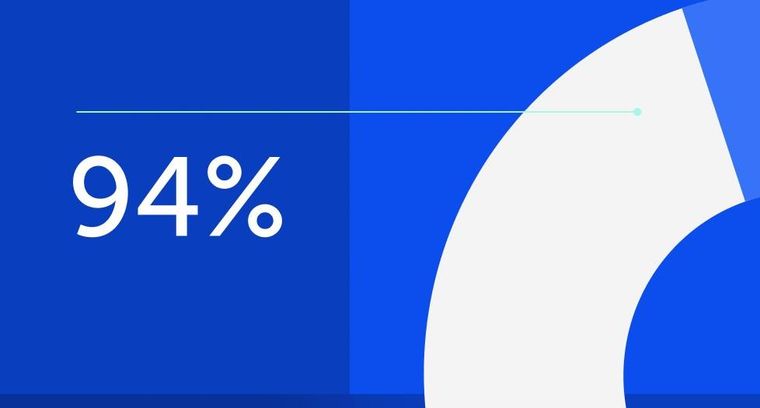
94% of researchers rate our articles as excellent or good
Learn more about the work of our research integrity team to safeguard the quality of each article we publish.
Find out more
REVIEW article
Front. Endocrinol., 05 April 2024
Sec. Reproduction
Volume 15 - 2024 | https://doi.org/10.3389/fendo.2024.1365602
The 25 kDa-sized protein Lipocalin 2 (LCN2) was originally isolated from human neutrophil granulocytes more than 30 years ago. LCN2 is an emerging player in innate immune defense, as it reduces bacterial growth due to its ability to sequester iron-containing bacterial siderophores. On the other hand, LCN2 also serves as a transporter for various hydrophobic substances due to its β-barrel shaped structure. Over the years, LCN2 has been detected in many other cell types including epithelial cells, astrocytes, and hepatocytes. Studies have clearly shown that aberrant expression of LCN2 is associated with a variety of disorders and malignancies, including several diseases of the reproductive system. Furthermore, LCN2 was proposed as a non-invasive prognostic and/or diagnostic biomarker in this context. Although several studies have shed light on the role of LCN2 in various disorders of the female and male reproductive systems, including tumorigenesis, a comprehensive understanding of the physiological function of LCN2 in the reproductive tract is still lacking. However, there is evidence that LCN2 is directly related to fertility, as global depletion of Lcn2 in mice has a negative effect on their pregnancy rate. Since LCN2 expression can be regulated by steroid hormones, it is not surprising that its expression fluctuates greatly during remodeling processes in the female reproductive tract, especially in the uterus. Well-founded details about the expression and regulation of LCN2 in a healthy reproductive state and also about possible changes during reproductive aging could contribute to a better understanding of LCN2 as a target in various diseases. Therefore, the present review summarizes current knowledge about LCN2 in the reproductive system, including studies in rodents and humans, and discusses changes in LCN2 expression during pathological events. The limited data suggest that LCN2 is expressed and regulated differently in healthy male and female reproductive organs.
Lipocalin 2 (LCN2) is a member of the lipocalin family of proteins known for its specific lipocalin fold that forms a hydrophobic pocket, thus allowing them to act as transport proteins (1). LCN2 was first isolated from human neutrophils and reported as neutrophil gelatinase-associated protein (NGAL) that served as bacterial iron sequester (2). It is believed that its multiple functions probably stem from its ability to act as a monomeric protein (25 kDa), as a homodimer (~46 kDa), and as a heterodimer (~135 kDa) in a complex with matrix metalloproteinase 9 (MMP9) (3). Importantly, only human LCN2 can form dimers (4), whereas murine LCN2 lacks Cys87 which is required for covalent disulfide bond formation (5).
Due to its pleiotropic nature, LCN2 was rediscovered and given several names, some of which being oncogene 24p3, siderocalin, p25, α2-microglobulin-related protein and migration-stimulating factor inhibitor (MSFI) (6–8). The protein was given the name siderocalin because LCN2 has the ability to reduce bacterial growth, as it can bind iron indirectly via bacterial siderophores (6, 9). In addition to its physiological role in host immune defense, aberrant expression of LCN2 has been observed under various pathophysiological conditions (10–12).
A role of LCN2 in reproductive tissue was suspected early on after its discovery. Because LCN2 was found to be strongly expressed in the uterine tissue of mice, the term ‘uterocalin’ for LCN2 was introduced (13). However, there are relatively few historical studies in the area of LCN2 in the reproductive system. Even less data is available on the molecular mechanisms by which LCN2 mediates signal transduction in the healthy reproductive system. Currently, three different putative LCN2 receptors, namely soluble carrier family 22 member 17 (SLC22A17), megalin and melanocortin-4 receptor (MC4R), have been described and comprehensively reviewed in (14). The role of these receptors in the reproductive tract is still poorly understood. However, there is evidence that sex hormones influence the expression of LCN2 in mice and humans (15–17).
With this review, our goal was to summarize the available knowledge on LCN2 in female and male reproductive organs. The focus was mainly on the female reproductive tract (uterus, vagina, and ovary). However, in the male reproductive tract, we concentrated on the expression of LCN2 in the testes and epididymis, as its expression in healthy and diseased prostates has been previously summarized (10).
The female reproductive system is tightly regulated by hormones and consists of the vagina, the cervix, the uterus, the fallopian tubes, and the ovaries (18). Ovaries produce and secrete steroid hormones and are responsible for the maturation and release of oocytes. Fertilization takes place in the fallopian tubes (also known as oviducts), after which the fertilized oocyte is transported to the uterus for implantation (19). The cervix not only connects the uterus to the vagina, through which sperm reach the site of fertilization and forms the birth canal, but also serves as a barrier to invading pathogens (20).
Although murine models are used for the research of reproductive organs, notable differences can be observed between human and murine reproductive systems. Unlike humans, which possess a pyriform uterus, mice have a bicornuate uterus consisting of uterine horns with multiple implantation sites that open into the uterine junction (21). Compared to the size of the reproductive tract, mice have rather small oviducts (21). Furthermore, the reproductive system of mice is more similar to that of rats than that of primates (22). Moreover, the time of gestation and the number of offspring differ between humans and rodents (22).
Although the existence of LCN2 in the uterus has been known for more than 25 years (23, 24), only few studies have addressed the expression, function, and signaling pathways of LCN2 in the female reproductive tract. This is likely due to the fact that so far little is known about the interaction of LCN2 with its various receptors as previously mentioned (14). In the following section, we summarize the studies on LCN2 expression in the female reproductive tract in chronological order.
Interestingly, the first reports of Lcn2 mRNA (then referred to as 24p3) in the murine female reproductive system were made almost at the same time by two different research groups in 1995 in the USA. Using Northern Blot analysis, Kasik and Rice (24) found high levels of Lcn2 in the glandular uterine epithelium of pregnant mice during gestation and shortly before giving birth. Liu and Nilsen-Hamilton investigated the expression of Lcn2 in a turpentine-induced mouse model of Acute Phase Response (APR) as well via Northern Blot analysis (23). In addition to a massive induction of Lcn2 in the liver during APR, a strongly enhanced expression of Lcn2 in murine uterine tissue under the same conditions was found (23). Interestingly, the Northern Blot analysis demonstrated that Lcn2 was not present in the placenta or fetus (23). Further experiments showed that Lcn2 is also up-regulated during normal pregnancy independently of APR (23). Two years later, the same research group from Iowa State University conducted another study on the expression of LCN2 protein and mRNA in the mice (13). In contrast to increased LCN2 expression in the uterus during APR, Western blot analysis showed no protein expression of LCN2 in the bloodstream or in the amniotic fluid during pregnancy (13). Instead, immunostaining identified the luminal and glandular uterine epithelium as the site of LCN2 protein synthesis (13). Interestingly, the expression levels of Lcn2 in the liver of the mice did not change during pregnancy, as analyzed by Northern blot analysis (13). It was particularly emphasized that the amount of LCN2 in the uterus during pregnancy and at birth was much higher than the increase detected in the liver during APR. This led to the assumption that LCN2 is involved in local inflammatory processes at parturition (13). In this context, a review article also proposed the theory that LCN2 is subject to separate, local regulation within different tissue types (25).
In 1996, Chu and co-workers demonstrated by Northern blot analysis that Lcn2 is strongly expressed in the uterus and vagina of adult mice, but not in ovarian tissue (26). Additionally, Western blot analysis revealed that LCN2 was not only detected in uterine tissue, but has also been reported as a component of uterine luminal fluid, secretions that are produced by uterine epithelial cells during estrus cycles in mature rodents (26). Furthermore, their analysis of glycopeptide linkage revealed evidence for N-linked but not O-linked glycosylation in LCN2. Thus, two potential sites for N-linked glycosylation at Asn81 and Asn85 of LCN2 can be derived from the primary structure (26).
The key finding of Huang and co-workers in 1999 was that uterine protein and mRNA expression of LCN2 (detected via Western and Northern blot analysis and immunohistochemical staining) is strongly dependent on natural hormonal fluctuations during the estrus cycle in mice (27). In line with this, a study by Burns et al. also shows that Lcn2 is regulated by hormonal influences in the murine ovary (28). In situ hybridization demonstrated that there was no detectable expression of Lcn2 in the wild type (WT) murine ovary, but depletion of the follicle-stimulating hormone receptor (FSHR) led to greatly increased Lcn2 levels (28).
