- Department of Pharmacy, Henan Provincial People’s Hospital, People’s Hospital of Zhengzhou University, School of Clinical Medicine, Henan University, Zhengzhou, Henan, China
Prior research has indicated that bisphosphonates (BPs) can improve periodontal disease because of their anti-osteoporosis properties. In vitro studies have shown that BPs induce cytotoxicity, inhibit wound healing, and thus affect periodontal disease. Denosumab and BPs have alternative indications. BP and denosumab are not known to correlate with gingival disorders. We assessed such a relationship by applying Bayesian and nonproportional analyses to data in the US FDA Adverse Event Reporting System (FAERS) database. The study analyzed BPs and denosumab-reported incidents with preferred terms found in the narrow Standardized MedDRA Queries for gingival disorders. A total of 5863 reported cases of gingival disorders were associated with five BPs (alendronate, pamidronate, ibandronate, risedronate, and zoledronate) and denosumab. More than 15% of patients with gingival disorders related to BPs and denosumab other than denosumab were hospitalized over short- or long-term periods. Our findings indicated BPs and denosumab had significant reporting odds ratios (ROR), proportional reporting ratios (PRR), and information components (IC) with respect to gingival disorders. Pamidronate had the highest association (ROR = 64.58, PRR = 57.99, IC = 5.71), while the weakest association was found with denosumab (ROR = 3.61, PRR = 3.60, IC = 1.77). Significant associations were found between the six drugs and gingival pain, gingival recession, gingivitis, periodontal disease, and periodontitis. In conclusion, our comprehensive overview of the correlations, clinical characteristics, and prognoses of BPs and denosumab-related gingival disorders suggests that these issues deserve continued surveillance and appropriate management.
1 Introduction
Drugs that counteract bone resorption, known as antiresorptive drugs (ARDs), interfere with bone metabolism to reduce abnormal bone remodeling or bone resorption (1). The most commonly used ARDs are calcium supplements, bisphosphonates (BPs), hormones, inhibitors of receptor activator of nuclear factor-κB ligand (RANKL), and other categories. BPs exert their antiresorptive effects through the inhibition of osteoclasts, which helps to restrict bone resorption and preserve bone density (2), while RANKL inhibitors block the activity of a crucial mediator in the regulation of osteoclast activity (3).
BPs can be classified into two main groups: nitrogen-containing (N-BP) and non-nitrogen-containing (NN-BP), with the former being more potent and more commonly prescribed. A commonly used RANKL inhibitor is the monoclonal antibody denosumab. These drugs are widely used in the treatment of various skeletal disorders, such as osteoporosis, malignancy-associated bone disease, and Paget’s disease (4, 5). Denosumab offers convenient administration and lacks renal metabolism, making it a potential alternative to BPs for treating osteoporosis and tumor bone metastasis. The most common adverse events for both classes of drugs were hypocalcemia, pain, and flu-like symptoms (6).
Gingival disease can lead to infection of the periodontal tissues and progressive loss of alveolar bone, ultimately resulting in tooth loss in severe cases (7). While previous studies have demonstrated the potential efficacy of BPs in improving periodontitis (8–10), it is noteworthy that the use of denosumab and BPs is associated with rare osteonecrosis of the jaw (ONJ) (11), which can cause alveolar bone loss, tooth mobility, and exacerbation of gum disease symptoms. Systematic analyses of these potential connections have not yet been reported. Therefore, this pharmacovigilance study aims to perform such an analysis utilizing data from the US FDA Adverse Event Reporting System (FAERS).
2 Methods
2.1 Data source
Data were gathered from the US Food and Drug Administration Public Data Open Project (Open FDA), which sourced raw data from the FAERS database. Extraction of data was carried out with OpenVigil2.1, an open pharmacovigilance data extraction, mining and analysis tool specifically designed for use with the FAERS database (12). OpenVigil2.1 operates solely on cleaned FDA data, with most duplicates and reports containing incomplete information being deleted.
In FAERS, adverse events are coded using preferred terms (PTs) from the Medical Dictionary for Regulatory Activities (MedDRA) (version 25.0). A specific PT may be assigned to multiple high-level terms (HLTs), high-level group terms (HLGTs), and system organ classes (SOCs). In addition, all PTs representing symptoms, signs, investigations, or diagnoses with potential relevance can be categorized into meaningful groups using Standardized MedDRA Queries (SMQs) to define a medical condition of interest. The present study incorporated the narrow SMQ of gingival disorders, which comprised 26 PTs (Table 1).
