Introduction
Insulin, a polypeptide hormone that is produced in the β-cells of the pancreatic islets of Langerhans, has multifaceted effects on metabolism in virtually all tissues. Insulin facilitates glucose entry into cells, stimulates glycogen formation in liver and muscle, and enhances fat and protein synthesis (1). Insulin also has a mitogenic function, stimulating cell growth and proliferation (2). By crossing the blood-brain barrier, insulin can affect feeding and cognition through CNS mechanisms (3). Binding of insulin to its receptor tyrosine kinase (RTK) triggers signal transduction, with phosphorylation of cellular substrates (IRS) and activation of phosphatidylinositol-3-kinase (PI3K), which initiates a chain of events directly involved in the metabolic and mitogenic effects of insulin (1, 4). The second pathway involves activation of mitogen-activated protein kinases (MAPK), which play a predominant role in the control of mitogenic effects of insulin (5). Disruption of the IRS/PI3K pathway leads to decreased tissue sensitivity to the metabolic action of insulin - a state of insulin resistance (IR), which is characteristic of patients with type 2 diabetes (T2D), obesity and arterial hypertension (6, 7).
The renin-angiotensin system (RAS) contributes to the pathophysiology of IR – thus angiotensin II (ANGII) disrupts insulin signaling by promoting phosphorylation of the insulin receptor and IRS-1 and PI3K which impairs their function (8–11). Given the close relationship between these signaling systems, we hypothesize that insulin itself may influence the RAS and regulate its function. We also discuss possible pathological consequences of RAS dysfunction due to impaired insulin signaling relevant to IR and diabetes complications.
Briefly about RAS - components and function
As a universal regulatory system, the RAS ensures normal functioning of basic life processes such as hemodynamics, fluid-salt and glucose homeostasis, behavior, and also participates in the regulation of immunity, apoptosis, inflammation and many others (10, 12–14). The major peptide effector of RAS, ANGII, is formed from the protein precursor angiotensinogen (AGT) by proteolytic processing involving renin and angiotensin-converting enzyme (ACE) (12). ANGII acts through two subtypes of G-protein-coupled receptors (GPCRs), the AT1R and AT2R receptors. Alternative processing of AGT involving ACE2 results in the formation of other angiotensins - ANG-(1-7) and ANGIV, which have their own receptors, Mas-receptor and IRAP (insulin-regulated aminopeptidase), respectively (12, 14–17).
Physiological effects of RAS are achieved through the interaction of counterbalancing activity pathways: “pathophysiological” ACE/ANGII/AT1R, whose activation is associated with hypertension, oxidative stress, hypertrophy, fibrosis and inflammation, and “protective” ACE/ANGII/AT2R, which has the opposite function, including hypotensive and anti-inflammatory effects (18). Alternative pathways involving ANG-(1-7) and AngIV in general function within the “protective” RAS axis (15–17).
In addition to the central RAS circulating in the blood system, there are local RAS in the brain, pancreas, kidneys, intestines, etc. involved in the regulation of the functional activity of these organs (18–21). Thus, pancreatic RAS, represented by a full set of functional components, including ANGII and ANG-(1-7) and their receptors, affects cell proliferation, apoptosis, oxidative stress, and inflammation (21–23). It has been shown that islet RAS regulates insulin biosynthesis and secretion, affects the mass of islet β−cells and is able to alter glucose homeostasis (24).
Evidence of the effect of insulin on the activity of RAS components
Insulin has a significant effect on RAS function by affecting the expression, secretion, and activity of its components (Table 1, Figure 1A).
