- 1Department of Cardiology, The First People’s Hospital of Wenling (The Affiliated Wenling Hospital of Wenzhou Medical University), Wenling, Zhejiang, China
- 2The Orthopaedic Center, The First People’s Hospital of Wenling (The Affiliated Wenling Hospital of Wenzhou Medical University), Wenling, Zhejiang, China
- 3Department of Internal Medicine, Yuhuan Second People’s Hospital, Yuhuan, Zhejiang, China
Vascular calcification is a common accompanying pathological change in many chronic diseases, which is caused by calcium deposition in the blood vessel wall and leads to abnormal blood vessel function. With the progress of medical technology, the diagnosis rate of vascular calcification has explosively increased. However, due to its mechanism’s complexity, no effective drug can relieve or even reverse vascular calcification. Irisin is a myogenic cytokine regulating adipose tissue browning, energy metabolism, glucose metabolism, and other physiological processes. Previous studies have shown that irisin could serve as a predictor for vascular calcification, and protect against hypertension, diabetes, chronic kidney disease, and other risk factors for vascular calcification. In terms of mechanism, it improves vascular endothelial dysfunction and phenotypic transformation of vascular smooth muscle cells. All the above evidence suggests that irisin plays a predictive and protective role in vascular calcification. In this review, we summarize the association of irisin to the related risk factors for vascular calcification and mainly explore the role of irisin in vascular calcification.
Introduction
Vascular calcification is a common complication of many diseases characterized by calcium deposition on the vessel wall, including diabetes, atherosclerosis, chronic kidney disease (CKD), and hypertension (1). Abnormal vascular calcification might impair vasomotor function and increase the risk of plaque rupture (2). The cohort studies showed that coronary artery calcification was independently associated with major adverse cardiovascular events (MACE) and was even the leading cause of death in CKD patients (3, 4). Due to the complexity of its mechanism, unfortunately, there is currently no effective drug to relieve vascular calcification. Therefore, exploring the mechanism of occurrence and development of vascular calcification and effective therapeutic targets is imperative.
Irisin, first reported in 2012 by Boström et al, is a 112-amino acid myogenic cytokine produced from the shed extracellular region of the transmembrane protein Fibronectin Type III Domain-Containing Protein 5 (FNDC5) (5). In the skeletal muscle cells, exercise could activate PGC-1α, thereby promoting the expression of FNDC5, which is processed into irisin (5). Interestingly, the translation initiation codon of human FNDC5 is atypical ATA rather than typical ATG (6). While the ATA codon in human FNDC5 may represent a null mutation, humans were presumed incapable of producing irisin (7, 8). The key findings using modified mass spectrometry techniques confirmed that irisin was real and expressed mainly at the atypical ATA start codon of FNDC5 (9). Subsequently, irisin has been shown to bind to its receptor αV/β5 integrin, thereby playing a protective role in bone remodeling and gut barrier function (10–12). Furthermore, Mu et al. found that the extracellular chaperone heat shock protein 90α (HSP90α) was involved in irisin-mediated integrin activation in mice (13). Exercise can lead to an increase in irisin levels, as well as the release of HSP90α. Then, HSP90α could promote αVβ5 activation, enabling irisin to act efficiently through its integrin receptor.
Subsequent research confirmed that exercise with the proper intensity and duration could stimulate the release of irisin, sequentially regulating glucose homeostasis, energy metabolism, and white adipose tissue browning (14–16). A meta-analysis containing 921 participants showed that exercise training could significantly increase irisin and decline insulin, glucose, and insulin resistance (17). Also, high-fat diets could increase the expression of Zfp57 by inhibiting the AMPK pathway, thereby inducing the inhibition of FNDC5 and further exacerbating insulin resistance in mouse muscle cells (18). The available evidence suggests that irisin takes part in a variety of metabolic processes and has protective effects against many metabolic diseases, such as diabetes, metabolic syndrome, obesity, etc. (19).
In addition to skeletal muscle, cardiac muscle is another source of irisin (20). As a myotropin, irisin is an important target for cardiac exercise rehabilitation, which can improve cardiac function after myocardial infarction (21). Irisin may alleviate ischemia-reperfusion injury by reducing oxidative stress, improving mitochondrial health, and inhibiting inflammation (22). Collectively, increasing evidence confirms the emerging protective effects of irisin in several cardiovascular diseases (23). In this review, we mainly focused on the role of irisin in risk factors and vascular calcification and provided useful clues for the application of irisin to relieve vascular calcification.
Irisin modulates risk factors for vascular calcification
Vascular calcification was previously thought to be a passive degenerative disease with aging. With a deeper understanding of molecular mechanisms, vascular calcification is defined as an active, reversible, and highly regulatable process affected by many factors (24). Currently recognized risk factors for vascular calcification include genetic factors, hypertension, diabetes, lipid metabolism disorders, inflammation, oxidative stress, imbalance of calcium and phosphorus homeostasis. Next, we mainly discuss the role of irisin in suppressing risk factors for vascular calcification (Figure 1).
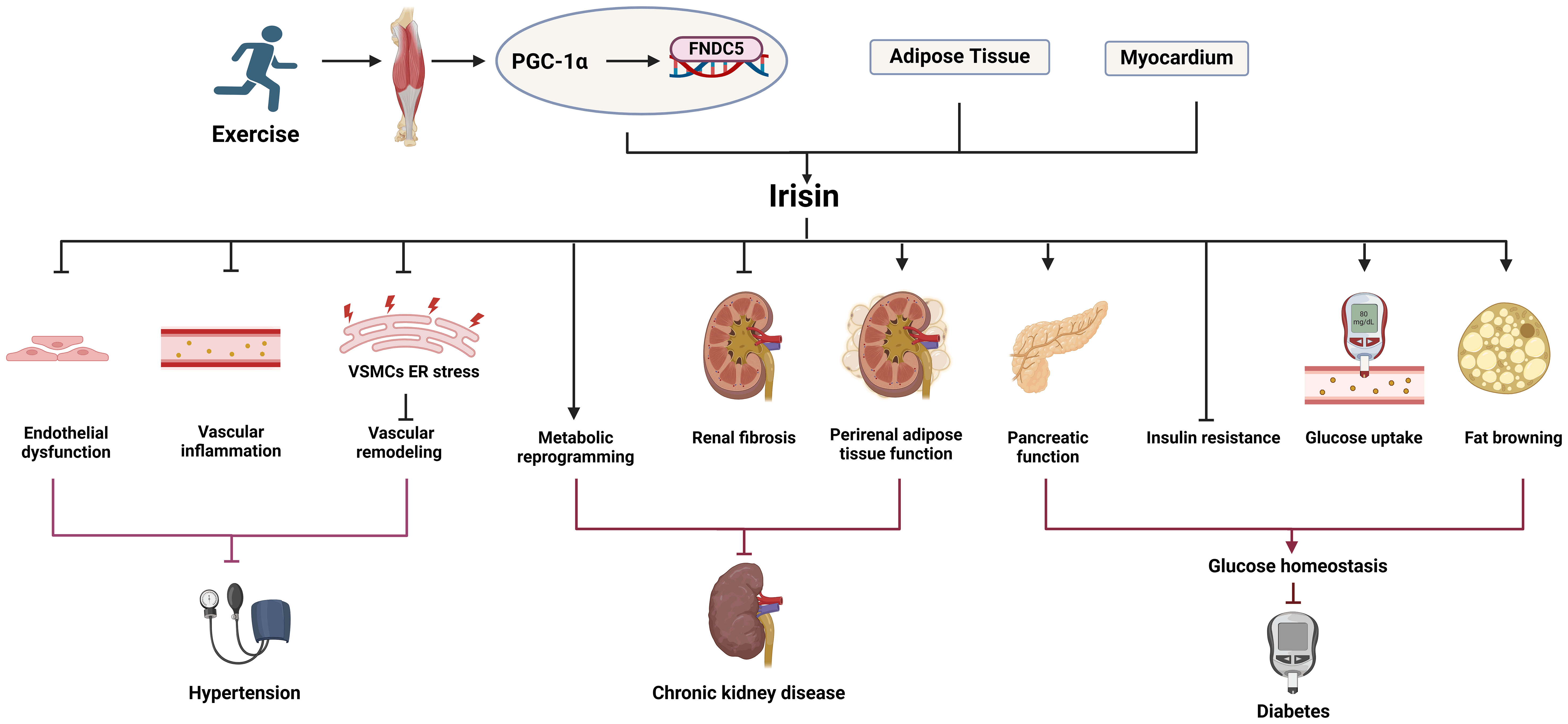
Figure 1 The role of irisin in the risk factors for vascular calcification. Irisin can inhibit hypertension, diabetes, chronic kidney disease, oxidative stress, inflammation and other risk factors related to vascular calcification.
