- Department of Endocrinology, Metabolism and Internal Medicine, Poznan University of Medical Sciences, Poznan, Poland
Dyslipidemia is one of the most common disorders worldwide, which, if left untreated, results in a multitude of complications. Thus proper diagnostics, which includes identifying of secondary causes of dyslipidemia is crucial. Endocrine disorders are an important cause of secondary dyslipidemia. This paper aims to review the publications on lipoprotein alterations in endocrine disorders from the past two years and provide an overview of the recent discoveries in this dynamically developing and large field. Significant changes in lipoprotein serum concentrations are present in most endocrinological diseases and can be modified with proper treatment. Some lipoproteins have also been proposed as markers in some endocrine diseases, e.g., thyroid carcinoma. From the scope of endocrine disorders, the largest number of studies explored the lipoprotein changes in polycystic ovary syndrome and in women during the menopausal and peri-menopausal period. Even though the association of thyroid disorders with dyslipidemia is already well studied, new research has delivered some exciting findings about lipoprotein alterations in euthyroid patients with either positive antithyroid peroxidase antibodies or reduced sensitivity to thyroid hormones. The problem of the adverse metabolic profile, including dyslipidemia in hypoprolactinemia has been recognized. Moreover, this review describes other significant discoveries encompassing lipoprotein alterations in disorders of the adrenals, thyroid, parathyroid glands, pituitary, and gonads. The up-to-date knowledge of the influence of endocrine disorders and hormonal changes on serum lipoproteins is prudent as it can significantly impact therapeutic decisions.
1 Introduction
Dyslipidemia is defined as an increase in any of the serum lipids (triglycerides, cholesterol, cholesterol esters, phospholipids, lipoproteins) or a decrease in serum high-density lipoprotein (1). It is one of the most common disorders affecting humankind. In 2008, the World Health Organization (WHO) estimated that around 39% of adults worldwide suffer from raised cholesterol levels. The prevalence was the highest in the European Region (54%) and both Americas (48%) (2). Dyslipidemia results in a multitude of complications, especially related to cardiovascular disease: coronary artery disease, peripheral artery disease, ischemic stroke, hypertension, aneurysms, and many more. Cardiovascular-related diseases are the leading cause of death (3). Thus, identifying and treating dyslipidemia is crucial. A standard serum lipid profile usually consists of total cholesterol (TC), low-density cholesterol (LDL-C), high-density cholesterol (HDL-C), and triglycerides (TG). It can be further expanded by the addition of more specialized tests, e.g., intermediate-density lipoproteins (IDL), very low-density lipoproteins (VLDL-C), apolipoprotein A1 (Apo A1), apolipoprotein B (Apo B), lipoprotein (a) [Lp (a)]. Most of the aforementioned particles are proatherogenic and deleterious to human health. However, two of them, namely Apo A1 and HDL-C, are generally considered antiatherogenic. The 2019 European Society of Cardiology emphasizes the importance of Apo B measurement as the Apo B containing lipoproteins have a central causal role in atherosclerosis development (4). The disease is usually caused by a sedentary lifestyle, lack of exercise, wrong diet (excess of calories, high saturated and trans fats intake, alcohol, etc), and genetic factors. However, changes in serum lipoproteins can often be observed in many endocrine disorders. Thus, knowing the associations between different endocrinological diseases and lipid metabolism is prudent. Most commonly, changes in serum lipoproteins occur secondary to the endocrine disorder. However, primary changes in serum lipoproteins may sometimes influence the course of endocrinological diseases. Lipid profile alterations associated with endocrine disorders are generally unfavorable and proatherogenic. Even though in some conditions, e.g., overt hyperthyroidism, a decrease in deleterious lipoproteins like low-density lipoprotein cholesterol (LDL-C) is seen, it cannot be viewed as unequivocally positive since the disease itself is associated with an increased cardiovascular risk (5). The problem of dyslipoproteinemia in endocrinological conditions is immense and would require a very long review. This paper aims to review the recent publications from the last two years on the role of lipoproteins in endocrine disorders and provide an overview of the new discoveries and current research topics in this dynamically developing field in a concise form. The summary of the most important hormonal and lipoprotein changes in selected endocrine disorders mentioned in this review is presented in Figure 1.
