- 1Cancer Center, The First Affiliated Hospital of Jilin University, Changchun, China
- 2Department of General Practice, The First Affiliated Hospital of Jilin University, Changchun, China
Background: The association between blood glucose and cognition is controversial. Klotho is an anti-aging protein with neural protective effects. This study aimed to use a population-based study to disentangle the relationship between blood glucose levels and cognitive function in older adults, and to explore the role of klotho in it.
Methods: A total of 1445 eligible participants from National Health and Nutrition Examination Survey (NHANES) 2011-2014 were included in our study. Cognitive function was assessed by Digit Symbol Substitution Test (DSST) and categorized into four quartiles (Q1-Q4). General characteristics and laboratory test results including serum klotho concentration and blood glucose levels were collected. Associations of cognitive function and klotho levels with blood glucose concentrations were explored through multivariate linear regression models. Mediation models were constructed to figure out the mediating role of klotho.
Results: All three multivariate linear regression models showed a negative correlation between blood glucose and cognitive function. (Model 1, β=-0.149, 95%CI: -0.202,-0.096, p=0.001; Model 2, β=-0.116, 95%CI: -0.167,-0.065, p=0.001; Model 3, β=-0.007, 95%CI: -0.118,-0.023, p=0.003). Mediation analysis showed that klotho mediated the statistical association between blood glucose level and cognitive function with proportions (%) of 12.5.
Conclusion: Higher blood glucose levels are associated with poorer cognitive performance in non-diabetic older adults, partially mediated through lower klotho levels.
1 Introduction
With global population aging, cognitive impairment has become an important public health issue (1). Numerous epidemiological studies have demonstrated that diabetes mellitus is one of the key risk factors for cognitive decline (2–4). Moreover, increased blood glucose levels are thought to be associated with cognitive impairment even among people without diabetes (5–7). For example, a prospective study found that a reverse U-shaped relationship was observed between fasting glucose and cognitive function, identifying a threshold for highest cognitive performance at 3.97–6.20 mmol/L fasting glucose (5)., indicating impaired fasting glucose may be associated with cognitive impairment. However, associations between blood glucose and cognition are inconsistent across different populations. A study among older Koreans showed that higher blood glucose was only associated with lower memory but not other cognitive domains (7), suggesting potential ethnic differences. Therefore, the relationship between glucose and cognition merits investigation across different ethnicities in the U.S.
The mechanisms underlying the impact of glucose on cognition remain elusive. Some studies have proposed that abnormalities in insulin signaling may be a key pathway (8, 9). In recent years, klotho, an aging-related protein, has gained considerable attention as a key regulator of the insulin/IGF pathway (10). Klotho increases insulin receptor affinity and enhances insulin sensitivity (11). Moreover, klotho exerts neuroprotective effects (12, 13). Hence, klotho may mediate the detrimental effects of glucose on cognition, but relevant evidence is scarce. Given the controversies over the relationship between blood glucose and cognition across populations and the uncertain mechanisms, research leveraging a representative U.S. cohort to examine the impact of glucose on cognition and the potential intermediary role of klotho is warranted. This will facilitate mechanistic understanding of the importance of glucose control for cognitive health.
2 Methods
2.1 Study population
The data analyzed in this study were from the National Health and Nutrition Examination Survey(NHANES, https://www.cdc.gov/nchs/nhanes/about_nhanes.htm). population. NHANES collects health and nutrition related data through interviews and physical examinations of a representative sample of the civilian non-institutionalized population. Written informed consent was obtained from each participant before participation in this study.
Cognitive function of the older population was measured in two cycles of NHANES, 2011-2012 and 2013-2014. A total of 3472 participants were included in the analysis after excluding participants under 60 years of old (n = 14,906). Subsequently, eligible participants needed to have complete data on cognitive function and blood glucose. 458 and 1569 participants who did not participate in the cognitive function test and did not participate in the blood glucose test were excluded, respectively. This resulted in an analytic sample of 1445 participants (see Figure 1).