In addition to the small number of studies of LCN2 in mice (Table 1), there are also few studies on its expression in human tissue. In a study analyzing 50 tissues of human origin by RNA dot-blot hybridization, the uterine tissue was positive for LCN2 (39). In their comprehensive study, Friedl and co-workers (40) used immunohistochemistry to examine various healthy human tissues for LCN2 protein expression. These results of the study report that LCN2 expression was not found in the female reproductive tract (ovaries, fallopian tube, endometrial glands or stroma, myometrium, uterine cervix). Unfortunately, not all images of the staining results from the screening are displayed in the publication, but the results of the staining have been summarized in tabular form.
According to Human Protein Atlas (https://www.proteinatlas.org/, last accessed on 21.3.2024), high expression of LCN2 mRNA was detected in the vagina and cervix, while protein expression was proven only in the cervix. Low or no LCN2 expression was detected in the ovary, endometrium, fallopian tube, and placenta (Table 2). There are no data on the tissue expression of human LCN2 in the vagina, but it was found via enzyme-linked immunosorbent assay that LCN2 is secreted into the vaginal fluid and participates in vaginal immune response (41).
A more recent study by De La Chesnaye and colleagues showed for the first time ovarian expression of LCN2 in rats (42). RT-qPCR showed that ovarian Lcn2 mRNA increased from date of birth to 30 days (pre-pubertal period) in rats (42). Western blot analysis showed that adult rats (without definite age mentioned) show stronger expression of LCN2 protein in ovaries than testes (42). Furthermore, cellular localization of LCN2 via immunostaining detected positive signal in granulosa cells (of primordial and growing follicles), in the oocyte, in the zona pellucida and in the antrum of developed follicles of adult rat ovaries (42).
The most recent study by our research group showed contrasting results for the expression of LCN2 in the ovary in mice, compared to the study described previously in rats. We found low but detectable mRNA and protein expression of LCN2 in the ovaries of B6N(Cg)-Esr1tm4.2Ksk/J mice through immunohistochemistry, Western blot, and RT-qPCR analysis (31, 43). The study revealed a correlation of increased LCN2 expression and estrogen receptor alpha (ERα, Esr1) deficiency in adult murine ovaries (31). In addition, in adult WT animals, the previously described estrus-dependent expression of LCN2 was confirmed in luminal and glandular uterine epithelium via immunohistochemical stainings (31). We have recently shown that LCN2 is also strongly expressed in parts of the oviduct and in the vagina of adult WT animals (Figure 1). Importantly, a subsequent study showed that the ovaries of Esr1-deficient mice exhibited iron accumulation, increased levels of LCN2, and signs of aging (43).
Figure 1 Schematic representation of LCN2 expression in the main components of the female reproductive system. The right side of the figure displays the primary compartments of the mouse female reproductive tract, including the ovary (O), oviduct (Ov), uterus (U), and vagina (V). The left side of the figure depicts a scheme of immunohistochemical staining of LCN2 in different parts of the female reproductive system based on previous research and our own findings using the anti-LCN2 antibody AF3508 from R&D Systems. Cells shown in shades of pink and purple indicated a lack of LCN2 expression, while cells with brown staining indicate positive LCN2 expression. The ovarian stroma consists of epithelial cells, fibroblast-like cells and smooth muscle cells. Growing ovarian follicles contain various cell types, including oocytes surrounded by granulosa and theca cells. Only cells in the Corpus luteum, which form from cells remaining in the pre-ovulatory follicle, were positive for LCN2. In both the oviduct and uterus, the luminal epithelium displayed positive staining for LCN2, while stromal cells remained unstained. Additionally, the glandular uterine epithelium showed positive LCN2 expression. In vaginal tissue, positive LCN2 staining was observed in several cells of the surface epithelium and stroma (lamina propria).
Limited data suggest that there are differences in LCN2 activities in mice (summarized in Table 1) and humans (summarized in Table 2), which may be related to the aforementioned ability of human LCN2 to form dimers. Furthermore, taking all reports into account, the conclusion can be drawn that LCN2, which is usually viewed as a systemic inflammation indicator, is regulated by specific local mechanisms within reproductive tissue without affecting the rest of the organism. Unfortunately, mechanistic insights into the mechanism of action of LCN2 in the female reproductive tract are still scarce. A study by Lee and co-workers suggested that post-translational modification of LCN2 may play a role in its biological function, as there is a phosphorylation site of protein kinase C at Ser88 in murine uterine protein (29).
We summarized the current knowledge on the expression of murine LCN2 in different compartments of the female reproductive tract in Figure 1 and included findings of our own (unpublished) immunohistochemical LCN2 stainings. Further comprehensive studies are needed to determine the precise cell type expressing LCN2, for instance, in the ovary, oviduct, and vagina and whether they are subject to hormonal fluctuations of the estrus cycle.
Throughout history, the expression of LCN2 in the reproductive system has been studied primarily in the context of pregnancy and reproduction. Studies gave evidence that LCN2 levels fluctuate during murine estrus cycle (27, 31). The murine estrus cycle consists of 4 phases, namely proestrus, estrus, metestrus, and diestrus which repeat every 4 to 5 days unless interrupted by pregnancy, pseudopregnancy, or anestrus (44). As already briefly mentioned, Huang et al. showed that the level of Lcn2 mRNA was high in the proestrus and estrus, then declined dramatically from the metestrus to diestrus in the uterus (27). Consistent with this observation, the LCN2 protein was abundant in the proestrus, decreased from estrus to metestrus, and declined to a very low level in the diestrus. We confirmed this finding and further showed that when hormone balance is disrupted by depletion of Esr1, the corresponding Esr1-deficient mice remain permanently in the diestrus (31). This is accompanied by a constant and massively reduced expression of LCN2 in uterine epithelium, which was observed in immunohistochemical staining (31).
Most existing publications studied LCN2 in the context of gestation, parturition or postpartum (13, 23, 24, 26). Liu and colleagues analyzed tissues stained with anti-LCN2 antiserum from mice on day 19 of pregnancy and 1 day postpartum (13). LCN2 was detectable in the luminal uterine epithelium (day 19). On day 1 after delivery, it was also found in the glandular epithelium. Kasik and Rice speculated that uterine Lcn2 mRNA (around birth) could be due to neutrophils in the uterus or stem from different cell types (such as myometrial cells) (24).
Following birth, the uterus undergoes a process called involution, whose goal is to return the uterus to its prepregnancy state. It has been observed that the postpartum involuting uterus is a major site of LCN2 expression (25). The authors speculate that in this context, LCN2 serves as an inducer of neutrophilic apoptosis, thus protecting the uterus from oxidative stress and carcinogenesis during the remodeling phase.
Others studied LCN2 in uterine tissue of rats (45) in context of pregnancy and involution. Western blot analysis show that LCN2 was strongly elevated on day 22 of pregnancy and on the first two days after delivery compared to non-pregnant uterine tissue. In pregnant and non-pregnant rats (in the proestrus), uterine LCN2 expression was restricted to the uterine luminal and glandular epithelium of the uterine and did not colocalize with Myeloperoxidase (detected via immunostaining), which is also up-regulated during postpartum involution but in different cellular compartments (in the vessel-rich layer and in the endometrial stroma).
The uterine tissue is also involution, as is the mammary gland. Northern blot analysis demonstrate that Lcn2 expression was barely detectable in the mammary gland of virgin, pregnant or lactating mice (46). In contrast, the involution process that induced strong tissue remodeling of the mouse mammary gland started with weaning and was accompanied by strong expression of Lcn2 mRNA and protein (46, 47). In situ hybridization demonstrated that Lcn2 expression was restricted to epithelial cells of mammary gland alveoli (46). Mechanistically, Bong and colleagues found that overexpression of Lcn2 drives mammary epithelial cells to apoptosis. Interestingly Western blot analysis show that the LCN2 protein is secreted from epithelium into the milk of lactating female mice, indicating an important function for the pups as well (47). Not less important, it was also speculate that Lcn2 and its receptor (Slc22a17 alias 24p3R) are involved in apoptotic signaling in the perinatal ovary, where numerous ovaries are lost through apoptosis (42).
LCN2 has been reported to play a role during pregnancy not only in rodents, but also in mares, where in situ hybridization showed that it was localized in the equine uterine glandular epithelium (48). Immunohistochemical stainings demonstrate that LCN2 protein is expressed in gravid endometrium and secreted into the luminal fluid (48). Therefore, it was speculated that LCN2 could function as a carrier for small substances from the mother to the fetus (48).
Several reports implicate LCN2 in the fertilization process (30, 49). A study by Watanabe and colleagues identified LCN2 produced by the female mouse reproductive tract as a sperm-capacitating agent that alters the membrane properties of sperm in preparation for fertilization (30). More specifically, LCN2 levels reach their peak during estrus at uterotubular junction, where sperm maturation is believed to begin, forcing the sperm to undergo lipid raft reorganization, cholesterol efflux, and glycosylphosphatidylinositol-anchored protein shedding necessary for fertilization. Furthermore, the same study reported that LCN2 has the ability to increase murine in vitro fertilization efficiency, making it a great target to study for clinical application.
On the other hand, other studies reported a negative correlation between LCN2 and fertilization (50, 51). More precisely, with the use of computer-assisted sperm analysis method, cytochemical staining and detection of the protein tyrosine phosphorylation pattern Lee and co-workers reported that the LCN2 protein play a role in stimulating flagellar motility but preventing acrosome reaction. The same group confirmed previous indications of the inhibitory effect of LCN2 on in vitro fertilization (51). To be precise, the presence of LCN2 reduced the response of sperm to bovine serum albumin (BSA) and calcium by suppressing intracellular pH elevation, calcium uptake, cAMP accumulation, and protein tyrosine phosphorylation of BSA/calcium-stimulated sperm.
