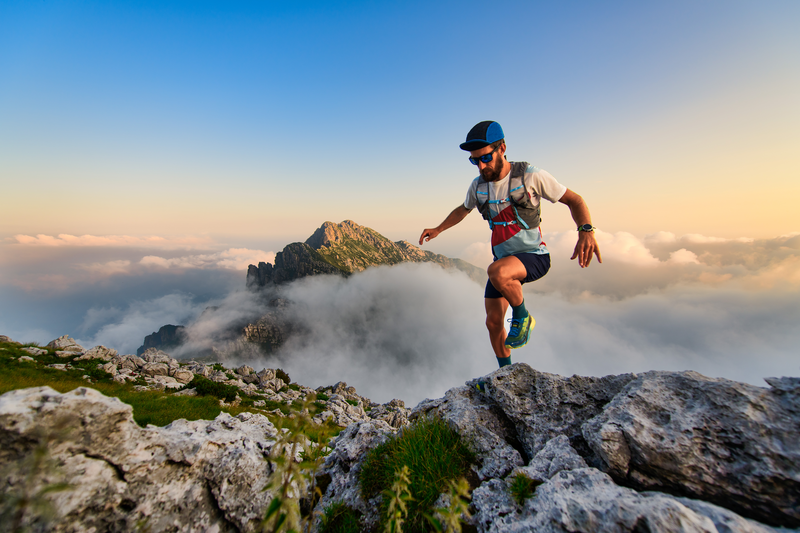
94% of researchers rate our articles as excellent or good
Learn more about the work of our research integrity team to safeguard the quality of each article we publish.
Find out more
SYSTEMATIC REVIEW article
Front. Endocrinol. , 21 March 2024
Sec. Pediatric Endocrinology
Volume 15 - 2024 | https://doi.org/10.3389/fendo.2024.1353334
Background & aims: Metabolic disease prevalence has increased in many regions, and is closely associated with dyslipidemia. Rapid growth refers to a significant increase in growth velocity above the normal range, particularly in infants and children, and is highly prevalent in congenital deficiency infants. But the association between dyslipidemia and rapid growth remains controversial. We performed this meta-analysis to investigate the lipid profile in subjects with and without postnatal rapid growth, and to determine what are the confounding factors.
Methods: Medline, EMBASE, China National Knowledge Infrastructure Chinese citation database and WANFANG database were searched (last search in May 2021). Publication bias was examined by constructing funnel plots, Egger’s linear regression test and Begg’s rank correlation test.
Results: The fixed effects model would be adopted if I2 is less than 25%, otherwise random effects model would be used. There were 11 articles involved with a total of 1148 participants (539 boys and 609 girls, mean age=7.4 years). Pooled analysis found that rapid growth was negatively associated with high-density lipoprotein cholesterol (HDL-C) (weighted mean difference=-0.068, 95%CI [-0.117, -0.020]), but not associated with triglycerides (TG), total cholesterol (TC), or low-density lipoprotein cholesterol (LDL-C). Stratified analysis suggested that increased TG were found in rapid growth subjects from developing countries. Higher TC was observed for rapid growth participants of follow-up age ≤8 years old, rapid growth duration ≤2 years, preterm, low birth weight, and from developing countries. But decreased TC was observed in small for gestational age (SGA) rapid growth subjects. Decreased LDL-C had been documented in rapid growth subjects of follow-up age >8 years old, from developed countries, and SGA. At last, rapid growth groups had lower HDL-C in infants of rapid growth duration >2 years and from developed countries.
Conclusion: Rapid growth is associated with lipid profiles, particularly during early childhood, and this relationship is influenced by factors such as the duration of growth, the level of national development, and birth weight. These findings are significant for the development of strategies to prevent metabolic diseases.
This review was registered in PROSPERO International Prospective Register of Systematic Reviews (www.crd.york.ac.uk/prospero/) with the registration number CRD42020154240.
“Developmental plasticity” (1) describes the remarkable ability of organ development to adapt to environmental signals during sensitive periods, a phenomenon that allows for significant growth adjustments. Research indicates that certain infants, particularly those born preterm, with low birth weight (LBW), or small for gestational age (SGA), often experience a more rapid postnatal growth rate, termed “catch-up growth,” to align with their peers (2, 3). This accelerated growth is especially pronounced when complemented by adequate postnatal nutrition (4), serving as a common compensatory mechanism for these infants (5).
Despite the widespread recognition of rapid growth, there remains no universally accepted standard or definition for this term (6). Generally, any growth rate exceeding the normal growth velocity is classified as rapid growth. This growth can be measured by changes in body height or weight (in centimeters or kilograms). However, a more widely accepted method involves using Z-scores, which compare an individual’s growth to sex and age-specific norms in the general population. A weight or height gain exceeding 0.67 standard deviation scores (SDS) within the first 24 months of life, equivalent to a significant percentile shift (e.g., from the 2nd to the 9th, or 9th to the 25th percentile), is commonly indicative of rapid growth in current research (5). Additionally, accelerated growth in head circumference and body mass index (BMI) is also considered. While rapid growth typically occurs before the age of two, the duration of this growth phase varies significantly across studies.
There is a growing body of evidence suggesting that postnatal growth patterns can have a lasting impact on the programming of fetal gene expression, potentially leading to long-term metabolic risks (7) such as obesity (8–10), glucose metabolism disorders (11, 12), and cardiovascular disease (13). Moreover, substantial evidence indicates a link between these conditions and the dysregulation of cholesterol metabolism (14–18). However, the relationship between rapid postnatal growth and subsequent serum lipid levels remains a subject of debate.
