shelhamer JH, Ottesen EA. Effects of infused histamine: correlation of plasma histamine levels and symptoms. J Allergy Clin Immunol. (1982) 69:283–9. doi: 10.1016/s0091–6749(82)80005–5
58. Lieberman P, Ewan PW. Anaphylaxis. In: Holgate S, Church M, Broide D, Martinez F, editors. Allergy, 4th ed. United Kingdom: Elsevier Health Sciences (2012). p. 331–46. Chapter 17.
59. Mayerhofer A, Bartke A, Amador AG, Began T. Histamine affects testicular steroid production in the golden hamster. Endocrinology. (1989) 125:560–2. doi: 10.1210/endo-125–1-560
60. Albrecht M, Frungieri MB, Gonzalez-Calvar S, Meineke V, Köhn FM, Mayerhofer A. Evidence for a histaminergic system in the human testis. Fertil Steril. (2005) 83:1060–3. doi: 10.1016/j.fertnstert.2004.12.002
61. Mondillo C, Varela ML, Abiuso AMB, Vázquez R. Potential negative effects of anti-histamines on male reproductive function. Reproduction. (2018) 155:R221–7. doi: 10.1530/REP-17–0685
62. González CR, González B, Matzkin ME, Muñiz JA, Cadet JL, Garcia-Rill EG, et al. Psychostimulant-induced testicular toxicity in mice: evidence of cocaine and caffeine effects on the local dopaminergic system. PloS One. (2015) 10:e0142713. doi: 10.1371/journal.pone.0142713
63. Davidoff MS, Schulze W, Middendorff R, Holstein AF. The Leydig cell of the human testis —A new member of the diffuse neuroendocrine system. Cell Tissue Res. (1993) 271:429–39. doi: 10.1007/bf02913725
64. Davidoff MS, Ungefroren H, Middendorff R, Koeva Y, Bakalska M, Atanassova N, et al. Catecholamine-synthesizing enzymes in the adult and prenatal human testis. Histochem Cell Biol. (2005) 124:313–23. doi: 10.1007/s00418-005-0024-x
65. Safina F, Tanaka S, Inagaki M, Tsuboi K, Sugimoto Y, Ichikawa A. Expression of l-histidine decarboxylase in mouse male germ cells. J Biol Chem. (2002) 19:14211–5. doi: 10.1074/jbc.M200702200
66. Pagotto RM, Monzon C, Moreno MB, Pignataro OP, Mondillo C. Proliferative effect of histamine on MA-10 Leydig tumor cells mediated through HRH2 activation, transient elevation in cAMP production, and increased extracellular signal-regulated kinase phosphorylation levels. Biol Reprod. (2012) 87:150. doi: 10.1095/biolreprod.112.102905
67. Gonzalez-Arto M, Hamilton TR, Gallego M, Gaspar-Torrubia E, Aguilar D, Serrano-Blesa E, et al. Evidence of melatonin synthesis in the ram reproductive tract. Andrology. (2016) 4:163–71. doi: 10.1111/andr.12117
68. Zwadlo-Klarwasser G, Braam U, Mühl-Zürbes P, Schmutzler W. Macrophages and lymphocytes: alternative sources of histamine. Agents Actions. (1994) 41:C99–100. doi: 10.1007/BF02007785
69. Rudd ML, Nicolas AN, Brown BL, Fischer-Stenger K, Stewart JK. Peritoneal macrophages express the serotonin transporter. J Neuroimmunol. (2005) 159:113–8. doi: 10.1016/j.jneuroim.2004.10.013
70. Matzkin ME, Valchi P, Riviere E, Rossi SP, Tavalieri YE, Muñoz de Toro MM, et al. Aging in the Syrian hamster testis: Inflammatory-oxidative status and the impact of photoperiod. Exp Gerontol. (2019) 124:110649. doi: 10.1016/j.exger.2019.110649
71. Otth C, Torres M, Ramírez A, Fernandez JC, Castro M, Rauch MC, et al. Novel identification of peripheral dopaminergic D2 receptor in male germ cells. J Cell Biochem. (2007) 100:141–50. doi: 10.1002/jcb.21037