Irisin and hypertension
Hypertension is a common chronic condition caused by a combination of genetic, environmental, and other factors, featured on elevated diastolic and/or systolic blood pressure. Chronically elevated blood pressure can lead to a gradual thickening of the aortic wall, dilation of the lumen, and eventually changes in the composition of the vessel wall and vascular calcification. In turn, vascular calcification might reduce the elasticity of artery walls, increase artery stiffness, and further increase blood pressure, forming a vicious cycle (25). The polymorphism in the gene encoding irisin (FNDC5 rs1746661) has been identified to be related to high systolic blood pressure and dyslipidemia in female patients with type 2 diabetes (T2DM) (26). Besides, a negative correlation has been shown between circulating irisin and blood pressure. Irisin levels decreased as blood pressure increased in preeclampsia and dialysis patients (27, 28). Compared to non-hypertensive diabetics, serum irisin is negatively correlated with blood pressure in hypertensive patients (29). All the above evidence indicates that irisin is significantly related to hypertension, and may be involved in the regulation of blood pressure.
Endothelial dysfunction, vascular inflammation, and vascular remodeling lead to increased peripheral resistance and vascular damage, which accelerates the rise in blood pressure (30). The subsequent mechanistic study showed that irisin supplementation could improve mesenteric artery endothelial dysfunction and reduce blood pressure in spontaneously hypertensive rats through AMPK-AKT-NO signaling pathway (31). Irisin could lower blood pressure by inhibiting oxidative stress and inflammation in Zucker diabetic adipose rats (32). Irisin might alleviate endoplasmic reticulum stress of vascular smooth muscle cells (VSMCs) by activating AMPK and inhibiting p38 signaling pathway, thereby protecting hypertension and vascular remodeling (33). Fortunately, irisin happens to be a multifunctional factor, acting on the above-mentioned pathophysiology and thus participating in the regulation of blood pressure.
Interestingly, treatment of amlodipine and valsartan (12 weeks) increased irisin levels in hypertensive patients (34). In addition, intravenous irisin effectively reduced blood pressure via Nrf2-mediated antioxidant effects in the paraventricular nucleus of spontaneously hypertensive rats (35). However, irisin might increase blood pressure and cardiac contractility when it was injected into the third ventricle of rats by activating neurons in the paraventricular nucleus of the hypothalamus (36). It implies that central and peripheral irisin may have different regulatory effects on blood pressure, and the specific mechanism still needs to be further explored.
Most of the above evidence suggests that irisin has a protective effect against hypertension. However, in Nω-Nitro-L-arginine methyl ester hydrochloride-induced models of hypertension, chronic irisin treatment with physiological doses did not reduce blood pressure (37). An observational study has also shown that irisin levels are positively correlated with systolic blood pressure and could serve as an independent predictor of hypertension. Subgroup analysis implied that irisin was associated with hypertension-related stroke significantly (38). The controversial results may be caused by different hypertension populations and different animal models of hypertension. In addition, previous studies have shown that central and peripheral irisin may have different regulation of blood pressure, and central irisin supplementation may lead to increased blood pressure, which may explain the correlation between irisin and hypertensive stroke. In the future, the effects of irisin should be evaluated separately for specific populations and different modes of administration and dosages.
Irisin and diabetes
Diabetes mellitus is a multi-etiological metabolic disease caused by insufficient insulin secretion and/or defective function, which is also a major cause of vascular calcification. A meta-analysis showed that the percentages of coronary artery calcium score (CACS)>0, CACS≥100, and CACS≥400 in diabetes patients were 29.3%-86.0%, 22.8%-65.0%, and 7.0%-37.8%, respectively (39). The PREDICT study suggested that CACS was a strong predictor of MACE in asymptomatic T2DM patients, with CACS 101-1000 patients having a 10.5-fold increased risk and CACS exceeding 1000 patients having a 19.8-fold increased risk of cardiovascular events (40). Obviously, diabetes is a major risk factor for vascular calcification.
It has been proved that irisin might regulate glucose metabolism, promote glucose utilization, and improve blood glucose disorders (41). Compared with healthy people, T2DM patients had lower circulating irisin levels, while the circulating irisin levels of long-standing T1DM patients and newly diagnosed T2DM patients were higher than that of the control group (42–47). Even, in patients with diabetic complications including diabetic retinopathy and diabetic nephropathy, irisin levels may be further reduced (48, 49). Therefore, irisin may be a promising predictor of diabetes’ development and progression. As for the elevated irisin levels in patients with new onset T2DM, a protective increase may be one of the reasons. The inconsistency in patients with different types of diabetes may be related to the different etiology of the two types of diabetes and the complexity of the mechanism of irisin regulating homeostasis.
Insulin resistance and abnormal pancreatic function are common pathological features of T2DM. A meta-analysis enrolling non-diabetic patients confirmed that circulating irisin was associated with insulin resistance (48). And irisin can increase energy expenditure, reduce body weight, and improve insulin sensitivity (50, 51). For example, irisin ameliorated insulin resistance by preserving the AMPK-insulin receptor signaling pathway in myoblasts (52). Pancreatic β-cells dysfunction is another common cause of diabetes, and chronic damage to pancreatic β-cells can further increase insulin resistance. Irisin can reduce the apoptosis of pancreatic β-cells by regulating apoptosis-related proteins (53). Both in vivo and in vitro studies have confirmed that irisin can promote the proliferation of pancreatic β-cells, increase insulin secretion and improve the function of pancreatic β-cells (53, 54). Additionally, irisin could alleviate insulin resistance and inflammation in pancreatic β-cells by activating PI3K/AKT/FOXO1 signaling pathway and inhibiting TLR4/NF-κB signaling pathway (55). In addition to the above effect, irisin promoted GLUT4 translocation and glucose uptake in skeletal muscle through AMPK signaling pathway and MAPK signaling pathway (56–58). Irisin could induce fat browning, increase insulin sensitivity and increase glucose uptake in adipose tissue (59–61). Studies have also shown that irisin can regulate hepatic glycogen synthesis and gluconeogenesis through multiple signaling pathways to maintain glucose homeostasis (62, 63).
Current results confirm that irisin plays a multi-dimensional protective role in diabetes by reducing insulin resistance, promoting β cell proliferation, increasing insulin secretion, improving glucose uptake in peripheral tissues, and regulating gluconeogenesis.
Irisin and CKD
There are many risk factors for vascular calcification in CKD patients, including hyperphosphatemia, hypercalcemia, increased mineralization, uremic toxins, and hemodynamic abnormalities caused by hemodialysis (64). Irisin was significantly decreased in CKD patients and its decreased levels are negatively associated with blood urea nitrogen and creatinine (65–69). For dialysis patients, the irisin levels of peritoneal dialysis patients were higher than those of hemodialysis patients, and the levels were related to the adequacy of peritoneal dialysis (70, 71). In addition, low serum irisin levels were associated with significantly increased risks of vascular calcification, cardiovascular disease, and cardiovascular and cerebrovascular disease mortality in hemodialysis patients (72–74). Also, irisin could independently predict carotid atherosclerosis and sarcopenia in peritoneal dialysis patients (75). Collectively, low irisin in CKD patients leads to loss of muscle as well as the occurrence and death of cardiovascular diseases.
Mechanistically, chronic renal failure can induce skeletal muscle atrophy in mice by inhibiting FNDC5 expression and enhancing skeletal muscle autophagy (76). Renal failure can inhibit irisin in muscle and cause the loss of cortical bone mass in mice (77). Peng et al. found that irisin can improve energy metabolism and reduce kidney injury and fibrosis (78). Mechanistically, muscle-specific overexpression of PGC-1α could increase the production of irisin in three models of kidney cell damage. In addition, irisin might correct metabolic reprogramming, increase the production of ATP in damaged kidney cells, and inhibit progressive kidney injury and fibrosis. Dojuksan can improve renal fibrosis through irisin-mediated muscle-kidney crosstalk (79). Furthermore, irisin has also been identified to prevent obesity-related CKD by regulating perirenal adipose tissue function in obese mice (80, 81). Irisin can improve CKD and its complications by regulating muscle-kidney and fat-kidney interactions. Unfortunately, as the typic feature of CKD, the correlation between calcium and phosphorus metabolism disorders and irisin has been poorly studied, which should be the focus of future attention.