Triglycerides (TG), a form of fat circulating in the bloodstream, serve as an energy source for the body’s cells. Elevated TG levels are associated with an increased risk of cardiovascular diseases (19). Total cholesterol (TC) is a comprehensive measure of cholesterol in the blood, including both low-density lipoprotein cholesterol (LDL-C) and high-density lipoprotein cholesterol (HDL-C). High TC levels are associated with atherosclerosis, a condition characterized by arterial plaque buildup that can lead to heart disease (20). LDL-C, often dubbed “bad cholesterol,” transports cholesterol to tissues and contributes to plaque formation (21), while HDL-C, known as “good cholesterol,” helps clear excess cholesterol from the bloodstream, thereby reducing the risk of heart disease (22). These lipid parameters were chosen for our study as they are pivotal indicators of cardiovascular health and are influenced by growth patterns, particularly during the rapid growth phase in early childhood. Elucidating the relationship between these lipid profiles and rapid growth is crucial for identifying potential risk factors and devising targeted interventions to foster cardiovascular health in children.
This meta-analysis aims to explore the lipid profiles of individuals with and without rapid postnatal growth and to identify influencing factors through subgroup analysis. The study parameters include TG, TC, LDL-C, and HDL-C in plasma.
This review was registered in PROSPERO International Prospective Register of Systematic Reviews (www.crd.york.ac.uk/prospero/) with the registration number CRD42020154240.
We followed the Meta-analysis of Observational Studies in Epidemiology (MOOSE) guidelines for the conduct of systematic reviews and meta-analyses of observational studies (23).
Eligibility for inclusion in our study was based on the following criteria:
(1) Studies must compare the levels of TG, TC, LDL-C, and HDL-C between subjects with rapid growth and those without.
(2) The definition of rapid growth must be explicit, encompassing the duration of rapid growth (e.g., rapid growth in height, weight, and BMI, often termed “catch-up growth,” “catch-up weight gain,” and “catch-up BMI gain” respectively), and the extent of rapid growth (measured by standard deviation scores, SDS). For instance, in our study, a height gain of more than 0.67 SDS from birth to 2 years old was considered clinically significant rapid growth, with TG levels assessed at the age of 8 years. Here, “catch-up growth” refers to the rapid growth mode, SDS>0.67 indicates the extent of rapid growth, and the duration is 2 years (from birth to 2 years old). The follow-up age is set at 8 years, with a follow-up duration of 6 years.
(3) The studies must provide data on the number of subjects, means, standard deviations, and 95% confidence intervals [CIs] for TG, TC, LDL-C, or HDL-C in both the rapid growth and control groups.
(4) Only observational studies were considered for inclusion in the analysis.
We systematically searched Medline (1966–May 2021) and Embase (1980–May 2021) for English studies (the start of their coverage). China National Knowledge Infrastructure Chinese citation database (CNKI) and WANFANG database were especially used to search articles in Chinese.
Considering that there are no universally accepted definitions of rapid growth, we reduced the restrictions of search terms and only entered “catch up growth” OR “rapid growth” OR “rapid weight gain” OR “rapid height gain”.
The following MeSH terms, words and phrases were used in the construction of the systematic search: (“catch-up growth” OR “rapid growth” OR “weight gain” OR “weight change” OR “height change” OR “height gain” OR “linear growth”) AND (“cholesterol” OR “lipoprotein” OR “triglyceride” OR “metabolic”) AND Humans [Mesh].
Two reviewers (Botian Chen and Defu Ma) independently screened titles and abstracts based on the inclusion criteria (The reviewers were trained to recognize the key words and concepts relevant to our research question, which included terms related to rapid growth, lipid profiles, and the specific age groups of interest. During the screening process, the reviewers manually excluded records that did not meet the criteria, such as those that were not original research studies, did not report the required lipid parameters, or were not focused on the relevant age groups. This manual screening was necessary to ensure that only studies with the highest relevance to our research question were included in the subsequent stages of the review.). We also searched the reference lists of all relevant articles to identify other potential studies. Then full-text articles were screened for eligibility. If necessary, we also emailed the authors when the required data were not reported in the articles.
All discrepancies were resolved by discussion and where needed, the third person (Qinghua Xin) arbitrated.
A structured checklist was meticulously designed to independently capture pertinent data by two reviewers, Botian Chen and Defu Ma. Discrepancies in data extraction were addressed through collaborative dialogue. In cases where data were replicated across multiple studies, the study with the largest sample size or the most comprehensive data presentation was prioritized for inclusion. The extracted data encompassed:
1) Author and publication year.
2) Study population: sample size, country, preterm/term or not, SGA/AGA or not, LBW or not.
3) Rapid growth metrics: encompassing growth velocity in anthropometric parameters such as height, weight, and head circumference, the duration of rapid growth, and the extent of growth acceleration.
4) Outcome measures: Means and standard deviations or 95% CIs of TC, TG, LDL-C, and HDL-C in rapid growth groups and control groups, and the follow-up age.
5) Study design: Given the nature of rapid growth, only observational studies were considered. In cohort studies, the follow-up duration was determined from the end of the rapid growth period to the time of outcome assessment. Studies were classified as cross-sectional if the follow-up age coincided with the end of the rapid growth period (0-year follow-up duration).
The methodological quality of the studies was assessed using the Newcastle-Ottawa Scale (24). A total score of 9 stars was allocated, with studies scoring 7 or above deemed to have high methodological quality (Grade A), and those scoring 1 to 6 as low quality (Grade B). Studies with a follow-up duration exceeding three years were deemed sufficiently long-term, and additional stars were awarded to studies with less than 25% missed follow-ups.
Q statistics were used to assess the heterogeneity among the pooled studies, with P<0.10 indicating statistical significance. Additionally, the I2 index was calculated to assess the statistical heterogeneity across studies, representing the proportion of total variability attributable to true heterogeneity. The fixed effects model was chosen when the I2 value was less than 25%; otherwise, the random effects model was used.
We created forest plots to display the means and 95% CIs for the summary analysis. As previously determined, subgroup meta-analyses were conducted to examine potential effect moderators, including rapid growth duration, growth pattern, follow-up age, nationality, and subject characteristics. Weighted mean differences (WMDs) and associated 95% CIs were reported. Furthermore, meta-regression analysis was conducted to assess whether the effect of rapid growth on serum lipid level was related to rapid growth duration and follow-up age. Publication bias was assessed using funnel plots, Egger’s linear regression test, and Begg’s rank correlation test.
