- 1Department of Endocrine, Hainan General Hospital, Hainan Affiliated Hospital of Hainan Medical University, Haikou, Hainan, China
- 2Department of Health Care Centre, Hainan General Hospital, Hainan Affiliated Hospital of Hainan Medical University, Haikou, China
Diabetes is a common chronic metabolic disease with complex causes and pathogenesis. As an immunomodulator, vitamin D has recently become a research hotspot in the occurrence and development of diabetes and its complications. Many studies have shown that vitamin D can reduce the occurrence of diabetes and delay the progression of diabetes complications, and vitamin D can reduce oxidative stress, inhibit iron apoptosis, promote Ca2+ influx, promote insulin secretion, and reduce insulin resistance. Therefore, the prevention and correction of vitamin D deficiency is very necessary for diabetic patients, but further research is needed to confirm what serum levels of vitamin D3 are maintained in the body. This article provides a brief review of the relationship between vitamin D and diabetes, including its acute and chronic complications.
1 Introduction
Diabetes mellitus (DM) is a group of metabolic diseases characterized by chronic hyperglycemia, caused by defects in insulin secretion and/or utilization. Over the past 30 years, the prevalence of diabetes in China has significantly increased. A thyroid iodine nutrition status and diabetes epidemiological survey conducted by the Endocrinology Branch of the Chinese Medical Association from 2015 to 2017 showed that the prevalence of diabetes among the Chinese population aged 18 and above was 11.2% (1). Vitamin D, a fat-soluble vitamin, not only plays a role in calcium and phosphorus regulation but is also closely related to diabetes, cardiovascular diseases, immune regulation, tumors, muscle strength enhancement, and fall prevention. Studies have shown that vitamin D is closely related to pancreatic function, immunity, genetic polymorphism, and the occurrence of diabetic complications. In recent years, the relationship between vitamin D and the development of diabetes has become a research focus in the field of diabetes. This article provides a brief overview of the effects of vitamin D on the pathogenesis and progression of complications in different types of diabetes.
2 Overview of vitamin D
Vitamin D, a derivative of steroids, belongs to the cyclopentane polyhydrophenyl compound class. It is chemically stable, except for being light-sensitive. There are two main sources of vitamin D: one is converted from 7-dehydrocholesterol in the skin under the influence of ultraviolet light; the other is from vitamin D2 in mushrooms exposed to sunlight and vitamin D3 in foods such as liver, milk, and cod liver oil. The vitamin D2 and D3 obtained from these sources are inactive forms, and they cannot be converted into each other, collectively referred to as vitamin D. To obtain biologically active 1,25(OH)2D3, it needs to undergo two hydroxylations in the body (Figure 1). Firstly, the inactive vitamin D is converted to 25(OH)D3 in the liver under the catalysis of the 25-hydroxylase enzyme. 25(OH)D3 is the main storage form in the body, and its level reflects the nutritional status of vitamin D. Then, 25(OH)D3 is further converted to 1,25(OH)2D3 in the kidneys under the action of 1α-hydroxylase. 1,25(OH)2D3 binds to vitamin D receptors(VDR) widely present in tissues and exerts its effects in the body (2).
3 Relationship between vitamin D levels and diabetes
3.1. Vitamin D and diabetes
A number of studies have been conducted in the population to explore the relationship between vitamin D3 and glycemic control (Table 1). Serum 25(OH)D3 levels have been shown to have a negative dose-response correlation with the risk of type 2 diabetes mellitus (T2DM) (3), and vitamin D supplementation reduces the risk of T2DM (4), decreases the risk of T2DM in pre-diabetic patients, and increases the chances of restoring normal glucose tolerance (5). However, the benefits of vitamin D3 for the prevention of T2DM may be limited to non-obese subjects (5) or patients with vitamin D deficiency (6).
Achieving blood glucose control is one of the goals of diabetes treatment. Serum 25(OH)D 3 levels can be an independent risk factor for increased levels of glycated hemoglobin in T2DM, with women being at a higher risk of vitamin D deficiency (7). In addition to finding that vitamin D can be a risk factor for glycemic control, it is interesting to note that in patients with T2DM, the combined administration of metformin and vitamin D resulted in better glycemic and glycosylated hemoglobin control compared to metformin alone (8). The efficacy of vitamin D on glycemic stability and insulin function was also found in patients with prediabetes (9). Similar results were obtained in animal experiments. Vitamin D supplementation reduced blood glucose, insulin levels, and improved insulin resistance (IR) in rats in a prediabetic model, and this efficacy was proportional to the dose of vitamin D supplementation (10). The above findings suggest that vitamin D helps in blood sugar control. However, there is still a need for larger studies in the future to examine the dose, duration, and most appropriate population for vitamin D supplementation to determine the relationship between vitamin D and glycemic control in diabetes. Serum 25(OH)D3 insufficiency is closely related to the development of type 1 diabetes mellitus (T1DM) in children (11).
3.2 Vitamin D and diabetic complications
Vitamin D is not only related to the occurrence and development of diabetes, but numerous studies have also confirmed that vitamin D deficiency is closely associated with diabetic complications (Figure 2).
Although vitamin D does not reduce all-cause mortality in older adults (12). However, there are still a series of studies proving that vitamin D can prevent cardiovascular risk in people with diabetes. The incidence of cardiovascular diseases in T2DM patients increases by 2 to 3 times (13), and a follow-up study of T2DM patients confirmed that vitamin D deficiency is the strongest correlating factor for the occurrence of cardiovascular diseases in these patients (14). Vitamin D deficiency is also related to endothelial dysfunction and atherosclerosis (15, 16). The non-high-density lipoprotein cholesterol/high-density lipoprotein cholesterol ratio (NHHR) is a new comprehensive index of atherosclerotic lipids (17). NHHR is negatively correlated with vitamin D in T2DM patients (18). A higher NHHR index indicates a greater tendency for peripheral cholesterol deposition, and an increased distribution of cholesterol to the periphery may lead to IR or pancreatic β-cell dysfunction (19), resulting in vitamin D deficiency. Supplementing vitamin D can slow down the progression of myocardial dysfunction in T2DM patients without complications (20).