2.2 Cognitive function
NHANES performed the Digit Symbol Substitution Test (DSST) for cognitive performance among participants aged 60 years or older. Completing the Digit Symbol Substitution Test (DSST) requires the integrity of executive function, processing speed, attention, spatial perception, and visual scanning cognitive abilities. The exercise is conducted using a paper form that has a key at the top containing 9 numbers paired with symbols. Participants have 2 minutes to copy the corresponding symbols in the 133 boxes that adjoin the numbers. The score is the total number of correct matches. A sample practice test is administered before the participants begin the main test. In NHANES, participants who could not correctly match the symbols with the numbers on their own during the pretest practice did not continue. Details on scoring can be found in the 1999-2000 NHANES CFQ questionnaire data file documentation https://wwwn.cdc.gov/Nchs/Nhanes/1999-2000/CFQ.htm.
2.3 Measurement of serum soluble klotho
Prior to analysis, all samples were stored at -80°C. Quantification of Klotho concentrations was performed using a commercially available enzyme-linked immunosorbent assay (ELISA) kit manufactured by IBL International (Japan). The laboratory methodology employed as well as quality assurance and quality control procedures have been described previously in the NHANES study documentation.
2.4 Measurement of blood glucose
After a 9-hour overnight fast, fasting blood glucose levels were measured in participants the following morning. These examinations were performed in adherence to established protocols for assessing fasting blood glucose.
2.5 Covariates
Covariates evaluated in this analysis included sex (male, female), race/ethnicity (Mexican American, Non-Hispanic Black, Non-Hispanic White, Other), educational attainment (less than high school, high school graduate, college graduate or above), socioeconomic status assessed by Poverty Income Ratio (cite SES source), smoking status (never, former, current), body mass index (<25.0, 25.0-29.9, ≥30.0 kg/m2), and drinking status (never, former, mild, moderate, heavy drinking) based on established categorizations.
2.6 Statistical analysis
To obtain population-representative statistics, participants were stratified into quartiles based on the median score of the Digit Symbol Substitution Test (DSST). Categorical variables were summarized as frequencies and percentages, with p-values from chi-squared tests reported. Continuous variables were expressed as mean ± standard deviation (SD), with p-values from Student’s t-tests presented. Associations of DSST scores and Klotho levels with blood glucose concentrations were explored through multivariate linear regression models.
Direct and indirect effects were evaluated to ascertain the mediating influence of Klotho levels on the relationship between blood glucose and DSST performance. Bootstrapping methodology was employed to determine the statistical significance of the mediation pathway. The magnitude of the mediation effect was quantified as the mediation effect percentage, calculated as (mediation effect/total effect) x 100. Statistical analyses were conducted using R statistical software (version 4.2.2, released 2022-10-31, http://www.r-project.org).
3 Results
Weighted characteristics of the 1445 participants included in the analyses are shown in Table 1. The weighted cognitive function stratified by sex, race, socioeconomic status, education, smoking status, alcohol drinking status, and glucose was statistically significantly different (P < 0.05).
Three multivariate linear regression models were constructed to explore the relationship between glucose and cognitive function (Table 2). In the crude model, the standardization coefficient of high glucose and cognitive function is -0.149(95%CI(-0.202,-0.096), p=0.001). After adjusting for sex and race (model 1), the standardization coefficient of high glucose and cognitive function is -0.116(95%CI(-0.167,-0.065), p=0.001). After further adjustment of education attainment, poverty income ratio, smoking status, and alcohol drinking status (model 2), the standardization coefficient of high glucose and cognitive function is -0.070(95%CI(-0.118,-0.023), p=0.003).
We further investigated the association between klotho and cognitive function (Table 3). After adjusting for all covariates, the results showed that the standardization coefficient between the highest quartile of klotho and cognitive function was 0.091(95%CI 0.031,0.152; p=0.003).
Furthermore, mediation analyses were conducted to explore the mediating effect of klotho. Figure 2 shows the mediating role of klotho in the relationship between glucose and sleep cognitive function. Klotho explained 12.5% of the association(p < 0.001).