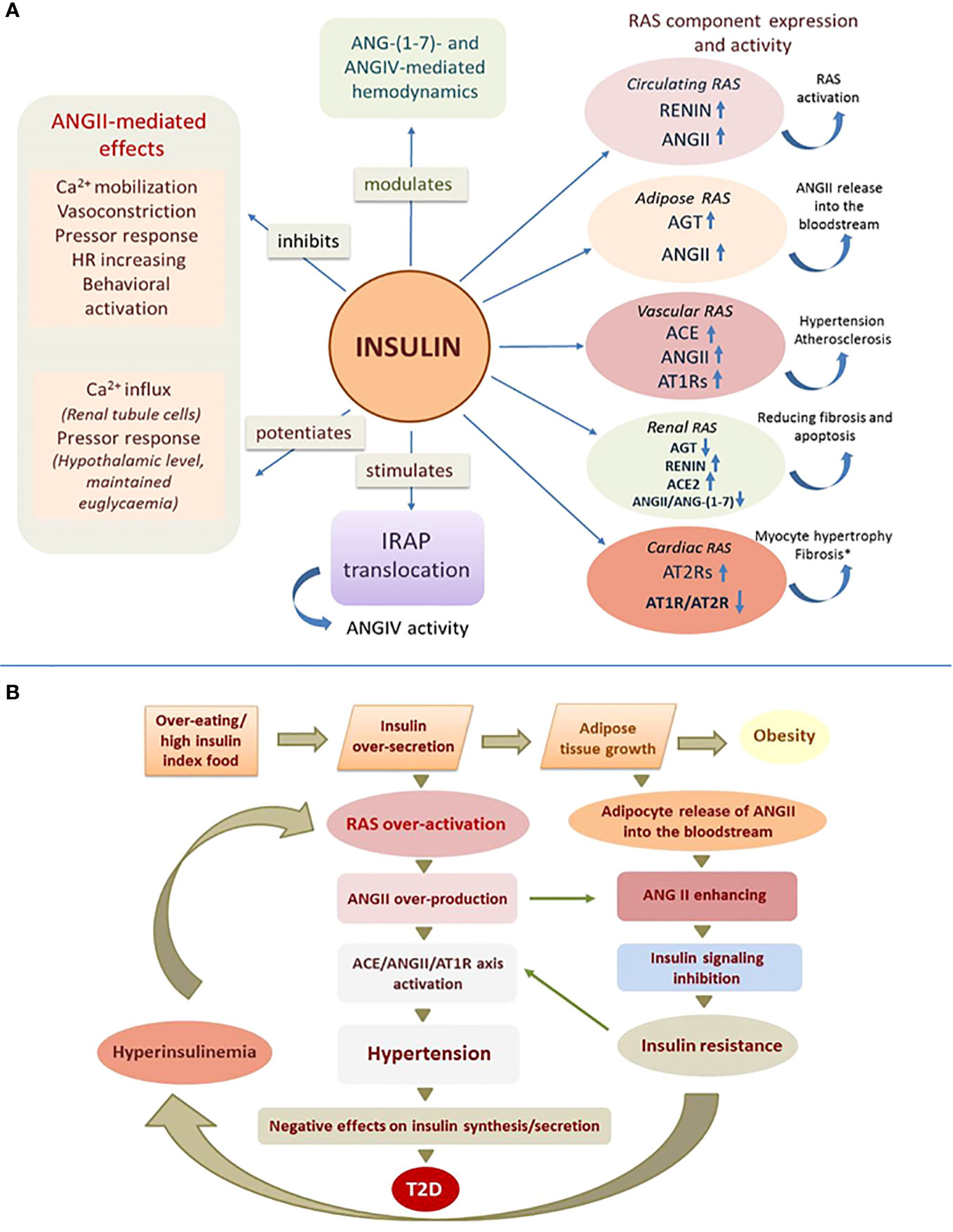
Figure 1 Effect of insulin on RAS function. (A) Insulin affects central and local RAS by acting on their receptors, enzymes and angiotensin peptides (experimentally confirmed effects, details in the text of the article); ANG, angiotensin; AGT, angiotensinogen; ACE, angiotensin-converting enzyme; AT1R and AT2R, angiotensin receptors; IRAP, insulin-regulated aminopeptidase; (↑) – increasing; (↓) – reducing; (*) - high concentrations of insulin. (B) Mechanism of angiotensin-dependent IR as a vicious circle.
Insulin promotes an increase in circulating ANGII levels
Insulin increases circulating ANGII levels by stimulating plasma renin activity (25) and also increases AGT expression and ANGII secretion in subcutaneous adipose tissue, which is an important source of circulating ANGII (26).
Insulin affects the expression of components of local RASs
In renal RAS, insulin stimulates renin production (renal cortex) (27), and suppresses AGT expression by interacting with a specific insulin-responsive element located in the promoter region of the AGT gene (proximal tubule cells) (28). In cultured podocytes, insulin increases the expression of ACE2, responsible for the production of ANG-(1-7), a functional ANGII antagonist, which shifts the ANGII/ANG-(1-7) ratio in favor of an “anti-ANG II” profile and contributes to reduced fibrosis and apoptosis (29).
In the vascular RAS (aortic endothelial cells) insulin suppresses AGT and renin expression but stimulates ACE biosynthesis (30). Insulin also induces AT1R overexpression in blood vessels, which may lead to increased biological efficacy of ANGII and thereby induce hypertension and atherosclerosis (31). In cardiac tissue, high concentrations of insulin cause the opposite effect, altering the AT1R/AT2R ratio in favor of an increase in AT2R, leading to pathological changes in the organ (32).
