- 1The First Clinical College, Shandong University of Traditional Chinese Medicine, Jinan, Shandong, China
- 2Gynecology Department, Shandong University of Traditional Chinese Medicine Affiliated Hospital, Jinan, Shandong, China
- 3Zhang Zhongjing College of Chinese Medicine, Nanyang Institute of Technology, Nanyang, Henan, China
Reproductive system diseases pose prominent threats to human physical and mental well-being. Besides being influenced by genetic material regulation and changes in lifestyle, the occurrence of these diseases is closely connected to exposure to harmful substances in the environment. Endocrine disrupting chemicals (EDCs), characterized by hormone-like effects, have a wide range of influences on the reproductive system. EDCs are ubiquitous in the natural environment and are present in a wide range of industrial and everyday products. Currently, thousands of chemicals have been reported to exhibit endocrine effects, and this number is likely to increase as the testing for potential EDCs has not been consistently required, and obtaining data has been limited, partly due to the long latency of many diseases. The ability to avoid exposure to EDCs, especially those of artificially synthesized origin, is increasingly challenging. While EDCs can be divided into persistent and non-persistent depending on their degree of degradation, due to the recent uptick in research studies in this area, we have chosen to focus on the research pertaining to the detrimental effects on reproductive health of exposure to several EDCs that are widely encountered in daily life over the past six years, specifically bisphenol A (BPA), phthalates (PAEs), polychlorinated biphenyls (PCBs), parabens, pesticides, heavy metals, and so on. By focusing on the impact of EDCs on the hypothalamic-pituitary-gonadal (HPG) axis, which leads to the occurrence and development of reproductive system diseases, this review aims to provide new insights into the molecular mechanisms of EDCs’ damage to human health and to encourage further in-depth research to clarify the potentially harmful effects of EDC exposure through various other mechanisms. Ultimately, it offers a scientific basis to enhance EDCs risk management, an endeavor of significant scientific and societal importance for safeguarding reproductive health.
Introduction
Estrogen, an essential class of sex hormones, plays a pivotal role in maintaining normal physiological functions within the human body. It has been demonstrated to have effects on many physiological events, including cardiovascular function, ovarian endocrine and reproductive function, and even male reproductive function. For example, premenopausal women exhibit a lower incidence and severity of hypertension compared to men of the same age. Our previous study established a close relationship between the shortage of estrogen and an increased risk of hypertension, which is closely related to estrogen receptors (1–3). Unlike the shortage of physiological estrogens, there are abundant xenoestrogens from industrial or natural sources in the ambient environment, which have been proved to be detrimental to a variety of physiological processes through estrogen receptors (ERs) and the subsequent cellular and endocrine signaling pathways by mimicking the endogenous ligand. Understanding this complexity is essential for comprehending the effects of endocrine disruptors on reproductive health.
Originally named xenoestrogens, endocrine disrupting chemicals (EDCs) have been officially defined by the USA Environmental Protection Agency (EPA) as external agents that disorder the natural processes involving hormone synthesis, secretion, transport, binding, action, or elimination (4). These chemicals may have estrogenic, anti-estrogenic, androgenic or anti-androgenic effects, which are crucial for maintaining homeostasis, reproductive functions, development, and behavior. The increasing prevalence of human endocrine-related diseases, particularly disorders of the female and male reproductive systems, and even certain cancers (e.g., breast cancer, endometrial cancer, ovarian cancer, cervical cancer, prostate cancer, and testicular cancer), has aroused people’s attention to EDCs (5–7). EDCs represent a global issue of great significance for humans and the environment8). With the advancement of science and technology and the development of society, humans are consistently exposed to EDCs through ingestion, inhalation, and skin contact from the embryonic stage, as they are widely present in cosmetics, detergents, pesticides, fungicides, pharmaceuticals, medical equipment, plastics, plasticizers, food products, food and beverage packaging, electronic components, and industrial solvents (Figure 1).
As is generally known, EDCs have the capacity to disrupt the normal functioning of the gonads, impacting the regulation of sex hormone biosynthesis controlled by the hypothalamic-pituitary-gonadal (HPG) axis (9, 10). The HPG axis serves as a principal modulator of reproductive function, driving processes such as the growth, development, maturation, and ovulation of follicles, as well as spermatogenesis and steroidogenesis (11, 12). The regulation of the physiological activities of the human reproductive system involves the hypothalamus, pituitary gland, and gonads, which regulate the secretion of gonadotropin-releasing hormone (GnRH), gonadotropins (Gn), and gonadal hormones (e.g., estrogen, progestogen, and androgen). The interactions among these hormones are critical for the proper control of the HPG axis. Most EDCs have diverse structures, including phenolics and non-phenolics of natural or industrial origin, and exhibit hormone-like or anti-hormone-like activities, making the female and male reproductive systems vulnerable to disruption by EDCs. Specifically, EDCs mimic sex hormones and interfere with the release of GnRH and Gn through various pathways, disrupting the HPG axis homeostasis and impairing folliculogenesis, ovulation, conception, spermatogenesis, sperm quality, and reproductive ability. Accordingly, EDCs play an adverse role in the reproductive system, at least in part, by affecting the HPG axis, causing abnormal sensitivity to steroid feedback mechanisms and deleterious effects on female and male reproductive organs and tissues. Further research showed that EDCs not only directly responsible for activating or inhibiting hormonal action but also indirectly modulating their action by autocrine or paracrine signaling, and even signal crosstalk.
Overall, the role of EDCs appears to be quite complicated and includes involvement in the physiology of reproductive organs and tissues (e.g., ovary, breast, endometrium, testis, and prostate), protein synthesis, lipid and bone metabolism, the occurrence and development of ovarian dysfunction, cancer, and other reproductive system-related diseases. Accumulating evidence from animal models and human epidemiological studies highlights the relationship between EDCs and an array of reproductive disorders. These disorders encompass uterine fibroids (UFs), endometriosis (EMs), polycystic ovary syndrome (PCOS), diminished ovarian reserve (DOR), premature ovarian insufficiency (POI), infertility, breast cancer (BC), endometrial cancer (EC), ovarian cancer (OC), cervical cancer (CC), cryptorchidism, hypospadias, prostate cancer (PC) and testicular cancer (TC). EDCs like bisphenol A (BPA), phthalates (PAEs), polychlorinated biphenyls (PCBs), parabens, pesticides, heavy metals, among others, have been implicated in these diseases, highlighting potential reproductive health risks of those EDCs during critical susceptibility periods.
EDCs are now recognized as serious threats to public health and one of the leading environmental risks globally. Given the grand challenges faced in the realm of reproductive health, it is imperative to augment research efforts in this sphere and urgent actions need to be taken to reduce extensive exposure to disruptive EDCs. Intending to inform future research and policy, this article aims to update the evidence supporting previously identified or increasingly likely associations of commonly encountered EDCs with female and male reproductive health over the past six years, which is indexed in PubMed, Scopus, and Web of Science regardless of conference abstracts. The exclusion criteria included all articles that were duplicates, unrelated, inaccessible, and not written in English. A large supplementary table summarizing all studies reviewed that reported significant or epidemiologically meaningful associations can be found in the appendix.
Research on EDCs in the female reproductive system
EDCs exert estrogenic effects, which is the starting point for studies on the occurrence and progression of endocrine-relevant diseases. Female ovaries serve as the primary gonads responsible for producing and releasing mature eggs, as well as secreting estrogen to regulate endometrial growth and menstrual periodic bleeding. By affecting estrogen receptor activity and interfering with estrogen-related signaling pathways, EDCs alter the estrogen level and feedback regulate the secretion of GnRH and Gn. The perturbation of the hypothalamic-pituitary-ovarian axis can impair the growth and development of eggs and periodic changes in the endometrium, leading to abnormal menstrual cycles, ovulation disorders, and premature ovarian aging, thereby reducing female reproductive ability and causing a series of female reproductive system disorders.
Chronic, long-term exposure to EDCs can cumulatively induce hormone-like effects, leading to endocrine dysfunction and adverse effects on the structure and function of female reproductive tissues and organs, potentially contributing to the development of numerous complex diseases, including UFs, EMs, PCOS, DOR, POI, infertility, EC, OC, CC, and BC. A comprehensive overview of common EDCs related to female reproductive system diseases is shown in Figure 2.
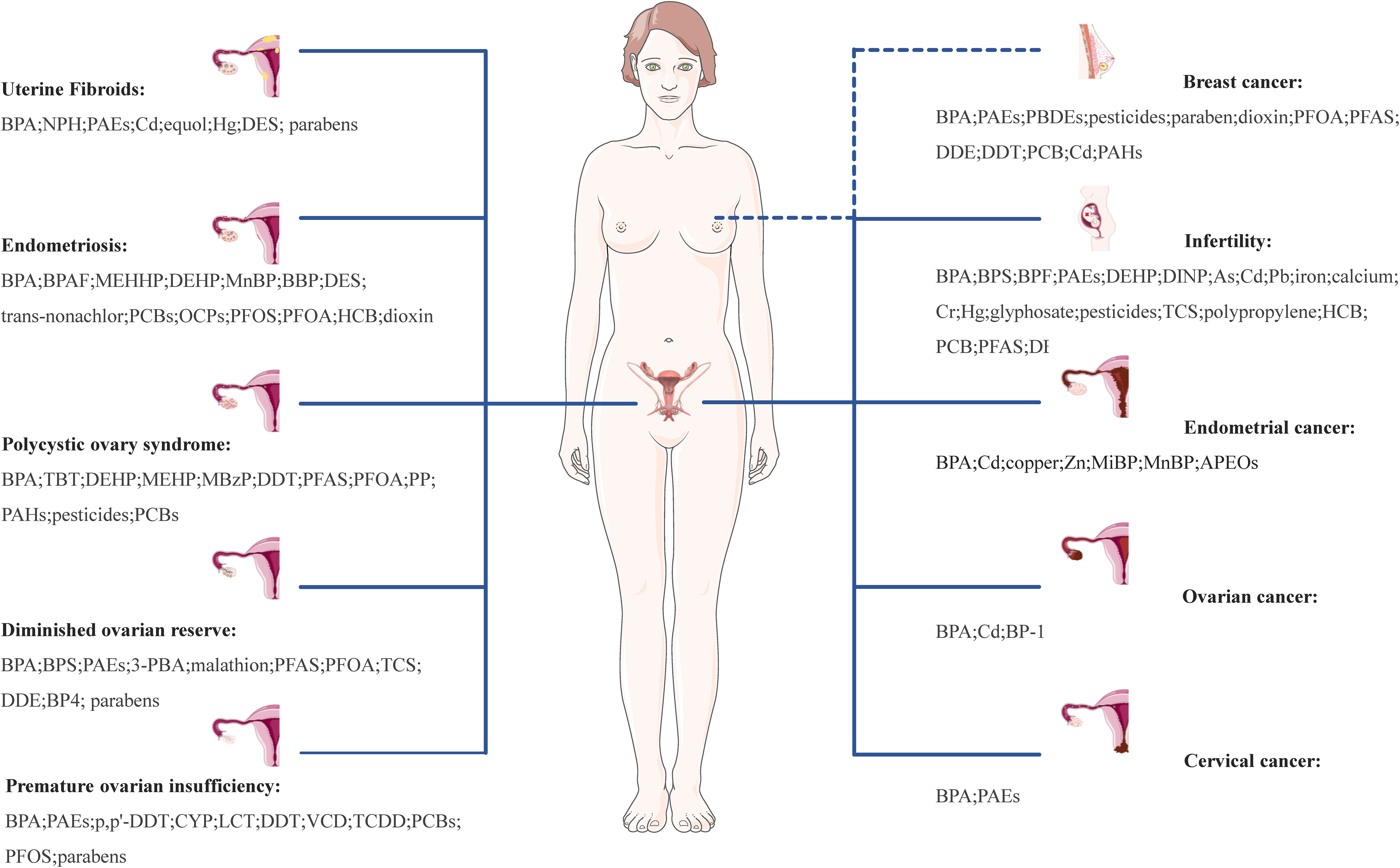
Figure 2 Effect of EDCs on female reproductive health. UFs, uterine fibroids; EMs, endometriosis; PCOS, polycystic ovary syndrome; DOR, diminished ovarian reserve; POI, premature ovarian insufficiency; EC, infertility, endometrial cancer; OC, ovarian cancer; CC, cervical cancer; PC, prostate cancer; TC, testicular cancer; BPA, bisphenol A; NPH, nonylphenol; PAEs, phthalates; Cd, cadmium; Hg, mercury; DES, diethylstilbestrol; BPAF, bisphenol AF; MEHHP, mono-(2-ethyl-5-hydroxyhexyl) phthalate; DEHP, di(2-ethylhexyl)phthalate; MnBP, mono-n-butyl phthalate; BBP, n-butylbenzyl phthalate; PCBs, polychlorinated biphenyls; OCPs, organochlorine pesticides; PFOS, perfluorooctane sulphonate; PFOA, perfluorooctanoic acid; HCB, hexachlorobenzene; TBT, tributyltin; MEHP, mono(2-ethylhexyl) phthalate; MBzP, monobenzyl phthalate; DDT, dichlorodiphenyltrichloroethane; PFAS, perfluorinated substances; PP, propylparaben; PAHs, polycyclic aromatic hydrocarbons; 3-PBA, 3-phenoxybenoic acid; TCS, triclosan; DDE, p,p’-dichlorodiphenyldichloroethylene; BP4, benzophenone-4; CYP, cypermethrin; LCT, lambda-cyhalothrin; VCD, 4-vinylcyclohexenediepoxide; TCDD, 2,3,7,8-tetrachlorodibenzo-p-dioxin; DINP, diisononyl phthalate; As, arsenic; Pb, lead; Cr, hexavalent chromium; Zn, zinc; MiBP, monoisobutyl phthalate; APEOs, alkylphenol ethoxylates; BP-1, benzophenone-1.
EDCs impact uterine leiomyoma cells’ proliferation ability leading to uterine fibroids (UFs)
Among women in their reproductive age, UFs are the prevailing benign tumors. Their growth is under the influence of sex steroids, leading to their appearance during the reproductive years and subsequent regression after menopause. The growth of fibroids is driven by higher levels of estrogen and progesterone in the body, making them a significant factor in the development of UFs. Conspicuously, exposure to various EDCs commonly found in plastics, cosmetics, and personal care products, including bisphenol A (BPA), phthalates (PAEs), cadmium (Cd), and diethylstilbestrol (DES), has been associated with an elevation in sex hormone levels and the development of UFs in women.
