- 1Department of Ophthalmology, Fourth People’s Hospital of Shenyang, Shenyang, China
- 2Department of Ophthalmology, Fourth Affiliated Hospital of Soochow University, Suzhou, China
- 3Department of Endocrinology, First Affiliated Hospital of Soochow University, Suzhou, China
Background: Diabetic retinopathy (DR) is a serious microvascular complication of diabetes mellitus. Research has identified a close relationship between fibroblast growth factor 21 (FGF21) and DR. FGF21 is a member of the FGF subfamily, which is activated by the Klotho coenzyme involved in the occurrence of DR. However, the association between FGF21, Klotho, and DR remains controversial.
Aim: To assess FGF21 and Klotho levels in patients with DR.
Methods: A literature search of the Web of Science, Wiley Online Library, PubMed, China National Knowledge Infrastructure and Wanfang databases was performed. The title or abstract search terms “diabetic retinopathy” and “DR” were used in combination with “fibroblast growth factor 21”, “FGF21”, and “Klotho”. Meta-analysis results are presented as standardized mean difference (SMD) with corresponding 95% confidence interval (CI).
Results: Fifteen studies were included in this meta-analysis. FGF21 levels in patients with DR were significantly higher than in non-DR patients with diabetes (SMD: 2.12, 95% CI [1.40, 2.84]). Klotho levels in patients with DR were significantly lower than in non-DR patients with diabetes (SMD: –0.63, 95% CI [–1.22, – 0.04]).
Conclusions: This systematic review is the first to evaluate the relationship between FGF21, Klotho levels, and DR. FGF21 levels were significantly higher in patients with DR. Fully elucidating the role of FGF21 will significantly contribute to the treatment of DR.
Introduction
Diabetes is a common metabolic disease characterized by abnormally high blood glucose levels. Epidemiological studies have reported that diabetes not only has an increasing annual incidence rate, but is also projected to become the seventh leading cause of death worldwide by 2030 (1), posing a serious threat to human health. Diabetic retinopathy (DR) is a serious microvascular complication of diabetes. In 2020, approximately 103 million individuals were affected by DR globally, and this number is projected to increase to 160 million by 2045 (2). DR is an ischemic disease characterized by early stage retinal neurodegeneration, retinal microaneurysms, and bleeding, as well as possible accompanying “cotton wool” patches, venous bead-like changes, and retinal microvascular abnormalities (3). DR is the main cause of visual dysfunction and blindness in working-age adults worldwide and is significantly associated with a risk for future cerebrovascular accidents, myocardial infarction, and congestive heart failure (4). Conventional treatment methods for DR, such as retinal laser photocoagulation, hypoglycemic drugs, and anti-vascular endothelial growth factor (anti-VEGF) therapy, are ineffective and are accompanied by numerous side effects (5). It is clear that DR imposes a significant public health burden worldwide, seriously threatening the vision and quality of life of affected individuals. As such, there is an urgent need to further clarify the precise pathological and physiological mechanisms of DR to improve prevention strategies for DR and develop new treatment strategies.
Recent research has identified a close relationship between fibroblast growth factor 21 (FGF21) and DR, which may be a therapeutic target for pathological vascular growth in patients (6–8). FGF21 is a member of the FGF subfamily, with a coding gene located on chromosome 19 that encodes a long-chain protein comprising 209 amino acids. FGF21 binds to FGF receptors 1–4 under the action of the Klotho coenzyme and enters the bloodstream, producing effects by binding to different receptors (9, 10). FGF21 is expressed in multiple tissues and organs of the human body, mainly in the liver, followed by the pancreas, adipose tissue, myocardial cells, skeletal muscles (11). FGF21, which participates in metabolic regulation in the bloodstream, originates mainly from the liver. However, the association between FGF21, Klotho, and DR remains controversial. The FGF21 and Klotho levels of DR patients were various among studies. To our knowledge, this systematic review is the first to evaluate the relationship between FGF21 and Klotho levels and DR.
Methods
Literature search
A literature search of the Web of Science, Wiley Online Library, PubMed, China National Knowledge Infrastructure (CNKI) and Wanfang databases was performed. The title or abstract search terms “diabetic retinopathy” and “DR” were used in combination with “fibroblast growth factor 21”, “FGF21”, and “Klotho”. The focus of the search period was 1980 to 2024, with publication languages limited to English and Chinese. The reference lists of the retrieved articles were manually searched to identify additional, potentially eligible studies. To date, no studies have been conducted on this topic. This systematic review and meta-analysis was registered with PROSPERO under accession number CRD42024501425. All items that should be reported for systematic reviews and meta-analyses are listed in the Supplementary Table 1.
Inclusion criteria
Meta-analysis was conducted on studies fulfilling the following criteria: sufficient data regarding FGF21, Klotho levels in DR patients and non-DR patients with diabetes; case-control design; and language limited to English and Chinese.
