- 1Medical College of Southeast University, Nanjing, Jiangsu, China
- 2Reproductive Medicine Center, Zhongda Hospital, Southeast University, Nanjing, Jiangsu, China
- 3Andrology Department of Integrative Medicine, Zhongda Hospital, Southeast University, Nanjing, Jiangsu, China
The increasing life expectancy observed in recent years has resulted in a higher prevalence of late-onset hypogonadism (LOH) in older men. LOH is characterized by the decline in testosterone levels and can have significant impacts on physical and mental health. While the underlying causes of LOH are not fully understood, there is a growing interest in exploring the role of inflammaging in its development. Inflammaging is a concept that describes the chronic, low-grade, systemic inflammation that occurs as a result of aging. This inflammatory state has been implicated in the development of various age-related diseases. Several cellular and molecular mechanisms have been identified as contributors to inflammaging, including immune senescence, cellular senescence, autophagy defects, and mitochondrial dysfunction. Despite the extensive research on inflammaging, its relationship with LOH has not yet been thoroughly reviewed in the literature. To address this gap, we aim to review the latest findings related to inflammaging and its impact on the development of LOH. Additionally, we will explore interventions that target inflammaging as potential treatments for LOH.
1 Introduction
Aging is a complex biological process characterized by physiological and biochemical changes in the human body. In the male context, a significant outcome of aging is the gradual reduction in the production of testosterone, a pivotal hormone with diverse physiological functions (1). The primary site of testosterone production is the Leydig cells situated in the interstitial tissue of the testes. However, these cells undergo structural and functional alterations with age, leading to decreased testosterone output (2). This reduction in testosterone levels corresponds with a condition termed Late-Onset Hypogonadism (LOH), which is marked by a range of symptoms including diminished libido, fatigue, sarcopenia, and mood fluctuations (3).
Studies indicate that luteinizing hormone (LH) levels remain relatively stable in older males, while testosterone secretion substantially declines (4). Comparative analysis of Leydig cell populations in young and older men reveals a significant reduction in Leydig cell numbers in the elderly (5). Moreover, aging Leydig cells experience disrupted redox balance, leading to intracellular reactive oxygen species (ROS) accumulation and subsequent oxidative stress. This oxidative stress disrupts signaling pathways vital for testosterone synthesis, resulting in reduced expression of key testosterone production molecules and impeding Leydig cell function (6).
Concomitantly, the aging process leads to a chronic low-grade inflammatory state termed inflammaging (7). C-reactive protein, interleukin-6, tumor necrosis factor-α, interleukin-1β, and other related markers are routinely utilized as straightforward serum biomarkers for assessing inflammaging (8). Circulating miRNA sequencing and quantification of circulating cell-free mitochondrial DNA copy numbers are also approaches for assessing systemic inflammation (9, 10). Unlike acute inflammation, inflammaging persists over time and is closely linked to the onset of several chronic and age-related ailments (8). Numerous studies consistently demonstrate elevated levels of inflammatory factors like TNF-α, IL-1β, and IL-6 in older males’ circulation (11–13). The activation of inflammatory signaling pathways, predominantly orchestrated by NF-KB and P38MAPK, assumes a central role in cellular aging. These pathways’ activation prompts the release of inflammatory mediators and proteases, culminating in the formation of the Senescence-Associated Secretory Phenotype (SASP) (14). Due to the lack of a protective blood-testis barrier, these inflammatory cytokines can easily enter the testicular interstitium through circulation, adversely affecting Leydig cell function (15). Additionally, the aging process can prompt the shift of M2-type testicular macrophages to pro-inflammatory M1-type testicular macrophages, releasing inflammatory cytokines and exacerbating testicular interstitial inflammation (16).
This article offers a comprehensive overview of recent research on inflammaging, immune senescence, cellular senescence, mitochondrial dysfunction, and the pathophysiological progression of LOH. Furthermore, we delve into the potential of targeting inflammaging as a promising strategy for future therapeutic interventions to slow down or halt the progression of age-related LOH.
2 Regulation of testosterone synthesis in Leydig cells
Testosterone synthesis within Leydig cells is primarily orchestrated by the hypothalamic-pituitary-gonadal (HPG) axis (17). The hypothalamus releases gonadotropin-releasing hormone (GnRH) in rhythmic pulses (18). GnRH interacts with pituitary gonadotroph receptors, stimulating LH secretion from the pituitary gland (19). LH binds to Leydig cell receptors, activating adenylyl cyclase. This triggers ATP to cyclic adenosine monophosphate (cAMP) conversion, activating Protein Kinase A (PKA) downstream (20). Cholesterol then enters Leydig cells, converting to free cholesterol. Steroidogenic acute regulatory protein (StAR) transports free cholesterol to mitochondria. P450scc enzyme in mitochondria cleaves free cholesterol to form pregnenolone (21, 22). Pregnenolone moves to the endoplasmic reticulum, becoming progesterone and dehydroepiandrosterone via enzymes like 3β-hydroxysteroid dehydrogenase (3β-HSD) and cytochrome P450 17A1 (CYP17A1) (23). Both progesterone and dehydroepiandrosterone are subsequently transformed into androstenedione. Ultimately, testosterone synthesis is realized through the catalytic action of 17β-hydroxysteroid dehydrogenase (17β-HSD) (24). During the aging process, certain changes in the body may affect testosterone synthesis by influencing the function of the HPG axis.
3 Immuno-senescence
Immuno-senescence, the gradual decline in immune system function with age, is a pivotal factor in the aging process (25). It involves reduced immune cell abundance, compromised efficiency, altered response patterns, heightened inflammation, and reduced tolerance (26). The aging process affecting peripheral blood immune cells can induce systemic inflammation, potentially disrupting the stable functioning of the hypothalamic-pituitary-gonadal (HPG) axis and resulting in impaired regulation of testosterone synthesis. Furthermore, the aging of testicular macrophages may contribute to localized inflammation, directly impeding the normal functionality of Leydig cells.
3.1 Changes in peripheral blood immune cells
Aging immune cells contribute to systemic inflammation and age-related disorders (27). Huang et al.’s single-cell sequencing study on human immune profiles across aging and gender revealed significant shifts in peripheral blood immune cells, contributing to systemic inflammation (28).
3.1.1 T cell alterations in aging males
T cells are the most significantly altered immune cell type in the peripheral blood of elderly males, characterized by a marked decrease in quantity, significant features of cellular aging, and upregulation of inflammatory gene expression. Firstly, single-cell sequencing studies suggest a significant reduction in the numbers of CD8+ T cells and CD4+ naïve T cells in the peripheral blood of elderly males (28). Martinez-Zamudio et al. noted senescent features in CD8+ T cells, including elevated intracellular Senescence-associated beta-galactosidase (SA-βGal) activity, telomere dysfunction, and impaired mitochondrial transcription factors in older males (29). In addition, elderly males exhibit elevated expression of specific genes like DUSP2, CXCR4, DDIT4, NF-KBIA, and JUNB in CD8+ T cells and CD4+ T cells, along with the activation of MAPK signaling pathways (28). These changes impair T cell function, causing reduced proliferation, increased lysosomal content, and excess pro-inflammatory cytokines (30, 31).
3.1.2 Natural killer cell dynamics in aging males
Natural Killer (NK) cells, crucial components in innate immunity, express CD56 and CD16 (32). Based on CD56 expression, they can be classified into CD56brightCD16+ and CD56dimCD16+ subpopulations. CD56brightCD16+ NK cells possess cytokine secretion capabilities and regulate immunity, while CD56dimCD16+ NK cells exhibit higher cytotoxicity and mainly engage in innate immunity (33). Aging affects these NK cell subsets in men’s peripheral blood, reducing CD56brightCD16+ and increasing CD56dimCD16+ NK cell absolute numbers and proportions (34). Moreover, a subset of CD56dimCD16+ NK cells has been observed to express elevated levels of CD57, a marker associated with highly differentiated cells (35). Some CD56dimCD16+CD57+ cells exhibit heightened expression of senescence-associated genes like ZFP36 and DUSP2 (28), elevating cytotoxicity and cytokine secretion (IL-6, TNF-α) (36–38).
3.1.3 Monocyte changes in aging males
The influence of aging on peripheral blood monocyte counts remains debated. Seidler S et al.’s study suggests minimal impact (39). However, recent sequencing studies reveal increased monocyte levels in aging individuals (28). However, multiple studies have consistently concluded that aging leads to an increase in the number of CD16+ monocytes, indicating phenotypic alterations in monocytes during the aging process (28, 39–41). Unlike classical CD14+ monocytes, CD16+ monocytes have an enhanced inflammatory potential that promotes the development of the SASP (42, 43). Simultaneously, the NF-KB signaling pathway, IL-1 signaling pathway, and inflammatory response signaling pathway in monocytes were markedly activated, with a concomitant significant upregulation in the expression of pro-inflammatory genes TNF, JUNB, and DDIT4 in aging men (28).
3.1.4 Dendritic cell dynamics in aging males
Dendritic cells play a pivotal role in the immune response as they serve as antigen-presenting cells to T cells. They encompass plasmacytoid dendritic cells (pDCs) and myeloid dendritic cells (mDCs) (44). Most scientific investigations have consistently concluded that the quantity of dendritic cells in the peripheral blood of healthy older adults does not exhibit noteworthy changes when compared to their younger counterparts (28, 45, 46). Nevertheless, shifts in pDCs and mDCs proportions during senescence remain debated (47–49). Regardless of mDCs abundance changes, they exhibit pronounced pro-inflammatory characteristics compared to pDCs. Senescent mDCs exhibit reduced phosphoinositide 3-kinase (PI3K) activity, amplifying NF-KB activation and subsequent pro-inflammatory cytokine generation (45). Senescent mDCs also show elevated CD68 expression, indicating sustained activation, leading to heightened pro-inflammatory cytokine production even without external stimuli (50).