All analyses were conducted using STATA (version 11; Stata Corp, College Station, TX, USA), with P < 0.05 considered statistically significant unless otherwise specified.
Initially, we identified 7047 studies, of which 2305 were excluded due to duplication. Upon further scrutiny, 11 studies were found to provide evidence of a relationship between rapid growth and lipid metabolism, comprising 9 English studies (25–33) and 2 Chinese studies (34, 35). Among these, 10 studies examined the associations between rapid growth and TG, 11 studies focused on TC, and 8 studies investigated LDL-C and HDL-C. The process of study selection is depicted in Figure 1.
Figure 1 Flow chart for study selection. TG, triglyceride; TC, total cholesterol; LDL-C, low density lipoprotein cholesterol; HDL-C, high density lipoprotein cholesterol.
Out of the 11 studies identified, seven were cross-sectional, three were cohort, and one was a case-control study. These studies were published between 2002 and 2017 and included a total of 1148 participants, with 1085 for TG, 1148 for TC, and 964 for LDL-C and HDL-C. The studies utilized population samples from various countries, including four from Italy, two from China, and one each from the Netherlands, England, Greece, Germany, and Cyprus. Sample sizes varied from 48 to 198 participants, with five studies having sample sizes under 100. Study design and exclusion and inclusion criteria were well-described in all included studies. And each of them gave a clear definition of rapid growth. The duration of rapid growth varied widely, ranging from 0.25 to 9.2 years, and the mean follow-up age also varied. Rapid growth ways included catch-up growth, catch-up weight gain, and catch-up BMI gain. The characteristics of the included studies are presented in Table 1.
The quality of the included studies was assessed and is presented in Supplementary Table 1. All studies had more than 7 scores, belonging to high-quality research. The mean score was 8.64. Because the lipid profile was shown at the start of the studies, all the included three cohort studies were not assigned a score according to the criteria of the Newcastle-Ottawa scale. Moreover, one study was not assigned a score because it did not have adequate follow-up time.
Twenty-eight valid data were extracted from the 10 studies. Table 2 shows the results of the summary analysis and stratified analysis. In the summary analysis, the heterogeneity test indicated a P-value of 0.105 and an I2 statistic of 26.0%, leading to the adoption of the random effects model. The results indicate that rapid growth was not significantly associated with TG, as shown by a pooled WMD of 0.034 (95%CI [-0.014, 0.081]). The forest diagram for the summary analysis is presented in Figure 2A. Furthermore, sensitivity analysis indicated that no single study significantly altered the pooled results.
Figure 2 Pooled analysis forest diagram of the effect of rapid growth on TG/TC/LDL-C/HDL-C (A) Pooled analysis forest diagram of the effect of rapid growth on TG; (B) Pooled analysis forest diagram of the effect of rapid growth on TC; (C) Pooled analysis forest diagram of the effect of rapid growth on LDL-C; (D) Pooled analysis forest diagram of the effect of rapid growth on HDL-C.
Categorizing the data by follow-up age, definitions of rapid growth, and participant characteristics, the stratified analyses revealed that none of these factors—follow-up age, rapid growth duration, rapid growth method, term or preterm birth status, SGA/AGA status, or LBW status—significantly affected the difference in TG levels between the rapid growth group and the control group. However, when subjects were categorized by their countries as developed or developing, rapid growth was associated with elevated TG levels in developing countries (WMD 0.122, 95%CI [0.002, 0.242]). The linear regression analysis showed that follow-up age (r=-0.001, P=0.886) and rapid growth duration (r=0.002, P=0.641) were not significantly associated with TG.
To evaluate publication bias, a funnel plot was constructed and is presented in Supplementary Figure 1A. The results indicate a symmetrical distribution. Meanwhile, the Begg’s test and Egger’s test also didn’t find publication bias, and the P values of the two tests were 0.707 and 0.664, respectively.
Twenty-nine valid data were extracted from the 11 studies. The heterogeneity was assessed with an I2 value of 31.5%, leading to the use of the random effects model for the summary analysis. The summary analysis indicated that rapid growth was not significantly associated with total cholesterol (TC), with a WMD of 0.058 (95% CI: [-0.044, 0.161]). Sensitivity analysis indicated that the pooled results were not significantly altered by any individual study. Table 3 shows the results of the summary analysis and stratified analyses, and Figure 2B shows the pooled analysis forest diagram.
Stratified analyses revealed a significant association between rapid growth and TC for participants with a follow-up age less than 8 years (WMD 0.113, 95% CI: [0.004, 0.222]) and for those with a rapid growth duration of less than 2 years (WMD 0.126, 95% CI: [0.004, 0.248]). Additionally, subjects who were preterm (WMD 0.175, 95% CI: [0.036, 0.315]), had LBW (WMD 0.212, 95% CI: [0.045, 0.379]), or were from developing countries (WMD 0.254, 95% CI: [0.097, 0.410]) showed higher TC levels in the rapid growth groups. However, infants with SGA who experienced rapid growth had lower TC levels compared to those without rapid growth (WMD -0.177, 95% CI: [-0.346, -0.008]). However, the different methods of rapid growth did not appear to influence TC levels. Meta-regression analyses showed no significant association between TC levels and follow-up age (r=-0.010, P=0.257) or rapid growth duration (r=-0.005, P=0.538).
The funnel plot is presented in Supplementary Figure 1B, with P-values for the Begg’s test and Egger’s test being 0.034 and 0.011, respectively. To address potential publication bias, the trim and fill method was used to recalculate the summary analysis, yielding results indicating no association between rapid growth and TC (WMD -0.062, 95% CI: [-0.172, 0.048]), consistent with the initial findings (WMD 0.058, 95% CI: [-0.044, 0.161]).
Twenty-four valid data were recruited from the 8 studies. The heterogeneity test indicated a P-value less than 0.1 (I2 = 52.5%), leading to the adoption of the random effects model for the combined analysis. The summary analysis showed no significant influence of rapid growth on LDL-C (WMD -0.042, 95%CI [-0.147, 0.062]). Sensitivity analysis indicated that the pooled results were not significantly altered by any single study. Table 4 shows the results of the summary analysis and stratified analyses, and Figure 2C shows the summary analysis forest diagram.