Data extraction and risk of bias
As part of the preliminary screening process, 2 reviewers independently used the search strategy and read titles and abstracts to exclude studies that did not fulfill the inclusion criteria. To determine whether the studies met the inclusion criteria, the two reviewers methodologically reviewed the full text. If the author’s information is incomplete, they can contact and crosscheck the author. If the conclusions of the two evaluators were inconsistent, the differences were resolved through discussion. If the discussion failed to resolve any differences, it was judged and arbitrated by a third researcher. The Cochrane Collaboration recommends the Newcastle-Ottawa Scale (NOS) as a tool to assess bias in observational studies (12, 13). Studies were rated according to the NOS, which ranged from zero to nine stars, and star scores were used to determine quality. There are three aspects in the NOS: the method for selecting case and control groups, their comparability, and the method for assessing exposure.
Statistical analysis
Heterogeneity among the included studies was assessed using the I2 statistic, and the data are expressed as standardized mean difference (SMD) and corresponding 95% confidence interval (CI). Fixed-effects models were used if I2 was < 50% and heterogeneity among studies was low or moderate; otherwise, random-effects models were used if I2 was > 50%. A sensitivity analysis was performed to evaluate the stability of the results. Begg’s and Egger’s tests were used to detect publication bias. P < 0.05 was set as the significance level. Data analysis was performed using Review Manager version 5.3. And the sensitivity analysis and publication bias were performed using Stata version 12.0.
Results
Literature search and study selection
The initial literature search retrieved 85 studies from the Web of Science, Wiley Online Library, PubMed, CNKI and databases. After screening, 15 studies including 1220 DR cases and 1447 controls were included (6, 7, 14–26). A flow-diagram illustrating the study selection process is presented in Figure 1. The characteristics of each of the included studies are summarized in Table 1. All 15 studies included in this meta-analysis fulfilled the criteria for the Newcastle-Ottawa Scale categories of selection, comparability, and exposure.
Results of meta-analysis
There 10 articles were about FGF21 and DR. While, in these 10 articles, one article used healthy people as control group, not the patients with non-DR diabetes. So, we have performed the analysis in different control groups (non-DR diabetes patients and healthy controls). FGF21 levels were significantly higher in patients with DR than in non-DR patients with diabetes (SMD:2.12, 95% CI [1.40, 2.84]; I2 = 97%) (6, 7, 14, 16, 17, 20, 21, 24, 25). Forest plot and funnel plot of FGF21 levels in patients with DR compared to those in non-DR diabetes are presented in Figures 2, 3. It was also found that FGF21 levels in patients with DR were significantly higher than that in healthy controls [SMD: 3.90, 95% CI (2.46, 5.35); I2 = 98%] (6, 7, 14, 17, 21, 22, 25). In addition, there was no difference in FGF21 levels between patients with non-proliferative DR (NPDR) and those with proliferative DR (PDR) [SMD: 0.89, 95% CI (–1.21, 2.99); I2 = 97%] (6, 14, 20–22). The Klotho level in patients with DR was significantly lower than that in non-DR patients with diabetes [SMD: –0.63, 95% CI (–1.22, – 0.04); I2 = 92%] (15, 18, 19, 23, 26). Forest plots and funnel plot of Klotho levels are presented in Figures 4, 5.
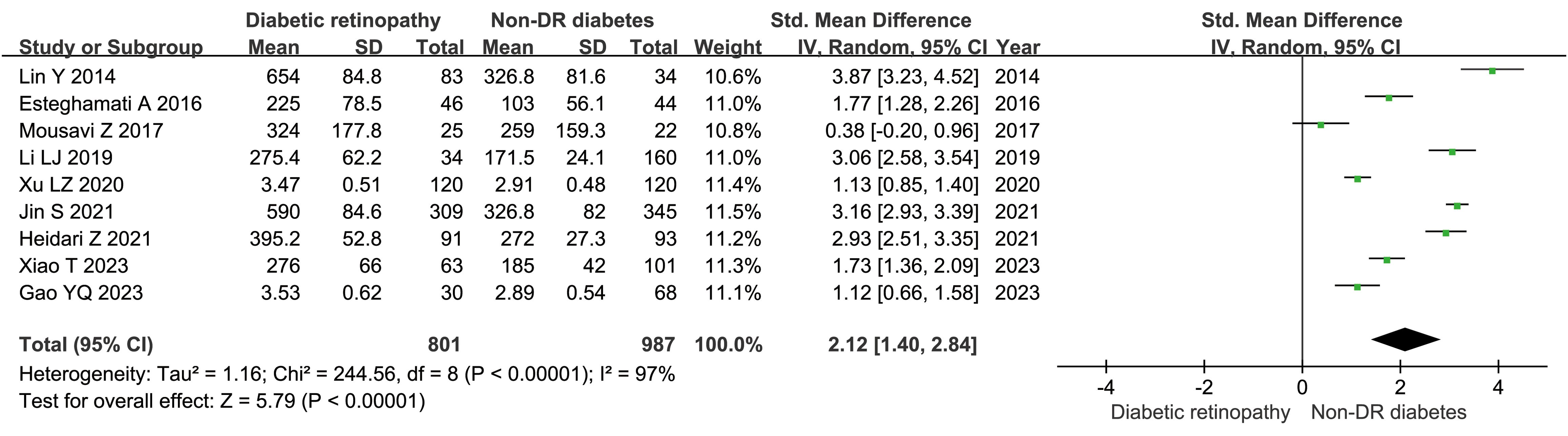
Figure 2. Forest plots of FGF21 level in patients with diabetic retinopathy compared to non-diabetic retinopathy diabetes patients. Diamond represents the pooled SMDs at 95% CI. SMD, standardized mean difference; CI, confidence interval.