Irisin and inflammation
Inflammation plays a critical role in the pathogenesis of vascular calcification. Macrophages or T lymphocytes infiltrating blood vessels are stimulated to produce TNF-α, IL-1β, IL-6, and other pro-inflammatory factors involved in vascular calcification (82, 83). Irisin could lower several inflammatory cytokines and increase anti-inflammatory factors (84). Irisin can reduce inflammatory markers such as MCP1, ICAM1, and VCAM1 in atherosclerotic APOE-/- mice (59). In mechanism, anti-inflammatory properties of irisin are connected with the reduced activity of NF-κB or AMPK signaling pathway activation (85, 86). Irisin could polarize M0 and M1 macrophages towards M2 phenotype by activating AMPK signaling (87). Besides, irisin could promote the differentiation of M2 macrophages by inducing the transcriptional activation of the JAK2-Stat6-dependent PPAR-gamma-associated anti-inflammatory system and NRF2-associated antioxidant genes (88). In addition, irisin alleviates inflammation by restraining the NLRP3 inflammasome and NF-κB pathway (89). Irisin can inhibit the formation of neutrophil extracellular trap and protect the pancreatic injury of mice, further elucidating the protective effect of irisin on acute inflammatory injury (90). Taken together, irisin plays an important role in the resistance to inflammation signaling pathway, providing a theoretical basis for its clinical transformation.
Irisin and oxidative stress
Oxidative stress can further induce mitochondrial dysfunction, endoplasmic reticulum stress, and lysosome dysfunction, leading to vascular calcification (91). At the molecular level, oxidative stress promotes the expression of MSX2, Runx2, SOX9, and ALP in cells and causes vascular calcification (92). In addition, oxidative stress facilitates the occurrence and development of vascular calcification through NADPH oxidase and its downstream ERK signaling pathway (93). By activating Nrf2/HO-1 signaling pathway, irisin could increase the expression of key antioxidant enzymes, such as catalase-9, superoxide dismutase, and glutathione peroxidase, and inhibit the production of hydrogen peroxide, so as to protect vascular endothelial cells from cellular oxidative damage (94). Irisin has been clearly confirmed to have an antioxidant function, and the antioxidant mechanism of irisin is associated with important cellular processes by reducing ROS and its complications, including regulation of mitochondrial fission and fusion, inhibition of inflammasome activation, improvement of autophagy, inhibition of endoplasmic reticulum stress and ferroptosis, and reversal of cell death (95).
Irisin inhibits vascular calcification
In a narrow sense, vascular calcification can be divided into three types according to the location: intima calcification, media calcification, and calcification of the extravascular membrane. Intima calcification is closely associated primarily with atherosclerotic plaque, while media calcification is considered to be the dominant form of vascular calcification in patients with CKD and hypertension (95). Multiple stimuli have been identified to induce endothelial cell dysfunction and VSMCs phenotypic transformation, leading to the development of vascular calcification (96). Previous studies have shown that irisin can relieve vascular calcification by acting on stem cells, vascular endothelial cells, and VSMCs (Table 1, Figure 2).
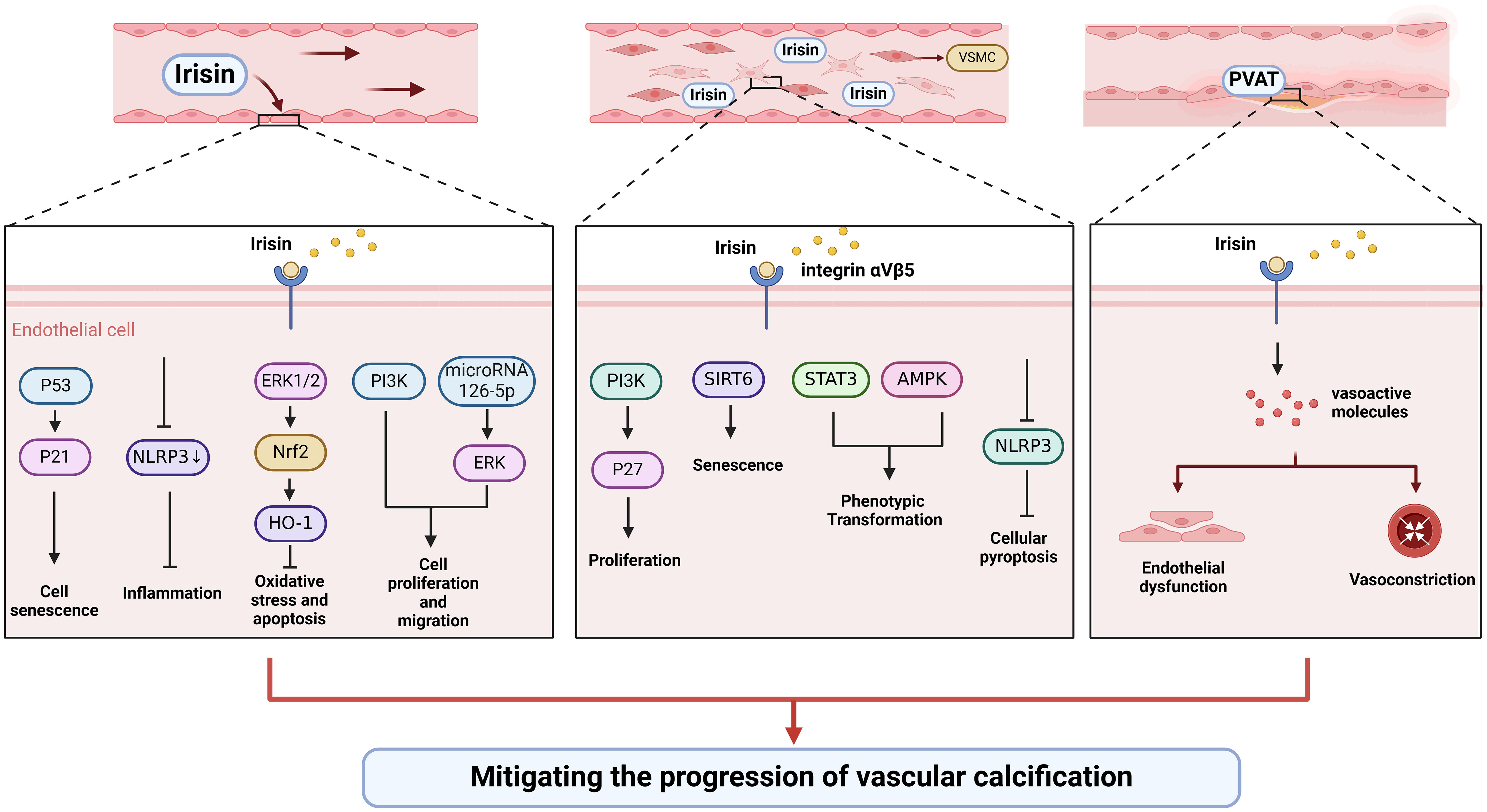
Figure 2 Irisin could alleviate vascular calcification by regulating endothelial cell function and phenotypic switching of VSMCs.
Irisin and vascular endothelial cells
Endothelial progenitor cells (EPCs) could differentiate and mature into endothelial cells, which are involved in vascular formation and repair after vascular endothelial injury (110). In overweight/obese children, circulating irisin is significantly increased, which is significantly associated with circulating EPCs (111). Exercise and dietary interventions could increase irisin levels and EPCs levels in obese adults (112). In addition, irisin could activate PI3K/Akt pathway and increase the number and function of EPCs in diabetic mice (97). Future studies should focus on how irisin modulates vascular calcification by regulating the number and function of EPCs.
More and more evidence shows that endothelial dysfunction is the primary cause of vascular calcification through the transition to mesenchymal and osteoblast lineages, secretion of calcification growth factors, induction of endothelial alkaline phosphatase (113). Improving the function of the vascular endothelium is essential to slow or reverse vascular calcification. Oxidative stress and inflammation are common causes of endothelial injury. Irisin could inhibit the activation of NLRP3 inflammasome and endothelial dysfunction (98, 99). Besides, irisin could improve endothelial function in T2DM by inhibiting oxidative/nitrifying stress (100). Irisin can protect diabetic myocardial microvascular endothelial cells from apoptosis and oxidative stress through ERK1/2/Nrf2/HO-1 pathway (94). In addition to being anti-inflammatory and antioxidant, irisin also inhibits endothelial cell mesenchymal transformation by regulating endothelial cell ROS accumulation and autophagy disorders, thus improving vascular fibrosis (101). Irisin antagonizes vascular endothelial cell proliferation and migration through the integrin αVβ5/PI3K pathway and microRNA126-5p-ERK signaling pathway (102–104). Besides, irisin inhibited endothelial cell senescence and G0/G1 phase cell cycle arrest through the p53/p21 pathway (102). Also, irisin might enhance the angiogenesis of mesenchymal stem cells and human umbilical vein endothelial cells (105, 114–117). Therefore, irisin can protect vascular endothelium in multiple directions.