Insulin modulates the action of angiotensin peptides
Insulin reduces ANGII-induced Ca2+ response in various cell types such as human skin fibroblasts and mesangial cells (33, 34) as well as in vascular smooth muscle cells (VSMCs) of various origins (34–37). In rat renal tissue (cortical thick ascending limb), insulin does not alter ANGII-induced Ca2+ release from intracellular reservoirs but stimulates Ca2+ influx (38). Insulin dose-dependently suppresses the vasoconstrictive effects of ANGII in arterial and venous systems, suggesting that insulin may modulate ANGII-mediated vascular function (39).
Intraperitoneal administration of 1 U of insulin abolishes ANGII-mediated activation of complex instrumental behavior in rats and halves the hypertensive effect of ANGII (40). Continuous insulin infusion (i.v.) reduces the pressor response to ANGII in rats and, conversely, increases it if euglycemia is artificially maintained (41). Centrally administered (i.c.v.) insulin potentiates ANGII pressor effects at the hypothalamic level (42).
Insulin regulates the activity of ANGII bioactive derivatives, ANG-(1-7) and ANGIV, which elicit responses generally opposite to ANGII. When rats were injected intraperitoneally with 1 U of insulin, the weak hypotensive effect of ANG-(1-7) changed into a biphasic hyper-hypotensive effect (43). In a similar experiment, the weak hypotensive effect of ANGIV on the background of insulin was changed to hypertensive (increase in blood pressure by 11-16%) with subsequent prolonged vasodilatation and tachyarrhythmia (44).
Insulin regulates IRAP function
The specific ANGIV receptor, IRAP, is an insulin-regulated aminopeptidase with which ANGIV interacts as an inhibitor (45). Expressed in the brain, heart, kidney, and blood vessels, IRAP is involved in the regulation of blood flow, glucose metabolism, and processes related to cognition, as well as several other functions (46). Insulin stimulates the translocation of IRAP together with the insulin-responsive glucose transporter GLUT4 from intracellular vesicles to the cell membrane, where IRAP performs its function by cleaving vasopressin, somatostatin and other active peptides (47).
Discussion
The study of the functional relationship between insulin and renin-angiotensin signaling systems, which play a key role in the control metabolism and homeostasis, seems particularly important due to the confirmed clinical association between IR and hypertension (48). Insulin signaling system and RAS are in a balanced relationship of reciprocal regulation, where the activity of each system depends to a certain extent on the functioning of the other. Thus, ANGII regulates insulin secretion by activating AT1Rs on the surface of β−cells (24) and plays an important role in the development of IR, which occurs in various pathological conditions, including diabetic (8–10). Insulin, in turn, affects central and local RASs by acting on their receptors, enzymes, and angiotensin effector peptides (Figure 1A).
We suggest that RAS activity is largely dependent on the state of the insulin signaling system and this may be extremely important for both normal body functioning and the development of pathology. This regulation can be flexible and efficient under physiological conditions and dramatically impaired under pathological conditions. Normally, insulin can increase ANGII concentrations and at the same time counterbalance the hyperactivity of this hormone by suppressing its effects, causing a kind of temporary “insulin-dependent resistance to ANGII”. Possible mechanisms for the inhibitory effect of insulin on ANGII signaling in this case could be chemical modification and inactivation of angiotensin receptors, as insulin-activated RTKs are able to phosphorylate GPCRs (49) or “borrow” components of GPCR signaling, including β-arrestins and G-protein-coupled kinases (50). In the absence of the hypoglycemic effect of insulin, which is characteristic of the IR state, insulin may, in contrast, enhance the hypertensive properties of ANGII (41) and thereby contribute to the hypertension that usually accompanies IR.
