- 1Department of Endocrinology and Metabolic Diseases, Polish Mother’s Memorial Hospital – Research Institute, Lodz, Poland
- 2Department of Paediatric Endocrinology, Medical University of Lodz, Lodz, Poland
Introduction: Sirtuin 1 (SIRT1) is known to be involved in sensing cellular energy levels and regulating energy metabolism. This study aimed to evaluate fasting serum SIRT1 levels in healthy children, and to analyse the influence of age, sex, puberty, body weight, height, and diet on its concentration.
Methods: 47 healthy children aged 4-14 with weight and height within normal range and no chronic disease were included into the study. Fasting serum SIRT1 concentrations were estimated by Enzyme Linked Immunosorbent Assay (ELISA).
Results: Results showed that serum SIRT1 concentrations in healthy children did not differ with respect to sex, age, height, weight and puberty. Whereas, it appeared that a higher frequency of fruits, vegetables and dairy products consumption was associated with an increase in serum SIRT1 levels.
Discussion: Studying SIRT1 in the context of children’s health may have implications for a broader understanding of growth processes, pubertal development, metabolic disorders and nutrition.
1 Introduction
The sirtuins are a family of nicotinamide adenine dinucleotide (NAD+)-dependent deacylases that regulate many cellular processes (1). Among the seven currently known types of sirtuins, sirtuin 1 (SIRT1) is involved in the cellular signal transduction and metabolism, DNA repair, inflammation as well as regulation of cellular senescence and aging (2–11). Despite numerous experimental studies on the importance of SIRT1 in the human body, there is very limited data on the level of blood concentration of this protein in humans. It should be noted that SIRT1 is directly engaged in intracellular GH signal transmission for IGF-1 secretion via modulation of JAK2/STAT pathway, also in the regulation of GH release in the central nervous system, as well as growth-plate chondrogenesis and longitudinal bone growth (12–15). Thus, it can be assumed that the concentration of SIRT1 should vary in dependence on age and growth rate in children.
On the other hand, it is known that changes in SIRT1 concentration affects the function of the hunger and satiety center, and the use of sirtuin activators promotes weight loss in obese individuals (16). Whereas in a fasting state, caloric restriction or malnutrition, SIRT1 intensifies catabolic processes and inhibits anabolic processes to maintain homeostasis (17–19). SIRT1 is also involved in regulating puberty (20). Moreover, there are some natural substances contained in daily consumed foods that act as sirtuin triggers (21–24). Thus, its concentration also probably varies depending on the body mass, stage of puberty, as well as diet.
The aim of this study was to evaluate fasting serum SIRT1 concentration in healthy children, and to analyse the influence of age, sex, body height, body mass and stage of puberty, as well as dietary habits and type and amount of nutrients intake.
2 Materials and methods
2.1 Study group
From among the children admitted to the Polish Mother Memorial Hospital - Research Institute in Lodz, Poland, the study group included those children who did not have any known healthy problems and who did meet the inclusion criteria and did not meet the exclusion criteria.
Inclusion criteria:
aged: 4-16 years, height and weight in the reference range (3rd-97th percentile for age and sex based on local percentile charts), completing a nutritional questionnaire, written consent of the legal representative to participate in the study.
Exclusion criteria:
chronic health problems which may influence the results (e.g. chronic diseases of the gastrointestinal tract, respiratory system, circulatory system, endocrine system, anorexia nervosa, undernutrition, obesity, short stature, excessive height, genetic disorders and syndromes), acute illness, no written consent of the legal representative to participate in the study.
Finally, 47 children were enrolled into the study group.
2.2 Auxological assessment
In each child: a detailed medical history was collected and a physical examination was performed. Height and weight measurements were taken in the morning on the day of hospital admission by the clinicians involved in this study (AF or RS). Children’s height was measured with an accuracy of 1 mm using a Harpenden stadiometer. Children were measured without shoes, with their heads in the Frankfort plane and their feet together. The measurement was performed three times and the average value was taken. Body weight was measured using an electronic scale, with an accuracy of 100 grams. During the measurement, the child was in underwear. Based on results of body height and weight, the standard deviation scores (SDS) in relation to the reference values for age and sex were calculated: for height - height standard deviation score (height SDS) and for body mass - weight SDS. Also, the body mass index (BMI) was calculated and expressed as BMI SDS with respect to the reference values for age and sex for Polish population (25, 26). The puberty stage was assessed according to Tanner scale (27).
2.3 Laboratory methods
The blood samples were taken in fasting state in the morning, to measure the routinely determined basic biochemical parameters as well as the concentrations of IGF-1 and IGFBP-3. An additional blood sample (2.4 ml) was taken in fasting state in the morning, for the determination of SIRT1 serum concentration. After collection, the blood was centrifuged to obtain serum. The serum with no signs of haemolysis was then frozen and stored at the appropriate temperature (see below), as required by the test manufacturer, until SIRT1 analysis.
All measurements were performed at the Centre for Medical Laboratory Diagnostics and Screening of the Polish Mother’s Memorial Hospital – Research Institute in Lodz, Poland.
SIRT1 concentration was determined by double-binding immunoenzymatic assay (ELISA) using 2 Human NAD-dependent deacetylase Sirtuin-1 (SIRT1/SIR2L1) ELISA Kits (Cusabio, Houston, TX, USA), according to the manufacturer’s instructions (User Manual; catalogue number: CSB-E15058h). The concentration of each sample was measured in duplicate. The sensitivity of the assay is 0.03 ng/ml, while the manufacturer’s guaranteed detection range of the assay is 0.15 ng/ml - 10 ng/ml, with an intra-assay coefficient of variation of less than 8% and an inter-assay coefficient of variation of less than 10%.
IGF-1 and IGFBP-3 concentrations were assessed using Immulite, DPC assays. For IGF-1, the WHO NIBSC 1st IRR 87/518 standard was used, with an analytical sensitivity of 20 ng/mL, a calibration ranges up to 1600 ng/mL, an intra-assay coefficient of variation: 3.1-4.3% and inter-assay coefficient of variation CV: 5,8-8,4%. The assay to assess IGFBP-3 was calibrated to the WHO NIBSC Reagent 93/560 standard, with an analytical sensitivity of 0.02 μg/mL, a calibration ranges up to 426 μg/mL, an intra-assay coefficient of variation of 3.5-5.6%, and an inter-assay coefficient of variation of 7.5-9.9%. IGF-1 concentration was expressed in terms of standard deviation for sex and age (IGF-I SDS), according to reference data (28). The molar ratio of IGF-1/IGFBP-3 was calculated assuming a molecular weight for IGF-1 of 7.5kDa and for IGFBP-3 of 42.0 kDa. The molar ratio of IGF-1 to IGFBP-3 is considered an indicator of the bioavailability of IGF-1 (29).