Irisin and VSMCs
VSMCs are the most abundant cell types, mainly located in the media, and their senescence, apoptosis, and phenotypic transformation have been confirmed to be the main causes of the development of vascular calcification (64). A histological study showed that irisin could increase the intima-media thickness, the number of VSMCs, and elastic layers in the media of the thoracic aorta (118). Irisin might inhibit vascular thickening and VSMCs proliferation through the integrin αVβ5/PI3K/P27 pathway (106). In addition, irisin can reduce the senescence of mouse VSMCs by increasing the stability of SIRT6, and irisin-rich extracellular vesicles can be used to delay vascular aging (107). As a typical feature of vascular calcification, irisin may be involved in the phenotypic transformation of VSMCs. For instance, irisin reversed PDGF-BB-induced VSMCs to secretory transformation through the STAT3 signaling pathway (108). Furthermore, irisin alleviated vascular calcification by activating autophagy and inhibiting NLRP3-mediated VSMCs pyroptosis in CKD (109). Irisin inhibited VSMC osteoblast transformation and mitochondrial dysfunction through AMPK/Drp1 signaling pathway to alleviate vascular calcification in CKD (109). Taken together, irisin might participate in the regulation of VSMCs proliferation, senescence, and phenotypic transformation to delay vascular calcification.
Discussion
Vascular calcification is one of the most difficult problems in cardiovascular diseases, and it is urgent for us to explore effective treatments to reduce adverse events and death and alleviate the burden of disease. Irisin is a so-called “exercise hormone” that is secreted into the circulation in response to stimulation such as physical exercise (119). Current evidence suggests that irisin can protect against hypertension by regulating endothelial function, inflammatory oxidative stress. In addition, irisin can improve insulin resistance, enhance islet function, and promote sugar utilization to optimize blood glucose levels. It also can modify kidney energy metabolism and adipose tissue function to protect kidney function. As a multifunctional cytokine, irisin can be involved in the inhibition of risk factors for vascular calcification through multiple pathways.
Circulating irisin has been shown to be significantly associated with hypertension, diabetes, CKD, and other risk factors for vascular calcification. Moreover, lower serum irisin levels are in connection with a higher prevalence and progression of vascular calcification, including abdominal aortic calcification, coronary artery calcification, and aortic valve calcification (72, 120–122). Therefore, irisin might be used as an indicator of risk factors and vascular calcification. However, more retrospective studies and cohort studies should be performed to confirm its ability and value as a marker.
In addition to being used as an indicator, irisin therapy can also improve hypertension, insulin resistance, etc. While most studies have shown a protective effect of irisin, there are also studies that suggest the opposite. For example, different parts of the injection of irisin have opposite effects on blood pressure. Therefore, the administration site and dose of irisin are worthy of further study. As an exercise-related cytokine, exercise can increase circulating irisin, but it is still unknown whether and how it reaches the lesion site to relieve vascular calcification.
Endothelial dysfunction and phenotypic transformation of VSMCs are two characteristics of vascular calcification. Irisin can directly protect endothelial function by regulating endothelial cell proliferation, senescence, interstitial transformation, and angiogenesis. And irisin can be used to defend against vascular calcification by regulating the proliferation, senescence, pyroptosis, and osteogenesis transformation of VSMCs. Besides, irisin has been identified to improve insulin sensitivity, inhibit bone resorption, and relieve bone-vascular interactions, thereby protecting vascular function (123). These studies elucidate the molecular mechanism of irisin to alleviate vascular calcification. In the future, the relationship between irisin and endothelial cells-VSMCs crosstalk is worth exploring.
Perivascular adipose tissue (PVAT) is a key endocrine organ around blood vessels that could secrete a large amount of metabolic vasoactive factors by endocrine and paracrine means (124). Under physiological conditions, PVAT exerts an anti-vasoconstriction effect by releasing many vasoactive molecules (125). Accumulating evidence points towards that obesity-driven adipose tissue dysfunction promotes chronic inflammatory states within the body, thereby contributing to the pathogenesis of cardiovascular disease (126). Recent research confirmed that irisin can improve the anti-contractile effect and endothelial dysfunction of PVAT in the thoracic aorta of high-fat-fed mice (127, 128). The protective mechanism of irisin may be related to the upregulation of the HO-1/adiponectin axis in PVAT. Therefore, irisin can indirectly mediate the regulation of vascular function by improving the function of PVAT. Furthermore, whether PVAT can secrete irisin to regulate vascular function is also the direction of future research.
Conclusion
In conclusion, irisin has a predictive and protective effect on vascular calcification and its risk factors. Future large clinical randomized controlled studies should be performed to verify the roles of irisin in vascular calcification.
Author contributions
SW: Writing – original draft. SH: Writing – original draft. YP: Writing – original draft, Writing – review & editing.
Funding
The author(s) declare financial support was received for the research, authorship, and/or publication of this article. The research was supported by the grants from: Zhejiang Provincial Program for Medicine and Health (2022KY446, 2023KY411, 2023KY1347), Social Development Science and Technology Foundation of Taizhou (21ywb115, 21ywb118, 20ywb143), Social Development Science and Technology Foundation of Wenling (2020S0180083, 2021S00156, 2021S00197, 2020S0180127).
Conflict of interest
The authors declare that the research was conducted in the absence of any commercial or financial relationships that could be construed as a potential conflict of interest.
Publisher’s note
All claims expressed in this article are solely those of the authors and do not necessarily represent those of their affiliated organizations, or those of the publisher, the editors and the reviewers. Any product that may be evaluated in this article, or claim that may be made by its manufacturer, is not guaranteed or endorsed by the publisher.
References
1. Kang JH, Kawano T, Murata M, Toita R. Vascular calcification and cellular signaling pathways as potential therapeutic targets. Life Sci (2024) 336:122309. doi: 10.1016/j.lfs.2023.122309
2. Jinnouchi H, Sato Y, Sakamoto A, Cornelissen A, Mori M, Kawakami R, et al. Calcium deposition within coronary atherosclerotic lesion: implications for plaque stability. Atherosclerosis (2020) 306:85–95. doi: 10.1016/j.atherosclerosis.2020.05.017
3. Jung CY, Yun HR, Park JT, Joo YS, Kim HW, Yoo TH, et al. Association of coronary artery calcium with adverse cardiovascular outcomes and death in patients with chronic kidney disease: results from the know-ckd. Nephrol Dial Transplant (2023) 38(3):712–21. doi: 10.1093/ndt/gfac194