72. Dirami G, Teerds KJ, Cooke BA. Effect of a dopamine agonist on the development of Leydig cell hyperplasia in Sprague-Dawley rats. Toxicol Appl Pharmacol. (1996) 141:169–77. doi: 10.1006/taap.1996.0273
73. González B, Gancedo SN, Janeir Garazatua SA, Roldán E, Vitullo AD, González CR. Dopamine receptor D1 contributes to cocaine epigenetic reprogramming of histone modifications in male germ cells. Front Cell Dev Biol. (2020) 8:216. doi: 10.3389/fcell.2020.00216
74. Raut S, Khambata K, Singh D, Balasinor NH. Dopamine receptor D2 regulates genes involved in germ cell movement and sperm motility in rat testes. Biol Reprod. (2024) 110:377–90. doi: 10.1093/biolre/ioad153
75. Ramírez AR, Castro MA, Angulo C, Ramió L, Montserrat Rivera M, Torres M, et al. The presence and function of dopamine type 2 receptors in boar sperm: a possible role for dopamine in viability, capacitation, and modulation of sperm motility. Biol Reprod. (2009) 80:753–61. doi: 10.1095/biolreprod.108.070961
76. Urra JA, Villaroel-Espíndola F, Covarrubias AA, Rodríguez-Gil JE, Ramírez-Reveco A, Concha II. Presence and function of dopamine transporter (DAT) in stallion sperm: dopamine modulates sperm motility and acrosomal integrity. PloS One. (2014) 9:e112834. doi: 10.1371/journal.pone.0112834
77. Mayerhofer A, Bartke A, Steger RW. Catecholamine effects on testicular testosterone production in the gonadally active and the gonadally regressed adult golden hamster. Biol Reprod. (1989) 40:752–61. doi: 10.1095/biolreprod40.4.752
78. Mayerhofer A, Calandra RS, Bartke A. Cyclic adenosine monophosphate (cAMP) does not mediate the stimulatory action of norepinephrine on testosterone production by the testis of the golden hamster. Life Sci. (1991) 48:1109–14. doi: 10.1016/0024-3205(91)90513-B
79. Mayerhofer A, Bartke A, Began T. Catecholamines stimulate testicular steroidogenesis in vitro in the Siberian hamster, Phodopus sungorus. Biol Reprod. (1993) 48:883–8. doi: 10.1095/biolreprod48.4.883
80. Mhaouty-Kodja S, Lozach A, Habert R, Tanneux M, Guigon C, Brailly-Tabard S, et al. Fertility and spermatogenesis are altered in a1b-adrenergic receptor knockout male mice. J Endocrinol. (2007) 195:281–92. doi: 10.1677/JOE-07–0071
81. Stojkov NJ, Baburski AZ, Bjelic MM, Sokanovic SJ, Mihajlovic AI, Drljaca DM, et al. In vivo blockade of α1-adrenergic receptors mitigates stress-disturbed cAMP and cGMP signaling in Leydig cells. Mol Hum Reprod. (2014) 20:77–88. doi: 10.1093/molehr/gat052
82. Cohen MS, Plaine L, Brown JS. The role of internal spermatic vein plasma catecholamine determinations in subfertile men with varicoceles. Fertil Steril. (1975) 26:1243–9. doi: 10.1016/s0015–0282(16)41541–4
83. Nagao Y. Viability of meiotic prophase spermatocytes of rats is facilitated in primary culture of dispersed testicular cells on collagen gel by supplementing epinephrine or norepinephrine: evidence that meiotic prophase spermatocytes complete meiotic divisions in vitro. In Vitro Cell Dev Biol. (1989) 25:1088–98. doi: 10.1007/BF02621259
84. Tunçel N, Gürer F, Aral E, Uzuner K, Aydın Y, Bayçu C. The effect of vasoactive intestinal peptide (VIP) on mast cell invasion/degranulation in testicular interstitium of immobilized/cold stressed and b-endorphin-treated rats. Peptides. (1996) 17:817–24.