2.4 Assessment of children’s dietary habits and type and frequency of nutrients intake
The assessment of the children’s dietary habits was carried out through dietary survey with parent, wherein the frequency of their offspring’s consumption of particular food categories over the preceding month was examined. The survey was administered by a physician-researcher. The survey was developed based on the CoCu Questionnaire validated on a population of german children (30). After obtaining permission from the authors to use the questionnaire, it was translated bilaterally, and then both versions were checked for compatibility. The survey consisted of two parts. The first part contained 14 questions about the composition of the diet and the second part of the questionnaire contained questions about eating habits and food culture. The parent was asked to rate how many servings of various foods the child consumes per day (fruits or vegetables, unsweetened dairy products, sweetened dairy products, sweetened beverages, whole wheat bread, white bread) or per week (meat, fish, french fries, potatoes, rice or pasta, ready meals, pastries, sweet or salty snacks). Reference portions were described in the text or illustrated with photos. The selection of food items was largely based on the Food Frequency Questionnaire FFQ and the healthy eating pyramid (31, 32). The second part of the survey included questions about eating habits (i.e. whether they follow any specific diet, number of meals they have per day). The questionnaire was included in the Supplementary Materials.
2.5 Statistical analysis
Statistical analysis of the collected data was performed using STATISTICA ver. 13.3 software (Statsoft, Poland). The Shapiro-Wilk test was used to assess normality of distribution, and the Levene’s test was used to assess equality of variance. Comparative analysis was performed using non-parametric tests for independent variables. Non-parametric The Kruskal–Wallis test by ranks and Mann-Whitney U test were used for intergroup comparisons of quantitative continuous variables. Intergroup comparisons of nominal/qualitative variables were performed using the Chi-square test. In addition, a correlation analysis of the variables was performed (Pearson’s correlation coefficient). Continuous variables were presented median and interquartile ranges (median (Q1-Q3)) and range, categorical variables by N (%)). Statistically significant differences were taken as p-values below 0.05.
2.6 Ethics approval
Approval was obtained from the Bioethics Committee at the Polish Mother’s Memorial Hospital – Research Institute in Lodz (Opinion No. 47/2020).
2.7 Informed consent statement
The legal representatives of all patients gave their informed written consent to participate in the study prior to their inclusion in the study.
3 Results
3.1 Study group characteristics
There were 47 healthy children included in the study. Mean age of children 10.35 ± 2.6 years, 57,5% were male. Study group characteristics is presented in Table 1.
3.2 The analysis of serum SIRT1 concentration in healthy children in dependence on age, sex and stage of puberty
Serum SIRT1 concentration in healthy children ranged from 0.04 to 0.96 ng/ml. The mean SIRT1 concentration in healthy children was 0.29 ± 0.21 ng/ml (mean ± SD), while the median value (Q1-Q3) was 0.26 (0.14-0.38) ng/ml. The normality of the distribution of SIRT1 concentration was assessed - no normal distribution was found (Shapiro - Wilk test, p=0.0002, Figure 1).
There was no significant correlation between SIRT1 concentration and age of children (r=0.16), SIRT1 concentration and weight of children (r=0.11), SIRT1 concentration and weight SDS values (r=-0.05), SIRT1 concentration and height of children (r=0.13), as well as SIRT1 concentration and height SDS values (r=-0.01), SIRT1 concentration and their BMI (r=0.05), SIRT1 concentration and BMI SDS values (r=-0.04). We also did not find correlations between SIRT1 and IGF-1 concentrations (r=0.11), SIRT1 concentration and IGF-1 SDS value (r=0.08), SIRT1 and IGFBP-3 concentrations (r=0.12), as well as between SIRT1 concentration and IGF-1/IGFBP-3 molar ratio (r=0.06). The mentioned results are presented in Figure 2 and Supplementary Figure 1.
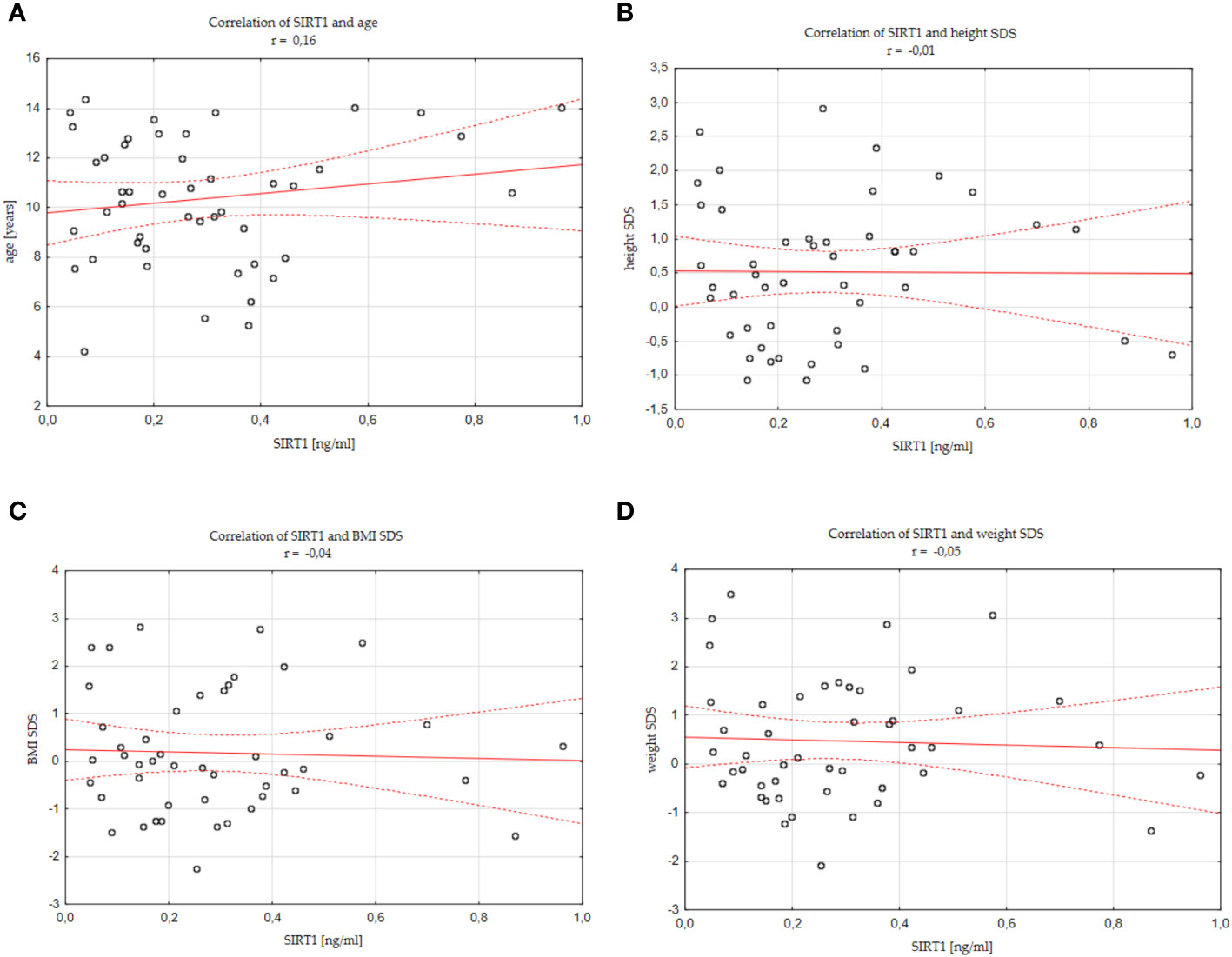
Figure 2 Correlations between SIRT1 concentration and individual analysed parameters (A) age, (B) height SDS, (C) BMI SDS, (D) weight SDS, standard deviation score; BMI, body mass index.