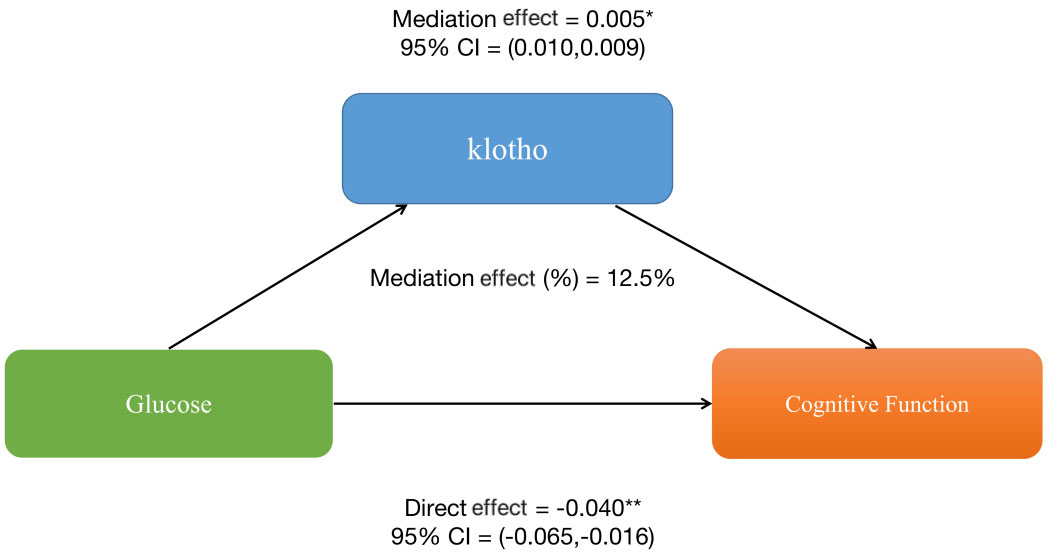
Figure 2 The mediation analysis of klotho on the relationship between glucose and cognitive function.
4 Discussion
4.1 Main findings
In this nationally representative U.S. sample, we validated the association between higher blood glucose levels and poorer cognitive performance measured by DSST even within non-diabetic range. The linear association was independent of potential confounders including socio-demographics, health behaviors, and adiposity. Our findings corroborate results from several previous epidemiological studies showing dose-response relationships between fasting or postprandial blood glucose levels and cognitive deficits in non-diabetic elderly adults (5–7). The persistent adverse impact of elevated glucose on cognition across the spectrum highlights the need for early screening and preventive interventions even before the diagnosis of diabetes.
Importantly, our study provides novel clinical evidence for the intermediary role of klotho in linking chronic hyperglycemia to cognitive decline. We found that higher serum klotho concentrations were independently associated with better DSST scores. Klotho partially mediated the association between elevated glucose and lower DSST performance. To our knowledge, this is the first population-based study discussing the involvement of klotho in glucose-associated cognitive impairment. The findings align with emerging preclinical evidence indicating neuroprotective properties of klotho against neuronal insulin resistance and oxidative stress (13–15). Our study extends the current literature by identifying klotho as a key intermediary linking circulatory glucose disturbance to cognitive aging in a general elderly population.
4.2 Potential mechanisms and clinical implications
4.2.1 Hyperglycemia-induced neuronal damage
Hyperglycemia can inflict neuronal damage through multiple molecular cascades relevant to cognitive decline. Elevated extracellular glucose enhances the formation of advanced glycation end-products (AGEs), which elicit inflammatory responses and oxidative stress in neurons (16). Excessive reactive oxygen species (ROS) production surpasses endogenous antioxidant capacity, resulting in oxidative damage to lipids, proteins and nucleic acids (17). ROS overproduction also activates stress-related signaling molecules including p38 mitogen-activated protein kinase (p38 MAPK) and c-Jun N-terminal kinase (JNK) (18). The activation of p38 MAPK/JNK pathways leads to aberrant hyperphosphorylation of tau, a hallmark of Alzheimer’s disease (19).
Additionally, hyperglycemia disrupts cellular proteostasis by inducing endoplasmic reticulum (ER) stress and mitochondrial dysfunction (20–22). ER stress can elicit neuronal apoptosis through caspase activation. Mitochondrial dysfunction caused by hyperglycemia not only reduces ATP production but also exacerbates ROS generation, establishing a vicious cycle to aggravate oxidative damage in neurons (23). Hyperglycemia also downregulates insulin signaling in the brain by attenuating insulin receptor substrates and Akt signaling, leading to impairments in glucose metabolism and plasticity (24).
These molecular mechanisms represent potential therapeutic targets. Pharmacological agents alleviating oxidative stress, neuroinflammation, ER stress, mitochondrial dysfunction, and insulin resistance could help mitigate hyperglycemia-induced neuronal injury. Lifestyle interventions including exercise, cognitive training, and caloric restriction may also counteract these pathological processes underlying glucose-associated cognitive decline (25–27).