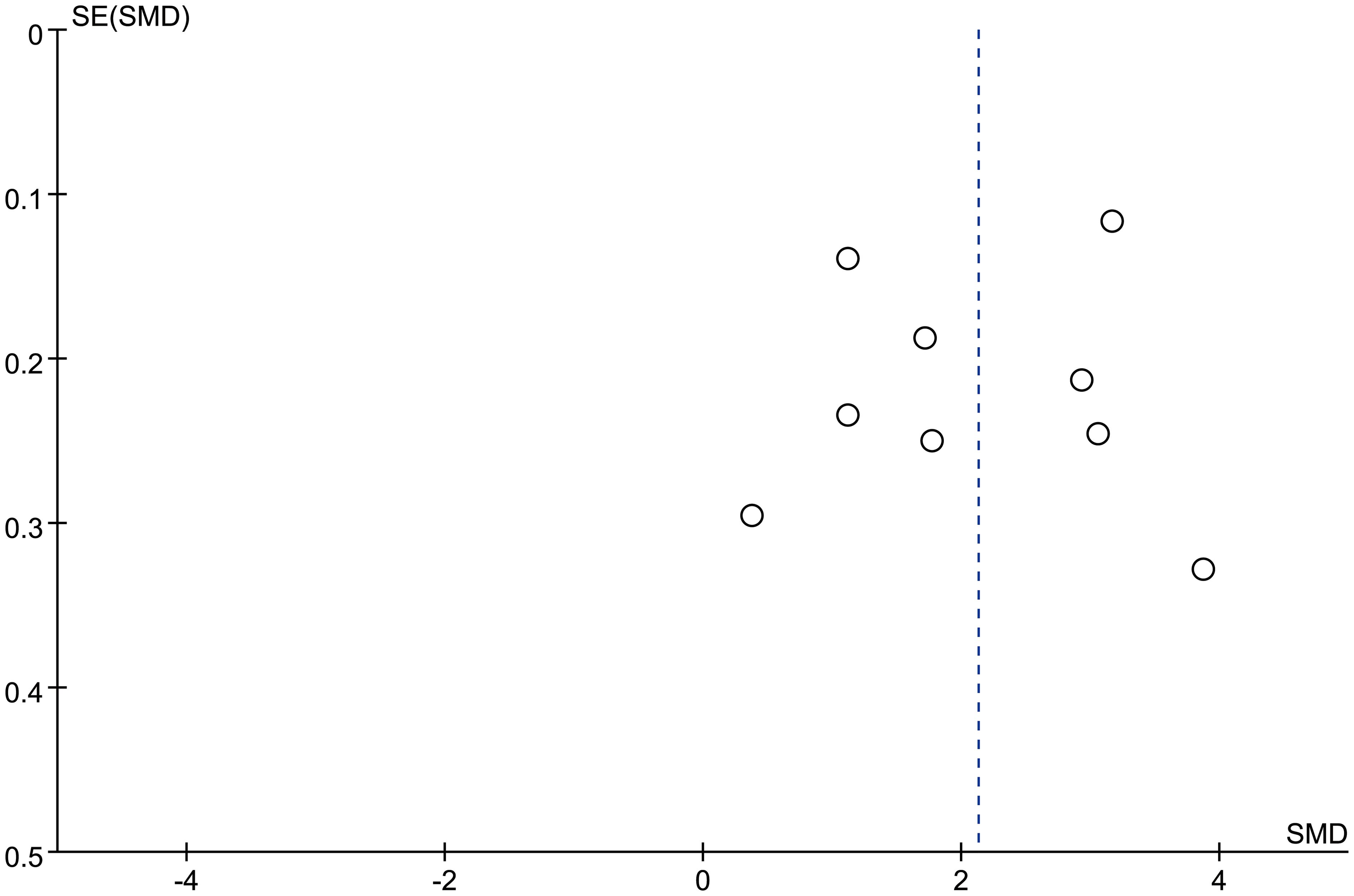
Figure 3. Funnel plots of FGF21 level in patients with diabetic retinopathy compared to non-diabetic retinopathy diabetes patients. SMD, standardized mean difference.

Figure 4. Forest plots of Klotho level in patients with diabetic retinopathy compared to non-diabetic retinopathy diabetes patients. Diamond represents the pooled SMDs at 95% CI. SMD, standardized mean difference; CI, confidence interval.