Stratified analyses revealed that LDL-C were lower in subjects with a follow-up age greater than 8 years (WMD -0.220, 95% CI: [-0.389, -0.050]), in those with small for gestational age (SGA) who experienced rapid growth compared to those without (WMD -0.268, 95% CI: [-0.407, -0.129]), and in participants from developed countries (WMD -0.168, 95% CI: [-0.297, -0.04]). In contrast, participants from developing countries with rapid growth exhibited higher LDL-C (WMD 0.132, 95%CI [0.014, 0.250]). Meta-regression analyses showed no significant association between LDL-C and follow-up age (r=-0.011, P=0.136) or rapid growth duration (r=-0.005, P=0.490).
The funnel plot, presented in Supplementary Figure 1C, indicates a symmetrical distribution. Additionally, the Begg’s test (P=0.333) and Egger’s test (P=0.307) did not detect any publication bias.
The studies included for HDL-C analysis were the same as those for LDL-C. In the summary analysis, the heterogeneity test indicated a P-value of 0.149 and an I2 statistic of 23.3%, leading to the use of the random effects model. The results indicated no significant difference in HDL-C between the rapid growth and control groups in the summary analysis (WMD -0.030, 95%CI [-0.074, 0.015]). The Begg’s test yielded a P-value of 0.206, while the funnel plot and Egger’s test (P=0.098) indicated the presence of publication bias. To address the publication bias, the trim and fill method was applied, revealing a negative association between rapid growth and HDL-C (WMD -0.068, 95%CI [-0.117, -0.020]). Sensitivity analysis indicated that no single study significantly influenced the pooled results. Table 5 presents the results of both the summary and stratified analyses, while Figure 2D illustrates the forest diagram from the summary analysis. The funnel plot is displayed in Supplementary Figures 1D, E.
Subgroup analyses identified that a rapid growth duration exceeding 2 years (WMD -0.084, 95% CI: [-0.159, -0.009]) and participants from developed countries (WMD -0.065, 95% CI: [-0.125, -0.004]) were associated with lower HDL-C in the rapid growth groups. Furthermore, rapid growth in SGA infants was associated with lower HDL-C compared to control groups (WMD -0.082, 95% CI: [-0.146, -0.018]). However, when the control groups were further refined, no significant difference in HDL-C was observed between rapid growth SGA infants and those with no rapid growth (SGA or AGA). Linear regression analysis revealed no significant association between HDL-C and follow-up age (r=0.001, P=0.787) or rapid growth duration (r=0.000, P=0.881).
This is the first meta-analysis to investigate the association between rapid growth and subsequent serum lipid levels. Our pooled analysis findings suggested that rapid growth was associated with lower HDL-C. Subgroup analyses revealed that follow-up age, definitions of rapid growth, and participant characteristics influence the associations between rapid growth and TG, TC, LDL-C, and HDL-C.
Dyslipidemia is an established risk factor for many noncommunicable diseases. In clinical research, LDL cholesterol is often calculated using the Friedewald formula: LDL cholesterol (mmol/l) = total cholesterol – HDL cholesterol -0.45*triglycerides (36). Triglyceride is regarded as an energy source for peripheral tissues. They mobilize from adipose tissue in the fasting/starved state. The rise of triglyceride is a risk factor for type II diabetes mellitus (37), metabolic syndrome (38), cardiovascular (39–42), cognitive function (43), and so on. TC is also positively associated with coronary heart disease (CHD) (44) and stroke (45, 46). Furthermore, lipids are transported in the blood by lipoproteins, which include HDL-C and LDL-C. However, the correlation between HDL-C concentration and non HDL-C in vascular risk is opposite. Many therapies reduce the risk of CHD, ischemic heart disease and stroke by lower LDL-C levels (47, 48) and elevating HDL-C levels (49, 50). Dyslipidemia prevalence has increased in many regions (51), especially in developing countries (52, 53). Early childhood represents a potentially modifiable critical period. A thorough understanding of the association between rapid growth and lipid metabolism may lead to new preventive strategies to combat metabolic disease.
It remains uncertain whether there is a specific time window during which rapid growth is beneficial for children (5). Some researchers thought that rapid growth early after birth is a normal adaptation to restore body size and does not impact metabolism (5, 32, 54, 55). Another explanation suggests that prolonged rapid growth is due to sustained higher caloric intake, a known risk factor for metabolism disturbance (56, 57). Our research found that subjects who experienced rapid growth before the age of 2 had higher TC, while those with rapid growth for more than 2 years had lower HDL-C. However, it is important to note that growth is continuous process, and determining the rapid growth at precise time points will always be challenging. Children who experience rapid growth for more than 2 years may have already begun this growth pattern before the age of 2. Conversely, we found that follow-up age did not affect the correlation between rapid growth and TG or HDL-C. However, the rapid growth group exhibited higher TC in subjects under 8 years of age and lower LDL-C in those over 8 years. In conclusion, early-life rapid growth appears to have a long-term beneficial effect on lipid metabolism.
SGA is typically defined as birth weight and/or length at least 2 standard deviations (SDs) below the mean for gestational age or at the 10th percentile. SGA subjects are a heterogeneous population and growth pattern plays an important role in influencing the lipid metabolic risk. It appears that SGA children are prone to various metabolic aberrations. Cianfarani et al. (26) observed that SGA children without rapid growth showed significantly reduced levels of TC than short-AGA subjects. Rabinowicz et al (58) found SGA preterm infants showed higher TG levels compared with age-matched AGA infants. However, the results of our research revealed that there is no difference in lipid profile between rapid growth SGA subjects and no rapid growth AGA infants, but rapid growth SGA showed lower TC and LDL-C when compared to no rapid growth SGA, suggesting that rapid growth is relatively safe for SGA to catch up with their peers. Our findings have significant implications for the feeding practices of SGA infants.