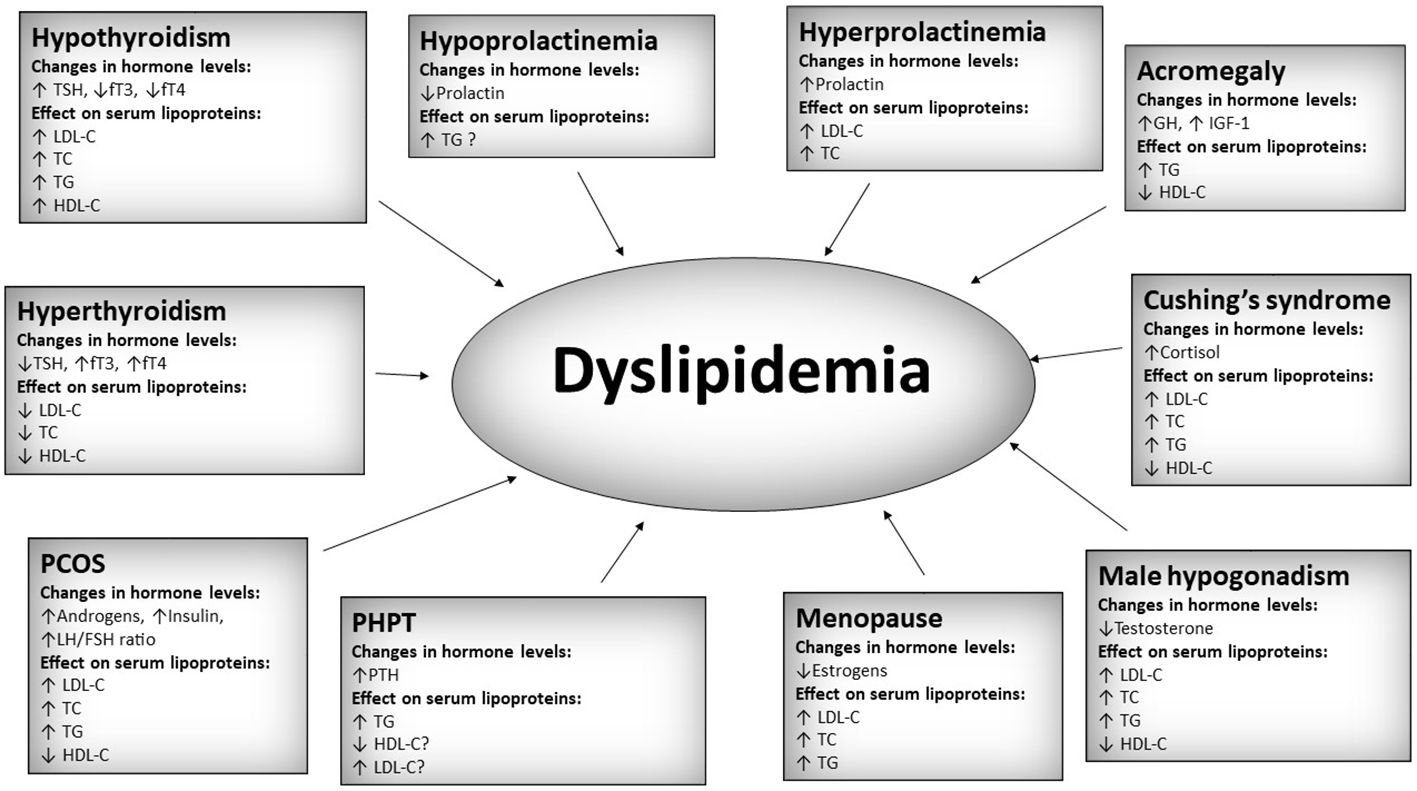
Figure 1 Summary of most important hormonal and lipoprotein changes in selected endocrine disorders. TSH, thyroid stimulating hormone; fT3, free triiodothyronine; fT4, free thyroxine; TC, total cholesterol; LDL-C, low-density cholesterol; HDL-C, high-density cholesterol; TG, triglycerides; PCOS, polycystic ovary syndrome; PHPT, primary hyperparathyroidism; PTH, parathormone; GH, growth hormone; IGF-1, insulin-like growth factor 1. A question mark “?” was added in cases of relatively low amount of data.
2 Thyroid
2.1 Hypothyroidism
Dyslipidemia is a very well-recognized manifestation of overt and subclinical hypothyroidism with thyroid stimulating hormone (TSH) >10 mIU/l. Hypothyroidism can be found in 1.4-13% of patients with hyperlipidemia (6). It is associated with increased TC, LDL-C, Lp (a), and sometimes decreased HDL-C levels (7). However, the data on mild subclinical hypothyroidism with TSH<10 mIU/l are equivocal. A meta-analysis of 35 studies delivered more data on this topic and has shown that even mild subclinical hypothyroidism is associated with an increase in TC, LDL-C, and TG, and a decrease in HDL-C. No differences were reported for VLDL-C, Apolipoprotein B, and Apolipoprotein A serum concentrations (8). Increased anti-thyroid peroxidase antibodies (anti-TPO) are the hallmark of Hashimoto’s thyroiditis diagnosis. Anti-TPO positivity in subclinical hypothyroidism is associated with a higher degree of dyslipidemia. In a cross-sectional study from India, patients with subclinical hypothyroidism and positive anti-TPO had significantly higher TC, LDL-C, and TG levels when compared to antibody-negative subclinical hypothyroid cases (9). Similar relationships were seen in euthyroid subjects with Hashimoto’s thyroiditis, who had higher TC, LDL-C, HDL-C, and TG than healthy euthyroid controls. In this patient group, anti-TPO correlated positively with total cholesterol, LDL-C, and TG (10). However, in another study, the prevalence of anti-TPO in euthyroid subjects was associated only with the presence of low HDL-C without the impact on other lipoprotein serum concentrations (11). The results of the studies mentioned above highlight the need for dyslipidemia screening among patients with anti-TPO positivity. Interestingly, some patients who are euthyroid and don’t have a history of thyroid disease might still be at risk of developing dyslipidemia due to impaired sensitivity to thyroid hormones. A study on 3573 euthyroid participants has shown that reduced thyroid hormone sensitivity is associated with metabolic syndrome and some alterations in lipoproteins, mainly TG (12).
Diagnosing subclinical hypothyroidism using age-adjusted TSH reference values in elderly patients is widely debated. Even though TSH levels are associated with TC, TG, and LDL-C levels in the geriatric population, the relationship varies in different age groups. TSH correlates positively with TC, TG, and LDL-C in the 71-80 age group, with TC in the 65-70 age group, but there was no significant correlation between any lipoproteins and TSH in the >80 years group (13). The impact of TSH on serum lipoproteins seems to be weaker with aging, which further supports the use of age-adjusted TSH reference ranges, at least in the eldest patient groups. However, other conclusions could be drawn from the latest research on Apolipoprotein E (ApoE), a lipoprotein that takes part in various lipid metabolism processes. One of its isoforms, ApoE4, is associated with Alzheimer’s disease. A study by Zhang et al. has found that exosomal transport of liver-derived ApoE4, which causes cognitive decline, is induced by aging-related hypothyroidism and that thyroid hormone levels correlate inversely with the exosomal ApoE4 content. Moreover, in this study, the treatment with levothyroxine has reduced the ApoE4 content of liver and serum exosomes compared with untreated subjects in a mice model (14). The study results are intriguing, but it is yet to be established in further studies if it can prevent cognitive decline.