85. Tunçel N, Aydin Y, Koşar M, Tunçel M. The effect of vasoactive intestinal peptide (VIP) on the testicular tissue histamine level of immobilized + cold stressed rats. Peptides. (1997) 18:913–5. doi: 10.1016/s0196–9781(97)00134–4
86. O’Reilly M, Alpert R, Jenkinson S, Gladue RP, Foo S, Trim S, et al. Identification of a histamine H4 receptor on human eosinophils – role in eosinophil chemotaxis. J Recept Signal Transduct Res. (2002) 22:431–48. doi: 10.1081/rrs-120014612
87. Khan UW, Rai U. Differential effects of histamine on Leydig cell and testicular macrophage activities in wall lizards: precise role of H1/H2 receptor subtypes. J Endocrinol. (2007) 194:441–8. doi: 10.1677/JOE-06–0225
88. Abiuso AM, Berensztein E, Pagotto RM, Pereyra EN, Medina V, Martinel Lamas DJ, et al. H4 histamine receptors inhibit steroidogenesis and proliferation in Leydig cells. J Endocrinol. (2014) 223:241–53. doi: 10.1530/JOE-14–0401
89. Mondillo C, Patrignani Z, Reche C, Rivera E, Pignataro O. Dual role of histamine in modulation of Leydig cell steroidogenesis via HRH1 and HRH2 receptor subtypes. Biol Reprod. (2005) 73:899–907. doi: 10.1095/biolreprod.105.041285
90. Mondillo C, Pagotto RM, Piotrkowski B, Reche CG, Patrignani ZJ, Cymeryng CB, et al. Involvement of nitric oxide synthase in the mechanism of histamineinduced inhibition of Leydig cell steroidogenesis via histamine receptor subtypes in Sprague–Dawley rats. Biol Reprod. (2009) 80:144–52. doi: 10.1095/biolreprod.108.069484
91. Gill M, Sareen ML, Sanyal SN. Effect of H2-receptor antagonists, cimetidine and ranitidine on reproductive functions in male mice. Indian J Exp Biol. (1991) 29:900–6.
92. Franca LR, Leal MC, Sasso-Cerri E, Vasconcelos A, Debeljuk L, Russell LD. Cimetidine (Tagamet) is a reproductive toxicant in male rats affecting peritubular cells. Biol Reprod. (2000) 63:1403–12. doi: 10.1095/biolreprod63.5.1403
93. Gupta A, Khosla R, Gupta S, Tiwary AK. Influence of histamine and H1-receptor antagonists on ejaculated human spermatozoa: role of intrasperm Ca2+. Indian J Exp Biol. (2004) 42:481–5.
94. Sasso-Cerri E, Cerri PS. Morphological evidences indicate that the interference of cimetidine on the peritubular components is responsible for detachment and apoptosis of Sertoli cells. Reprod Biol Endocrinol. (2008) 6:18. doi: 10.1186/1477–7827-6–18
95. Aprioku JS, Ibeachu C, Amah-Tariah FS. Differential effects of H2 receptor antagonists on male reproductive function and hepatic enzymes in wistar rats. Asian J BioMed Pharm Sci. (2014) 4:1–6.
96. Kuzminov O, Ostapiv D, Alekhina T. Evaluation of cytotoxic action of antihistamines – desloratadine and loratadine – using bulls spermatozoa as a test object. Vìsnik Dnìpropetrovs′kogo Unìversitetu. Serìâ Bìologìâ. (2014) 5:3–6. doi: 10.15421/021401
97. Tinajero JC, Fabbri A, Dufau ML. Regulation of corticotropin-releasing factor secretion from Leydig cells by serotonin. Endocrinology. (1992) 130:1780–8. doi: 10.1210/endo.130.4.1312425
98. Hedger MP, Khatab S, Gonzales G, de Kretser DM. Acute and short-term actions of serotonin administration on the pituitary-testicular axis in the adult rat. Reprod Fertil Dev. (1995) 7:1101–9. doi: 10.1071/rd9951101
99. Aragón MA, Ayala ME, Marín M, Avilés A, Damián-Matsumura P, Domínguez R. Serotoninergic system blockage in the prepubertal rat inhibits spermatogenesis development. Reproduction. (2005) 129:717–27. doi: 10.1530/rep.1.00598
100. Díaz-Ramos J, Flores-Flores M, Ayala ME, Aragón-Martínez A. Impaired serotonin communication during juvenile development in rats diminishes adult sperm quality. Syst Biol Reprod Med. (2018) 64:340–7. doi: 10.1080/19396368.2018.1472825