A prospective study on T2DM patients found that higher serum 25(OH)D3 levels can reduce the risk of diabetic retinopathy, diabetic nephropathy, and diabetic neuropathy, and serum 25(OH)D3 levels within a certain range (10-106 nmol/L) have a linear dose-response relationship with diabetic retinopathy and diabetic nephropathy (21). Inflammation has been shown to contribute to the occurrence and development of diabetic retinopathy (22–27). The pathogenesis of diabetic retinopathy is related to inflammation and fibrosis (23), and it has been reported that the production of pro-inflammatory cytokines such as IL-1, TNF-α, and VEGF increases in the vitreous body of patients with diabetic retinopathy and in animal models of the retina (22, 24). High glucose-induced upregulation of pro-inflammatory cytokines can lead to the destruction of the blood-retinal barrier (BRB), cell death, and angiogenesis (22, 25). Supplementing vitamin D can protect retinal epithelial cells from high glucose-induced oxidative stress, inflammation and ferroptosis which may be one of the mechanisms by which vitamin D prevents the progression of diabetic retinopathy (28, 29).
Diabetic nephropathy is a major cause of disability and death in middle-aged and elderly patients with T2DM, significantly affecting their quality of life and safety. Progressive proteinuria and renal function deterioration are the main clinical symptoms of diabetic nephropathy (30–32). A study on elderly T2DM patients in China found that the incidence of vitamin D deficiency was significantly higher in patients with proteinuria than in those without (33). In animal experiments, vitamin D can prevent podocyte damage, thereby reducing proteinuria and glomerulosclerosis (34).
Peripheral nerve damage is also a common complication of diabetes mellitus. Patients lacking vitamin D are more likely to experience nerve function deficits associated with diabetic peripheral neuropathy than those with sufficient vitamin D (35). Among the Kurdish population, lower levels of vitamin D and higher levels of HbA1c are predictive risk factors for painful diabetic peripheral neuropathy (36). Controlling blood glucose alone cannot prevent the progression of peripheral neuropathy in T2DM. Chen T (37) found that monthly intramuscular injections of high-dose vitamin D improved peripheral neuropathy. This may provide new ideas for treating diabetic peripheral nerves. However, more research is needed to determine the exact course of treatment and dosage. In a study of diabetes mellitus in children (38), children with vitamin D deficiency did not complain of peripheral neuropathy, but sensory nerve action potential of sural nerve and motor peroneal nerve velocity were statistically significantly lower in diabetic patients with vitamin D deficiency compared to diabetic patients with normal vitamin D levels.
Diabetic ketosis is one of the acute complications of diabetes mellitus. Serum 25(OH)D3 levels are lower in ketosis-prone T2DM compared to non-ketosis-prone T2DM (39, 40), and serum 25(OH)D3 levels are related to the severity of pancreatitis concurrent with diabetic ketoacidosis (41).
4 Vitamin D and the pathogenesis of diabetes
4.1 Vitamin D and pancreatic function
Vitamin D may affect pancreatic function through several signaling pathways (Figure 3). Glucose-6-phosphatase (G6Pase) and phosphoenolpyruvate carboxykinase (PEPCK) are two key enzymes that convert non-carbohydrate substances into glucose (42, 43). Their abnormal expression is closely associated with enhanced gluconeogenesis and considered a marker for T2DM (44). Combined treatment with vitamin D and aerobic exercise can upregulate protein kinase B (AKT) in liver cells of T2DM rats, downregulate PEPCK and G6Pase expression, improve liver function, and alleviate IR (45). Vitamin D-binding protein (VDBP) is the primary plasma carrier maintaining vitamin D and its metabolites. Deficiency of VDBP may lead to pancreatic α-cell atrophy and proliferation, alters Na+ channel conductance, reduces cellular activation by glucose, and decreases the rate of glucagon secretion in vivo, potentially increasing the incidence of late-onset T1DM (46). A double-blind, randomized, controlled clinical trial (47) showed that vitamin D supplementation improved β-cell function in patients with serum 25(OH)D3 levels below 12 ng/mL compared to placebo.
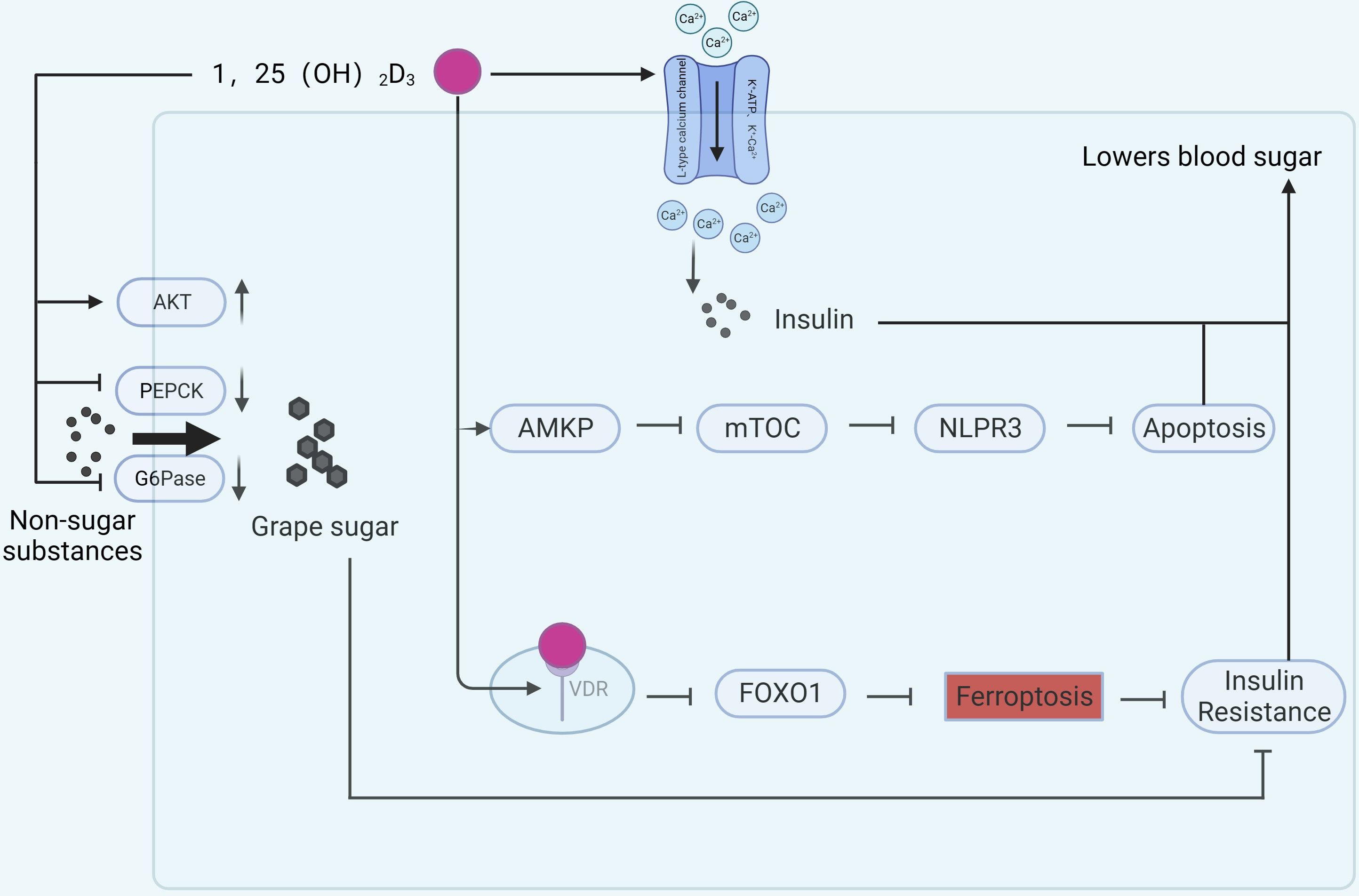
Figure 3 Mechanisms of vitamin D involvement in pancreatic function. (AKT, protein kinase B; PEACK, phosphoenolpyruvate carboxykinase; G6Pase, Glucose-6-phosphatase; AMPK, Adenosine 5’-monophosphate (AMP)-activated protein kinase; mTOR, mammalian target of rapamycin).