2.2 Data extraction
This retrospective study gathered data from the FAERS database between Q4 2003 and Q2 2023. Nitrogen-containing BPs (alendronate, ibandronate, pamidronate, risedronate, and zoledronate) and a RANKL inhibitor (denosumab) were identified as primary suspects in the reports. Duplicate entries with the same Individual Safety Report number were eliminated prior to analysis. In this report, the SMQ-narrow and PT dimensions are used to analyze the association of gingival disease with BPs and denosumab.
2.3 Data mining
Following the fundamental principles of Bayesian and non-proportional analysis, we utilized reporting odds ratio (ROR) (13), proportional reporting ratio (PRR) (14), and Bayesian confidence propagation neural network (BCPNN) algorithms (15) to investigate the correlation between drugs and the negative impact of gingival disorders. The equations and criteria for the three algorithms are shown in Table 2; if any of the three algorithms met the criteria, it was considered a positive signal.
2.4 Statistical analysis
A descriptive analysis was carried out to summarize the clinical traits of patients suffering from gingival disorders linked to BPs and denosumab. The continuous variable was presented as mean ± standard deviation (SD), and the frequency variable was expressed as a percentage. Pearson’s chi-square or Fisher’s exact test were used to compare the intervention and hospitalization rates of various ARDs. All statistical analyses were performed using IBM® SPSS® Statistics (version 26). Results with a P value less than 0.05 were deemed statistically significant.
3 Results
3.1 Descriptive analysis
The clinical features of patients with BPs- and denosumab-induced gingival disorders are presented in Table 3. The median age of patients who received BPs was 63.2 ± 9.2 years, whereas the median age of those who received denosumab was 71.0 ± 10.7 years. Female patients were affected more often than male patients. Over 60% of patients using alendronate, ibandronate, pamidronate, risedronate, and denosumab came from the United States, compared with 42.7% for zoledronate. Reports associated with BPs were most highly concentrated from 2009 through 2013, and while reports associated with denosumab were concentrated from 2014 to 2018.