BPA
BPA was first synthesized in 1981 and has been utilized in the production of plastics since the 1950s (13, 14). In addition to its role as a constituent of plastics, BPA is commonly found in epoxy resin linings for metal-based food and beverage cans. Furthermore, it is utilized in various consumer products such as thermal paper, medical equipment, toys, electronics, and water pipes (15, 16). It is worth noting that BPA can leach into food, water, or medical supplies through physical manipulation or repetitive use. The United States Environmental Protection Agency (US-EPA) has established a safety level for BPA at 50 μg/kg/d (micrograms per kilogram per day), whereas the European Food Safety Authority (EFSA) lowered its temporary tolerable daily intake to 4 μg/kg/d (17). This demonstrated that BPA is well-known as one of the most infamous EDCs, even at very low concentrations, BPA can also be combined with ERs and activate ER-dependent transcription, resulting in the corresponding biological effect, and causing negative effects on human health.
BPA exerts a significant impact on the development of UFs through environmental exposure and lifestyle factors (18, 19). Experimental studies using human uterine leiomyoma (UL) cells have demonstrated that BPA, at low concentrations ranging from 10-6μM to 10μM, enhances cell proliferation by facilitating hormonally regulated progression, while higher concentrations (100 μM-200μM) inhibit growth (20). Further investigations, involving intervention with BPA in primary and subcultures of human UF cells, have provided compelling evidence suggesting that BPA induces dose and time-dependent promotion of leiomyoma cell growth. This effect is achieved through the activation of signaling pathways such as GPR30-EGFR and MAPK/ERK/c-fos (21). Additional investigations have shown that BPA and its analog, nonylphenol (NPH), can activate the TGF-β signaling pathway through estrogen receptor-α (ER-α) signaling, thereby modulating the proliferation of UL cells in vitro (22). The application of RNA-seq analysis has revealed that BPA and NPH, acting as external stimulants with phenolic estrogen properties, induce the upregulation of inflammatory factors in uterine leiomyomas (23). By combining ChIP-seq and RNA-seq methods, researchers have gained new insights into the pathogenesis of UFs, specifically how BPA and its derivative can facilitate fibrosis through the PI3K/AKT signaling pathway (24).
Recent research highlights BPA’s significant influence on ER activity, inflammation, and related signaling pathways, which in turn affect the proliferation and apoptosis of UL cells, ultimately promoting fibroid growth and development. However, a study focusing on reproductive-aged Black women did not find consistent and strong associations between urinary BPA concentrations and the incidence or growth of UFs (25). Consequently, in order to comprehensively evaluate the relationship between BPA and UFs, large-scale multi-species research is necessary.
PAEs
PAEs, as a plasticizer, are extensively used in certain detergents, medical products, and even as coatings for oral drugs and dietary supplements (26). They are also known to have endocrine-disrupting properties owing to their ability to interfere with the action or metabolism of sex hormones (27). The urinary concentration of PAE metabolites is commonly used as a representative biomarker of exposure to di (2-ethylhexyl) phthalate (DEHP). An in-depth analysis of baseline data obtained from the Midlife Women’s Health Study (MWHS) revealed a strong positive association between higher levels of ΣDEHP and related phthalate molar sums with the risk of prior fibroid diagnosis, particularly in women who experienced weight gain and obesity from early adulthood to midlife (28). Additionally, a study combining questionnaire survey data and high-performance liquid chromatography measurements found higher urine concentrations of DEHP in women with UFs, demonstrating positive associations between DEHP and uterine volume (29). A preliminary study investigating PAEs exposure and the burden of UFs among women undergoing surgical treatment found consistent associations between DEHP metabolites and uterine volume that were independent of race or ethnicity (30). Furthermore, in a mouse xenograft model, human fibroid tissue exposed to DEHP exhibited increased proliferation, tumor growth, and collagen production compared to tissue from animals fed a control substance (31). In vitro experiments using human uterine leiomyoma cells demonstrated that DEHP exposure enhanced cell viability, promoted anti-apoptotic protein expression, and induced the expression of HIF-1α and COX-2 (32). Other epidemiological and in vitro studies have shown that the major DEHP metabolite, mono (2-ethyl-5-hydroxyhexyl) phthalate (MEHHP), enhances the survival of leiomyoma cells through activation of the tryptophan-kynurenine-AHR pathway (33). Additionally, analysis of the TREE cohort data revealed positive associations between certain PAE exposures and the risks of UFs and endometriosis, which may be mediated by oxidatively generated DNA damage (34). Compared to conventional PAEs, a case-control study among Korean women before menopause conducted organophosphate esters (OPEs) and some alternative plasticizers are associated with increased risks of UFs (35).
However, a prospective ultrasound study showed little evidence of the effect of the PAE biomarkers mixture on uterine leiomyomata incidence (36). It is worth noting that the interplay of racism and sexism may potentially influence the research findings (37). Taking into consideration the simultaneous exposure to chemicals through common sources and metabolic pathways, multivariable logistic regression, WQS regression, and BKMR regression models were employed to investigate the joint effects of ten commonly encountered endocrine-disrupting chemicals, including urinary metabolites of PAEs and mono (2-ethylhexyl) phthalate (MEHP), revealing negative associations with uterine leiomyomas and endometriosis (26).
Although controversies persist, the majority of findings suggest that PAEs and their metabolites may contribute to oxidative stress and inflammatory responses, thereby promoting proliferative and anti-apoptotic activities in human uterine leiomyoma cells.
Other EDCs
In addition to the detrimental effects of BPA and PAEs on UFs development, analyses using simple and multiple linear regression revealed that elevated blood cadmium (Cd) levels were positively correlated with UF development in a group of 308 premenopausal women aged 30-49 years (38). Notably, a study conducted on fibroid cells indicated that exposure to Cd-induced changes in gene expression, leading to increased cellular movement and invasiveness (39). Furthermore, continuous exposure to Cd resulted in the transformation of benign human UL cells into malignant cells in vitro, which was accompanied by the downregulation of genes encoding for extracellular matrix components and the upregulation of genes involved in ECM degradation (40). Mechanistic research revealed that Cd-induced proliferation of human UL cells occurred through activation of Histone H3 and Aurora B via Fork head box M1/Cyclin D1 interactions downstream of MAPK and a nongenomic GPER/p-src/EGFR/MAPK signaling pathway that did not directly involve ERα (41, 42). Another noteworthy EDCs, diethylstilbestrol (DES), a synthetic nonsteroidal estrogen once used to support pregnancy and prevent and treat osteoporosis could lead to various uteropathies (43). A nationwide retrospective observational study on 529 families of DES-treated women showed that DES was associated with a higher incidence of UFs in women and highlighted a multigenerational and likely transgenerational effect of this EDC in humans (44). Female Eker rats exposed to DES 11μg/d on days 10, 11, and 12 after birth reprogramed the methylome and found an augmenting estrogen activity of myometrial stem cells, leading to a “hyper-inflammatory phenotype” and an increased hormone-dependent risk of UFs (45, 46). Further investigation into the molecular mechanisms implicated that early life exposure to DES suppressed nucleotide excision repair in rat myometrial stem cells through TGF-β signaling (47). Moreover, studies demonstrated that Vitamin D3 could mitigate the DNA damage caused by developmental DES exposure in uterine myometrial stem cells, effectively reducing the incidence of UF development (48). In comprehensive statistical analyses aiming to explore the associations between chemical mixtures and gynecological disorders, equol and mercury (Hg) were identified as the most significant chemicals linked to uterine leiomyomata (49, 50). In one study of reproductive-aged Black women, urinary concentrations of some phenols and parabens were weakly and non-monotonically associated with negative UFs growth (25). Parabens are commonly utilized as preservatives in various foods, including sauces, jams, and soft drinks, as well as in cosmetic products (51). Research in rodents has demonstrated that parabens can disrupt ovarian function and reduce fertility (52). It is biologically plausible that parabens act in an anti-estrogenic manner in the uterus, which could explain the finding of a weak inverse association between paraben exposure biomarkers and UFs incidence.
In vitro and in vivo studies have shown that BPA, NPH, DEHP, Cd, DES, Hg, and equol can cause DNA damage or impact epigenetic processes to fibroid pathogenesis, and early adolescence may be a window of susceptibility for fibroid development (53). These findings hold promise for the development of new prevention strategies for fibroids. However, further research at more realistic exposure levels is necessary to identify critical biological pathways and accurately assess the risks associated with windows of exposure to the aforementioned EDCs, taking into consideration underlying mechanisms and species-specific differences.
EDCs enhance invasive and proliferative activities of endometrial cells in endometriosis (EMs)
EMs, being a hormone-dependent chronic inflammatory disease, has a multifactorial etiology involving genetic, hormonal, immunologic, and environmental factors. Characterized by the implantation and growth of endometrial tissue outside the uterine cavity, EMs are strongly associated with high estrogen secretion. Numerous studies have indicated a potential link between exposure to EDCs, particularly BPA and PAEs, and abnormal secretion of estrogen in the HPG axis, thereby playing a role in the etiology of EMs (54–58). Nevertheless, further research is required to systematically evaluate the probability and strength of these exposure-outcome relationships.
BPA
Exposure to BPA increased the odds of having EMs in a dose-response relationship (59), and oxidative stress perhaps plays a potential role in the endocrine-disruptive (60). Histopathological examinations of the uterus in CD1 mice exposed to varying doses of BPA revealed an accumulation of collagen and an elevated number of F4/80 positive macrophages, resembling characteristics associated with an EMs-like phenotype (61). Similarly, experiments using a mouse model of EMs demonstrated that BPA exposure led to an upregulation of ER-β expression and an increase in both the size and number of endometriotic lesions. Co-administration of an ER-β antagonist blunted the effects of BPA on lesion number and volume, suggesting that BPA promotes endometriotic lesion formation and growth in an ERβ-dependent manner. Molecular mechanism studies have demonstrated that BPA modulates the WDR5/TET2 complex, resulting in the regulation of ER-β expression in eutopic endometrium and driving the development of EMs (62). Additionally, BPA treatment of endometriosis stromal cells (ESCs) under serum-free revealed that ESC proliferation was most pronounced upon exposure to 1000 pmol BPA (63). BPA was also found to enhance the invasion of human ESCs by upregulating the expressions of matrix metalloproteinase-2 (MMP-2) and MMP-9 through the GPER-mediated MAPK/ERK signaling pathway, thereby increasing the risk of peritoneal EMs (64). Furthermore, bisphenol AF (BPAF) has been found to disrupt normal ovarian signaling to a greater extent than BPA when administered through the diet in a mouse model of EMs, resulting in increased growth of EMs lesions (65).
Moreover, evidence suggests that BPA and its substitutes may have consequences across generations, compromising the ovarian function of subsequent cohorts. These previous studies confirm the increased incidence of EMs-like lesions associated with BPA exposure, highlighting the importance of conducting further epidemiological studies to evaluate the potential association between BPA and EMs using biomarker analysis.
PAEs
The results of the systematic review as of July 2018 revealed PAEs were positively associated with the prevalence of EMs (66). In Asia, research has demonstrated that exposure to MEHHP, a metabolite of PAEs, may pose a potential risk for women with EMs (67), similar to how DEHP affects women’s reproductive prognosis and ovarian function (68). Endometriotic tissue is featured by high prostaglandin levels and progesterone resistance. Microarray studies showed that DEHP upregulated aldo-keto reductases expression in EMs patients and enhanced progesterone resistance (69). Furthermore, treatment of endometrial cells with DEHP may increase extracellular signal-regulated kinase phosphorylation and regulate Pak4 expression collaborate with the increase in migration and invasion of endometrial cells, and therefore with the development of EMs (70). The significant increase in endometrial stromal cells invasiveness observed after DEHP exposure could be a link between DEHP exposure and increased EMs likelihood (71). Additionally, nonobese diabetic/severe combined immunodeficiency mice fed with DEHP have exhibited greater growth and proliferation of endometrial implants compared to those fed with a vehicle (70). PAEs are also suspected of promoting EMs through mechanisms other than binding to estradiol receptors (72). Exposure to mono-n-butyl phthalate (MnBP), a PAE metabolite, in Women with EMs, had been found to potentially affect the health and steroidogenesis of human granulosa cells (73). Chronic exposure to low-dose n-butyl benzyl phthalate (BBP), a widely used plasticizer, has been found to have weak estrogenic activity and may potentially promote the survival of endometriotic tissue through CD44-expressing plasmacytoid dendritic cells (74). This highlights the critical need for policy and regulatory initiatives aimed at identifying and controlling the health-related impacts of plastics. Simultaneously, it underscores the necessity for well-designed cohort studies with significant sample sizes to provide further insights.
In vitro results have indicated the capacity of PAEs to induce EMs. However, no meaningful relationship was found between the concentration of BPA metabolites in urinary samples and EMs, as determined through biomarker analysis. Likewise, similar results were derived from the study on PAEs (75). Given the weak strength of the results, additional research is warranted to unravel the mechanisms underlying the interaction between PAEs and EMs.
Other EDCs
The risk of developing EMs in adult life was significantly increased by exposure to DES in utero (76). A case report noted that all daughters and subsequent granddaughters who were prenatally exposed to DES presented with EMs, suggesting a role of DES in the pathogenesis of this disease (77). Animal studies have shown that DES induces erratic estrous cycles and abnormal hormone levels, demonstrating its efficacy in inducing EMs and suggesting its potential as an alternative approach in rat models (78). Integrating metabolic and cytokine profiling, a comprehensive approach has provided support for the association between EMs and certain persistent organic pollutants (POPs) like trans-nonachlor, polychlorinated biphenyls (PCBs) 114 and dioxin-like toxic equivalents from PCBs (79). PCBs are a halogenated aromatic group of ubiquitous, persistent environmental pollutants, and they can be present in high concentrations in fatty foods such as meat, fish and dairy products (80). Findings from the ENDO Study indicated an association between adipose-to-serum ratio and adipose estrogenic PCB and organochlorine pesticides (OCPs) mixtures and incident EMs (81). OCPs are synthetic pesticides that have been widely used in agricultural programs and epidemics in the past decades. Owing to the multiple toxicities and environmental persistence, OCPs have been banned or restricted in many countries. Higher levels of perfluorooctane sulphonate (PFOS) and perfluorooctanoic acid (PFOA) were observed in EMs patients, as measured in women aged 20-50 years from the National Health and Nutrition Examination Survey (82). Experimental evidence from research on human endometrial stromal cells and endometrial stromal cells indicates that hexachlorobenzene (HCB) induces cell migration and invasion, and enhances aromatase expression levels (83). Female rats exposed to varying doses of HCB for 30 days exhibited enhanced growth of endometriotic lesions and associated abnormal changes characteristic of EMs (84). In vivo mouse model confirmed that dioxins and dioxin-like PCBs promoted the development of EMs via enhancing 17β-estradiol biosynthesis and inflammatory response mediated by aryl hydrocarbon (85, 86). Dioxin, a byproduct of combustion and various industrial production, has irreversible “teratogenic, carcinogenic, and mutagenic” toxicity. Human can be exposed to dioxins and their by-products by consuming high-fat foods such as meat, poultry, milk, egg. However, one research analyzed plasma levels of PCBs and did not find any clear association between exposure to PCBs and the risk of EMs (87).