Our study focused solely on the impact of rapid growth on lipid metabolism. To date, epidemiological and clinical studies have shown that many diseases may originate from developmental trajectories in early life (4, 5). For example, some studies have reported that catch-up weight gains predicted increased risk for high glucose concentrations, high blood pressure (59), and cardiovascular disease (60). It has been suggested that interventions aimed at limiting excessive postnatal weight gain might prevent the development of central obesity, insulin resistance, and cardiovascular disease risks (61). However, other evidence showed that rapid growth may have positive effects on development and growth. A cohort study in southern Brazil presented that for SGA children, the rapid growth group had 65% fewer subsequent hospital admissions and 75% lower mortality to age 5 years (62). Rapid growth in very low birth weight infants may reduce bronchial responsiveness (63), decrease short stature risk, and enhance neurodevelopment outcomes (64). Moreover, rapid growth has a negative relationship with insulin resistance and a positive relationship with prealbumin and IGF-1 (65). In summary, various health outcomes can be linked to early-life growth trajectories, and the mechanisms that connect early growth patterns with later outcomes are intricate.
Our study had several limitations. The first limitation is the heterogeneity, as discussed above. Differences in follow-up age, definitions of rapid growth, characteristics of participants, and the chosen control groups, would all affect the interpretation of the conclusions. Although we employed a stratified analysis method, it may not have adequately accounted for all confounding factors. Efforts should be directed towards standardizing the definition of rapid growth, encompassing growth durations, anthropometric parameters, and the extent of growth, to enhance the comparability of results across studies. Secondly, the majority of the included studies have small sample sizes. A large-scale, population-based prospective cohort study is needed to illustrate relative problems. Thirdly, our study is based solely on observational studies, which may not account for all confounding factors, including genetic variation (66–68). Therefore, further exploration of causal inferences is warranted. What’s more, the rapid growth time in our study refers to the time to evaluate the rapid growth, and the specific rapid growth time can only be determined through cohort study. In addition, publication bias is potential limitation.
In conclusion, our study demonstrates a significant association between rapid growth in early childhood and changes in lipid profiles, which may have profound implications for long-term cardiovascular health. These findings emphasize the importance of early growth patterns in the development of metabolic disorders and suggest that interventions targeting rapid growth could be crucial in reducing the risk of cardiovascular diseases in later life. Our research contributes to the growing body of evidence linking early life growth trajectories with adult health outcomes, offering a novel perspective on the critical window of early childhood for preventive strategies. Furthermore, our study provides actionable information for clinicians to monitor and manage the lipid profiles of children experiencing rapid growth, potentially leading to improved health outcomes and a reduction in the burden of cardiovascular diseases in the population.
BC: Conceptualization, Data curation, Formal Analysis, Methodology, Visualization, Writing – original draft, Writing – review & editing. DM: Conceptualization, Data curation, Formal Analysis, Funding acquisition, Methodology, Writing – original draft, Writing – review & editing. YC: Data curation, Formal Analysis, Writing – review & editing. WY: Writing – review & editing. QX: Writing – review & editing.
The author(s) declare financial support was received for the research, authorship, and/or publication of this article. This research was supported by a Grant from the National Natural Science Foundation of China (Nos. 81202193 and 81573130) and the Beijing Natural Science Foundation (Nos. S160004, 7172117, and 7122103) to Defu Ma.
The authors declare that the research was conducted in the absence of any commercial or financial relationships that could be construed as a potential conflict of interest.
All claims expressed in this article are solely those of the authors and do not necessarily represent those of their affiliated organizations, or those of the publisher, the editors and the reviewers. Any product that may be evaluated in this article, or claim that may be made by its manufacturer, is not guaranteed or endorsed by the publisher.
The Supplementary Material for this article can be found online at: https://www.frontiersin.org/articles/10.3389/fendo.2024.1353334/full#supplementary-material
1. Gluckman PD, Hanson MA. Living with the past: evolution, development, and patterns of disease. Sci (New York NY). (2004) 305:1733–6. doi: 10.1126/science.1095292
2. Ong KK, Preece MA, Emmett PM, Ahmed ML, Dunger DB. Size at birth and early childhood growth in relation to maternal smoking, parity and infant breast-feeding: longitudinal birth cohort study and analysis. Pediatr Res. (2002) 52:863–7. doi: 10.1203/00006450-200212000-00009
3. Albertsson-Wikland K, Wennergren G, Wennergren M, Vilbergsson G, Rosberg S. Longitudinal follow-up of growth in children born small for gestational age. Acta Paediatrica (Oslo Norway 1992). (1993) 82:438–43. doi: 10.1111/j.1651-2227.1993.tb12718.x
4. Ong KK, Kennedy K, Castaneda-Gutierrez E, Forsyth S, Godfrey KM, Koletzko B, et al. Postnatal growth in preterm infants and later health outcomes: a systematic review. Acta Paediatrica (Oslo Norway 1992). (2015) 104:974–86. doi: 10.1111/apa.13128
5. Martin A, Connelly A, Bland RM, Reilly JJ. Health impact of catch-up growth in low-birth weight infants: systematic review, evidence appraisal, and meta-analysis. Maternal Child Nutr. (2017) 13(1):10.1111/mcn.12297. doi: 10.1111/mcn.12297
6. Baird J, Fisher D, Lucas P, Kleijnen J, Roberts H, Law C. Being big or growing fast: systematic review of size and growth in infancy and later obesity. BMJ (Clinical Res ed). (2005) 331:929. doi: 10.1136/bmj.38586.411273.E0
7. Barker DJ, Osmond C. Infant mortality, childhood nutrition, and ischaemic heart disease in England and Wales. Lancet (London England). (1986) 1:1077–81. doi: 10.1016/S0140-6736(86)91340-1