2.2 Hyperthyroidism
Hyperthyroidism is associated with decreased TC, LDL-C, Lp (a), Apo B, and HDL-C serum concentration (7). It is prudent to remember that despite lower levels of the majority of proatherogenic lipid particles, hyperthyroidism is still associated with an increased cardiovascular risk (15). The treatment of overt hyperthyroidism is associated with a reversal of the observed changes – mainly an increase in TC, LDL-C, and HDL-C. However, the impact of subclinical hyperthyroidism treatment on lipid metabolism is uncertain. According to recent studies, definitive subclinical hyperthyroidism therapy is associated foremost with an increase in LDL-C, while the effect on other lipoproteins remains small or uncertain (16, 17). Still, the increase in LDL-C, which is a significant atherosclerosis factor, should make clinicians vigilant of the need for lipid profile check-ups in such patients. Amiodarone-induced hyperthyroidism is one of the most problematic adverse events, which often limits the use of this drug in cardiac patients. Recently, machine learning models, which can predict the occurrence of amiodarone-induced hyperthyroidism, were described. This study found that the most important baseline risk factors for amiodarone-induced thyroid dysfunction were higher HDL-C, TSH, lower free thyroxine(FT4), alkaline phosphatase, and LDL-C, and shorter duration of treatment (18). Such tools are still far from being used in routine clinical practice, but they could significantly improve patients’ safety if available.
2.3 Thyroid cancer
Thyroid cancer itself is not associated directly with alternations in serum lipoprotein levels. However, serum lipoprotein levels are routinely evaluated in patients with thyroid cancer after thyroidectomy because of the hormone imbalances that can occur after the surgery. However, recent research suggests they can also be used as a marker for thyroid cancer risk. A study, which contained data from over 3 million participants from health checkups provided by the Korean National Health Insurance Service, has reported that repeatedly measured low HDL-C levels are a risk factor for thyroid cancer. The hazard ratios for thyroid cancer occurrence were 1.243, 1.404, 1.486, and 1.680 for each subsequent low HDL-C measurement. The link of this association was the strongest in patients with metabolic disease, e.g., central obesity, high blood pressure, impaired fasting glucose, and hyperlipidemia (19). The monocyte-to-high-density lipoprotein ratio (MHR) is an inflammation and oxidative stress marker. A recent paper has discovered that patients with papillary thyroid carcinoma (PTC) have higher MHR values. However, the diagnostic accuracy of MHR in diagnosing PTC was borderline acceptable (20). MHR is a very cheap and accessible parameter and could be an interesting adjunctive tool in thyroid nodule workup in the future. However, more research must be performed.
3 Primary hyperparathyroidism
Primary hyperparathyroidism is associated with an increased risk of cardiovascular disease. There are some reports about the association of primary hyperparathyroidism with dyslipidemia, mainly consisting of increased TG and, in some populations, also, higher LDL-C and lower HDL-C serum concentrations. However, the data is somewhat inconclusive at this moment (21–24). A recent meta-analysis of 34 studies assessed if parathyroidectomy can modify cardiometabolic risk factors. Unfortunately, no change was found in total cholesterol, LDL, HDL, or triglycerides after surgery. However, the procedure decreased other parameters like glucose, insulin, and blood pressure values (25).
4 Polycystic ovary syndrome
Polycystic ovary syndrome (PCOS) is known to be associated with metabolic syndrome and dyslipidemia. All sorts of detrimental lipid profile changes have been reported in PCOS. The lipid profile of PCOS patients is characterized by higher TG, LDL-C, non-HDL-C, and lower HDL-C (26). Recent research on the Chinese population has found that dyslipidemia is present in 41.3% of patients with PCOS and is predominately characterized by low HDL-C. Interestingly, patients with different clinical features of PCOS were found to have different alterations in their lipid profiles. Patients with clinically apparent hyperandrogenism had decreased HDL-C and Apo A serum concentrations. Patients with insulin resistance had increased TG, LDL, Apo B, TG/LDL, and Apo B/Apo A ratios. Patients with dysglycemia or type 2 diabetes had increased TC and TG levels (27). The Apo B/Apo A1 ratio is an interesting parameter, which in PCOS patients is positively associated with the prevalence of metabolic syndrome, its components, aminotransferase elevation, insulin resistance, and correlates with androgen levels (28). Another typical lipoprotein alteration in PCOS is an increased level of lipoprotein (a) compared to healthy controls, which has been recently confirmed by a meta-analysis of 23 studies (29). Dyslipidemia in PCOS is not only associated with increased cardiovascular risk but also with the risk of adverse pregnancy-related outcomes. A recent study by Cai et al. on PCOS women who underwent ovulation stimulation shows that increased total TC, LDL-C, TG, and Apo B are associated with a lower chance of clinical pregnancy and live birth, with total cholesterol, LDL-C, and Apo B is also related to the risk of miscarriage. From the above-mentioned lipid profile parameters, high levels of Apo B seem to be the most important, with odds ratios for pregnancy, live birth, and miscarriage standing at 0.34, 0.39, and 3.82, respectively (30). However, another study that examined 1470 women with PCOS who underwent their first cycle of in vitro fertilization/intracytoplasmic sperm injection reported no differences in cumulative pregnancy outcomes among the dyslipidemia and the control groups, with the exception of a negative association between total cholesterol serum concentrations and the cumulative live birth rate (31).