The most important report on the implication of LCN2 in the fertilization process is probably the one that says that Lcn2KO mice show compromised fertility (52). The study reports that although Lcn2 deficient males appear to be fertile, Lcn2-deficient females show a significantly (p < 0.05) decreased pregnancy rate compared to WT littermates when crossed to WT or Lcn2-deficient males. Taken together, these data indicate that sperm maturation and fertilization are highly dependent on the LCN2 status of the female individual.
The male reproductive system consists of external genitals such as penis, scrotum, and testes and the internal organs, including the vas deferens, urethra, and prostate gland. The interaction of the individual parts is controlled by the hypothalamic-pituitary-gonadal hormone axis and is essential for male fertility. Only a brief overview is given here, since the various processes are very complex and precisely regulated and are explained elsewhere (53, 54). The male reproductive organs have various functions including production of testosterone, spermatogenesis, sperm maturation and –storage, and the release of the sperm. The testes have both an endocrine function (e.g., testosterone production) and an exocrine function (spermatogenesis). Sperm maturation and storage occur in the epididymis. The sperms are transported via the Vas deferens to the prostate and urethra. The prostate gland produces the fluid secretions that support the sperms. Both sperm and urine from the bladder are excreted through the urethra to the outside via the penis. It is important to keep in mind that there are various differences in the male reproductive tract between species, such as gross anatomy and histology. Compared to humans, for example, rodents have a multilobed prostate and additional glands (54).
According to Human Protein Atlas (https://www.proteinatlas.org/, last accessed on 21.3.2024), Lcn2 mRNA expression was not detected in the testes, epididymis, and seminal vesicles, while only low expression is reported in healthy prostate. The data correspond to the study by Friedl and co-workers who analyzed the expression of the LCN2 in the human prostate gland and found strong expression in glandular foci and a positive signal in epithelial cells (40). Furthermore, RNA dot-blot hybridization with a probe containing the LCN2 cDNA sequence showed specific expression in human prostate tissue (39). To date, no further data are available on the physiological expression and function of LCN2 in the male human reproductive tract.
In contrast to the limited data available on human reproductive tissues, there are some studies on rodent models that investigated LCN2 in different parts of the male reproductive system. Chronologically, Chu and colleagues in 1996, were the first to mention LCN2 in the male reproductive tract (26). Northern blot analysis of various tissues of adult mice revealed a strong expression of Lcn2 in the epididymis, but not in the testes, prostate, seminal vesicles, or coagulating glands (26). Four years later, the group confirmed their findings by immunolocalization and Northern blot analysis (37). LCN2 was expressed in epithelial cells and the lumen of mouse epididymis (37). Both the mRNA and the LCN2 protein were already detectable in 2-week-old mice and remained at a constant level during the observed period (up to 12-weeks). Interestingly, a decreasing gradient of LCN2 expression was detected from the caput to the caudal region of the epididymis, as well as its association with sperm. The finding was confirmed by another research group, who detected the presence of LCN2 in the caput region of the epididymis via immunolocalization (38). In another study, it was proposed that LCN2 is responsible for internalization of the protein–ligand complex by spermatozoid cells (55). Most notably, LCN2 has been reported to serve as a physiological mechanism for the delivery of ferric ion to epididymal spermatozoa (55). Taking into account the findings, it was concluded that LCN2 is an important component in spermatozoa processing.
In the initial studies mentioned above on LCN2 in the male reproductive tract, no expression was detected in the testes, but the studies of the following years showed contrasting results. To better classify the ambiguous results, the histological structure and function of the testes are briefly described. Histologically, testes consist of tubular glands covered by the tunica albuginea (54). They comprise testicular tubules (seminiferous tubules), including Sertoli cells and germ cells (spermatogonia, spermatocytes, spermatids) and the interstitial compartment with the endocrine Leydig cells (54). Sertoli cells function as the main cells required for maturation and differentiation of germ cells (54).
Tanaka and colleagues found contrasting results regarding Lcn2 expression in murine testes. Northern bot analysis showed no expression in wild type mice from 19.5-dpc up to 8 weeks (32). In the same study, Lcn2 expression was determined in germ cells and somatic cells via RT-PCR after fluorescence-activated cell sorting of testicular gonadal cells from wild type (13.5-dpc and 3-week-old) mice (32). It was assumed that the somatic cells are mainly Sertoli cells, but no Leydig cell markers, which also belong to the somatic cells, were examined (32). A subsequent study by the same group showed, that primary Sertoli cells, obtained from the testes of 7-day-old mice through enzymatic digestion followed by magnetic activated cell sorting, exhibited undetectable levels of Lcn2 (33). Additionally, they reported that its expression in juvenile primary murine Sertoli cells is highly dependent on germ cells, more specifically spermatogonial cells (33). The freshly isolated primary Sertoli cells were positive for Lcn2, but loose expression levels when cultured for 1 week alone but not in coculture with primary spermatogonial cells. Interestingly, in vitro treatment of the Sertoli cells with spermatogonial cell-conditioned medium caused nuclear factor-B (NF-κB) pathway mediated transcription of the Lcn2 gene (33), providing first insights into the signaling mechanisms responsible for LCN2 expression in the testes. Moreover, it was shown that Lcn2 is regulated in a different manner in testicular cells compared to inflammatory immune cells, as commonly known inducers (Interleukin-1β and lipopolysaccharide) fail to induce Lcn2 in an immortalized Sertoli-B cell line (33). Furthermore, transcriptional regulation of the Lcn2 gene in juvenile Sertoli cells was independent of IκBζ (33).
In 2009, it was speculated that artificially generated electromagnetic fields (EMF) affect sperm motility and could affect LCN2 expression in mice (34). This study provides further insights into the expression of LCN2 on mRNA and protein level in healthy testes. Total testicular mRNA, isolated from untreated adult 8-week-old mice, showed strong Lcn2 expression demonstrated by RT-PCR (34). When mice were exposed to a 3 mT (50 Hz) magnetic field (for 6 days a week and 4 hours a day) for 8 weeks, Lcn2 mRNA expression and protein levels decreased significantly (34). Mechanistically, it was discussed that LCN2 is involved in EMF-induced apoptosis in germ cells.
Years later, a research group analyzed the pattern of Lcn2 expression with increasing age in mice and provided the first evidence for Lcn2 in murine Leydig cells (35). In situ hybridization demonstrated that Lcn2 levels show an age-dependent increase in testes from day 1 to 8 months in Leydig cells, then reduce by the twelfth month, portraying Lcn2 as a developmentally regulated gene (35). However, while that study detected LCN2 protein expression in Leydig cell, it reported an absence of LCN2 in Sertoli and germ cells (35).
A study by De La Chesnaye and coworkers in 2018 studied LCN2 for the first time in adult rat testes and reported positive expression solely in germ cells (42). In addition, the mRNA expression profile and cellular location of LCN2 were analyzed in gonads collected from fetal rats at 21 days post-coitum, as well as neonatal rats at 0, 2, 4, 6, 12, 20 and 30 postnatal days (42). Interestingly, expression in testes collected at all stages was lower than in ovaries of female rats. In this study, the expression of the LCN2 receptor SLC22A17 was shown in germ cells at different developmental stages (42). On the contrary, a consecutive study done by the same group in 2020, reporting detectable LCN2 expression in Sertoli and Leydig cells (56). Although both publications contain manufacturer information on the antibody used (which was the same), only one of the studies provides information on the catalog number, therefore, it cannot be completely ruled out that different antibodies were used. Unfortunately, this discrepancy is not addressed in the study, so it is not possible to definitively say where exactly LCN2 is expressed in the rat testes.
Recently, studies observed high expression of Lcn2 in adult Leydig cells via in situ hybridization (36). This is in line with the most recent publication by our group studying LCN2 in reproductive tissues. There, moderate expression of Lcn2 mRNA and protein expression was found in the testes of adult mice (31). In addition, immunolocalization confirmed positive signals in interstitial Leydig cells (31). Furthermore, this study found Esr1-dependent expression of LCN2, since it was greatly increased in Esr1-deficient testes (31).
In summary, it can be concluded that the expression and function of LCN2 in the male reproductive tract are not yet fully understood. Although research suggests that LCN2 plays a vital role in sperm maturation, to understand the exact mechanisms by which LCN2 exerts its functions, a detailed screening of LCN2 expression in different compartments with a well-established antibody (such as anti-LCN2 AF3508 from R&D Systems) would be essential. Therefore, we have schematically summarized the immunohistochemical localization of LCN2 expression in the male reproductive tissues (of mouse testis and epididymis) in Figure 2 that we have found in unpublished work. It is important to understand which cells express LCN2 and which putative receptors are essential. In this way, the molecular mechanisms of its action can be finally uncovered.
Figure 2 Schematic representation of LCN2 expression in the main components of the male reproductive system. The right side of the figure shows the main components involved in sperm maturation and development in the murine male reproductive tract, namely the testis (T) and epididymis (E) which can be histologically divided into the caput (Cap), corpus, and cauda (Cau) regions. The left side of the figure is a schematic representation of immunohistochemical staining of LCN2 in the different compartments using the anti-LCN2 antibody AF3508 from R&D Systems. Cells shown in shades of pink and purple represent cells negative for LCN2, while cells with brown staining are positive for LCN2. Spermatogenesis begins gradually in the germ cells located in the seminiferous tubules of the testis. Positive staining for LCN2 was only observed in interstitial located, hormone producing Leydig cells. The spermatozoa (sperm), produced in the testis, are transported for maturation and storage to the epididymis. In the caput epididymis, a high expression of LCN2 is visible in the lumen where sperm maturation occurs. A decline in LCN2 expression can be observed when comparing sperm in the lumen of the cauda to the caput epididymis segment.