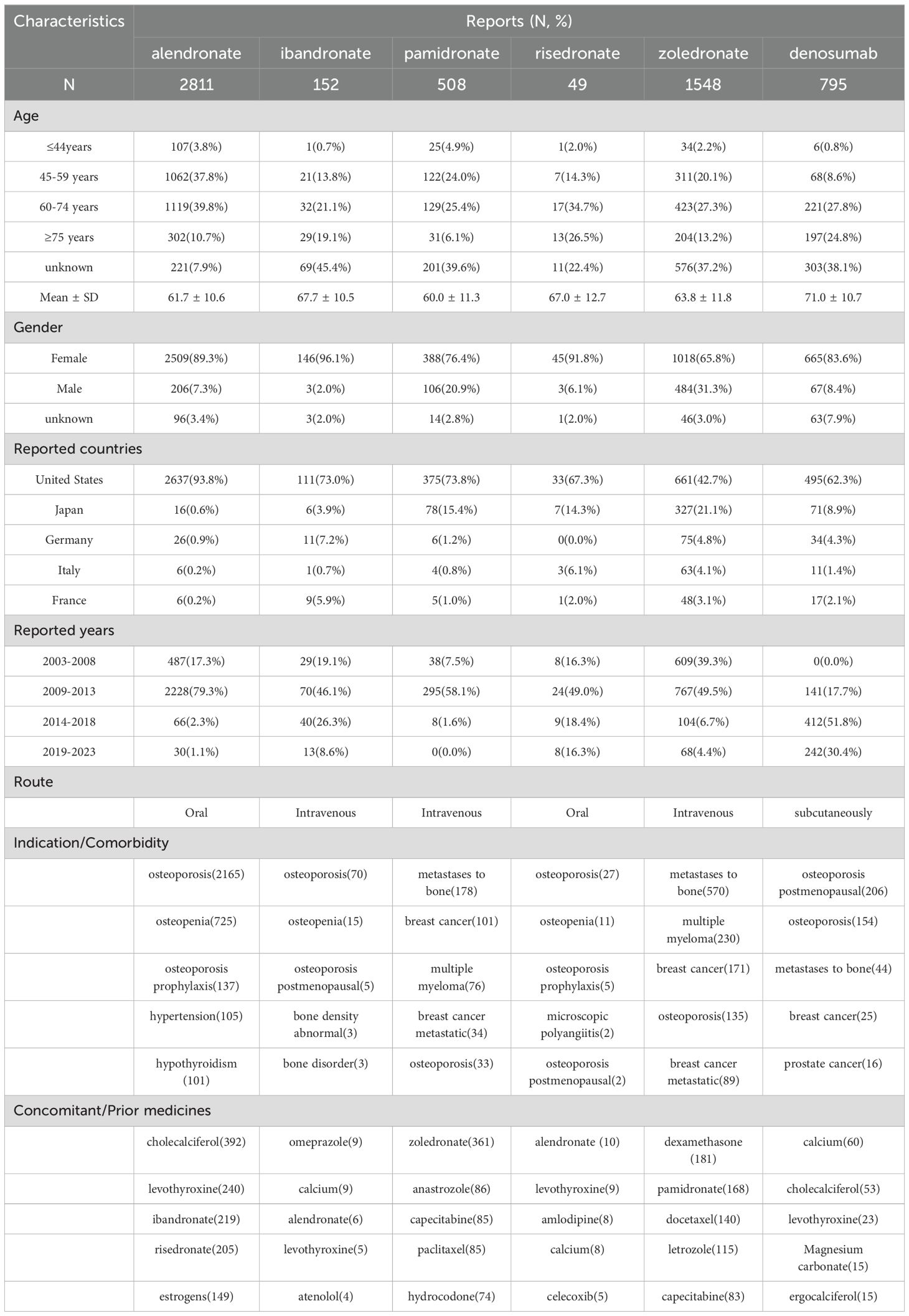
Table 3. The clinical characteristics of patients with BPs- and denosumab-related gingival disorders.
The therapeutic uses of the drugs in this study were different. Alendronate, ibandronate, and risedronate were mainly used to treat osteoporosis and osteopenia and as prophylaxis for osteoporosis. Zoledronate, pamidronate, and denosumab were used to treat osteoporosis, but they were also used to treat primary tumors, including breast cancers, and metastases to bone. The most commonly reported concomitant and prior medications were calcium supplements, other BPs, levothyroxine, antitumor agents, and nonsteroidal anti-inflammatory drugs.
3.2 Bayesian and nonproportional analyses
From Q4 2003 through Q2 2023, a total of 13,165,131 cases were recorded, including 24,025 cases of gingival disorders. Signal detection of BPs and denosumab-related gingival disease is shown in Table 4. The numbers of reported cases of gingival disorders associated with alendronate, pamidronate, risedronate, zoledronate, ibandronate, and denosumab were 2811, 508, 49, 1548, 152, and 795, respectively. Based on the aforementioned criteria, all six drugs showed statistical significance in terms of ROR, PRR, and information component (IC). Of these, pamidronate had the strongest association with gingival disorders (ROR = 64.58, PRR = 57.99, IC = 5.71), while the weakest association was found with denosumab (ROR = 3.61, PRR = 3.60, IC = 1.77).
The analysis focused on the reported occurrences of BPs and denosumab with PTs present in the narrow SMQ for gingival disorders, along with their corresponding PRR values (Table 5). Gingival bleeding, gingival disorder, gingival pain, gingival recession, gingivitis, periodontal disease, and periodontitis were the PTs that exhibited the most common occurrence in gingival diseases, accounting for more than 80% of the PTs. The PRR values of the drugs and the common PTs were greater than 2, and ibandronate, denosumab, and gingival bleeding were excluded. Adverse events with more than two reported cases were selected for correlation analysis with all drugs (Figure 1). The findings revealed high correlations between the six drugs and gingival pain, gingival recession, gingivitis, periodontal disease, and periodontitis.