3.2 Changes of testicular macrophages
3.2.1 Function of testicular macrophages
Testicular macrophages are pivotal in maintaining testicular immune privilege, supporting spermatogenesis, and modulating testosterone synthesis (51–53). They are categorized into interstitial and peritubular macrophages based on phenotypic traits (54). These cells originate from embryonic and bone marrow progenitors, adopting an anti-inflammatory state with TGF-β and IL-10 secretion to support testosterone synthesis and spermatogenesis (52, 55). Furthermore, these macrophages possess the capability to secrete 25-hydroxycholesterol, facilitating the process of testosterone synthesis in Leydig cells (56).
3.2.2 Influence of aging on testicular macrophages
In early investigations, transmission electron microscopy was employed to examine age-related alterations in testicular macrophages, revealing abnormalities in cellular structures such as mitochondria and the Golgi apparatus (57). However, the mechanisms underlying these changes in aging macrophages remained unclear due to methodological limitations. With the advent and progression of sequencing technologies, we have attained a more comprehensive understanding of the genetic, phenotypic, and quantitative changes in aging macrophages. Aging increases pro-inflammatory macrophages. Nie et al. identified elevated hyperactivated (M1-type) macrophages and heightened cytokine secretion in elderly human testes (58). Mouse research supported these findings, revealing three macrophage subpopulations. Subpopulation 3 (senescence-specific) increased significantly in aged mice, showing a hyperactivated state and inflammation-related gene expression. Aging enriched genes related to subpopulations 1 and 2, linked to type I interferon secretion and Toll-like receptor 4 signaling (16).
3.3 Effect of immuno-senescence on HPG axis
Alterations in peripheral blood immune cells during immunosenescence significantly contribute to heightened proinflammatory status in men. This senescent inflammatory state profoundly impacts the HPG axis function (59). The aging process of the hypothalamus is intricately linked to the activation of the NF-KB signaling pathway. The activation of the NF-KB signaling pathway exerts an impact on the functionality of Gonadotropin-Releasing Hormone (GnRH) neurons, impeding GnRH gene transcription and culminating in an anomalous release of GnRH within the hypothalamus (60). Studies have identified an augmented presence of pro-inflammatory cytokines, particularly IL-6, in the pituitary tissue of aging mice. This inflammatory condition markedly hinders the tissue repair mechanisms of pituitary stem cells (61). Aging notably expands pro-inflammatory testicular macrophages, increasing pro-inflammatory cytokine secretion and gene expression. This macrophage shift significantly influences the testicular inflammatory microenvironment during aging (62). Systemic and localized inflammation may exacerbate Leydig cellular aging, leading to mitochondrial dysfunction and inhibition of autophagy (Figure 1).
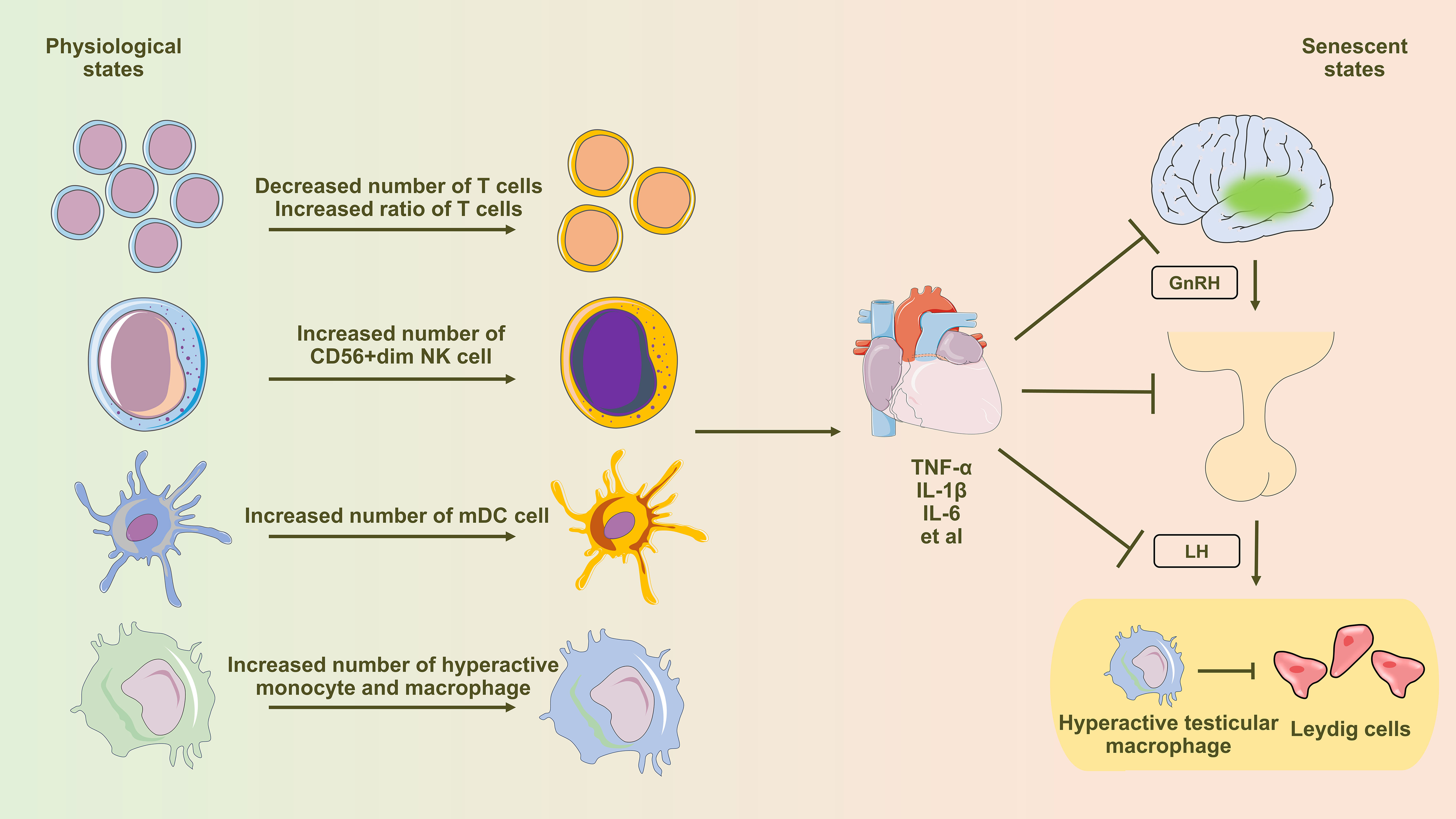
Figure 1 Diagram illustrating the influence of immune senescence-induced inflammaging on testosterone synthesis in Leydig cells. Aging profoundly affects peripheral blood immune cells, inducing notable alterations in T cells, NK cells, dendritic cells, monocytes, and macrophages. This shift leads to an elevated presence of pro-inflammatory immune cells and an excessive production of pro-inflammatory cytokines, subsequently influencing the regular functionality of the hypothalamic-pituitary-gonadal (HPG) axis via the circulatory system. Testicular macrophages, under the influence of senescence, transition towards the M1 subtype (hyperactivated), characterized by heightened secretion of abundant pro-inflammatory factors. These factors are then released through paracrine signaling, directly impacting the functionality of Leydig cells.
4 Cellular senescence
Cellular senescence is characterized by the cessation of cell proliferation and functionality under specific circumstances, accompanied by distinct alterations in morphology, physiology, and molecular attributes (63). This intricate biological phenomenon is intricately linked to the typical progression of organisms, the aging trajectory, and the onset of various diseases.
4.1 Basic change of senescent Leydig cells
The pioneering work of Hayflick and Moorhead unveiled senescent cells’ inability to undergo customary cell division as essential for cell cycles (64). Subsequent investigations have encompassed telomere length and telomerase function as principal determinants contributing to cell cycle arrest (65, 66). Morphological and structural changes often characterize senescent cells, manifesting as an enlarged, flattened morphology and irregular organelles (67). SA-βGal serves as a widely employed biomarker, offering insights into the extent of cellular senescence to some extent, although not serving as an obligatory indicator (68). Simultaneously, the expression of specific cell cycle inhibitory proteins, notably p16 and p21, experiences significant upregulation in senescent cells (69). Jin et al. employed transmission electron microscopy to detect find fewer, disorganized mitochondria in senescent Leydig cells (70). Jeong et al. observed pronounced elevation in SA-βgal levels, along with heightened expressions of p16, p19, and p21, in aging Leydig cells (71).
4.2 SASP in senescent Leydig cells
SASP is crucial in cellular aging, where senescent cells release various molecules affecting the microenvironment and nearby cells (72). Persistent SASP can trigger inflammation and age-related disorders (73–75).
4.2.1 Activation of NF-KB pathways
The NF-KB signaling pathway is pivotal in immune responses, inflammation, proliferation, and apoptosis (76). Activation pathways typically involve cell surface receptors, viral infections, bacterial components, and cytokines (77). NF-KB translocates from cytoplasm to nucleus, binding SASP-related gene promoters, inducing transcription (78). Furthermore, NF-KB can indirectly enhance the expression of the transcription factor C/EBPβ, subsequently facilitating direct regulation of SASP gene expression by C/EBPβ (79, 80). Within Leydig cells, Tao Shang and colleagues demonstrated significant activation of the NF-KB signaling pathway (81). Upon exposure to inflammatory induction, mouse Leydig progenitor cells and the TM3 cell line exhibited a substantial increase in the levels of pro-inflammatory cytokines in the cell culture supernatants.
4.2.2 Activation of MAPK pathways
The MAPK signaling pathway, including ERK1/2, JNK, and P38 MAPK, is pivotal in cell processes like proliferation, differentiation, apoptosis, and inflammation (82, 83). Adam Freund et al. suggested P38MAPK’s autonomous role in SASP, enhancing NF-KB’s transcriptional activity for SASP activation (84). Simultaneously, P38MAPK exerts an indirect influence by potentiating the activation of transcription factors such as ATF2, AP-1, and CREB1 (85, 86). Consequently, these activated transcription factors interplay in the regulation of SASP. P38MAPK activation was found in aging Leydig cells, while ERK1/2 or JNK activation wasn’t prominent (87). This suppresses key testosterone synthesis molecules expression in Leydig cells, ultimately reducing serum testosterone levels.
