- 1Department of Endocrinology and Metabolism, National Hospital Organization Kyoto Medical Center, Kyoto, Japan
- 2Department of Endocrinology, Metabolism, and Hypertension Research, Clinical Research Institute, National Hospital Organization Kyoto Medical Center, Kyoto, Japan
- 3Department of Molecular and Integrative Physiology, University of Michigan, Ann Arbor, MI, United States
- 4Department of Pathology, University of Michigan, Ann Arbor, MI, United States
- 5Michigan Center for Translational Pathology, University of Michigan, Ann Arbor, MI, United States
- 6Rogel Cancer Center, University of Michigan, Ann Arbor, MI, United States
- 7Department of Radiology, National Hospital Organization Kyoto Medical Center, Kyoto, Japan
- 8Department of Urology, National Hospital Organization Kyoto Medical Center, Kyoto, Japan
- 9Department of Diagnostic Pathology, National Hospital Organization Kyoto Medical Center, Kyoto, Japan
- 10Department of Pathology, Tohoku University Graduate School of Medicine, Sendai, Japan
- 11Clinical Research Institute, National Hospital Organization Kyoto Medical Center, Kyoto, Japan
- 12Division of Metabolism, Endocrinology, and Diabetes, University of Michigan, Ann Arbor, MI, United States
Double somatic mutations in CTNNB1 and GNA11/Q have recently been identified in a small subset of aldosterone-producing adenomas (APAs). As a possible pathogenesis of APA due to these mutations, an association with pregnancy, menopause, or puberty has been proposed. However, because of its rarity, characteristics of APA with these mutations have not been well characterized. A 46-year-old Japanese woman presented with hypertension and hypokalemia. She had two pregnancies in the past but had no history of pregnancy-induced hypertension. She had regular menstrual cycle at presentation and was diagnosed as having primary aldosteronism after endocrinologic examinations. Computed tomography revealed a 2 cm right adrenal mass. Adrenal venous sampling demonstrated excess aldosterone production from the right adrenal gland. She underwent right laparoscopic adrenalectomy. The resected right adrenal tumor was histologically diagnosed as adrenocortical adenoma and subsequent immunohistochemistry (IHC) revealed diffuse immunoreactivity of aldosterone synthase (CYP11B2) and visinin like 1, a marker of the zona glomerulosa (ZG), whereas 11β-hydroxylase, a steroidogenic enzyme for cortisol biosynthesis, was mostly negative. CYP11B2 IHC-guided targeted next-generation sequencing identified somatic CTNNB1 (p.D32Y) and GNA11 (p.Q209H) mutations. Immunofluorescence staining of the tumor also revealed the presence of activated β-catenin, consistent with features of the normal ZG. The expression patterns of steroidogenic enzymes and related proteins indicated ZG features of the tumor cells. PA was clinically and biochemically cured after surgery. In conclusion, our study indicated that CTNNB1 and GNA11-mutated APA has characteristics of the ZG. The disease could occur in adults with no clear association with pregnancy or menopause.
Introduction
Aldosterone-producing adenoma (APA) is a major form of primary aldosteronism (PA). In the past decade, there has been significant progress in the determination of genetic causes of APA. The use of next-generation sequencing (NGS) in APA has resulted in the identification of somatic mutations responsible for excess aldosterone production. These affected genes include KCNJ5 (1), ATP1A1 (2), ATP2B3 (2), CACNA1D (3, 4), CACNA1H (5, 6), and CLCN2 (7–9). These aldosterone-driver genes encode ion channels or transporters. Mutations in these genes directly or indirectly increase intracellular calcium levels resulting in enhanced tumor cell aldosterone synthase (CYP11B2) expression and inappropriate aldosterone production (10). More recently, somatic mutations in CADM1 (11) and SLC30A1 (12) have also been identified as rare genetic causes of APA. An immunohistochemistry (IHC)-based sequencing approach that targets CYP11B2-expressing regions using formalin-fixed, paraffin-embedded (FFPE) tissue has enabled detection of these somatic mutations in the vast majority of APAs (13–16).
As in other adrenocortical tumors such as adrenocortical carcinoma and cortisol-producing adenoma, somatic activating mutations in exon 3 of the CTNNB1 gene, that encodes β-catenin, have also been identified in 2-5% of APA (17–19). A recent study reported double somatic mutations of GNA11 or GNAQ in CTNNB1-mutated APAs (20). As a possible pathogenesis of APA harboring these double mutations, an association with pregnancy, menopause, or puberty has been proposed based on the disease onset and increased tumor expression of luteinizing hormone/choriogonadotropin receptor (LHCGR) (20). However, due to its rare incidence, characteristics of APA with these double mutations have not been well characterized. Herein, we report the detailed clinical course of a Japanese woman with APA harboring somatic CTNNB1 and GNA11 mutations. Notably, the present case had no history of pregnancy-associated hypertension or irregular menstrual cycles at presentation.
Materials and methods
Immunohistochemistry
IHC was performed on 10% FFPE tissue sections as described previously (21). The following primary antibodies were used: CYP11B2 (MilliporeSigma, MABS1251; diluted 1:1250; RRID, AB_2783793), 17α-hydroxylase/17, 20 lyase (CYP17A1) (LSBio, LS-B14227; diluted 1:2000; RRID, AB_2857939), 11β-hydroxylase (CYP11B1) (clone 80-7-3; kindly provided by Dr. Celso Gomez-Sanchez; diluted 1:50; RRID, AB_2650563), and visinin like 1 (VSNL1) (MilliporeSigma, MABN762; diluted 1:1000; RRID, AB_2832208).