Intraperitoneal injection of 1,25(OH)2D3 treats dexamethasone-induced IR in rats and improves islet function. The reason is related to the alteration of calcium ions after activation of L-type voltage-dependent calcium channel (VDCC), K+-ATP, K+-Ca2+, and Kv channels by 1,25(OH)2D3, followed by activation of downstream PKC, PKA, and so on, which promotes insulin secretion (48). In vitro studies indicate that vitamin D can activate AMP-dependent protein kinase, inhibit the mammalian target of rapamycin (mTOR) pathway, thereby inhibiting the activation of the NLRP3 inflammasome and reducing β-cell apoptosis, promoting insulin release (49). Vitamin D may also reduce β-cell apoptosis in T2DM by inhibiting nuclear factor κB and downregulating the expression of divalent metal transporter 1 (DMT1) , alleviating pancreatic iron overload (50).
Ferroptosis, a newly discovered form of cell death, is considered a crucial factor in the pathogenesis of many inflammatory diseases (51). Ferroptosis is also considered a new target for diabetes (52). In the rat diabetes model, ferroptosis-related indicators such as GPX4 and SLC7A11 were downregulated (53) and ACSL4 was upregulated (54). 1,25(OH)2D3 treatment not only lowered blood glucose, but also reversed the changes in the above metrics. The mechanism is related to 1,25(OH)2D3/VDR/FOXO1. Binding of 1,25(OH)2D3 to the VDR inhibits ferroptosis in pancreatic β-cells and ameliorates IR by down-regulating FOXO1 expression (54). Vitamin D induces cellular autophagy while inhibiting streptozotocin-induced β-cell apoptosis, increasing insulin secretion and increasing β-cell resistance to cellular stresses encountered in hyperglycemic states (55). Excessive or prolonged exposure to nitric oxide leads to β-cell dysfunction, whereas vitamin D induces and maintains high levels of the A20 gene protein and reduces nitric oxide levels, thus serving to protect β-cell function (56).
4.2 Vitamin D and immune function
Type 1 diabetes is an autoimmune disease. Vitamin D, by binding with its receptor, reduces pro-inflammatory cytokines in immune cells and has an immunomodulatory effect (57, 58). CD4+ T lymphocytes are the primary immune-mediated cells in the development of T1DM (59). Vitamin D supplementation can downregulate cathepsin G (Cat G) expression, hindering CD4+ T lymphocyte activation, thus enhancing pancreatic β-cell function (60). Systemic lupus erythematosus (SLE) is an immune disease. It has been found that IR is more prevalent in SLE patients than in controls (61), while serum vitamin D is negatively correlated with CD4+/CD8+ T cells (62), IFN-α levels (63), IL-17, IL-23 (64) in SLE patients. 25(OH)D3 is further converted into 1,25(OH)2D3 in the kidneys by 1α-hydroxylase, which is expressed by antigen-presenting cells (65), indicating an association of the immune microenvironment with active vitamin D production, suggesting that vitamin D may mediate the disease process in T1DM. Complement can trigger the contraction of CD4+ type 1 helper T cell responses by inducing the intrinsic expression of VDR and vitamin D-activating enzyme CYP27B1, enabling T cells to both activate and respond to vitamin D (66). Vitamin D deficiency can epigenetically suppress Jarid2 expression in hepatic stellate cells (HSCs) and activate the Mef2/PGC1a pathway, leading to fat macrophage infiltration. Macrophages secrete MiR-106b-5p, inducing downregulation of the PIK3CA/PIK3R1/PDPK1/AKT signaling pathway, promoting fat IR (67). Vitamin D supplementation can reduce IR in diabetic rats by lowering phosphorylation levels of insulin receptor substrate 1 (IRS1), leading to impaired glucose transporter 4 and reduced glucose uptake, as well as increasing peroxisome proliferator-activated receptor γ expression and reducing nuclear factor κB phosphorylation levels (10). In addition, vitamin D increases insulin secretion and sensitivity by up-regulating mitogen-activated protein kinase phosphorylase-1 (MKP-1), inhibiting lipopolysaccharide-induced p38 phosphorylation, suppressing the production of IL-6 and TNF-α in human monocytes (68), and increasing the activity of the antioxidant system (69). Vitamin D has a strong protective effect on chronic kidney diseases (70, 71). The possible mechanism is that 1,25(OH)2D3 reduces oxidative stress by increasing renal antioxidant capacity, inhibiting hyperglycemia-induced cell apoptosis, preventing podocyte damage, promoting anti-inflammatory action, and improving endothelial function (72–74) (Figure 4). In T1DM, some patients may experience a clinical remission period, also known as the “honeymoon phase”, after receiving insulin therapy in the early stage of the disease, in which the islet function of the patient may partially or completely return to normal levels, and vitamin D may reduce the concentration of serum TNF-α through immunomodulatory effects, thereby reducing the inflammatory response during the honeymoon phase and prolonging the duration of the honeymoon phase (75), but the amount of vitamin D supplementation needs to be further studied.