Several in vitro studies have demonstrated that EDCs, represented by BPA, PAE and DES, could cause inflammation, invasion, change in cytokines, increased oxidative stress, viability, resistance to hydrogen peroxide, and proliferation of endometrial cells. It is important to note that the mechanism of EDC exposure in relation to EMs is still not fully understood, mainly due to the complex pathophysiology of this gynecological disease. These findings underscore the importance of considering mixtures when assessing exposure-disease relationships. Consequently, further large-scale and homogeneous studies are needed to focus on the interactions and to draw conclusions about the influence of these EDCs on the development of EMs.
EDCs promote the characteristic performance of polycystic ovary syndrome (PCOS)
PCOS is a heterogeneous disease characterized by hyperandrogenism, ovulatory dysfunction, and polycystic ovarian morphology, ultimately causing anovulatory infertility in women. The etiology of PCOS has not been elucidated, which may be due to the interaction between specific genetic and environmental factors. Extensive evidence has shown that EDCs can provoke abnormalities in the HPG axis, even at low concentrations, thereby leading to metabolic disorders and suggesting a possible etiological mechanism underlying PCOS (88, 89).
BPA
Evidence from numerous studies conducted on infertile women supports a strong association between BPA and PCOS. A meta-analysis showed that PCOS patients had significantly higher BPA levels than those of control groups (90). In fact, as much as 48.28% of PCOS patients were found to have detectable BPA in their urine samples. Furthermore, subsequent investigations revealed a positive correlation between BPA and testosterone levels, serum insulin levels, and the incidence of insulin resistance in PCOS patients (91). Moreover, distinct patterns in the steroid hormone biosynthesis and metabolism of linoleic acid, linolenic acid, and sphingolipids were observed in the blood plasma of BPA-exposed patients, providing valuable insights into the underlying metabolic disorders in PCOS (92). Animal experiments studying molecular mechanisms of action provided insights on the effects of BPA exposure on ovulatory, hormonal, mitochondrial dysfunction, and senescence all hallmarks of PCOS phenotype (93). Additionally, female rats subjected to BPA, tributyltin (TBT), or a combination of both from postnatal day 1-16 exhibited irregular estrus cycles, reduced corpora lutea and antral follicles, and an increased occurrence of atretic follicles and cysts (94). Collectively, the aforementioned reports collectively illustrate the detrimental role of BPA in the development and pathogenesis of PCOS. The molecular effect of BPA on PCOS can be summarized as altering ovarian steroidogenesis (95), exacerbating the state of hyperandrogenism, affecting oocyte development, folliculogenesis, and aggravating metabolic parameters. A study on a single generation revealed the combined effects of parental obesity and transgenerational reproductive toxicity of BPA (96). More intricate research has indicated that ancestral exposure to BPA can induce PCOS-like phenotypes in subsequent unexposed generations by activating cancerous pathways, altering arginine-proline metabolism, insulin signaling, AMPK, HOTAIR regulatory pathways, as well as modulating the upstream regulators ESR1 and TGF signaling in the ovary (97). Moreover, a randomized controlled experiment found higher serum levels of BPA replacement chemicals in women with PCOS (98, which is supported by similar findings in a case-control study (99).
Due to the inherent challenges in fully aligning human and animal study results, it remains difficult to ascertain the exact role of BPA and its replacement chemicals in the pathogenesis of PCOS. Consequently, it is imperative to conduct prospective-designed studies focusing on the early origins of PCOS to shed light on the potential health risks associated with BPA exposure in women with this condition.
PAEs
Evidence from clinical research confirms that women with PCOS have higher levels of DEHP in their follicular fluid (FF) compared to control subjects, potentially contributing to pregnancy loss following in vitro fertilization (IVF) (100). A nested case-control study focusing on women undergoing IVF provides further support for the role of DEHP in PCOS pathogenesis, as urinary metabolites of PAE metabolites were found to be related to PCOS development (101). It is noteworthy that concentrations of PAE metabolites may also play a role in obesity, glucose, and lipid impairment in women with PCOS (102). The TREE cohort study specifically investigated the individual and joint associations of urinary PAE metabolites with PCOS, indicating that mono (2-ethylhexyl) phthalate (MEHP), monobenzyl phthalate (MBzP), and the overall sum of DEHP were associated with a higher prevalence of PCOS (103). Furthermore, PAEs were found to be linked to metabolic disturbances, including insulin resistance indices and serum triglycerides, in adolescents with PCOS (104). However, a study evaluating DEHP and MEHP levels in adolescents found no significant differences between those with and without PCOS (105).
Considering that PCOS is a complex endocrine and metabolic disorder encompassing reproductive and psychological dysfunctions, exposure to PAEs approximately contributes to PCOS progression through metabolic disruption. Nonetheless, given the existence of contradictory research findings, further investigation is required to identify the complete underlying mechanism of the characteristic performance of PCOS.
Other EDCs
Polycystic ovaries, chronic anovulation, and hyperandrogenism are classic features of PCOS. Gas Chromatography Mass-Mass Spectrometer analysis revealed that dichlorodiphenyltrichloroethane (DDT), an organochlorine pesticide, may play a critical role in PCOS pathogenesis by modulating reproductive hormone levels involved in androgen catabolism (106). Exposure to tributyltin (TBT), an organometallic xenobiotic, has been shown to induce reproductive, metabolic, and cardiovascular abnormalities similar to PCOS (107), making it a useful tool in the creation of PCOS rat models (108). The relationship between PCOS and exposure to perfluorinated substances (PFAS) has yielded contradictory findings, with some studies reporting a positive association (109, 110), while others report contradictory findings (111). PFAS is a kind of hard to degradation of synthetic chemicals that can mimic fatty acid and bio-accumulate in adipose tissues, activate the peroxisome proliferator-activated receptor and other pathways to play an endocrine role. Exposure to contaminated food, water, and soil can cause reproductive and developmental toxicity of PFAS to humans (112–114). Epidemiological research has demonstrated elevated levels of PFOA, a PFAS member, in the serum of women with PCOS, which were associated with irregular menstrual cycles (115). Experimental interventions involving PFOA in female mice have shown to be ovotoxic (116). Propylparaben (PP), an antimicrobial preservative commonly used in cosmetics, personal care products, and pharmaceuticals, has been shown to exert detrimental effects on ovarian estradiol secretion and ovulation in both mice and human ovarian granulosa tumor-derived cell lines (KGN) (117). In addition, polycyclic aromatic hydrocarbons (PAHs) (118), pesticides (119), and PCBs (120) are some of the EDCs detected in various matrices such as the serum, plasma, FF, and urine of PCOS patients.
Therefore, EDCs discussed above have the potential to affect hormonal function, ultimately affecting the function of sexual steroids, disrupting metabolic processes, compromising reproductive health, and exacerbating the risk associated with PCOS pathogenesis. Large-scale, double-blind, placebo-controlled randomized clinical trials should be conducted to confirm the findings from the aforementioned studies and gain a better understanding of the underlying mechanisms of action.
EDCs reduce the number of follicles causing diminished ovarian reserve (DOR)
The decline in ovarian reserve function represents the initial phase of ovarian aging or failure, which can be attributed to a diminished number of recruitable follicles in the ovaries or a decline in the fertilization capacity of oocytes. The ovary plays a significant role in feedback regulation of the HPG axis and female fertility by providing the eggs and sex steroid hormones for fertilization and the maintenance of reproductive function. Environmental factors have been recognized as significant contributors to the diminishment of ovarian resesrve and reproductive damage (19).
BPA
According to clinical practice, the decline in ovarian reserves can be assessed using the antral follicle count (AFC), which is the number of antral follicles measured through transvaginal ultrasound. A systematic review revealed a negative correlation between BPA and AFC, as well as sex hormone-binding globulin (121). Animal experiments also showed that BPA exposure caused irregular estrous cyclicity and reduced the number of follicles at all levels, including AFC (122). Studies conducted in northern China and South Korea have further conveyed that BPA and bisphenol S (BPS) disrupt steroidogenesis, inhibit follicle growth, and heighten the risk of DOR (123, 124).
Alongside AFC, serum indicators like anti-müllerian hormone (AMH), follicle-stimulating hormone (FSH), and estradiol (E2) in serum are used to measure ovarian reserve. Despite certain studies reporting an inverse association between BPA and AFC and AMH, it is essential for multiracial clinical research to investigate the relationship between BPA and these markers of DOR (125).
PAEs
The EARTH Study investigated the effect of female exposure to PAEs on ovarian reserve, as measured by AFC. Mean AFC exhibited significant decreases with increasing concentrations of DEHP, with decreases of 24%, 19%, and 14% in the 2nd, 3rd, and 4th quartiles, respectively, compared to women in the 1st quartile of urine DEHP (126). Cross-sectional research conducted in China also revealed that PAEs exposure may lower ovarian reserve by downregulating the concentrations of corticosterone and cortisol in FF (127). Additionally, PAEs have been shown to enhance oxidative stress, leading to follicle death, faster depletion of ovarian reserve, and earlier reproductive senescence (128). In vivo and in vitro experiments found that DEHP treatment drives ovarian dysfunction by disrupting the normal expression of ovarian function-related genes, inhibiting the proliferation of granulosa cells, and reducing healthy follicles via the SLC39A5/NF-κB/NLRP3 axis (129).
Although less research has been conducted on the correlation between PAE exposure and the progression of DOR compared to BPA, increasing evidence from animal experiments indicates that certain PAEs can modulate endogenous steroid hormone signaling. Consequently, there is growing concern about accelerated follicle loss and reproductive aging in humans. Therefore, comprehensive analysis is necessary to examine the interplay between PAEs and markers of ovarian reserve.
Pesticides
Pesticides comprise semi-volatile persistent organic pollutants, categorized by their use as biocides, fungicides, bactericides, insecticides, and herbicides (130, 131). These pesticides have been widely used due to the increasing global population and the accompanying demand for larger food supplies. The widespread application of insecticides in the agricultural field as well as in household practices is one of the potential environmental risk factors affecting the function of women’s ovaries. Pyrethroids, a widely utilized class of insecticides, are typically evaluated through the detection of their metabolites in urine. Unconditional logistic regression analysis has revealed a significant correlation between the concentrations of 3-phenoxybenzoic acid (3-PBA), a well-known metabolite of frequently utilized pyrethrin insecticides, and an elevated risk of ovarian dysfunction in individuals with POI (132). Additionally, malathion, a broad-spectrum insecticide employed in agricultural settings and public health pest control, has been linked to oxidative stress, DNA damage, granulosa cell apoptosis, and autophagy, which contribute to reproductive toxicity (133).
Consequently, insecticides persist in the environment and enter organisms through various exposure routes, including ingestion, resulting in a profound reduction in follicle count. Moreover, these chemicals have the ability to disrupt the expression of genes related to ovarian function and modify epigenetic markers, thereby posing potential hazards to human reproductive health.
Other EDCs
Exposure to PFAS has been found to disrupt the initial stages of folliculogenesis and reduce ovarian reserve by interfering with ovarian enzyme activities involved in steroidogenesis or by inhibiting kisspeptin signaling in the hypothalamus (134). Greater exposure to PFOA has been observed in patients with DOR and poses a threat to embryo development by altering the metabolic composition of FF (135). Triclosan (TCS), a lipid-soluble phenolic compound with broad-spectrum antibacterial properties, can be exposed to the general population through dermal and mucosal contact with consumer products. A prospective cohort study including 109 women seeking fertility treatment found that urinary TCS concentrations were inversely associated with AFC, thus affecting ovarian reserve (136). A French case-control study detected 17 POPs in serum samples, with only p,p’-dichlorodiphenyldichloroethylene (DDE) being significantly associated with an increased risk of DOR (137). Another case-control study utilizing Bayesian kernel machine and logistic regressions, validated the combined and individual effects of EDCs on DOR, with a particular emphasis on the influence of benzophenone-4 (BP4) (138). In addition, PP biomarker concentrations were inversely associated with ovarian antral follicle count (139). Animal research has shown that parabens exposure can lead to changes in fertility, fecundity, and reproductive parameters, accelerating ovarian aging (140, 141). In vitro experiments have shown that PP exposure of mature ovarian follicles resulted in growth inhibition under culture conditions (142). A pilot study observed modest inverse associations between methylparaben and ovarian volume, such that ovarian volume was −4.28% smaller with every two-fold increase in methylparaben (143). However, an epidemiological study of humans reported methyl (MP), ethyl (EP), butyl (BP) and izobutyl paraben (iBuP) parabens were not associated any with parameters of ovarian reserve (139).
Exposure to EDCs has been observed to reduce the number of functional follicles in the ovary by activating specific signaling pathways, disrupting intercellular communication between oocytes and granulosa cells, and inducing oxidative stress. These effects ultimately result in DOR and have long-lasting detrimental impacts on reproductive function (123, 144). However, a study conducted on women attending a fertility clinic found no association between mixtures of phenols and PAE metabolites in urine and ovarian reserve (145). Due to the limited number of studies and inconsistent findings, the potential impact of these EDCs on adverse female reproductive outcomes remains uncertain.
EDCs accelerate reproductive aging causing premature ovarian insufficient (POI)
POI, defined as a loss of ovarian function, represents an intermediate stage between DOR and premature ovarian failure (POF). It is characterized by the premature depletion of follicles, leading to amenorrhea, subfertility, or infertility. POI is a complex process influenced by various factors, and the increasing incidence of this condition suggests that environmental factors may serve as primary causes alongside genetic factors.
BPA
The proposed role of EDCs in the development of DOR indicates that compounds such as BPA and other plasticizers pose a significant threat to reproductive health and contribute to reproductive aging. Studies have identified higher levels of BPA and mono-butyl phthalate (MBP) in the serum of women with POI, suggesting that these chemicals may act as contributing risk factors for POI (146). Exposure to BPA during fetal development disrupts the balanced activation of primordial follicles. This disruption leads to the depletion of the non-renewable pool of primordial follicles (PFs), while fetal exposure to BPA induces excessive endoplasmic reticulum stress in granulosa cells, accelerating primordial follicle activation and the subsequent development of POI (147). Numerous rodent studies have demonstrated that BPA can disrupt hormone cyclicity, likely through alterations in estrogen regulation, underscoring the significant impact of BPA on ovarian function. However, a study conducted in China measuring the concentration of BPA and serum levels of reproductive hormones found no significant association between BPA and FSH and AMH levels (148).
The inconsistencies in these findings can be attributed to the inherent limitations of retrospective case-control studies that rely on post-diagnostic assessment of exposure levels. It is crucial to note that the single sampling approach can still adequately reflect the average exposure of the population to BPA. Nevertheless, further evaluation through population-based studies conducted in occupational or environmental settings is needed to fully comprehend the associations between BPA exposure and its related health outcomes.