4.2.2 Neuroprotective mechanisms of klotho
Klotho may counteract the detrimental effects of hyperglycemia on cognition through diverse mechanisms (Figure 3). By increasing insulin receptor affinity, klotho can enhance insulin sensitivity and restore neuronal insulin signaling (28, 29). Through regulating redox systems, klotho suppresses ROS generation and inhibits ROS-induced activation of p38 MAPK/JNK pathways, curbing aberrant phosphorylation of tau (11). Previous studies have shown Sirtuin 1 is involved in insulin release and the regulation of klotho. Klotho may antagonize oxidative stress, activate cellular autophagy, and inhibit neuroinflammation to reduce cognitive impairment by affecting the AMPK/SIRT1 signaling pathway, which plays an important role in glucose regulation, neuron proliferation, oxidative stress, and cognition (30–32).. Klotho also upregulates antioxidant enzymes like superoxide dismutase to bolster neuronal defenses against oxidative damage (33).
Moreover, klotho protects against ER stress-induced apoptosis by blocking ASK1 signaling and inducing autophagy (11). Autophagy degrades misfolded proteins accumulated during ER stress to maintain proteostasis. Klotho preserves mitochondrial homeostasis by inhibiting stress-related signaling molecules and apoptosis pathways (34–36). By alleviating hyperglycemia-induced oxidative, ER and mitochondrial stress, klotho maintains neuronal integrity and function.
Our clinical findings complement these experimental studies to support the neuroprotective role of klotho against glucose toxicity. Further research is needed to elucidate the precise molecular events and signaling pathways linking klotho to neuronal resilience. Uncovering these mechanisms may unveil new possibilities for klotho-based therapies.
Strategies to enhance klotho activity, such as caloric restriction, could offer innovative avenues for mitigating cognitive aging related to glucose dysregulation (37). Exercise training increases klotho levels and improves cognition in older adults, likely through a klotho-dependent pathway (38). Some phytochemicals like curcumin and resveratrol have demonstrated ability to upregulate klotho expression (39, 40). Developing nutritional or pharmacological approaches to boost klotho function warrants investigation for dementia prevention and treatment.
4.3 Research strengths, limitations and future directions
Major strengths of the study include the population-based national sample, exploration of a novel intermediary mechanism, and rigorous statistical approach adjusting for potential confounders. However, several limitations should be acknowledged. The cross-sectional nature precludes causal inference. While we selected instrumental variables meeting stringent criteria, residual confounding cannot be excluded. Generalizability to other populations requires further verification. Owing to data constraints, we did not have comprehensive cognitive assessments or neuroimaging biomarkers to enable detailed investigation across cognitive domains.
Future large-scale longitudinal studies incorporating multi-domain cognitive test batteries, neuroimaging, and biomarker assessments are warranted to validate the interrelationships between glucose, klotho, and domain-specific cognitive trajectories. Clinical trials are needed to establish causal impacts of interventions modulating klotho on cognition. Animal models could help elucidate precise molecular mechanisms underlying the cognitive protection afforded by klotho. Elucidating the role of klotho in neuronal maintenance and resilience may uncover innovative prevention opportunities against cognitive aging and dementia related to metabolic disturbance.
5 Conclusion
In summary, our study provides novel clinical evidence that higher blood glucose levels are associated with poorer cognitive performance in non-diabetic older adults, partially mediated through lower klotho levels. Our findings underscore the importance of early glycemic control and highlight klotho as a potential intermediary linking glucose disturbance to cognitive aging. Further research into the links between glucose, klotho and cognition may open promising translational opportunities for dementia prevention.
Data availability statement
The original contributions presented in the study are included in the article/supplementary material. Further inquiries can be directed to the corresponding authors.
Ethics statement
The studies involving humans were approved by National Center for Health Statistics Ethics Review Board. The studies were conducted in accordance with the local legislation and institutional requirements. The participants provided their written informed consent to participate in this study.