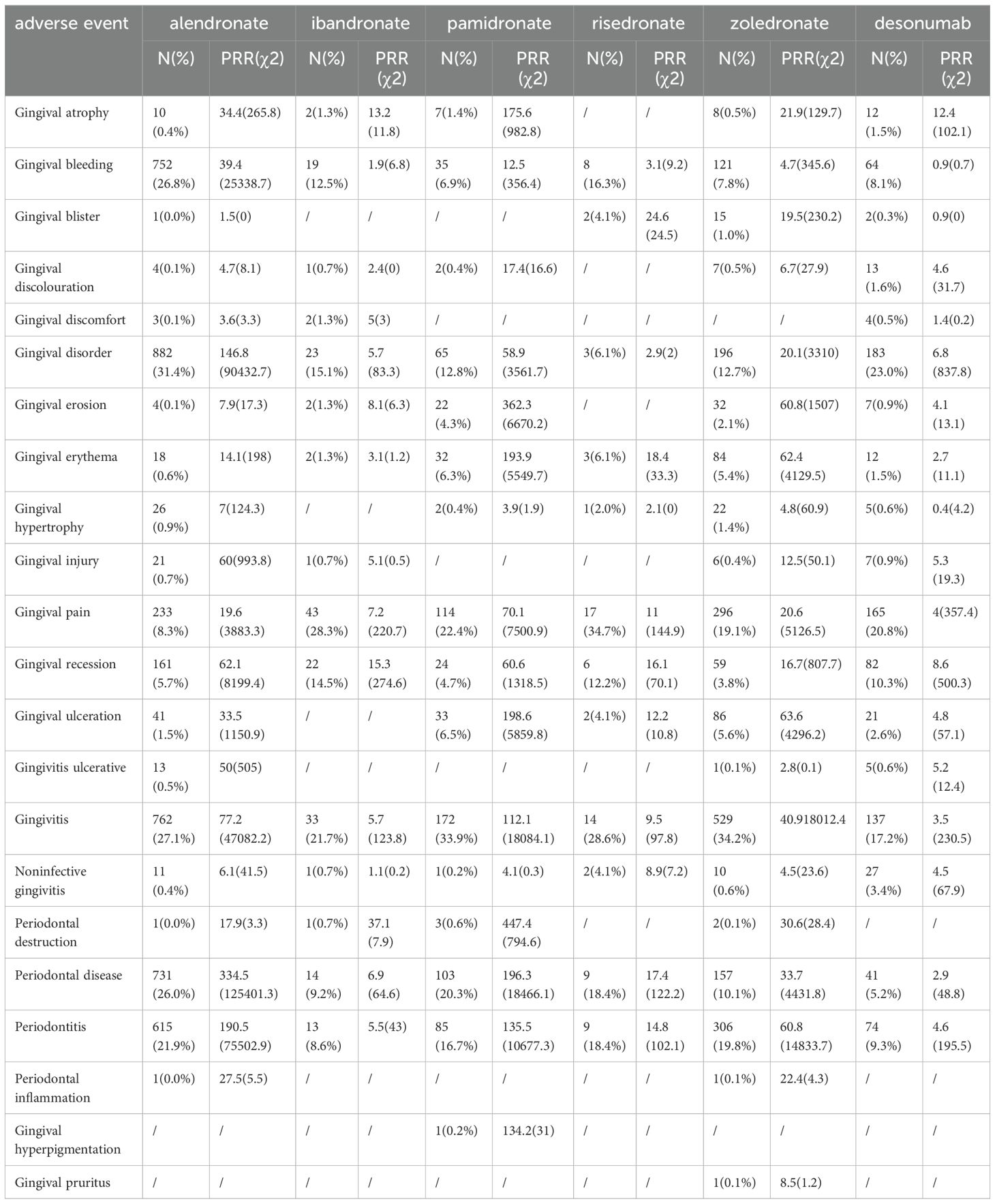
Table 5. The correlation analysis of PTs included the narrow SMQ for BPs and denosumab with gingival disease.
3.3 The rates of intervention and hospitalization linked to gingival disorders caused by BPs and denosumab
To assess the impact of BPs and denosumab-related gingival disease, the proportion of patients who underwent intervention and/or were hospitalized was analyzed (Figure 2). Of the six drugs, alendronate was associated with the highest hospitalization rate for BP-related gingival disorders (69.37%). The rates associated with ibandronate, pamidronate, risedronate, zoledronate, and denosumab were 15.13%, 36.22%, 30.61%, 19.70%, and 6.24%, respectively. The intervention rate associated with risedronate was 4.08%, significantly higher than for other drugs.