Immunofluorescence staining
Immunofluorescence (IF) was performed on FFPE sections of 5 μm thickness. After deparaffinization, the slides were boiled for 15 minutes in pH 6, citrate-based buffer (Vector Laboratories) for epitope retrieval followed by 10% normal goat serum (Abcam) blocking for 1 hour. The primary antibodies to β-catenin (Cell Signaling Technology, 9562; diluted 1:100; RRID, AB_331149) and KCNJ5 (G protein-activated inward rectifier potassium channel 4) (from Dr. Celso Gomez-Sanchez; clone 36-33-5; diluted 1:1000; RRID, AB_3086774) (22) were incubated overnight at 4˚C. The fluorescent-conjugated secondary antibodies (Jackson ImmunoResearch, 111-545-144; diluted 1:100; RRID, AB_2338052 and Thermo Fisher Scientific, A-11032; diluted 1:100; RRID, AB_2534091) were then incubated for 1 hour at room temperature followed by autofluorescence quenching with TrueBlack® Lipofuscin Autofluorescence Quencher (Biotium) for 30 seconds. Finally, coverslips were mounted with 4’,6-diamidino-2-phenylindole (DAPI).
DNA and RNA isolation
Genomic DNA (gDNA) and RNA from APA and adjacent normal adrenal tissue were isolated separately from serial FFPE tissue sections using the AllPrep DNA/RNA FFPE kit (QIAGEN) as described previously (23). gDNA and RNA were used for targeted NGS and quantitative real-time RT-PCR (qPCR), respectively.
Targeted NGS
Ion Torrent™-based targeted NGS (Thermo Fisher Scientific) was used for sequencing analysis. The custom Ion AmpliSeq™ panel for targeted NGS included the full coding regions of following genes: KCNJ5, ATP1A1, ATP2B3, CACNA1D, CACNA1H, CLCN2, CADM1, SLC30A1, CTNNB1, GNAS, and GNA11. The methods for targeted NGS, including library preparation, sequencing, and variant calling, were performed as described previously (23).
Quantitative real-time RT-PCR
RNA was reverse transcribed using the high-capacity complementary DNA (cDNA) archive kit (Life Technologies). qPCR was performed using the StepOnePlus™ Real-Time PCR systems (Applied Biosystems) (23). The primer-probe sets for CYP11B2 were designed in house and manufactured by IDT DNA (24). The following primer-probe sets were purchased from Thermo Fisher Scientific: LHCGR (Hs00174885_m1), GNRHR (gonadotropin-releasing hormone receptor) (Hs00171248_m1), and ACTB (β-actin) (Hs01060665_g1). ACTB transcript was used as an internal control for quantitative normalization. The delta-delta threshold cycle method was used to calculate fold changes in mRNA expression over adjacent normal adrenal.
This study was approved by the institutional review boards at the National Hospital Organization Kyoto Medical Center (20–038) and the University of Michigan (HUM00083056). The patient provided written consent for the use of specimen in this study and publication of this article.
Results
Case presentation
A 46-year-old Japanese woman was referred to us for the investigation of PA. She had been hypertensive at least for 4 months (her blood pressure was 216/105 mmHg at initial visit of the referring hospital). She had two pregnancies at the ages of 22 and 23 but had no history of pregnancy-associated hypertension or other complications according to her Maternal and Child Handbooks (25). Although she had menopause-like symptoms such as headaches, sweating, and fatigue, her menstrual cycle was regular at the time of presentation. She had urolithiasis at the age of 40. Computed tomography (CT) for the evaluation of urolithiasis detected a right adrenal tumor. However, no further investigation was performed at that time. She had no family history of endocrine disorders.
Laboratory testing showed hypokalemia and elevated plasma aldosterone concentration with suppressed renin (Table 1). She was diagnosed as having PA based on the results of captopril challenge test (Table 1) (26). Concomitant cortisol excess was not documented (Table 1). Adrenal CT revealed a 2 cm right adrenal mass (Figures 1A, B). Left adrenal was intact by imaging. Adrenal venous sampling indicated excess aldosterone production from the right adrenal gland (Table 2). 131I-6β-iodomethyl-19-norcholesterol (NP-59) scintigraphy with dexamethasone suppression further demonstrated increased tracer uptake in the right adrenal lesion (Figure 1C). She underwent right laparoscopic adrenalectomy. The resected tumor was histologically diagnosed as adrenocortical adenoma according to the criteria of Weiss (27) and also harboring the foci of pseudoglandular formations (Figures 2A, B). Notably, Ki-67 labeling index was high (6% at hotspots) (Figure 2C). After surgery, her blood pressure and serum potassium were both normalized. Based on the primary aldosteronism surgical outcome (PASO) study criteria (28), PA was clinically and biochemically cured after surgery (Table 3). No tumor recurrence was observed by imaging study performed at 2 years after surgery.