PAEs
PAEs are known to exert toxicity on the reproductive system and human development. The reserve of PFs, which represents an individual’s potential fertility, plays a major role in determining the female reproductive lifespan. Epidemiological studies and experimental research have indicated that both acute and chronic exposure to PAEs disrupt the estrous cycle, hastens the recruitment of PFs, and accelerates ovarian reproductive aging, ultimately leading to the development of POF (149). Clinical studies and preclinical findings demonstrate that PAEs and PAHs overactivate the calcium signaling pathway and the PI3K/Akt pathway, leading to the recruitment and depletion of PFs, ultimately precipitating the onset of POI (150). PAEs exposure also decrease the expression of AMH, and raise serum FSH levels, thereby increasing the risk of premature menopause and exacerbating the symptoms of POF (151). Additionally, a case-control study conducted in China indicated that higher urinary concentrations of PAEs may impair ovarian function and increase the odds of POF (152). Furthermore, findings from mouse studies indicate that prenatal exposure to environmentally relevant mixtures of PAEs accelerates biomarkers of reproductive aging, leading to decreased testosterone and inhibin B levels, elevated FSH and LH levels, and a decrease in the percentage of PFs, with these effects extending across multiple generations (153). In humans, dibutyl phthalate, which is associated with decreased hormone production and DOR, can reach the ovary and impact its function (154). Inflammatory factors, particularly tumor necrosis factor (TNF) production, have been proposed as significant contributors to the induction of apoptosis in the mammalian ovary by both natural and synthetic environmental estrogens such as di-2-ethylhexyl phthalate and BPA (155).
While the investigation of the effects of chemicals in PAEs on reproductive function is still limited, studies have demonstrated that PAEs hasten the activation and depletion of PFs, disrupt menstrual cycle, and influence reproductive aging. These findings suggest the involvement of epigenetic mechanisms underlying the impact of PAEs on these endpoints, but warrants further investigation is required to confirm this hypothesis.
Pesticides
Pesticides are considered to have an impact on neuroendocrine regulation of the gonadal axis and effects on ovaries at different levels. Case-control studies conducted on the female population in China have revealed a correlation between exposure to OCPs, particularly p,p’-DDT, and lower levels of AMH, with a dose-response relationship observed for the risk of POI (156). Female mice exposed to acceptable daily intake or chronic reference doses of cypermethrin (CYP) from gestational day 0.5 until 44 weeks old exhibit an ovarian phenotype resembling human POI, characterized by increased apoptosis, decreased cell proliferation, and downregulation of genes involved in steroidogenesis (157). Moreover, in a study involving sexually mature female rats, lambda-cyhalothrin (LCT), a type II pyrethroid pesticide, was administered at two different doses, resulting in a simultaneous reduction of gonadotropic hormone, estradiol, and progesterone levels, as well as degenerative changes in the ovaries (158). These findings indicate the reproductive effects of LCT.
It is noticeable that many of the pesticides are toxic to non-target organisms, including, aquatic organisms, birds, domestic animals, and particularly humans. Exposure to pesticides can potentially cause generalized oxidative stress, disrupt the secretion of gonadal hormones, and negatively impact oocyte quality, implantation rate, and pregnancy rate. Therefore, the government should strengthen the management of the agricultural environment, formulate a more detailed and perfect agricultural protection plan, and reduce the use of pesticides while solving agricultural pests.
Other EDCs
The compound 4-vinylcyclohexenediepoxide (VCD), which serves as a flame retardant, antioxidant, and plasticizer, has been found to selectively damage primordial and primary ovarian follicles, deplete the follicular reserve, and induce a mouse model of POI (159). Clinical case-control studies show that dioxin-like PCBs have been significantly associated with POI (156). 2,3,7,8-tetrachlorodibenzo-p-dioxin (TCDD), a by-product of organic synthesis and burning, can rapidly accumulate in human and animal tissues, leading to reduced fertility. Pregnant Sprague-Dawley rats exposed to TCDD exhibit diminished ovarian reserves, and the offspring of these rats show inhibited follicular development in adulthood (160). Systematic reviews of the literature and a knowledge synthesis also pointed out that TCDD alters estrous cyclicity and impairs follicular development (161). Zhang et al. suggested that PFOS exposure may suppress ovarian hormone production and impair follicular development, resulting in the loss of ovarian function and earlier menopause among POI patients (162). In addition, parabens accelerate ovarian dysfunction in a 4-vinylcyclohexene diepoxide-induced ovarian failure model (163).
Therefore, the increasing data from epidemiological studies and experimental animal models indicate that exposure to the above EDCs can exhaust and deplete follicular cells, resulting in an earlier age of menopause, POI, and even POF. As prospective studies on EDC exposure levels in humans are challenging, conducting large-scale animal or cell experiments in the future could potentially address this limitation and offer significant insights into the relationship between EDCs and ovarian function.
EDCs contribute to lower probabilities of implantation and clinical pregnancy
Infertility rates in humans have witnessed a significant increase worldwide since the turn of the 21st century. The impaired secretory function of the HPG axis and the morphology and function of the female reproductive system may ultimately lead to female infertility. Exposure to certain EDCs, either alone or in combination, can disrupt the endocrine system and compromise the integrity of human stem cells. As a result, difficulties in conceiving or carrying a pregnancy to term emerge, thereby establishing EDCs as crucial environmental risk factors for infertility (164, 165).
BPA
Both epidemiological and experimental evidence demonstrate that all bisphenols affects female infertility and subfertility (166, 167). This effect extends to BPA substitutes like BPS and bisphenol F (BPF), which exhibit parallel endocrine-disrupting effects. In vitro maturation experiments involving bovine cumulus-oocyte complexes (COCs) exposed to BPA or BPS for 24 hours present heightened spindle abnormalities in MII oocytes and chromosome misalignment across various concentrations, ultimately leading to infertility (168). BPS is present in culture media used in assisted reproductive technology (ART) and cell culture, which may contribute to a decrease in the success rate of ART (169). Interventions using human, mouse, and human granulosa cell line (KGN) models have demonstrated that BPA exposure can abnormally influence ovarian functions, leading to abnormal folliculogenesis through the activation of autophagy in granulosa cells via the AMPK/mTOR/ULK1 pathway (170). BPA-induced apoptosis, mediated by GPER-dependent activation of the ROS/Ca2+-ASK1-JNK pathway in KGN cells, has also been linked to the occurrence of female infertility (171). Data from the National Health and Nutrition Examination Surveys manifested that a combination of phenol and PAE metabolites is linked to infertility among reproductive-age women, particularly BPA and DEHP. And the probability of infertility increased dramatically as the quantiles of the total mixture concentration increased (172). However, some research has suggested that BPA exposure is not associated with increased embryo implantation failure, decreased fertilized oocytes, or decreased oocyte counts (173).
Collectively, most experiments indicated an impairment of female infertility with BPA, notably during perinatal. This impairment occurs through various mechanisms, including disruption of gonadotropin signaling, sex steroid hormone production, histone modifications, and miRNA expression. Additionally, BPA exposure during early life may have transgenerational effects. It would be more informative to evaluate BPA exposure prior to disease onset rather than at the time of diagnosis. Larger and more diverse epidemiological research is needed to refine the above finding.
PAEs
Significantly higher values of certain PAEs, particularly DEHP, suggest a possible involvement of these compounds as competing factors in reproductive issues, especially in women with idiopathic infertility (174). One study identified preliminary evidence suggesting an association between DEHP and infertility in women (175). Urinary PAE metabolite concentrations have been found to be negatively associated with AMH hormone concentrations in the FF of women undergoing fertility treatment. This association may have implications for antral follicle recruitment and fertility treatment outcomes (176). High DEHP exposure and its correlation with infertility have been confirmed in a study conducted in Jordan (177). Furthermore, DEHP metabolites in Sweden and Estonia have shown a significant inverse association with the ovarian sensitivity index, potentially leading to altered ovarian function and infertility in women (178). Studies have also indicated that paternal mixtures of urinary DEHP metabolite concentrations are related to higher rates of infertility treatment failure (179). Moreover, findings from studies using FF aspirated from individual follicles indicate that PAE-induced endocrine defects observed in vitro in follicular cells may also occur in humans at environmentally relevant exposure levels (180). Research conducted on Wistar rats has demonstrated that exposure to DEHP or diisononyl phthalate (DINP) can disrupt ovarian follicle recruitment and ultimately maturation, potentially resulting in severe consequences for female fertility (181).
Studies have identified preliminary evidence suggesting an association between DEHP and infertility in women may contribute to lower probabilities of implantation, clinical pregnancy, and live birth by changing estrous cyclicity, aggravating oxidative stress, and damaging oocyte quality. It is worth noting that the results of these studies should be interpreted with caution due to the limitations of different research subjects, methods, and quantities.
Heavy metals
Environmental degradation can increase the likelihood of human exposure to heavy metals (HM) from natural sources and human activities. Such exposure can lead to various health consequences, including reproductive problems. A cross-sectional analysis from 2013 to 2018 NHANES data revealed urinary arsenic (As) and cadmium (Cd) were associated with female infertility, while blood/urine lead (Pb) was found to be related to infertility in overweight/obese women and those in advanced age (182). Cd has been shown to induce apoptosis of the human granulosa cell line KGN through mitochondrial dysfunction-mediated pathways and may cause female reproductive toxicity (183). Pb exposure affects ovary development, folliculogenesis, and steroidogenesis in rats through the activation of the IRE1α-JNK signaling pathway, posing a hazard to reproductive health (184). The microenvironment provided by the follicular fluid (FF) affects the quality of oocytes. An excess of iron and calcium in the FF reduces the rate of good quality embryos, while an excess of potassium impairs the blastocyst rate (185). Increased use and improper disposal of hexavalent chromium (Cr), an environmental contaminant, can disrupt oocyte development in rats by elevating oxidative stress, causing DNA double-strand breaks, microtubule disruption, and aberrant chromosome segregation. These effects may result in embryo lethality, infertility, or birth defects (186). In addition, mercury impairs the function of human primary endometrial stromal cells, impacting human fertility and posing a hazard to the outcomes of IVF procedures (187).
Exposure to heavy metals is known to have detrimental effects on oocyte maturation, ovulation, and fertilization. This occurs through the induction of oxidative stress and damage to biological molecules, ultimately impacting female reproductive capacity and assisted reproduction treatments. Although the precise causal link remains incompletely understood, these findings have significant implications for the management of patients with subfertility. To mitigate potential harm to the reproductive system, it is crucial to minimize exposure to metal pollutants in daily life and carefully consider the intake of specific trace elements.
Other EDCs
Apart from the aforementioned BPA, PAEs, and heavy metals, numerous other EDCs have detrimental effects on reproductive health. Glyphosate, the primary ingredient in glyphosate-based herbicides, has been shown to disrupt female reproduction in various animal models including fish, chicks, rats, mice, and ewe lambs. Its effects can include prolonged time-to-conceive, spontaneous abortion, stillbirths, and developmental defects (188, 189). These impacts are believed to be mediated by alterations in estrogen receptors and molecules involved in estrogenic pathways. Chlorpyrifos (CPF), a very effective, low-cost, and easily accessible acaricide insecticide, either as a consequence of hormonal changes or by direct local action, induces proliferative changes in the uterus of the rat (190). This result may suggest that CPF chronic exposure could affect reproduction or act as a risk factor in the development of uterine proliferative pathologies. Endosulfan, one of the major cyclodiene pesticides, is also shown to cause ovarian regression in females, alterations in hormone synthesis, follicular maturation, ovulation process, and ovarian cycle, which leads to an increase in infertility (189, 191). A population-level study revealed a heightened correlation between pesticide metabolite concentrations in urine and the risk of infertility among individuals with a high body mass index (192). Additionally, a 12-month prospective follow-up study involving 1,182 couples attempting to achieve pregnancy discovered an elevated risk of menstrual abnormalities and low fertility associated with high levels of TCS, a common ingredient in personal care and household products (193). The concentration of polypropylene in the urine of infertile women, determined through verified gas chromatography ion volley mass spectrometry, has been found to be related to ovarian reserve parameters (139). This suggests that polypropylene may have the potential to reduce fertility. Analysis of POPs in the blood and FF collected from infertile women undergoing assisted reproductive technology treatments in Sweden has revealed an association between HCB levels and clinical pregnancy and live birth outcomes (194). Subsequent studies have found that higher concentrations of PCBs and pesticides in FF are associated with thinner endometrial thickness, reduced numbers of retrieved oocytes, and decreased fertilization rates in intracytoplasmic sperm injection procedures (195). Self-reported lifestyle factors and EDCs concentrations in FF have shown that the presence of PFAS increases ovarian sensitivity to hormone stimulation while reducing embryo quality and fertility (196, 197), especially preconception exposure (198). In vitro models examining the effects of DES on the human ovarian cortex provide compelling evidence that it decreases the density of unilaminar follicles and negatively impacts fertility (199). Additionally, studies using environmentally relevant doses of TBT to disrupt mammalian ovarian function in vitro and in vivo have demonstrated impaired ovarian function and fertility due, in part, to abnormal sensitivity to steroid feedback mechanisms and detrimental effects on the ovaries (200, 201). Ethyl paraben and mixtures of benzophenones, TCS, and BPA were associated with infertility among women in the United States (202). Within a large chemical mixture, inverse associations levels of DEHP metabolites and methylparaben, and possibly PFOA, with ovarian sensitivity index are observed in Sweden and Estonia women, suggesting that these chemicals may contribute to altered ovarian function and infertility (178). Interestingly, the study conducted in Asians announced that the joint effect of paraben mixture on couple fecundity was non-significant (203).
There is convincing evidence that EDCs can potentially harm human health and reproductive potential. The above-mentioned studies have demonstrated that oocytes in the FF can be directly exposed to these chemicals, resulting in endocrine-mediated effects that can lead to ovulation failure. Furthermore, certain EDCs have been shown to cause endometrial dysfunction and implantation failure, potentially through the involvement of oxidative stress, inflammatory and apoptotic mediators. These effects ultimately reduce pregnancy rates and pose a threat to female reproductive function. It is imperative to advocate for sensible lifestyle modifications in both females and males aiming to conceive, in order to minimize EDC exposure. To sum up, the results of these studies should be validated in-depth, and the differences in the body’s response and the interaction between the environment and genes need to be clarified through subsequent prospective population research.
EDCs interfere with some endocrine and intracrine targets relevant to breast cancer (BC)
The mammary gland serves as the targeted regulatory organ of the hypothalamic-pituitary-ovarian axis. Imbalances in this axis can lead to hormonal secretion disorders, which in turn affect the breast by causing mutations in cell and tissue proliferation and division. These alterations also impact the expression of oncogenes and tumor suppressor genes, ultimately contributing to the pathogenesis of BC. BC, a hormone-dependent specific tumor of the reproductive system, accounts for approximately 30% of female cancers and represents one of the leading causes of death in women (204, 205). The secretion of estrogen and progesterone from the ovaries stimulates the proliferation of myoepithelial cells within ducts, with estrogen being the primary sex hormone responsible for mammary gland development during critical life stages (206). Risk factors for BC can be categorized into familial, hereditary, and environmental exposure. The estrogenic properties of EDCs may be potentially linked to the increasing rates of BC. Demographic research has confirmed the relationship between the incidence of BC and EDCs, such as BPA, PAEs, pesticides, parabens, polybrominated diphenyl ethers (PBDEs), and other environmental estrogens compounds.