Currently, the standard first-line therapy in PCOS is combined oral contraceptives(COC), with anti-androgen medication being usually the second-line therapy. The efficacy of anti-androgens in PCOS has been evaluated in a large meta-analysis, which has shown that combination therapy with COC and anti-androgens results in a worsened lipid profile with higher TC, LDL-C, and TG (32). The addition of letrozole (an aromatase inhibitor) to ethinylestradiol and cyproterone acetate tablets can result in the improvement of a multitude of hormonal and metabolic parameters when compared to ethinylestradiol and cyproterone acetate therapy alone. The observed lipoprotein changes included lower TC, LDL-C, TG, and higher HDL-C levels. Notably, such treatment was not associated with any differences in the number of adverse events (33).
Natural medicine therapies and nutraceuticals are getting increasing attention in PCOS. In a double-blind, randomized, placebo-controlled trial, the supplementation of crocin resulted in a significant increase in HDL-C and stabilized cholesterol, LDL, and TG levels, which rose during the follow-up period in the placebo group (34). Fenugreek is another option for plan-based treatment for women with PCOS. Two recent studies showed that a Fenugreek seed extract has a beneficial effect on serum lipoproteins, causing a small but significant increase of HDL-C after 12 weeks of therapy in one study (35) and a decrease in TC, LDL-C, and TG when compared to placebo in another trial (36). The benefits of propolis administration in PCOS have been examined by a recent randomized, triple-blinded, placebo-controlled trial. The use of Propolis was associated with reducing the LDL-C to HDL-C ratio (37). A meta-analysis of the three so far published randomized controlled trials about the effects of resveratrol treatment in PCOS did not show any important benefit on serum lipoprotein concentrations apart from lowering total cholesterol levels (38). Even though the changes in serum lipoproteins caused by the use of nutraceuticals are mild or modest, some of those substances seem to be a beneficial and safe add-on to PCOS therapy.
Phthalates are esters of phthalic acid, which are used as plasticizers and belong to the group of endocrine disruptors. They also have an impact on serum lipoprotein levels in women with PCOS. The sum of phthalates in the urine correlates with LDL-C, TG, and TC to HDL-C ratio, while Mono-methyl-phthalate urine levels are associated with TC, LDL-C, TG, and TC to HDL-C ratio (39). Thus, exposure to phthalates should be avoided to minimize the degree of dyslipidemia in PCOS patients.
Idiopathic hirsutism is one of the differential diagnoses of PCOS and, besides PCOS, one of the most common causes of hirsutism in women. In a recent study on 334 patients with idiopathic hirsutism, Mahmoudieh et al. showed that contrary to PCOS, idiopathic hirsutism is not associated with any changes in serum lipoprotein levels or other metabolic risk factors (40).
5 Menopause
Estrogens have a crucial role in maintaining correct lipid metabolism. Thus, in the peri-menopausal and post-menopausal periods, when the serum concentrations of estrogens drop, dyslipidemia becomes more prevalent in women. Menopause is associated with higher lipoprotein levels, including higher TG, TC, and LDL-C. However, no difference in HDL-C is observed between pre- and postmenopausal women (41). A study by Karppinen et al. has examined changes in the circulating metabolome by targeted nuclear magnetic resonance metabolomics in women in the transition period from perimenopause to early menopause. In this Finnish cohort study, 85 significant changes in the metabolome were associated with menopause, of which 64 have been directly explained by the hormonal changes during menopause. In this study, the most prominent changes in the metabolome associated with menopause were a significant increase in Apo B, VLDL-C, triglycerides and particles, LDL-cholesterol and particles, and HDL triglycerides, glycerol, and leucine and a decrease in 3-hydroxybutyrate, and citrate serum concentrations. HRT was associated with increased medium-to-large HDL particle count and decreased small-to-medium LDL particle count (42).
A recent meta-analysis of 21 studies involving 1573 women with premature ovarian insufficiency showed that the disease is associated with an adverse lipid profile, consisting of increased total cholesterol, LDL-C, TG, and decreased HDL-C (43). Women who develop premature ovarian insufficiency transit into the postmenopausal period much earlier than healthy women and thus are exposed for a longer time to the proatherogenic changes related to estrogen deficiency. That is why early check-ups of the lipid profile and fast introduction of proper treatment are of utmost importance in those women.
Currently, hormone replacement therapy (HRT) is indicated for the alleviation of vasomotor symptoms of menopause. Research on the influence of HRT on cardiovascular risk is still ongoing, and some very interesting papers on this topic were published recently. A meta-analysis of 14 randomized controlled trials assessed the effect of HRT in the form of 17β-estradiol plus norethisterone acetate on serum lipoprotein (a) and apolipoproteins. The meta-analysis showed that such treatment causes a mean decrease of 67.59 mg/L in lipoprotein (a), of 3.71 mg/dl in Apo B, and has no effect on apolipoprotein A1 and A2 levels (44). A study on nearly 90,000 postmenopausal women recruited from the UK biobank has shown that increased lipoprotein (a) was associated with an increased risk of coronary heart disease. Moreover, it has been found, similarly to the previously described meta-analysis, that HRT decreases lipoprotein (a) levels. However, interestingly, no evidence of lower lipoprotein (a) associated risks was found between HRT users and nonusers (45). Correct timing of HRT and avoiding late HRT commencing might be crucial for preventing harmful changes in HDL-C in postmenopausal women.
6 Male hypogonadism
Hypogonadism is associated with an unfavorable lipid profile consisting of increased TC, LDL-C, TG and low HDL-C. However, a recent study on 7268 men participating in a health examination suggests that the association between testosterone and LDL-C is nonlinear. Instead, the study reports an inverted U-shaped association between the prevalence of testosterone <3.5ng/ml and the deciles of LDL-C, thus implying a higher risk of testosterone <3.5 ng/ml in patients with both high and low LDL-C levels. Moreover, a negative association of testosterone with the deciles of TG and a positive with the deciles of HDL-C is also shown (46). The results of this study suggest that not only high LDL-C but also low LDL-C serum concentrations, especially when combined with high TG and low HDL-C, should indicate screening for male hypogonadism.