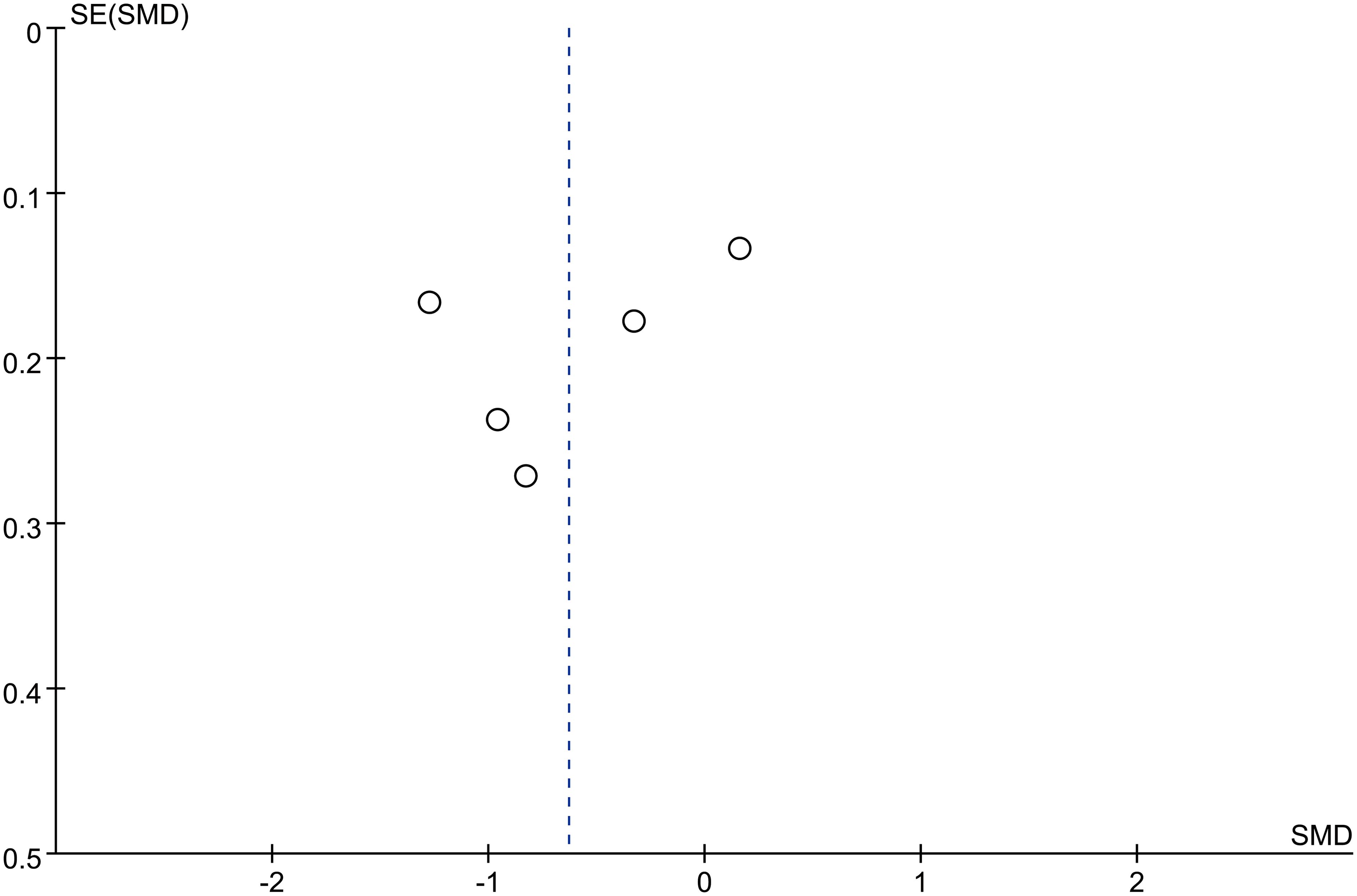
Figure 5. Funnel plots of Klotho level in patients with diabetic retinopathy compared to non-diabetic retinopathy diabetes patients. SMD, standardized mean difference.
Sensitivity analysis and publication bias
Each study was subjected to a sensitivity analysis to determine its influence. Sensitivity analysis showed no significant differences from our previous estimates, indicating that a single study had a marginal impact on the overall estimate (Supplementary Figures 1, 2). Accordingly, the meta-analysis yields stable results. A thorough and comprehensive search of the databases was conducted. Begg’s and Egger’s tests were conducted to identify whether publication bias was present in the reviewed studies. The results (P > 0.05) indicated that there was no publication bias (Supplementary Figures 3–6).
Discussion
This systematic review is the first to evaluate FGF21 and Klotho levels in patients with DR. In our previous research, we found the leptin and chemerin levels in patients with DR were significantly higher than those in non-DR patients (27). In this meta-analysis, fourteen independent studies were included in the meta-analysis. We conclude that FGF21 levels were significantly higher in patients with DR than non-DR patients with diabetes, and that Klotho levels were significantly lower in patients with DR than non-DR patients with diabetes.