4. Chen J, Budoff MJ, Reilly MP, Yang W, Rosas SE, Rahman M, et al. Coronary artery calcification and risk of cardiovascular disease and death among patients with chronic kidney disease. JAMA Cardiol (2017) 2(6):635–43. doi: 10.1001/jamacardio.2017.0363
5. Bostrom P, Wu J, Jedrychowski MP, Korde A, Ye L, Lo JC, et al. A Pgc1-alpha-dependent myokine that drives brown-fat-like development of white fat and thermogenesis. Nature (2012) 481(7382):463–8. doi: 10.1038/nature10777
6. Trettel CDS, Pelozin BRA, Barros MP, Bachi ALL, Braga PGS, Momesso CM, et al. Irisin: an anti-inflammatory exerkine in aging and redox-mediated comorbidities. Front Endocrinol (Lausanne) (2023) 14:1106529. doi: 10.3389/fendo.2023.1106529
7. Albrecht E, Norheim F, Thiede B, Holen T, Ohashi T, Schering L, et al. Irisin - a myth rather than an exercise-inducible myokine. Sci Rep (2015) 5:8889. doi: 10.1038/srep08889
8. Erickson HP. Irisin and fndc5 in retrospect: an exercise hormone or a transmembrane receptor? Adipocyte (2013) 2(4):289–93. doi: 10.4161/adip.26082
9. Jedrychowski MP, Wrann CD, Paulo JA, Gerber KK, Szpyt J, Robinson MM, et al. Detection and quantitation of circulating human irisin by tandem mass spectrometry. Cell Metab (2015) 22(4):734–40. doi: 10.1016/j.cmet.2015.08.001
10. Kim H, Wrann CD, Jedrychowski M, Vidoni S, Kitase Y, Nagano K, et al. Irisin mediates effects on bone and fat via alphav integrin receptors. Cell (2018) 175(7):1756–68 e17. doi: 10.1016/j.cell.2018.10.025
11. Bi J, Zhang J, Ren Y, Du Z, Li T, Wang T, et al. Irisin reverses intestinal epithelial barrier dysfunction during intestinal injury via binding to the integrin alphavbeta5 receptor. J Cell Mol Med (2020) 24(1):996–1009. doi: 10.1111/jcmm.14811
12. Estell EG, Le PT, Vegting Y, Kim H, Wrann C, Bouxsein ML, et al. Irisin directly stimulates osteoclastogenesis and bone resorption in vitro and in vivo. Elife (2020) 9. doi: 10.7554/eLife.58172
13. Mu A, Wales TE, Zhou H, Draga-Coleta SV, Gorgulla C, Blackmore KA, et al. Irisin acts through its integrin receptor in a two-step process involving extracellular hsp90alpha. Mol Cell (2023) 83(11):1903–20 e12. doi: 10.1016/j.molcel.2023.05.008
14. Zhang Y, Li R, Meng Y, Li S, Donelan W, Zhao Y, et al. Irisin stimulates browning of white adipocytes through mitogen-activated protein kinase P38 map kinase and Erk map kinase signaling. Diabetes (2014) 63(2):514–25. doi: 10.2337/db13-1106
15. Fatouros IG. Is irisin the new player in exercise-induced adaptations or not? A 2017 update. Clin Chem Lab Med (2018) 56(4):525–48. doi: 10.1515/cclm-2017-0674
16. He X, Hua Y, Li Q, Zhu W, Pan Y, Yang Y, et al. Fndc5/irisin facilitates muscle-adipose-bone connectivity through ubiquitination-dependent activation of runt-related transcriptional factors Runx1/2. J Biol Chem (2022) 298(3):101679. doi: 10.1016/j.jbc.2022.101679
17. Mohammad Rahimi GR, Hejazi K, Hofmeister M. The effect of exercise interventions on irisin level: A systematic review and meta-analysis of randomized controlled trials. EXCLI J (2022) 21:524–39. doi: 10.17179/excli2022-4703
18. Guo Q, Zhang B, Du H, Zhu R, Sun X, Fan X, et al. High-fat diet and palmitate inhibits fndc5 expression via Ampk-Zfp57 pathway in mouse muscle cells. Chem Biol Interact (2023) 369:110265. doi: 10.1016/j.cbi.2022.110265
19. Bao JF, She QY, Hu PP, Jia N, Li A. Irisin, a fascinating field in our times. Trends Endocrinol Metab (2022) 33(9):601–13. doi: 10.1016/j.tem.2022.06.003
20. Aydin S, Kuloglu T, Aydin S, Eren MN, Celik A, Yilmaz M, et al. Cardiac, skeletal muscle and serum irisin responses to with or without water exercise in young and old male rats: cardiac muscle produces more irisin than skeletal muscle. Peptides (2014) 52:68–73. doi: 10.1016/j.peptides.2013.11.024
21. Qin S, Tian Z, Boidin M, Buckley BJR, Thijssen DHJ, Lip GYH. Irisin is an effector molecule in exercise rehabilitation following myocardial infarction (Review). Front Physiol (2022) 13:935772. doi: 10.3389/fphys.2022.935772
22. Wang Y, Liu H, Sun N, Li J, Peng X, Jia Y, et al. Irisin: A promising target for ischemia-reperfusion injury therapy. Oxid Med Cell Longev (2021) 2021:5391706. doi: 10.1155/2021/5391706
23. Fu J, Li F, Tang Y, Cai L, Zeng C, Yang Y, et al. The emerging role of irisin in cardiovascular diseases. J Am Heart Assoc (2021) 10(20):e022453. doi: 10.1161/JAHA.121.022453
24. Li Y, Sun Z, Zhang L, Yan J, Shao C, Jing L, et al. Role of macrophages in the progression and regression of vascular calcification. Front Pharmacol (2020) 11:661. doi: 10.3389/fphar.2020.00661
25. Clemmer JS, Shafi T, Obi Y. Physiological mechanisms of hypertension and cardiovascular disease in end-stage kidney disease. Curr Hypertens Rep (2022) 24(10):413–24. doi: 10.1007/s11906-022-01203-7
26. Brondani LA, Boelter G, Assmann TS, Leitao CB, Canani LH, Crispim D. Irisin-encoding gene (Fndc5) variant is associated with changes in blood pressure and lipid profile in type 2 diabetic women but not in men. Metabolism (2015) 64(9):952–7. doi: 10.1016/j.metabol.2015.05.005
27. Zhang LJ, Xie Q, Tang CS, Zhang AH. Expressions of irisin and urotensin ii and their relationships with blood pressure in patients with preeclampsia. Clin Exp Hypertens (2017) 39(5):460–7. doi: 10.1080/10641963.2016.1273945
28. Wang PX, Fan ZJ, Wu LY, Wang SY, Zhang JL, Dong XT, et al. Serum irisin levels are negatively associated with blood pressure in dialysis patients. Hypertens Res (2023) 46(12):2738–45. doi: 10.1038/s41440-023-01449-x
29. Li B, Yao Q, Guo S, Ma S, Dong Y, Xin H, et al. Type 2 diabetes with hypertensive patients results in changes to features of adipocytokines: leptin, irisin, lgr4, and sfrp5. Clin Exp Hypertens (2019) 41(7):645–50. doi: 10.1080/10641963.2018.1529779
30. Zhu B, Wang B, Zhao C, Wang Y, Zhou Y, Lin J, et al. Irisin regulates cardiac responses to exercise in health and diseases: A narrative review. J Cardiovasc Transl Res (2023) 16(2):430–42. doi: 10.1007/s12265-022-10310-4
31. Fu J, Han Y, Wang J, Liu Y, Zheng S, Zhou L, et al. Irisin lowers blood pressure by improvement of endothelial dysfunction via Ampk-Akt-enos-no pathway in the spontaneously hypertensive rat. J Am Heart Assoc (2016) 5(11). doi: 10.1161/JAHA.116.003433
32. Huang Y, Chen Q, Jiang Q, Zhao Z, Fang J, Chen L. Irisin lowers blood pressure in Zucker diabetic rats by regulating the functions of renal angiotensin ii type 1 receptor via the inhibition of the Nf-kappab signaling pathway. Peptides (2022) 147:170688. doi: 10.1016/j.peptides.2021.170688
33. Li RL, Zhuo CL, Yan X, Li H, Lin L, Li LY, et al. Irisin attenuates vascular remodeling in hypertensive mice induced by ang ii by suppressing ca(2+)-dependent endoplasmic reticulum stress in Vsmcs. Int J Biol Sci (2024) 20(2):680–700. doi: 10.7150/ijbs.84153
34. Celik HT, Akkaya N, Erdamar H, Gok S, Kazanci F, Demircelik B, et al. The effects of valsartan and amlodipine on the levels of irisin, adropin, and perilipin. Clin Lab (2015) 61(12):1889–95. doi: 10.7754/clin.lab.2015.150420
35. Huo CJ, Yu XJ, Sun YJ, Li HB, Su Q, Bai J, et al. Irisin lowers blood pressure by activating the Nrf2 signaling pathway in the hypothalamic paraventricular nucleus of spontaneously hypertensive rats. Toxicol Appl Pharmacol (2020) 394:114953. doi: 10.1016/j.taap.2020.114953
36. Zhang W, Chang L, Zhang C, Zhang R, Li Z, Chai B, et al. Central and peripheral irisin differentially regulate blood pressure. Cardiovasc Drugs Ther (2015) 29(2):121–7. doi: 10.1007/s10557-015-6580-y