We also compared the SIRT1 concentration in individual subgroups of children, differentiated by gender (girls vs boys), age (younger than 10 years vs older or equal to 10 years old), stage of puberty (prepubertal vs pubertal), body weight and height (BMI SDS/hSDS greater or equal to 0 vs less than 0) and IGF1 concentration (IGF-1 SDS above or equal to 0 vs below 0). There were no statistical differences between SIRT 1 levels in the analysed subgroups (Table 2).
3.3 Dependence of SIRT1 concentration on the frequency of consumption of particular types of food
Based on the declared daily fruits and vegatables intake estimated in the overview survey described in Materials and methods, we found that children who consumed at least 4-5 portions of fruits or vegetables per day had significantly higher levels of SIRT1 than children who consumed less (0-3 portions per day) [0.41 (0.23 – 0.6) ng/ml vs 0.2 (0.1 – 0.34) ng/ml, p=0.02, Figure 3]. A tendency towards higher SIRT1 concentration was also found in the group of children eating at least 2-3 or more servings of fruits and vegetables, compared to those eating 0 or a maximum of 1 serving per day [0.29 (0.18 – 0.44) ng/ml vs 0.17 (0.14 – 0.3) ng/ml, p=0.068].
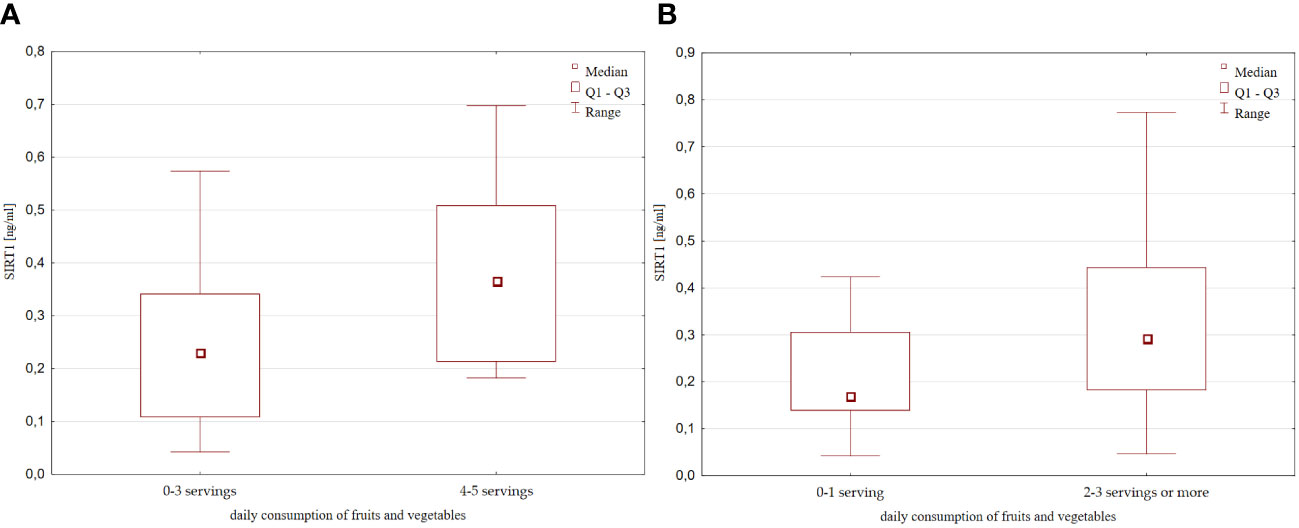
Figure 3 SIRT1 concentration with respect to the daily fruits and vegetable consumption (A) 0-3 servings vs 4-5 servings per day; (B) 0-1 serving vs 2-3 servings or more per day;.
Moreover, SIRT1 concentration increased with declared frequency of fruits and vegetables consumption (Figure 4).
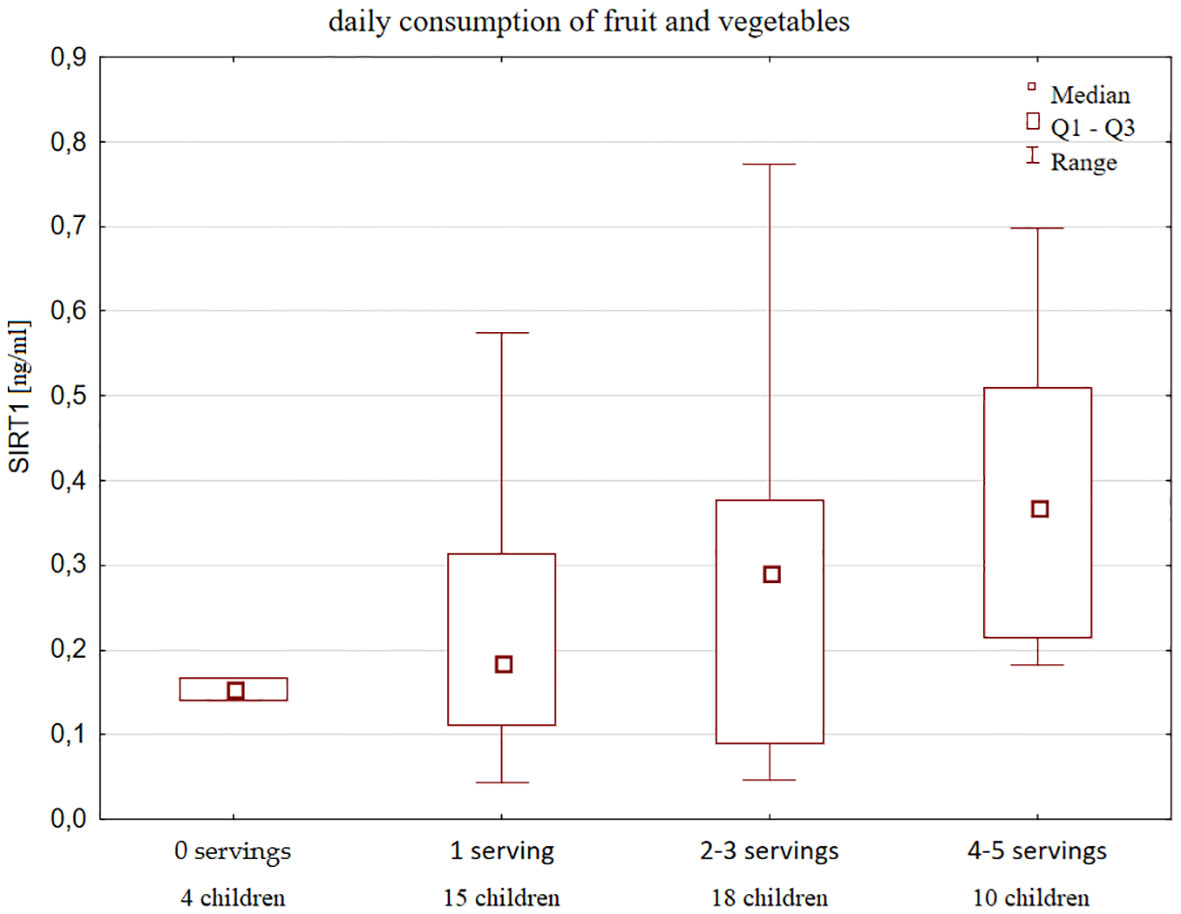
Figure 4 Serum SIRT1 concentration according to the declared frequency of fruits and vegetables intake in healthy children and a number of children in each group.