The presented article summarizes that there are only limited, partially ambiguous studies on the physiological expression and function of LCN2 in the reproductive tract. However, there are several studies that focus on LCN2 in different diseases and pathologies of both male and female reproductive tracts. In the following sections, LCN2 is examined in the context of various diseases.
Steroid hormones, especially estrogens, are essential players in a variety of biological processes in the female reproductive tissue. They enable folliculogenesis, successful ovulation, and mediate proliferation and differentiation of uterine tissue (57). Studies show that the LCN2 promoter contains an estrogen-responsive element and its expression can be controlled by estrogens (17). If hormone levels are not in balance, hormone deficiency conditions can occur, for example, hypogonadism or during menopause. Since LCN2 is influenced by natural hormonal fluctuations of the estrous cycle (27), it is likely that its expression is affected under such conditions. In a study by Liu and co-workers, the dependence of LCN2 on hormones was investigated using an ovariectomized mouse model, which is a valuable tool for understanding estrogen deficiency (16). In situ hybridization and Western blot analysis gave evidence that estrogen is the main hormone for inducing LCN2 levels in uterine tissue (16). On the other hand, deletion of Esr1 in mice, one of the receptors through which estrogens can mediate signals, does not induce LCN2 in uterine epithelium (16). This is in line with the findings of our recent study using Esr1-deficient mice (31). Since the mice in the studies by Liu et al. had their ovaries removed due to the experimental model (16), the authors were unable to make any statement about the expression of LCN2 in the ovary. Kessel et al. showed that the depletion of Esr1 in female mice leads to a massive increase in LCN2 expression in the ovary (31).
Obesity affects not only LCN2 expression in adipocytes (58), but also in the reproductive tract. A study using rats showed that ovarian LCN2 strongly decreased in pups from obese mothers compared with pups of mothers fed control diet (56). This scenario was as well observed in rat testicles of pups from obese mothers compared with control animals (56). However, the molecular mechanisms of Lcn2 regulation are poorly understood, but there is a general assumption that SLC22A17 and megalin play essential roles (56). Further studies should aim to understand the molecular mechanisms, which in turn will also allow conclusions to be drawn about the physiological function of LCN2.
Hormonal imbalances, including a low level of estrogen and high levels of testosterone, are common in patient with polycystic ovarian syndrome (PCOS) (59). LCN2 is likely involved in PCOS as obesity is linked to this condition (59) and LCN2 is known to be strongly expressed by adipocytes (58). However, there are limited studies on the subject, and existing data is inconclusive. As previously reported, human LCN2 has the ability to form complexes with MMP9 and regulate its function (3). A study conducted on 80 subjects (40 healthy and 40 patients with PCOS) reported lower serum levels of LCN2 as well as LCN2/MMP9 complex in PCOS patients (60). Another study found no difference in serum concentration and activity of LCN2/MMP9 complex between the diseased and healthy groups (61). Sahin and co-workers also found no difference in serum LCN2 levels between patients with PCOS and control patients (62). On the contrary, Cakal and colleagues reported increased serum levels of LCN2 in woman with PCOS (30 patients) compared to body mass index-matched controls (30 woman) (63). There are two studies that report that LCN2 is not affected by PCOS, but that obesity in patients with PCOS is associated with alterations of serum LCN2 levels (64, 65). Loss of weight significantly decreases LCN2 levels in obese/overweight PCOS patients (64, 65). Possible hormonal fluctuations in patients of these studies are a possible reason for the different results, suggesting the notion that hormonal differences might affect LCN2 or activity of the LCN2/MMP9 complex (61). More comprehensive studies on urine, blood, and tissue are needed to understand the role of LCN2 in PCOS.
Shortly after the discovery of LCN2, it became obvious that it plays a role in fertilization, implantation, and pregnancy in mice (13, 23, 24, 26).
However, LCN2 has also been implicated in pregnancy complications in humans. Meta-analysis comparing women with healthy pregnancy and preeclampsia (PE) detected that high circulating LCN2 is associated with PE, which may be independent of the trimesters for blood sampling and the severity of PE (66). In line, Stepan and colleagues found that maternal LCN2 serum levels increased significantly in PE patients (67).
Based on these findings, it is likely that elevated circulating LCN2 expression is associated with increased vascular and metabolic risk in patients with PE. Furthermore, meta-analysis of data comparing gestational diabetes mellitus (GDM) patients to parturient with normal glucose tolerance identified higher blood LCN2 in GDM patients (68). Proteomics analysis of amniotic fluid obtained from patients diagnosed with a sonographic short cervix (< 25 mm) revealed a proteomic signature of increased risk of imminent delivery containing increased LCN2 protein (69).
During early pregnancy, stimulation of uterine tissue with lipopolysaccharide or injection of Escherichia coli leads to a strong induction of LCN2 (16). In addition, LCN2 is secreted in the vaginal fluid and is involved in the vaginal immune response (41). There is a positive correlation between the increase in leukocyte infiltration and the level of LCN2 in the vaginal fluid of woman suffering from vaginal inflammation (70). Interestingly, LCN2 expression was found to be increased during vulvovaginal candidiasis infection, but decreased in bacterial vaginosis, two common vaginal disorders in reproductively aged women (41). The differences may be related to different microbiota composition in the different disorders and the authors speculate that low levels of LCN2 might be favorable for bacterial processes that use siderophores to obtain iron for proliferation (41). Another study in connection with group B Staphylococcus (GBS) infections during pregnancy assumes that LCN2 prevents iron uptake by GBS and thus restrict their growth (71). The immune defense activity of LCN2 in the vagina appears to be controlled by hormones, as treatment with topical estrogen concentrations led to an increase in LCN2 in vaginal douche samples of patients and in immortalized vaginal epithelial cell lines (72). All these findings shed light on the antibacterial activity of LCN2 in the female reproductive tract, which has previously been found in non-reproductive tissues (52).
When endometrial-like tissue develops outside the uterine cavity, it is often associated with pelvic pain and infertility (73). This condition is known as endometriosis and often affects women in reproductive age (73). Nevertheless, the exact cause of this condition is not fully understood, but is associated with pro-inflammatory processes (73). Consistently, there is a significantly higher expression of serum LCN2 in patients with endometriosis compared to the control group (73). This increase was accompanied by increased quantities of the tumor marker cancer antigen 125 and the inflammatory C-reactive protein (74). In endometriosis patients, there was not only a systemic increase in LCN2, but also locally in endometrial cysts, as demonstrated by immunohistochemical staining (74). However, others did not find significant differences in serum LCN2 levels in endometriosis patients compared to the control group or between different stages of the disease, but the MMP9/LCN2 complex ratio was higher (75). Since endometriosis patients have an almost three times higher incidence of developing cancer, it is also likely that LCN2 is involved in the transition (76). Depending on their findings, Yamada and co-workers speculated that LCN2 is involved in the progression of ovarian carcinoma resulting from endometriosis (77).
Furthermore, a behavioral study showed that induced endometriosis in mice led to increased depression and is also reflected in the induction of the expression of various targets in the brain, including the induction of the LCN2 protein and mRNA in the amygdala in females with endometriosis (78). A study in mice that dealt with the molecular interactions that LCN2 plays in endometriosis found that LCN2 expression induces epithelial-to-mesenchymal transition (EMT) in stressed primary uterine endometrial cells promoting onset of endometriosis (79). More studies are essential to clarify how LCN2 is related to the initiation and progression of endometriosis. It would also be informative to investigate whether and how LCN2 fluctuates during the menstrual cycle, which has not been considered in existing studies and could possibly explain the differences seen.
Gynecological cancers can originate from various sites in the normal tissues of the female reproductive tract (80). LCN2 expression has been shown to be up-regulated in ovarian, cervical tissue, and endometrial cancer, as well as human ovarian cancer cell lines [https://www.proteinatlas.org/, last accessed on 21.3.2024].
Hao and co-workers found an up-regulated expression of LCN2 in ovarian cancer patients, as well as in different ovarian cancer cell lines compared to normal tissue and cell lines (81). Using human ovarian cancer cell lines, the study provided evidence on the mechanism of LCN2 signaling ovarian cancer (81). LCN2 promotes tumor progression in ovarian cancer cells by activating the ERK/GSK3β/β-catenin signaling pathway (81).
Interestingly, LCN2 could be a suitable non-invasive biomarker for ovarian cancer as it is elevated not only in tissue, but also in serum and urine of patients (82–84). The expression of immunoreactive NGAL (irNGAL) in ovarian tumors changes with disease grade and this change is reflected in the concentration of NGAL/LCN2 in peripheral blood, which allows LCN2 to be used as a biomarker of tumor progression (83). Additionally, LCN2 has been detected among the most highly up-regulated genes in ovarian serous papillary carcinomas (85). Furthermore, LCN2 has been reported to increase intracellular iron levels in ovarian cancer cells but decrease oxidative stress, suggesting antioxidant capacity and allowing cancer cells to survive in stressful endometriotic cysts (77). Taken together, the data clearly demonstrate a positive correlation of LCN2 with tumor progression and targeting LCN2 or its signaling pathways could be a therapeutic strategy for targeting ovarian cancer.