4.3 Vitamin D polymorphism and diabetes
Polymorphism in the VDR gene plays a role in the control and progression of T1DM, with higher levels of vitamin D providing protection for pancreatic cells (76). Polymorphisms in the 25-hydroxylase (CYP2R1) gene, rs12794714 and rs10766196, are associated with a higher risk of T1DM (77). It was found that CYP2R1 mRNA expression in the livers of mice fed a high-fat diet was significantly lower than in those fed a low-fat diet (78). As well, an activity analysis of the isolated liver showed that obese mice produced significantly less 25(OH)D3 than lean mice, indicating that reduced circulating 25(OH)D3 is partly due to the decreased expression of CYP2R1 in obese mice. The onset of T1DM might also be related to polymorphism in the CYP27B1 gene located on chromosome 12, where polymorphisms in the CYP27B1 gene could lead to reduced levels of 1α-hydroxylase, thereby affecting the conversion of vitamin D to 1,25(OH)2D3 and increasing the susceptibility to T1DM (79). Tangjittipokin (80) found that VDR gene-related variations of ApaI (rs7975232), TaqI (rs731236), and BsmI (rs1544410) were negatively associated with vitamin D and IL-10 levels in children with T1DM. Alleles of DHCR7, GC, CYP2R1, and CYP24A1 play a synergistic role in susceptibility to type 1 diabetes by functioning in the vitamin D pathway and serum vitamin D levels (81). A genome-wide association Meta-analysis study by Jiang X et al. (82) of 79,366 European individuals suggests that CYP24A1 (rs17216707) is negatively correlated with 25(OH)D3 levels. The correlation between VDR gene rs739837 polymorphism and susceptibility to T2DM and gestational diabetes mellitus (GDM) (83) pointed out that the VDR gene rs739837 polymorphism is significantly correlated with susceptibility to T2DM. Studies in gestational diabetes mellitus (84, 85) confirmed that single nucleotide polymorphisms (SNPs) mutations at VDR-rs10783219 and MTNR1B-rs10830962 significantly increased the risk of GDM, ApaI-rs79785232, BsmI-rs1544410, FokI-rs2228570 and TaqI-rs731236 are associated with GDM occurrence in the Saudi Arabian region (Table 2).
5 Conclusion and prospects
In recent years, from D to D, the role of vitamin D in the occurrence and development of diabetes has gained attention. The relationship between vitamin D and the onset, progression of diabetes, the ideal daily dosage of vitamin D supplementation, and the optimal serum 25(OH)D3 levels for maximum benefits in diabetes risk individuals, early-stage patients, blood sugar control, and diabetes-related complications still require more reliable clinical studies and basic experiments for confirmation. Current research suggests that moderate supplementation of vitamin D can improve the onset and progression of diabetes and its complications, but routine large-dose supplementation is not recommended. It is believed that the relationship between the two will be further verified in the near future.
Author contributions
CL: Conceptualization, Writing – review & editing. JF: Writing – original draft. YY: Writing – review & editing. JL: Writing – review & editing. YH: Conceptualization, Supervision, Writing – review & editing. TF: Conceptualization, Supervision, Writing – review & editing.
Funding
The author(s) declare that financial support was received for the research, authorship, and/or publication of this article. This work was supported by Hainan Province Clinical Medical Center, Hainan Province Science and Technology Special Fund (No. ZDYF2019156) and Hainan Provincial Natural Science Foundation of China (No. 824RC545).
Acknowledgments
Thanks to DT and ZD for their help with the figures production.
Conflict of interest
The authors declare that the research was conducted in the absence of any commercial or financial relationships that could be construed as a potential conflict of interest.
Publisher’s note
All claims expressed in this article are solely those of the authors and do not necessarily represent those of their affiliated organizations, or those of the publisher, the editors and the reviewers. Any product that may be evaluated in this article, or claim that may be made by its manufacturer, is not guaranteed or endorsed by the publisher.
References
1. Li Y, Teng D, Shi X, Qin G, Qin Y, Quan H, et al. Prevalence of diabetes recorded in mainland China using 2018 diagnostic criteria from the American Diabetes Association: national cross sectional study. BMJ. (2020) 369:m997. doi: 10.1136/bmj.m997
2. Christakos S, Dhawan P, Verstuyf A, Verlinden L, Carmeliet G. Vitamin D: metabolism, molecular mechanism of action, and pleiotropic effects. Physiol Rev. (2016) 96:365–408. doi: 10.1152/physrev.00014.2015. (1522-1210 (Electronic)).
3. Wang M, Zhou T, Li X, Ma H, Liang Z, Fonseca VA, et al. Baseline vitamin D status, sleep patterns, and the risk of incident type 2 diabetes in data from the UK biobank study. Diabetes Care. (2020) 43:2776–84. doi: 10.2337/dc20-1109
4. Hsia DS, Nelson J, Vickery EM, Rasouli N, LeBlanc ES, Kim S, et al. Effect of vitamin D on regression to normal glucose regulation and individual glycemic measures: A secondary analysis among participants adherent to the trial protocol in the randomized clinical trial vitamin D and type 2 diabetes (D2d) study. Diabetes Res Clin Pract. (2023) 202:110792. doi: 10.1016/j.diabres.2023.110792
5. Zhang Y, Tan H, Tang J, Li J, Chong W, Hai Y, et al. Effects of vitamin D supplementation on prevention of type 2 diabetes in patients with prediabetes: A systematic review and meta-analysis. Diabetes Care. (2020) 43:1650–8. doi: 10.2337/dc19-1708
6. Pittas AG, Dawson-Hughes B, Sheehan P, Ware JH, Knowler WC, Aroda VR, et al. Vitamin D supplementation and prevention of type 2 diabetes. N Engl J Med. (2019) 381:520–30. doi: 10.1056/NEJMoa1900906
7. Wang C, Li H, Huo L, Wang Q, Zhang T, He X, et al. Serum 25-hydroxyvitamin D levels in type 2 diabetes patients in north China: seasonality and the association between vitamin D status and glycosylated hemoglobin levels. Int J Clin Pract. (2023) 2023:4151224. doi: 10.1155/2023/4151224
8. Cojic M, Kocic R, Klisic A, Kocic G. The effects of vitamin D supplementation on metabolic and oxidative stress markers in patients with type 2 diabetes: A 6-month follow up randomized controlled study. Front Endocrinol (Lausanne). (2021) 12:610893. doi: 10.3389/fendo.2021.610893
9. Zou Y, Guo B, Yu S, Wang D, Qiu L, Jiang Y. Effect of vitamin D supplementation on glycose homeostasis and islet function in vitamin D deficient or insufficient diabetes and prediabetes: a systematic review and meta-analysis. J Clin Biochem Nutr. (2021) 69:229–37. doi: 10.3164/jcbn.20-165
10. Krisnamurti DGB, Louisa M, Poerwaningsih EH, Tarigan TJE, Soetikno V, Wibowo H, et al. Vitamin D supplementation alleviates insulin resistance in prediabetic rats by modifying IRS-1 and PPARgamma/NF-kappaB expressions. Front Endocrinol (Lausanne). (2023) 14:1089298. doi: 10.3389/fendo.2023.1089298
11. Liu C, Lu M, Xia X, Wang J, Wan Y, He L, et al. Correlation of serum vitamin D level with type 1 diabetes mellitus in children: a meta-analysis. Nutr Hosp. (2015) 32:1597–4. 1699-5198 (Electronic). doi: 10.3305/nh.2015.32.4.9198
12. Neale RE, Baxter C, Romero BD, McLeod DSA, English DR, Armstrong BK, et al. The D-Health Trial: a randomised controlled trial of the effect of vitamin D on mortality. Lancet Diabetes Endocrinol. (2022) 10:120–8. doi: 10.1016/S2213-8587(21)00345-4. 2213-8595 (Electronic).