Although no correlation was observed between SIRT1 and IGF-1 levels in the whole analysed group, after dividing children into groups according to the amount of vegetables and fruits consumed, we found that children eating more fruits and vegetables (at least 2-3 servings per day), despite higher levels of SIRT1, had also significantly lower IGF-1 concentration ([149.6 (87.4 – 349.8) ng/ml vs 379 (156.2 – 502.3) ng/ml, p=0.01, Figure 5], and IGF-1 SDS values [-0.69 (-1.59 – 0.2) vs 0.37 (-0.4 – 0.68), p=0.006, Figure 5], as well as decreased IGF-1/IGFBP-3 molar ratio [0.22 (0.18 – 0.38) vs 0.4 (0.22 – 0.5), p=0.02]. Furthermore, those children were found to have lower BMI [16.64 (15 – 18.67) vs 18.24 (16.22 – 21.2), p=0.056, Figure 5] and BMI SDS [-0.39 (-0.92 – 0.31) vs 0.3 (-0.34 – 1.6), p=0.088, Figure 5]. Nevertheless, they did not differ with respect to height, gender and age.
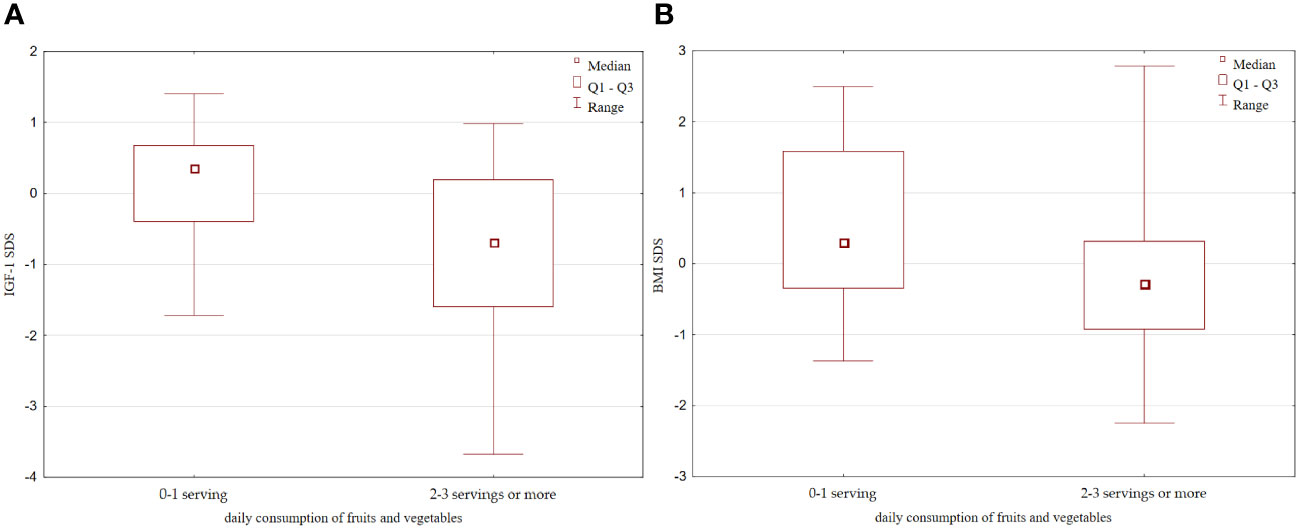
Figure 5 Differences in: (A) IGF-1 SDS; (B) BMI SDS with respect to the daily fruits and vegetables consumption. IGFF-1, Insulin-like growth factor 1; IGFBP-3, insulin-like growth factor binding protein-3.
SIRT1 concentration was also slightly but significantly higher in the group of children consuming unsweetened dairy products more frequently; that is, with consumption at least 2-3 times a day compared to consumption of 0-1 once a day [0.34 (0.2 – 0.54) ng/ml vs 0.18 (0.14 – 0.29) ng/ml, p=0.018, Figure 6]. There were no significant differences in height, weight and IGF-1 concentration with respect to the frequency of the consumption of dairy products.
No differences in SIRT1 levels were detected with respect to the consumption frequency of bread, rice, pasta, fish, meat, sweets and sugary drinks, nor in relation to a specific diet or a number of daily meals. Data on those individual food groups that were compared in the survey are included in the Supplementary Materials.
4 Discussion
SIRT1 is a protein that is primarily localized intracellularly (in a nucleus of liver, muscle, and white adipose tissue and in a cytoplasm of pancreatic and endothelial cells) (3, 5). It has been detected in the human adult serum (33) and its concentration appears to be altered in various disease states. SIRT1 serum reduction was shown in Alzheimer’s disease and mild cognitive impairment (33), obesity (34) and lung diseases (35), suggesting that serum SIRT1 may be a potential biomarker for various aging-associated diseases. On the other hand, increased serum SIRT1 levels were noticed in acute ischemic stroke (36), asthma (37) systemic lupus erythematosus (38) and frailty (39). To quantify SIRT1 levels in serum samples enzyme-linked immunosorbent assays (ELISA) technique was used most frequently.
To our knowledge, this study represents the first attempt to assess the serum concentration of SIRT1 in children and to explore the potential influencing factors. When compared to studies conducted in the adult population, the levels of SIRT1 observed in children within our study exhibited slightly lower concentration (35, 37, 40–43). There was one earlier study (44) focusing on SIRT1 levels in children in the context of growth. In this study SIRT1 levels were notably higher than the values reported in our observation. However, the authors of the cited report presented a small sample size with only male children and did not refer to other potential factors that may influence SIRT1 concentration in a population of healthy children (44). Nevertheless, the observed differences in serum concentration could be attributed to variations in the assay sources or the laboratory techniques (35, 37, 40–43).