The components of SASP secreted by senescent Leydig cells can exert paracrine effects on neighboring cells (88). It is noteworthy that the C/EBPβ, ATF2, AP-1, and CREB1 molecules belong to the family of basic leucine zipper transcription factors (89). Indeed, these molecules are intricately involved in the regulation of SASP (90). Importantly, within Leydig cells, they assume a pivotal role in orchestrating testosterone synthesis pathways (91, 92).
5 Mitochondrial dysfunction
Leydig cellular senescence is closely linked with mitochondrial dysfunction. Aging induces oxidative stress in cells, thereby influencing the expression levels of crucial molecules involved in testosterone synthesis on the mitochondria. This, in turn, initiates processes such as apoptosis and necroptosis, culminating in mitochondrial dysfunction.
5.1 Role of mitochondria in testosterone synthesis
Mitochondria, vital intracellular organelles, play a crucial role in testosterone synthesis within Leydig cells. Firstly, they are central to generating ATP, the essential energy substrate required for testosterone synthesis (93). Secondly, mitochondria are integral to the intricate signaling cascade governing testosterone synthesis, as key molecules like StAR and CYP11A1 localize within these organelles (94–96). Additionally, mitochondria regulate oxidative stress and apoptosis in Leydig cells, highlighting their multifaceted involvement (97, 98).
5.2 Mitochondrial dysfunction in senescent Leydig cells
Mitochondrial dysfunction significantly contributes to impaired testosterone synthesis in senescent Leydig cells (99). The expression of StAR on the outer mitochondrial membrane of senescent Leydig cells shows a notable reduction (100), leading to structural perturbations in Leydig cell mitochondria and subsequent impairment of mitochondrial oxidative respiratory chain function (101). Dysfunctional mitochondria in Leydig cells can lead to reduced ATP synthesis and an accumulation of intracellular ROS (102). Excess ROS triggers the activation of inflammatory vesicles, initiating cellular pyroptosis (103). This process involves the NLRP3 inflammasome, where NF-KB activation, in response to inflammatory stimuli, amplifies the transcription of inflammasome-related genes (104). NLRP3 activation recruits the adaptor molecule ASC, initiating caspase-1 activation. Caspase-1 cleaves pro-IL-1β into IL-1β and pro-IL-18 into IL-18, resulting in their secretion and subsequent apoptosis (105). In response to inflammatory stimuli, Leydig cells exhibit increased intracellular NLRP3 and ASC expression, caspase-1 cleavage, conversion of IL-1β and IL-18, apoptosis activation, and reduced expression of testosterone-synthesizing molecules (106). Collectively, these changes culminated in the inhibition of testosterone synthesis.
6 Autophagy deficiency
6.1 Role of autophagy in Leydig cells
Autophagy, an intrinsic catabolic process, maintains intracellular stability by degrading impaired cellular components (107). Eukaryotic cells primarily classify autophagy into three major types: macroautophagy, microautophagy, and chaperone-mediated autophagy (108). Given its specificity toward organelles and cellular contents, autophagy further subdivides into types such as mitophagy, ER-phagy, and lipophagy (109–111). In Leydig cells, autophagy regulates oxidative stress, cholesterol uptake, apoptosis, and pyroptosis, influencing testosterone synthesis capacity (106, 112–114).
6.2 Autophagy deficiency and its consequences in Leydig cells
Mitochondria exhibit dynamic behavior within the cell, continuously undergoing fusion and division processes (115). The occurrence of mitophagy is intricately linked to the processes of mitochondrial fusion and division. Mitochondrial fission stimulates the expression of pre-mitochondrial fission proteins, thereby triggering a corresponding alteration in the extent of mitophagy (116). Recent research on Leydig cells highlighted elevated N6-methyladenosine (m6A) levels in primary Leydig cells from senescent mice. This m6A increase impedes intracellular autophagy, influencing testosterone synthesis functionality (117). Reduced autophagy induces redox imbalance in Leydig cells, accumulating intracellular ROS (114). Dysregulated ROS levels disturb mitochondrial fusion equilibrium, impacting autophagy. Yi et al. attributed cadmium-induced apoptosis in Leydig cells to mitochondrial fragmentation, causing impaired function and elevated superoxide and ROS levels, inhibiting mitochondrial autophagy (118). Diminished autophagy in Leydig cells also links to pyroptosis initiation. Inflammatory stimuli reduce autophagy, activating cellular inflammasome and initiating pyroptosis (106) (Figure 2).
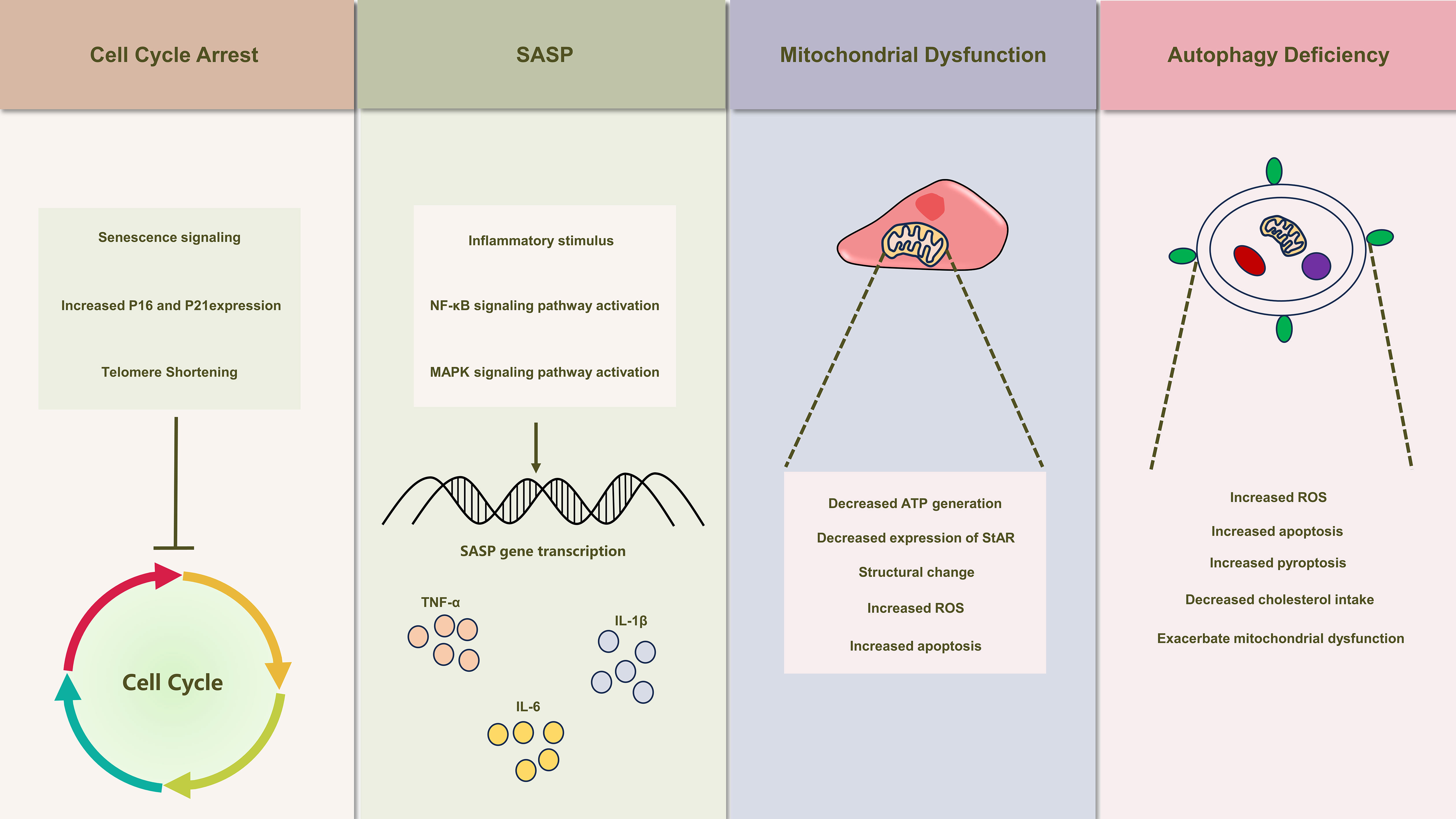
Figure 2 Illustrates the schematic depiction of senescence-induced alterations in Leydig cells. Leydig cell senescence manifests a spectrum of modifications, encompassing cell cycle arrest, the emergence of senescence-associated secretory phenotypes (SASP), mitochondrial dysfunction, and autophagy deficiency. These alterations also play a crucial role in the development of testicular inflammaging, leading to substantial inhibition of Leydig cell function.
7 Possible therapeutic strategies
The established clinical intervention for LOH is testosterone replacement therapy (TRT). Nevertheless, TRT is accompanied by unavoidable side effects (119). We present various alternative interventions, different from TRT, capable of ameliorating LOH symptoms by mitigating inflammaging (Table 1).
7.1 Physical exercise
Physical exercise emerges as a natural and comprehensive approach to health management, demonstrating a visible impact on clinical symptoms associated with various diseases (120). In the context of LOH, engagement in suitable physical exercise holds the potential to alleviate systemic inflammation, enhance cellular antioxidant stress capacity, and elevate testosterone levels in patients. In a randomized controlled trial involving individuals aged 45-75, participation in interval aerobic exercise at an intensity reaching 90% of the maximum heart rate three times a week yielded a substantial reduction in the circulating levels of C-reactive protein and TNF-α in the study participants (121). Several clinical trials have substantiated that older individuals participating in regular physical exercise manifest heightened levels of antioxidant capacity markers in their blood, including glutathione peroxidase, total nitrite/nitrate, and total oxyradical scavenging capacity, in comparison to their sedentary counterparts (122). Moreover, moderate-intensity aerobic exercise has demonstrated a noteworthy capacity to substantially elevate testosterone levels in elderly men (123).