13. Ma CX, Ma XN, Guan CH, Li YD, Mauricio D, Fu SB. Cardiovascular disease in type 2 diabetes mellitus: progress toward personalized management. Cardiovasc Diabetol. (2022) 21:74. doi: 10.1186/s12933-022-01516-6
14. de la Guia-Galipienso F, Martinez-Ferran M, Vallecillo N, Lavie CJ, Sanchis-Gomar F, Pareja-Galeano H. Vitamin D and cardiovascular health. Clin Nutr. (2021) 40:2946–57. doi: 10.1016/j.clnu.2020.12.025
15. Kim DH, Meza CA, Clarke H, Kim JS, Hickner RC. Vitamin D and endothelial function. Nutrients. (2020) 12. doi: 10.3390/nu12020575
16. Rodriguez AJ, Scott D, Srikanth V, Ebeling P. Effect of vitamin D supplementation on measures of arterial stiffness: a systematic review and meta-analysis of randomized controlled trials. Clin Endocrinol (Oxf). (2016) 84:645–57. doi: 10.1111/cen.13031
17. Sheng GA-O, Liu D, Kuang M, Zhong Y, Zhang S, Zou YA-OX. Utility of non-high-density lipoprotein cholesterol to high-density lipoprotein cholesterol ratio in evaluating incident diabetes risk. Diabetes Metab Syndr Obes. (2022) 31:1677–86. doi: 10.2147/DMSO.S355980. 15(1178-7007 (Print).
18. Zhen R, Ban J, Jia Z, Liu Y, Li Z, Chen S. The relationship between non-HDL-C /HDL-C ratio (NHHR) and vitamin D in type 2 diabetes mellitus. Diabetes Metab Syndr Obes. (2023) 16:2661–73. doi: 10.2147/DMSO.S414673
19. Narvaez CJ, Simmons KM, Brunton J, Salinero A, Chittur SV, Welsh JE. Induction of STEAP4 correlates with 1,25-dihydroxyvitamin D3 stimulation of adipogenesis in mesenchymal progenitor cells derived from human adipose tissue. J Cell Physiol. (2013) 228:2024–36. doi: 10.1002/jcp.24371
20. Obert P, Nottin S, Philouze C, Aboukhoudir F. Major impact of vitamin D(3) deficiency and supplementation on left ventricular torsional mechanics during dobutamine stress in uncomplicated type 2 diabetes. Nutr Metab Cardiovasc Dis. (2023) 33(11):2269–79. doi: 10.1016/j.numecd.2023.06.017
21. Chen X, Wan Z, Geng T, Zhu K, Li R, Lu Q, et al. Vitamin D status, vitamin D receptor polymorphisms, and risk of microvascular complications among individuals with type 2 diabetes: A prospective study. Diabetes Care. (2023) 46:270–7. doi: 10.2337/dc22-0513
22. Stitt AW, Curtis TM, Chen M, Medina RJ, McKay GJ, Jenkins A, et al. The progress in understanding and treatment of diabetic retinopathy. Prog Retin Eye Res. (2016) 51:156–86. doi: 10.1016/j.preteyeres.2015.08.001
23. Platania CBM, Leggio GM, Drago F, Salomone S, Bucolo C. Computational systems biology approach to identify novel pharmacological targets for diabetic retinopathy. Biochem Pharmacol. (2018) 158:13–26. doi: 10.1016/j.bcp.2018.09.016
24. Lazzara F, Fidilio A, Platania CBM, Giurdanella G, Salomone S, Leggio GM, et al. Aflibercept regulates retinal inflammation elicited by high glucose via the PlGF/ERK pathway. Biochem Pharmacol. (2019) 168:341–51. doi: 10.1016/j.bcp.2019.07.021
25. Platania CBM, Lazzara F, Fidilio A, Fresta CG, Conti F, Giurdanella G, et al. Blood-retinal barrier protection against high glucose damage: The role of P2X7 receptor. Biochem Pharmacol. (2019) 168:249–58. doi: 10.1016/j.bcp.2019.07.010
26. D'Amico AG, Maugeri G, Reitano R, Bucolo C, Saccone S, Drago F, et al. PACAP modulates expression of hypoxia-inducible factors in streptozotocin-induced diabetic rat retina. J Mol Neurosci. (2015) 57:501–9. doi: 10.1007/s12031-015-0621-7
27. Platania CBM, Maisto R, Trotta MC, D'Amico M, Rossi S, Gesualdo C, et al. Retinal and circulating miRNA expression patterns in diabetic retinopathy: An in silico and in vivo approach. Br J Pharmacol. (2019) 176:2179–94. doi: 10.1111/bph.14665
28. Tohari AM, Almarhoun M, Alhasani RH, Biswas L, Zhou X, Reilly J, et al. Protection by vitamin D against high-glucose-induced damage in retinal pigment epithelial cells. Exp Cell Res. (2020) 392:112023. doi: 10.1016/j.yexcr.2020.112023
29. Zhan D, Zhao J, Shi Q, Lou J, Wang W. 25-hydroxyvitamin D3 inhibits oxidative stress and ferroptosis in retinal microvascular endothelial cells induced by high glucose through down-regulation of miR-93. BMC Ophthalmol. (2023) 13:1471–2415. doi: 10.1186/s12886-022-02762-8
30. Khatib ST, Hemadneh MK, Hasan SA, Khazneh E, Zyoud SH. Quality of life in hemodialysis diabetic patients: a multicenter cross-sectional study from Palestine. BMC Nephrol. (2018) 19:49. doi: 10.1186/s12882-018-0849-x
31. Foundation NK. KDOQI clinical practice guideline for diabetes and CKD: 2012 update. Am J Kidney Dis. (2012) 60:850–86. doi: 10.1053/j.ajkd.2012.07.005. (1523-6838 (Electronic)).