8. Salgin B, Norris SA, Prentice P, Pettifor JM, Richter LM, Ong KK, et al. Even transient rapid infancy weight gain is associated with higher BMI in young adults and earlier menarche. Int J Obes (2005). (2015) 39:939–44. doi: 10.1038/ijo.2015.25
9. Alves JG, Vasconcelos SA, de Almeida TS, Lages R, Just E. Influence of catch-up growth on abdominal fat distribution in very low birth weight children - cohort study. J Pediatr Endocrinol Metab JPEM. (2015) 28:153–6. doi: 10.1515/jpem-2014-0191
10. Belfort MB, Gillman MW, Buka SL, Casey PH, McCormick MC. Preterm infant linear growth and adiposity gain: trade-offs for later weight status and intelligence quotient. J Pediatr. (2013) 163:1564–9.e2. doi: 10.1016/j.jpeds.2013.06.032
11. Veening MA, Van Weissenbruch MM, Delemarre-Van De Waal HA. Glucose tolerance, insulin sensitivity, and insulin secretion in children born small for gestational age. J Clin Endocrinol Metab. (2002) 87:4657–61. doi: 10.1210/jc.2001-011940
12. Liu C, Wu B, Lin N, Fang X. Insulin resistance and its association with catch-up growth in Chinese children born small for gestational age. Obes (Silver Spring Md). (2017) 25:172–7. doi: 10.1002/oby.21683
13. Leunissen RW, Kerkhof GF, Stijnen T, Hokken-Koelega A. Timing and tempo of first-year rapid growth in relation to cardiovascular and metabolic risk profile in early adulthood. Jama. (2009) 301:2234–42. doi: 10.1001/jama.2009.761
14. DeBose-Boyd RA. Significance and regulation of lipid metabolism. Semin Cell Dev Biol. (2018) 81:97. doi: 10.1016/j.semcdb.2017.12.003
15. Zhou M, Zhu L, Cui X, et al. The triglyceride to high-density lipoprotein cholesterol (TG/HDL-C) ratio as a predictor of insulin resistance but not of beta cell function in a Chinese population with different glucose tolerance status. Lipids Health Dis. (2016) 15:104. doi: 10.1186/s12944-016-0270-z
16. Bahendeka S, Wesonga R, Mutungi G, Muwonge J, Neema S, Guwatudde D. Prevalence and correlates of diabetes mellitus in Uganda: a population-based national survey. Trop Med Int Health TM IH. (2016) 21:405–16. doi: 10.1111/tmi.12663
17. van Schie MC, Jainandunsing S, van Lennep JER. Monogenetic disorders of the cholesterol metabolism and premature cardiovascular disease. Eur J Pharmacol. (2017) 816:146–53. doi: 10.1016/j.ejphar.2017.09.046
18. von Muhlen D, Langer RD, Barrett-Connor E. Sex and time differences in the associations of non-high-density lipoprotein cholesterol versus other lipid and lipoprotein factors in the prediction of cardiovascular death (The Rancho Bernardo Study). Am J Cardiol. (2003) 91:1311–5. doi: 10.1016/s0002-9149(03)00319-9
19. Boullart AC, de Graaf J, Stalenhoef AF. Serum triglycerides and risk of cardiovascular disease. Biochim Biophys Acta. (2012) 1821:867–75. doi: 10.1016/j.bbalip.2011.10.002
20. Jung E, Kong SY, Ro YS, Ryu HH, Shin SD. Serum cholesterol levels and risk of cardiovascular death: A systematic review and a dose-response meta-analysis of prospective cohort studies. Int J Environ Res Public Health. (2022) 19:8272. doi: 10.3390/ijerph19148272
21. Ference BA, Yoo W, Alesh I, Mahajan N, Mirowska KK, Mewada A, et al. Effect of long-term exposure to lower low-density lipoprotein cholesterol beginning early in life on the risk of coronary heart disease: a Mendelian randomization analysis. J Am Coll Cardiol. (2012) 60:2631–9. doi: 10.1016/j.jacc.2012.09.017
22. Nicholls SJ, Nelson AJ. HDL and cardiovascular disease. Pathology. (2019) 51:142–7. doi: 10.1016/j.pathol.2018.10.017
23. Stroup DF, Berlin JA, Morton SC, Olkin I, Williamson GD, Rennie D, et al. Meta-analysis of observational studies in epidemiology: a proposal for reporting. Meta-analysis Of Observational Studies in Epidemiology (MOOSE) group. JAMA. (2000) 283:2008–12. doi: 10.1001/jama.283.15.2008
24. Wells G SB, O’Connell D, Peterson J, Welch V, Losos M, Tugwell P. The Newcastle-Ottawa Scale (NOS) for assessing the quality of nonrandomised studies in meta-analyses (2013). Available online at: http://www.ohri.ca/programs/clinical_epidemiology/oxford.asp.
25. Cianfarani S, Geremia C, Scott CD, Germani D. Growth, IGF system, and cortisol in children with intrauterine growth retardation: is catch-up growth affected by reprogramming of the hypothalamic-pituitary-adrenal axis? Pediatr Res. (2002) 51:94–9. doi: 10.1203/00006450-200201000-00017
26. Cianfarani S, Maiorana A, Geremia C, Scirè G, Spadoni GL, Germani D. Blood glucose concentrations are reduced in children born small for gestational age (SGA), and thyroid-stimulating hormone levels are increased in SGA with blunted postnatal catch-up growth. J Clin Endocrinol Metab. (2003) 88:2699–705. doi: 10.1210/jc.2002-021882
27. Toumba M, Hadjidemetriou A, Topouzi M, Savva SC, Demetriadou R, Kanaris C, et al. Evaluation of the auxological and metabolic status in prepubertal children born small for gestational age. J Pediatr Endocrinol Metab JPEM. (2005) 18:677–88. doi: 10.1515/JPEM.2005.18.7.677
28. Mohn A, Chiavaroli V, Cerruto M, Blasetti A, Giannini C, Bucciarelli T, et al. Increased oxidative stress in prepubertal children born small for gestational age. J Clin Endocrinol Metab. (2007) 92:1372–8. doi: 10.1210/jc.2006-1344
29. Torre P, Ladaki C, Scire G, Spadoni GL, Cianfarani S. Catch-up growth in body mass index is associated neither with reduced insulin sensitivity nor with altered lipid profile in children born small for gestational age. J Endocrinol Invest. (2008) 31:760–4. doi: 10.1007/BF03349254
30. Gohlke BC, Stutte S, Bartmann P, Woelfle J. Does gender-specific BMI development modulate insulin sensitivity in extremely low birth weight infants? J Pediatr Endocrinol Metab JPEM. (2009) 22:827–35. doi: 10.1515/JPEM.2009.22.9.827
31. Leunissen RW, Gao Y, Cianflone K, Stijnen T, Hokken-Koelega AC. Growth patterns during childhood and the relationship with acylation-stimulating protein. Clin Endocrinol. (2010) 72:775–80. doi: 10.1111/j.1365-2265.2010.03771.x
32. Embleton ND, Korada M, Wood CL, Pearce MS, Swamy R, Cheetham TD. Catch-up growth and metabolic outcomes in adolescents born preterm. Arch Dis Childhood. (2016) 101:1026–31. doi: 10.1136/archdischild-2015-310190
33. Giapros V, Vavva E, Siomou E, Kolios G, Tsabouri S, Cholevas V, et al. Low-birth-weight, but not catch-up growth, correlates with insulin resistance and resistin level in SGA infants at 12 months. J Maternal-fetal Neonatal Med. (2017) 30:1771–6. doi: 10.1080/14767058.2016.1224838
34. Wang J, Yang MT, Chen XX, Huang K, Tong XT, Chen MQ. Relationship between catch-up growth of premature infants and insulin resistance. Chin J Appl Clin Pediatr. (2015) 8):593–6.