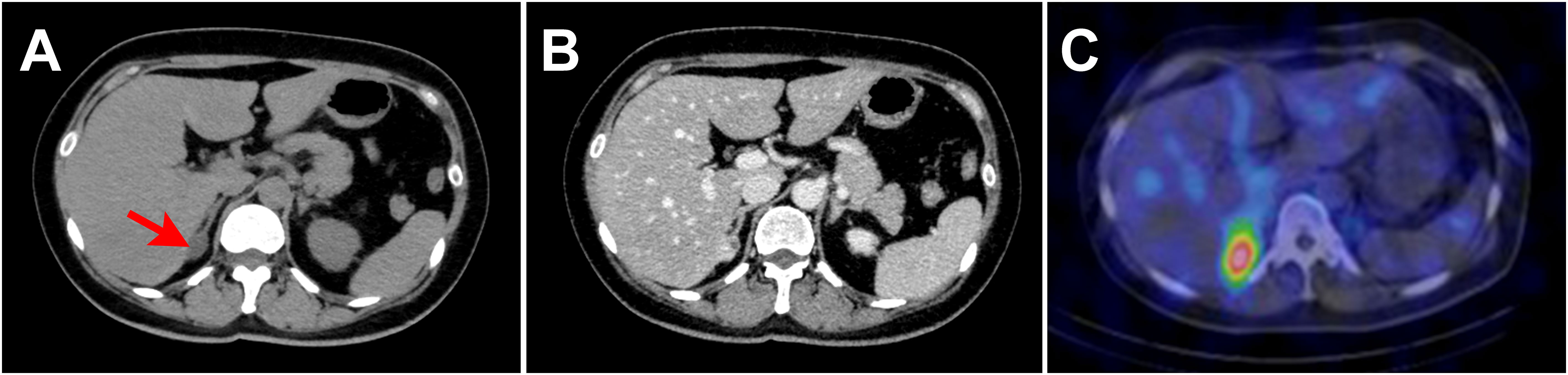
Figure 1 Imaging findings. (A, B). Computed tomography (CT) revealed a 2 cm right adrenal mass (red arrow in (A)). The mean Hounsfield unit of the adrenal tumor on unenhanced CT was 14.0. (A). Unenhanced CT. (B). Contrast enhanced CT. (C). NP-59 scintigraphy with dexamethasone suppression showed increased tracer uptake in the right adrenal lesion.
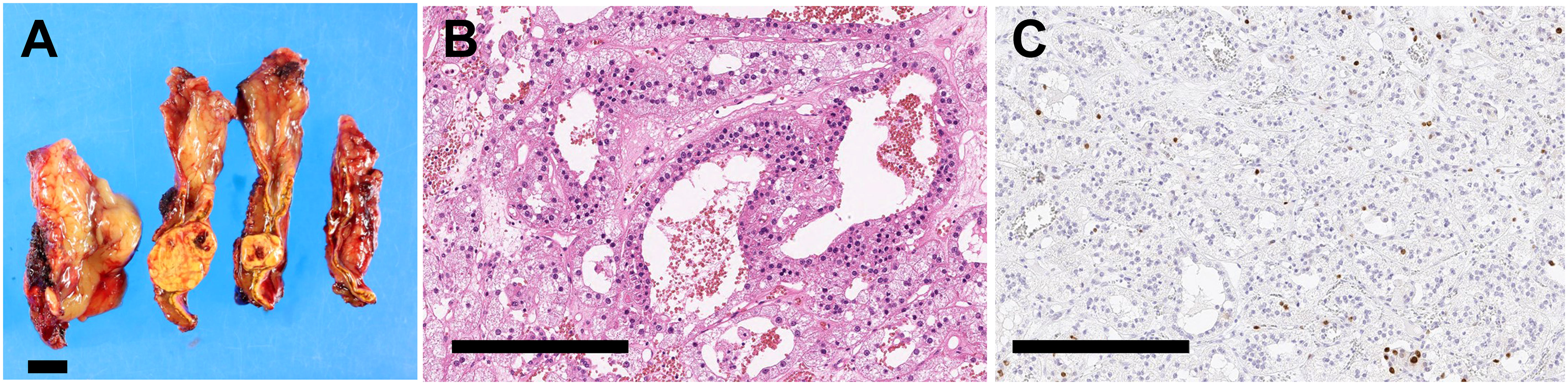
Figure 2 Histopathology of resected adrenal tumor. (A). Cut surfaces of resected adrenal tissue showing a yellow nodule with a diameter of 2.0 cm. Scale bar, 1 cm. (B, C). High magnification photomicrographs of adrenal tumor. Scale bars, 300 µm. (B). Hematoxylin and eosin staining. (C) Ki-67 staining.
Histopathologic and genetic characteristics of the resected tumor
IHC revealed diffuse immunoreactivity of CYP11B2 in tumor cells suggestive of neoplastic production of aldosterone (Figures 3A, B). VSNL1, a marker for the normal zona glomerulosa (ZG) (29), was also abundant in the tumor (Figures 3C, D). Consistent with normal suppression of cortisol after 1 mg dexamethasone suppression test, immunoreactivity of CYP17A1 and CYP11B1, both required for cortisol biosynthesis, was markedly low (Figures 3E–H). The adjacent adrenal tissue demonstrated paradoxical hyperplasia of the ZG, a hyperplastic ZG with negative CYP11B2 immunoreactivity, and aldosterone-producing micronodules (30). There were no atrophic changes in the zona fasciculata (ZF) or zona reticularis (ZR) of the adjacent adrenal tissue (Supplementary Figure 1A). In the ZR, normal dehydroepiandrosterone sulfotransferase (DHEA-ST) immunoreactivity was observed (Supplementary Figure 1B).