RAS hyperactivation is characteristic of the IR state, but in this case a chicken-or-egg situation may be observed (51). We propose to consider the mechanism of development of IR as a vicious circle (Figure 1B), which is triggered by any causes that contributes to a chronic increase in insulin release (overeating, preference for high insulin index foods such as sweets, baked goods, etc.). Excess insulin secretion stimulates overproduction of ANGII in circulating and local RASs and overgrowth of adipose tissue, which in response to insulin increases synthesis and release of ANGII into the bloodstream, further increasing its concentration (25). Since ANGII can inhibit insulin signaling, its ever-increasing levels contribute to IR. Under conditions of IR, insulin inadequately regulates glucose homeostasis and other metabolic processes due to disruption of the IRS/PI3K signaling pathway, whereas MAPK-pathway signaling is preserved, which contributes to the maintenance or even enhancement of other, non-metabolic effects of insulin (5). In these conditions insulin can affect tissues that are not directly involved in metabolism and retain insulin reactivity, thereby causing specific responses with potentially dangerous consequences including arterial hypertension (5). Because insulin promotes activation of predominantly prohypertensive components of the RAS, hypertension in patients with IR may be largely related to hyperactivation of the RAS due to compensatory hyperinsulinemia accompanying IR. Further activation of the ACE/ANGII/AT1R axis under these conditions, especially in the vascular endothelium, aggravates hypertension characteristic of obesity and IR. Excess ANGII levels in blood and tissues in IR promote profibrotic, inflammatory and hypertrophic processes causing remodeling and dysfunction of cardiovascular and renal tissues. On the other hand, the state of IR is characterized by chronic vasoconstriction in the area of insulin secretion due to high expression of AT1Rs of islet RAS on the background of high levels of ANGII, which dramatically reduces islet blood flow and negatively affects insulin synthesis and release, leading to glucose intolerance (22, 52). Further aggravation of disturbed insulin signaling in the presence of increasing ANGII, eventually leads to metabolic syndrome and T2D (53). We believe that our results may illuminate the relationship between T2D, cardiovascular disease and renal dysfunction, whose molecular mechanisms include among others IR and hyperactivity of the RAS (54).
Each of the angiotensin peptides can cause different, even opposite effects - for example, the specific action of ANGII is determined by the type of receptor it interacts with - AT1R or AT2R (17). ANGIV and ANG-(1-7), which have their own receptors, can also be AT1R and/or AT2R agonists and, depending on concentration, exert both AT1R-mediated vasoconstrictor and AT2R-mediated vasodilator effects (55–57). Insulin, by influencing the expression and function of various angiotensin receptors, may be a factor regulating their effective concentration and availability for ligands, which ultimately determines the spectrum of physiologic effects of various angiotensin peptides and maintains a physiologically adequate balance between the “pathophysiologic” and “protective” branches of the RAS.
Most hormones, including insulin, have a window of optimal physiological concentrations (58). Secretory deficiency or excess of insulin, as well as impaired signaling leading to insulin dysfunction, can disrupt the balance of regulatory interactions between insulin and RAS. When drugs affecting insulin secretion and signal transduction (insulin secretagogues and sensitizers) or insulin therapy are used, the functional activity of the RAS may shift towards the “pathophysiological” axis (59–62). This may be the cause of increased blood pressure during experimental sustained hyperinsulinemia (63) as well as the development of hypertension in pregnant women during insulin therapy for gestational diabetes mellitus (64). Various complications such as angiopathy, nephropathy, retinopathy, neuropathy, inflammation, etc., characteristic of the diabetic state (65–67) may in fact result from RAS dysregulation against insulin dysfunction due to pathology and/or medication.
In our opinion, insulin is directly involved in the flexible regulation of RAS by influencing the expression and activity of its enzymes, effector peptides and receptors. Impaired insulin signaling can lead to dysregulation of RAS function resulting in serious complications characteristic of the diabetic state. We hypothesize that IR is a consequence of RAS hyperactivation, which is provoked by excessive insulin secretion promoted by poor diet and/or other causes. Normalization of insulin secretion, primarily through dietary correction, as well as pharmacotherapy that restores the functional balance of insulin and renin-angiotensin signaling systems seem essential for the prevention and treatment of IR, hypertension and diabetic complications.
Author contributions
TZ: Conceptualization, Validation, Writing – original draft. ST: Supervision, Writing – original draft, Conceptualization. AK: Data curation, Supervision, Validation, Writing – review & editing.
Funding
The author(s) declare that no financial support was received for the research, authorship, and/or publication of this article.
Conflict of interest
The authors declare that the research was conducted in the absence of any commercial or financial relationships that could be construed as a potential conflict of interest.
Publisher’s note
All claims expressed in this article are solely those of the authors and do not necessarily represent those of their affiliated organizations, or those of the publisher, the editors and the reviewers. Any product that may be evaluated in this article, or claim that may be made by its manufacturer, is not guaranteed or endorsed by the publisher.