It was proven that, SIRT1 play an important role in detection of cellular energy levels and regulation of energy metabolism and increase in response to fasting, caloric restriction and malnutrition (3). Several reports have shown that serum SIRT1 levels correlated negatively with BMI and were elevated in patients with anorexia nervosa (1, 45). It is well known that caloric restriction contributes to a state of growth hormone resistance and is associated with a decrease in serum IGF-1 levels, which is a main mediator of growth hormone (GH) action in peripheral tissues. SIRT1 is known to influence the process of intracellular GH signal transduction for IGF-1 synthesis (46). In situations of fasting or nutrient deficiencies, SIRT1 has been found to decrease the release of IGF-1 from the liver through the STAT5 pathway and enhance resistance to GH by promoting the release of GH from the pituitary gland (13, 47, 48). Furthermore, the presence of SIRT1 in the hypothalamus, particularly in neurons expressing the growth hormone receptor (GHR), and in chondrocytes, suggests potential relevance to the context of growth regulation (12, 14, 15, 49). It has been also suggested that SIRT1 regulate kisspeptin expression in the hypothalamus, affecting the timing of puberty onset (20). Therefore, it seemed to us that SIRT1 levels might vary depending on child height, or pubertal stage. However, we did not observe any significant differences in serum SIRT1 levels in relation to, age, height and IGF-1 levels as well as pubertal development. Although it has been found that SIRT1 levels decrease with age (50), in our paediatric cohort with a limited age range these differences may not be apparent.
As our results did not reflect associations between SIRT1 serum levels and height, weight or puberty, the intracellular function of SIRT1 may be defined by factors other than blood concentration. However, taking into account data from the literature, further investigation regarding SIRT1 involvement in growth disorders as well as weight and pubertal disturbances is warranted.
As SIRT1 is engaged in responding to metabolic imbalances, the amount of SIRT1 depends on the availability and type of nutrients (51). We assessed that SIRT1 serum levels were higher in children that consume more fruits and vegetables (at least 2-3 portions per day). Interestingly, those children were thinner and had lower IGF-1 serum levels, whereas their height was not affected the reduced IGF-1 serum levels may be related to sirtuins (46), but too many factors affect IGF-1 values to draw conclusions on this topic. Our outcomes are consistent with studies showing that sirtuin 1 may be activated by certain polyphenols, a class of naturally-occurring phytochemicals, which are compounds of some dietary products - fruits and vegetables in particular. The best known SIRT1 activator, resveratrol can be found in grapes, blueberries and grape products such as red wine (21, 23, 51–53). Piceatannol is a metabolite of resveratrol detected in grapes, passion fruit and white tea (23, 24). Quercetin, flavonoid polyphenol is present in fruits (peaches), vegetables (onions, garlic) and nuts (22, 54). Other dietary polyphenols that activates SIRT1 is fisetin, which can be found in apples, kiwi, dactyls, strawberries and blueberries among others (22, 53, 55). Some data suggest that besides polyphenols, dairy components may also serve as SIRT1 activators. Regarding our results, children with high consumption of dairy products had significantly increased SIRT1 serum concentration. Correspondingly, in vitro study in muscle and adipose cells indicate that systemic effects of high dairy feeding resulted in increased SIRT1 gene expression and activity in those cells (56). In addition, leucine, which is present in dairy food was proven to increase SIRT1 expression (57). Despite studies indicate that both natural and synthetic sirtuin activating compounds increase SIRT1 activity in vivo, the precise mechanism by which they activate SIRT1 remains unclear (58, 59). According to data from the literature resveratrol and others sirtuin 1 activators have demonstrated promising outcomes in a wide range of age-related diseases including obesity, diabetes, inflammation, cardiovascular disease, among others (60–64). Huang et al. summarized the current experience regarding resveratrol treatment in people with obesity, finding a significant improvement in metabolic complications and body weight reduction (65). A favourable effect of resveratrol has also been shown in certain diseases in children, such as ADHD or muscular dystrophies (66, 67).
Sirtfood is a novel food concept, according to which compounds from diet can affect sirtuins (53). Sirtfoods are said to induce a calorie restriction state and reduce nutrient consumption without causing malnutrition (68). The vast majority of data show that foods containing sirtuins (Sirtfoods) may produce pleiotropic, beneficial effects on health, alleviating metabolic disorders (53). Formulating a dietary regimen that integrates sirtuin-activating components derived from both the Asian and Mediterranean diets presents a potentially efficacious strategy in the prevention of chronic diseases. This approach holds promise for fostering health and supporting healthy aging (69). However, low bioavailability and rapid metabolism of polyphenols are issues that need to be scientifically addressed (70, 71). Further research is needed to evaluate dietary impact on sirtuin 1 and Sirtfoods-associated clinical implications.
Despite our study is limited by a small sample size, it is important to highlight that it represents the first investigation focusing on assessing SIRT1 serum levels in children which may offer valuable insights into the role of SIRT1 in pediatric physiology and its potential relevance to various aspects of child health. The preliminary findings presented here may serve as a basis for further research in this area and contribute to our understanding of SIRT1 implications in childhood development and disease, with an emphasis on growth and nutrition. Future studies with larger sample sizes and a wider age range are warranted to validate and expand upon the findings of this pioneering investigation.
5 Conclusions/highlights
1. Serum sirtuin 1 concentrations in healthy children did not differ with respect to sex, age, pubertal development, axiological parameters and IGF-1 levels.
2. Higher frequency of fruits, vegetables and dairy products consumption appeared to increase serum sirtuin 1 levels.
Data availability statement
The raw data supporting the conclusions of this article will be made available by the authors, without undue reservation.
Ethics statement
The studies involving humans were approved by Bioethics Committee at the Polish Mother’s Memorial Hospital – Research Institute in Lodz (Opinion No. 47/2020). The studies were conducted in accordance with the local legislation and institutional requirements. Written informed consent for participation in this study was provided by the participants’ legal guardians/next of kin.
Author contributions
AF: Conceptualization, Data curation, Funding acquisition, Investigation, Methodology, Project administration, Resources, Software, Validation, Visualization, Writing – original draft, Writing – review & editing. AL: Supervision, Writing – review & editing. RS: Conceptualization, Formal analysis, Funding acquisition, Investigation, Methodology, Project administration, Supervision, Validation, Visualization, Writing – review & editing.
Funding
The author(s) declare financial support was received for the research, authorship, and/or publication of this article. Study was funded by the Polish Mother’s Memorial Hospital – Research Institute Internal Grant No. 15GW/2021 financed by the Ministry of Science and Higher Education subsidy.
Conflict of interest
The authors declare that the research was conducted in the absence of any commercial or financial relationships that could be construed as a potential conflict of interest.
Publisher’s note
All claims expressed in this article are solely those of the authors and do not necessarily represent those of their affiliated organizations, or those of the publisher, the editors and the reviewers. Any product that may be evaluated in this article, or claim that may be made by its manufacturer, is not guaranteed or endorsed by the publisher.