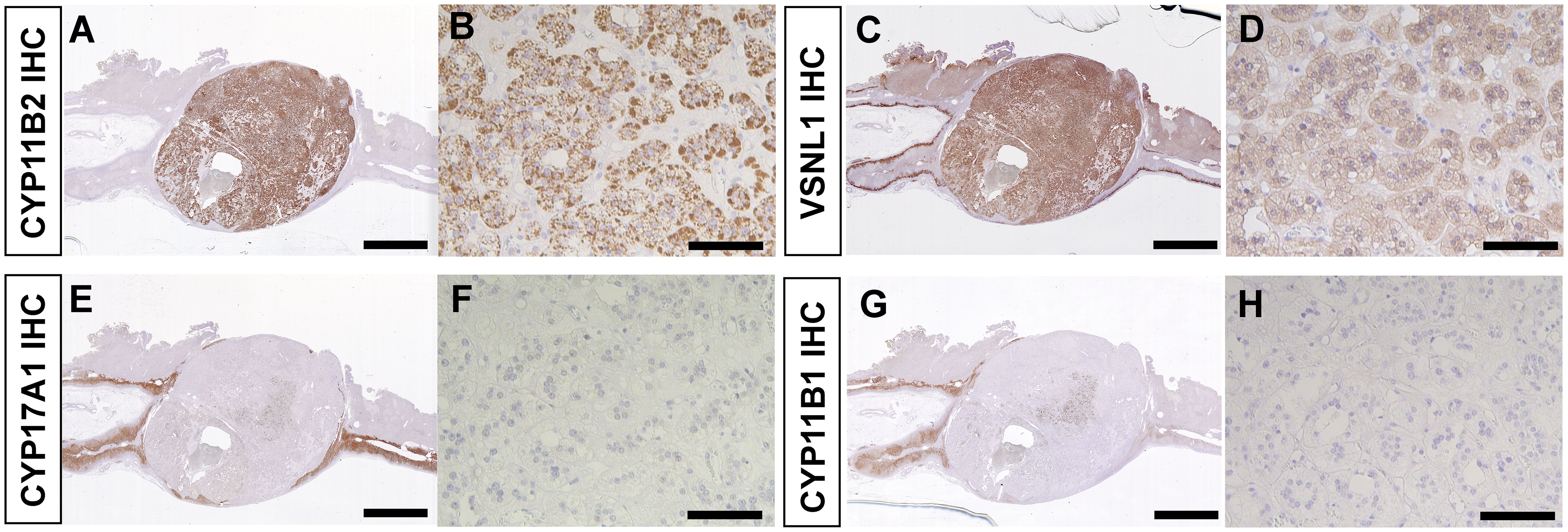
Figure 3 Immunohistochemistry of resected adrenal tumor. (A, B). CYP11B2 IHC. (C, D). VSNL1 IHC. (E, F). CYP17A1 IHC. (G, H). CYP11B1 IHC. (A, C, E, G). Scanned images of stained slides. Scale bars, 5 mm. (B, D, F, H). High magnification photomicrographs of adrenal tumor. Scale bars, 100 µm.
Targeted NGS identified double somatic CTNNB1 (p.D32Y) and GNA11 (p.Q209H) mutations with similar variant allele frequencies (Table 4). Using our method, these mutations were not detected in adjacent adrenal gDNA, suggesting their somatic origin. qPCR revealed high tumor expression of CYP11B2 mRNA (599-fold over adjacent normal adrenal), confirming accurate sample collection. In agreement with previous studies (20, 31), LHCGR and GNRHR mRNA levels were also elevated within the tumor compared with those in adjacent normal adrenal (148-fold and 56-fold, respectively).
We further tested β-catenin protein localization using IF staining to assess Wnt/β-catenin activation status (Figures 4A–D). In IF staining, KCNJ5 was used as a plasma membrane marker. A subset of tumor cells revealed nuclear and/or cytoplasmic immunoreactivity of β-catenin, suggesting activated status, which is seen in the ZG of normal adrenal glands (32).
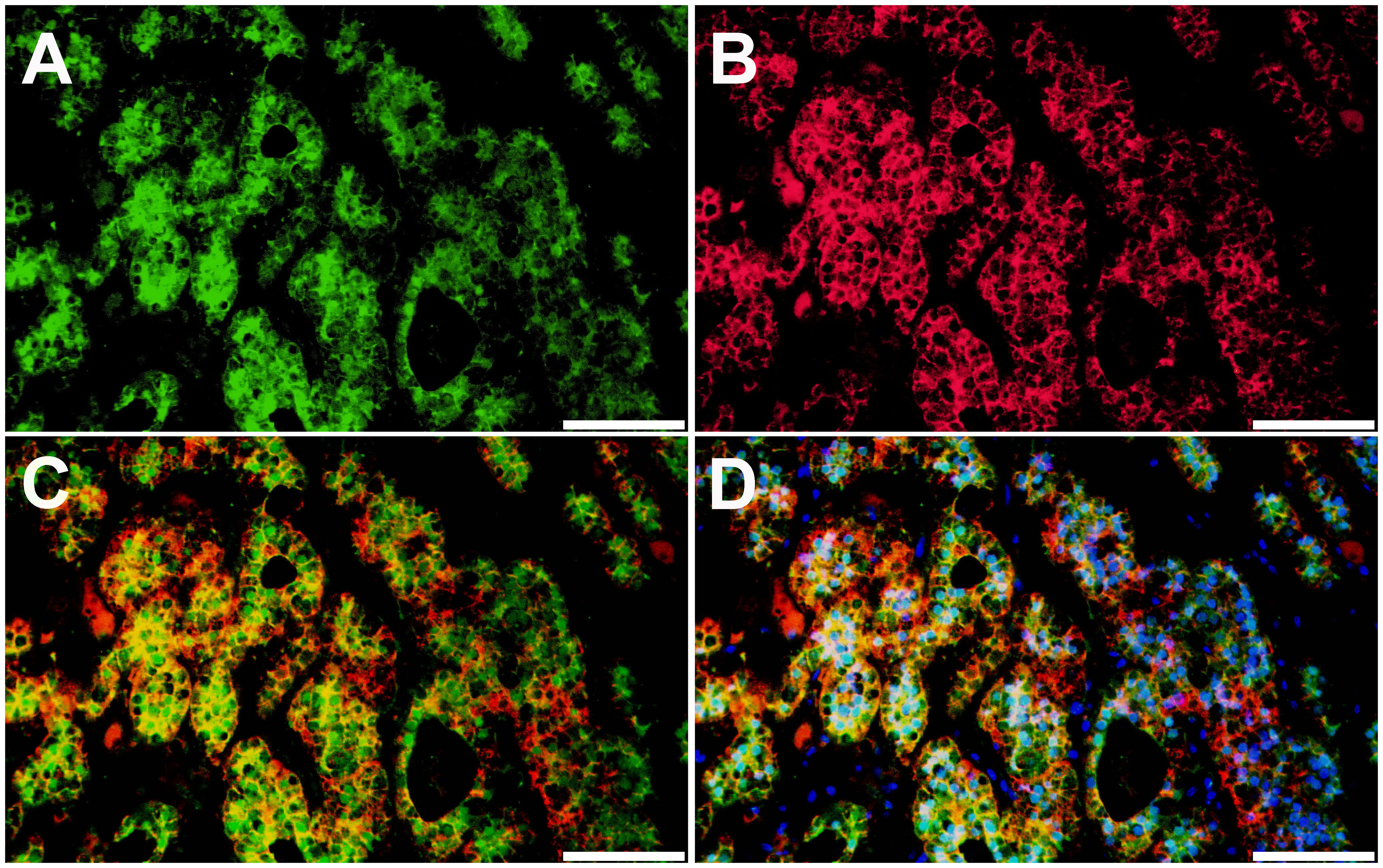
Figure 4 Localization of β-catenin protein in aldosterone-producing adenoma harboring somatic CTNNB1 and GNA11 mutations. β-catenin protein expression patterns in aldosterone-producing adenoma were assessed by immunofluorescence (IF) staining. (A). IF for β-catenin (β-catenin, green). (B). IF for KCNJ5 (KCNJ5, red). (C). IF for β-catenin and KCNJ5. (D). IF for β-catenin and KCNJ5 with DAPI (DAPI, blue). Scale bars, 100 µm.