7.2 Anti-inflammation and antioxidant interventions
Adrenomedullin (ADM), a peptide hormone synthesized primarily in the adrenal medulla, has a broader presence, including the testes (124, 125). ADM exerts potent protective effect in inflammatory contexts (126). Rat primary Leydig cells synthesize ADM and express its receptors, suggesting its autocrine and paracrine roles in safeguarding testosterone production (127). ADM potentially protects Leydig cells during inflammation by curbing ROS production, stabilizing mitochondria, inhibiting MAPK and NF-KB signaling, and enhancing autophagy, which counters apoptosis and pyroptosis (128, 129). Mitochondria-targeted antioxidants interact directly with mitochondria to counteract oxidative stress and damage (130). SS peptides, specifically SS-31, have been extensively researched for their impact on mitochondrial function (131). SS-31 operates at the cellular level, reducing and neutralizing ROS generation, effectively mitigating oxidative stress within cells and mitochondria (132). It plays a critical role in maintaining mitochondrial membrane integrity, thereby balancing mitochondrial fusion and fission, vital for cellular energy metabolism and function (133). Evidence also suggests that SS-31 exhibits anti-inflammatory properties, modulating inflammatory mediator production and cellular responses to inflammation (134). Its potential spans various inflammatory and age-related conditions, including cardiovascular diseases, kidney ailments, and Alzheimer’s disease (135, 136). Currently, clinical trials for the therapeutic application of ADM and SS-31 in specific diseases have progressed to phase II trials (137). Further investigations are imperative to establish the safety profile of these drugs in clinical settings and ascertain its efficacy for addressing LOH.
7.3 Cell transplantation
In recent years, cell transplantation has garnered growing attention as a therapeutic approach for a diverse range of diseases. Cell transplantation methods employed for addressing LOH encompass Leydig cell transplantation, Leydig stem cell transplantation, and stem cell transplantation. Luo et al. conducted a study that introduced an autofluorescence-based technique for the isolation and purification of Leydig cells. Subsequently, these isolated cells were transplanted subcutaneously into denuded mice, resulting in elevated testosterone levels (138). ARORA et al. demonstrated that the concurrent subcutaneous transplantation of Leydig stem cells, supporting cells, and myoid cells facilitated the differentiation and maturation of Leydig cells, leading to the secretion of testosterone (139). In rats, tail vein injection of bone marrow mesenchymal stem cells (MSCs) has been observed to elevate serum testosterone levels. This effect is attributed to the reduction of oxidative stress and senescence phenotype in Leydig cells (140).
7.4 Traditional medicine
Several herbal remedies from Traditional Chinese Medicine, aiming to enhance sex hormone levels and male sexual function, show promise in addressing LOH. Our team developed “Yangjing Capsules,” demonstrating positive impacts on Leydig cell testosterone synthesis (141, 142). Complex herbal compositions pose challenges in pinpointing therapeutic elements and understanding clinical efficacy. Recent research focuses on individual herbs and extracts, verifying direct effects on Leydig cell testosterone synthesis. Icariin, from Epimedium, mimics sex hormones, enhances immunity, and reduces inflammation (143, 144). Studies confirm icariin stimulates testosterone synthesis through pathways involving the upregulation of testosterone synthesis-associated molecules, mitochondrial function preservation, and other mechanisms (145, 146). Polygonatum sibiricum polysaccharides, extracted from Polygonatum sibiricum, renowned for kidney and sexual function enhancement, exhibit antioxidative and anti-inflammatory effects (147). These polysaccharides safeguard testosterone synthesis in mice by reducing oxidative stress, preserving mitochondrial function, and inhibiting apoptosis (148). Additionally, a range of herbs has been explored for their potential to support and protect testosterone synthesis (149), warranting comprehensive investigation across various experimental domains. Ashwagandha, commonly referred to as Withania somnifera, is an herb extensively employed in Indian traditional medicine. It holds promise in ameliorating symptoms associated with LOH through potential benefits, encompassing stress reduction, antioxidant properties, anti-inflammatory effects, immune modulation, and facilitation of testosterone synthesis, among other mechanisms (150–152).
8 Conclusions
In the elderly population, chronic aseptic low-grade inflammation, referred to as inflammaging, contributes to the emergence and progression of diverse age-associated conditions. Both systemic and localized immuno-senescence expose Leydig cells to an inflammatory milieu. Simultaneously, SASP stemming from Leydig cellular senescence, along with factors such as mitochondrial dysfunction and impaired autophagy, amplify the inflammatory response within Leydig cells. These combined factors culminate in the impairment of testosterone synthesis function, sparking the onset of LOH (Figure 3). Notably, investigations concerning the influence of treatments targeting inflammation and mitochondrial function on LOH remain limited. This research gap assumes paramount significance in fulfilling the medical necessities and enhancing the well-being of elderly men, warranting extensive further exploration and translational efforts.
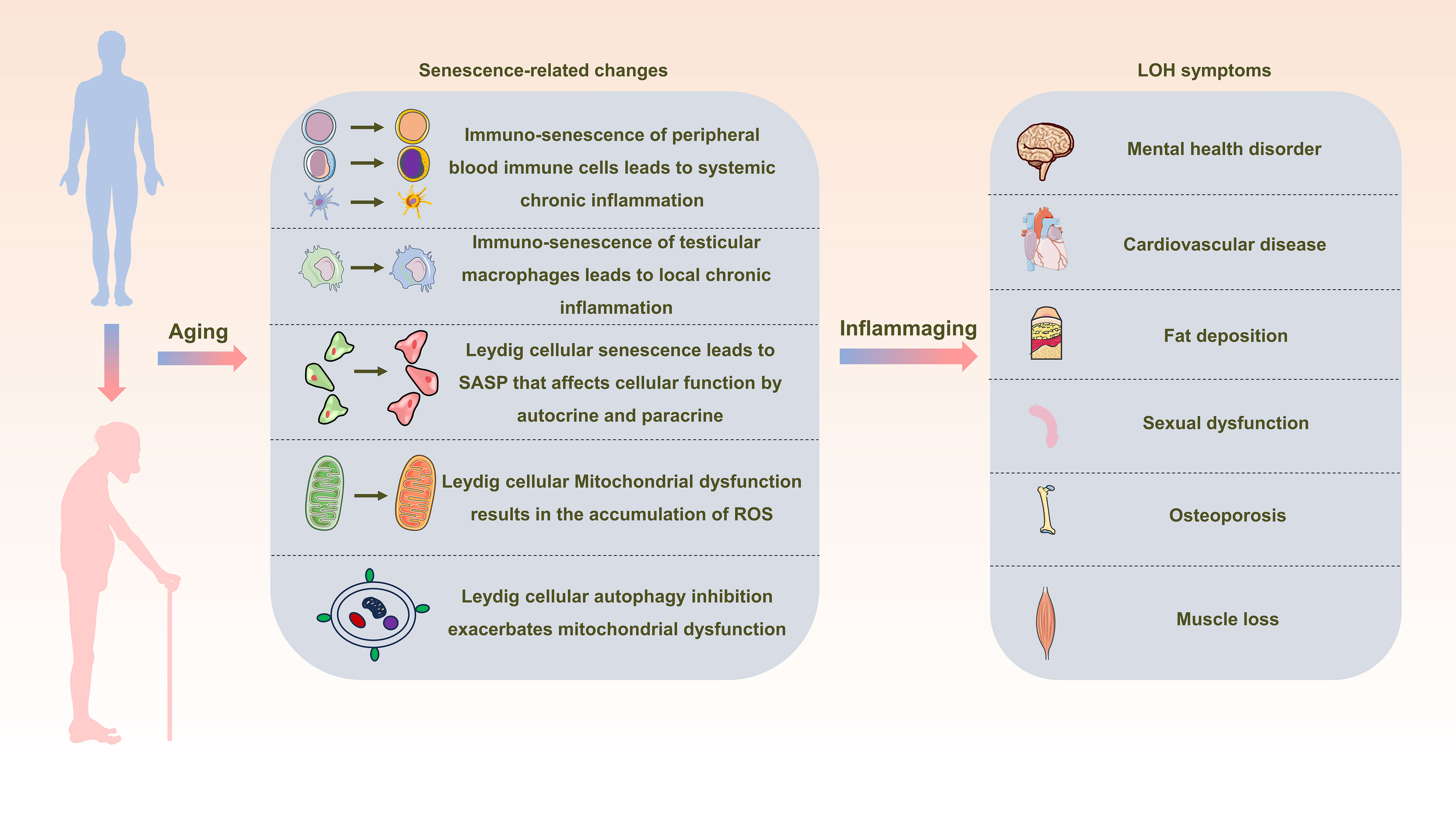
Figure 3 Schematic representation depicting the interrelation between inflammaging and late-onset hypogonadotropic hypogonadism (LOH). As aging progresses, alterations in peripheral blood immune cells, testicular macrophages, and Leydig cells become pronounced, creating a milieu of widespread and localized inflammation. This state, termed inflammaging, disrupts the intricate process of testosterone synthesis within Leydig cells, consequently initiating and advancing the development of LOH in aging males.
Author contributions
DX: Conceptualization, Writing – original draft, Writing – review & editing. YJ: Funding acquisition, Writing – review & editing. BJ: Funding acquisition, Writing – review & editing.
Funding
The author(s) declare financial support was received for the research, authorship, and/or publication of this article. This work was supported by the National Natural Science Foundation of China (Grant No. 82074440, 82004376).
Conflict of interest
The authors declare that the research was conducted in the absence of any commercial or financial relationships that could be construed as a potential conflict of interest.
Publisher’s note
All claims expressed in this article are solely those of the authors and do not necessarily represent those of their affiliated organizations, or those of the publisher, the editors and the reviewers. Any product that may be evaluated in this article, or claim that may be made by its manufacturer, is not guaranteed or endorsed by the publisher.