Supplementary material
The Supplementary Material for this article can be found online at: https://www.frontiersin.org/articles/10.3389/fendo.2024.1356612/full#supplementary-material
References
1. Haigis MC, Sinclair DA. Mammalian sirtuins: b iological insights and disease relevance. Annu Rev Pathol: Mech Dis. (2010) 5:253–95. doi: 10.1146/annurev.pathol.4.110807.092250
2. Bonkowski MS, Sinclair DA. Slowing ageing by design: the rise of NAD+ and sirtuin-activating compounds. Nat Rev Mol Cell Biol. (2016) 17:679–90. doi: 10.1038/nrm.2016.93
3. Nogueiras R, Habegger KM, Chaudhary N, Finan B, Banks AS, Dietrich MO, et al. SIRTUIN 1 AND SIRTUIN 3: PHYSIOLOGICAL MODULATORS OF METABOLISM. Physiol Rev. (2012) 92:1479–514. doi: 10.1152/physrev.00022.2011
4. Hwang Jw, Yao H, Caito S, Sundar IK, Rahman I. Redox regulation of SIRT1 in inflammation and cellular senescence. Free Radic Biol Med. (2013) 61:95–110. doi: 10.1016/j.freeradbiomed.2013.03.015
5. Xie L, Huang R, Liu S, Wu W, Su A, Li R, et al. A positive feedback loop of SIRT1 and miR17HG promotes the repair of DNA double-stranded breaks. Cell Cycle. (2019) 18:2110–23. doi: 10.1080/15384101.2019.1641388
6. Alves-Fernandes DK, Jasiulionis MG. The role of SIRT1 on DNA damage response and epigenetic alterations in cancer. Int J Mol Sci. (2019) 20:3153. doi: 10.3390/ijms20133153
7. Kauppinen A, Suuronen T, Ojala J, Kaarniranta K, Salminen A. Antagonistic crosstalk between NF-κB and SIRT1 in the regulation of inflammation and metabolic disorders. Cell Signal. (2013) 25:1939–48. doi: 10.1016/j.cellsig.2013.06.007
8. Pang J, Xiong H, Ou Y, Yang H, Xu Y, Chen S, et al. SIRT1 protects cochlear hair cell and delays age-related hearing loss via autophagy. Neurobiol Aging. (2019) 80:127–37. doi: 10.1016/j.neurobiolaging.2019.04.003
9. Zhou S, Tang X, Chen HZ. Sirtuins and insulin resistance. Front Endocrinol (Lausanne). (2018) 9:748. doi: 10.3389/fendo.2018.00748
10. Yang Y, Liu Y, Wang Y, Chao Y, Zhang J, Jia Y, et al. Regulation of SIRT1 and its roles in inflammation. Front Immunol. (2022) 13:831168. doi: 10.3389/fimmu.2022.831168
11. Kitada M, Ogura Y, Monno I, Koya D. Sirtuins and type 2 diabetes: role in inflammation, oxidative stress, and mitochondrial function. Front Endocrinol (Lausanne). (2019) 10:187. doi: 10.3389/fendo.2019.00187
12. Kang X, Yang W, Wang R, Xie T, Li H, Feng D, et al. Sirtuin-1 (SIRT1) stimulates growth-plate chondrogenesis by attenuating the PERK-eIF-2α-CHOP pathway in the unfolded protein response. J Biol Chem. (2018) 293:8614–25. doi: 10.1074/jbc.M117.809822
13. Yamamoto M, Iguchi G, Fukuoka H, Suda K, Bando H, Takahashi M, et al. SIRT1 regulates adaptive response of the growth hormone–insulin-like growth factor-I axis under fasting conditions in liver. Proc Natl Acad Sci USA. (2013) 110:14948–53. doi: 10.1073/pnas.1220606110
14. de Lima JBM, Ubah C, Debarba LK, Ayyar I, Didyuk O, Sadagurski M. Hypothalamic GHR-SIRT1 axis in fasting. Cells. (2021) 10:891. doi: 10.3390/cells10040891
15. Satoh A, Brace CS, Ben-Josef G, West T, Wozniak DF, Holtzman DM, et al. SIRT1 promotes the central adaptive response to diet restriction through activation of the dorsomedial and lateral nuclei of the hypothalamus. J Neurosci. (2010) 30:10220–32. doi: 10.1523/JNEUROSCI.1385-10.2010
16. Ramadori G, Fujikawa T, Fukuda M, Anderson J, Morgan DA, Mostoslavsky R, et al. SIRT1 deacetylase in POMC neurons is required for homeostatic defenses against diet-induced obesity. Cell Metab. (2010) 12:78–87. doi: 10.1016/j.cmet.2010.05.010
17. Opstad TB, Sundfør T, Tonstad S, Seljeflot I. Effect of intermittent and continuous caloric restriction on Sirtuin1 concentration depends on sex and body mass index. Nutr Metab Cardiovasc Dis. (2021) 31:1871–8. doi: 10.1016/j.numecd.2021.03.005
18. Gillum MP, Erion DM, Shulman GI. Sirtuin-1 regulation of mammalian metabolism. Trends Mol Med. (2011) 17:8–13. doi: 10.1016/j.molmed.2010.09.005
19. Bordone L, Guarente L. Calorie restriction, SIRT1 and metabolism: understanding longevity. Nat Rev Mol Cell Biol. (2005) 6:298–305. doi: 10.1038/nrm1616
20. Vazquez MJ, Toro CA, Castellano JM, Ruiz-Pino F, Roa J, Beiroa D, et al. SIRT1 mediates obesity- and nutrient-dependent perturbation of pubertal timing by epigenetically controlling Kiss1 expression. Nat Commun. (2018) 9:4194. doi: 10.1038/s41467-018-06459-9
21. Gertz M, Nguyen GTT, Fischer F, Suenkel B, Schlicker C, Fränzel B, et al. A molecular mechanism for direct sirtuin activation by resveratrol. PloS One. (2012) 7:e49761. doi: 10.1371/journal.pone.0049761
22. Iside C, Scafuro M, Nebbioso A, Altucci L. SIRT1 activation by natural phytochemicals: an overview. Front Pharmacol. (2020) 11:1225. doi: 10.3389/fphar.2020.01225
23. Dai H, Sinclair DA, Ellis JL, Steegborn C. Sirtuin activators and inhibitors: Promises, achievements, and challenges. Pharmacol Ther. (2018) 188:140–54. doi: 10.1016/j.pharmthera.2018.03.004
24. Piotrowska H, Kucinska M, Murias M. Biological activity of piceatannol: Leaving the shadow of resveratrol. Mutat Res/Reviews Mutat Res. (2012) 750:60–82. doi: 10.1016/j.mrrev.2011.11.001
25. Kułaga Z, Grajda A, Gurzkowska B, Góźdź M, Wojtyło M, Swiąder A, et al. Polish 2012 growth references for preschool children. Eur J Pediatr. (2013) 172:753–61. doi: 10.1007/s00431-013-1954-2
26. Kułaga Z, Litwin M, Tkaczyk M, Palczewska I, Zajączkowska M, Zwolińska D, et al. Polish 2010 growth references for school-aged children and adolescents. Eur J Pediatr. (2011) 170:599–609. doi: 10.1007/s00431-010-1329-x
27. Tanner JM, Whitehouse RH. Clinical longitudinal standards for height, weight, height velocity, weight velocity, and stages of puberty. Arch Dis Child. (1976) 51:170–9. doi: 10.1136/adc.51.3.170
28. Elmlinger MW, Kühnel W, Weber MM, Ranke MB. Reference ranges for two automated chemiluminescent assays for serum insulin-like growth factor I (IGF-I) and IGF-binding protein 3 (IGFBP-3). Clin Chem Lab Med. (2004) 42:654–64. doi: 10.1515/CCLM.2004.112
29. Haj-Ahmad LM, Mahmoud MM, Sweis NWG, Bsisu I, Alghrabli AM, Ibrahim AM, et al. Serum IGF-1 to IGFBP-3 molar ratio: A promising diagnostic tool for growth hormone deficiency in children. J Clin Endocrinol Metab. (2023) 108(4):986–94. doi: 10.1210/clinem/dgac609
30. Poulain T, Spielau U, Vogel M, Körner A, Kiess W. CoCu: A new short questionnaire to evaluate diet composition and culture of eating in children and adolescents. Clin Nutr. (2019) 38:2858–65. doi: 10.1016/j.clnu.2018.12.020
31. Jarosz M. Nowa Piramida Zdrowego Żywienia i Stylu Życia Dzieci i Młodzieży. Żyw Człow Meta. (2019) 46(1):13–4. Available at: https://ncez.pzh.gov.pl/dzieci-i-mlodziez/piramida-zdrowego-zywienia-i-stylu-zycia-dzieci-i-mlodziezy-2/.