References
1. Norton L, Shannon C, Gastaldelli A, DeFronzo RA. Insulin: The master regulator of glucose metabolism. Metabolism (2022) 129:155142. doi: 10.1016/j.metabol.2022.155142
2. Ish-Shalom D, Christoffersen CT, Vorwerk P, Sacerdoti-Sierra N, Shymko RM, Naor D, et al. Mitogenic properties of insulin and insulin analogues mediated by the insulin receptor. Diabetologia (1997) 40 Suppl 2:S25–31. doi: 10.1007/s001250051393
3. Banks WA, Owen JB, Erickson MA. Insulin in the brain: there and back again. Pharmacol Ther (2012) 136:82–93. doi: 10.1016/j.pharmthera.2012.07.006
4. Cheatham B, Kahn CR. Insulin action and the insulin signaling network. Endocr Rev (1995) 16:117–42. doi: 10.1210/edrv-16-2-117
5. Brosolo G, Da Porto A, Bulfone L, Vacca A, Bertin N, Scandolin L, et al. Insulin resistance and high blood pressure: mechanistic insight on the role of the kidney. Biomedicines (2022) 10:2374. doi: 10.3390/biomedicines10102374
6. Li M, Chi X, Wang Y, Setrerrahmane S, Xie W, Xu H. Trends in insulin resistance: insights into mechanisms and therapeutic strategy. Signal Transduct Target Ther (2022) 7:216. doi: 10.1038/s41392-022-01073-0
7. Di Giuseppe G, Ciccarelli G, Soldovieri L, Capece U, Cefalo CMA, Moffa S, et al. First-phase insulin secretion: can its evaluation direct therapeutic approaches? Trends Endocrinol Metab (2023) 27:216–30. doi: 10.1016/j.tem.2023.02.001. S1043-2760(23)00030-9.
8. Underwood PC, Adler GK. The renin angiotensin aldosterone system and insulin resistance in humans. Curr Hypertens Rep (2013) 15:59–70. doi: 10.1007/s11906-012-0323-2
9. Velloso LA, Folli F, Perego L, Saad MJ. The multi-faceted cross-talk between the insulin and angiotensin II signaling systems. Diabetes Metab Res Rev (2006) 22:98–107. doi: 10.1002/dmrr.611
10. Perkins JM, Davis SN. The renin-angiotensin-aldosterone system: a pivotal role in insulin sensitivity and glycemic control. Curr Opin Endocrinol Diabetes Obes (2008) 15:147–52. doi: 10.1097/MED.0b013e3282f7026f
11. Valente V, Izzo R, Manzi MV, De Luca MR, Barbato E, Morisco C. Modulation of insulin resistance by renin angiotensin system inhibitors: implications for cardiovascular prevention. Monaldi Arch Chest Dis (2021) 91(2). doi: 10.4081/monaldi.2021.1602
12. Forrester SJ, Booz GW, Sigmund CD, Coffman TM, Kawai T, Rizzo V, et al. Angiotensin II signal transduction: an update on mechanisms of physiology and pathophysiology. Physiol Rev (2018) 98:1627–738. doi: 10.1152/physrev.00038.2017
13. Gong X, Hu H, Qiao Y, Xu P, Yang M, Dang R, et al. The involvement of renin-angiotensin system in lipopolysaccharide-induced behavioral changes, neuroinflammation, and disturbed insulin signaling. Front Pharmacol (2019) 10:318. doi: 10.3389/fphar.2019.00318
14. Cantero-Navarro E, Fernández-Fernández B, Ramos AM, Rayego-Mateos S, Rodrigues-Diez RR, Sánchez-Niño MD, et al. Renin-angiotensin system and inflammation update. Mol Cell Endocrinol (2021) 529:111254. doi: 10.1016/j.mce.2021.111254
15. Santos RAS, Oudit GY, Verano-Braga T, Canta G, Steckelings UM, Bader M. The renin-angiotensin system: going beyond the classical paradigms. Am J Physiol Heart Circ Physiol (2019) 316:H958–70. doi: 10.1152/ajpheart.00723.2018
16. Iwai M, Horiuchi M. Devil and angel in the renin-angiotensin system: ACE-angiotensin II-AT1 receptor axis vs. ACE2-angiotensin-(1-7)-Mas receptor axis. Hypertens Res (2009) 32:533–6. doi: 10.1038/hr.2009.74
17. Paz Ocaranza M, Riquelme JA, García L, Jalil JE, Chiong M, Santos RAS, et al. Counter-regulatory renin-angiotensin system in cardiovascular disease. Nat Rev Cardiol (2020) 17:116–29. doi: 10.1038/s41569-019-0244-8
18. Urmila A, Rashmi P, Nilam G, Subhash B. Recent advances in the endogenous brain renin-angiotensin system and drugs acting on it. J Renin Angiotensin Aldosterone Syst (2021) 2021:9293553. doi: 10.1155/2021/9293553
19. Ichihara A, Kobori H, Nishiyama A, Navar LG. Renal renin-angiotensin system. Contrib Nephrol (2004) 143:117–1130. doi: 10.1159/000078716
20. Zamolodchikova TS, Shoibonov BB, Tolpygo SM. Local renin-angiotensin system of small intestine. Eksp Klin Gastroenterol (2016) 12:97–104.