LCN2 mRNA and protein expression were strong in human patients with endometrial hyperplasia and, suggesting that it is involved in the tumorigenic process leading to endometrial carcinoma (86). Furthermore, there is a gradual increase in LCN2 in patients with endometrial hyperplasia and carcinoma (87). Consistent with this, LCN2 was found to be a component of the key signature in the onset of endometrial cancer in the glandular uterine epithelium, as its expression increased in precancerous atypical endometrial hyperplasia (88). A study with 85 high-grade endometrial cancer tissue samples showed strong LCN2 expression using immunohistochemical detection (88). Multivariate analysis revealed a positive correlation between LCN2 expression and shorter overall survival of these patients (89). Others found that increased LCN2 tissue expression is associated with aggressive characteristics and a poor prognosis in women with endometrial cancer (90). In line, immunohistochemical localization of LCN2 (as well as its receptor SLC22A17) was indicated LCN2 to be a prognostic factor in endometrial cancer (91). In endometrial cancer, there is not only a strong expression of LCN2 in the tissue, but also in the serum of the patients. In a study that included 123 women, out of whom 52 had endometrial cancer, significantly lower median serum levels of LCN2 were found in a group of patients with normal endometrium compared to the endometrial cancer group (92).
In vitro experiments confirmed the role of LCN2 in enhancing endometrial carcinoma cell survival and migration by mediating cytokine production (93). In addition, Lin and colleagues used the human endometrial carcinoma cell line RL95-2 to gain new insights into the LCN2 signaling mechanism and found that it is induced in a time-dependent manner by glucocorticoid stimulation (dexamethasone) and under starvation conditions (94). Furthermore, forced expression of LCN2 led to increased proliferation and invasion in the human endometrial cancer cell lines Ishikawa and HEC1B (87). A study by Miyamoto and co-workers provided information on signaling pathways of LCN2 in endometrial cancer (95). Their results showed that LCN2 promotes the survival of endometrial cancer cell lines via the PI3K pathway in a stressful environment and in an iron-dependent manner (95). Furthermore, others found that stimulation with LCN2 protein purified from mouse uterine fluid induced apoptosis in the RL95-2 endometrial cancer cell line (94). However, estrogen could not induce Lcn2 expression in these cells (94). LCN2 expression is speculated to be involved in balance between cell death and proliferation in uterine tissue remodeling and is important in the progression of endometrial cancer.
Persistent infection with the human papilloma virus is a risk factor for the development of cervical cancer, including cervical adenomas and cervical squamous carcinomas (96). By analyzing 90 clinical specimens, it was found that elevated LCN2 tissue expression in cervical cancer is correlated with tumor metastasis and cancer progression (97). In addition, serum levels of LCN2 were also increased significantly patient with cervical cancer, suggesting it as a potential non-invasive biomarker for the diagnosis and prognosis of cervical cancer (97, 98). In vitro and in vivo studies in a xenograft model confirmed tumor-promoting role and provided evidence that LCN2 mediates cervical cancer through the EMT signaling pathway (97).
All mayor findings on LCN2 expression and role in female reproductive tract cancers have been summarized in a tabular form in Table 3.
LCN2 is a known acute phase protein in non-reproductive tissues (52). Interestingly, a study examined Lcn2 in the context of uropathogenic infections (99). In murine cauda epididymis, Lcn2 mRNA was significantly increased after infection with uropathogenic E. coli, highlighting the antibacterial activity of LCN2 in reproductive tissues as seen in female uterine tissue (16, 99).
Male infertility can be caused by diseases of the testicles themselves or impaired testicular function, including defects in spermatogenesis, Leydig cell degeneration or poor sperm quality (100). One study from 2017, detected a strongly increased expression of Lcn2 expression in the testes in mice as a consequence of induced infertility by busulfan treatment or bilateral cryptorchidism (35). Although the study did not examine molecular signaling mechanisms, the authors speculated that LCN2 is involved in germ cell apoptosis. Tanaka and colleagues conducted a study on the involvement of spermatogonia in testicular gene regulation. They discovered that germ cell-deficient mice completely lose the expression of certain genes, including Lcn2 (32). Furthermore, they examined Lcn2 expression in a juvenile spermatogonial depleted (jsd/jsd) mouse mutant, which has severe defects in spermatogonial differentiation (seminiferous tubules containing only type A spermatogonial germ cells and Sertoli cells), and in an artificial cryptorchid model (32). Interestingly, both models exhibited high expression of Lcn2 in the testis, suggesting a potential relationship between Lcn2 and germ cell apoptosis (32).
Kessel et al. analyzed LCN2 expression in testes from adult infertile Esr1-deficient mice (31). Interestingly, LCN2 protein expression was strongly increased in these animals compared with age-matched WT animals (31). These studies provide evidence that LCN2 has not only a physiological but also a pathophysiological role in the testes. However, it is mandatory to understand the underlying molecular mechanism, which likely involves estrogen receptor signaling.
Apart from its role in benign prostate diseases and the development and progression of prostate cancer (10, 101), LCN2 has hardly been studied at all in diseases of the male reproductive tract. In 2005, a study analyzed adult male germ cell tumors and found a high increase in LCN2 in teratomas without seminomas but not in other subtypes, classifying it as an suitable predictor (102).
For a more comprehensive understanding, it is also important to investigate the expression and localization of LCN2 in healthy human testes and in various diseases such as testicular cancer.
The presence of LCN2 has been confirmed in both male and female healthy reproductive systems by multiple studies. However, the available studies show that LCN2 has so far been studied much more intensively in a pathological context than in a physiological context. Nevertheless, we can speculate about the different functions of LCN2 in the female and male reproductive tract. Although it appears that in the female reproductive system LCN2 is involved in tissue reorganization during the menstrual cycle and pregnancy, the mechanism of its action and the cells responsible for its production have not yet been determined. However, it has been shown to serve as a component of the maturation and motility component of sperm in male reproductive organs. Furthermore, several studies report the deregulation of LCN2 in numerous reproductive tissue pathologies, including fertility defects. Considering that the literature overview provides clear evidence that LCN2 is a key component in fertility, and that no information on the actual mechanisms of its action is currently available, further research on this topic would be beneficial to understand its precise function in the reproductive system, as well as its therapeutic potential.
MK: Conceptualization, Data curation, Writing – original draft, Writing – review & editing. PBMS: Data curation, Visualization, Writing – review & editing. RW: Data curation, Funding acquisition, Supervision, Writing – review & editing. SKS: Conceptualization, Data curation, Supervision, Writing – original draft, Writing – review & editing.
The author(s) declare financial support was received for the research, authorship, and/or publication of this article. RW is supported by the German Research Foundation (grants WE2554/13-1, WE2554/15-1, and WE 2554/17-1), the Deutsche Krebshilfe (grant 70115581), and the Interdisciplinary Centre for Clinical Research within the Faculty of Medicine at the RWTH Aachen University (grant PTD 1-5). The funders had no role in the design of this article or in the decision to publish it.
The authors declare that the research was conducted in the absence of any commercial or financial relationships that could be construed as a potential conflict of interest.
The author(s) declared that they were an editorial board member of Frontiers, at the time of submission. This had no impact on the peer review process and the final decision.
All claims expressed in this article are solely those of the authors and do not necessarily represent those of their affiliated organizations, or those of the publisher, the editors and the reviewers. Any product that may be evaluated in this article, or claim that may be made by its manufacturer, is not guaranteed or endorsed by the publisher.
APR, acute phase response; BSA, bovine serum albumin; E. coli, Escherichia coli; EMF, electromagnetic field; EMT, epithelial-to-mesenchymal transition; Esr1, ERα, estrogen receptor alpha; FSHR, follicle-stimulating hormone receptor; GBS, group b Staphylococcus; GDM, gestational diabetes mellitus; irNGAL, immunoreactive NGAL; LCN2, lipocalin 2; MC4R, melanocortin-4 receptor; MMP9, matrix metalloproteinase 9; MSFI, migration-stimulating factor inhibitor; NF-κB, nuclear factor kappa B; NGAL, neutrophil gelatinase-associated protein; PCOS, polycystic ovarian syndrome; PE, preeclampsia; RT-PCR, conventional reverse transcription polymerase chain reaction; RT-qPCR, real-time quantitative polymerase chain reaction; SLC22A17, soluble carrier family 22 member 17; WT, wild type.
1. Flower DR. The lipocalin protein family: structure and function. Biochem J. (1996) 318:1–14. doi: 10.1042/bj3180001
2. Kjeldsen L, Johnsen AH, Sengeløv H, Borregaard N. Isolation and primary structure of NGAL, a novel protein associated with human neutrophil gelatinase. J Biol Chem. (1993) 268:10425–32. doi: 10.1016/S0021-9258(18)82217-7
3. Yan L, Borregaard N, Kjeldsen L, Moses MA. The high molecular weight urinary matrix metalloproteinase (MMP) activity is a complex of gelatinase B/MMP-9 and neutrophil gelatinase-associated lipocalin (NGAL). Modulation of MMP-9 activity by NGAL. J Biol Chem. (2001) 276:37258–65. doi: 10.1074/jbc.M106089200
4. Cramer EP, Glenthøj A, Häger M, Juncker-Jensen A, Engelholm LH, Santoni-Rugiu E, et al. No effect of NGAL/lipocalin-2 on aggressiveness of cancer in the MMTV-PyMT/FVB/N mouse model for breast cancer. PloS One. (2012) 7:e39646. doi: 10.1371/journal.pone.0039646
5. Holmes MA, Paulsene W, Jide X, Ratledge C, Strong RK. Siderocalin (Lcn 2) also binds carboxymycobactins, potentially defending against mycobacterial infections through iron sequestration. Structure. (2005) 13:29–41. doi: 10.1016/j.str.2004.10.009
6. Goetz DH, Holmes MA, Borregaard N, Bluhm ME, Raymond KN, Strong RK. The neutrophil lipocalin NGAL is a bacteriostatic agent that interferes with siderophore-mediated iron acquisition. Mol Cell. (2002) 10:1033–43. doi: 10.1016/s1097-2765(02)00708-6
7. Flower DR, North AC, Attwood TK. Mouse oncogene protein 24p3 is a member of the lipocalin protein family. Biochem Biophys Res Commun. (1991) 180:69–74. doi: 10.1016/s0006-291x(05)81256-2
8. Schor SL, Grey AM, Ellis I, Schor AM, Coles B, Murphy R. Migration stimulating factor (MSF): its structure, mode of action and possible function in health and disease. Symp Soc Exp Biol. (1993) 47:235–51.