FGF21 is a key regulator of retinal lipid and glucose metabolism. It can metabolize lipoproteins in the adipose tissue and maintain adipocyte phospholipid homeostasis. FGF21 also increases lipid utilization during amino acid starvation. FGF21 acts by regulating the activities of peroxisome proliferator-activated receptors (PPARs) and peroxisome proliferator-activated receptor γ coactivator-1 (PGC-1). FGF21 is crucial in PPAR-α agonists to improve metabolic processes in obese mice (28). FGF21 inhibits the growth of pathological retinal neovascularization through adiponectin (APN). FGF21 promotes APN expression in the blood circulation in a dose-dependent manner (29). Fu et al. reported that APN could inhibit neovascularization of the retina and choroid in mice (30). The use of long-acting FGF21 analogs can increase the concentration of retinal APN in mice, indicating that FGF21 has important effects on metabolic functions (31). To determine whether APN mediates the protective effect of FGF21 on retinal neovascularization, the retinal vascular systems of mice with and without long-acting FGF21 analogs were compared under oxygen-induced retinopathy in APN gene knockout mice. Studies have shown that APN deficiency exacerbates retinal neovascularization and eliminates the beneficial effects of long-acting FGF21 analogs in reducing hypoxic retinal neovascularization. In addition, APN can inhibit retinal neovascularization by reducing the level of tumor necrosis-alpha (TNF-α) (32). In summary, FGF21 inhibits the growth of pathological retinal neovascularization by targeting APN and reducing TNF-α, which is a key risk factor for hypoxia-induced retinopathy (33).
High blood glucose levels can induce oxidative stress, which is a key factor in DR (34). Photosensitive cells are the most metabolically active cells in the human body and are prone to metabolic disorders and oxidative stress (35). Fu et al. (36) reported that the use of FGF21 in insulin-deficient diabetic mice reversed retinal neuron defects caused by diabetes, improved the function and morphology of photoreceptors, and reduced inflammation of photoreceptors. NRF2 regulates oxidative stress and inflammatory response, and interleukin (IL)-1β is an inflammatory factor causing retinal neurovascular injury. FGF21 protects photoreceptor cells from oxidative stress by upregulating the expression of the NRF2 protein in the DR retina and decreasing the production of IL-1β (37, 38). Photosensitive cells can release inflammatory products that stimulate the surrounding cells (39) and cause changes in retinal vascular permeability in diabetic mouse models (40). In addition, the effect of long-term FGF21 administration on the inhibition of retinal vascular leakage in in vivo and in vitro models has been verified (41). Retinal tissue is highly sensitive to metabolism, and photoreceptors contain the largest number of mitochondria in human cells. Among all cells in the retina, photoreceptors mount the largest response to retinal oxidative stress and inflammation caused by diabetes. In patients with PDR and subsequent retinitis pigmentosa, retinal neovascularization is slow. Therefore, enhancing the levels of antioxidants in photoreceptor cells can prevent neurovascular damage in DR; however, this is, to some extent, independent of the APN-TNF-α pathway (36).
Experimental evidence suggests that FGF21 is beneficial in DR. In the present study, we confirmed that FGF21 levels were significantly higher in patients with DR than in those without DR. It is difficult to explain this phenomenon in patients with DR. Some researchers have named this phenomenon “FGF21 resistance” (42, 43). Although this hypothesis is supported, the mechanism underlying FGF21 resistance has not yet been elucidated. Once the role of FGF21 is fully elucidated, however, we believe that it will contribute significantly to the treatment of DR.
The FGF21 coenzyme Klotho has been found to be expressed in the human retina, optic nerve, and lens (44, 45). Some evidence suggests that Klotho regulates many mechanisms involved in maintaining retinal cell homeostasis and function (44, 46, 47). First, Klotho-knockout mice exhibit several morphological changes compared with wild-type mice, including reduced pigmentation of the retinal pigment epithelium layer, enlarged choroidal vessels, thinning and deformation of the basement membrane, and signs of degeneration of the outer photoreceptor layer (46). Proteomic analysis has shown that proteins involved in eye development, visual perception, and mitochondrial function are downregulated in Klotho-knockout mice (47). Thus, Klotho-knockout mice have reduced retinal function, with functional defects comparable to those observed in insulin-like growth factor-1 knockout mice (44). Several experimental models have shown that depletion of Klotho negatively affects important functions of retinal cells, including oxidative stress, VEGF-A secretion, and phagocytosis, thereby activating mechanisms that may contribute to the onset and progression of DR. However, there is some evidence that treatment with recombinant Klotho improves retinal function.
The present study had some limitations. First, the duration of diabetes and disease severity varied among the included studies, and data regarding NPDR and PDR were limited. Second, the languages of literature search were limited to English and Chinese. As such, high-quality research investigating the role(s) of FGF21 and Klotho in the treatment of DR is warranted. It is, therefore, important to interpret the results of this meta-analysis cautiously.
Conclusion
This systematic review is the first to evaluate the relationship between FGF21 and Klotho levels and DR. FGF21 levels were significantly higher in patients with DR. Fully characterizing the role of FGF21 will significantly contribute to the treatment of DR.
Data availability statement
The original contributions presented in the study are included in the article/Supplementary Material. Further inquiries can be directed to the corresponding authors.
Author contributions
YJ: Writing – original draft. WZ: Writing – original draft. YX: Writing – original draft. XZ: Data curation, Writing – original draft. XS: Conceptualization, Writing – original draft, Writing – review & editing.
Funding
The author(s) declare that no financial support was received for the research, authorship, and/or publication of this article.
Acknowledgments
We would like to thank Editage (www.editage.cn) for English language editing.
Conflict of interest
The authors declare that the research was conducted in the absence of any commercial or financial relationships that could be construed as a potential conflict of interest.