37. Aydogdu N, Yalcinkaya Yavuz O, Tastekin E, Tayfur P, Kaya O, Kandemir N. The effects of irisin on nomega-nitro-L-arginine methyl ester hydrochloride-induced hypertension in rats. Balkan Med J (2019) 36(6):337–46. doi: 10.4274/balkanmedj.galenos.2019.2019.5.113
38. Chen K, Zhou M, Wang X, Li S, Yang D. The role of myokines and adipokines in hypertension and hypertension-related complications. Hypertens Res (2019) 42(10):1544–51. doi: 10.1038/s41440-019-0266-y
39. Sow MA, Magne J, Salle L, Nobecourt E, Preux PM, Aboyans V. Prevalence, determinants and prognostic value of high coronary artery calcium score in asymptomatic patients with diabetes: A systematic review and meta-analysis. J Diabetes Complications (2022) 36(8):108237. doi: 10.1016/j.jdiacomp.2022.108237
40. Elkeles RS, Godsland IF, Feher MD, Rubens MB, Roughton M, Nugara F, et al. Coronary calcium measurement improves prediction of cardiovascular events in asymptomatic patients with type 2 diabetes: the predict study. Eur Heart J (2008) 29(18):2244–51. doi: 10.1093/eurheartj/ehn279
41. Zhang H, Wu X, Liang J, Kirberger M, Chen N. Irisin, an exercise-induced bioactive peptide beneficial for health promotion during aging process. Ageing Res Rev (2022) 80:101680. doi: 10.1016/j.arr.2022.101680
42. Espes D, Lau J, Carlsson PO. Increased levels of irisin in people with long-standing type 1 diabetes. Diabetes Med (2015) 32(9):1172–6. doi: 10.1111/dme.12731
43. Du XL, Jiang WX, Lv ZT. Lower circulating irisin level in patients with diabetes mellitus: A systematic review and meta-analysis. Horm Metab Res (2016) 48(10):644–52. doi: 10.1055/s-0042-108730
44. Xuan X, Lin J, Zhang Y, Zhou L, Xu L, Jia J, et al. Serum irisin levels and clinical implication in elderly patients with type 2 diabetes mellitus. J Clin Med Res (2020) 12(9):612–7. doi: 10.14740/jocmr4261
45. Yildiz Kopuz TN, Dagdeviren M, Fisunoglu M. Serum irisin levels in newly diagnosed type-ii diabetic patients: no association with the overall diet quality but strong association with fruit intake. Clin Nutr ESPEN (2022) 49:357–64. doi: 10.1016/j.clnesp.2022.03.022
46. Akyuz A, Mert B, Ozkaramanli Gur D, Mucip Efe M, Aykac H, Alpsoy S, et al. Association of lower serum irisin levels with diabetes mellitus: irrespective of coronary collateral circulation, and syntax score. North Clin Istanb (2021) 8(6):607–14. doi: 10.14744/nci.2021.73669
47. Hou Q, Song R, Zhao X, Yang C, Feng Y. Lower circulating irisin levels in type 2 diabetes mellitus patients with chronic complications: A meta-analysis. Heliyon (2023) 9(11):e21859. doi: 10.1016/j.heliyon.2023.e21859
48. Qiu S, Cai X, Yin H, Zugel M, Sun Z, Steinacker JM, et al. Association between circulating irisin and insulin resistance in non-diabetic adults: A meta-analysis. Metabolism (2016) 65(6):825–34. doi: 10.1016/j.metabol.2016.02.006
49. Shelbaya S, Abu Shady MM, Nasr MS, Bekhet MM, Mageed YA, Abbas M. Study of irisin hormone level in type 2 diabetic patients and patients with diabetic nephropathy. Curr Diabetes Rev (2018) 14(5):481–6. doi: 10.2174/1573399813666170829163442
50. Ulualan G, Kiraz ZK, Kirel B. Relation of serum irisin levels to obesity and non-alcoholic fatty liver disease. Turk J Pediatr (2022) 64(2):246–54. doi: 10.24953/turkjped.2020.3003
51. Shen S, Liao Q, Chen X, Peng C, Lin L. The role of irisin in metabolic flexibility: beyond adipose tissue browning. Drug Discovery Today (2022) 27(8):2261–7. doi: 10.1016/j.drudis.2022.03.019
52. Yano N, Zhang L, Wei D, Dubielecka PM, Wei L, Zhuang S, et al. Irisin counteracts high glucose and fatty acid-induced cytotoxicity by preserving the Ampk-insulin receptor signaling axis in C2c12 myoblasts. Am J Physiol Endocrinol Metab (2020) 318(5):E791–805. doi: 10.1152/ajpendo.00219.2019
53. Liu S, Du F, Li X, Wang M, Duan R, Zhang J, et al. Effects and underlying mechanisms of irisin on the proliferation and apoptosis of pancreatic beta cells. PloS One (2017) 12(4):e0175498. doi: 10.1371/journal.pone.0175498
54. Natalicchio A, Marrano N, Biondi G, Spagnuolo R, Labarbuta R, Porreca I, et al. The myokine irisin is released in response to saturated fatty acids and promotes pancreatic beta-cell survival and insulin secretion. Diabetes (2017) 66(11):2849–56. doi: 10.2337/db17-0002
55. Zheng S, Chen N, Kang X, Hu Y, Shi S. Irisin alleviates ffa induced beta-cell insulin resistance and inflammatory response through activating Pi3k/Akt/Foxo1 signaling pathway. Endocrine (2022) 75(3):740–51. doi: 10.1007/s12020-021-02875-y
56. Xin C, Liu J, Zhang J, Zhu D, Wang H, Xiong L, et al. Irisin improves fatty acid oxidation and glucose utilization in type 2 diabetes by regulating the ampk signaling pathway. Int J Obes (Lond) (2016) 40(3):443–51. doi: 10.1038/ijo.2015.199
57. Huh JY, Mougios V, Kabasakalis A, Fatouros I, Siopi A, Douroudos II, et al. Exercise-induced irisin secretion is independent of age or fitness level and increased irisin may directly modulate muscle metabolism through ampk activation. J Clin Endocrinol Metab (2014) 99(11):E2154–61. doi: 10.1210/jc.2014-1437
58. Ye X, Shen Y, Ni C, Ye J, Xin Y, Zhang W, et al. Irisin reverses insulin resistance in C2c12 cells via the P38-mapk-pgc-1alpha pathway. Peptides (2019) 119:170120. doi: 10.1016/j.peptides.2019.170120
59. Zhang Y, Xie C, Wang H, Foss RM, Clare M, George EV, et al. Irisin exerts dual effects on browning and adipogenesis of human white adipocytes. Am J Physiol Endocrinol Metab (2016) 311(2):E530–41. doi: 10.1152/ajpendo.00094.2016
60. Li H, Zhang Y, Wang F, Donelan W, Zona MC, Li S, et al. Effects of irisin on the differentiation and browning of human visceral white adipocytes. Am J Transl Res (2019) 11(12):7410–21.
61. Tsai YC, Wang CW, Wen BY, Hsieh PS, Lee YM, Yen MH, et al. Involvement of the P62/nrf2/ho-1 pathway in the browning effect of irisin in 3t3-L1 adipocytes. Mol Cell Endocrinol (2020) 514:110915. doi: 10.1016/j.mce.2020.110915
62. Mo L, Shen J, Liu Q, Zhang Y, Kuang J, Pu S, et al. Irisin is regulated by car in liver and is a mediator of hepatic glucose and lipid metabolism. Mol Endocrinol (2016) 30(5):533–42. doi: 10.1210/me.2015-1292
63. Liu TY, Shi CX, Gao R, Sun HJ, Xiong XQ, Ding L, et al. Irisin inhibits hepatic gluconeogenesis and increases glycogen synthesis via the Pi3k/Akt pathway in type 2 diabetic mice and hepatocytes. Clin Sci (Lond) (2015) 129(10):839–50. doi: 10.1042/CS20150009
64. Wang S, Hu S. The role of sirtuins in osteogenic differentiation of vascular smooth muscle cells and vascular calcification. Front Cardiovasc Med (2022) 9:894692. doi: 10.3389/fcvm.2022.894692
65. Wen MS, Wang CY, Lin SL, Hung KC. Decrease in irisin in patients with chronic kidney disease. PloS One (2013) 8(5):e64025. doi: 10.1371/journal.pone.0064025
66. Liu JJ, Liu S, Wong MD, Tan CS, Tavintharan S, Sum CF, et al. Relationship between circulating irisin, renal function and body composition in type 2 diabetes. J Diabetes Complications (2014) 28(2):208–13. doi: 10.1016/j.jdiacomp.2013.09.011
67. Ebert T, Focke D, Petroff D, Wurst U, Richter J, Bachmann A, et al. Serum levels of the myokine irisin in relation to metabolic and renal function. Eur J Endocrinol (2014) 170(4):501–6. doi: 10.1530/EJE-13-1053
68. Yang S, Xiao F, Pan L, Zhang H, Ma Z, Liu S, et al. Association of serum irisin and body composition with chronic kidney disease in obese Chinese adults: A cross-sectional study. BMC Nephrol (2015) 16:16. doi: 10.1186/s12882-015-0009-5