BPA
Research indicates that the mean concentration of BPA is higher in cancerous patients compared to non-cancerous individuals, potentially increasing the risk of BC incidence (207). Following in-depth research reported that BPA may induce metastatic aggression in low metastatic human BC cells through PGC-1α mediated mitochondrial biogenesis and epithelial-mesenchymal plasticity (208). Notably, co-exposure to DEHP and BPA increased the incidence and reduced the latency of mammary tumors, which seemed to enhance the susceptibility of carcinogen-induced tumors (209). Despite DEHP exhibiting no apparent estrogenic activity, it can still disrupt ER activity to increase serum estradiol levels. Nevertheless, a recent meta-analysis, comprising 9 case–control studies involving 7820 BC cases, found no associations between BPA and BC (210).
Large-scale epidemiological studies are essential to determine the causal relationship between BPA and BC. The ER is a key transcriptional factor that drives the oncogenesis and growth of hormonally sensitive breast cells in collaboration with other growth factors. At least, BPA exposure could be a risk factor for ductal hyperplasia, possibly through an estrogen-dependent mechanism.
PAEs
Studies have indicated a positive association between PAEs and BC risk (211). Differential increases in serum PAE concentrations have been observed in women with BC compared to those without the disease in Mexico and Toluca City, thereby supporting the hypothesis of a positive association between PAE exposure and BC incidence (212). It has been suggested that PAEs could exert multiple parallel interactions with ERα signaling, emphasizing adverse health outcomes in BC cell proliferation (213). Furthermore, evidence indicates that the activation of the aryl hydrocarbon receptor (AhR) can promote cancer cell metastasis. Both MEHP and TCDD can induce migration and invasion in MCF7 human BC cells, with this promotion being partly AhR dependent (214). What’s interesting is that the co-exposure can produce antagonistic effect. Moreover, other authors found that PAEs metabolites could upregulate the expression of disintegrin and metalloproteinase domain 33 (ADAM33), which plays a significant role in reducing BC risk (215).
As a commonly used plasticizer, PAEs are widely utilized in industrial, medical, and life-related fields, and are considered to be ubiquitous environmental hormones. The complex underlying mechanisms of BC development, the wide variety of PAEs, and ethnic differences in study populations may have contributed to the contrary findings.
PBDEs
Polybrominated diphenyl ethers (PBDEs) are synthetic halogenated compounds that have been widely distributed as environmental contaminants due to their extensive use as additive flame retardants in various household products, such as furniture and hard plastic coatings in appliances. Owing to their endocrine-disrupting effects, PBDEs have garnered significant interest in their potential connection with BC. Certain PBDE congeners have been shown to stimulate the proliferation of human BC cells (216). Recent studies involving Chinese women have revealed a positive correlation between BC growth and exposure to BDE-47, one of the PBDE congeners that has received intense focus due to its widespread existence in the environment and adverse health effects (217, 218). However, evidence from case-control studies in France and California has shown no significant associations between PBDEs and BC (219, 220). Additionally, significant differences in PBDE levels were not observed when examining malignant and benign tumor tissues (221).
Due to their structural similarities to PCBs, PBDEs are also considered suspected human chemical carcinogens. Although the mechanism by which PBDEs cause cancer is not well understood, efforts should be made to minimize daily exposure, as they enter the food chain and accumulate in higher predators that are eventually consumed by humans.
Pesticides
When considering the links between breast cancer (BC) and environmental factors in humans, the most compelling data, apart from diet, arises from pesticide exposure in farming, which has been associated with an increased incidence of BC. The mammary glands of both male and female rats and mice are susceptible to non-organochlorine (such as vinclozolin, atrazine, glyphosate, chlorpyrifos) and organochlorine (including endosulfan, methoxychlor, hexachlorobenzene) pesticides (222, 223). Experimental models have demonstrated various effects of these compounds, including alterations in mammary development, impaired cell proliferation and steroid receptor expression and signaling, heightened malignant cellular transformation and tumor development, and increased angiogenesis.
So far, most studies have focused on individual pesticides or the direct modulating effects of compounds on sex hormone receptors. Few prospective studies have investigated OCPs in relation to overall or BC-specific mortality following the diagnosis of BC. Therefore, evidence from in vivo and epidemiological studies needs to be further expanded.
Parabens
Epidemiological evidence also suggests a connection between paraben exposure and the development of BC. For instance, one study indicated that the highest quintiles of urinary total parabens in women were linked to an increased risk of BC (224). Additionally, parabens have been detected in breast tissue, including breast tumor tissue (225, 226). Specifically, parabens have been observed to enhance the proliferation of BC cell lines at biologically relevant levels, mainly in an ER-dependent manner (227). Mechanistic research has also revealed that exposure to PP during pregnancy and lactation increases epithelial cell proliferation, decreases collagen thickness, and alters the immune cell profile in the mammary gland (228). However, conflicting conclusions have been reported in some studies. For instance, a case-control study involving a multiethnic cohort showed an inverse relationship between total paraben exposure and BC risk in postmenopausal women (229). It is worth noting that one major difference between these studies is the collection of post-diagnostic urinary samples in one case and pre-diagnostic urinary samples in the other, leading to potential difficulties in comparing the results. Furthermore, contradictory findings have emerged from studies examining the association of personal care product use (a potential source of parabens) and BC risk, with a weak inverse association found in the Norwegian Women and Cancer Cohort (230) and a 10% to 15% higher risk of BC in moderate-to-frequent users of beauty products compared to less frequent users in the Sister Study (231).
Research increasingly suggests that exposure to low doses of parabens during sensitive developmental periods can lead to changes in mammary gland morphology and gene expression. EDCs encountered during critical periods of breast cell proliferation and differentiation, such as puberty and pregnancy, have the potential to affect mammary gland development, but further elucidation of this relationship is necessary (232). The main concerns regarding parabens use in consumer products are their potential mimicry of endogenous hormones (226), possible cross-talks with other signal transduction pathways that are pivotal in the development of BC (233), and modulation of key enzymes involved in local estrogen metabolism.
Other EDCs
The World Health Organization (WHO) and the Endocrine Society have both highlighted the carcinogenic role of dioxin. A prospective cohort study in the USA suggested a positive relationship between municipal solid waste incinerator (MSWI)-produced dioxin exposure and invasive BC, with a higher risk for women living within 5 km of MSWIs (234). However, a case–control study nested within the French E3N prospective cohort did not show an increased risk of breast cancer from higher airborne dioxin exposure, possibly due to the small population size (235). Given that dioxin is classified as a known human carcinogen by the International Agency for Research on Cancer (IARC) and its pathogenic mechanism is plausible, it is reasonable to propose a possible role in breast cancer development. Additionally, exposure to PFOA promotes proliferation, migration, and invasion potential in human breast epithelial cells (236). Positive associations between breast cancer risk and perfluoroalkylated substances, including PFOA, have been observed in French women (220). However, a nested case-control study among California teachers showed no correlation between serum PFAS levels measured after diagnosis with breast cancer risk (237). Specifically, circulating PFOS concentration was associated with estrogen receptor–positive tumors, while low concentrations of PFOS and PFOA were associated with estrogen receptor–negative tumors (220). In other words, women younger than 50 years old had a higher risk, and this risk increased when tumors were estrogen receptor–positive. A follow-up study suggested that the inhibition of mammary gland development by PFOA might elevate the risk of developing mammary tumors through the activation of signaling pathways associated with tumorigenesis (238).
In addition, the association between DDT or its metabolites in lipid, serum, or plasma and BC incidences is noteworthy. Higher DDE and DDT levels were linked to worse overall survival in the Carolina Breast Cancer Study (239). Studies have shown that intrauterine and infant DDT exposure increases the risk of premenopausal BC, while DDT exposure after infancy elevates BC risk in the early postmenopausal years (240). This emphasizes that time of first exposure and the levels of exposure at younger ages are critical for accurate assessment of the BC risk. On the contrary, a cross-sectional study investigating the potential impact of organochlorine chemicals on mammographic breast density found no correlation between higher DDE levels in women and BC risk (241). Furthermore, a hospital-based case-control study in Chinese women found a positive association between certain PCB exposures and elevated incidence of BC (242). Moreover, a meta-analysis highlighted the increased risk of BC associated with five specific polychlorinated biphenyls (PCB 99, PCB 105, PCB 118, PCB 138, and PCB 183) (223).
Regarding Cd, a recent meta-analysis of cohort studies discovered only a marginal positive relationship between dietary cadmium intake and BC, with no clear mechanism (243, 244). In vitro and animal model studies have demonstrated that this heavy metal possesses estrogen-like properties, can increase migration and epithelial–mesenchymal transition of BC cells, and can promote the production of active oxygen species (245, 246). It is important to note that developing breast tissue is susceptible to select EDCs during childhood and adolescence. Polycyclic aromatic hydrocarbons (PAHs), a class of EDCs, are believed to potentially delay the onset of breast development in girls (247). PAHs are byproducts of incomplete combustion of coal, oil, natural gas, wood, and paper. Notably, it is particularly noteworthy that more than 150 types of PAH have been detected in the smoke emitted by smokers so far. PAH-enriched EDC mixtures have been shown to enhance aryl hydrocarbon receptor and antiapoptotic signaling, promoting a proliferative phenotype in BC cells (248).
Given that most studies on the relationship between EDCs and BC risk are case-control studies, it is inevitable that there may be potential biases in exposure estimates during sample collection. Prospective studies focusing on the association between duration, dose, and age of EDCs exposure, and BC risk should be considered. Additionally, the influence of androgen levels is also significant in BC development, and it is crucial to explore the effect of EDCs on testosterone and BC risk. For now, caution should be taken when individuals, including BC patients or individuals with a high risk of BC, make decisions on common products containing those EDCs.
EDCs facilitate the development of estrogen-dependent endometrial cancer (EC)
EC is the sixth most commonly diagnosed cancer in women. The most prevalent symptom is abnormal uterine bleeding, while vaginal discharge and pyometra can also occur. Prolonged exposure of the endometrium to estrogen in the context of a dysfunctional HPG axis and deficient progesterone conversion can facilitate the development of endometrial hyperplasia and potentially EC. The majority of ECs are estrogen-dependent type I cancers, and excessive exposure to EDCs heighten the associated risk.
Chronic exposure to BPA has been shown to impair steroid hormone signaling in the uterus of mice, potentially contributing to the development of uterine hyperplasia and cancer (249). Research on the risks of EDCs to EC has been extensive. Apart from the receptor-mediated mechanism, BPA may exert its carcinogenic role through epigenetic mutations, thereby altering developmental pathways and cellular processes (250). A low-dose BPA-gene signature has demonstrated predictive value for survival outcomes in EC patients (251). Analysis using inductively coupled plasma optical emission spectrometry has explored risk factors for EC and blood cadmium (Cd) levels, indicating that intake or environmental exposure to Cd may result in estrogen-dependent diseases, including EC (252, 253). Moreover, high dietary Cd intake might be associated with poorer outcomes in women with EC (254). With growing evidence suggesting a relationship between altered serum trace metal concentration and EC, further research on a larger group of patients is required to validate and evaluate our findings. Especially regarding serum copper and zinc (Zn) concentrations, which have shown a potential link to altered trace metal levels and EC (255, 256). A nested case-control study conducted within the Multiethnic Cohort revealed a positive association between the urinary excretion of monoisobutyl phthalate (MiBP) and MnBP and the risk of EC (257). In addition, the occurrence of EC was found to be associated with the use of alkylphenol ethoxylates (APEOs), nonionic surfactants widely utilized as emulsifiers (258).
Mounting evidence suggests a link between EDCs and the incidence and development of hormone-related tumors, with EC serving as a representative example. Suspected EDCs, including BPA, MiBP, MnBP, and APEOs, are thought to induce genotoxicity via multiple pathways, thereby increasing the rate of EC development. Furthermore, excessive accumulation of non-essential heavy metals like copper in the body has been linked to the occurrence of EC. Due to the burden of gynecological cancer on female reproductive health, additional studies are urgently needed to identify risk factors. The prevention and treatment of EC should be based on reducing exposure to the above EDCs.
EDCs induce cell aberrant proliferation and metastasis in ovarian cancer (OC)
The available data have shown that OC is associated with a number of estrogen-regulated pathways, similar to CC, which are also partially regulated by HPG axis function. The concealment of early symptoms of OC, coupled with the lack of accurate non-invasive screening methods, contributes to more than 70% of patients have progressed to advanced stages when diagnosed, resulting in OC accounting for over two-thirds of gynecological cancer-related deaths (259). Multiple factors, including genetic, endocrinology and environmental, may contribute to the process of OC formation, of which EDCs are an established risk factor.
Gene Ontology analysis revealed that exposure to 100 nM BPA resulted in the dysregulation of numerous genes, specifically 159 genes associated with OC (260). In silico analyses utilizing gene expression data from The Cancer Genome Atlas (TCGA) and the Genotype-Tissue Expression (GTEx) databases demonstrated that 14 out of 94 genes were exclusively dysregulated in the presence of BPA, while the remaining 80 genes already exhibited dysregulated expression patterns as a consequence of the disease itself (261). Although EDCs do not typically modify DNA sequences, each category of EDC has its specific mechanism of action, which can differ from others. Heavy metal Cd increases the expression of P-glycoprotein in the ovarian cell line SKOV-3, which may negatively affect anticancer treatment (262). Benzophenone-1 (BP-1), an endocrine-disrupting compound found in personal care products, exhibited estrogen-like effects. Molecular dynamics simulations, in combination with yeast-based assays, demonstrated the persistent binding of BP-1 to the ligand binding domain of ERα, leading to the abnormal proliferation and metastasis of OC cells (263).
These studies underscore the potential role of EDCs with sex-hormone activity in the etiology of OC. Despite being relatively uncommon, OC is a highly lethal disease. Exposure to EDCs such as BPA, Cd, and BP-1, commonly found in plasticizers, cosmetics, and other products, may contribute to the progression of OC through epimutations. The diverse range of changes observed with different compounds suggests the involvement of multiple mechanisms in promoting female reproductive pathology. These findings provide insights for implementing preventive measures aimed at reducing exposure to the mentioned EDCs, subsequently affecting disease progression.
EDCs regulate the cell cycle in cervical cancer (CC)
CC, along with EC and OC, has a potentially impaired HPG axis function, leading to abnormal hormone secretion pulse rhythms, and is the most commonly diagnosed cancer among women and a leading cause of cancer-related deaths. While human papillomavirus (HPV) is a necessary but insufficient cause of CC, other significant cofactors, including certain EDCs, also play a prominent role. High levels of BPA and PAEs exposure have been observed in women with CC, with the metabolite MEHP, a byproduct of DEHP, exhibiting the highest concentration (264). Additional research is needed to investigate the potential synergistic proliferation of CC resulting from the interplay between EDCs and HPV, given the correlation observed between urine samples from CC women and HPV infection.