In recent years, the awareness of testosterone replacement therapy(TRT) and its use in hypogonadal men has increased. There has been a long debate about whether TRT increases the cardiovascular risk in hypogonadal men. Fortunately, a recent multicenter, randomized, double-blind, placebo-controlled trial showed that TRT was non-inferior to placebo in the incidence of major adverse cardiac events (47). Moreover, a meta-analysis of 16 randomized controlled trials showed that TRT significantly lowers LDL-C levels. Still, the effect on HDL remains unclear due to the imprecision or heterogeneity of the analyzed studies (48). Not only testosterone but also gonadotrophins seem to be associated with lipoprotein levels. A recent paper reported that the follicle-stimulating hormone (FSH) in hypogonadal males with type 2 diabetes correlates with TC, LDL-C, and TG levels. Interestingly, this finding was independent of testosterone serum concentration (49). Thus, FSH might be a new marker of dyslipidemia in this patient group.
7 Adrenal disorders
It is long known that hypercortisolism is associated with an altered lipid profile. Dyslipidemia can be observed in Cushing’s syndrome of any etiology (pituitary, adrenal, ectopic). Cushing’s syndrome typically presents with increased TC, LDL-C, TG, and decreased HDL-C. Surgical or pharmacological treatment can normalize serum lipoprotein levels, but sometimes dyslipidemia persists even after resolution of hypercortisolemia (50). Less information is available about hyperaldosteronism. A meta-analysis of 30 studies examined the differences in serum lipoproteins between patients with primary hyperaldosteronism and essential hypertension. It has shown that patients with primary hyperaldosteronism have lower triglycerides, LDL-C, and TC levels compared to essential hypertension subjects (51). Even though this observation’s mechanism is unknown, the increased cardiovascular risk in primary aldosteronism patients seems to be unrelated to dyslipidemia.
Interestingly, even in patients with neither clinically apparent signs of adrenal disease nor a presence of an adrenal tumor, the morphology of the adrenal gland might affect its function and impact lipid metabolism. This was shown in a study on 588 diabetic patients without any comorbidities or medications affecting adrenal gland function. Researchers in this study measured adrenal limb thickness and found it to be inversely correlated with HDL-C (52).
8 Acromegaly and growth hormone deficiency
Acromegaly is associated with dyslipidemia development, which can occur in up to 74% of patients (53). The main lipoprotein changes observed in acromegaly include hypertriglyceridemia and reduced HDL-C serum concentrations (54). Recent studies have shown, that dyslipidemia improves upon somatostatin analogue treatment. Long-acting somatostatin analog treatment results in a decrease in TG to HDL-C ratio and Lp (a) and an increase in HDL-C. The reduction in mean growth hormone levels is positively associated with the decline in TG, Lp (a), and an increase in HDL-C (55). Pegvisomant is a growth hormone (GH) receptor antagonist that effectively lowers the insulin-like growth factor 1 (IGF-1) levels and improves symptom control in acromegaly. Even though previous studies did not find many alterations in serum lipoproteins after pegvisomant treatment, according to a recent study assessing the outcomes of a 10-year-long, continuous pegvisomant treatment, the use of this drug is associated with a decrease in TC and LDL-C. A combined therapy, consisting of pegvisomant with the addition of a somatostatin analog, has been shown to lower TC and LDL-C even stronger than pegvisomant monotherapy (56).
9 Hyperprolactinemia and hypoprolactynemia
Hyperprolactinemia is an established, well-known clinical entity. Lipid profile abnormalities in patients with prolactinomas usually are higher TC and LDL-C serum concentrations (57). However, the potential clinical significance of hypoprolactinemia has been recognized only recently. A recent study reported that women with iatrogenic hypoprolactinemia caused by cabergoline treatment have higher serum concentrations of CRP, fibrinogen, uric acid, HbA1C, 2-h post-challenge glucose, TG, and lower levels of testosterone, free androgen index and HDL-C. Fortunately, all those changes were reversible after cabergoline dose reduction and normalization of prolactin levels (58). The results of this study show that the goal of dopamine agonist treatment should be the achievement of normoprolactinemia, and overtreatment leading to hypoprolactinemia should be avoided.
10 Conclusions
The knowledge of lipoprotein alterations in endocrine disorders has developed further. Most of the recent research focused on thyroid disease, menopause, PCOS, and hypogonadism. The up-to-date knowledge of the influence of endocrine disorders and hormonal changes on serum lipoproteins is prudent as it can significantly impact therapeutic decisions.
Author contributions
MO: Conceptualization, Investigation, Writing – original draft, Writing – review & editing. ES: Conceptualization, Writing – review & editing. MR: Conceptualization, Writing – review & editing.
Funding
The author(s) declare that no financial support was received for the research, authorship, and/or publication of this article.
Conflict of interest
The authors declare that the research was conducted in the absence of any commercial or financial relationships that could be construed as a potential conflict of interest.
The author(s) declared that they were an editorial board member of Frontiers, at the time of submission. This had no impact on the peer review process and the final decision.
Publisher’s note
All claims expressed in this article are solely those of the authors and do not necessarily represent those of their affiliated organizations, or those of the publisher, the editors and the reviewers. Any product that may be evaluated in this article, or claim that may be made by its manufacturer, is not guaranteed or endorsed by the publisher.
References
1. Shattat GF. A review article on hyperlipidemia: types, treatments and new drug targets. BioMed Pharmacol J. (2015) 7:399–409. doi: 10.13005/bpj/504
2. World Health Organization. Global status report on noncommunicable diseases (2011). Available online at: https://iris.who.int/handle/10665/44579.