9. Yang J, Goetz D, Li JY, Wang W, Mori K, Setlik D, et al. An iron delivery pathway mediated by a lipocalin. Mol Cell. (2002) 10:1045–56. doi: 10.1016/s1097-2765(02)00710-4
10. Schröder SK, Asimakopoulou A, Weiskirchen R. Lipocalin 2 as a potential diagnostic and/or prognostic biomarker in prostate, lung and liver cancer. Clinics Oncol. (2018) 1:1–14.
11. Chakraborty S, Kaur S, Guha S, Batra SK. The multifaceted roles of neutrophil gelatinase associated lipocalin (NGAL) in inflammation and cancer. Biochim Biophys Acta. (2012) 1826:129–69. doi: 10.1016/j.bbcan.2012.03.008
12. Santiago-Sánchez GS, Pita-Grisanti V, Quiñones-Díaz B, Gumpper K, Cruz-Monserrate Z, Vivas-Mejía PE. Biological functions and therapeutic potential of lipocalin 2 in cancer. Int J Mol Sci. (2020) 21:4365. doi: 10.3390/ijms21124365
13. Liu Q, Ryon J, Nilsen-Hamilton M. Uterocalin: a mouse acute phase protein expressed in the uterus around birth. Mol Reprod Dev. (1997) 46:507–14. doi: 10.1002/(SICI)1098-2795(199704)46:4<507::AID-MRD9>3.0.CO;2-S
14. Schröder SK, Gasterich N, Weiskirchen S, Weiskirchen R. Lipocalin 2 receptors: facts, fictions, and myths. Front Immunol. (2023) 14:1229885. doi: 10.3389/fimmu.2023.1229885
15. Guo H, Zhang Y, Brockman DA, Hahn W, Bernlohr DA, Chen X. Lipocalin 2 deficiency alters estradiol production and estrogen receptor signaling in female mice. Endocrinology. (2012) 153:1183–93. doi: 10.1210/en.2011-1642
16. Liu YF, Li MY, Yan YP, Wei W, Li B, Pan HY, et al. ERα-dependent stimulation of LCN2 in uterine epithelium during mouse early pregnancy. Reproduction. (2020) 159:493–501. doi: 10.1530/REP-19-0616
17. Seth P, Porter D, Lahti-Domenici J, Geng Y, Richardson A, Polyak K. Cellular and molecular targets of estrogen in normal human breast tissue. Cancer Res. (2002) 62:4540–4.
18. Chumduri C, Turco MY. Organoids of the female reproductive tract. J Mol Med (Berl). (2021) 99:531–53. doi: 10.1007/s00109-020-02028-0
19. Georgadaki K, Khoury N, Spandidos DA, Zoumpourlis V. The molecular basis of fertilization (Review). Int J Mol Med. (2016) 38:979–86. doi: 10.3892/ijmm.2016.2723
20. Agostinis C, Mangogna A, Bossi F, Ricci G, Kishore U, Bulla R. Uterine immunity and microbiota: A shifting paradigm. Front Immunol. (2019) 10:2387. doi: 10.3389/fimmu.2019.02387
21. Ratajczak CK, Fay JC, Muglia LJ. Preventing preterm birth: the past limitations and new potential of animal models. Dis Mod Mech. (2010) 3:407–14. doi: 10.1242/dmm.001701
22. Malik M, Roh M, England SK. Uterine contractions in rodent models and humans. Acta Physiol (Oxf). (2021) 231:e13607. doi: 10.1111/apha.13607
23. Liu Q, Nilsen-Hamilton M. Identification of a new acute phase protein. J Biol Chem. (1995) 270:22565–70. doi: 10.1074/jbc.270.38.22565
24. Kasik JW, Rice EJ. An increase in expression of the lipocalin 24p3 is found in mouse uterus coincident with birth. Am J Obstet Gynecol. (1995) 173:613–7. doi: 10.1016/0002-9378(95)90291-0
25. Nilsen-Hamilton M, Liu Q, Ryon J, Bendickson L, Lepont P, Chang Q. Tissue involution and the acute phase response. Ann N Y Acad Sci. (2003) 995:94–108. doi: 10.1111/j.1749-6632.2003.tb03213.x
26. Chu ST, Huang HL, Chen JM, Chen YH. Demonstration of a glycoprotein derived from the 24p3 gene in mouse uterine luminal fluid. Biochem J. (1996) 316:545–50. doi: 10.1042/bj3160545
27. Huang HL, Chu ST, Chen YH. Ovarian steroids regulate 24p3 expression in mouse uterus during the natural estrous cycle and the preimplantation period. J Endocrinol. (1999) 162:11–9. doi: 10.1677/joe.0.1620011
28. Burns KH, Owens GE, Ogbonna SC, Nilson JH, Matzuk MM. Expression profiling analyses of gonadotropin responses and tumor development in the absence of inhibins. Endocrinology. (2003) 144:4492–507. doi: 10.1210/en.2003-0476
29. Lee YC, Lin SD, Yu HM, Chen ST, Chu ST. Phosphorylation of the 24p3 protein secreted from mouse uterus in vitro and in vivo. J Protein Chem. (2001) 20:563–9. doi: 10.1023/a:1013321213822
30. Watanabe H, Takeo T, Tojo H, Sakoh K, Berger T, Nakagata N, et al. Lipocalin 2 binds to membrane phosphatidylethanolamine to induce lipid raft movement in a PKA-dependent manner and modulates sperm maturation. Development. (2014) 141:2157–64. doi: 10.1242/dev.105148
31. Kessel JC, Weiskirchen R, Schröder SK. Expression analysis of lipocalin 2 (LCN2) in reproductive and non-reproductive tissues of Esr1-deficient mice. Int J Mol Sci. (2023) 24:9280. doi: 10.3390/ijms24119280
32. Tanaka K, Tamura H, Tanaka H, Katoh M, Futamata Y, Seki N, et al. Spermatogonia-dependent expression of testicular genes in mice. Dev Biol. (2002) 246:466–79. doi: 10.1006/dbio.2002.0671
33. Fujino RS, Tanaka K, Morimatsu M, Tamura K, Kogo H, Hara T. Spermatogonial cell-mediated activation of an IkappaBzeta-independent nuclear factor-kappaB pathway in Sertoli cells induces transcription of the lipocalin-2 gene. Mol Endocrinol. (2006) 20:904–15. doi: 10.1210/me.2005-0423
34. Mohammadi Roushandeh A, Halabian R, Parisa M, Soleimani Rad J, Sadeghzadeh B, Samadikuchaksaraei A, et al. Down-regulation of lipocalin 2 expression in mouse testis after exposure to electromagnetic field. Iran J Med Sci. (2009) 34:265–70.