The regulation of cancer gene expression involves a cooperative interplay between epigenetics and genetics. EDCs not only have the ability to regulate hormone levels in the body through HPG but also can affect the biological processes of OC through various pathways such as epimutations. There are still significant knowledge gaps in understanding the correlation between OC and EDCs due to the multifactorial nature of cancer development.
Aside from BC, EC, OC, and CC, tumors can also emerge in other gynecological sites such as the vagina and fallopian tubes. EDCs, particularly BPA, present various threats to the occurrence and progression of gynecological cancers. However, determining the carcinogenic effects of EDCs is challenging due to the difficulty in evaluating lifelong exposure. While adults typically require higher concentrations of EDCs to experience toxic effects, developmental stages can be affected by low doses, and cumulative exposure to the environment can lead to the manifestation of diseases later in life. The exact amounts of EDCs causing cancer are still unclear, but it is hypothesized that during the embryonic period, puberty, menopause, and when the ovaries are in a degenerating state, they may be more vulnerable to the effects of exogenous chemicals in the form of EDCs. In other words, EDCs can have an impact even at low doses and can induce unpredictable interactions, thus establishing their recognition as potential carcinogens. Conducting additional studies using ex vivo and in vivo models may help bridge knowledge gaps regarding the effects of BPA, other EDCs, or their mixtures on carcinogenesis.
Research on EDCs in the male reproductive system
EDCs negatively affect the female reproductive system through various mechanisms including, but not limited to, the regulation of the hypothalamic-pituitary-ovarian axis. Similarly, the male reproductive function is primarily regulated by the hypothalamic-pituitary-testicular axis. EDCs affect hypothalamus that stimulates pituitary gland to secrete Gn, which regulate spermatogenesis through Leydig cell androgen secretion and Sertoli cell activation. EDCs can also negatively impact the function of the male reproductive organs, particularly the testis, which is crucial for spermatogenesis and male hormone secretion. EDCs can affect spermatogenesis, compromise sperm quality, and contribute to the onset of Testicular Dysgenesis Syndrome (TDS). TDS encompasses conditions like cryptorchidism, hypospadias, and testicular changes that can increase the risk of developing cancer. Importantly, more and more evidence showed that EDCs, such as BPA, DES, PCB, and pesticides, can also be involved in the development of TDS and other male reproductive system-related disorders, even cancer, posing a substantial threat to male reproductive health. A comprehensive overview of common EDCs related to male reproductive system diseases is shown in Figure 3.
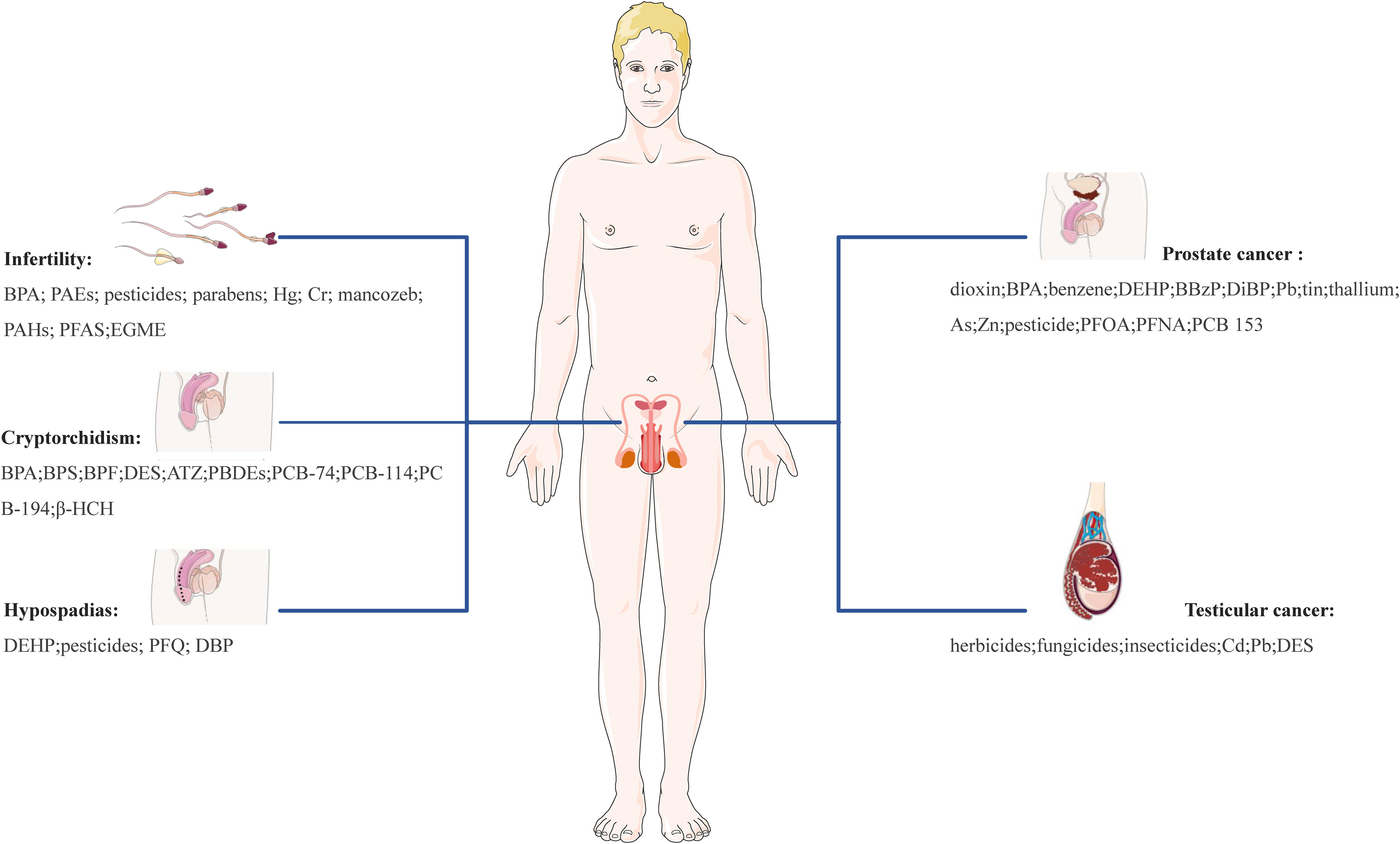
Figure 3 Effect of EDCs on male reproductive health. EGME, ethylene glycol monomethyl ether; ATZ, atrazine; PBDEs, polybrominated diphenyl ethers; β-HCH, β-hexachlorocyclohexane; PFQ, pyrifluquinazon; DBP, dibutyl phthalate; PFNA, perfluorononanoic acid.
EDCs damage semen quality and lead to male infertility
Infertility affects approximately 15% of the global population, with male factors responsible for 40–50% of these cases (265). The increasing incidence of male infertility can be attributed to various factors, including hormonal imbalances, anatomical causes, sexually transmitted diseases, genetic, environmental and lifestyle factors (266, 267). While the mechanisms leading to male infertility are not fully understood, changes in hormonal balance, particularly during sperm development, may impact sperm quality (268). Since the hypothalamic-pituitary-testicular tightly controls male reproductive system, EDCs acting as anti-androgens or mimicking estrogens may interfere with these mechanisms and have a profound impact on spermatogenesis. Experimental evidence suggests that increased exposure to EDCs may contribute to the decline in sperm counts, as well as impaired sperm motility and morphology (8, 269–271). Furthermore, the analysis of semen parameters, such as sperm count, motility, and morphology, provides valuable insights into testicular function and serves as a tool in fertility evaluation.
BPA
BPA can have a negative impact on sperm quality and directly affect seminal plasma, thereby influencing the testicular environment (272). Studies using animal models and in vitro methods have revealed that exposure to BPA is linked to decreased sperm count, impaired sperm motility and velocity, reduced epididymis weight, impaired insulin signaling and glucose regulation, and lower levels of Gn, testicular steroids, and testosterone in the serum (273–275). A study involving 74 and 83 male workers from factories with and without occupational exposure found that increased BPA exposure was associated with higher hydroxymethylation levels, suggesting potential adverse effects on male fertility (276). Furthermore, studies on reproductive-aged Chinese men have shown an association between BPA and altered serum hormone levels, such as low testosterone levels, ultimately leading to reduced male fertility (277, 278). Low testosterone levels are a major cause of male infertility, as this hormone is crucial for most processes throughout the entire male reproductive tract. However, a study involving 146 couples undergoing in vitro fertilization found that BPA concentrations did not significantly impact sperm concentration or motility parameters (279). Similarly, no statistically significant association was found between BPA concentration in the urine and parameters of seminal fluid (280, 281).
The correlations between BPA exposure and fertility in different populations of males have been investigated, but the results remain contradictory and inconclusive (282–284). The inconsistent findings are possibly due to the variations in BPA exposure levels. While male infertility cannot solely be attributed to BPA exposure, higher concentrations of BPA may contribute to infertility (285). Additionally, in epoxy resin workers occupationally exposed to BPA, alterations of sperm LINE-1 hydroxymethylation were observed (286). Furthermore, a Chinese cohort study found that hypermethylation of LINE-1 was significantly associated with tobacco smoking in men at risk of infertility (287). Therefore, daily exposure to BPA through lifestyle factors such as smoking can regulate male hormones through the HPG axis or damage sperm quality through epigenetic modifications, potentially leading to male infertility. In the future, multiple sample studies should be conducted under standardized BPA exposure levels, and men should be encouraged to develop healthy lifestyles, such as quitting smoking, to avoid unnecessary BPA exposure as much as possible.
PAEs
The serum levels of PAE metabolites have been identified as a reliable predictor for male infertility (288. Numerous studies have consistently reported a positive association between urinary levels of PAEs and at least one semen parameter indicative of low semen quality, with some studies also indicating sperm DNA damage (289). Paternal mixtures of urinary concentrations of DEHP have been relevant to higher infertility treatment failure (179), providing compelling evidence of the link between PAE metabolite levels and decreased sperm quality. Additionally, environmentally relevant concentrations of specific PAEs in seminal plasma have been linked with reduced semen volume, sperm motility, viability, and morphological alterations in sperm heads, resulting in semen volume and sperm viability falling below reference values (290).
Moreover, mechanistic research suggests that sperm protamine serves as a molecular biomarker during spermatozoa apoptotic processes, potentially mediating the association between PAE exposure and spermatozoa apoptosis (291). Furthermore, lipophilic phthalic acid esters have been found to impair the human sperm acrosomal reaction likely through the inhibition of the phospholipase A2-signaling pathway (292). MEHP has been shown to induce telomere structure and function disorder, mediating cell cycle dysregulation and apoptosis through c-Myc and its upstream transcription factors in a mouse spermatogonia-derived cell line (293). In addition, chronic dietary administration of lower levels of diethyl phthalate has been observed to induce murine testicular germ cell inflammation and sperm pathologies, highlighting the undesirable male reproductive outcomes following chronic DEP exposure (294). In conclusion, the levels of PAEs and their metabolites in serum, urine, and seminal plasma are closely related to sperm parameters. Detecting PAEs in infertile men may clarify the mechanisms of PAEs causing sperm toxicity and provide credible evidence that PAEs could pose a serious threat to male infertility by inducing sperm apoptosis in the future.
Pesticides
Evidence from one research suggests that serum concentrations of multiple OCPs may be correlated with altered serum testosterone levels, indicating potential adverse effects of pesticide exposure on male reproductive health (295). Numerous studies consistently demonstrate associations between pesticide exposure and compromised sperm parameters, particularly sperm motility and sperm DNA integrity (296). Notably, ancestral exposure to p,p’-DDE has been linked to impaired testis histology, decreased the sperm fertility in the F1 generation, and transgenerational sperm DNA hypomethylation of paternally imprinted genes (297). DDE, a metabolite of organochlorine insecticide DDT, has been shown to have anti-androgenic effects. In a cross-sectional study of Chinese men, higher levels of serum γ-hexachlorobenzene (γ-HCH), a seed mixing and sterilization agent, were correlated with decreased sperm motility, and this association remained statistically significant even after adjusting for multiple testing (298). Further research confirms that HCH impairs human sperm motility by affecting lysine glutarylation and mitochondrial functions (299). Moreover, a human investigation reported that urine concentrations of pyrethroid metabolites were linked to elevated rates of sperm aneuploidy (300, 301). Exposure to organophosphate pesticides has also been found to decrease sperm count, concentration, total and progressive motility, and normal sperm morphology, potentially via a testosterone-independent mechanism (302).
Extensive in vitro and in vivo studies have been conducted to investigate the effects and potential mechanisms of pesticides on the male reproductive system, uncovering a spectrum of negative impacts that may ultimately culminate in infertility (303). Widely used globally, carbamates have been found to disrupt various signaling pathways, such as those mediated by acetylcholine or kisspeptin, compromising steroidogenesis and spermatogenesis (304). The benzoylurea pesticide Lufenuron has been associated with significant histological and histochemical damage in mammals, resulting in impaired testicular function and subsequent infertility (305). Additionally, dimethoate (DM), a prominent organophosphate insecticide used for pest control in vegetables, fruits, and agricultural crops, has been found to have detrimental effects on sperm morphology, fertility, and embryos, posing potential health risks to humans and non-target organisms due to its persistence in soils and crops (306). Furthermore, amitraz (AMZ), a formamidine pesticide widely used as an insecticide and acaricide, has been shown to induce alterations in bovine sperm, potentially affecting male fertility at concentrations found in the environment (307). Similarly, exposure to other pesticides such as diazinon (DZN) (308), pyridaben (PDB) (309), bifenthrin (BF) (310), flurochloridone (FLC) (311), fipronil (FPN) (312), etoxazole (ETX) (313), imidacloprid (IMI) (314, 315), atrazine (ATR) (316, 317), cypermethrin (CYP) (314, 318), chlorpyrifos (CPF) (314, 319–322), chlorpyrifos (323, 324), simazine (325), endosulfan (191), and deltamethrin (318) could lead to a significant decrease in sperm count, motility, normality, vitality, and an increase in deformity rates. Moreover, a significant decrease in daily sperm production, epididymal sperm count, motile, viable, and hypo-osmotic swelling-tail swelled sperm was observed in mancozeb-treated rats (326). Mancozeb, a contact fungicide and a polymeric complex of manganese and zinc salts of ethylene-bis-dithiocarbamate, is linked to histological alterations in the testis and a decrease in testosterone biosynthesis. Additionally, piperonyl butoxide (PBO), a semi-synthetic methylenedioxyphenyl compound, is commonly used as a synergist to enhance the insecticidal effect of pesticides for agricultural and household use. Studies have indicated that PBO considerably reduces sperm motility, kinematics, and acrosome-reacted and capacitated spermatozoa (327). The use of the pesticides and their synergists may directly or indirectly cause disorders in male fertility. Furthermore, exposure to these pesticides can trigger inflammation, mitochondrial defects, DNA damage, and epigenetic gene methylation, resulting in toxic and pathological effects on cells related to the reproductive system and causing lasting and serious consequences for reproductive organs and male reproductive health. Therefore, greater efforts are warranted to protect ourselves and our environment from the detrimental effects of these pesticides.