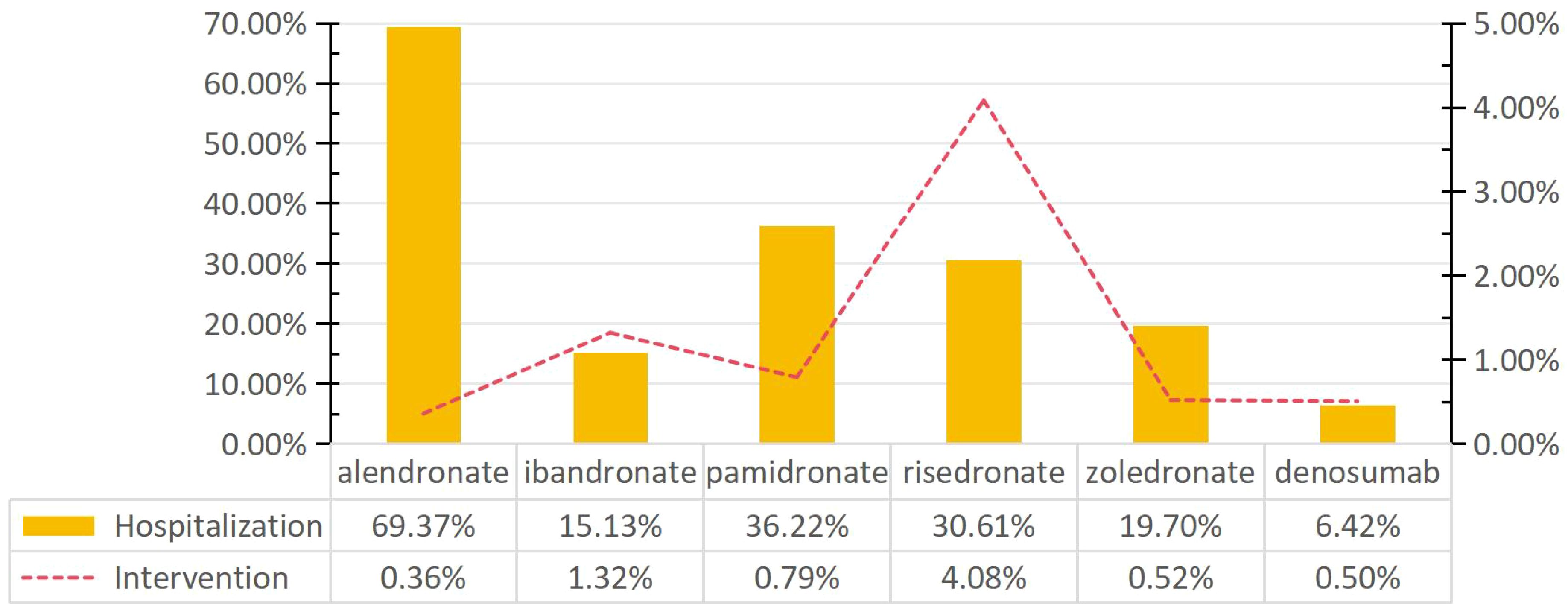
Figure 2. Rates of intervention and hospitalization for BPs- and denosumab-related gingival disorders.
4 Discussion
The purpose of this study was to analyze the association between BPs and denosumab with gingival disease, as well as the clinical characteristics and differences of the various drug interventions. To our knowledge, this analysis, which was based on FAERS database receipts from Q4 2003 to Q2 2023, is the first and largest such analysis of real-world data.
The study showed that BPs and denosumab were relevant to gingival disease. In the study of drugs, pamidronate and alendronate had strong correlations with gingival disorders, with respective ROR values of 64.58 and 51.20. In contrast, denosumab showed a relatively weaker association (ROR = 3.61). When analyzing the correlation of each drug with PTs included in the SMQ of gingival disorders, pamidronate and alendronate demonstrated the strongest correlations with the highest number of reported PTs (gingivitis, gingival pain, periodontal disease, periodontitis, gingival disorder, gingival bleeding, gingival ulceration, and gingival recession), while the lowest correlation was observed with denosumab.
Gingival lesions associated with BPs and denosamab are more prevalent in middle-aged and elderly women. The reported cases mainly fall within the 60-74 age group, and the proportion of alendronate-reported cases was 40%. A possible reason for these associations may be that the predominant indication for using these ARDs is post-menopausal osteoporosis. In addition, a reason why usage is lower in higher age ranges is that the metabolism of BPs depends on kidney function, and decreased renal function in older patients may increase the risk of adverse reactions. The management of osteoporosis and bone metastasis often requires prolonged use of ARDs. In clinical practice, other BPs are frequently administered sequentially for convenience or to mitigate adverse reactions. This study revealed that over 15% of patients who had used Alendronate, Pamidronate, and Zoledronate had previously been treated with other BPs, with Pamidronate accounting for 71.07%.