35. Kang Z, Qiao N, Tan Z, Tang Z, Li Y. Expression patterns and changes of the LCN2 gene in the testes of induced cryptorchidism and busulfan-treated mice. Syst Biol Reprod Med. (2017) 63:364–9. doi: 10.1080/19396368.2017.1355416
36. Yanai S, Baba T, Inui K, Miyabayashi K, Han S, Inoue M, et al. Gene expression and functional abnormalities in XX/Sry Leydig cells. Sci Rep. (2021) 11:719. doi: 10.1038/s41598-020-80741-z
37. Chu ST, Lee YC, Nein KM, Chen YH. Expression, immunolocalization and sperm-association of a protein derived from 24p3 gene in mouse epididymis. Mol Reprod Dev. (2000) 57:26–36. doi: 10.1002/1098-2795(200009)57:1<26::AID-MRD5>3.0.CO;2-4
38. Cooper TG, Wagenfeld A, Cornwall GA, Hsia N, Chu ST, Orgebin-Crist MC, et al. Gene and protein expression in the epididymis of infertile c-ros receptor tyrosine kinase-deficient mice. Biol Reprod. (2003) 69:1750–62. doi: 10.1095/biolreprod.103.017566
39. Cowland JB, Borregaard N. Molecular characterization and pattern of tissue expression of the gene for neutrophil gelatinase-associated lipocalin from humans. Genomics. (1997) 45:17–23. doi: 10.1006/geno.1997.4896
40. Friedl A, Stoesz SP, Buckley P, Gould MN. Neutrophil gelatinase-associated lipocalin in normal and neoplastic human tissues. Cell type-specific Pattern expression. Histochem J. (1999) 31:433–41. doi: 10.1023/a:1003708808934
41. Beghini J, Giraldo PC, Linhares IM, Ledger WJ, Witkin SS. Neutrophil gelatinase-associated lipocalin concentration in vaginal fluid: Relation to bacterial vaginosis and vulvovaginal candidiasis. Reprod Sci. (2015) 22:964–8. doi: 10.1177/1933719115570914
42. De La Chesnaye E, Manuel-Apolinar L, Damasio L, Olivares A, Palomino MA, Santos I, et al. Expression profiling of lipocalin-2 and 24p3 receptor in murine gonads at different developmental stages. Exp Ther Med. (2018) 16:213–21. doi: 10.3892/etm.2018.6196
43. Schröder SK, Krizanac M, Kim P, Kessel JC, Weiskirchen R. Ovaries of estrogen receptor 1-deficient mice show iron overload and signs of aging. Front Endocrinol (Lausanne). (2024) 15:1325386. doi: 10.3389/fendo.2024.1325386
44. Vidal JD, Filgo AJ. Evaluation of the estrous cycle, reproductive tract, and mammary gland in female mice. Curr Protoc Mouse Biol. (2017) 7:306–25. doi: 10.1002/cpmo.35
45. Elkhalil AO, Nilsen-Hamilton M, Yoshizawa F, Sugita S. Expression of SIP24 in the peripartum and postpartum rat uterus. Connect Tissue Res. (2005) 46:235–41. doi: 10.1080/03008200500346160
46. Bong J-J, Seol M-B, Kim H-H, Han O, Back K, Baik M. The 24p3 gene Is induced during involution of the mammary gland and induces apoptosis of mammary epithelial cells. Mol Cells. (2004) 17:29–34. doi: 10.1016/S1016-8478(23)13001-9
47. Ryon J, Bendickson L, Nilsen-Hamilton M. High expression in involuting reproductive tissues of uterocalin/24p3, a lipocalin and acute phase protein. Biochem J. (2002) 367:271–7. doi: 10.1042/BJ20020026
48. Haneda S, Nagaoka K, Nambo Y, Kikuchi M, Nakano Y, Li J, et al. Expression of uterine lipocalin 2 and its receptor during early- to mid-pregnancy period in mares. J Reprod Dev. (2017) 63:127–33. doi: 10.1262/jrd.2016-096
49. Lingwood D. Lipocalin 2 as a membrane-reorganizing agent. Sci Signal. (2014) 7:pe19. doi: 10.1126/scisignal.2005563
50. Lee YC, Liao C, Li PT, Tzeng WF, Chu ST. Mouse lipocalin as an enhancer of spermatozoa motility. Mol Biol Rep. (2003) 30:165–72. doi: 10.1023/a:1024985024661
51. Lee YC, Elangovan N, Tzeng WF, Chu ST. Mouse uterine 24p3 protein as a suppressor of sperm acrosome reaction. Mol Biol Rep. (2005) 32:237–45. doi: 10.1007/s11033-005-7096-5
52. Berger T, Togawa A, Duncan GS, Elia AJ, You-Ten A, Wakeham A, et al. Lipocalin 2-deficient mice exhibit increased sensitivity to Escherichia coli infection but not to ischemia-reperfusion injury. Proc Natl Acad Sci U.S.A. (2006) 103:1834–9. doi: 10.1073/pnas.0510847103
53. Cunha GR, Sinclair A, Ricke WA, Robboy SJ, Cao M, Baskin LS. Reproductive tract biology: Of mice and men. Differentiation. (2019) 110:49–63. doi: 10.1016/j.diff.2019.07.004
54. Knoblaugh SE, True L, Tretiakova M, Hukkanen RR. Male reproductive system. In: Comparative Anatomy and Histology (Second Edition): A mouse, rat, and human atlas. Treuting PM, Dintzis SM, Montine KS, editors. London, San Diego, Cambridge, Kidlington: Elsevier, Academic Press Inc (2017) p. 335–63.
55. Elangovan N, Lee YC, Tzeng WF, Chu ST. Delivery of ferric ion to mouse spermatozoa is mediated by lipocalin internalization. Biochem Biophys Res Commun. (2004) 319:1096–104. doi: 10.1016/j.bbrc.2004.05.091
56. De La Chesnaye E, Manuel-Apolinar L, Damasio L, Castrejón E, López-Ballesteros R, Revilla-Monsalve MC, et al. The gonadal expression pattern of lipocalin-2 and 24p3 receptor is modified in the gonads of the offspring of obese rats. Mol Med Rep. (2020) 22:1409–19. doi: 10.3892/mmr.2020.11226
57. Hamilton KJ, Hewitt SC, Arao Y, Korach KS. Estrogen hormone biology. Curr Top Dev Biol. (2017) 125:109–46. doi: 10.1016/bs.ctdb.2016.12.005
58. Yan QW, Yang Q, Mody N, Graham TE, Hsu CH, Xu Z, et al. The adipokine lipocalin 2 is regulated by obesity and promotes insulin resistance. Diabetes. (2007) 56:2533–40. doi: 10.2337/db07-0007
59. Chen X, Jia X, Qiao J, Guan Y, Kang J. Adipokines in reproductive function: a link between obesity and polycystic ovary syndrome. J Mol Endocrinol. (2013) 50:R21–37. doi: 10.1530/JME-12-0247
60. Diamanti-Kandarakis E, Livadas S, Kandarakis SA, Margeli A, Papassotiriou I. Serum concentrations of atherogenic proteins neutrophil gelatinase-associated lipocalin and its complex with matrix metalloproteinase-9 are significantly lower in women with polycystic ovary syndrome: hint of a protective mechanism. Eur J Endocrinol. (2008) 158:525–31. doi: 10.1530/EJE-07-0822
61. Ranjbaran J, Farimani M, Tavilani H, Ghorbani M, Karimi J, Poormonsefi F, et al. Matrix metalloproteinases 2 and 9 and MMP9/NGAL complex activity in women with PCOS. Reproduction. (2016) 151:305–11. doi: 10.1530/REP-15-0340
62. Sahin SB, Cure MC, Ugurlu Y, Ergul E, Gur EU, Alyildiz N, et al. Epicardial adipose tissue thickness and NGAL levels in women with polycystic ovary syndrome. J Ovarian Res. (2014) 7:24. doi: 10.1186/1757-2215-7-24
63. Cakal E, Ozkaya M, Engin-Ustun Y, Ustun Y. Serum lipocalin-2 as an insulin resistance marker in patients with polycystic ovary syndrome. J Endocrinol Invest. (2011) 34:97–100. doi: 10.1007/BF03347037
64. Panidis D, Tziomalos K, Koiou E, Kandaraki EA, Tsourdi E, Delkos D, et al. The effects of obesity and polycystic ovary syndrome on serum lipocalin-2 levels: a cross-sectional study. Reprod Biol Endocrinol. (2010) 8:151. doi: 10.1186/1477-7827-8-151
65. Koiou E, Tziomalos K, Katsikis I, Kandaraki EA, Kalaitzakis E, Delkos D, et al. Weight loss significantly reduces serum lipocalin-2 levels in overweight and obese women with polycystic ovary syndrome. Gynecol Endocrinol. (2012) 28:20–4. doi: 10.3109/09513590.2011.588745
66. Wei Y, Li L, Wang F, Fu L, Li Z, Hu Y. Circulating neutrophil gelatinase-associated lipocalin and preeclampsia: a meta-analysis. J Matern Fetal Neonatal Med. (2023) 36:2197100. doi: 10.1080/14767058.2023.2197100
67. Stepan H, Philipp A, Reiche M, Klostermann K, Schrey S, Reisenbüchler C, et al. Serum levels of the adipokine lipocalin-2 are increased in preeclampsia. J Endocrinol Invest. (2010) 33:629–32. doi: 10.1007/BF03346661
68. Zhu J, Jiang S, Jiang X, Luo K, Huang X, Hua F. Association of blood lipocalin-2 levels with gestational diabetes mellitus: A systematic review and meta-analysis. Horm Metab Res. (2022) 54:677–85. doi: 10.1055/a-1909-1922
69. Gudicha DW, Romero R, Gomez-Lopez N, Galaz J, Bhatti G, Done B, et al. The amniotic fluid proteome predicts imminent preterm delivery in asymptomatic women with a short cervix. Sci Rep. (2022) 12:11781. doi: 10.1038/s41598-022-15392-3
70. Beghini J, Giraldo PC, Eleutério J, Amaral RL, Polpeta NC, Gonçalves AK. Vaginal inflammation: Association between leukocyte concentration and levels of immune mediators. Am J Reprod Immunol. (2016) 75:126–33. doi: 10.1111/aji.12475
71. Scholl J, Nasioudis D, Boester A, Speleotes M, Grunebaum A, Witkin SS. Group B streptococcus alters properties of vaginal epithelial cells in pregnant women. Am J Obstet Gynecol. (2016) 214:383.e1–5. doi: 10.1016/j.ajog.2015.12.053
72. Stanton A, Mowbray C, Lanz M, Brown K, Hilton P, Tyson-Capper A, et al. Topical estrogen treatment augments the vaginal response to Escherichia coli flagellin. Sci Rep. (2020) 10:8473. doi: 10.1038/s41598-020-64291-y