Discussion
The Wnt/β-catenin signaling pathway plays an important role in adrenocortical development, homeostasis, and regeneration (33). In the non-pathologic human adrenal cortex, activated β-catenin (nuclear and/or cytoplasmic expression) is restricted to the ZG, where physiologic aldosterone biosynthesis occurs. In contrast, non-activated β-catenin (cell membrane expression) is predominant in the ZF (32). Aberrant Wnt/β-catenin signaling was reported to lead to various adrenal disorders and dysregulated steroidogenesis (33). Although the prevalence of somatic CTNNB1 mutation is relatively low in APA, activated β-catenin, i.e., nuclear and/or cytoplasmic localization of β-catenin, was reported in the majority of APA (34). A recent study investigating intra-tumor heterogeneity in APA demonstrated that β-catenin was activated mainly in CYP11B2-expressing regions of the tumor (16). The adrenal tumor from the present case showed diffuse CYP11B2 immunoreactivity. Like ZG cells, a subset of tumor cells demonstrated rosette-like structure and activated β-catenin (Figure 4). The intense tumor expression of VSNL1, one of the ZG markers, also supports a ZG identity of the tumor (Figures 3C, D).
Zhou et al. (20) recently demonstrated the coexistence of gain-of-function mutations in GNA11 or its close homolog, GNAQ, in 16 of 27 CTNNB1-mutated APAs (59%). The GNA11 and GNAQ genes encode G-protein subunit alpha 11 (G11) and G-protein subunit alpha q (Gq), respectively. Gq/11 act as important modulators of angiotensin II receptor activation, which is one of the main physiologic regulators of aldosterone production in ZG cells (35). The mutations in GNA11/Q in APA have always been detected in the highly conserved p.Q209 residue that is crucial for GTPase activation. These mutations inhibit GTPase activity, resulting in constitutive activation of downstream signaling and enhanced aldosterone production (20). High tumor expression of LHCGR and GNRHR in APAs with CTNNB1 (and GNA11/Q) mutations has been a rationale for the link between the disease onset and pregnancy, menopause, or puberty (20, 31). In Zhou’s study above, double mutations of CTNNB1 and GNA11/Q were more often seen in women than men (15 vs. 1) and the disease onset of 12 out of 16 cases (75%) was associated with pregnancy, menopause, or puberty (20). Our present case also showed elevated expression of LHCGR and GNRHR mRNA in the tumor compared with that in adjacent adrenal. However, the pathophysiologic role of high tumor expression of LHCGR and GNRHR mRNA in our case is unclear since her disease onset was not directly associated with pregnancy or menopause. Of particular note, one of the 16 cases in Zhou’s study had the same combination of mutations as our case (CTNNB1 p.D32Y and GNA11 p.Q209H) and the patient had no history of hypertension during her past 10 pregnancies (20).
Previous studies also reported aberrant expression of G protein-coupled receptors, including LHCGR, GNRHR, 5-hydroxytryptamine (serotonin) receptor 4 (HTR4), and melanocortin 2 receptor (MC2R) in APAs (36, 37). In addition, some of patients with PA were reported to show enhanced aldosterone production in response to luteinizing hormone (LH), human chorionic gonadotropin (hCG), or gonadotropin-releasing hormone (GnRH) (38–42). Gagnon et al. (41) investigated genetic characteristics of GnRH/LH-responsive PA, including APA, bilateral macronodular adrenal hyperplasia, and other rarer forms. In their cohort, 17 patients with APA underwent in vivo GnRH and/or LH tests; 6, 10, and 1 had both, only GnRH, and only LH tests, respectively. Among 16 APAs tested for GnRH, 6 and 3 APAs showed positive and partial response, respectively. Positive response to LH was observed in 5 out of 7 APAs tested. Sequencing analysis of 15 APAs that had in vivo GnRH and/or LH tests revealed 3 KCNJ5 (1 tested for GnRH and LH, no response; 1 tested for GnRH, partial response; 1 tested for LH, positive response), 1 ATP1A1 (tested for GnRH, no response), and 1 CACNA1D mutations (tested for GnRH, no response). Of particular interest, there were no CTNNB1-mutated APAs in their cohort (41). Another study by Kishimoto et al. (40) demonstrated that GNRHR and LHCGR mRNA levels were higher and the response to GnRH was greater in APAs with no known mutations (mutation hotspots of KCNJ5, ATP1A1, ATP2B3, CACNA1D, and CTNNB1 genes were screened) (n=9) compared with those with KCNJ5 hotspot mutations (n=13). Genetic causes of GnRH/LH-responsive APAs appear to be heterogeneous and largely unknown. Further dedicated studies are needed.