Author contributions
XL: Conceptualization, Investigation, Writing – original draft, Data curation, Software. YL: Data curation, Software, Writing – original draft, Formal Analysis, Validation. XC: Formal Analysis, Writing – original draft, Conceptualization, Investigation, Methodology. HY: Resources, Visualization, Writing – review & editing. FL: Data curation, Formal Analysis, Investigation, Software, Writing – review & editing. NC: Investigation, Methodology, Resources, Supervision, Writing – review & editing. JC: Project administration, Resources, Supervision, Validation, Writing – review & editing. WL: Project administration, Supervision, Validation, Writing – review & editing.
Funding
The author(s) declare that no financial support was received for the research, authorship, and/or publication of this article.
Conflict of interest
The authors declare that the research was conducted in the absence of any commercial or financial relationships that could be construed as a potential conflict of interest.
Publisher’s note
All claims expressed in this article are solely those of the authors and do not necessarily represent those of their affiliated organizations, or those of the publisher, the editors and the reviewers. Any product that may be evaluated in this article, or claim that may be made by its manufacturer, is not guaranteed or endorsed by the publisher.
References
1. Prince M, Bryce R, Albanese E, Wimo A, Ribeiro W, Ferri CP. The global prevalence of dementia: a systematic review and metaanalysis. Alzheimer's dementia. (2013) 9.1:63–75. doi: 10.1016/j.jalz.2012.11.007
2. Crane PK, Walker R, Hubbard RA, Li G, Nathan DM, Zheng H, et al. Glucose levels and risk of dementia. New Engl J Med. (2013) 369.6:540–8. doi: 10.1056/NEJMoa1215740
3. Xu WL, Qiu CX, Wahlin A, Winblad B, Fratiglioni L. Diabetes mellitus and risk of dementia in the Kungsholmen project: a 6-year follow-up study. Neurology. (2004) 63.7:1181–6. doi: 10.1212/01.wnl.0000140291.86406.d1
4. Cheng G, Huang C, Deng H, Wang H. Diabetes as a risk factor for dementia and mild cognitive impairment: a meta-analysis of longitudinal studies. Internal Med J. (2012) 42.5:484–91. doi: 10.1111/j.1445-5994.2012.02758.x
5. Liu Z, Zaid M, Hisamatsu T, Tanaka S, Fujiyoshi A, Miyagawa N, et al. Elevated fasting blood glucose levels are associated with lower cognitive function, with a threshold in non-diabetic individuals: A population-based study. J Epidemiol. (2020) 30:121–7. doi: 10.2188/jea.JE20180193
6. Kerti L, Witte AV, Winkler A, Grittner U, Rujescu D, Flöel A. Higher glucose levels associated with lower memory and reduced hippocampal microstructure. Neurology. (2013) 81.20:1746–52. doi: 10.1212/01.wnl.0000435561.00234.ee
7. Yaffe K, Kanaya A, Lindquist K, Simonsick EM, Harris T, Shorr RI, et al. The metabolic syndrome, inflammation, and risk of cognitive decline. Jama. (2004) 292.18:2237–42. doi: 10.1001/jama.292.18.2237
8. Rawlings AM, Sharrett AR, Schneider AL, Coresh J, Albert M, Couper D, et al. Diabetes in midlife and cognitive change over 20 years: a cohort study. Ann Internal Med. (2014) 161.11:785–93. doi: 10.7326/M14-0737
9. Akhtar A, Sah SP. Insulin signaling pathway and related molecules: Role in neurodegeneration and Alzheimer's disease. Neurochem Int. (2020) 135:104707. doi: 10.1016/j.neuint.2020.104707
10. Zeldich E, Chen CD, Colvin TA, Bove-Fenderson EA, Liang J, Tucker Zhou TB, et al. The neuroprotective effect of Klotho is mediated via regulation of members of the redox system. J Biol Chem. (2014) 289.35:24700–15. doi: 10.1074/jbc.M114.567321
11. Brobey RK, German D, Sonsalla PK, Gurnani P, Pastor J, Hsieh CC, et al. Klotho protects dopaminergic neuron oxidant-induced degeneration by modulating ASK1 and p38 MAPK signaling pathways. PloS One. (2010) 10(10):e0139914. doi: 10.1371/journal.pone.0139914
12. Yamamoto M, Clark JD, Pastor JV, Gurnani P, Nandi A, Kurosu H, et al. Regulation of oxidative stress by the anti-aging hormone klotho. J Biol Chem. (2005) 280.45:38029–34. doi: 10.1074/jbc.M509039200
13. Zhang H, Yu L, Yun G. Reduced serum levels of klotho are associated with mild cognitive impairment in patients with type 2 diabetes mellitus. Diabetes Metab Syndr Obes. (2023) 16:129–37. doi: 10.2147/DMSO.S394099
14. Abraham CR, Mullen PC, Tucker-Zhou T, Chen CD, Zeldich E. Klotho is a neuroprotective and cognition-enhancing protein. Vitam Horm. (2016) 101:215–38. doi: 10.1016/bs.vh.2016.02.004
15. Norwitz NG, Saif N, Ariza IE, Isaacson RS. Precision nutrition for alzheimer’s prevention in apoE4 carriers. Nutrients. (2021) 13:1362. doi: 10.3390/nu13041362
16. Ruiz HH, Ramasamy R, Schmidt AM. Advanced glycation end products: building on the concept of the "Common soil" in metabolic disease. Endocrinology. (2020) 161:bqz006. doi: 10.1210/endocr/bqz006
17. Jakubczyk K, Dec K, Kałduńska J, Kawczuga D, Kochman J, Janda K. Reactive oxygen species - sources, functions, oxidative damage. Pol Merkur Lekarski. (2020) 48:124–7.