References
1. Yeap BB, Manning L, Chubb S, Handelsman DJ, Almeida OP, Hankey GJ, et al. Progressive impairment of testicular endocrine function in ageing men: Testosterone and dihydrotestosterone decrease, and luteinizing hormone increases, in men transitioning from the 8th to 9th decades of life. Clin Endocrinol (Oxf) (2018) 88:88–95. doi: 10.1111/cen.13484
2. Zirkin BR, Papadopoulos V. Leydig cells: formation, function, and regulation. Biol Reprod (2018) 99:101–11. doi: 10.1093/biolre/ioy059
3. Nieschlag E. Late-onset hypogonadism: a concept comes of age. Andrology (2020) 8:1506–11. doi: 10.1111/andr.12719
4. Camacho EM, Huhtaniemi IT, O’Neill TW, Finn JD, Pye SR, Lee DM, et al. Age-associated changes in hypothalamic-pituitary-testicular function in middle-aged and older men are modified by weight change and lifestyle factors: longitudinal results from the European Male Ageing Study. Eur J Endocrinol (2013) 168:445–55. doi: 10.1530/EJE-12-0890
5. Mularoni V, Esposito V, Di Persio S, Vicini E, Spadetta G, Berloco P, et al. Age-related changes in human Leydig cell status. Hum Reprod (2020) 35:2663–76. doi: 10.1093/humrep/deaa271
6. Chung JY, Chen H, Zirkin B. Sirt1 and Nrf2: regulation of Leydig cell oxidant/antioxidant intracellular environment and steroid formationdagger. Biol Reprod (2021) 105:1307–16. doi: 10.1093/biolre/ioab150
7. Fulop T, Larbi A, Pawelec G, Khalil A, Cohen AA, Hirokawa K, et al. Immunology of aging: the birth of inflammaging. Clin Rev Allergy Immunol (2023) 64:109–22. doi: 10.1007/s12016-021-08899-6
8. Franceschi C, Campisi J. Chronic inflammation (inflammaging) and its potential contribution to age-associated diseases. J Gerontol Biol Sci Med Sci (2014) 69 Suppl 1:S4–09. doi: 10.1093/gerona/glu057
9. Pinti M, Cevenini E, Nasi M, De Biasi S, Salvioli S, Monti D, et al. Circulating mitochondrial DNA increases with age and is a familiar trait: Implications for “inflamm-aging”. Eur J Immunol (2014) 44:1552–62. doi: 10.1002/eji.201343921
10. Olivieri F, Rippo MR, Procopio AD, Fazioli F. Circulating inflamma-miRs in aging and age-related diseases. Front Genet (2013) 4:121. doi: 10.3389/fgene.2013.00121
11. Abd ES, Al-Shreef FM. Inflammatory cytokines and immune system modulation by aerobic versus resisted exercise training for elderly. Afr Health Sci (2018) 18:120–31. doi: 10.4314/ahs.v18i1.16
12. Krabbe KS, Pedersen M, Bruunsgaard H. Inflammatory mediators in the elderly. Exp Gerontol (2004) 39:687–99. doi: 10.1016/j.exger.2004.01.009
13. Maggio M, Basaria S, Ceda GP, Ble A, Ling SM, Bandinelli S, et al. The relationship between testosterone and molecular markers of inflammation in older men. J Endocrinol Invest (2005) 28:116–19.
14. Lopes-Paciencia S, Saint-Germain E, Rowell MC, Ruiz AF, Kalegari P, Ferbeyre G. The senescence-associated secretory phenotype and its regulation. Cytokine (2019) 117:15–22. doi: 10.1016/j.cyto.2019.01.013
15. Pelletier RM. The blood-testis barrier: the junctional permeability, the proteins and the lipids. Prog Histochem Cytochem (2011) 46:49–127. doi: 10.1016/j.proghi.2011.05.001
16. Zhang W, Xia S, Xiao W, Song Y, Tang L, Cao M, et al. A single-cell transcriptomic landscape of mouse testicular aging. J Adv Res (2022) 53:219–34. doi: 10.1016/j.jare.2022.12.007
17. Kaprara A, Huhtaniemi IT. The hypothalamus-pituitary-gonad axis: Tales of mice and men. Metabolism (2018) 86:3–17. doi: 10.1016/j.metabol.2017.11.018
18. Xie Q, Kang Y, Zhang C, Xie Y, Wang C, Liu J, et al. The role of kisspeptin in the control of the hypothalamic-pituitary-gonadal axis and reproduction. Front Endocrinol (Lausanne) (2022) 13:925206. doi: 10.3389/fendo.2022.925206
19. McCann SM, MArubayashi U, Sun HQ, Yu WH. Control of follicle-stimulating hormone and luteinizing hormone release by hypothalamic peptides. Ann N Y Acad Sci (1993) 687:55–9. doi: 10.1111/j.1749-6632.1993.tb43853.x
20. Choi J, Smitz J. Luteinizing hormone and human chorionic gonadotropin: origins of difference. Mol Cell Endocrinol (2014) 383:203–13. doi: 10.1016/j.mce.2013.12.009
21. Clark BJ. ACTH action on stAR biology. Front Neurosci (2016) 10:547. doi: 10.3389/fnins.2016.00547
22. Miller WL. Steroid hormone synthesis in mitochondria. Mol Cell Endocrinol (2013) 379:62–73. doi: 10.1016/j.mce.2013.04.014
23. Hebert-Schuster M, Rotta BE, Kirkpatrick B, Guibourdenche J, Cohen M. The interplay between glucose-regulated protein 78 (GRP78) and steroids in the reproductive system. Int J Mol Sci (2018) 19(7):1842. doi: 10.3390/ijms19071842
24. Payne AH, Hales DB. Overview of steroidogenic enzymes in the pathway from cholesterol to active steroid hormones. Endocr Rev (2004) 25:947–70. doi: 10.1210/er.2003-0030
25. Wang Y, Dong C, Han Y, Gu Z, Sun C. Immunosenescence, aging and successful aging. Front Immunol (2022) 13:942796. doi: 10.3389/fimmu.2022.942796
26. Pawelec G. Age and immunity: what is “immunosenescence”? Exp Gerontol (2018) 105:4–09. doi: 10.1016/j.exger.2017.10.024
27. Franceschi C, Garagnani P, Parini P, Giuliani C, Santoro A. Inflammaging: a new immune-metabolic viewpoint for age-related diseases. Nat Rev Endocrinol (2018) 14:576–90. doi: 10.1038/s41574-018-0059-4
28. Huang Z, Chen B, Liu X, Li H, Xie L, Gao Y, et al. Effects of sex and aging on the immune cell landscape as assessed by single-cell transcriptomic analysis. Proc Natl Acad Sci U S (2021) 118(33):e2023216118. doi: 10.1073/pnas.2023216118
29. Martinez-Zamudio RI, Dewald HK, Vasilopoulos T, Gittens-Williams L, Fitzgerald-Bocarsly P, Herbig U. Senescence-associated beta-galactosidase reveals the abundance of senescent CD8+ T cells in aging humans. Aging Cell (2021) 20:e13344. doi: 10.1111/acel.13344
30. Baixauli F, Acin-Perez R, Villarroya-Beltri C, Mazzeo C, Nunez-Andrade N, Gabande-Rodriguez E, et al. Mitochondrial respiration controls lysosomal function during inflammatory T cell responses. Cell Metab (2015) 22:485–98. doi: 10.1016/j.cmet.2015.07.020
31. Desdin-Mico G, Soto-Heredero G, Aranda JF, Oller J, Carrasco E, Gabande-Rodriguez E, et al. T cells with dysfunctional mitochondria induce multimorbidity and premature senescence. Science (2020) 368:1371–76. doi: 10.1126/science.aax0860
32. Poznanski SM, Ashkar AA. What defines NK cell functional fate: phenotype or metabolism? Front Immunol (2019) 10:1414. doi: 10.3389/fimmu.2019.01414
33. Wendt K, Wilk E, Buyny S, Buer J, Schmidt RE, Jacobs R. Gene and protein characteristics reflect functional diversity of CD56dim and CD56bright NK cells. J Leukoc Biol (2006) 80:1529–41. doi: 10.1189/jlb.0306191
34. Khummuang S, Chuensirikulchai K, Pata S, Laopajon W, Chruewkamlow N, Mahasongkram K, et al. Characterization and functional analysis of novel circulating NK cell sub-populations. Int Immunol (2019) 31:515–30. doi: 10.1093/intimm/dxz027
35. Bjorkstrom NK, Riese P, Heuts F, Andersson S, Fauriat C, Ivarsson MA, et al. Expression patterns of NKG2A, KIR, and CD57 define a process of CD56dim NK-cell differentiation uncoupled from NK-cell education. Blood (2010) 116:3853–64. doi: 10.1182/blood-2010-04-281675
36. Le Garff-Tavernier M, Beziat V, Decocq J, Siguret V, Gandjbakhch F, Pautas E, et al. Human NK cells display major phenotypic and functional changes over the life span. Aging Cell (2010) 9:527–35. doi: 10.1111/j.1474-9726.2010.00584.x
37. Liu M, Meng Y, Zhang L, Han Z, Feng X. High-efficient generation of natural killer cells from peripheral blood with preferable cell vitality and enhanced cytotoxicity by combination of IL-2, IL-15 and IL-18. Biochem Biophys Res Commun (2021) 534:149–56. doi: 10.1016/j.bbrc.2020.12.012
38. Rink L, Cakman I, Kirchner H. Altered cytokine production in the elderly. Mech Ageing Dev (1998) 102:199–209. doi: 10.1016/s0047-6374(97)00153-x
39. Seidler S, Zimmermann HW, Bartneck M, Trautwein C, Tacke F. Age-dependent alterations of monocyte subsets and monocyte-related chemokine pathways in healthy adults. BMC Immunol (2010) 11:30. doi: 10.1186/1471-2172-11-30
40. Hearps AC, Martin GE, Angelovich TA, Cheng WJ, Maisa A, Landay AL, et al. Aging is associated with chronic innate immune activation and dysregulation of monocyte phenotype and function. Aging Cell (2012) 11:867–75. doi: 10.1111/j.1474-9726.2012.00851.x