Gingivitis associated with BPs and denosamab often has a significant impact on quality of life, often requiring either short- or long-term hospitalization and other interventions to prevent permanent impairment or damage. More than 15% of patients with gingival disease associated with BPs required either short- or long-term hospitalization. In particular, alendronate had the highest hospitalization rate (69.37%), which was significantly higher than that of the other drugs (Pearson’s chi-squared test, P < 0.05). In contrast, the intervention rate for gingival disease related to alendronate was 0.36%, which was lower than that of other drugs. Furthermore, we observed that risedronate was associated with the highest risk of intervention for gingival disease compared to other drugs (4.08%, Pearson’s chi-squared test, P > 0.05).
Previous studies have indicated that BPs impede the healing process of the oral mucosa and lead to delayed wound closure (16). BP and denosamab may cause rare osteonecrosis of the jaw, which can lead to oral ulcers, alveolar bone loss, tooth loosening, etc. In vitro studies have provided additional evidence showcasing the genotoxic and cytotoxic effects of BPs on oral tissues (11). These agents have been shown to inhibit cell proliferation, metabolism, viability, and migration, alter cell morphology, and induce apoptosis and inflammation (17). The impacts of BPs on various types of oral cells, such as gingival fibroblasts, oral keratinocytes, periodontal ligament fibroblasts, periodontal ligament stem cells, and oral fibroblasts, have been investigated in several studies, and the findings suggest that BPs can lead to reduced cell proliferation, migration, and metabolism, as well as decreased viability and increased apoptosis (18–21).
N-BPs in particular have been found to inhibit the activity of transforming growth factor β (TGF-β) (23), which is an important factor in the differentiation of fibroblasts into myofibroblasts and thus the promotion of wound healing, cell migration, viability, and proliferation (22). Through this mechanism, N-BPs have been shown to impair re-epithelialization of oral mucosal tissue and to reduce wound healing (23). It has also been suggested that N-BPs can increase the production of reactive oxygen species (ROS) by inhibiting farnesyl pyrophosphate. ROS generation may regulate cell growth factors and signal pathways to reduce cell migration and proliferation (21). Furthermore, N-BP was found to down-regulate cyclin D1 expression in orofacial mesenchymal stem cells (24), resulting in cell division arrest at the G0/G1 phase and the hindering of cell proliferation. The induction of ROS generation by N-BP, as well as the upregulation of production of endogenous (TNF, TNF-receptor associated factor, and death domain) and exogenous (B-cell lymphoma 2, inhibitor of apoptosis, and caspase) apoptotic pathway-related factors in oral tissues (19), may explain the increased rate of gum tissue cell apoptosis (25).
Similarly, denosumab has been shown to inhibit the secretion of interferon-γ in gingival tissue and to enhance the cytotoxicity of natural killer cells (26), leading to an imbalance in the gingival microenvironment. Furthermore, denosumab upregulates the production of the pro-apoptotic factors Bad, Bax, and Bim, inhibits osteoclast activity, and delays the healing of oral bone injuries (27). The research investigating these mechanistic connections have mainly involved in vitro tests or experiments in non-human animals; therefore, additional clinical research or longer clinical studies are needed.
This study benefits from real-world research and data mining techniques; however, we also recognize several limitations. Firstly, our methods were unable to distinguish accurate data from false or inaccurately reported data. Secondly, while we were able to extract statistics from the basic patient information, the accuracy of concomitant diseases and medication histories was unclear, introducing potential confounding factors and uncertainties into our analysis. Thirdly, data mining using Bayesian and nonproportional analyses can only establish statistical associations and not causal relationships between adverse events and drugs.
Despite these limitations, our findings identified a significant association between reports of gingival disorders and patients taking BPs and denosamab. However, the lack of research on BPs and denosamab-related gingival disorders makes it challenging to establish a definitive link between the drugs and the adverse events. Further clinical, anatomical, or imaging studies involving large-scale human populations are necessary to provide more comprehensive explanations.
Data availability statement
The original contributions presented in the study are included in the article/supplementary material. Further inquiries can be directed to the corresponding author.
Author contributions
LW: Data curation, Formal analysis, Writing – original draft. WZ: Formal analysis, Methodology, Visualization, Writing – review & editing. CZ: Formal analysis, Methodology, Visualization, Writing – review & editing. ZF: Conceptualization, Writing – review & editing.
Funding
The author(s) declare that no financial support was received for the research, authorship, and/or publication of this article.
Conflict of interest
The authors declare that the research was conducted in the absence of any commercial or financial relationships that could be construed as a potential conflict of interest.