Publisher’s note
All claims expressed in this article are solely those of the authors and do not necessarily represent those of their affiliated organizations, or those of the publisher, the editors and the reviewers. Any product that may be evaluated in this article, or claim that may be made by its manufacturer, is not guaranteed or endorsed by the publisher.
Supplementary material
The Supplementary Material for this article can be found online at: https://www.frontiersin.org/articles/10.3389/fendo.2024.1390035/full#supplementary-material
Supplementary Table 1 | Preferred reporting items for systematic review and meta-analyses (PRISMA) checklist.
Supplementary Figure 1 | The sensitivity analysis results of FGF21 level in patients with diabetic retinopathy compared to non-diabetic retinopathy diabetes patients.
Supplementary Figure 2 | The sensitivity analysis results of Klotho level in patients with diabetic retinopathy compared to non-diabetic retinopathy diabetes patients.
Supplementary Figure 3 | The Begg’s test of FGF21 level in patients with diabetic retinopathy compared to non-diabetic retinopathy diabetes patients.
Supplementary Figure 4 | The Egger’s test of FGF21 level in patients with diabetic retinopathy compared to non-diabetic retinopathy diabetes patients.
Supplementary Figure 5 | The Begg’s test of Klotho level in patients with diabetic retinopathy compared to non-diabetic retinopathy diabetes patients.
Supplementary Figure 6 | The Egger’s test of Klotho level in patients with diabetic retinopathy compared to non-diabetic retinopathy diabetes patients.
References
1. Cho NH, Shaw JE, Karuranga S, Huang Y, Da RFJ, Ohlrogge AW, et al. IDF Diabetes Atlas: Global estimates of diabetes prevalence for 2017 and projections for 2045. Diabetes Res Clin Pract. (2018) 138:271–81. doi: 10.1016/j.diabres.2018.02.023
2. Teo ZL, Tham YC, Yu M, Chee ML, Rim TH, Cheung N, et al. Global prevalence of diabetic retinopathy and projection of burden through 2045: systematic review and meta-analysis. Ophthalmology. (2021) 128:1580–91. doi: 10.1016/j.ophtha.2021.04.027
3. Wong TY, Cheung CM, Larsen M, Sharma S, Simo R. Diabetic retinopathy. Nat Rev Dis Primers. (2016) 2:16012. doi: 10.1038/nrdp.2016.12
4. Modjtahedi BS, Wu J, Luong TQ, Gandhi NK, Fong DS, Chen W. Severity of diabetic retinopathy and the risk of future cerebrovascular disease, cardiovascular disease, and all-cause mortality. Ophthalmology. (2021) 128:1169–79. doi: 10.1016/j.ophtha.2020.12.019
5. Olsen TW. Anti-VEGF pharmacotherapy as an alternative to panretinal laser photocoagulation for proliferative diabetic retinopathy. JAMA. (2015) 314:2135–6. doi: 10.1001/jama.2015.15409
6. Lin Y, Xiao YC, Zhu H, Xu QY, Qi L, Wang YB, et al. Serum fibroblast growth factor 21 levels are correlated with the severity of diabetic retinopathy. J Diabetes Res. (2014) 2014:929756. doi: 10.1155/2014/929756
7. Esteghamati A, Momeni A, Abdollahi A, Khandan A, Afarideh M, Noshad S, et al. Serum fibroblast growth factor 21 concentrations in type 2 diabetic retinopathy patients. Ann Endocrinol (Paris). (2016) 77:586–92. doi: 10.1016/j.ando.2016.01.005
8. Tomita Y, Ozawa N, Miwa Y, Ishida A, Ohta M, Tsubota K, et al. Pemafibrate prevents retinal pathological neovascularization by increasing FGF21 level in a murine oxygen induced retinopathy model. Int J Mol Sci. (2019) 20:5878. doi: 10.3390/ijms20235878
9. Nishimura T, Nakatake Y, Konishi M, Itoh N. Identification of a novel FGF, FGF-21, preferentially expressed in the liver. Biochim Biophys Acta. (2000) 1492:203–6. doi: 10.1016/S0167-4781(00)00067-1
10. Woo YC, Xu A, Wang Y, Lam KS. Fibroblast growth factor 21 as an emerging metabolic regulator: clinical perspectives. Clin Endocrinol (Oxf). (2013) 78:489–96. doi: 10.1111/cen.12095
11. Keuper M, Haring HU, Staiger H. Circulating FGF21 levels in human health and metabolic disease. Exp Clin Endocrinol Diabetes. (2020) 128:752–70. doi: 10.1055/a-0879-2968
12. Wells GA, Shea B, O’Connell D, et al. The Newcastle-Ottawa Scale (NOS) for Assessing the Quality of Non randomized Studies in Meta-Analyses . Available online at: http://www.ohri.ca/programs/clinical_epidemiology/oxford.asp (Accessed 2014 Aug 5).
13. Higgins JPT, Green S. Cochrane Handbook for Systematic Reviews of Interventions Version 5.1.0 . Available online at: http://www.cochrane-handbook.org (Accessed 2014 Aug).