3. Mc Namara K, Alzubaidi H, Jackson JK. Cardiovascular disease as a leading cause of death: how are pharmacists getting involved? Integr Pharm Res Pract. (2019) 8:1–11. doi: 10.2147/IPRP
4. Mach F, Baigent C, Catapano AL, Koskinas KC, Casula M, Badimon L, et al. 2019 ESC/EAS Guidelines for the management of dyslipidaemias: lipid modification to reduce cardiovascular risk: The Task Force for the management of dyslipidaemias of the European Society of Cardiology (ESC) and European Atherosclerosis Society (EAS). Eur Heart J. (2020) 41(1):111–88.
5. Szczepanek-Parulska E, Sokolowski J, Dmowska D, Klimek J, Stasikowski T, Zdebski P, et al. Lipid profile abnormalities associated with endocrine disorders. Endokrynol Pol. (2022) 73(5):863–71. doi: 10.5603/EP.a2022.0059
6. Duntas LH, Brenta G. A renewed focus on the association between thyroid hormones and lipid metabolism. Front Endocrinol. (2018) 9:511. doi: 10.3389/fendo.2018.00511
7. Rizos CV, Elisaf MS, Liberopoulos EN. Effects of thyroid dysfunction on lipid profile. Open Cardiovasc Med J. (2011) 5:76–84. doi: 10.2174/1874192401105010076
8. Treister-Goltzman Y, Yarza S, Peleg R. Lipid profile in mild subclinical hypothyroidism: systematic review and meta-analysis. Minerva Endocrinol. (2021) 46:428–40. doi: 10.23736/S2724-6507.20.03197-1
9. Kumar M, Dheeraj D, Kant R, Kumar A. The association between anti-thyroid peroxidase antibody and dyslipidemia in subclinical hypothyroidism among the rural population of Central India. Cureus. (2022) 14:e22317. doi: 10.7759/cureus.22317
10. Cengiz H, Demirci T, Varim C, Tamer A. The effect of Thyroid Autoimmunity on Dyslipidemia in patients with Euthyroid Hashimoto Thyroiditis. Pak J Med Sci. (2021) 37:1365–70. doi: 10.12669/pjms.37.5.3883
11. Kim HJ, Park SJ, Park HK, Byun DW, Suh K, Yoo MH. Thyroid autoimmunity and metabolic syndrome: a nationwide population-based study. Eur J Endocrinol. (2021) 185:707–15. doi: 10.1530/EJE-21-0634
12. Lv F, Cai X, Li Y, Zhang X, Zhou X, Han X, et al. Sensitivity to thyroid hormone and risk of components of metabolic syndrome in a Chinese euthyroid population. J Diabetes. (2023) 15(10):900–10. doi: 10.1111/1753-0407.13441
13. Ni W, Zhang M, Wang X, Li X, Wang Q, Wang Y, et al. Age-specific serum thyrotropin reference range for the diagnosis of subclinical hypothyroidism and its association with lipid profiles in the elderly population. Sci Rep. (2022) 12:20872. doi: 10.1038/s41598-022-24182-w
14. Zhang M, Gong W, Zhang D, Ji M, Chen B, Chen B, et al. Ageing related thyroid deficiency increases brain-targeted transport of liver-derived ApoE4-laden exosomes leading to cognitive impairment. Cell Death Dis. (2022) 13:1–13. doi: 10.1038/s41419-022-04858-x
15. Iglesias P, Benavent M, López G, Arias J, Romero I, Díez JJ. Hyperthyroidism and cardiovascular disease: an association study using big data analytics. Endocrine. (2024) 83:405–13. doi: 10.1007/s12020-023-03482-9
16. Kamiński G, Olejarz M, Szczepanek-Parulska E, Ruchała M, Saracyn M. Changes of lipid profile in subclinical hyperthyroidism and following restoration of euthyroidism. Pol Arch Intern Med. (2022) 132:16277. doi: 10.20452/pamw.16277
17. Russo L, Nguyen TNH, Kyrilli A, Robin M, Lassen PB, Moreno-Reyes R, et al. Metabolic changes after radioiodine correction of grade 1 and grade 2 subclinical hyperthyroidism. Eur Thyroid J. (2021) 10:382–9. doi: 10.1159/000512734
18. Lu YT, Chao HJ, Chiang YC, Chen HY. Explainable machine learning techniques to predict amiodarone-induced thyroid dysfunction risk: multicenter, retrospective study with external validation. J Med Internet Res. (2023) 25:e43734. doi: 10.2196/43734
19. Kim J, Kim MK, Baek KH, Song KH, Han K, Kwon HS. Repeated low high-density lipoprotein cholesterol and the risk of thyroid cancer: A nationwide population- based study in Korea. Endocrinol Metab Seoul Korea. (2022) 37:303–11. doi: 10.3803/EnM.2021.1332
20. Xu H, Pang Y, Li X, Zha B, He T, Ding H. Monocyte to high-density lipoprotein cholesterol ratio as an independent risk factor for papillary thyroid carcinoma. J Clin Lab Anal. (2021) 35:e24014. doi: 10.1002/jcla.24014
21. Procopio M, Barale M, Bertaina S, Sigrist S, Mazzetti R, Loiacono M, et al. Cardiovascular risk and metabolic syndrome in primary hyperparathyroidism and their correlation to different clinical forms. Endocrine. (2014) 47:581–9. doi: 10.1007/s12020-013-0091-z
22. Luboshitzky R, Chertok-Schaham Y, Lavi I, Ishay A. Cardiovascular risk factors in primary hyperparathyroidism. J Endocrinol Invest. (2009) 32:317–21. doi: 10.1007/BF03345719
23. Bibik EE, Dobreva EA, Elfimova AR, Miliutina AP, Eremkina AK, Gorbacheva AM, et al. Primary hyperparathyroidism in young patients is associated with metabolic disorders: a prospective comparative study. BMC Endocr Disord. (2023) 23:57. doi: 10.1186/s12902-023-01302-9