Publisher’s note
All claims expressed in this article are solely those of the authors and do not necessarily represent those of their affiliated organizations, or those of the publisher, the editors and the reviewers. Any product that may be evaluated in this article, or claim that may be made by its manufacturer, is not guaranteed or endorsed by the publisher.
References
1. Stavropoulos A, Bertl K, Pietschmann P, Pandis N, Schiødt M, Klinge B. The effect of antiresorptive drugs on implant therapy: Systematic review and meta-analysis. Clin Oral Implants Res. (2018) 29:54–92. doi: 10.1111/clr.13282
2. Drake MT, Clarke BL, Khosla S. Bisphosphonates: mechanism of action and role in clinical practice. Mayo Clin Proc. (2008) 83:1032–45. doi: 10.4065/83.9.1032
3. Hanley DA, Adachi JD, Bell A, Brown V. Denosumab: mechanism of action and clinical outcomes. Int J Clin Pract. (2012) 66:1139–46. doi: 10.1111/ijcp.12022
4. Russell RG. Bisphosphonates: the first 40 years. Bone. (2011) 49:2–19. doi: 10.1016/j.bone.2011.04.022
5. Tsourdi E, Rachner TD, Rauner M, Hamann C, Hofbauer LC. Denosumab for bone diseases: translating bone biology into targeted therapy. Eur J Endocrinol. (2011) 165:833–40. doi: 10.1530/EJE-11-0454
6. Jara MA, Varghese J, Hu MI. Adverse events associated with bone-directed therapies in patients with cancer. Bone. (2022) 158:115901. doi: 10.1016/j.bone.2021.115901
7. Koide Y, Kataoka Y, Hasegawa T, Ota E, Noma H. Effect of systemic bisphosphonate administration on patients with periodontal disease: a systematic review and meta-analysis protocol. BMJ Open. (2022) 12:e57768. doi: 10.1136/bmjopen-2021-057768
8. Penoni DC, Leao A, Torres SR, Farias MLF, Fernandes TM, Crivelli M, et al. Effects of bone fragility and antiresorptive drugs on periodontal disease and tooth loss: A longitudinal study. JDR Clin Trans Res. (2018) 3:378–87. doi: 10.1177/2380084418787451
9. Akram Z, Abduljabbar T, Kellesarian SV, Abu Hassan MI, Javed F, Vohra F. Efficacy of bisphosphonate as an adjunct to nonsurgical periodontal therapy in the management of periodontal disease: a systematic review. Br J Clin Pharmacol. (2017) 83:444–54. doi: 10.1111/bcp.13147
10. Pavanelli A, de Menezes BS, Pereira E, de Souza Morais FA, Cirelli JA, de Molon RS. Pharmacological therapies for the management of inflammatory bone resorption in periodontal disease: A review of preclinical studies. BioMed Res Int. (2022) 2022:5832009. doi: 10.1155/2022/5832009
11. Beth-Tasdogan NH, Mayer B, Hussein H, Zolk O, Peter JU. Interventions for managing medication-related osteonecrosis of the jaw. Cochrane Database Syst Rev. (2022) 7:CD12432. doi: 10.1002/14651858.CD012432.pub3
12. Kass-Hout TA, Xu Z, Mohebbi M, Nelsen H, Baker A, Levine J, et al. OpenFDA: an innovative platform providing access to a wealth of FDA’s publicly available data. J Am Med Inform Assoc. (2016) 23:596–600. doi: 10.1093/jamia/ocv153
13. Sakaeda T, Tamon A, Kadoyama K, Okuno Y. Data mining of the public version of the FDA Adverse Event Reporting System. Int J Med Sci. (2013) 10:796–803. doi: 10.7150/ijms.6048
14. Slattery J, Alvarez Y, Hidalgo A. Choosing thresholds for statistical signal detection with the proportional reporting ratio. Drug Saf. (2013) 36:687–92. doi: 10.1007/s40264-013-0075-1
15. Hauben M, Bate A. Decision support methods for the detection of adverse events in post-marketing data. Drug Discovery Today. (2009) 14:343–57. doi: 10.1016/j.drudis.2008.12.012
16. Landesberg R, Cozin M, Cremers S, Woo V, Kousteni S, Sinha S, et al. Inhibition of oral mucosal cell wound healing by bisphosphonates. J Oral Maxillofac Surg. (2008) 66:839–47. doi: 10.1016/j.joms.2008.01.026