69. Kaluzna M, Hoppe K, Schwermer K, Ibrahim AY, Pawlaczyk K, Ziemnicka K. Adropin and irisin levels in relation to nutrition, body composition, and insulin resistance in patients with end-stage renal disease on chronic hemodialysis and peritoneal dialysis. Pol Arch Med Wewn (2016) 126(7-8):474–82. doi: 10.20452/pamw.3466
70. Rodriguez-Carmona A, Perez Fontan M, Sangiao Alvarellos S, Garcia Falcon T, Pena Bello ML, Lopez Muniz A, et al. Serum levels of the adipomyokine irisin in patients with chronic kidney disease. Nefrologia (2016) 36(5):496–502. doi: 10.1016/j.nefro.2016.05.019
71. Tan Z, Ye Z, Zhang J, Chen Y, Cheng C, Wang C, et al. Serum irisin levels correlated to peritoneal dialysis adequacy in nondiabetic peritoneal dialysis patients. PloS One (2017) 12(4):e0176137. doi: 10.1371/journal.pone.0176137
72. He L, He WY A, Yang WL, Zhang AH. Lower serum irisin levels are associated with increased vascular calcification in hemodialysis patients. Kidney Blood Press Res (2018) 43(1):287–95. doi: 10.1159/000487689
73. Dong X, Fu W, Deng Y, Jia L, Lin N, Li W, et al. Lower serum irisin levels are associated with the increasing mortality of cardiovascular and cerebrovascular diseases in hemodialysis patients. Ann Palliat Med (2021) 10(6):6052–61. doi: 10.21037/apm-21-406
74. Arcidiacono T, Magni G, Macrina L, Sirtori M, Belloni C, Premaschi S, et al. Serum irisin may predict cardiovascular events in elderly patients with chronic kidney disease stage 3-5. J Ren Nutr (2022) 32(3):282–91. doi: 10.1053/j.jrn.2021.05.007
75. Lee MJ, Lee SA, Nam BY, Park S, Lee SH, Ryu HJ, et al. Irisin, a novel myokine is an independent predictor for sarcopenia and carotid atherosclerosis in dialysis patients. Atherosclerosis (2015) 242(2):476–82. doi: 10.1016/j.atherosclerosis.2015.08.002
76. Pan YJ, Zhou SJ, Feng J, Bai Q, LT A, Zhang AH. Urotensin ii induces mice skeletal muscle atrophy associated with enhanced autophagy and inhibited irisin precursor (Fibronectin type iii domain containing 5) expression in chronic renal failure. Kidney Blood Press Res (2019) 44(4):479–95. doi: 10.1159/000499880
77. Kawao N, Kawaguchi M, Ohira T, Ehara H, Mizukami Y, Takafuji Y, et al. Renal failure suppresses muscle irisin expression, and irisin blunts cortical bone loss in mice. J Cachexia Sarcopenia Muscle (2022) 13(1):758–71. doi: 10.1002/jcsm.12892
78. Peng H, Wang Q, Lou T, Qin J, Jung S, Shetty V, et al. Myokine mediated muscle-kidney crosstalk suppresses metabolic reprogramming and fibrosis in damaged kidneys. Nat Commun (2017) 8(1):1493. doi: 10.1038/s41467-017-01646-6
79. Jiang S, Oh DS, Dorotea D, Son E, Kim DS, Ha H. Dojuksan ameliorates tubulointerstitial fibrosis through irisin-mediated muscle-kidney crosstalk. Phytomedicine (2021) 80:153393. doi: 10.1016/j.phymed.2020.153393
80. Han F, Kan C, Wu D, Kuang Z, Song H, Luo Y, et al. Irisin protects against obesity-related chronic kidney disease by regulating perirenal adipose tissue function in obese mice. Lipids Health Dis (2022) 21(1):115. doi: 10.1186/s12944-022-01727-6
81. Hammoud SH, AlZaim I, Al-Dhaheri Y, Eid AH, El-Yazbi AF. Perirenal adipose tissue inflammation: novel insights linking metabolic dysfunction to renal diseases. Front Endocrinol (Lausanne) (2021) 12:707126. doi: 10.3389/fendo.2021.707126
82. Bessueille L, Magne D. Inflammation: A culprit for vascular calcification in atherosclerosis and diabetes. Cell Mol Life Sci (2015) 72(13):2475–89. doi: 10.1007/s00018-015-1876-4
83. Lopez-Mejias R, Gonzalez-Gay MA. Il-6: linking chronic inflammation and vascular calcification. Nat Rev Rheumatol (2019) 15(8):457–9. doi: 10.1038/s41584-019-0259-x
84. Slate-Romano JJ, Yano N, Zhao TC. Irisin reduces inflammatory signaling pathways in inflammation-mediated metabolic syndrome. Mol Cell Endocrinol (2022) 552:111676. doi: 10.1016/j.mce.2022.111676
85. Mazur-Bialy AI, Pochec E, Zarawski M. Anti-inflammatory properties of irisin, mediator of physical activity, are connected with Tlr4/Myd88 signaling pathway activation. Int J Mol Sci (2017) 18(4):701. doi: 10.3390/ijms18040701
86. Jiang X, Hu Y, Zhou Y, Chen J, Sun C, Chen Z, et al. Irisin protects female mice with Lps-induced endometritis through the ampk/nf-kappab pathway. Iran J Basic Med Sci (2021) 24(9):1247–53. doi: 10.22038/ijbms.2021.56781.12678
87. Ye W, Wang J, Lin D, Ding Z. The immunomodulatory role of irisin on osteogenesis via Ampk-mediated macrophage polarization. Int J Biol Macromol (2020) 146:25–35. doi: 10.1016/j.ijbiomac.2019.12.028
88. Tu Y, Liu J, Kong D, Guo X, Li J, Long Z, et al. Irisin drives macrophage anti-inflammatory differentiation via jak2-stat6-dependent activation of ppargamma and nrf2 signaling. Free Radic Biol Med (2023) 201:98–110. doi: 10.1016/j.freeradbiomed.2023.03.014
89. Li Q, Tan Y, Chen S, Xiao X, Zhang M, Wu Q, et al. Irisin alleviates lps-induced liver injury and inflammation through inhibition of nlrp3 inflammasome and nf-kappab signaling. J Recept Signal Transduct Res (2021) 41(3):294–303. doi: 10.1080/10799893.2020.1808675
90. Han F, Ding ZF, Shi XL, Zhu QT, Shen QH, Xu XM, et al. Irisin inhibits neutrophil extracellular traps formation and protects against acute pancreatitis in mice. Redox Biol (2023) 64:102787. doi: 10.1016/j.redox.2023.102787
91. Nguyen NT, Nguyen TT, Park KS. Oxidative stress related to plasmalemmal and mitochondrial phosphate transporters in vascular calcification. Antioxidants (Basel) (2022) 11(3):494. doi: 10.3390/antiox11030494
92. Alesutan I, Feger M, Tuffaha R, Castor T, Musculus K, Buehling SS, et al. Augmentation of phosphate-induced osteo-/chondrogenic transformation of vascular smooth muscle cells by homoarginine. Cardiovasc Res (2016) 110(3):408–18. doi: 10.1093/cvr/cvw062
93. Huang M, Zheng L, Xu H, Tang D, Lin L, Zhang J, et al. Oxidative stress contributes to vascular calcification in patients with chronic kidney disease. J Mol Cell Cardiol (2020) 138:256–68. doi: 10.1016/j.yjmcc.2019.12.006
94. Zhu D, Zhang X, Wang F, Ye Q, Yang C, Liu D. Irisin rescues diabetic cardiac microvascular injury via erk1/2/nrf2/ho-1 mediated inhibition of oxidative stress. Diabetes Res Clin Pract (2022) 183:109170. doi: 10.1016/j.diabres.2021.109170
95. Zhao H, Liu H, Liu Y, Jin J, He Q, Lin B. The role of extracellular vesicles in vascular calcification in chronic kidney disease. Front Med (Lausanne) (2022) 9:997554. doi: 10.3389/fmed.2022.997554
96. Sutton NR, Malhotra R, St Hilaire C, Aikawa E, Blumenthal RS, Gackenbach G, et al. Molecular mechanisms of vascular health: insights from vascular aging and calcification. Arterioscler Thromb Vasc Biol (2023) 43(1):15–29. doi: 10.1161/ATVBAHA.122.317332
97. Zhu G, Wang J, Song M, Zhou F, Fu D, Ruan G, et al. Irisin increased the number and improved the function of endothelial progenitor cells in diabetes mellitus mice. J Cardiovasc Pharmacol (2016) 68(1):67–73. doi: 10.1097/FJC.0000000000000386
98. Deng X, Huang W, Peng J, Zhu TT, Sun XL, Zhou XY, et al. Irisin alleviates advanced glycation end products-induced inflammation and endothelial dysfunction via inhibiting ros-nlrp3 inflammasome signaling. Inflammation (2018) 41(1):260–75. doi: 10.1007/s10753-017-0685-3
99. Xin C, Zhang J, Hao N, Wang J, Liu H, Wei H, et al. Irisin inhibits nlrp3 inflammasome activation in hg/hf incubated cardiac microvascular endothelial cells with H/R injury. Microcirculation (2022) 29(8):e12786. doi: 10.1111/micc.12786
100. Zhu D, Wang H, Zhang J, Zhang X, Xin C, Zhang F, et al. Irisin improves endothelial function in type 2 diabetes through reducing oxidative/nitrative stresses. J Mol Cell Cardiol (2015) 87:138–47. doi: 10.1016/j.yjmcc.2015.07.015