73. Horne AW, Missmer SA. Pathophysiology, diagnosis, and management of endometriosis. BMJ. (2022) 379:e070750. doi: 10.1136/bmj-2022-070750
74. Guney G, Taskin MI, Laganà AS, Tolu E, Aslan F, Hismiogullari AA, et al. Neutrophil gelatinase-associated lipocalin serum level: A potential noninvasive biomarker of endometriosis. Med (Baltimore). (2023) 102:e35539. doi: 10.1097/MD.0000000000035539
75. Bostanci Durmus A, Dincer Cengiz S, Yılmaz H, Candar T, Gursoy AY, Sinem Caglar G. The levels of matrix metalloproteinase-9 and neutrophil gelatinase-associated lipocalin in different stages of endometriosis. J Obstet Gynaecol. (2019) 39:991–5. doi: 10.1080/01443615.2019.1584889
76. Yu HC, Lin CY, Chang WC, Shen BJ, Chang WP, Chuang CM, et al. Increased association between endometriosis and endometrial cancer: a nationwide population-based retrospective cohort study. Int J Gynecol Cancer. (2015) 25:447–52. doi: 10.1097/IGC.0000000000000384
77. Yamada Y, Miyamoto T, Kashima H, Kobara H, Asaka R, Ando H, et al. Lipocalin 2 attenuates iron-related oxidative stress and prolongs the survival of ovarian clear cell carcinoma cells by up-regulating the CD44 variant. Free Radic Res. (2016) 50:414–25. doi: 10.3109/10715762.2015.1134795
78. Li T, Mamillapalli R, Ding S, Chang H, Liu ZW, Gao XB, et al. Endometriosis alters brain electrophysiology, gene expression and increases pain sensitization, anxiety, and depression in female mice. Biol Reprod. (2018) 99:349–59. doi: 10.1093/biolre/ioy035
79. Liao CJ, Li PT, Lee YC, Li SH, Chu ST. Lipocalin 2 induces the epithelial-mesenchymal transition in stressed endometrial epithelial cells: possible correlation with endometriosis development in a mouse model. Reproduction. (2014) 147:179–87. doi: 10.1530/REP-13-0236
80. Taylor AH, Tortolani D, Ayakannu T, Konje JC, Maccarrone M. (Endo)cannabinoids and gynaecological cancers. Cancers (Basel). (2020) 13:37. doi: 10.3390/cancers13010037
81. Hao P, Li H, Wu A, Zhang J, Wang C, Xian X, et al. Lipocalin2 promotes cell proliferation and migration in ovarian cancer through activation of the ERK/GSK3β/β-catenin signaling pathway. Life Sci. (2020) 262:118492. doi: 10.1016/j.lfs.2020.118492
82. Coticchia CM, Curatolo AS, Zurakowski D, Yang J, Daniels KE, Matulonis UA, et al. Urinary MMP-2 and MMP-9 predict the presence of ovarian cancer in women with normal CA125 levels. Gynecol Oncol. (2011) 123:295–300. doi: 10.1016/j.ygyno.2011.07.034
83. Lim R, Ahmed N, Borregaard N, Riley C, Wafai R, Thompson EW, et al. Neutrophil gelatinase-associated lipocalin (NGAL) an early-screening biomarker for ovarian cancer: NGAL is associated with epidermal growth factor-induced epithelio-mesenchymal transition. Int J Cancer. (2007) 120:2426–34. doi: 10.1002/ijc.22352
84. Cho H, Kim JH. Lipocalin2 expressions correlate significantly with tumor differentiation in epithelial ovarian cancer. J Histochem Cytochem. (2009) 57:513–21. doi: 10.1369/jhc.2009.953257
85. Santin AD, Zhan F, Bellone S, Palmieri M, Cane S, Bignotti E, et al. Gene expression profiles in primary ovarian serous papillary tumors and normal ovarian epithelium: identification of candidate molecular markers for ovarian cancer diagnosis and therapy. Int J Cancer. (2004) 112:14–25. doi: 10.1002/ijc.20408
86. Liao CJ, Huang YH, Au HK, Wang LM, Chu ST. The cancer marker neutrophil gelatinase-associated lipocalin is highly expressed in human endometrial hyperplasia. Mol Biol Rep. (2012) 39:1029–36. doi: 10.1007/s11033-011-0828-9
87. Miyamoto T, Kashima H, Suzuki A, Kikuchi N, Konishi I, Seki N, et al. Laser-captured microdissection-microarray analysis of the genes involved in endometrial carcinogenesis: stepwise up-regulation of lipocalin2 expression in normal and neoplastic endometria and its functional relevance. Hum Pathol. (2011) 42:1265–74. doi: 10.1016/j.humpath.2010.07.027
88. Ren X, Liang J, Zhang Y, Jiang N, Xu Y, Qiu M, et al. Single-cell transcriptomic analysis highlights origin and pathological process of human endometrioid endometrial carcinoma. Nat Commun. (2022) 13:6300. doi: 10.1038/s41467-022-33982-7
89. Srdelić Mihalj S, Kuzmić-Prusac I, Zekić-Tomaš S, Šamija-Projić I, Čapkun V. Lipocalin-2 and matrix metalloproteinase-9 expression in high-grade endometrial cancer and their prognostic value. Histopathology. (2015) 67:206–15. doi: 10.1111/his.12633
90. Mannelqvist M, Stefansson IM, Wik E, Kusonmano K, Raeder MB, Øyan AM, et al. Lipocalin 2 expression is associated with aggressive features of endometrial cancer. BMC Cancer. (2012) 12:169. doi: 10.1186/1471-2407-12-169
91. Miyamoto T, Asaka R, Suzuki A, Takatsu A, Kashima H, Shiozawa T. Immunohistochemical detection of a specific receptor for lipocalin2 (solute carrier family 22 member 17, SLC22A17) and its prognostic significance in endometrial carcinoma. Exp Mol Pathol. (2011) 91:563–8. doi: 10.1016/j.yexmp.2011.06.002
92. Cymbaluk-Płoska A, Chudecka-Głaz A, Pius-Sadowska E, Machaliński B, Sompolska-Rzechuła A, Kwiatkowski S, et al. The role of lipocalin-2 serum levels in the diagnostics of endometrial cancer. Cancer biomark. (2019) 24:315–24. doi: 10.3233/CBM-181942
93. Lin HH, Liao CJ, Lee YC, Hu KH, Meng HW, Chu ST. Lipocalin-2-induced cytokine production enhances endometrial carcinoma cell survival and migration. Int J Biol Sci. (2011) 7:74–86. doi: 10.7150/ijbs.7.74
94. Lin HH, Li WW, Lee YC, Chu ST. Apoptosis induced by uterine 24p3 protein in endometrial carcinoma cell line. Toxicology. (2007) 234:203–15. doi: 10.1016/j.tox.2007.02.017
95. Miyamoto T, Kashima H, Yamada Y, Kobara H, Asaka R, Ando H, et al. Lipocalin 2 enhances migration and resistance against cisplatin in endometrial carcinoma cells. PloS One. (2016) 11:e0155220. doi: 10.1371/journal.pone.0155220
96. Burd EM. Human papillomavirus and cervical cancer. Clin Microbiol Rev. (2003) 16:1–17. doi: 10.1128/CMR.16.1.1-17.2003
97. Chung IH, Wu TI, Liao CJ, Hu JY, Lin YH, Tai PJ, et al. Overexpression of lipocalin 2 in human cervical cancer enhances tumor invasion. Oncotarget. (2016) 7:11113–26. doi: 10.18632/oncotarget.7096
98. Vitkauskaitė A, Celiešiūtė J, Paškauskas S, Skrodenienė E, Nadišauskienė RJ, Burkauskienė A, et al. Associations among serum lipocalin-2 concentration, human papilloma virus and clinical stage of cervical cancer. Medicina (Kaunas). (2019) 55:229. doi: 10.3390/medicina55060229
99. Klein B, Bhushan S, Günther S, Middendorff R, Loveland KL, Hedger MP, et al. Differential tissue-specific damage caused by bacterial epididymo-orchitis in the mouse. Mol Hum Reprod. (2020) 26:215–27. doi: 10.1093/molehr/gaaa011
100. Sharma A, Minhas S, Dhillo WS, Jayasena CN. Male infertility due to testicular disorders. J Clin Endocrinol Metab. (2021) 106:e442–59. doi: 10.1210/clinem/dgaa781
101. Tung MC, Hsieh SC, Yang SF, Cheng CW, Tsai RT, Wang SC, et al. Knockdown of lipocalin-2 suppresses the growth and invasion of prostate cancer cells. Prostate. (2013) 73:1281–90. doi: 10.1002/pros.22670
Keywords: Lipocalin 2 (LCN2), uterocalin, fertility, reproductive system/tract, cancer, estrogen, biomarker
Citation: Krizanac M, Mass Sanchez PB, Weiskirchen R and Schröder SK (2024) Overview of the expression patterns and roles of Lipocalin 2 in the reproductive system. Front. Endocrinol. 15:1365602. doi: 10.3389/fendo.2024.1365602
Received: 04 January 2024; Accepted: 25 March 2024;
Published: 05 April 2024.
Edited by:
Fred Sinowatz, Ludwig Maximilian University of Munich, GermanyReviewed by:
James Arthur MacLean, Washington State University, United StatesCopyright © 2024 Krizanac, Mass Sanchez, Weiskirchen and Schröder. This is an open-access article distributed under the terms of the Creative Commons Attribution License (CC BY). The use, distribution or reproduction in other forums is permitted, provided the original author(s) and the copyright owner(s) are credited and that the original publication in this journal is cited, in accordance with accepted academic practice. No use, distribution or reproduction is permitted which does not comply with these terms.
*Correspondence: Ralf Weiskirchen, cndlaXNraXJjaGVuQHVrYWFjaGVuLmRl; Sarah K. Schröder, c2FzY2hyb2VkZXJAdWthYWNoZW4uZGU=
Disclaimer: All claims expressed in this article are solely those of the authors and do not necessarily represent those of their affiliated organizations, or those of the publisher, the editors and the reviewers. Any product that may be evaluated in this article or claim that may be made by its manufacturer is not guaranteed or endorsed by the publisher.
Research integrity at Frontiers
Learn more about the work of our research integrity team to safeguard the quality of each article we publish.