17. Pabst AM, Ziebart T, Koch FP, Taylor KY, Al-Nawas B, Walter C. The influence of bisphosphonates on viability, migration, and apoptosis of human oral keratinocytes–in vitro study. Clin Oral Investig. (2012) 16:87–93. doi: 10.1007/s00784-010-0507-6
18. McLeod NM, Moutasim KA, Brennan PA, Thomas G, Jenei V. In vitro effect of bisphosphonates on oral keratinocytes and fibroblasts. J Oral Maxillofac Surg. (2014) 72:503–9. doi: 10.1016/j.joms.2013.08.007
19. Scheper MA, Badros A, Chaisuparat R, Cullen KJ, Meiller TF. Effect of zoledronic acid on oral fibroblasts and epithelial cells: a potential mechanism of bisphosphonate-associated osteonecrosis. Br J Haematol. (2009) 144:667–76. doi: 10.1111/j.1365-2141.2008.07504.x
20. Soydan SS, Araz K, Senel FV, Yurtcu E, Helvacioglu F, Dagdeviren A, et al. Effects of alendronate and pamidronate on apoptosis and cell proliferation in cultured primary human gingival fibroblasts. Hum Exp Toxicol. (2015) 34:1073–82. doi: 10.1177/0960327115569808
21. Taniguchi N, Osaki M, Onuma K, Ishikawa M, Ryoke K, Kodani I, et al. Bisphosphonate-induced reactive oxygen species inhibit proliferation and migration of oral fibroblasts: A pathogenesis of bisphosphonate-related osteonecrosis of the jaw. J Periodontol. (2020) 91:947–55. doi: 10.1002/JPER.19-0385
22. Komatsu Y, Ibi M, Chosa N, Kyakumoto S, Kamo M, Shibata T, et al. Zoledronic acid suppresses transforming growth factor-beta-induced fibrogenesis by human gingival fibroblasts. Int J Mol Med. (2016) 38:139–47. doi: 10.3892/ijmm.2016.2582
23. Marcuzzi A, Zanin V, Crovella S, Pontillo A. Comments on ‘‘Geranylgeraniol–a new potential therapeutic approach to bisphosphonate associated osteonecrosis of the jaw” by Ziebart T et al. (2011). Oral Oncol. (2011) 47:436–437, 438. doi: 10.1016/j.oraloncology.2011.02.017
24. Wang Y, Akintoye SO. Primary cilia enhance osteogenic response of jaw mesenchymal stem cells to hypoxia and bisphosphonate. J Oral Maxillofac Surg. (2021) 79:2487–98. doi: 10.1016/j.joms.2021.07.027
25. Wiziack ZP, Oliveira SI, Servat-Medina L, Jorge MP, Lima Neto LG, Hass V, et al. Standardized arrabidaea chica extract shows cytoprotective effects in zoledronic acid-treated fibroblasts and osteoblasts. Clin Cosmet Investig Dent. (2020) 12:327–33. doi: 10.2147/CCIDE.S259158
26. Kaur K, Sun Y, Kanayama K, Morinaga K, Hokugo A, Nishimura I, et al. Augmentation of IFN-gamma by bone marrow derived immune cells in the presence of severe suppression of IFN-gamma in gingivae induced by zoledronic acid and denosumab in Hu-BLT mice model of ONJ. Front Endocrinol (Lausanne). (2023) 14:1111627. doi: 10.3389/fendo.2023.1111627
Keywords: antiresorptive drugs, FAERS, gingival disorders, adverse drug reactions, bisphosphonates, denosumab
Citation: Wang L, Zhang W, Zhao C-l and Fu Z-h (2024) Bisphosphonate- and disumab-related gingival disorders: case analysis from the U.S. Food and Drug Administration Adverse Event Reporting System. Front. Endocrinol. 15:1367607. doi: 10.3389/fendo.2024.1367607
Received: 21 February 2024; Accepted: 07 August 2024;
Published: 22 August 2024.
Edited by:
Eleonore Fröhlich, Medical University of Graz, AustriaReviewed by:
Ali Tafazoli, Queen’s University, CanadaYunzhou Fan, The Ohio State University, United States
Copyright © 2024 Wang, Zhang, Zhao and Fu. This is an open-access article distributed under the terms of the Creative Commons Attribution License (CC BY). The use, distribution or reproduction in other forums is permitted, provided the original author(s) and the copyright owner(s) are credited and that the original publication in this journal is cited, in accordance with accepted academic practice. No use, distribution or reproduction is permitted which does not comply with these terms.
*Correspondence: Zhong-hua Fu, zhaocl725@zzu.edu.cn