21. Leung PS. The physiology of a local renin-angiotensin system in the pancreas. J Physiol (2007) 580:31–7. doi: 10.1113/jphysiol.2006.126193
22. Cheng Q, Leung PS. An update on the islet renin-angiotensin system. Peptides (2011) 32:1087–95. doi: 10.1016/j.peptides.2011.03.003
23. Graus-Nunes F, Souza-Mello V. The renin-angiotensin system as a target to solve the riddle of endocrine pancreas homeostasis. BioMed Pharmacother (2019) 109:639–45. doi: 10.1016/j.biopha.2018.10.191
24. Ramracheya RD, Muller DS, Wu Y, Whitehouse BJ, Huang GC, Amiel SA, et al. Direct regulation of insulin secretion by angiotensin II in human islets of Langerhans. Diabetologia (2006) 49(2):321–31. doi: 10.1007/s00125-005-0101-7
25. Trovati M, Massucco P, Anfossi G, Cavalot F, Mularoni E, Mattiello L, et al. Insulin influences the renin-angiotensin-aldosterone system in humans. Metabolism (1989) 38:501–3. doi: 10.1016/0026-0495(89)90207-2
26. Harte A, McTernan P, Chetty R, Coppack S, Katz J, Smith S, et al. Insulin-mediated upregulation of the renin angiotensin system in human subcutaneous adipocytes is reduced by rosiglitazone. Circulation (2005) 111:1954–61. doi: 10.1161/01.CIR.0000161954.17870.5D
27. Jost-Vu E, Horton R, Antonipillai I. Altered regulation of renin secretion by insulin-like growth factors and angiotensin II in diabetic rats. Diabetes (1992) 41:1100–5. doi: 10.2337/diab.41.9.1100
28. Chen X, Zhang SL, Pang L, Filep JG, Tang SS, Ingelfinger JR, et al. Characterization of a putative insulin-responsive element and its binding protein(s) in rat angiotensinogen gene promoter: regulation by glucose and insulin. Endocrinology (2001) 142:2577–85. doi: 10.1210/endo.142.6.8214
29. Márquez E, Riera M, Pascual J, Soler MJ. Albumin inhibits the insulin-mediated ACE2 increase in cultured podocytes. Am J Physiol Renal Physiol (2014) 306:F1327–34. doi: 10.1152/ajprenal.00594.2013
30. Kamide K, Rakugi H, Nagai M, Takiuchi S, Matsukawa N, Higaki J, et al. Insulin-mediated regulation of the endothelial renin-angiotensin system and vascular cell growth. J Hypertens (2004) 22:121–7. doi: 10.1097/00004872-200401000-00021
31. Nickenig G, Röling J, Strehlow K, Schnabel P, Böhm M. Insulin induces upregulation of vascular AT1 receptor gene expression by posttranscriptional mechanisms. Circulation (1998) 98(22):2453–60. doi: 10.1161/01.cir.98.22.2453
32. Samuelsson AM, Bollano E, Mobini R, Larsson BM, Omerovic E, Fu M, et al. Hyperinsulinemia: effect on cardiac mass/function, angiotensin II receptor expression, and insulin signaling pathways. Am J Physiol Heart Circ Physiol (2006) 291:H787–96. doi: 10.1152/ajpheart.00974.2005
33. Ceolotto G, Valente R, Baritono E, Reato S, Iori E, Monari A, et al. Effect of insulin and angiotensin II on cell calcium in human skin fibroblasts. Hypertension (2001) 37:1486–91. doi: 10.1161/01.hyp.37.6.1486
34. Inishi Y, Okuda T, Arakawa T, Kurokawa K. Insulin attenuates intracellular calcium responses and cell contraction caused by vasoactive agents. Kidney Int (1994) 45:1318–25. doi: 10.1038/ki.1994.172
35. Touyz RM, Schiffrin EL. Tyrosine kinase signaling pathways modulate angiotensin II-induced calcium ([Ca2+]i) transients in vascular smooth muscle cells. Hypertension (1996) 27:1097–103. doi: 10.1161/01.hyp.27.5.1097
36. Kahn AM, Seidel CL, Allen JC, O’Neil RG, Shelat H, Song T. Insulin reduces contraction and intracellular calcium concentration in vascular smooth muscle. Hypertension (1993) 22:735–42. doi: 10.1161/01.hyp.22.5.735
37. Saito F, Hori MT, Fittingoff M, Hino T, Tuck ML. Insulin attenuates agonist-mediated calcium mobilization in cultured rat vascular smooth muscle cells. J Clin Invest (1993) 92:1161–7. doi: 10.1172/JCI116685