101. Pan JA, Zhang H, Lin H, Gao L, Zhang HL, Zhang JF, et al. Irisin ameliorates doxorubicin-induced cardiac perivascular fibrosis through inhibiting endothelial-to-mesenchymal transition by regulating Ros accumulation and autophagy disorder in endothelial cells. Redox Biol (2021) 46:102120. doi: 10.1016/j.redox.2021.102120
102. Chen J, Li K, Shao J, Lai Z, Gao R, Wang C, et al. Irisin suppresses nicotine-mediated atherosclerosis by attenuating endothelial cell migration, proliferation, cell cycle arrest, and cell senescence. Front Cardiovasc Med (2022) 9:851603. doi: 10.3389/fcvm.2022.851603
103. Bi J, Zhang J, Ren Y, Du Z, Zhang Y, Liu C, et al. Exercise hormone irisin mitigates endothelial barrier dysfunction and microvascular leakage-related diseases. JCI Insight (2020) 5(13). doi: 10.1172/jci.insight.136277
104. Zhang Y, Song H, Zhang Y, Wu F, Mu Q, Jiang M, et al. Irisin inhibits atherosclerosis by promoting endothelial proliferation through microrna126-5p. J Am Heart Assoc (2016) 5(9). doi: 10.1161/JAHA.116.004031
105. Liao Q, Qu S, Tang LX, Li LP, He DF, Zeng CY, et al. Irisin exerts a therapeutic effect against myocardial infarction via promoting angiogenesis. Acta Pharmacol Sin (2019) 40(10):1314–21. doi: 10.1038/s41401-019-0230-z
106. Li K, Chen J, Wang C, Shao J, Lai Z, Yu X, et al. Irisin ameliorates nicotine-mediated atherosclerosis via inhibition of the pi3k pathway. Ann Transl Med (2021) 9(9):805. doi: 10.21037/atm-21-2072
107. Chi C, Fu H, Li YH, Zhang GY, Zeng FY, Ji QX, et al. Exerkine fibronectin type-iii domain-containing protein 5/irisin-enriched extracellular vesicles delay vascular ageing by increasing sirt6 stability. Eur Heart J (2022) 43(43):4579–95. doi: 10.1093/eurheartj/ehac431
108. Song H, Xu J, Lv N, Zhang Y, Wu F, Li H, et al. Irisin reverses platelet derived growth factor-bb-induced vascular smooth muscle cells phenotype modulation through stat3 signaling pathway. Biochem Biophys Res Commun (2016) 479(2):139–45. doi: 10.1016/j.bbrc.2016.07.052
109. Wang PW, Pang Q, Zhou T, Song XY, Pan YJ, Jia LP, et al. Irisin alleviates vascular calcification by inhibiting vsmc osteoblastic transformation and mitochondria dysfunction via Ampk/Drp1 signaling pathway in chronic kidney disease. Atherosclerosis (2022) 346:36–45. doi: 10.1016/j.atherosclerosis.2022.02.007
110. Asahara T, Masuda H, Takahashi T, Kalka C, Pastore C, Silver M, et al. Bone marrow origin of endothelial progenitor cells responsible for postnatal vasculogenesis in physiological and pathological neovascularization. Circ Res (1999) 85(3):221–8. doi: 10.1161/01.res.85.3.221
111. De Meneck F, Victorino de Souza L, Oliveira V, do Franco MC. High irisin levels in overweight/obese children and its positive correlation with metabolic profile, blood pressure, and endothelial progenitor cells. Nutr Metab Cardiovasc Dis (2018) 28(7):756–64. doi: 10.1016/j.numecd.2018.04.009
112. Huang J, Wang S, Xu F, Wang D, Yin H, Lai Q, et al. Exercise training with dietary restriction enhances circulating irisin level associated with increasing endothelial progenitor cell number in obese adults: an intervention study. PeerJ (2017) 5:e3669. doi: 10.7717/peerj.3669
113. Zhang L, Yao J, Yao Y, Bostrom KI. Contributions of the endothelium to vascular calcification. Front Cell Dev Biol (2021) 9:620882. doi: 10.3389/fcell.2021.620882
114. Kan T, He Z, Du J, Xu M, Cui J, Han X, et al. Irisin promotes fracture healing by improving osteogenesis and angiogenesis. J Orthop Translat (2022) 37:37–45. doi: 10.1016/j.jot.2022.07.006
115. Yang F, Wang Z, Li B, He Y, Du F, Tian S, et al. Irisin enhances angiogenesis of mesenchymal stem cells to promote cardiac function in myocardial infarction via Pi3k/Akt activation. Int J Stem Cells (2021) 14(4):455–64. doi: 10.15283/ijsc21005
116. Zhang M, Xu Y, Jiang L. Irisin attenuates oxidized low-density lipoprotein impaired angiogenesis through akt/mtor/S6k1/nrf2 pathway. J Cell Physiol (2019) 234(10):18951–62. doi: 10.1002/jcp.28535
117. Wu F, Song H, Zhang Y, Zhang Y, Mu Q, Jiang M, et al. Irisin induces angiogenesis in human umbilical vein endothelial cells in vitro and in zebrafish embryos in vivo via activation of the erk signaling pathway. PloS One (2015) 10(8):e0134662. doi: 10.1371/journal.pone.0134662
118. Altaweel R, Shatarat A, Badran D, Abu Tarboush NM. The effects of irisin on the rat thoracic aorta: A histological study. Folia Morphol (Warsz) (2022) 81(4):923–30. doi: 10.5603/FM.a2021.0107
119. Tsourdi E, Anastasilakis AD, Hofbauer LC, Rauner M, Lademann F. Irisin and bone in sickness and in health: A narrative review of the literature. J Clin Med (2022) 11(22). doi: 10.3390/jcm11226863
120. Wang SS, Li JM, Hu P, Guo YC, Liu XB, Wang JA, et al. Circulating irisin level as a biomarker for pure aortic stenosis and aortic valve calcification. J Cardiovasc Transl Res (2022) 16(2):443–52. doi: 10.1007/s12265-022-10327-9
121. Zhou SJ, Wang XX, Tang W, Han QF, He L, Zhang AH. Lower serum irisin levels are associated with increased abdominal aortic calcification in peritoneal dialysis patients. Kidney Dis (Basel) (2021) 7(3):219–26. doi: 10.1159/000512514
122. Hisamatsu T, Miura K, Arima H, Fujiyoshi A, Kadota A, Kadowaki S, et al. Relationship of serum irisin levels to prevalence and progression of coronary artery calcification: A prospective, population-based study. Int J Cardiol (2018) 267:177–82. doi: 10.1016/j.ijcard.2018.05.075
123. Csiky B, Sagi B, Emmert V, Wittmann I, Sulyok E. Cardiometabolic effects of irisin in patients with end-stage renal disease on regular hemo- or peritoneal dialysis. Blood Purif (2022) 51(5):450–7. doi: 10.1159/000517529
124. Ozen G, Daci A, Norel X, Topal G. Human perivascular adipose tissue dysfunction as a cause of vascular disease: focus on vascular tone and wall remodeling. Eur J Pharmacol (2015) 766:16–24. doi: 10.1016/j.ejphar.2015.09.012
125. Xia N, Li H. The role of perivascular adipose tissue in obesity-induced vascular dysfunction. Br J Pharmacol (2017) 174(20):3425–42. doi: 10.1111/bph.13650
126. AlZaim I, de Rooij L, Sheikh BN, Borgeson E, Kalucka J. The evolving functions of the vasculature in regulating adipose tissue biology in health and obesity. Nat Rev Endocrinol (2023) 19(12):691–707. doi: 10.1038/s41574-023-00893-6
127. Hou N, Liu Y, Han F, Wang D, Hou X, Hou S, et al. Irisin improves perivascular adipose tissue dysfunction via regulation of the heme oxygenase-1/adiponectin axis in diet-induced obese mice. J Mol Cell Cardiol (2016) 99:188–96. doi: 10.1016/j.yjmcc.2016.09.005
Keywords: irisin, vascular calcification, risk factors, predictor, protective role
Citation: Wang S, Hu S and Pan Y (2024) The emerging roles of irisin in vascular calcification. Front. Endocrinol. 15:1337995. doi: 10.3389/fendo.2024.1337995
Received: 14 November 2023; Accepted: 19 January 2024;
Published: 09 February 2024.
Edited by:
Guanghong Jia, University of Missouri, United StatesReviewed by:
Ibrahim AlZaim, Aarhus University, DenmarkCopyright © 2024 Wang, Hu and Pan. This is an open-access article distributed under the terms of the Creative Commons Attribution License (CC BY). The use, distribution or reproduction in other forums is permitted, provided the original author(s) and the copyright owner(s) are credited and that the original publication in this journal is cited, in accordance with accepted academic practice. No use, distribution or reproduction is permitted which does not comply with these terms.
*Correspondence: Yuping Pan, panyuping3@126.com