24. Dobreva EA, Gorbacheva AM, Bibik EE, Eremkina AK, Elfimova AR, Salimkhanov R, et al. Cardiovascular and metabolic status in patients with primary hyperparathyroidism: a single-center experience. Front Endocrinol. (2023) 14:1266728. doi: 10.3389/fendo.2023.1266728
25. Yavari M, Feizi A, Haghighatdoost F, Ghaffari A, Rezvanian H. The influence of parathyroidectomy on cardiometabolic risk factors in patients with primary hyperparathyroidism: a systematic review and meta-analysis. Endocrine. (2021) 72:72–85. doi: 10.1007/s12020-020-02519-7
26. Wild RA, Rizzo M, Clifton S, Carmina E. Lipid levels in polycystic ovary syndrome: systematic review and meta-analysis. Fertil Steril. (2011) 95:1073–9. doi: 10.1016/j.fertnstert.2010.12.027
27. Guo F, Gong Z, Fernando T, Zhang L, Zhu X, Shi Y. The lipid profiles in different characteristics of women with PCOS and the interaction between dyslipidemia and metabolic disorder states: A retrospective study in Chinese population. Front Endocrinol. (2022) 13:892125. doi: 10.3389/fendo.2022.892125
28. He H, Feng J, Zhang S, Wang Y, Li J, Gao J, et al. The apolipoprotein B/A1 ratio is associated with metabolic syndrome components, insulin resistance, androgen hormones, and liver enzymes in women with polycystic ovary syndrome. Front Endocrinol. (2021) 12:773781. doi: 10.3389/fendo.2021.773781
29. Masson W, Barbagelata L, Lobo M, Lavalle-Cobo A, Corral P, Nogueira JP. Plasma lipoprotein(a) levels in polycystic ovary syndrome: A systematic review and meta-analysis. High Blood Press Cardiovasc Prev Off J Ital Soc Hypertens. (2023) 30:305–17. doi: 10.1007/s40292-023-00585-2
30. Cai WY, Luo X, Ma HL, Shao XG, Wu XK. Association between preconception serum lipid concentrations and treatment outcomes in women with PCOS who underwent ovulation induction. Reprod BioMed. (2022) 45:805–14. doi: 10.1016/j.rbmo.2022.04.013
31. Jiang X, Lu X, Cai M, Liu Y, Guo Y. Impact of dyslipidemia on the cumulative pregnancy outcomes after first ovarian stimulation. Front Endocrinol. (2022) 13. doi: 10.3389/fendo.2022.915424
32. Alesi S, Forslund M, Melin J, Romualdi D, Peña A, Tay CT, et al. Efficacy and safety of anti-androgens in the management of polycystic ovary syndrome: a systematic review and meta-analysis of randomised controlled trials. eClinicalMedicine. (2023) 63:102162. doi: 10.1016/j.eclinm.2023.102162
33. Lu K, Li N, Zhang M, Lin J, Chen J. Effects of letrozole combined with ethinylestradiol and cyproterone acetate tablets on serum sex hormones and lipid metabolism in patients with polycystic ovary syndrome. Am J Transl Res. (2023) 15:1271–80.
34. Rahimi G, Shams S, Aslani MR. Effects of crocin supplementation on inflammatory markers, lipid profiles, insulin and cardioprotective indices in women with PCOS: A randomized, double-blind, placebo-controlled trial. Phytother Res PTR. (2022) 36:2605–15. doi: 10.1002/ptr.7474
35. Sankhwar P, Jaiswar SP, Yadav S, Awasthi V, Goel A, Kumar P, et al. Beneficial effects of a novel fenugreek seed extract (Trigonella foenum-graecum, furocyst®) in women with polycystic ovary syndrome (PCOS): A follow-up compliance clinical investigation. J Am Nutr Assoc. (2022) 42:1–9. doi: 10.1080/27697061.2022.2145526
36. Singh A, Gainder S, Banerjee P, Goel A, Kumar P, Mondal B, et al. Efficacy of a proprietary fenugreek seed extract (Trigonella foenum-graecum, furocyst®) in women with polycystic ovary syndrome (PCOS): a randomized, double-blind, placebo-controlled study. J Am Nutr Assoc. (2022) 0:1–9. doi: 10.1080/27697061.2022.2126410
37. Abbasi E, Bagherniya M, Soleimani D, Ghasemi-Tehrani H, Abbaspour M, Clark CCT, et al. The effects of propolis supplementation on high-sensitivity C-reactive protein, testosterone hormone, and metabolic profile in women with polycystic ovary syndrome: A randomized, triple-blinded, placebo-controlled clinical trial. Phytother Res PTR. (2023) 37(11):5366–77. doi: 10.1002/ptr.7977
38. Larik MO, Ahmed A, Khan L, Iftekhar MA. Effects of resveratrol on polycystic ovarian syndrome: a systematic review and meta-analysis of randomized controlled trials. Endocrine. (2024) 83:51–9. doi: 10.1007/s12020-023-03479-4
39. Milankov A, Milanović M, Milošević N, Sudji J, Pejaković S, Milić N, et al. The effects of phthalate exposure on metabolic parameters in polycystic ovary syndrome. Clin Chim Acta Int J Clin Chem. (2023) 540:117225. doi: 10.1016/j.cca.2023.117225
40. Mahmoudieh L, Amiri M, Rahmati M, Habibi Moeini AS, Sarvghadi F, Azizi F, et al. Idiopathic hirsutism and metabolic status: A population-based prospective cohort study. J Clin Endocrinol Metab. (2022) 108:114–23. doi: 10.1210/clinem/dgac538
41. Ambikairajah A, Walsh E, Cherbuin N. Lipid profile differences during menopause: a review with meta-analysis. Menopause N Y N. (2019) 26:1327–33. doi: 10.1097/GME.0000000000001403