101. Collin O, Damber JE, Bergh A. 5-hydroxytryptamine—a local regulator of testicular blood flow and vasomotion in rats. J Reprod Fert. (1996) 106:17–22. doi: 10.1530/jrf.0.1060017
102. Rocha CS, Martins A, Rato L, Silva BM, Oliveira PF, Alves MG. Melatonin alters the glycolytic profile of Sertoli cells: Implications for male fertility. Mol Hum Reprod. (2014) 20:1067–76. doi: 10.1093/molehr/gau080
103. Yang WC, Tang KQ, Fu CZ, Riaz H, Zhang Q, Zan LS. Melatonin regulates the development and function of bovine Sertoli cells via its receptors MT1 and MT2. Anim Reprod Sci. (2014) 147:10–6. doi: 10.1016/j.anireprosci.2014.03.017
104. Valenti S, Guido R, Giusti M, Giordano G. In vitro acute and prolonged effects of melatonin on purified rat Leydig cell steroidogenesis and adenosine 3’,5’-monophosphate production. Endocrinology. (1995) 136:5357–62. doi: 10.1210/endo.136.12.7588282
105. Wu CS, Leu SF, Yang HY, Huang BM. Melatonin inhibits the expression of steroidogenic acute regulatory protein and steroidogenesis in MA-10 cells. J Androl. (2001) 22:245–54. doi: 10.1002/j.1939-4640.2001.tb02177.x
106. Yang M, Guan S, Tao J, Zhu K, Lv D, Wang J, et al. Melatonin promotes male reproductive performance and increases testosterone synthesis in mammalian Leydig cells. Biol Reprod. (2021) 104:1322–36. doi: 10.1093/biolre/ioab046
107. Mukherjee A, Haldar C. Photoperiodic regulation of melatonin membrane receptor (MT1R) expression and steroidogenesis in testis of adult golden hamster, Mesocricetus auratus. J Photochem Photobiol B. (2014) 140:374–80. doi: 10.1016/j.jphotobiol.2014.08.022
108. Mukherjee A, Haldar C. Melatonin membrane receptor (MT1R) expression and nitro-oxidative stress in testis of golden hamster, Mesocricetus auratus: an age-dependent study. Exp Gerontol. (2015) 69:211–20. doi: 10.1016/j.exger.2015.06.022
109. Lopez Vicchi F, Luque GA, Brie B, Nogueira JP, Garcia Tornadu I, Becu-Villalobos D. Dopaminergic drugs in type 2 diabetes and glucose homeostasis. Pharmacol Res. (2016) 109:74–80. doi: 10.1016/j.phrs.2015.12.029
110. Paravati S, Rosani A, Warrington SJ. Physiology, Catecholamines. Treasure Island, FL: StatPearls Publishing (2023).
111. Lin SH, Lee LT, Yang YK. Serotonin and mental disorders: a concise review on molecular neuroimaging evidence. Clin Psychopharmacol Neurosci. (2014) 12:196–202. doi: 10.9758/cpn.2014.12.3.196
112. Romanelli RJ, Wu FM, Gamba R, Mojtabai R, Segal JB. Behavioral therapy and serotonin reuptake inhibitor pharmacotherapy in the treatment of obsessive-compulsive disorder: a systematic review and meta-analysis of head-to-head randomized controlled trials. Depress Anxiety. (2014) 31:641–52. doi: 10.1002/da.22232
113. Rios ER, Venâncio ET, Rocha NF, Woods DJ, Vasconcelos S, Macedo D, et al. Melatonin: pharmacological aspects and clinical trends. Int J Neurosci. (2010) 120:583–90. doi: 10.3109/00207454.2010.492921
114. Srinivasan V. Therapeutic potential of melatonin and its analogs in Parkinson’s disease: focus on sleep and neuroprotection. Ther Adv Neurol Disord. (2011) 4:297–317. doi: 10.1177/1756285611406166
115. Wang L, Wang C, Choi WS. Use of melatonin in cancer treatment: where are we? Int J Mol Sci. (2022) 23:3779. doi: 10.3390/ijms23073779
116. Yanai K, Yoshikawa T, Church MK. Efficacy and safety of non-brain penetrating H1-antihistamines for the treatment of allergic diseases. Curr Top Behav Neurosci. (2022) 59:193–214. doi: 10.1007/7854_2021_265
117. Elenkov IJ, Wilder RL, Chrousos GP, Vizi ES. The sympathetic nerve–an integrative interface between two supersystems: the brain and the immune system. Pharmacol Rev. (2000) 52:595–638.