41. Nyugen J, Agrawal S, Gollapudi S, Gupta S. Impaired functions of peripheral blood monocyte subpopulations in aged humans. J Clin Immunol (2010) 30:806–13. doi: 10.1007/s10875-010-9448-8
42. Merino A, Buendia P, Martin-Malo A, Aljama P, Ramirez R, Carracedo J. Senescent CD14+CD16+ monocytes exhibit proinflammatory and proatherosclerotic activity. J Immunol (2011) 186:1809–15. doi: 10.4049/jimmunol.1001866
43. Ong SM, Hadadi E, Dang TM, Yeap WH, Tan CT, Ng TP, et al. The pro-inflammatory phenotype of the human non-classical monocyte subset is attributed to senescence. Cell Death Dis (2018) 9:266. doi: 10.1038/s41419-018-0327-1
44. Macri C, Pang ES, Patton T, O’Keeffe M. Dendritic cell subsets. Semin Cell Dev Biol (2018) 84:11–21. doi: 10.1016/j.semcdb.2017.12.009
45. Agrawal A, Agrawal S, Cao JN, Su H, Osann K, Gupta S. Altered innate immune functioning of dendritic cells in elderly humans: a role of phosphoinositide 3-kinase-signaling pathway. J Immunol (2007) 178:6912–22. doi: 10.4049/jimmunol.178.11.6912
46. Jing Y, Shaheen E, Drake RR, Chen N, Gravenstein S, Deng Y. Aging is associated with a numerical and functional decline in plasmacytoid dendritic cells, whereas myeloid dendritic cells are relatively unaltered in human peripheral blood. Hum Immunol (2009) 70:777–84. doi: 10.1016/j.humimm.2009.07.005
47. Agrawal A, Sridharan A, Prakash S, Agrawal H. Dendritic cells and aging: consequences for autoimmunity. Expert Rev Clin Immunol (2012) 8:73–80. doi: 10.1586/eci.11.77
48. Della BS, Bierti L, Presicce P, Arienti R, Valenti M, Saresella M, et al. Peripheral blood dendritic cells and monocytes are differently regulated in the elderly. Clin Immunol (2007) 122:220–28. doi: 10.1016/j.clim.2006.09.012
49. Shodell M, Siegal FP. Circulating, interferon-producing plasmacytoid dendritic cells decline during human ageing. Scand J Immunol (2002) 56:518–21. doi: 10.1046/j.1365-3083.2002.01148.x
50. Agrawal A, Tay J, Ton S, Agrawal S, Gupta S. Increased reactivity of dendritic cells from aged subjects to self-antigen, the human DNA. J Immunol (2009) 182:1138–45. doi: 10.4049/jimmunol.182.2.1138
51. Hutson JC. Interactions between testicular macrophages and Leydig cells. J Androl (1998) 19:394–98. doi: 10.1002/j.1939-4640.1998.tb02030.x
52. Shechter R, London A, Schwartz M. Orchestrated leukocyte recruitment to immune-privileged sites: absolute barriers versus educational gates. Nat Rev Immunol (2013) 13:206–18. doi: 10.1038/nri3391
53. Smith LB, O’Shaughnessy PJ, Rebourcet D. Cell-specific ablation in the testis: what have we learned? Andrology (2015) 3:1035–49. doi: 10.1111/andr.12107
54. Mossadegh-Keller N, Gentek R, Gimenez G, Bigot S, Mailfert S, Sieweke MH. Developmental origin and maintenance of distinct testicular macrophage populations. J Exp Med (2017) 214:2829–41. doi: 10.1084/jem.20170829
55. Fijak M, Meinhardt A. The testis in immune privilege. Immunol Rev (2006) 213:66–81. doi: 10.1111/j.1600-065X.2006.00438.x
56. Lukyanenko YO, Chen JJ, Hutson JC. Production of 25-hydroxycholesterol by testicular macrophages and its effects on Leydig cells. Biol Reprod (2001) 64:790–96. doi: 10.1095/biolreprod64.3.790
57. Giannessi F, Giambelluca MA, Scavuzzo MC, Ruffoli R. Ultrastructure of testicular macrophages in aging mice. J Morphol (2005) 263:39–46. doi: 10.1002/jmor.10287
58. Nie X, Munyoki SK, Sukhwani M, Schmid N, Missel A, Emery BR, et al. Single-cell analysis of human testis aging and correlation with elevated body mass index. Dev Cell (2022) 57:1160–76. doi: 10.1016/j.devcel.2022.04.004
59. Cai D, Khor S. “Hypothalamic microinflammation” Paradigm in aging and metabolic diseases. Cell Metab (2019) 30:19–35. doi: 10.1016/j.cmet.2019.05.021
60. Zhang G, Li J, Purkayastha S, Tang Y, Zhang H, Yin Y, et al. Hypothalamic programming of systemic ageing involving IKK-beta, NF-kappaB and GnRH. Nature (2013) 497:211–16. doi: 10.1038/nature12143
61. Vennekens A, Laporte E, Hermans F, Cox B, Modave E, Janiszewski A, et al. Interleukin-6 is an activator of pituitary stem cells upon local damage, a competence quenched in the aging gland. Proc Natl Acad Sci U S (2021) 118(25):e2100052118. doi: 10.1073/pnas.2100052118
62. Hales DB. Testicular macrophage modulation of Leydig cell steroidogenesis. J Reprod Immunol (2002) 57:3–18. doi: 10.1016/s0165-0378(02)00020-7
63. Roger L, Tomas F, Gire V. Mechanisms and regulation of cellular senescence. Int J Mol Sci (2021) 22(23):13173. doi: 10.3390/ijms222313173
64. Hayflick L, Moorhead PS. The serial cultivation of human diploid cell strains. Exp Cell Res (1961) 25:585–621. doi: 10.1016/0014-4827(61)90192-6
65. Giardini MA, Segatto M, Da SM, Nunes VS, Cano MI. Telomere and telomerase biology. Prog Mol Biol Transl Sci (2014) 125:1–40. doi: 10.1016/B978-0-12-397898-1.00001-3
66. Rossiello F, Jurk D, Passos JF, D’Adda DFF. Telomere dysfunction in ageing and age-related diseases. Nat Cell Biol (2022) 24:135–47. doi: 10.1038/s41556-022-00842-x
67. Habiballa L, Salmonowicz H, Passos JF. Mitochondria and cellular senescence: Implications for musculoskeletal ageing. Free Radic Biol Med (2019) 132:3–10. doi: 10.1016/j.freeradbiomed.2018.10.417
68. Lee BY, Han JA, Im JS, Morrone A, Johung K, Goodwin EC, et al. Senescence-associated beta-galactosidase is lysosomal beta-galactosidase. Aging Cell (2006) 5:187–95. doi: 10.1111/j.1474-9726.2006.00199.x
69. Xie J, Wang Y, Lu L, Liu L, Yu X, Pei F. Cellular senescence in knee osteoarthritis: molecular mechanisms and therapeutic implications. Ageing Res Rev (2021) 70:101413. doi: 10.1016/j.arr.2021.101413
70. Xiaohu J, Su G, Yuying Z, Simin C, Wenyan W, Jingjing Y, et al. Traditional Chinese Medicine formula Wubi Shanyao Pills protects against reproductive aging by activating SIRT1/3 to reduce apoptosis. J Ethnopharmacol (2023) 318:116976. doi: 10.1016/j.jep.2023.116976
71. Jeong MG, Song H, Shin JH, Jeong H, Kim HK, Hwang ES. Transcriptional coactivator with PDZ-binding motif is required to sustain testicular function on aging. Aging Cell (2017) 16:1035–42. doi: 10.1111/acel.12631
72. Birch J, Gil J. Senescence and the SASP: many therapeutic avenues. Genes Dev (2020) 34:1565–76. doi: 10.1101/gad.343129.120
73. Coryell PR, Diekman BO, Loeser RF. Mechanisms and therapeutic implications of cellular senescence in osteoarthritis. Nat Rev Rheumatol (2021) 17:47–57. doi: 10.1038/s41584-020-00533-7
74. He S, Sharpless NE. Senescence in health and disease. Cell (2017) 169:1000–11. doi: 10.1016/j.cell.2017.05.015
75. Midha A, Pan H, Abarca C, Andle J, Carapeto P, Bonner-Weir S, et al. Unique human and mouse beta-cell senescence-associated secretory phenotype (SASP) reveal conserved signaling pathways and heterogeneous factors. Diabetes (2021) 70:1098–116. doi: 10.2337/db20-0553
76. Oeckinghaus A, Hayden MS, Ghosh S. Crosstalk in NF-kappaB signaling pathways. Nat Immunol (2011) 12:695–708. doi: 10.1038/ni.2065
77. Vallabhapurapu S, Karin M. Regulation and function of NF-kappaB transcription factors in the immune system. Annu Rev Immunol (2009) 27:693–733. doi: 10.1146/annurev.immunol.021908.132641
78. Salminen A, Kauppinen A, Kaarniranta K. Emerging role of NF-kappaB signaling in the induction of senescence-associated secretory phenotype (SASP). Cell Signal (2012) 24:835–45. doi: 10.1016/j.cellsig.2011.12.006
79. Sebastian T, Malik R, Thomas S, Sage J, Johnson PF. C/EBPbeta cooperates with RB:E2F to implement Ras(V12)-induced cellular senescence. EMBO J (2005) 24:3301–12. doi: 10.1038/sj.emboj.7600789
80. Stein B, Cogswell PC, Baldwin AJ. Functional and physical associations between NF-kappa B and C/EBP family members: a Rel domain-bZIP interaction. Mol Cell Biol (1993) 13:3964–74. doi: 10.1128/mcb.13.7.3964-3974.1993
81. Shang T, Zhang X, Wang T, Sun B, Deng T, Han D. Toll-like receptor-initiated testicular innate immune responses in mouse Leydig cells. Endocrinology (2011) 152:2827–36. doi: 10.1210/en.2011-0031
82. Kim EK, Choi EJ. Compromised MAPK signaling in human diseases: an update. Arch Toxicol (2015) 89:867–82. doi: 10.1007/s00204-015-1472-2
83. Kyriakis JM, Avruch J. Mammalian MAPK signal transduction pathways activated by stress and inflammation: a 10-year update. Physiol Rev (2012) 92:689–737. doi: 10.1152/physrev.00028.2011