14. Mousavi Z, Bonakdaran S, Sahebkar A, Yaghoubi G, Yaghoubi MA, Davoudian N, et al. The relationship between serum levels of fibroblast growth factor 21 and diabetic retinopathy. EXCLI J. (2017) 16:1249–56. doi: 10.17179/excli2017-672
15. Zhang L, Liu T. Clinical implication of alterations in serum Klotho levels in patients with type 2 diabetes mellitus and its associated complications. J Diabetes Complicat. (2018) 32:922–30. doi: 10.1016/j.jdiacomp.2018.06.002
16. Li LJ, Zhang M, Li ZX, Han XP, Zheng JC. Correlation between serum fibroblast growth factor 21 level and diabetic kidney disease and diabetic retinopathy in type 2 diabetic patients. Chin J Diabetes. (2019) 27:812–5. doi: 10.3969/j.issn.1006-6187.2019.11.003
17. Xu LZ, Tan HT, Zhang SQ, Liu F. Serum levels of fibroblast growth factor 21 in patients with type 2 diabetic retinopathy and its clinical significance. Chin J Gen Pract. (2020) 18:959–61. doi: 10.16766/j.cnki.issn.1674-4152.001402
18. Corcillo A, Fountoulakis N, Sohal A, Farrow F, Ayis S, Karalliedde J. Low levels of circulating anti-ageing hormone Klotho predict the onset and progression of diabetic retinopathy. Diabetes Vasc Dis Res. (2020) 17:2033390146. doi: 10.1177/1479164120970901
19. Ji B, Wei H, Ding Y, Liang H, Yao L, Wang H, et al. Protective potential of klotho protein on diabetic retinopathy: Evidence from clinical and in vitro studies. J Diabetes Invest. (2020) 11:162–9. doi: 10.1111/jdi.13100
20. Jin S, Xia N, Han L. Association between serum fibroblast growth factor 21 level and sight-threatening diabetic retinopathy in Chinese patients with type 2 diabetes. BMJ Open Diabetes Res Care. (2021) 9:e002126. doi: 10.1136/bmjdrc-2021-002126
21. Heidari Z, Hasanpour M. The serum fibroblast growth factor 21 is correlated with retinopathy in patients with type 2 diabetes mellitus. Diabetes Metab Syndr. (2021) 15:102296. doi: 10.1016/j.dsx.2021.102296
22. Cai LY, Luo G. Expression and significance of serum chemokine and fibroblast growth factor 21 in patients with diabetic retinopathy. J Pract Clin Med. (2021) 25:76–80. doi: 10.7619/jcmp.20211123
23. Wang F, Meng XD, Peng J. Changes of serum Klotho, NEP and Vaspin levels in patients with diabetic retinopathy and their clinical significance. Int J Lab Med. (2022) 43:791–5. doi: 10.3969/j.issn.1673-4130.2022.07.005
24. Gao YQ, Liang N. Predictive value of serum irisin, FGF-21, TNF-α and IL-6 combined detection for retinopathy in patients with type 2 diabetes mellitus. Lab Med Clinic. (2023) 20:3037–40. doi: 10.3969/j.issn.1672-9455.2023.20.021
25. Xiao T, Cai ZW, Lin J, Gao XL. Correlation between serum fibroblast growth factor 21 and retinal vessel diameter in type 2 diabetes mellitus. J Chronic Med. (2023) 24:1064–6. doi: 10.16440/J.CNKI.1674-8166.2023.07.29
26. Hu W. Correlation between Serum FGF23 and Klotho Protein Levels and Microvascular Complications in Type 2 Diabetes Mellitus. China: Xian Medical University (2022).
27. Jiang Y, Fan H, Xie J, Xu Y, Sun X. Association between adipocytokines and diabetic retinopathy: a systematic review and meta-analysis. Front Endocrinol (Lausanne). (2023) 14:1271027. doi: 10.3389/fendo.2023.1271027
28. Fruchart JC. Pemafibrate (K-877), a novel selective peroxisome proliferator activated receptor alpha modulator for management of atherogenic dyslipidemia. Cardiovasc Diabetol. (2017) 16:124. doi: 10.1186/s12933-017-0602-y
29. Struik D, Dommerholt MB, Jonker JW. Fibroblast growth factors in control of lipid metabolism: from biological function to clinical application. Curr Opin Lipidol. (2019) 30:235–43. doi: 10.1097/MOL.0000000000000599
30. Fu Z, Lofqvist CA, Shao Z, Sun Y, Joyal JS, Hurst CG, et al. Dietary ω-3 polyunsaturated fatty acids decrease retinal neovascularization by adipose endoplasmic reticulum stress reduction to increase adiponectin. Am J Clin Nutr. (2015) 101:879–88. doi: 10.3945/ajcn.114.099291
31. Lin Z, Tian H, Lam KS, Lin S, Hoo RC, Konishi M, et al. Adiponectin mediates the metabolic effects of FGF21 on glucose homeostasis and insulin sensitivity in mice. Cell Metab. (2013) 17:779–89. doi: 10.1016/j.cmet.2013.04.005
32. Higuchi A, Ohashi K, Kihara S, Walsh K, Ouchi N. Adiponectin suppresses pathological microvessel formation in retina through modulation of tumor necrosis factor-alpha expression. Circ Res. (2009) 104:1058–65. doi: 10.1161/CIRCRESAHA.109.194506