32. Bonner R, Albajrami O, Hudspeth J, Upadhyay A. Diabetic kidney disease. Prim Care. (2020) 47:645–59. doi: 10.1016/j.pop.2020.08.004
33. Zhang L, Guo Q, Xu Y, Wei W, Wang Y. Association between serum 25- hydroxyvitamin D and albuminuiria in middle-aged and older Chinese patients with type 2 diabetes. BMC Endocr Disord. (2023) 23:189. doi: 10.1186/s12902-023-01440-0
34. Kuhlmann A, Haas Cs Fau - Gross M-L, Gross Ml Fau - Reulbach U, Reulbach U Fau - Holzinger M, Holzinger M Fau - Schwarz U, Schwarz U Fau - Ritz E, et al. 1,25-Dihydroxyvitamin D3 decreases podocyte loss and podocyte hypertrophy in the subtotally nephrectomized rat. Am J Physiol Renal Physiol. (2003) 286:F526–533. doi: 10.1152/ajprenal.00316.2003. (1931-857X (Print)).
35. Pang C, Yu H, Cai Y, Song M, Feng F, Gao L, et al. Vitamin D and diabetic peripheral neuropathy: A multi-centre nerve conduction study among Chinese patients with type 2 diabetes. Diabetes Metab Res Rev. (2023) 39:e3679. doi: 10.1002/dmrr.3679
36. Hassan AB, Al-Dosky AHA. Vitamin D status and its association with inflammatory markers among Kurdish type 2 diabetic patients with painful diabetic peripheral neuropathy. Steroids. (2023) 199:109289. doi: 10.1016/j.steroids.2023.109289
37. Chen T, Xing X, Huang L, Tu M, Lai X, Wen S, et al. Efficacy and safety of high-dose intramuscular vitamin D(2) injection in type 2 diabetes mellitus with distal symmetric polyneuropathy combined with vitamin D insufficiency: study protocol for a multicenter, randomized, double-blinded, and placebo-controlled trial. Front Endocrinol (Lausanne). (2023) 14:1202917. doi: 10.3389/fendo.2023.1202917
38. Polat İ, Can Yılmaz G, Dedeoğlu Ö. Vitamin D and nerve conduction in pediatric type-1 diabetes mellitus. Brain Dev. (2022) 44:336–42. doi: 10.1016/j.braindev.2022.01.001. (1872-7131 (Electronic)).
39. He X, Luo Y, Hao J, Wang C, Gan K, Zhen Y, et al. Association between serum vitamin D levels and ketosis episodes in hospitalized patients with newly diagnosed ketosis-prone type 2 diabetes. Diabetes Metab Syndr Obes. (2022) 15:3821–9. doi: 10.2147/DMSO.S389609
40. Nóvoa-Medina YA-O, Barreiro-Bautista M, Perdomo-Quinteiro M, González-Martín JA-O, Quinteiro-González S, Domínguez Á, et al. 25OHVitamin D levels in a canarian pediatric population with and without type 1 diabetes: the role of acidosis. Nutrients. (2023) 15:3067. doi: 10.3390/nu15133067. (2072-6643 (Electronic)).
41. Lu S, Wei D, Yin C, Xiong J, Zhu L, Yan S, et al. Correlation between PCT, 25(OH)D, PTX-3, AMS levels and the severity of diabetic ketoacidosis complicated by pancreatitis. BMC Endocr Disord. (2021) 21:136. doi: 10.1186/s12902-021-00792-9
42. Barthel A, Schmoll D, Kruger KD, Bahrenberg G, Walther R, Roth RA, et al. Differential regulation of endogenous glucose-6-phosphatase and phosphoenolpyruvate carboxykinase gene expression by the forkhead transcription factor FKHR in H4IIE-hepatoma cells. Biochem Biophys Res Commun. (2001) 285:897–902. doi: 10.1006/bbrc.2001.5261
43. Zhu YX, Hu HQ, Zuo ML, Mao L, Song GL, Li TM, et al. Effect of oxymatrine on liver gluconeogenesis is associated with the regulation of PEPCK and G6Pase expression and AKT phosphorylation. BioMed Rep. (2021) 15:56. doi: 10.3892/br.2021.1432
44. Sharma R, Kumari M, Prakash P, Gupta S, Tiwari SA-O. Phosphoenolpyruvate carboxykinase in urine exosomes reflect impairment in renal gluconeogenesis in early insulin resistance and diabetes. Am J Physiol Renal Physiol. (2020) 318:F720–31. doi: 10.1152/ajprenal.00507.2019. (1522-1466 (Electronic)).