84. Freund A, Patil CK, Campisi J. p38MAPK is a novel DNA damage response-independent regulator of the senescence-associated secretory phenotype. EMBO J (2011) 30:1536–48. doi: 10.1038/emboj.2011.69
85. Kim HA, Seo GY, Kim PH. Macrophage-derived BAFF induces AID expression through the p38MAPK/CREB and JNK/AP-1 pathways. J Leukoc Biol (2011) 89:393–98. doi: 10.1189/jlb.1209787
86. Liu P, Gao Q, Guan L, Sheng W, Hu Y, Gao T, et al. Atorvastatin attenuates isoflurane-induced activation of ROS-p38MAPK/ATF2 pathway, neuronal degeneration, and cognitive impairment of the aged mice. Front Aging Neurosci (2020) 12:620946. doi: 10.3389/fnagi.2020.620946
87. Luo D, Qi X, Xu X, Yang L, Yu C, Guan Q. Involvement of p38 MAPK in Leydig cell aging and age-related decline in testosterone. Front Endocrinol (Lausanne) (2023) 14:1088249. doi: 10.3389/fendo.2023.1088249
88. Acosta JC, Banito A, Wuestefeld T, Georgilis A, Janich P, Morton JP, et al. A complex secretory program orchestrated by the inflammasome controls paracrine senescence. Nat Cell Biol (2013) 15:978–90. doi: 10.1038/ncb2784
89. Deppmann CD, Alvania RS, Taparowsky EJ. Cross-species annotation of basic leucine zipper factor interactions: Insight into the evolution of closed interaction networks. Mol Biol Evol (2006) 23:1480–92. doi: 10.1093/molbev/msl022
90. Salotti J, Johnson PF. Regulation of senescence and the SASP by the transcription factor C/EBPbeta. Exp Gerontol (2019) 128:110752. doi: 10.1016/j.exger.2019.110752
91. Manna PR, Dyson MT, Stocco DM. Role of basic leucine zipper proteins in transcriptional regulation of the steroidogenic acute regulatory protein gene. Mol Cell Endocrinol (2009) 302:1–11. doi: 10.1016/j.mce.2008.12.009
92. Martin LJ, Nguyen HT. Basic leucine zipper transcription factors as important regulators of leydig cells’ Functions. Int J Mol Sci (2022) 23(21):12887. doi: 10.3390/ijms232112887
93. Roger AJ, Munoz-Gomez SA, Kamikawa R. The origin and diversification of mitochondria. Curr Biol (2017) 27:R1177–92. doi: 10.1016/j.cub.2017.09.015
94. Brzoskwinia M, Pardyak L, Kaminska A, Tworzydlo W, Hejmej A, Marek S, et al. Flutamide treatment reveals a relationship between steroidogenic activity of Leydig cells and ultrastructure of their mitochondria. Sci Rep (2021) 11:13772. doi: 10.1038/s41598-021-93292-8
95. Galano M, Li Y, Li L, Sottas C, Papadopoulos V. Role of constitutive STAR in leydig cells. Int J Mol Sci (2021) 22(4):2021. doi: 10.3390/ijms22042021
96. Midzak A, Rone M, Aghazadeh Y, Culty M, Papadopoulos V. Mitochondrial protein import and the genesis of steroidogenic mitochondria. Mol Cell Endocrinol (2011) 336:70–9. doi: 10.1016/j.mce.2010.12.007
97. Midzak AS, Chen H, Aon MA, Papadopoulos V, Zirkin BR. ATP synthesis, mitochondrial function, and steroid biosynthesis in rodent primary and tumor Leydig cells. Biol Reprod (2011) 84:976–85. doi: 10.1095/biolreprod.110.087460
98. Miquel J. Can antioxidant diet supplementation protect against age-related mitochondrial damage? Ann N Y Acad Sci (2002) 959:508–16. doi: 10.1111/j.1749-6632.2002.tb02120.x
99. Midzak AS, Chen H, Papadopoulos V, Zirkin BR. Leydig cell aging and the mechanisms of reduced testosterone synthesis. Mol Cell Endocrinol (2009) 299:23–31. doi: 10.1016/j.mce.2008.07.016
100. Culty M, Luo L, Yao ZX, Chen H, Papadopoulos V, Zirkin BR. Cholesterol transport, peripheral benzodiazepine receptor, and steroidogenesis in aging Leydig cells. J Androl (2002) 23:439–47. doi: 10.1002/j.1939-4640.2002.tb02251.x
101. Galano M, Papadopoulos V. Role of constitutive STAR in mitochondrial structure and function in MA-10 leydig cells. Endocrinology (2022) 163(8):bqac091. doi: 10.1210/endocr/bqac091
102. Beattie MC, Adekola L, Papadopoulos V, Chen H, Zirkin BR. Leydig cell aging and hypogonadism. Exp Gerontol (2015) 68:87–91. doi: 10.1016/j.exger.2015.02.014
103. Zhou R, Yazdi AS, Menu P, Tschopp J. A role for mitochondria in NLRP3 inflammasome activation. Nature (2011) 469:221–25. doi: 10.1038/nature09663
104. Afonina IS, Zhong Z, Karin M, Beyaert R. Limiting inflammation-the negative regulation of NF-kappaB and the NLRP3 inflammasome. Nat Immunol (2017) 18:861–69. doi: 10.1038/ni.3772
105. Chen X, Liu G, Yuan Y, Wu G, Wang S, Yuan L. NEK7 interacts with NLRP3 to modulate the pyroptosis in inflammatory bowel disease via NF-kappaB signaling. Cell Death Dis (2019) 10:906. doi: 10.1038/s41419-019-2157-1
106. Li MY, Zhu XL, Zhao BX, Shi L, Wang W, Hu W, et al. Adrenomedullin alleviates the pyroptosis of Leydig cells by promoting autophagy via the ROS-AMPK-mTOR axis. Cell Death Dis (2019) 10:489. doi: 10.1038/s41419-019-1728-5
107. Mizushima N, Komatsu M. Autophagy: renovation of cells and tissues. Cell (2011) 147:728–41. doi: 10.1016/j.cell.2011.10.026
108. Duraes FV, Niven J, Dubrot J, Hugues S, Gannage M. Macroautophagy in endogenous processing of self- and pathogen-derived antigens for MHC class II presentation. Front Immunol (2015) 6:459. doi: 10.3389/fimmu.2015.00459
109. Gubas A, Dikic I. ER remodeling via ER-phagy. Mol Cell (2022) 82:1492–500. doi: 10.1016/j.molcel.2022.02.018
110. Wang L, Klionsky DJ, Shen HM. The emerging mechanisms and functions of microautophagy. Nat Rev Mol Cell Biol (2023) 24:186–203. doi: 10.1038/s41580-022-00529-z
111. Youle RJ, Narendra DP. Mechanisms of mitophagy. Nat Rev Mol Cell Biol (2011) 12:9–14. doi: 10.1038/nrm3028
112. Gao F, Li G, Liu C, Gao H, Wang H, Liu W, et al. Autophagy regulates testosterone synthesis by facilitating cholesterol uptake in Leydig cells. J Cell Biol (2018) 217:2103–19. doi: 10.1083/jcb.201710078
113. Wang M, Zhu CQ, Zeng L, Cheng L, Ma L, Zhang M, et al. Melatonin regulates the cross-talk between autophagy and apoptosis by SIRT3 in testicular Leydig cells. Biochem Biophys Res Commun (2021) 555:182–89. doi: 10.1016/j.bbrc.2021.03.138
114. Yahyavy S, Valizadeh A, Saki G, Khorsandi L. Taurine induces autophagy and inhibits oxidative stress in mice Leydig cells. Jbra Assist Reprod (2020) 24:250–56. doi: 10.5935/1518-0557.20190079
115. Tandler B, Hoppel CL, Mears JA. Morphological pathways of mitochondrial division. Antioxidants (Basel) (2018) 7(2):30. doi: 10.3390/antiox7020030
116. Twig G, Elorza A, Molina AJ, Mohamed H, Wikstrom JD, Walzer G, et al. Fission and selective fusion govern mitochondrial segregation and elimination by autophagy. EMBO J (2008) 27:433–46. doi: 10.1038/sj.emboj.7601963
117. Chen Y, Wang J, Xu D, Xiang Z, Ding J, Yang X, et al. m(6)A mRNA methylation regulates testosterone synthesis through modulating autophagy in Leydig cells. Autophagy (2021) 17:457–75. doi: 10.1080/15548627.2020.1720431
118. Yi L, Shang XJ, Lv L, Wang Y, Zhang J, Quan C, et al. Cadmium-induced apoptosis of Leydig cells is mediated by excessive mitochondrial fission and inhibition of mitophagy. Cell Death Dis (2022) 13:928. doi: 10.1038/s41419-022-05364-w
119. Barbonetti A, D’Andrea S, Francavilla S. Testosterone replacement therapy. Andrology (2020) 8:1551–66. doi: 10.1111/andr.12774
120. Collado-Mateo D, Lavin-Perez AM, Penacoba C, Del CJ, Leyton-Roman M, Luque-Casado A, et al. Key factors associated with adherence to physical exercise in patients with chronic diseases and older adults: an umbrella review. Int J Environ Res Public Health (2021) 18(4):2023. doi: 10.3390/ijerph18042023
121. Pedersen LR, Olsen RH, Anholm C, Astrup A, Eugen-Olsen J, Fenger M, et al. Effects of 1 year of exercise training versus combined exercise training and weight loss on body composition, low-grade inflammation and lipids in overweight patients with coronary artery disease: a randomized trial. Cardiovasc Diabetol (2019) 18:127. doi: 10.1186/s12933-019-0934-x
122. Franzoni F, Ghiadoni L, Galetta F, Plantinga Y, Lubrano V, Huang Y, et al. Physical activity, plasma antioxidant capacity, and endothelium-dependent vasodilation in young and older men. Am J Hypertens (2005) 18:510–16. doi: 10.1016/j.amjhyper.2004.11.006
123. Leitao AE, Vieira M, Pelegrini A, Da SE, Guimaraes A. A 6-month, double-blind, placebo-controlled, randomized trial to evaluate the effect of Eurycoma longifolia (Tongkat Ali) and concurrent training on erectile function and testosterone levels in androgen deficiency of aging males (ADAM). Maturitas (2021) 145:78–85. doi: 10.1016/j.maturitas.2020.12.002