Because of its rare incidence, clinical characteristics of the patients with APA harboring double CTNNB1 and GNA11/Q mutations are not well characterized. Our case had typical clinical characteristics of PA with no excess cortisol co-secretion. Although the histologic findings were compatible with adrenocortical adenoma according to the criteria of Weiss (27), the tumor cells had unusually high Ki-67 labeling index for an adenoma (43). The present case was therefore closely followed up after surgery. Post-operative clinical course was indeed excellent with achievement of clinical and biochemical cure and no tumor recurrence was observed. Our present case also indicates that the occurrence of APA with double CTNNB1 and GNA11 somatic mutations is not always associated with pregnancy or menopause. In conclusion, we present a case of APA with double somatic mutations in CTNNB1 and GNA11. Detailed clinical and histologic examination will provide useful information for better characterization of patients with PA caused by these rare mutations.
Data availability statement
The datasets presented in this article are not readily available because of ethical and privacy restrictions. Requests to access the datasets should be directed to the corresponding author.
Ethics statement
The studies involving humans were approved by National Hospital Organization Kyoto Medical Center and the University of Michigan. The studies were conducted in accordance with the local legislation and institutional requirements. The participants provided their written informed consent to participate in this study. Written informed consent was obtained from the individual(s) for the publication of any potentially identifiable images or data included in this article.
Author contributions
All authors made individual contributions to authorship. KN and WR conceived the idea of molecular analysis. KN drafted the manuscript. AB and AU performed molecular analysis. KN, YH, TM, and HO were involved in the care of the patient. KM, YY, and HS were involved in histologic diagnosis. AY, NS-A, and TT provided input for the case and manuscript. All authors contributed to the article and approved the submitted version.
Funding
The author(s) declare that financial support was received for the research, authorship, and/or publication of this article. This research was supported by NIH grants R01DK106618 and R01DK043140 to WR. This work was also supported by grants from Japan Heart Foundation and Takeda Science Foundation to KN.
Acknowledgments
We would like to thank Dr. Celso E. Gomez-Sanchez at the University of Mississippi for providing the monoclonal antibodies against human CYP11B1 and KCNJ5. We also thank medical staff at National Hospital Organization Kyoto Medical Center.
Conflict of interest
KN received a research grant from AstraZeneca, which is unrelated to the current work.
The remaining authors declare that the research was conducted in the absence of any commercial or financial relationships that could be construed as a potential conflict of interest.
Publisher’s note
All claims expressed in this article are solely those of the authors and do not necessarily represent those of their affiliated organizations, or those of the publisher, the editors and the reviewers. Any product that may be evaluated in this article, or claim that may be made by its manufacturer, is not guaranteed or endorsed by the publisher.
Supplementary material
The Supplementary Material for this article can be found online at: https://www.frontiersin.org/articles/10.3389/fendo.2024.1286297/full#supplementary-material
References
1. Choi M, Scholl UI, Yue P, Bjorklund P, Zhao B, Nelson-Williams C, et al. K+ channel mutations in adrenal aldosterone-producing adenomas and hereditary hypertension. Science. (2011) 331:768–72. doi: 10.1126/science.1198785
2. Beuschlein F, Boulkroun S, Osswald A, Wieland T, Nielsen HN, Lichtenauer UD, et al. Somatic mutations in ATP1A1 and ATP2B3 lead to aldosterone-producing adenomas and secondary hypertension. Nat Genet. (2013) 45:440–4, 444e1-2. doi: 10.1038/ng.2550
3. Scholl UI, Goh G, Stolting G, de Oliveira RC, Choi M, Overton JD, et al. Somatic and germline CACNA1D calcium channel mutations in aldosterone-producing adenomas and primary aldosteronism. Nat Genet. (2013) 45:1050–4. doi: 10.1038/ng.2695
4. Azizan EA, Poulsen H, Tuluc P, Zhou J, Clausen MV, Lieb A, et al. Somatic mutations in ATP1A1 and CACNA1D underlie a common subtype of adrenal hypertension. Nat Genet. (2013) 45:1055–60. doi: 10.1038/ng.2716
5. Scholl UI, Stolting G, Nelson-Williams C, Vichot AA, Choi M, Loring E, et al. Recurrent gain of function mutation in calcium channel CACNA1H causes early-onset hypertension with primary aldosteronism. Elife. (2015) 4:e06315. doi: 10.7554/eLife.06315
6. Nanba K, Blinder AR, Rege J, Hattangady NG, Else T, Liu CJ, et al. Somatic CACNA1H mutation as a cause of aldosterone-producing adenoma. Hypertension. (2020) 75:645–9. doi: 10.1161/HYPERTENSIONAHA.119.14349