45. Hoseini Z, Behpour N, Hoseini R. Vitamin D improves the antidiabetic effectiveness of aerobic training via modulation of Akt, PEPCK, and G6Pase expression. Diabetol Metab Syndr. (2023) 15:184. doi: 10.1186/s13098-023-01158-y
46. Viloria K, Nasteska D, Briant LJB, Heising S, Larner DP, Fine NHF, et al. Vitamin-D-binding protein contributes to the maintenance of alpha cell function and glucagon secretion. Cell Rep. (2020) 31:107761. doi: 10.1016/j.celrep.2020.107761
47. Rasouli N, Brodsky IG, Chatterjee R, Kim SH, Pratley RE, Staten MA, et al. Effects of vitamin D supplementation on insulin sensitivity and secretion in prediabetes. J Clin Endocrinol Metab. (2022) 107:230–40. doi: 10.1210/clinem/dgab649
48. Mendes AKB, Sulis PM, Cavalari FC, Padilla DPR, Aragon M, Gaspar JM, et al. 1alpha,25-(OH)(2) vitamin D(3) prevents insulin resistance and regulates coordinated exocytosis and insulin secretion. J Nutr Biochem. (2022) 99:108864. doi: 10.1016/j.jnutbio.2021.108864
49. Wu M, Lu L, Guo K, Lu J, Chen H. Vitamin D protects against high glucose-induced pancreatic beta-cell dysfunction via AMPK-NLRP3 inflammasome pathway. Mol Cell Endocrinol. (2022) 547:111596. doi: 10.1016/j.mce.2022.111596
50. Zhao Y, Mei G, Zhou F, Kong B, Chen L, Chen H, et al. Vitamin D decreases pancreatic iron overload in type 2 diabetes through the NF-κB-DMT1 pathway. J Nutr Biochem. (2021) 99:108870. doi: 10.1016/j.jnutbio.2021.108870. (1873-4847 (Electronic)).
51. Li JY, Yao YM, Tian YP. Ferroptosis: A trigger of proinflammatory state progression to immunogenicity in necroinflammatory disease. Front Immunol. (2021) 12:701163. doi: 10.3389/fimmu.2021.701163. (1664-3224 (Electronic)).
52. Yang XD, Yang YY. Ferroptosis as a novel therapeutic target for diabetes and its complications. Front Endocrinol (Lausanne). (2022) 29:853822. doi: 10.3389/fendo.2022.853822. (1664-2392 (Print)).
53. Zhou Y. The Protective Effects of Cryptochlorogenic Acid on β-Cells Function in Diabetes in vivo and vitro via Inhibition of Ferroptosis. Diabetes Metab Syndr Obes. (2020) 8:1921–31. doi: 10.2147/DMSO.S249382. (1178-7007 (Print)).
54. Ding Y, Wu Q. 1,25D/VDR inhibits pancreatic β cell ferroptosis by downregulating FOXO1 expression in diabetes mellitus. Cell Signal. (2023) 105. doi: 10.1016/j.cellsig.2022.110564. (1873-3913 (Electronic)).
55. Wang Y, He D, Ni C, Zhou H, Wu S, Xue Z, et al. Vitamin D induces autophagy of pancreatic β-cells and enhances insulin secretion. Mol Med Rep. (2016) 14:44–50. doi: 10.3892/mmr.2016.5531. (1791-3004 (Electronic)).
56. Nord JA, Wynia-Smith SL, Gehant AL, Jones Lipinski RA, Naatz A, Rioja I, et al. N-terminal BET bromodomain inhibitors disrupt a BRD4-p65 interaction and reduce inducible nitric oxide synthase transcription in pancreatic beta-cells. Front Endocrinol (Lausanne). (2022) 13:923925. doi: 10.3389/fendo.2022.923925
57. LB E, Ismailova A, Dimeloe S, Hewison M, White JH. Vitamin D and immune regulation: antibacterial, antiviral, anti-inflammatory. JBMR Plus. (2021) 5:e10405. doi: 10.1002/jbm4.10405
58. Zughaier SM, Lubberts E, Bener A. Editorial: immune-modulatory effects of vitamin D. Front Immunol. (2020) 11:596611. doi: 10.3389/fimmu.2020.596611
59. Atkinson MA, Roep BO, Posgai A, Wheeler DCS, Peakman M. The challenge of modulating beta-cell autoimmunity in type 1 diabetes. Lancet Diabetes Endocrinol. (2019) 7:52–64. doi: 10.1016/S2213-8587(18)30112-8
60. Lai X, Liu X, Cai X, Zou FA-O. Vitamin D supplementation induces CatG-mediated CD4(+) T cell inactivation and restores pancreatic β-cell function in mice with type 1 diabetes. Am J Physiol Endocrinol Metab. (2021) 322:E74–84. doi: 10.1152/ajpendo.00066.2021. (1522-1555 (Electronic)).
61. García-Carrasco M, Mendoza-Pinto C, Munguía-Realpozo P, Etchegaray-Morales I, Vélez-Pelcastre SK, Méndez-Martínez S, et al. Insulin resistance and diabetes mellitus in patients with systemic lupus erythematosus. Endocr Metab Immune Disord Drug Targets. (2023) 23:503–14. doi: 10.2174/1871530322666220908154253. (2212-38736).
62. Zhao M, Duan XH, Wu ZZ, Gao CC, Wang N, Zheng ZH. Severe vitamin D deficiency affects the expression of autophagy related genes in PBMCs and T-cell subsets in active systemic lupus erythematosus. Am J Clin Exp Immunol. (2017) 6:43–51. (2164-7712 (Print)).
63. Abdel Galil SM, El-Shafey AM, Abdul-Maksoud RS, El-Boshy M. Interferon alpha gene expression and serum level association with low vitamin D levels in Egyptian female patients with systemic lupus erythematosus. Lupus. (2017) 27:199–209. doi: 10.1177/0961203317716321. (1477-0962 (Electronic)).
64. Shahin D, El-Farahaty RM, Houssen ME, Machaly SA, Sallam M, ElSaid TO, et al. Serum 25-OH vitamin D level in treatment-naïve systemic lupus erythematosus patients: Relation to disease activity, IL-23 and IL-17. Lupus. (2017) 26:917–26. doi: 10.1177/0961203316682095. (1477-0962 (Electronic)).
65. Harrison SR, Li D, Jeffery LE, Raza K, Hewison M. Vitamin D, autoimmune disease and rheumatoid arthritis. Calcif Tissue Int. (2020) 106:58–75. doi: 10.1007/s00223-019-00577-2
66. Chauss D, Freiwald T, McGregor R, Yan B, Wang L, Nova-Lamperti E, et al. Autocrine vitamin D signaling switches off pro-inflammatory programs of T(H)1 cells. Nat Immunol. (2022) 23:62–74. doi: 10.1038/s41590-021-01080-3
67. Oh J, Riek AE, Bauerle KT, Dusso A, McNerney KP, Barve RA, et al. Embryonic vitamin D deficiency programs hematopoietic stem cells to induce type 2 diabetes. Nat Commun. (2023) 14:3278. doi: 10.1038/s41467-023-38849-z
68. Zhang Y, Leung DY, Richers BN, Liu Y, Remigio LK, Riches DW, et al. Vitamin D inhibits monocyte/macrophage proinflammatory cytokine production by targeting MAPK phosphatase-1. J Immunol. (2012) 188:2127–35. doi: 10.4049/jimmunol.1102412
69. Fathi F, Sadek KM, Khafaga AF, Al Senosy AW, Ghoniem HA, Fayez S, et al. Vitamin D regulates insulin and ameliorates apoptosis and oxidative stress in pancreatic tissues of rats with streptozotocin-induced diabetes. Environ Sci pollut Res Int. (2022) 29:90219–29. doi: 10.1007/s11356-022-22064-2
70. Nakhoul NA-O, Thawko T, Farber E, Dahan I, Tadmor H, Nakhoul R, et al. The therapeutic effect of active vitamin D supplementation in preventing the progression of diabetic nephropathy in a diabetic mouse model. J Diabetes Res. (2020) 2020:7907605. doi: 10.1155/2020/7907605. (2314-6753 (Electronic)).