42. Karppinen JE, Törmäkangas T, Kujala UM, Sipilä S, Laukkanen J, Aukee P, et al. Menopause modulates the circulating metabolome: evidence from a prospective cohort study. Eur J Prev Cardiol. (2022) 29:1448–59. doi: 10.1093/eurjpc/zwac060
43. Cai WY, Luo X, Wu W, Song J, Xie NN, Duan C, et al. Metabolic differences in women with premature ovarian insufficiency: a systematic review and meta-analysis. J Ovarian Res. (2022) 15:109. doi: 10.1186/s13048-022-01041-w
44. Tang Z, Găman MA, Prabahar K, Mei H. The effect of 17β-estradiol plus norethisterone acetate treatment on lipoprotein (a), atherogenic and anti-atherogenic apolipoproteins levels in postmenopausal women: A meta-analysis of randomized controlled trials. Exp Gerontol. (2023) 172:112055. doi: 10.1016/j.exger.2022.112055
45. Honigberg MC, Trinder M, Natarajan P. Lipoprotein(a), menopausal hormone therapy, and risk of coronary heart disease in postmenopausal individuals. JAMA Cardiol. (2022) 7:565–8. doi: 10.1001/jamacardio.2022.0716
46. Lee JH, Jung HD, Choi JD, Kang JY, Yoo TK, Park YW. Non-linear association between testosterone and LDL concentrations in men. Andrology. (2023) 11:1107–13. doi: 10.1111/andr.13393
47. Lincoff AM, Bhasin S, Flevaris P, Mitchell LM, Basaria S, Boden WE, et al. Cardiovascular safety of testosterone-replacement therapy. N Engl J Med. (2023) 389:107–17. doi: 10.1056/NEJMoa2215025
48. Mangolim AS, Brito L de AR, Nunes-Nogueira VDS. Effectiveness of testosterone replacement in men with obesity: a systematic review and meta-analysis. Eur J Endocrinol. (2021) 186:123–35. doi: 10.1530/EJE-21-0473
49. Xu X, Li C, Diao H, Guo Y, Zhao Y, Zhao W, et al. Elevated circulating follicle stimulating hormone level is associated with dyslipidemia in male type 2 diabetes mellitus patients: A cross-sectional study. Diabetes Metab Res Rev. (2023) 40(1):e3718. doi: 10.1002/dmrr.3718
50. Pivonello R, Isidori AM, De Martino MC, Newell-Price J, Biller BMK, Colao A. Complications of Cushing’s syndrome: state of the art. Lancet Diabetes Endocrinol. (2016) 4:611–29. doi: 10.1016/S2213-8587(16)00086-3
51. Manosroi W, Phudphong P, Atthakomol P, Phimphilai M. The differences of serum lipid profiles between primary aldosteronism and essential hypertension: a meta-analysis and systematic review. BMC Endocr Disord. (2022) 22:217. doi: 10.1186/s12902-022-01135-y
52. Liu Y, Zou X, Zhao W, Yao X, Wang L, Zhou L, et al. Adrenal limb thickness is associated with metabolism profiles in patients with diabetes: A cross-sectional study. J Diabetes. (2023) 16(2):e13479. doi: 10.1111/1753-0407.13479
53. Rolla M, Jawiarczyk-Przybyłowska A, Halupczok-Żyła J, Kałużny M, Konopka BM, Błoniecka I, et al. Complications and comorbidities of acromegaly—Retrospective study in polish center. Front Endocrinol. (2021) 12:642131. doi: 10.3389/fendo.2021.642131
54. Gadelha MR, Kasuki L, Lim DST, Fleseriu M. Systemic complications of acromegaly and the impact of the current treatment landscape: an update. Endocr Rev. (2019) 40:268–332. doi: 10.1210/er.2018-00115
55. Shao XQ, Chen ZY, Wang M, Yang YP, Yu YF, Liu WJ, et al. Effects of long-acting somatostatin analogues on lipid metabolism in patients with newly diagnosed acromegaly: A retrospective study of 120 cases. Horm Metab Res Horm Stoffwechselforschung Horm Metab. (2022) 54:25–32. doi: 10.1055/a-1717-9332
56. Pirchio R, Auriemma RS, Montini ME, Vergura A, Pivonello R, Colao A. Control of acromegaly in more than 90% of patients after 10 years of pegvisomant therapy: an European referral centre real-life experience. J Endocrinol Invest. (2023) 46:1027. doi: 10.1007/s40618-022-01980-7
57. Glezer A, Santana MR, Bronstein MD, Donato J, Jallad RS. The interplay between prolactin and cardiovascular disease. Front Endocrinol. (2023) 13:1018090. doi: 10.3389/fendo.2022.1018090
Keywords: endocrine disorders, lipoproteins, HDL-cholesterol, LDL-cholesterol, triglycerides, lipoprotein (a), apolipoprotein B, apolipoprotein A
Citation: Olejarz M, Szczepanek-Parulska E and Ruchala M (2024) Lipoprotein alterations in endocrine disorders - a review of the recent developments in the field. Front. Endocrinol. 15:1354098. doi: 10.3389/fendo.2024.1354098
Received: 11 December 2023; Accepted: 19 March 2024;
Published: 02 April 2024.
Edited by:
Rajesh Katare, University of Otago, New ZealandReviewed by:
Piotr Glinicki, Centre of Postgraduate Medical Education, PolandCopyright © 2024 Olejarz, Szczepanek-Parulska and Ruchala. This is an open-access article distributed under the terms of the Creative Commons Attribution License (CC BY). The use, distribution or reproduction in other forums is permitted, provided the original author(s) and the copyright owner(s) are credited and that the original publication in this journal is cited, in accordance with accepted academic practice. No use, distribution or reproduction is permitted which does not comply with these terms.
*Correspondence: Michal Olejarz, bWwub2xlamFyekBnbWFpbC5jb20=