The understanding of the potential molecular mechanism of pesticide-induced toxicity on sperm provides a theoretical basis for the future treatment of male infertility. The findings unequivocally demonstrate that pesticides can have detrimental effects on semen quality, which in turn impairs the normal functioning of male reproductive function. Moreover, a study examining the effects of environmental pyrethroids on sperm morphology in men with oligozoospermia further consolidates this point (328, 329). Additionally, epidemiological evidence supports the correlation between pesticides and male fertility, particularly in relation to semen quality, DNA fragmentation, and chromosome aneuploidy among workers and the exposed population (330). It is hypothesized that pesticides may impact fertility by directly affecting spermatogenesis through reduced antioxidant capacity, impairment of testicular production of testosterone, systemic alterations in testosterone metabolism, mitochondrial dysfunction, or by influencing the HPG axis to steroidogenesis and inducing changes in epigenetic modifications.
Other EDCs
In various in vitro and in vivo studies, parabens have been associated with weak estrogenic and anti-androgenic actions (331). One study demonstrated that paraben use during pregnancy results in significant changes in the mitochondrial bioenergetics and antioxidant capacity of testicular germ cells, as well as the antioxidant capacity of several F1 generation organs, contributing to the understanding of male infertility in future generations (332). Parabens, especially butyl, can affect the enzymatic antioxidant system in rat testes, leading to a decrease in cellular antioxidant capacity, which may be related to male infertility (333). However, a couple-based prospective cohort study showed paraben exposure in male partners was not associated with fecundity (203).
Heavy metals cause toxicity by impacting the HPG axis, testicular function, spermatogenesis and sperm processing. Higher levels of Hg are associated with decreased semen quality and higher levels of DNA damage in men and lead to lower fertility and higher spontaneous abortion rates (334). Cr III, classified as an endocrine-disrupting chemical, and its compound Cr III picolinate (CrPic3), impact male fertility by altering reactive oxygen species, testosterone levels, and sperm parameters, such as sperm motility and abnormal sperm count (335).
A meta-analysis of 272 occupationally exposed men and 247 unexposed men found a significant decrease in sperm motility in participants occupationally exposed to high levels of solvents (336). Full-time firefighters have been reported to be at increased risk of infertility due to their occupational exposure to several endocrine-disrupting solvents, such as flame retardants and PAHs (337). Of special interest is their exposure to PFAS as the main constituent of aqueous film-forming firefighting foams (338). A human stem cell-based model of spermatogenesis showed that PFAS lower the expression of markers for spermatogonia and primary spermatocytes without directly affecting germ cell viability, suggesting potential long-term effects on male fertility through the depletion of the spermatogonial stem cell pool and abnormalities in primary spermatocytes (339). Additionally, ethylene glycol monomethyl ether (EGME) reduced the expression of spermatocyte-specific genes in rats and mice (340). And ethylene glycol-based chemicals are commonly used as solvents, antifreeze agents, and coolants in commercial and industrial applications.
Epidemiological studies have revealed an increase in fertility disorders, characterized by a higher incidence of lower sperm quality parameters and a greater occurrence of cryptorchidism and hypospadias (341, 342). However, the regulation of spermatogenesis involves numerous and complex underlying biochemical processes that contribute to its dysfunction, including the developmental age of exposure, short- and long-term effects, dosage methods, and dose-related responses. While these EDCs may result in impairments in semen parameters, irrefutable evidence linking these EDCs to the known male fertility decline is elusive. This is partly due to the complexity of conducting longitudinal studies needed to establish causality and the ethical concerns associated with conducting prospective clinical trials involving human exposures. Furthermore, only a limited number of human studies have been conducted to assess the potential long-term or epigenetic effects of low-dose exposures on future generations. Given the current gap in understanding how the environment affects male fertility, it is imperative to minimize exposure to these EDCs and, where feasible, implement prospective cohort studies to clarify the adverse effects on male reproductive health.
EDCs affect testicular descent into the scrotal position leading to cryptorchidism
Cryptorchidism, a condition characterized by undescended testes, can significantly impair germ cells and reduce fertility, while also increasing the risk of testicular torsion, hernia, and malignancy. Moreover, it has been a recognized maternal and perinatal risk factor, which can lead to premature birth, low birth weight, and developmental delay of the fetus, thus affecting the growth and development of newborn males.
One prospective analysis revealed a positive association between levels of Bisphenol A (BPA) in maternal sera, placenta, and sera from boys with cryptorchidism. Specifically, higher concentrations of maternal serum BPA during 10-17 weeks of gestation were linked to an increased incidence of cryptorchidism in offspring (343). Additionally, a study focusing on children with cryptorchidism highlighted significantly elevated plasma levels of BPA, BPS, and BPF relative to control subjects (344). Additionally, a retrospective analysis found a heightened occurrence of cryptorchidism in sons of men who were exposed to DES during prenatal development. The transgenerational effects of DES were observed in animal studies as well as in the offspring of women exposed to DES (345). Furthermore, prenatal exposure to environmentally relevant levels of PBDEs leads to testicular dysgenesis in male offspring, which is characterized by decreased anogenital index and testicular organ coefficient, increased incidence of cryptorchidism (346). Moreover, prenatal exposure to atrazine (ATZ), an herbicide used for preventing broadleaf weeds in crops and turf grasses, was shown to induce cryptorchidism and hypospadias in male mouse offspring, potentially through the downregulation of insulin-like 3 (347). In the Norwegian HUMIS study, exposure to PCB-74, PCB-114, PCB-194, and the estrogenic organochlorine pesticide β-hexachlorocyclohexane (β-HCH) may also increase the risk of cryptorchidism (348). However, in the French West, there was no observed association between maternal or cord plasma concentration of Chlordecone, an organochlorine pesticide, and the risk of overall malformations or undescended testes (349). Cryptorchidism displayed spatial heterogeneity, with the main cluster in an area of former coal mining and metallurgic industry in northern France, suggesting environmental factors associated with higher incidence (350). Nevertheless, the research results on the relationship between EDCs and cryptorchidism are not consistent. Other epidemiological studies have found no associations between in-utero exposure to PCBs, dioxins, or HCB and cryptorchidism (351).
Several studies noted that some EDCs pass across the placenta in humans throughout pregnancy and different levels of bioaccumulation depending on the fetal organ. Male fetuses appear to be more susceptible to the effects of EDCs compared to female fetuses. While a correlation exists between the occurrence of cryptorchidism and EDCs, a significant association between EDC exposure and the incidence of cryptorchidism has not been definitively established. Consequently, it is crucial to comprehensively evaluate the reproductive toxicity of environmental endocrine disruptors. Further studies are required to explore these specific issues.
EDCs interfere with the development of male genitals leading to hypospadias
Hypospadias is one of the most common congenital malformations in the urinary system of children, yet its etiology remains unknown. It is characterized by the urethral opening developing on the ventral side of the penis. Xenobiotic chemicals, including certain drugs and environmental contaminants, especially EDCs, are believed to be the primary factors contributing to the development of hypospadias.
The observation of the intervention of DEHP in female SD rats found that DEHP significantly alters the oxidative balance in male fetal, inducing cryptorchidism and hypospadias by reducing oxidative stress (352). Most retrospective studies reported parental occupational exposure to pesticides was associated with at least a twofold increased risk of hypospadias (353, 354). The VHEMBE birth cohort study examined levels of EDCs in maternal perinatal serum and urine and further correlated this data with the genitourinary examination results of boys in their offspring. The study found that higher prenatal exposure to pyrethroid insecticides was linked to an elevated risk of hypospadias (355). Exposure of pregnant rats to the pesticide pyrifluquinazon (PFQ) and dibutyl phthalate (DBP) during sexual differentiation of the reproductive tract disrupted the androgen signaling pathway and induced hypospadias (356). Nevertheless, slightly increased risks of cryptorchidism were observed in association with all crop acreages near homes during pregnancy, especially for orchards, and no association was observed for hypospadias in French (357). So, residential exposure to pesticide-active ingredients has shown diverse patterns of association with birth defects. Likewise, a specific study investigating the presence of EDCs in breast milk samples of mothers with and without hypospadias-affected baby boys did not find any conclusive evidence linking hypospadias with exposure to POPs (358).
Therefore, it is evident that there are remaining challenges in comprehensively understanding the impact of environmental factors on the occurrence of hypospadias. A vast majority of research claims that being heavily exposed to agricultural pesticides occupies an important position in the pathogenesis of hypospadias, despite the limitation of modest case numbers. These discrepancies could be attributed to individual variations in the absorption, metabolism, or distribution of EDCs. Nonetheless, these studies do provide valuable information on the quantification of exposures. To comprehensively assess the potential combined effects of multiple EDC exposures, the development of multi-exposure models is warranted.
EDCs improve the carcinogenic effect of prostate cancer (PC)
The development and maintenance of the prostate gland, like the testes, rely on the local activity of androgens in the body and the regulation of the HPG axis. EDCs produce reproductive toxicity by interfering with the normal function of male hormones. The prostate grows very slowly after sexual maturity and usually causes symptoms only in the severe advanced stage, such as PC. PC, a hormone-dependent cancer, has emerged as a serious global health concern, significantly impacting men’s well-being (359). EDCs represent a major environmental and health issue, as they can disrupt normal prostate functions, stimulate PC growth, and impair immune and neuronal systems.
There are currently some EDCs that can be considered carcinogenic, like dioxin for PC (360). Chronic low-dose exposure to BPA throughout life has been associated with an increased risk of prostate disease. This can occur through interference with genes involved in intraprostatic androgen and estrogen production, as well as other genes implicated in PC development (361–363). Epigenetic markers, such as DNA methylation and histone modifications, may also contribute to these effects (364). BPS, an alternative to BPA, has been found to contribute to the progression of PC by regulating the expression of genes COL1A1 and COL1A2 (365). Furthermore, benzene, a volatile organic compound found in petroleum and crude oil products, has been found to increase the odds of developing PC two-fold, even at low ambient concentrations (366). A forty-year systematic review supports that the most tumorigenic EDC groups were PAEs, heavy metals, and pesticides (367). In a study involving 80 PC patients, frequently detected metabolites of PAEs, such as DEHP, DiBP, and butyl-benzyl (BBzP), were quantified in urine samples using liquid chromatography or electrospray ionization tandem mass spectrometry. The results demonstrated an association between exposure to these metabolites and the occurrence of PC, particularly in abdominally obese men (368). Another exploratory case-control study examining heavy metal toxicities in benign PC estimated Pb and Cd levels using atomic absorption spectrophotometry, demonstrating an increased risk of PC associated with heavy metal toxicity (369). Furthermore, blood cadmium levels exhibited an inverse association with PC, while blood lead, urinary tin, and thallium levels exhibited positive associations (370). A multiple metal analysis about the association between serum heavy metals and PC disclosed that the serum levels of As and Zn increased the risk of PC (371). The risks posed by exposure to these metals are particularly pronounced in PC patients compared to other EDCs. Another study examined the combined effects of pesticides and established PC loci, providing evidence of potential mechanisms through which pesticides may heighten the risk of PC (372). A prospective nested case-control study of serum concentrations of each of the PFAS and the risk of aggressive PC shows that serum concentrations of PFAS did not establish an association with an increased risk of aggressive PC (373). Despite this, in vitro experiments verified the promotive role of PFOA and perfluorononanoic acid (PFNA) in the growth of prostate cancer cells (374). Additionally, the cytotoxicity and proliferation of a prostate cancer cell line exposed to various dilutions of PFOA and PCB 153 were monitored, and the results suggest that even at picomolar concentrations, PFOA and PCB153 can increase the proliferation of PC (375).
The aforementioned research provided novel insights into understanding the role of some EDCs in PC and brought attention to the environmental association with male reproductive system cancer risks and progression. Multiple findings have highlighted that even low-concentration exposure to various types of EDCs can induce distinct effects on PC cells, emphasizing the importance of investigating the impact of EDC compounds across all stages of the disease. The disruption of HPG axis secretion arising from EDCs exposure may represent a significant step in the aberrant development leading to PC, while the combined effects of EDC mixtures augment the risk of PC beyond that attributed to individual EDC exposures. Consequently, more prospective studies should compensate for the limitations of mechanistic experiments and incorporate comparable toxicological data.
EDCs disrupt the homeostasis of testicular cells leading to testicular cancer (TC)
TC is one of the most common malignancies in young men though the pathogenesis is not completely clear. Cryptorchidism, hypospadias, oligospermia, genetic factors, and EDCs are recognized as high-risk factors for TC. The dysfunction of HPG axis caused by EDCs, resulting in the change of male hormone levels, is the direct cause of cryptorchidism malignant transformation, and may also be the cause of TC. Findings from a systematic review and meta-analysis consistently demonstrate that maternal EDCs exposure, as opposed to postnatal exposure in adult males, is associated with an increased risk of TC (5).
Emerging evidence supported the link between male reproductive system diseases and tumors, especially male infertile and TC. Epidemiology has confirmed the likelihood of illness of TC has increased by more than 60% in infertile men (376). Furthermore, EDCs such as PFOS negatively impact testis-specific biomarkers insulin-like peptide 3 and androstenedione (377). Glyphosate formulations are widely used as a non-selective systemic herbicide and have been shown to affect testicular weight in male guinea pigs, severely impairing testicular growth performance and inducing reproductive toxicity (378). A 20-year ecological study of TC carried out in Brazil manifested that fungicides and insecticides increased age-standardized mortality rates (379). Long-term exposure to Cd and Pb compounds, known to induce acute reproductive toxicity (380), has been associated with significantly higher blood Cd levels in patients with TC, highlighting the potential role of Cd as an important parameter in TC prediction (381). Animal experiments have also demonstrated that the severity of testes injury increases with increasing Cd concentration, which can potentially impact testosterone synthesis (382). In another study, neonatal exposure to EDCs resulted in infertility and testicular tumors in 65% of DES-treated mice (383). Moreover, studies on the mechanism by which EDCs promote the development of TC cells have shown that the connection and non-connection functions of Cx43 and MAPK signaling pathways may be involved in the process of EDCs disrupting the balance of TC cell growth in vivo (384).