71. Hong SH, Kim YB, Choi HS, Jeong TD, Kim JT, Sung YA. Association of vitamin D deficiency with diabetic nephropathy. Endocrinol Metab. (2020) 36(1):106–13. doi: 10.3803/EnM.2020.826. (2093-5978 (Electronic)).
72. Deng X, Cheng J, Shen M. Vitamin D improves diabetic nephropathy in rats by inhibiting renin and relieving oxidative stress. J Endocrinol Invest. (2016) 39:657–66. doi: 10.1007/s40618-015-0414-4
73. Li YC. Podocytes as target of vitamin D. Curr Diabetes Rep. (2011) 7:35–40. doi: 10.2174/157339911794273964. (1875-6417 (Electronic)).
74. Doorenbos CR, van den Born J, Navis G, de Borst MH. Possible renoprotection by vitamin D in chronic renal disease: beyond mineral metabolism. Nat Rev Nephrol. (2009) 5:691–700. doi: 10.1038/nrneph.2009.185
75. Nwosu BA-O, Parajuli S, Jasmin G, Fleshman J, Sharma RB, Alonso LA-O, et al. Ergocalciferol in new-onset type 1 diabetes: A randomized controlled trial. J Endocr Soc. (2021) 6:bvab179. doi: 10.1210/jendso/bvab179. (2472-1972 (Electronic)).
76. Habibian N, Amoli MM, Abbasi F, Rabbani A, Alipour A, Sayarifard F, et al. Role of vitamin D and vitamin D receptor gene polymorphisms on residual beta cell function in children with type 1 diabetes mellitus. Pharmacol Rep. (2019) 71:282–8. doi: 10.1016/j.pharep.2018.12.012
77. Nam HK, Rhie YJ, Lee KH. Vitamin D level and gene polymorphisms in Korean children with type 1 diabetes. Pediatr Diabetes. (2019) 20:750–8. doi: 10.1111/pedi.12878
78. Roizen JD, Long C, Casella A, O'Lear L, Caplan I, Lai M, et al. Obesity decreases hepatic 25-hydroxylase activity causing low serum 25-hydroxyvitamin D. J Bone Miner Res. (2019) 34:1068–73. doi: 10.1002/jbmr.3686
79. Voutsadakis IA-O. Vitamin D receptor (VDR) and metabolizing enzymes CYP27B1 and CYP24A1 in breast cancer. Mol Biol Rep. (2020) 47:9821–30. doi: 10.1007/s11033-020-05780-1. (1573-4978 (Electronic)).
80. Tangjittipokin W, Umjai P, Khemaprasit K, Charoentawornpanich P, Chanprasert C, Teerawattanapong N, et al. Vitamin D pathway gene polymorphisms, vitamin D level, and cytokines in children with type 1 diabetes. Gene. (2021) 791:145691. doi: 10.1016/j.gene.2021.145691. (1879-0038 (Electronic)).
81. Almeida JA-O, Rodrigues D, Guimarães J, Lemos MA-O. Vitamin D pathway genetic variation and type 1 diabetes: A case-control association study. Genes (Basel). (2020) 11:897. doi: 10.3390/genes11080897. (2073-4425 (Electronic)).
82. Jiang X, O'Reilly PF, Aschard H, Hsu YH, Richards JB, Dupuis J, et al. Genome-wide association study in 79,366 European-ancestry individuals informs the genetic architecture of 25-hydroxyvitamin D levels. Nat Commun. (2018) 9:260. doi: 10.1038/s41467-017-02662-2
83. Zeng Q, Zou D, Wei Y, Ouyang Y, Lao Z, Guo R. Association of vitamin D receptor gene rs739837 polymorphism with type 2 diabetes and gestational diabetes mellitus susceptibility: a systematic review and meta-analysis. Eur J Med Res. (2022) 27:65. doi: 10.1186/s40001-022-00688-x
84. Mo M, Shao B, Xin X, Luo W, Si S, Jiang W, et al. The association of gene variants in the vitamin D metabolic pathway and its interaction with vitamin D on gestational diabetes mellitus: A prospective cohort study. Nutrients. (2021) 13:4220. doi: 10.3390/nu13124220. (2072-6643 (Electronic)).
Keywords: vitamin D, diabetes mellitus, diabetic complications, insulin resistance, pathogenesis
Citation: Li C, Fu J, Ye Y, Li J, He Y and Fang T (2024) The impact of vitamin D on the etiopathogenesis and the progression of type 1 and type 2 diabetes in children and adults. Front. Endocrinol. 15:1360525. doi: 10.3389/fendo.2024.1360525
Received: 23 December 2023; Accepted: 25 March 2024;
Published: 08 April 2024.
Edited by:
Mohammed S. Razzaque, Lake Erie College of Osteopathic Medicine, United StatesReviewed by:
Pradeep Kumar Dabla, G B Pant Institute of Postgraduate Medical Education and Research (GIPMER), IndiaBenjamin Udoka Nwosu, Hofstra University, United States
Copyright © 2024 Li, Fu, Ye, Li, He and Fang. This is an open-access article distributed under the terms of the Creative Commons Attribution License (CC BY). The use, distribution or reproduction in other forums is permitted, provided the original author(s) and the copyright owner(s) are credited and that the original publication in this journal is cited, in accordance with accepted academic practice. No use, distribution or reproduction is permitted which does not comply with these terms.
*Correspondence: Tuanyu Fang, ZmFuZ3R1YW55dV8yMDEwQDE2My5jb20=; Yangli He, MTY2OTQxMzUxOEBxcS5jb20=
†These authors share first authorship