35. Wei HX, Ding YL. Effect of catch-up growth in low birth weight premature infants on insulin resistance. Hainan Med J. (2016) 17:2144–6,7.
36. Friedewald WT, Levy RI, Fredrickson DS. Estimation of the concentration of low-density lipoprotein cholesterol in plasma, without use of the preparative ultracentrifuge. Clin Chem. (1972) 18:499–502. doi: 10.1093/clinchem/18.6.499
37. Banks WA, Farr SA, Salameh TS, Niehoff ML, Rhea EM, Morley JE, et al. Triglycerides cross the blood-brain barrier and induce central leptin and insulin receptor resistance. Int J Obes (2005). (2018) 42:391–7. doi: 10.1038/ijo.2017.231
38. Eckel RH, Grundy SM, Zimmet PZ. The metabolic syndrome. Lancet (London England). (2005) 365:1415–28. doi: 10.1016/S0140-6736(05)66378-7
39. Abdel-Maksoud MF, Hokanson JE. The complex role of triglycerides in cardiovascular disease. Semin Vasc Med. (2002) 2:325–33. doi: 10.1055/s-2002-35403
40. Budoff M. Triglycerides and triglyceride-rich lipoproteins in the causal pathway of cardiovascular disease. Am J Cardiol. (2016) 118:138–45. doi: 10.1016/j.amjcard.2016.04.004
41. Wang X, Ye P, Cao R, Yang X, Xiao W, Zhang Y, et al. Triglycerides are a predictive factor for arterial stiffness: a community-based 4.8-year prospective study. Lipids Health Dis. (2016) 15:97. doi: 10.1186/s12944-016-0266-8
42. Bansal S, Buring JE, Rifai N, Mora S, Sacks FM, Ridker PM. Fasting compared with nonfasting triglycerides and risk of cardiovascular events in women. Jama. (2007) 298:309–16. doi: 10.1001/jama.298.3.309
43. Farr SA, Yamada KA, Butterfield DA, Abdul HM, Xu L, Miller NE, et al. Obesity and hypertriglyceridemia produce cognitive impairment. Endocrinology. (2008) 149:2628–36. doi: 10.1210/en.2007-1722
44. Nagasawa SY, Okamura T, Iso H, Tamakoshi A, Yamada M, Watanabe M, et al. Relation between serum total cholesterol level and cardiovascular disease stratified by sex and age group: a pooled analysis of 65 594 individuals from 10 cohort studies in Japan. J Am Heart Assoc. (2012) 1:e001974. doi: 10.1161/JAHA.112.001974
45. Huxley R, Lewington S, Clarke R. Cholesterol, coronary heart disease and stroke: a review of published evidence from observational studies and randomized controlled trials. Semin Vasc Med. (2002) 2:315–23. doi: 10.1055/s-2002-35402
46. Lawlor DA, Owen CG, Davies AA, Whincup PH, Ebrahim S, Cook DG, et al. Sex differences in the association between birth weight and total cholesterol. A meta-analysis. Ann Epidemiol. (2006) 16:19–25. doi: 10.1016/j.annepidem.2005.04.006
47. Law MR, Wald NJ, Rudnicka AR. Quantifying effect of statins on low density lipoprotein cholesterol, ischaemic heart disease, and stroke: systematic review and meta-analysis. BMJ (Clinical Res ed). (2003) 326:1423. doi: 10.1136/bmj.326.7404.1423
48. Expert Panel on Detection, Evaluation, Treatment of High Blood Cholesterol in Adults. Executive summary of the third report of the national cholesterol education program (NCEP) expert panel on detection, evaluation, and treatment of high blood cholesterol in adults (Adult treatment panel III). Jama. (2001) 285:2486–97. doi: 10.1001/jama.285.19.2486
49. Robins SJ, Collins D, Wittes JT, Papademetriou V, Deedwania PC, Schaefer EJ, et al. Relation of gemfibrozil treatment and lipid levels with major coronary events: VA-HIT: a randomized controlled trial. Jama. (2001) 285:1585–91. doi: 10.1001/jama.285.12.1585
50. Genest J Jr., Marcil M, Denis M, Yu L. High density lipoproteins in health and in disease. J Invest Med. (1999) 47:31–42.