38. Hus-Citharel A, Bouby N, Iturrioz X, Llorens-Cortes C. Multiple cross talk between hangiotensin II, bradykinin, and insulin signaling in the cortical thick ascending limb of rat kidney. Endocrinology (2010) 151:3181–94. doi: 10.1210/en.2009-1237
39. Yagi S, Takata S, Kiyokawa H, Yamamoto M, Noto Y, Ikeda T, et al. Effects of insulin on vasoconstrictive responses to norepinephrine and angiotensin II in rabbit femoral artery and vein. Diabetes (1988) 37:1064–67. doi: 10.2337/diab.37.8.1064
40. Tolpygo SM, Pevtsova EI, Shoibonov BB, Kotov AV. Comparative study of the effects of free bound and carrier protein angiotensin II in experimental hypoglycemia and hyperglycemia. Bull Exp Biol Med (2014) 156:419–22. doi: 10.1007/s10517-014-2364-9
41. Takatori S, Mizote M, Zamami Y, Kurosaki Y, Kawasaki H. Effects of insulin on vascular responses to spinal cord stimulation and vasoactive agents in pithed rats. Br J Pharmacol (2003) 140:1137–45. doi: 10.1038/sj.bjp.0705539
42. Mayer MA, Höcht C, Giani JF, Muñoz MC, Carranza A, Taira CA, et al. Central insulin-angiotensin II interaction in blood pressure regulation in fructose overloaded rats. Regul Pept (2013) 185:37–43. doi: 10.1016/j.regpep.2013.06.001
43. Tolpygo SM, Shoibonov BB, Lagutina LV. (2015). Participation angiotensin 1-7 free and bound with proteins in compensation disorders behavior and hemodynamics under experimental hypo- and hyperglycemia in rats, in: Proc. of the 4th International Interdisciplinary Conference “Modern problems in systemic regulation of physiological functions”, Moscow: Pero. pp. 622–26.
44. Tolpygo SM, Pevtsova EI, Kotov AV. Behavioral and hemodynamic effects of free and protein-bound angiotensin IV in rats in experimental hypo- and hyperglycemia: comparative aspects. Bull Exp Biol Med (2015) 159:297–301. doi: 10.1007/s10517-015-2946-1
45. Albiston AL, Diwakarla S, Fernando RN, Mountford SJ, Yeatman HR, Morgan B, et al. Identification and development of specific inhibitors for insulin-regulated aminopeptidase as a new class of cognitive enhancers. Br J Pharmacol (2011) 164:37–47. doi: 10.1111/j.1476-5381.2011.01402.x
46. Mattorre B, Tedeschi V, Paldino G, Fiorillo MT, Paladini F, Sorrentino R. The emerging multifunctional roles of ERAP1, ERAP2 and IRAP between antigen processing and renin-angiotensin system modulation. Front Immunol (2022) 13:1002375. doi: 10.3389/fimmu.2022.1002375
47. Fernando RN, Albiston AL, Chai SY. The insulin-regulated aminopeptidase IRAP is colocalised with GLUT4 in the mouse hippocampus–potential role in modulation of glucose uptake in neurones? Eur J Neurosci (2008) 28:588–98. doi: 10.1111/j.1460-9568.2008.06347.x
48. Reaven GM. Insulin resistance/compensatory hyperinsulinemia, essential hypertension, and cardiovascular disease. J Clin Endocrinol Metab (2003) 88(6):2399–403. doi: 10.1210/jc.2003-030087
49. Doronin S, Shumay E, Wang HY, Malbon CC. Akt mediates sequestration of the beta(2)-adrenergic receptor in response to insulin. J Biol Chem (2002) 277:15124–31. doi: 10.1074/jbc.M108771200
50. Girnita L, Worrall C, Takahashi S, Seregard S, Girnita A. Something old, something new and something borrowed: emerging paradigm of insulin-like growth factor type 1 receptor (IGF-1R) signaling regulation. Cell Mol Life Sci (2014) 71:2403–27. doi: 10.1007/s00018-013-1514-y
51. Jia G, Sowers JR. Hypertension in diabetes: an update of basic mechanisms and clinical disease hypertension. Hypertension (2021) 78:1197–205. doi: 10.1161/HYPERTENSIONAHA.121.17981
52. Carlsson PO, Berne C, Jansson L. Angiotensin II and the endocrine pancreas: effects on islet blood flow and insulin secretion in rats. Diabetologia (1998) 41:127–33. doi: 10.1007/s001250050880
53. Czech MP. Insulin action and resistance in obesity and type 2 diabetes. Nat Med (2017) 23:804–14. doi: 10.1038/nm.4350