33. Kociok N, Radetzky S, Krohne TU, Gavranic C, Joussen AM. Pathological but not physiological retinal neovascularization is altered in TNF-Rp55-receptor-deficient mice. Invest Ophthalmol Vis Sci. (2006) 47:5057–65. doi: 10.1167/iovs.06-0407
34. Madsen-Bouterse SA, Kowluru RA. Oxidative stress and diabetic retinopathy: pathophysiological mechanisms and treatment perspectives. Rev Endocr Metab Disord. (2008) 9:315–27. doi: 10.1007/s11154-008-9090-4
35. Kern TS, Berkowitz BA. Photoreceptors in diabetic retinopathy. J Diabetes Investig. (2015) 6:371–80. doi: 10.1111/jdi.12312
36. Fu Z, Wang Z, Liu CH, Gong Y, Cakir B, Liegl R, et al. Fibroblast growth factor 21 protects photoreceptor function in type 1 diabetic mice. Diabetes. (2018) 67:974–85. doi: 10.2337/db17-0830
37. Wang MX, Zhao J, Zhang H, Li K, Niu LZ, Wang YP, et al. Potential protective and therapeutic roles of the nrf2 pathway in ocular diseases: an update. Oxid Med Cell Longev. (2020) 2020:9410952. doi: 10.1155/2020/9410952
38. Wooff Y, Man SM, Aggio-Bruce R, Natoli R, Fernando N. IL-1 family members mediate cell death, inflammation and angiogenesis in retinal degenerative diseases. Front Immunol. (2019) 10:1618. doi: 10.3389/fimmu.2019.01618
39. Tonade D, Liu H, Palczewski K, Kern TS. Photoreceptor cells produce inflammatory products that contribute to retinal vascular permeability in a mouse model of diabetes. Diabetologia. (2017) 60:2111–20. doi: 10.1007/s00125-017-4381-5
40. Honasoge A, Nudleman E, Smith M, Rajagopal R. Emerging insights and interventions for diabetic retinopathy. Curr Diabetes Rep. (2019) 19:100. doi: 10.1007/s11892-019-1218-2
41. Tomita Y, Fu Z, Wang Z, Cakir B, Cho SS, Britton W, et al. Long-acting FGF21 inhibits retinal vascular leakage in in vivo and in vitro models. Int J Mol Sci. (2020) 21:1188. doi: 10.3390/ijms21041188
42. Liu JJ, Foo JP, Liu S, Lim SC. The role of fibroblast growth factor 21 in diabetes and its complications: A review from clinical perspective. Diabetes Res Clin Pract. (2015) 108:382–9. doi: 10.1016/j.diabres.2015.02.032
43. So WY, Leung PS. Fibroblast growth factor 21 as an emerging therapeutic target for type 2 diabetes mellitus. Med Res Rev. (2016) 36:672–704. doi: 10.1002/med.21390
44. Reish NJ, Maltare A, McKeown AS, Laszczyk AM, Kraft TW, Gross AK, et al. The age-regulating protein klotho is vital to sustain retinal function. Invest Ophthalmol Vis Sci. (2013) 54:6675–85. doi: 10.1167/iovs.13-12550
45. Zhang Y, Wang L, Wu Z, Yu X, Du X, Li X. The expressions of klotho family genes in human ocular tissues and in anterior lens capsules of age-related cataract. Curr Eye Res. (2017) 42:871–5. doi: 10.1080/02713683.2016.1259421
46. Kokkinaki M, Abu-Asab M, Gunawardena N, Ahern G, Javidnia M, Young J, et al. Klotho regulates retinal pigment epithelial functions and protects against oxidative stress. J Neurosci. (2013) 33:16346–59. doi: 10.1523/JNEUROSCI.0402-13.2013
Keywords: FGF21, Klotho, diabetic retinopathy, fibroblast growth factor 21, DR
Citation: Jiang Y, Zhang W, Xu Y, Zeng X and Sun X (2024) Relationship of fibroblast growth factor 21, Klotho, and diabetic retinopathy: a meta-analysis. Front. Endocrinol. 15:1390035. doi: 10.3389/fendo.2024.1390035
Received: 22 February 2024; Accepted: 08 August 2024;
Published: 27 August 2024.
Edited by:
Honghua Yu, Guangdong Provincial People’s Hospital, ChinaReviewed by:
Qi Feng Liu, First People’s Hospital of Kunshan, ChinaKunbei Lai, Sun Yat-sen University, China
Copyright © 2024 Jiang, Zhang, Xu, Zeng and Sun. This is an open-access article distributed under the terms of the Creative Commons Attribution License (CC BY). The use, distribution or reproduction in other forums is permitted, provided the original author(s) and the copyright owner(s) are credited and that the original publication in this journal is cited, in accordance with accepted academic practice. No use, distribution or reproduction is permitted which does not comply with these terms.
*Correspondence: Xin Sun, c3VueGluNzdAMTI2LmNvbQ==; Xiandong Zeng, emVuZ194aWFuZG9uZzE5NjlAMTYzLmNvbQ==
†These authors have contributed equally to this work