32. Kowalkowska J, Wadolowska L, Hamulka J, Wojtas N, Czlapka-Matyasik M, Kozirok W, et al. Reproducibility of a short-form, multicomponent dietary questionnaire to assess food frequency consumption, nutrition knowledge, and lifestyle (SF-FFQ4PolishChildren) in Polish children and adolescents. Nutrients. (2019) 11:2929. doi: 10.3390/nu11122929
33. Kumar R, Chaterjee P, Sharma PK, Singh AK, Gupta A, Gill K, et al. Sirtuin1: A promising serum protein marker for early detection of Alzheimer’s disease. PloS One. (2013) 8:e61560. doi: 10.1371/journal.pone.0061560
34. Mariani S, Fiore D, Persichetti A, Basciani S, Lubrano C, Poggiogalle E, et al. Circulating SIRT1 increases after intragastric balloon fat loss in obese patients. Obes Surg. (2016) 26:1215–20. doi: 10.1007/s11695-015-1859-4
35. Yanagisawa S, Papaioannou AI, Papaporfyriou A, Baker JR, Vuppusetty C, Loukides S, et al. Decreased serum sirtuin-1 in COPD. Chest. (2017) 152:343–52. doi: 10.1016/j.chest.2017.05.004
36. Liu Y, Jia S, Liang X, Dong M, Xu X, Lu C, et al. Prognostic value of Sirtuin1 in acute ischemic stroke and its correlation with functional outcomes. Med (Baltimore). (2018) 97:e12959. doi: 10.1097/MD.0000000000012959
37. Wang Y, Li D, Ma G, Li W, Wu J, Lai T, et al. Increases in peripheral SIRT1: a new biological characteristic of asthma. Respirol. (2015) 20:1066–72. doi: 10.1111/resp.12558
38. Yang C, Li R, Xu WD, Huang AF. Increased levels of sirtuin-1 in systemic lupus erythematosus. Int J Rheumatic Dis. (2022) 25:869–76. doi: 10.1111/1756-185X.14360
39. Ma L, Niu H, Sha G, Zhang Y, Liu P, Li Y. Serum SIRT1 is associated with frailty and adipokines in older adults. J Nutrition Health Aging. (2019) 23:246–50. doi: 10.1007/s12603-018-1149-7
40. Gonçalinho GHF, Nascimento JR de O, Mioto BM, Amato RV, Moretti MA, Strunz CMC, et al. Effects of coffee on sirtuin-1, homocysteine, and cholesterol of healthy adults: does the coffee powder matter? J Clin Med. (2022) 11:2985. doi: 10.3390/jcm11112985
41. Charążka B, Siejka A. Correlations between serum sirtuin levels and cardiovascular risk factors in women with polycystic ovary syndrome. Adv Med Sci. (2022) 67:123–8. doi: 10.1016/j.advms.2022.01.004
42. Ozkan Kurtgoz P, Karakose S, Cetinkaya CD, Erkus E, Guney I. Evaluation of sirtuin 1 (SIRT1) levels in autosomal dominant polycystic kidney disease. Int Urol Nephrol. (2022) 54:131–5. doi: 10.1007/s11255-021-02862-2
43. Mansur AP, Roggerio A, Goes MFS, Avakian SD, Leal DP, Maranhão RC, et al. Serum concentrations and gene expression of sirtuin 1 in healthy and slightly overweight subjects after caloric restriction or resveratrol supplementation: A randomized trial. Int J Cardiol. (2017) 227:788–94. doi: 10.1016/j.ijcard.2016.10.058
44. Kaplan DS, Canak A, Isık E, Orkmez M, Kumru B. Relationship of fibroblast growth factor 21, sirtuin 1, visfatin, and regulators in children with short stature. Growth Factors. (2018) 36:172–7. doi: 10.1080/08977194.2018.1513504
45. Amerio A, Escelsior A, Martino E, Strangio A, Aguglia A, Marcatili M, et al. The association between blood SIRT1 and ghrelin, leptin, and antibody anti-hypothalamus: A comparison in normal weight and anorexia nervosa. J Pers Med. (2023) 13:928. doi: 10.3390/jpm13060928
46. Fedorczak A, Lewiński A, Stawerska R. Involvement of sirtuin 1 in the growth hormone/insulin-like growth factor 1 signal transduction and its impact on growth processes in children. Int J Mol Sci. (2023) 24:15406. doi: 10.3390/ijms242015406
47. Fazeli PK, Klibanski A. Determinants of GH resistance in malnutrition. J Endocrinol. (2014) 220:R57–65. doi: 10.1530/JOE-13-0477
48. Wójcik M, Krawczyńska A, Antushevich H, Herman AP. Post-receptor inhibitors of the GHR-JAK2-STAT pathway in the growth hormone signal transduction. Int J Mol Sci. (2018) 19(7):1843. doi: 10.3390/ijms19071843
49. Yamamoto M, Takahashi Y. The essential role of SIRT1 in hypothalamic-pituitary axis. Front Endocrinol (Lausanne). (2018) 9:605. doi: 10.3389/fendo.2018.00605
50. Zhong Y, Chen AF, Zhao J, Gu YJ, Fu GX. Serum levels of cathepsin D, sirtuin1, and endothelial nitric oxide synthase are correlatively reduced in elderly healthy people. Aging Clin Exp Res. (2016) 28:641–5. doi: 10.1007/s40520-015-0472-7
51. Howitz KT, Bitterman KJ, Cohen HY, Lamming DW, Lavu S, Wood JG, et al. Small molecule activators of sirtuins extend Saccharomyces cerevisiae lifespan. Nature. (2003) 425:191–6. doi: 10.1038/nature01960
52. Malaguarnera L. Influence of resveratrol on the immune response. Nutrients. (2019) 11:946. doi: 10.3390/nu11050946
53. Akan OD, Qin D, Guo T, Lin Q, Luo F. Sirtfoods: new concept foods, functions, and mechanisms. Foods. (2022) 11:2955. doi: 10.3390/foods11192955