18. Zhang J, Wang X, Vikash V, Ye Q, Wu D, Liu Y, et al. ROS and ROS-mediated cellular signaling. Oxid Med Cell Longev. (2016) 2016:4350965. doi: 10.1155/2016/4350965
19. Cao M, Liu F, Ji F, Liang J, Liu L, Wu Q, et al. Effect of c-Jun N-terminal kinase (JNK)/p38 mitogen-activated protein kinase (p38 MAPK) in morphine-induced tau protein hyperphosphorylation. Behav Brain Res. (2013) 237:249–55. doi: 10.1016/j.bbr.2012.09.040
20. Wang L, Guo L, Lu L, Sun H, Shao M, Beck SJ, et al. Synaptosomal mitochondrial dysfunction in 5XFAD mouse model of Alzheimer's disease. PloS One. (2016) 11(3):e0150441. doi: 10.1371/journal.pone.0150441
21. Djelti F, Dhenain M, Terrien J, Picq JL, Hardy I, Champeval D, et al. Champeval D,et al. Impaired fasting blood glucose is associated to cognitive impairment and cerebral atrophy in middle-aged non-human primates. Aging (Albany NY). (2016) 9(1):173–86. doi: 10.18632/aging.101148
22. Martins I. Nutrition therapy regulates caffeine metabolism with relevance to NAFLD and induction of type 3 diabetes. J Diabetes Metab Disord. (2017) 4:019. doi: 10.24966/DMD-201X
23. Rizwan H, Pal S, Sabnam S, Pal A. High glucose augments ROS generation regulates mitochondrial dysfunction and apoptosis via stress signalling cascades in keratinocytes. Life Sci. (2020) 241:117148. doi: 10.1016/j.lfs.2019.117148
24. Maiese K. New insights for oxidative stress and diabetes mellitus. Oxid Med Cell Longev. (2015) 2015:875961. doi: 10.1155/2015/875961
25. Pifferi F, Aujard F. Caloric restriction, longevity and aging: Recent contributions from human and non-human primate studies. Prog Neuropsychopharmacol Biol Psychiatry. (2019) 95:109702. doi: 10.1016/j.pnpbp.2019.109702
26. Kivipelto M, Mangialasche F, Ngandu T. Lifestyle interventions to prevent cognitive impairment, dementia and Alzheimer disease. Nat Rev Neurol. (2018) 14:653–66. doi: 10.1038/s41582-018-0070-3
27. Dominguez LJ, Veronese N, Vernuccio L, Catanese G, Inzerillo F, Salemi G, et al. Nutrition, physical activity, and other lifestyle factors in the prevention of cognitive decline and dementia. Nutrients. (2021) 13:4080. doi: 10.3390/nu13114080
28. Geng L, Liao B, Jin L, Yu J, Zhao X, Zhao Y, et al. β-Klotho promotes glycolysis and glucose-stimulated insulin secretion via GP130. Nat Metab. (2022) 4:608–26. doi: 10.1038/s42255-022-00572-2
29. Xu Y, Sun Z. Molecular basis of klotho: from gene to function in aging. Endocr Rev. (2015) 36.2:174–93. doi: 10.1210/er.2013-1079
30. Su H, Gao D, Chen Y, Zuo Z. The relationship between klotho and SIRT1 expression in renal aging related disease. Int J Gen Med. (2022) 15:7885–93. doi: 10.2147/IJGM.S384119
31. Gao D, Zuo Z, Tian J, Ali Q, Lin Y, Lei H, et al. Activation of SIRT1 attenuates klotho deficiency-induced arterial stiffness and hypertension by enhancing AMP-activated protein kinase activity. Hypertension. (2016) 68:1191–9. doi: 10.1161/HYPERTENSIONAHA.116.07709