124. Li YY, Hwang IS, O WS, Tang F. Adrenomedullin peptide: gene expression of adrenomedullin, its receptors and receptor activity modifying proteins, and receptor binding in rat testis–actions on testosterone secretion. Biol Reprod (2006) 75:183–88. doi: 10.1095/biolreprod.106.052274
125. Sakata J, Shimokubo T, Kitamura K, Nishizono M, Iehiki Y, Kangawa K, et al. Distribution and characterization of immunoreactive rat adrenomedullin in tissue and plasma. FEBS Lett (1994) 352:105–08. doi: 10.1016/0014-5793(94)00928-7
126. Xian X, Sakurai T, Kamiyoshi A, Ichikawa-Shindo Y, Tanaka M, Koyama T, et al. Vasoprotective activities of the adrenomedullin-RAMP2 system in endothelial cells. Endocrinology (2017) 158:1359–72. doi: 10.1210/en.2016-1531
127. Chan YF, WS O, Tang F. Adrenomedullin in the rat testis. I: Its production, actions on testosterone secretion, regulation by human chorionic gonadotropin, and its interaction with endothelin 1 in the leydig cell. Biol Reprod (2008) 78:773–79. doi: 10.1095/biolreprod.107.060871
128. Hu W, Shi L, Li MY, Zhou PH, Qiu B, Yin K, et al. Adrenomedullin protects Leydig cells against lipopolysaccharide-induced oxidative stress and inflammatory reaction via MAPK/NF-kappaB signalling pathways. Sci Rep (2017) 7:16479. doi: 10.1038/s41598-017-16008-x
129. Zhou PH, Hu W, Zhang XB, Wang W, Zhang LJ. Protective effect of adrenomedullin on rat leydig cells from lipopolysaccharide-induced inflammation and apoptosis via the PI3K/akt signaling pathway ADM on rat leydig cells from inflammation and apoptosis. Mediators Inflammation (2016) 2016:7201549. doi: 10.1155/2016/7201549
130. Szeto HH, Schiller PW. Novel therapies targeting inner mitochondrial membrane–from discovery to clinical development. Pharm Res (2011) 28:2669–79. doi: 10.1007/s11095-011-0476-8
131. Chavez JD, Tang X, Campbell MD, Reyes G, Kramer PA, Stuppard R, et al. Mitochondrial protein interaction landscape of SS-31. Proc Natl Acad Sci USA (2020) 117:15363–73. doi: 10.1073/pnas.2002250117
132. Shang L, Ren H, Wang S, Liu H, Hu A, Gou P, et al. SS-31 protects liver from ischemia-reperfusion injury via modulating macrophage polarization. Oxid Med Cell Longev (2021) 2021:6662156. doi: 10.1155/2021/6662156
133. Peng X, Wang K, Zhang C, Bao JP, Vlf C, Gao JW, et al. The mitochondrial antioxidant SS-31 attenuated lipopolysaccharide-induced apoptosis and pyroptosis of nucleus pulposus cells via scavenging mitochondrial ROS and maintaining the stability of mitochondrial dynamics. Free Radic Res (2021) 55:1080–93. doi: 10.1080/10715762.2021.2018426
134. Mo Y, Deng S, Zhang L, Huang Y, Li W, Peng Q, et al. SS-31 reduces inflammation and oxidative stress through the inhibition of Fis1 expression in lipopolysaccharide-stimulated microglia. Biochem Biophys Res Commun (2019) 520:171–78. doi: 10.1016/j.bbrc.2019.09.077
135. Ding XW, Robinson M, Li R, Aldhowayan H, Geetha T, Babu JR. Mitochondrial dysfunction and beneficial effects of mitochondria-targeted small peptide SS-31 in Diabetes Mellitus and Alzheimer’s disease. Pharmacol Res (2021) 171:105783. doi: 10.1016/j.phrs.2021.105783
136. Zhu Y, Luo M, Bai X, Li J, Nie P, Li B, et al. SS-31, a mitochondria-targeting peptide, ameliorates kidney disease. Oxid Med Cell Longev (2022) 2022:1295509. doi: 10.1155/2022/1295509
137. Kita T, Ashizuka S, Takeda T, Matsumoto T, Ohmiya N, Nakase H, et al. Adrenomedullin for biologic-resistant Crohn’s disease: A randomized, double-blind, placebo-controlled phase 2a clinical trial. J Gastroenterol Hepatol (2022) 37:2051–59. doi: 10.1111/jgh.15945
138. Luo P, Feng X, Deng R, Wang F, Zhang Y, Li X, et al. An autofluorescence-based isolation of Leydig cells for testosterone deficiency treatment. Mol Cell Endocrinol (2021) 535:111389. doi: 10.1016/j.mce.2021.111389
139. Arora H, Zuttion M, Nahar B, Lamb D, Hare JM, Ramasamy R. Subcutaneous leydig stem cell autograft: A promising strategy to increase serum testosterone. Stem Cells Transl Med (2019) 8:58–65. doi: 10.1002/sctm.18-0069
140. Wang Z, Yang T, Liu S, Chen Y. Effects of bone marrow mesenchymal stem cells on ovarian and testicular function in aging Sprague-Dawley rats induced by D-galactose. Cell Cycle (2020) 19:2340–50. doi: 10.1080/15384101.2020.1806434
141. Gu Y, Zhang X, Sun D, Zhao H, Cai B, Gao C, et al. The stimulative effect of yangjing capsule on testosterone synthesis through nur77 pathway in leydig cells. Evid Based Complement Alternat Med (2015) 2015:408686. doi: 10.1155/2015/408686
142. Sun D, Dong W, Jin B, Chen G, Cai B, Deng W, et al. Mechanisms of yangjing capsule in leydig cell apoptosis and testosterone synthesis via promoting stAR expression. Biol Pharm Bull (2018) 41:1401–05. doi: 10.1248/bpb.b18-00205
143. Bi Z, Zhang W, Yan X. Anti-inflammatory and immunoregulatory effects of icariin and icaritin. BioMed Pharmacother (2022) 151:113180. doi: 10.1016/j.biopha.2022.113180
144. Zhao H, Shan Y, Ma Z, Yu M, Gong B. A network pharmacology approach to explore active compounds and pharmacological mechanisms of epimedium for treatment of premature ovarian insufficiency. Drug Des Devel Ther (2019) 13:2997–3007. doi: 10.2147/DDDT.S207823
145. Sun J, Wang D, Lin J, Liu Y, Xu L, Lv R, et al. Icariin protects mouse Leydig cell testosterone synthesis from the adverse effects of di(2-ethylhexyl) phthalate. Toxicol Appl Pharmacol (2019) 378:114612. doi: 10.1016/j.taap.2019.114612
146. Sun J, Xu W, Zheng S, Lv C, Lin J, Chen S, et al. Icariin promotes mouse Leydig cell testosterone synthesis via the Esr1/Src/Akt/Creb/Sf-1 pathway. Toxicol Appl Pharmacol (2022) 441:115969. doi: 10.1016/j.taap.2022.115969
147. Shen F, Song Z, Xie P, Li L, Wang B, Peng D, et al. Polygonatum sibiricum polysaccharide prevents depression-like behaviors by reducing oxidative stress, inflammation, and cellular and synaptic damage. J Ethnopharmacol (2021) 275:114164. doi: 10.1016/j.jep.2021.114164
148. Han C, Zhu Y, Yang Z, Fu S, Zhang W, Liu C. Protective effect of Polygonatum sibiricum against cadmium-induced testicular injury in mice through inhibiting oxidative stress and mitochondria-mediated apoptosis. J Ethnopharmacol (2020) 261:113060. doi: 10.1016/j.jep.2020.113060
149. Zhang Q, Yang C, Zhang M, Lu X, Cao W, Xie C, et al. Protective effects of ginseng stem-leaf saponins on D-galactose-induced reproductive injury in male mice. Aging (Albany NY) (2021) 13:8916–28. doi: 10.18632/aging.202709
150. Alanazi HH, Elfaki E. The immunomodulatory role of withania somnifera (L.) dunal in inflammatory diseases. Front Pharmacol (2023) 14:1084757. doi: 10.3389/fphar.2023.1084757
151. Speers AB, Cabey KA, Soumyanath A, Wright KM. Effects of withania somnifera (Ashwagandha) on stress and the stress- related neuropsychiatric disorders anxiety, depression, and insomnia. Curr Neuropharmacol (2021) 19:1468–95. doi: 10.2174/1570159X19666210712151556
Keywords: Leydig cell, late-onset hypogonadism (LOH), inflammaging, mitochondrial, senescence-associated secretory phenotype (SASP)
Citation: Xing D, Jin Y and Jin B (2024) A narrative review on inflammaging and late-onset hypogonadism. Front. Endocrinol. 15:1291389. doi: 10.3389/fendo.2024.1291389
Received: 09 September 2023; Accepted: 02 January 2024;
Published: 17 January 2024.
Edited by:
Gabriele Christine Saretzki, Newcastle University, United KingdomReviewed by:
Mariana Toricelli, Association for Research Incentive Fund (AFIP), BrazilMarianna Cacciapuoti, San Giovanni di Dio Hospital, Italy
Xiang Qi, Jinan University, China
Copyright © 2024 Xing, Jin and Jin. This is an open-access article distributed under the terms of the Creative Commons Attribution License (CC BY). The use, distribution or reproduction in other forums is permitted, provided the original author(s) and the copyright owner(s) are credited and that the original publication in this journal is cited, in accordance with accepted academic practice. No use, distribution or reproduction is permitted which does not comply with these terms.
*Correspondence: Baofang Jin, hexiking@126.com
†ORCID: Dong Xing, orcid.org/0000-0002-5814-781X
Yihan Jin, orcid.org/0009-0008-6191-5665
Baofang Jin, orcid.org/0000-0002-5604-2897