54. Cui Z, Zhao X, Amevor FK, Du X, Wang Y, Li D, et al. Therapeutic application of quercetin in aging-related diseases: SIRT1 as a potential mechanism. Front Immunol. (2022) 13:943321. doi: 10.3389/fimmu.2022.943321
55. Khan N, Syed DN, Ahmad N, Mukhtar H. Fisetin: a dietary antioxidant for health promotion. Antioxid Redox Signal. (2013) 19:151–62. doi: 10.1089/ars.2012.4901
56. Bruckbauer A, Zemel MB. Effects of dairy consumption on SIRT1 and mitochondrial biogenesis in adipocytes and muscle cells. Nutr Metab (Lond). (2011) 8:91. doi: 10.1186/1743-7075-8-91
57. Li H, Xu M, Lee J, He C, Xie Z. Leucine supplementation increases SIRT1 expression and prevents mitochondrial dysfunction and metabolic disorders in high-fat diet-induced obese mice. Am J Physiol Endocrinol Metab. (2012) 303:E1234–44. doi: 10.1152/ajpendo.00198.2012
58. Hubbard BP, Sinclair DA. Small molecule SIRT1 activators for the treatment of aging and age-related diseases. Trends Pharmacol Sci. (2014) 35:146–54. doi: 10.1016/j.tips.2013.12.004
59. Huang Y, Lu J, Zhan L, Wang M, Shi R, Yuan X, et al. Resveratrol-induced Sirt1 phosphorylation by LKB1 mediates mitochondrial metabolism. J Biol Chem. (2021) 297:100929. doi: 10.1016/j.jbc.2021.100929
60. Milne JC, Lambert PD, Schenk S, Carney DP, Smith JJ, Gagne DJ, et al. Small molecule activators of SIRT1 as therapeutics for the treatment of type 2 diabetes. Nature. (2007) 450:712–6. doi: 10.1038/nature06261
61. García-Martínez BI, Ruiz-Ramos M, Pedraza-Chaverri J, Santiago-Osorio E, Mendoza-Núñez VM. Effect of resveratrol on markers of oxidative stress and sirtuin 1 in elderly adults with type 2 diabetes. Int J Mol Sci. (2023) 24:7422. doi: 10.3390/ijms24087422
62. Li H, Xia N, Hasselwander S, Daiber A. Resveratrol and vascular function. Int J Mol Sci. (2019) 20:2155. doi: 10.3390/ijms20092155
63. Najjar RS, Feresin RG. Protective role of polyphenols in heart failure: molecular targets and cellular mechanisms underlying their therapeutic potential. Int J Mol Sci. (2021) 22:1668. doi: 10.3390/ijms22041668
64. Chen J, Lou R, Zhou F, Li D, Peng C, Lin L. Sirtuins: Key players in obesity-associated adipose tissue remodeling. Front Immunol. (2022) 13:1068986. doi: 10.3389/fimmu.2022.1068986
65. Huang H, Chen G, Liao D, Zhu Y, Pu R, Xue X. The effects of resveratrol intervention on risk markers of cardiovascular health in overweight and obese subjects: a pooled analysis of randomized controlled trials. Obes Rev. (2016) 17:1329–40. doi: 10.1111/obr.12458
66. Kawamura K, Fukumura S, Nikaido K, Tachi N, Kozuka N, Seino T, et al. Resveratrol improves motor function in patients with muscular dystrophies: an open-label, single-arm, phase IIa study. Sci Rep. (2020) 10:20585. doi: 10.1038/s41598-020-77197-6
67. Rafeiy-Torghabeh M, Ashraf-Ganjouei A, Moradi K, Bagheri S, Mohammadi MR, Akhondzadeh S. Resveratrol adjunct to methylphenidate improves symptoms of attention-deficit/hyperactivity disorder: a randomized, double-blinded, placebo-controlled clinical trial. Eur Child Adolesc Psychiatry. (2021) 30:799–807. doi: 10.1007/s00787-020-01562-z
68. Lilja S, Bäck H, Duszka K, Hippe B, Suarez L, Höfinger I, et al. Fasting and fasting mimetic supplementation address sirtuin expression, miRNA and microbiota composition. Funct Foods Health Dis. (2020) 10:439. doi: 10.31989/ffhd.v10i10.752
69. Pallauf K, Giller K, Huebbe P, Rimbach G. Nutrition and healthy ageing: calorie restriction or polyphenol-rich ‘MediterrAsian’ diet? Oxid Med Cell Longev. (2013) 2013:707421. doi: 10.1155/2013/707421
70. Cueva C, Silva M, Pinillos I, Bartolomé B, Moreno-Arribas MV. Interplay between dietary polyphenols and oral and gut microbiota in the development of colorectal cancer. Nutrients. (2020) 12:625. doi: 10.3390/nu12030625
Keywords: sirtuin 1, growth, puberty, IGF-1, diet, healthy children
Citation: Fedorczak A, Lewiński A and Stawerska R (2024) Sirtuin 1 serum concentration in healthy children - dependence on sex, age, stage of puberty, body weight and diet. Front. Endocrinol. 15:1356612. doi: 10.3389/fendo.2024.1356612
Received: 15 December 2023; Accepted: 21 February 2024;
Published: 11 March 2024.
Edited by:
Giulio Maltoni, IRCCS University Hospital of Bologna Sant Orsola Polyclinic, ItalyReviewed by:
Gerdi Tuli, Regina Margherita Hospital, ItalyAgnieszka Domin, University of Rzeszow, Poland
Copyright © 2024 Fedorczak, Lewiński and Stawerska. This is an open-access article distributed under the terms of the Creative Commons Attribution License (CC BY). The use, distribution or reproduction in other forums is permitted, provided the original author(s) and the copyright owner(s) are credited and that the original publication in this journal is cited, in accordance with accepted academic practice. No use, distribution or reproduction is permitted which does not comply with these terms.
*Correspondence: Renata Stawerska, renata.stawerska@icloud.com