118. Flierl MA, Rittirsch D, Huber-Lang M, Sarma JV, Ward PA. Catecholamines—Crafty weapons in the inflammatory arsenal of immune/inflammatory cells or opening pandora’s box. Mol Med. (2008) 14:195–204. doi: 10.2119/2007-00105.Flierl
119. Gaskill PJ, Khoshbouei H. Dopamine and norepinephrine are embracing their immune side and so should we. Curr Opin Neurobiol. (2022) 77:102626. doi: 10.1016/j.conb.2022.102626
120. Channer B, Matt SM, Nickoloff-Bybel EA, Pappa V, Agarwal Y, Wickman J, et al. Dopamine, immunity, and disease. Pharmacol Rev. (2023) 75:62–158. doi: 10.1124/pharmrev.122.000618
121. Maryanovich M, Zahalka AH, Pierce H, Pinho S, Nakahara F, Asada N, et al. Adrenergic nerve degeneration in bone marrow drives aging of the hematopoietic stem cell niche. Nat Med. (2018) 24:782–91. doi: 10.1038/s41591-018-0030-x
122. Matzkin ME, Miquet JG, Fang Y, Hill CM, Turyn D, Calandra RS, et al. Alterations in oxidative, inflammatory and apoptotic events in short-lived and long-lived mice testes. Aging (Albany NY). (2016) 8:95–110. doi: 10.18632/aging.100875
123. Bressan D, Battistoni G, Hannon GJ. The dawn of spatial omics. Science. (2023) ñ381:eabq4964. doi: 10.1126/science.abq4964
124. Lewis SM, Asselin-Labat ML, Nguyen Q, Berthelet J, Tan X, Wimmer VC, et al. Spatial omics and multiplexed imaging to explore cancer biology. Nat Meth. (2021) 18:997–1012. doi: 10.1038/s41592–021-01203–6
125. Guo J, Sosa E, Chitiashvili T, Nie X, Rojas EJ, Oliver E, et al. Single-cell analysis of the developing human testis reveals somatic niche cell specification and fetal germline stem cell establishment. Cell Stem Cell. (2021) 28:764–78.e4. doi: 10.1016/j.stem.2020.12.004
126. Nie X, Munyoki SK, Sukhwani M, Schmid N, Missel A, Emery BR, et al. Single-cell analysis of human testis aging and correlation with elevated body mass index. Dev Cell. (2022) 57:1160–76.e5. doi: 10.1016/j.devcel.2022.04.004
127. Lahiri S, Aftab W, Walenta L, Strauss L, Poutanen M, Mayerhofer A, et al. ALDI-IMS combined with shotgun proteomics identify and localize new factors in male infertility. Life Sci Alliance. (2021) 4:e202000672. doi: 10.26508/lsa.202101015
Keywords: catecholamines, serotonin, histamine, melatonin, testis, male infertility, aging
Citation: Frungieri MB and Mayerhofer A (2024) Biogenic amines in the testis: sources, receptors and actions. Front. Endocrinol. 15:1392917. doi: 10.3389/fendo.2024.1392917
Received: 28 February 2024; Accepted: 27 May 2024;
Published: 20 June 2024.
Edited by:
Sudhanshu Bhushan, University of Giessen, GermanyReviewed by:
Luís Pedro Rato, Instituto Politécnico da Guarda, PortugalAlison Jane Carey, Queensland University of Technology, Australia
Copyright © 2024 Frungieri and Mayerhofer. This is an open-access article distributed under the terms of the Creative Commons Attribution License (CC BY). The use, distribution or reproduction in other forums is permitted, provided the original author(s) and the copyright owner(s) are credited and that the original publication in this journal is cited, in accordance with accepted academic practice. No use, distribution or reproduction is permitted which does not comply with these terms.
*Correspondence: Monica Beatriz Frungieri, bW9uaWNhZnJ1bmdpZXJpQGdtYWlsLmNvbQ==