7. Scholl UI, Stolting G, Schewe J, Thiel A, Tan H, Nelson-Williams C, et al. CLCN2 chloride channel mutations in familial hyperaldosteronism type II. Nat Genet. (2018) 50:349–54. doi: 10.1038/s41588-018-0048-5
8. Fernandes-Rosa FL, Daniil G, Orozco IJ, Goppner C, El Zein R, Jain V, et al. A gain-of-function mutation in the CLCN2 chloride channel gene causes primary aldosteronism. Nat Genet. (2018) 50:355–61. doi: 10.1038/s41588-018-0053-8
9. Dutta RK, Arnesen T, Heie A, Walz M, Alesina P, Soderkvist P, et al. A somatic mutation in CLCN2 identified in a sporadic aldosterone-producing adenoma. Eur J Endocrinol. (2019) 181:K37–41. doi: 10.1530/EJE-19-0377
10. Scholl UI. Genetics of primary aldosteronism. Hypertension. (2022) 79:887–897. doi: 10.1161/HYPERTENSIONAHA.121.16498
11. Wu X, Azizan EAB, Goodchild E, Garg S, Hagiyama M, Cabrera CP, et al. Somatic mutations of CADM1 in aldosterone-producing adenomas and gap junction-dependent regulation of aldosterone production. Nat Genet. (2023) 55:1009–21. doi: 10.1038/s41588-023-01403-0
12. Rege J, Bandulik S, Nanba K, Kosmann C, Blinder AR, Plain A, et al. Somatic SLC30A1 mutations altering zinc transporter ZnT1 cause aldosterone-producing adenomas and primary aldosteronism. Nat Genet. (2023) 55:1623–31. doi: 10.1038/s41588-023-01498-5
13. Nanba K, Omata K, Else T, Beck PCC, Nanba AT, Turcu AF, et al. Targeted molecular characterization of aldosterone-producing adenomas in white Americans. J Clin Endocrinol Metab. (2018) 103:3869–76. doi: 10.1210/jc.2018-01004
14. Nanba K, Omata K, Gomez-Sanchez CE, Stratakis CA, Demidowich AP, Suzuki M, et al. Genetic characteristics of aldosterone-producing adenomas in blacks. Hypertension. (2019) 73:885–92. doi: 10.1161/HYPERTENSIONAHA.118.12070
15. Nanba K, Yamazaki Y, Bick N, Onodera K, Tezuka Y, Omata K, et al. Prevalence of somatic mutations in aldosterone-producing adenomas in Japanese patients. J Clin Endocrinol Metab. (2020) 105:e4066–e4073. doi: 10.1210/clinem/dgaa595
16. De Sousa K, Boulkroun S, Baron S, Nanba K, Wack M, Rainey WE, et al. Genetic, cellular, and molecular heterogeneity in adrenals with aldosterone-producing adenoma. Hypertension. (2020) 75:1034–44. doi: 10.1161/HYPERTENSIONAHA.119.14177
17. Akerstrom T, Maharjan R, Sven Willenberg H, Cupisti K, Ip J, Moser A, et al. Activating mutations in CTNNB1 in aldosterone producing adenomas. Sci Rep. (2016) 6:19546. doi: 10.1038/srep19546
18. Scholl UI, Healy JM, Thiel A, Fonseca AL, Brown TC, Kunstman JW, et al. Novel somatic mutations in primary hyperaldosteronism are related to the clinical, radiological and pathological phenotype. Clin Endocrinol (Oxf). (2015) 83:779–89. doi: 10.1111/cen.12873
19. Wu VC, Wang SM, Chueh SJ, Yang SY, Huang KH, Lin YH, et al. The prevalence of CTNNB1 mutations in primary aldosteronism and consequences for clinical outcomes. Sci Rep. (2017) 7:39121. doi: 10.1038/srep39121
20. Zhou J, Azizan EAB, Cabrera CP, Fernandes-Rosa FL, Boulkroun S, Argentesi G, et al. Somatic mutations of GNA11 and GNAQ in CTNNB1-mutant aldosterone-producing adenomas presenting in puberty, pregnancy or menopause. Nat Genet. (2021) 53:1360–72. doi: 10.1038/s41588-021-00906-y
21. Kitamura T, Blinder AR, Nanba K, Tsuiki M, Mishina M, Okuno H, et al. ACTH-independent production of 11-oxygenated androgens and glucocorticoids in an adrenocortical adenoma. Eur J Endocrinol. (2022) 187:K39–45. doi: 10.1530/EJE-22-0508
22. Yang Y, Gomez-Sanchez CE, Jaquin D, Aristizabal Prada ET, Meyer LS, Knosel T, et al. Primary aldosteronism: KCNJ5 mutations and adrenocortical cell growth. Hypertension. (2019) 74:809–16. doi: 10.1161/HYPERTENSIONAHA.119.13476
23. Nanba K, Chen AX, Omata K, Vinco M, Giordano TJ, Else T, et al. Molecular heterogeneity in aldosterone-producing adenomas. J Clin Endocrinol Metab. (2016) 101:999–1007. doi: 10.1210/jc.2015-3239
24. Pezzi V, Mathis JM, Rainey WE, Carr BR. Profiling transcript levels for steroidogenic enzymes in fetal tissues. J Steroid Biochem Mol Biol. (2003) 87:181–9. doi: 10.1016/j.jsbmb.2003.07.006
25. Takeuchi J, Sakagami Y, Perez RC. The mother and child health handbook in Japan as a health promotion tool: An overview of its history, contents, use, benefits, and global influence. Glob Pediatr Health. (2016) 3:2333794X16649884. doi: 10.1177/2333794X16649884
26. Naruse M, Katabami T, Shibata H, Sone M, Takahashi K, Tanabe A, et al. Japan Endocrine Society clinical practice guideline for the diagnosis and management of primary aldosteronism 2021. Endocr J. (2022) 69:327–59. doi: 10.1507/endocrj.EJ21-0508
27. Weiss LM. Comparative histologic study of 43 metastasizing and nonmetastasizing adrenocortical tumors. Am J Surg Pathol. (1984) 8:163–9. doi: 10.1097/00000478-198403000-00001