51. Halcox JP, Banegas JR, Roy C, Dallongeville J, De Backer G, Guallar E, et al. Prevalence and treatment of atherogenic dyslipidemia in the primary prevention of cardiovascular disease in Europe: EURIKA, a cross-sectional observational study. BMC Cardiovasc Disord. (2017) 17:160. doi: 10.1186/s12872-017-0591-5
52. Supiyev A, Nurgozhin T, Zhumadilov Z, Peasey A, Hubacek JA, Bobak M. Prevalence, awareness, treatment and control of dyslipidemia in older persons in urban and rural population in the Astana region, Kazakhstan. BMC Public Health. (2017) 17:651. doi: 10.1186/s12889-017-4629-5
53. Gao N, Yu Y, Zhang B, Yuan Z, Zhang H, Song Y, et al. Dyslipidemia in rural areas of North China: prevalence, characteristics, and predictive value. Lipids Health Dis. (2016) 15:154. doi: 10.1186/s12944-016-0328-y
54. Lei X, Chen Y, Ye J, Ouyang F, Jiang F, Zhang J. The optimal postnatal growth trajectory for term small for gestational age babies: a prospective cohort study. J Pediatr. (2015) 166:54–8. doi: 10.1016/j.jpeds.2014.09.025
55. Bouhours-Nouet N, Dufresne S, de Casson FB, Mathieu E, Douay O, Gatelais F, et al. High birth weight and early postnatal weight gain protect obese children and adolescents from truncal adiposity and insulin resistance: metabolically healthy but obese subjects? Diabetes Care. (2008) 31:1031–6. doi: 10.2337/dc07-1647
56. Ibanez L, Lopez-Bermejo A, Diaz M, de Zegher F. Catch-up growth in girls born small for gestational age precedes childhood progression to high adiposity. Fertil Steril. (2011) 96:220–3. doi: 10.1016/j.fertnstert.2011.03.107
57. Crowther NJ, Cameron N, Trusler J, Toman M, Norris SA, Gray IP. Influence of catch-up growth on glucose tolerance and beta-cell function in 7-year-old children: results from the birth to twenty study. Pediatrics. (2008) 121:e1715–22. doi: 10.1542/peds.2007-3147
58. Rabinowicz S, Levkovitz O, Leibovitch L, Schushan-Eisen I, Morag I, Rosen C, et al. Increased risk for early hypertriglyceridemia in small for gestational age preterm infants. Eur J Pediatr. (2020) 179:1873–9. doi: 10.1007/s00431-020-03764-8
59. Victora CG, Adair L, Fall C, Hallal PC, Martorell R, Richter L, et al. Maternal and child undernutrition: consequences for adult health and human capital. Lancet (London England). (2008) 371:340–57. doi: 10.1016/S0140-6736(07)61692-4
60. Barker DJ, Osmond C, Forsen TJ, Kajantie E, Eriksson JG. Trajectories of growth among children who have coronary events as adults. New Engl J Med. (2005) 353:1802–9. doi: 10.1056/NEJMoa044160
61. Ibanez L, Ong K, Dunger DB, de Zegher F. Early development of adiposity and insulin resistance after catch-up weight gain in small-for-gestational-age children. J Clin Endocrinol Metab. (2006) 91:2153–8. doi: 10.1210/jc.2005-2778
62. Victora CG, Barros FC, Horta BL, Martorell R. Short-term benefits of catch-up growth for small-for-gestational-age infants. Int J Epidemiol. (2001) 30:1325–30. doi: 10.1093/ije/30.6.1325
63. Mai XM, Gaddlin PO, Nilsson L, Leijon I. Early rapid weight gain and current overweight in relation to asthma in adolescents born with very low birth weight. Pediatr Allergy Immunol. (2005) 16:380–5. doi: 10.1111/j.1399-3038.2005.00290.x
64. Latal-Hajnal B, von Siebenthal K, Kovari H, Bucher HU, Largo RH. Postnatal growth in VLBW infants: significant association with neurodevelopmental outcome. J Pediatr. (2003) 143:163–70. doi: 10.1067/S0022-3476(03)00243-9
65. Sebastiani G, Diaz M, Bassols J, Aragonés G, López-Bermejo A, de Zegher F, et al. The sequence of prenatal growth restraint and post-natal catch-up growth leads to a thicker intima-media and more pre-peritoneal and hepatic fat by age 3-6 years. Pediatr Obes. (2016) 11:251–7. doi: 10.1111/ijpo.12053
66. Morgan AR, Thompson JM, Murphy R, Black PN, Lam WJ, Ferguson LR, et al. Obesity and diabetes genes are associated with being born small for gestational age: results from the Auckland Birthweight Collaborative study. BMC Med Genet. (2010) 11:125. doi: 10.1186/1471-2350-11-125
67. Freathy RM, Bennett AJ, Ring SM, Shields B, Groves CJ, Timpson NJ, et al. Type 2 diabetes risk alleles are associated with reduced size at birth. Diabetes. (2009) 58:1428–33. doi: 10.2337/db08-1739
Keywords: rapid growth, triglyceride, total cholesterol, low density lipoprotein, high density lipoprotein
Citation: Chen B, Chen Y, Wang Y, Xin Q and Ma D (2024) The association between rapid growth and lipid profile: a systematic review and meta-analysis. Front. Endocrinol. 15:1353334. doi: 10.3389/fendo.2024.1353334
Received: 10 December 2023; Accepted: 27 February 2024;
Published: 21 March 2024.
Edited by:
Semra Çaglar Çetinkaya, University of Health Sciences (Turkey), TürkiyeReviewed by:
Roshan Kumar Mahat, Dharanidhar Medical College and Hospital, IndiaCopyright © 2024 Chen, Chen, Wang, Xin and Ma. This is an open-access article distributed under the terms of the Creative Commons Attribution License (CC BY). The use, distribution or reproduction in other forums is permitted, provided the original author(s) and the copyright owner(s) are credited and that the original publication in this journal is cited, in accordance with accepted academic practice. No use, distribution or reproduction is permitted which does not comply with these terms.
*Correspondence: Defu Ma, bWFkZWZ1QGJqbXUuZWR1LmNu
Disclaimer: All claims expressed in this article are solely those of the authors and do not necessarily represent those of their affiliated organizations, or those of the publisher, the editors and the reviewers. Any product that may be evaluated in this article or claim that may be made by its manufacturer is not guaranteed or endorsed by the publisher.
Research integrity at Frontiers
Learn more about the work of our research integrity team to safeguard the quality of each article we publish.