32. Rostamzadeh F, Moosavi-Saeed Y, Yeganeh-Hajahmadi M. Interaction of klotho and sirtuins. Exp Gerontol. (2023) 182:112306. doi: 10.1016/j.exger.2023.112306
33. Zeldich E, Chen CD, Boden E, Howat B, Nasse JS, Zeldich D, et al. Klotho is neuroprotective in the superoxide dismutase (SOD1G93A) mouse model of ALS. J Mol Neurosci. (2019) 69:264–85. doi: 10.1007/s12031-019-01356-2
34. Ikushima M, Rakugi H, Ishikawa K, Maekawa Y, Yamamoto K, Ohta J, et al. Anti-apoptotic and anti-senescence effects of Klotho on vascular endothelial cells. Biochem Biophys Res Commun. (2006) 339.3:827–32. doi: 10.1016/j.bbrc.2005.11.094
35. James MI. Single gene inactivation with implications to diabetes and multiple organ dysfunction syndrome. J Clin Epigenet. (2017) 3:24. doi: 10.21767/2472-1158
36. Martins IJ. Anti-aging genes improve appetite regulation and reverse cell senescence and apoptosis in global populations. Adv Aging Res. (2016) 5:9–26. doi: 10.4236/aar.2016.51002
37. Hu M-C, Shiizaki K, Kuro-o M, Moe OW. Fibroblast growth factor 23 and klotho: physiology and pathophysiology of an endocrine network of mineral metabolism. Annu Rev Physiol. (2013) 75:503. doi: 10.1146/annurev-physiol-030212-183727
38. Prud'homme GJ, Kurt M, Wang Q. Pathobiology of the klotho antiaging protein and therapeutic considerations. Front Aging. (2022) 3:931331. doi: 10.3389/fragi.2022.931331
39. Mansoor SR, Hashemian M, Khalili-Fomeshi M, Ashrafpour M, Moghadamnia AA, Ghasemi-Kasman M. Upregulation of klotho and erythropoietin contributes to the neuroprotection induced by curcumin-loaded nanoparticles in experimental model of chronic epilepsy. Brain Res Bull. (2018) 142:281–8. doi: 10.1016/j.brainresbull.2018.08.010
Keywords: glucose, klotho, cognitive function, cognitive impairment, hyperglycemia
Citation: Liu X, Li Y, Chen X, Yin H, Li F, Chen N, Cui J and Li W (2024) Revisiting the mechanisms linking blood glucose to cognitive impairment: new evidence for the potential important role of klotho. Front. Endocrinol. 15:1323407. doi: 10.3389/fendo.2024.1323407
Received: 17 October 2023; Accepted: 21 February 2024;
Published: 05 March 2024.
Edited by:
Lei Sha, China Medical University, ChinaReviewed by:
Ian James Martins, University of Western Australia, AustraliaDorota Formanowicz, Poznan University of Medical Sciences, Poland
Copyright © 2024 Liu, Li, Chen, Yin, Li, Chen, Cui and Li. This is an open-access article distributed under the terms of the Creative Commons Attribution License (CC BY). The use, distribution or reproduction in other forums is permitted, provided the original author(s) and the copyright owner(s) are credited and that the original publication in this journal is cited, in accordance with accepted academic practice. No use, distribution or reproduction is permitted which does not comply with these terms.
*Correspondence: Naifei Chen, Y2hlbm5haWZlaUBqbHUuZWR1LmNu; Jiuwei Cui, Y3VpandAamx1LmVkdS5jbg==; Wei Li, bGl3ZWk2NkBqbHUuZWR1LmNu
†These authors have contributed equally to this work and share first authorship