28. Williams TA, Lenders JWM, Mulatero P, Burrello J, Rottenkolber M, Adolf C, et al. Outcomes after adrenalectomy for unilateral primary aldosteronism: an international consensus on outcome measures and analysis of remission rates in an international cohort. Lancet Diabetes Endocrinol. (2017) 5:689–99. doi: 10.1016/S2213-8587(17)30135-3
29. Seccia TM, Caroccia B, Gomez-Sanchez EP, Vanderriele PE, Gomez-Sanchez CE, Rossi GP. Review of markers of zona glomerulosa and aldosterone-producing adenoma cells. Hypertension. (2017) 70:867–74. doi: 10.1161/HYPERTENSIONAHA.117.09991
30. Williams TA, Gomez-Sanchez CE, Rainey WE, Giordano TJ, Lam AK, Marker A, et al. International histopathology consensus for unilateral primary aldosteronism. J Clin Endocrinol Metab. (2021) 106:42–54. doi: 10.1210/clinem/dgaa484
31. Teo AE, Garg S, Shaikh LH, Zhou J, Karet Frankl FE, Gurnell M, et al. Pregnancy, primary aldosteronism, and adrenal CTNNB1 mutations. N Engl J Med. (2015) 373:1429–36. doi: 10.1056/NEJMoa1504869
32. Boulkroun S, Samson-Couterie B, Golib-Dzib JF, Amar L, Plouin PF, Sibony M, et al. Aldosterone-producing adenoma formation in the adrenal cortex involves expression of stem/progenitor cell markers. Endocrinology. (2011) 152:4753–63. doi: 10.1210/en.2011-1205
33. Little DW 3rd, Dumontet T. LaPensee CR and Hammer GD. beta-catenin in adrenal zonation and disease. Mol Cell Endocrinol. (2021) 522:111120. doi: 10.1016/j.mce.2020.111120
34. Berthon A, Drelon C, Ragazzon B, Boulkroun S, Tissier F, Amar L, et al. WNT/beta-catenin signalling is activated in aldosterone-producing adenomas and controls aldosterone production. Hum Mol Genet. (2014) 23:889–905. doi: 10.1093/hmg/ddt484
35. Nogueira EF, Bollag WB, Rainey WE. Angiotensin II regulation of adrenocortical gene transcription. Mol Cell Endocrinol. (2009) 302:230–6. doi: 10.1016/j.mce.2008.08.024
36. Saner-Amigh K, Mayhew BA, Mantero F, Schiavi F, White PC, Rao CV, et al. Elevated expression of luteinizing hormone receptor in aldosterone-producing adenomas. J Clin Endocrinol Metab. (2006) 91:1136–42. doi: 10.1210/jc.2005-1298
37. Ye P, Mariniello B, Mantero F, Shibata H, Rainey WE. G-protein-coupled receptors in aldosterone-producing adenomas: a potential cause of hyperaldosteronism. J Endocrinol. (2007) 195:39–48. doi: 10.1677/JOE-07-0037
38. Zwermann O, Suttmann Y, Bidlingmaier M, Beuschlein F and Reincke M. Screening for membrane hormone receptor expression in primary aldosteronism. Eur J Endocrinol. (2009) 160:443–51. doi: 10.1530/EJE-08-0711
39. Albiger NM, Sartorato P, Mariniello B, Iacobone M, Finco I, Fassina A, et al. A case of primary aldosteronism in pregnancy: do LH and GNRH receptors have a potential role in regulating aldosterone secretion? Eur J Endocrinol. (2011) 164:405–12. doi: 10.1530/EJE-10-0879
40. Kishimoto R, Oki K, Yoneda M, Gomez-Sanchez CE, Ohno H, Kobuke K, et al. Gonadotropin-releasing hormone stimulate aldosterone production in a subset of aldosterone-producing adenoma. Med (Baltimore). (2016) 95:e3659. doi: 10.1097/MD.0000000000003659
41. Gagnon N, Caceres-Gorriti KY, Corbeil G, El Ghoyareb N, Ludwig N, Latour M, et al. Genetic characterization of gnRH/LH-responsive primary aldosteronism. J Clin Endocrinol Metab. (2018) 103:2926–35. doi: 10.1210/jc.2018-00087
42. Castinetti F, Guerin C, Louiset E, Lacroix A. HCG-responsive aldosteronoma with transient secretion during pregnancy confirmed through HCG-stimulated adrenal venous sampling. Front Endocrinol (Lausanne). (2023) 14:1153374. doi: 10.3389/fendo.2023.1153374
Keywords: primary aldosteronism, aldosterone-producing adenoma, CYP11B2, somatic mutation, CTNNB1, GNA11
Citation: Nanba K, Blinder AR, Udager AM, Hirokawa Y, Miura T, Okuno H, Moriyoshi K, Yamazaki Y, Sasano H, Yasoda A, Satoh-Asahara N, Rainey WE and Tagami T (2024) Double somatic mutations in CTNNB1 and GNA11 in an aldosterone-producing adenoma. Front. Endocrinol. 15:1286297. doi: 10.3389/fendo.2024.1286297
Received: 31 August 2023; Accepted: 21 February 2024;
Published: 05 March 2024.
Edited by:
Norlela Sukor, Universiti Kebangsaan Malaysia Medical Center (UKMMC), MalaysiaReviewed by:
Andrea Gutierrez Maria, Eunice Kennedy Shriver National Institute of Child Health and Human Development (NIH), United StatesAlexander Akhmedov, University of Zurich, Switzerland
Copyright © 2024 Nanba, Blinder, Udager, Hirokawa, Miura, Okuno, Moriyoshi, Yamazaki, Sasano, Yasoda, Satoh-Asahara, Rainey and Tagami. This is an open-access article distributed under the terms of the Creative Commons Attribution License (CC BY). The use, distribution or reproduction in other forums is permitted, provided the original author(s) and the copyright owner(s) are credited and that the original publication in this journal is cited, in accordance with accepted academic practice. No use, distribution or reproduction is permitted which does not comply with these terms.
*Correspondence: Kazutaka Nanba, knamba@umich.edu
†ORCID: Kazutaka Nanba, orcid.org/0000-0002-6475-5141