54. Kadowaki T, Maegawa H, Watada H, Yabe D, Node K, Murohara T, et al. Interconnection between cardiovascular, renal and metabolic disorders: A narrative review with a focus on Japan. Diabetes Obes Metab (2022) 24:2283–96. doi: 10.1111/dom.14829
55. Le MT, Vanderheyden PM, Szaszák M, Hunyady L, Vauquelin G. Angiotensin IV is a potent agonist for constitutive active human AT1 receptors. Distinct roles of the N-and C-terminal residues of angiotensin II during AT1 receptor activation. J Biol Chem (2002) 277:23107–10. doi: 10.1074/jbc.C200201200
56. Yang R, Smolders I. Dupont AG Blood pressure and renal hemodynamic effects of angiotensin fragments. Hypertens Res (2011) 34:674–83. doi: 10.1038/hr.2011.24
57. Vinh A, Widdop RE, Drummond GR, Gaspari TA. Chronic angiotensin IV treatment reverses endothelial dysfunction in ApoE-deficient mice. Cardiovasc Res (2008) 77:178–87. doi: 10.1093/cvr/cvm021
58. Kolb H, Kempf K, Röhling M, Martin S. Insulin: too much of a good thing is bad. BMC Med (2020) 18:224. doi: 10.1186/s12916-020-01688-6
59. Campbell JE, Newgard CB. Mechanisms controlling pancreatic islet cell function in insulin secretion. Nat Rev Mol Cell Biol (2021) 22:142–58. doi: 10.1038/s41580-020-00317-7
60. Kalra S, Arora S, Kapoor N. Classification of non-insulin glucose lowering drugs. J Pak Med Assoc (2022) 72:181–2. doi: 10.47391/JPMA.22-003
61. Cheng R, Taleb N, Stainforth-Dubois M, Rabasa-Lhoret R. The promising future of insulin therapy in diabetes mellitus. Am J Physiol Endocrinol Metab (2021) 320:E886–90. doi: 10.1152/ajpendo.00608.2020
62. Su J, Tang L, Luo Y, Xu J, Ouyang S. Research progress on drugs for diabetes based on insulin receptor/insulin receptor substrate. Biochem Pharmacol (2023) 217:115830. doi: 10.1016/j.bcp.2023.115830
63. Brands MW, Hildebrandt DA, Mizelle HL, Hall JE. Sustained hyperinsulinemia increases arterial pressure in conscious rats. Am J Physiol (1991) 260:R764–8. doi: 10.1152/ajpregu.1991.260.4.R764
64. Sun T, Meng F, Zang S, Li Y, Zhang R, Yu Z, et al. The effects of insulin therapy on maternal blood pressure and weight in women with gestational diabetes mellitus. BMC Pregnancy Childbirth (2021) 21:657. doi: 10.1186/s12884-021-04066-z
65. Nguyen DV, Shaw LC, Grant MB. Inflammation in the pathogenesis of microvascular complications in diabetes. Front Endocrinol (Lausanne) (2012) 3:170. doi: 10.3389/fendo.2012.00170
66. Tsuchiya K. Role of insulin action in the pathogenesis of diabetic complications. Diabetol Int (2022) 13:591–8. doi: 10.1007/s13340-022-00601-1
Keywords: insulin, renin-angiotensin system, insulin resistance, signaling cross-talk, angiotensin, diabetes mellitus
Citation: Zamolodchikova TS, Tolpygo SM and Kotov AV (2024) Insulin in the regulation of the renin-angiotensin system: a new perspective on the mechanism of insulin resistance and diabetic complications. Front. Endocrinol. 15:1293221. doi: 10.3389/fendo.2024.1293221
Received: 12 September 2023; Accepted: 08 January 2024;
Published: 23 January 2024.
Edited by:
Hilda Ghadieh, University of Balamand, LebanonReviewed by:
Marcia Hiriart, Universidad Nacional Autonoma de Mexico, MexicoCopyright © 2024 Zamolodchikova, Tolpygo and Kotov. This is an open-access article distributed under the terms of the Creative Commons Attribution License (CC BY). The use, distribution or reproduction in other forums is permitted, provided the original author(s) and the copyright owner(s) are credited and that the original publication in this journal is cited, in accordance with accepted academic practice. No use, distribution or reproduction is permitted which does not comply with these terms.
*Correspondence: Tatyana S. Zamolodchikova, tatyanazam@yandex.ru