Although only certain studies have reported an increased risk for TC upon EDCs exposure and the mechanism of action is still ambiguous, the role of EDCs as a threat to male reproductive health cannot be ignored. Exposure to increased EDCs can disrupt androgen expression, disrupt hormone receptor binding, lead to HPG axis imbalance, interfere with steroidogenesis and hormonal metabolism, or modify epigenetic mechanisms, all of which can lead to TDS and other reproductive system cancer diseases. The chronic exposition of cancer cells to EDCs should be considered as a potential obstacle in cancer therapy as many studies showed that exposure to these compounds promotes radio- and chemo-resistance. Despite ongoing research, the precise action modes of certain EDCs in hormone-sensitive cancers and their effects on tumor growth and metastases, particularly in humans, remain poorly understood. Given the wide prevalence and extensive use of EDCs, continued assessment of their long-term reproductive health effects, particularly in carcinogenesis, is essential to eradicate the worst of them and to sensitize the population to minimize their use.
Conclusion and future prospective
The global health impact of EDCs has become a major public problem, initially and primarily focused on their actions through the HPG axis to induce hormone-like effects. However, research now clearly demonstrates that the mechanisms of action of EDCs are far more diverse. The mechanisms by which EDCs mediate specific cellular functions (e.g., cell growth, proliferation, differentiation, apoptosis and carcinogenesis) lead to the occurrence and development of the above-mentioned reproductive system-related diseases through various common pathways. These pathways involve the genomic regulation of target gene transcription, the non-genomic rapid signal transduction mediated by membrane-bound ERs or other receptors, the autocrine or paracrine signaling pathways involving growth factors and cytokines, and epigenetic alterations majorly in the form of DNA methylation, histone modifications (Figure 4). This broader understanding of their actions not only implicates reproductive toxicity but also highlights their potent carcinogenic and cancer-promoting effects. EDCs exist in various forms and are widespread in numerous products. Certain substances can enter the body through inhalation and dietary intake, exerting impacts on the reproductive system and significantly affecting reproductive health, potentially leading to UFs, EMs, PCOS, DOR, POI, infertile, BC, EC, OC, CC, cryptorchidism, hypospadias, PC and TC. In light of the recent reclassification of certain EDCs as human carcinogens, further investigation into the association between the aforementioned outcomes and reproductive system diseases should be conducted with larger sample sizes.
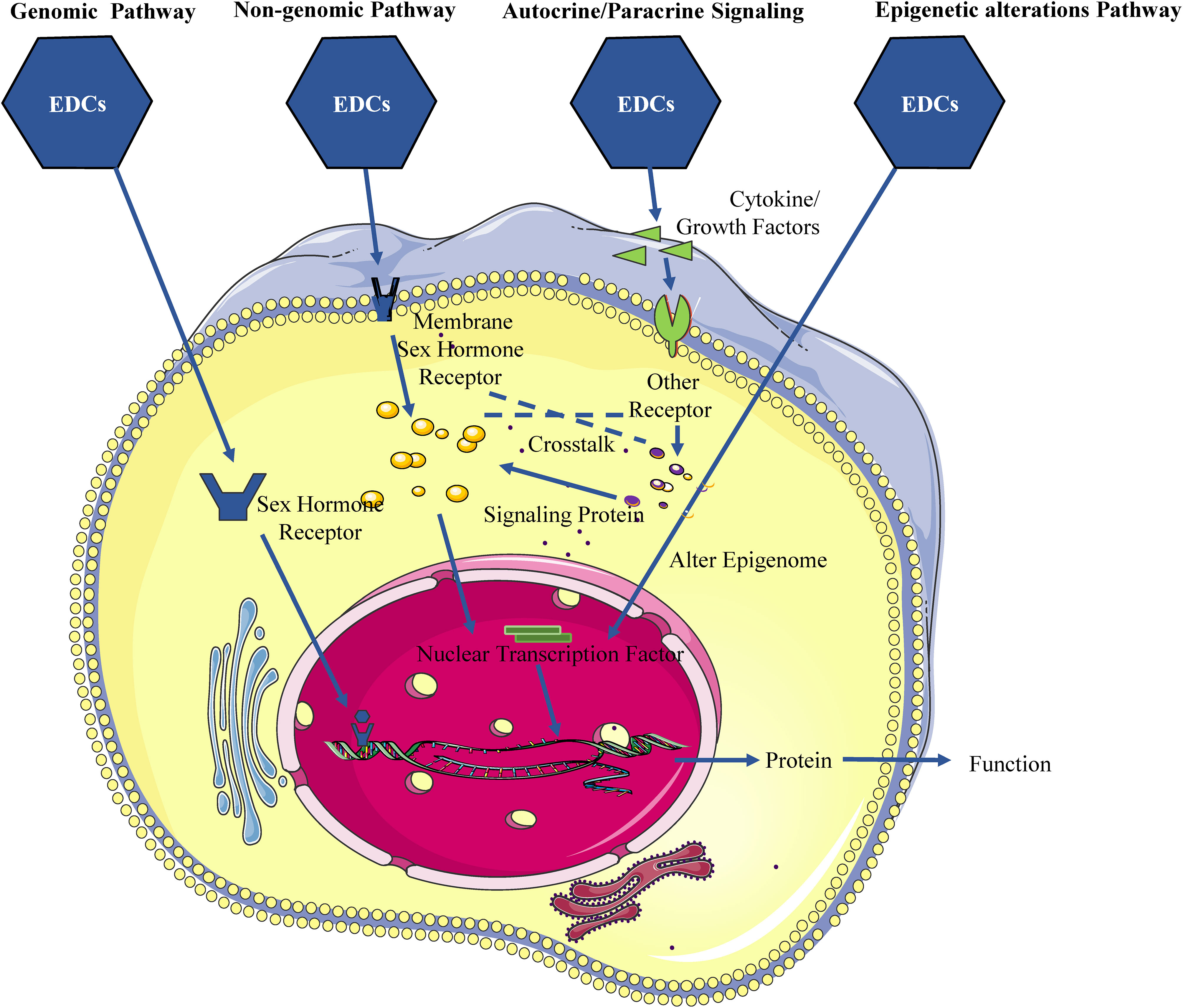
Figure 4 Molecular mechanisms of EDCs-genomic pathways, non-genomic pathways, autocrine/paracrine signaling and epigenetic alterations.
Supplementary Table 1 provides detailed information on studies that demonstrate the association and mechanism of action between EDCs exposure and reproductive health. The complex feedback mechanisms involved in the hormone-like or anti-hormone-like activity of EDCs can be detected through various methods, such as ligand binding assay, transcriptional assay, protein assay, cell assay, animal assay, and signal pathway analysis. As a result, differences arise in defining pathogenic concentration dependence, agonist or antagonist properties, and ligand or pathway responses, leading to potentially contradictory research findings regarding the same EDCs. A quick and cost-effective solution for preliminary hazard assessment of chemicals that do not undergo animal testing, to identify which chemicals can act as an EDC is a significant advantage and a practical necessity.
Notwithstanding the fact that multiple social and structural determinants of health contribute to the marked racial-, ethnic-, and gender-based disparities in endocrine, EDCs exposure undoubtedly generally tend to accumulate and resist degradation, ampling the risk of endocrine diseases and reducing the quality of life. Vulnerable populations carry a disproportionate burden of multiple reproductive disorders and their associated comorbidities and medical costs. Addressing these concerns will necessitate political determination to develop and implement different remediation policies based on different degrees of degradation levels to limit the use of these harmful EDCs. The incorporate occupational and environmental history-taking into medical practice would facilitate the diagnosis and treatment of reproductive system diseases. Nonetheless, implementing personalized education programs, modifying unhealthy dietary habits, choosing fresh food instead of processed food, washing fruits and vegetables to remove residual pesticides, avoiding heating or microwaving food in plastic containers, minimizing exposure to EDC chemicals, and taking relevant protective measures in occupations with high exposure are fundamental strategies to reduce the risk of diseases related to the reproductive system. These measures are crucial in minimizing exposure during the development of female pregnancy and male sexual organs, preventing the accumulation of EDCs in the body, and mitigating the genetic effects of intergenerational relationships.
The existing research on EDCs and their effects on reproductive health still has several areas for improvement. The aforementioned studies have several limitations, primarily stemming from their retrospective nature and lack of specific focus on investigating the association between EDCs half-life and reproductive system diseases. To comprehensively characterize the effects of individual exposures and their combinations, future research should aim to collect multiple exposure data and consider the presence of EDC mixtures, as stronger associations have been observed with the development of cancer for mixtures compared to individual chemicals (385). Additionally, efforts should be directed toward identifying potential etiologic agents or mixtures of compounds that may act synergistically or through specific mechanistic pathways. It is crucial to capture exposure data during critical developmental periods to shed light on the relationship between exposure and disease, with a particular emphasis on precaution, prevention, and potential multigenerational effects. Indeed, in different species and at different times of the life cycle, an EDC can have different effects on different cell or tissue types. Large-scale, well-designed longitudinal prospective research is needed to gather information from diverse populations worldwide and conduct more robust epidemiological studies to confirm the mechanisms of action and the importance of critical exposure windows, in order to fully comprehend the impact of EDCs on reproductive health.
Author contributions
JP: Writing – original draft. PL: Investigation, Writing – review & editing. XY: Investigation, Writing – review & editing. ZZ: Writing – review & editing. JL: Conceptualization, Writing – review & editing.
Funding
The author(s) declare financial support was received for the research, authorship, and/or publication of this article. This research was supported by the Shandong Provincial Natural Science Foundation of China (ZR2021MH079).
Conflict of interest
The authors declare that the research was conducted in the absence of any commercial or financial relationships that could be construed as a potential conflict of interest.
Publisher’s note
All claims expressed in this article are solely those of the authors and do not necessarily represent those of their affiliated organizations, or those of the publisher, the editors and the reviewers. Any product that may be evaluated in this article, or claim that may be made by its manufacturer, is not guaranteed or endorsed by the publisher.
Supplementary material
The Supplementary Material for this article can be found online at: https://www.frontiersin.org/articles/10.3389/fendo.2023.1324993/full#supplementary-material
Abbreviations
UFs, uterine fibroids; EMs, endometriosis; PCOS, polycystic ovary syndrome; DOR, diminished ovarian reserve; POI, premature ovarian insufficiency; EC, infertility, endometrial cancer; OC, ovarian cancer; CC, cervical cancer; PC, prostate cancer; TC, testicular cancer; BPA,bisphenol A; NPH, nonylphenol; PAEs, phthalates; Cd, cadmium; Hg, mercury; DES, diethylstilbestrol; BPAF, bisphenol AF; MEHHP, mono-(2-ethyl-5-hydroxyhexyl) phthalate; DEHP, di(2-ethylhexyl)phthalate; MnBP, mono-n-butyl phthalate; BBP, n-butylbenzyl phthalate; PCBs, polychlorinated biphenyls; OCPs, organochlorine pesticides; PFOS, perfluorooctane sulphonate; PFOA, perfluorooctanoic acid; HCB, hexachlorobenzene; TBT, tributyltin; MEHP, mono(2-ethylhexyl) phthalate; MBzP, monobenzyl phthalate; DDT, dichlorodiphenyltrichloroethane; PFAS, perfluorinated substances; PP, propylparaben; PAHs, polycyclic aromatic hydrocarbons; 3-PBA, 3-phenoxybenoic acid; TCS, triclosan; DDE, p,p’-dichlorodiphenyldichloroethylene; BP4, benzophenone-4; CYP, cypermethrin; LCT, lambda-cyhalothrin; VCD, 4-vinylcyclohexenediepoxide; TCDD, 2,3,7,8-tetrachlorodibenzo-p-dioxin; DINP, diisononyl phthalate; As, arsenic; Pb, lead; Cr, hexavalent chromium; Zn, zinc; MiBP, monoisobutyl phthalate; APEOs, alkylphenol ethoxylates; BP-1, benzophenone-1; EGME, ethylene glycol monomethyl ether; ATZ, atrazine; PBDEs, polybrominated diphenyl ethers; β-HCH, β-hexachlorocyclohexane; PFQ, pyrifluquinazon; DBP, dibutyl phthalate; PFNA, perfluorononanoic acid.
References
1. Xue B, Zhang Z, Beltz TG, Johnson RF, Guo F, Hay M, et al. Estrogen receptor-β in the paraventricular nucleus and rostroventrolateral medulla plays an essential protective role in aldosterone/salt-induced hypertension in female rats. Hypertension (2013) 61:1255–62. doi: 10.1161/HYPERTENSIONAHA.111.00903
2. Xue B, Zhang Z, Beltz TG, Guo F, Hay M, Johnson AK. Estrogen regulation of the brain renin-angiotensin system in protection against angiotensin II-induced sensitization of hypertension. Am J Physiol Heart Circ Physiol (2014) 307:H191–8. doi: 10.1152/ajpheart.01012.2013
3. Xue B, Zhang Z, Beltz TG, Guo F, Hay M, Johnson AK. Genetic knockdown of estrogen receptor-alpha in the subfornical organ augments ANG II-induced hypertension in female mice. Am J Physiol Regul Integr Comp Physiol (2015) 308:R507–16. doi: 10.1152/ajpregu.00406.2014
4. Yilmaz B, Terekeci H, Sandal S, Kelestimur F. Endocrine disrupting chemicals: exposure, effects on human health, mechanism of action, models for testing and strategies for prevention. Rev Endocr Metab Disord (2020) 21:127–47. doi: 10.1007/s11154-019-09521-z
5. Bräuner EV, Lim YH, Koch T, Uldbjerg CS, Gregersen LS, Pedersen MK, et al. Endocrine disrupting chemicals and risk of testicular cancer: A systematic review and meta-analysis. J Clin Endocrinol Metab (2021) 106:e4834–60. doi: 10.1210/clinem/dgab523
6. Lin Y, An R, Wu C, Liu H, Deng J, Tan H, et al. Serum microcystin-LR levels and risk of gestational diabetes mellitus: A Chinese nested case-control study. Front Endocrinol (Lausanne) (2022) 13:1047866. doi: 10.3389/fendo.2022.1047866
7. Corpuz-Hilsabeck M, Culty M. Impact of endocrine disrupting chemicals and pharmaceuticals on Sertoli cell development and functions. Front Endocrinol (Lausanne) (2023) 14:1095894. doi: 10.3389/fendo.2023.1095894
8. Kahn LG, Philippat C, Nakayama SF, Slama R, Trasande L. Endocrine-disrupting chemicals: implications for human health. Lancet Diabetes Endocrinol (2020) 8:703–18. doi: 10.1016/S2213-8587(20)30129-7
9. Czarnywojtek A, Borowska M, Dyrka K, Moskal J, Kościński J, Krela-Kaźmierczak I, et al. The influence of various endocrine disruptors on the reproductive system. Endokrynol Pol (2023) 74:221–33. doi: 10.5603/EP.a2023.0034
10. Lei B, Yang Y, Xu L, Zhang X, Yu M, Yu J, et al. Molecular insights into the effects of tetrachlorobisphenol A on puberty initiation in Wistar rats. Sci Total Environ (2023) 911:168643. doi: 10.1016/j.scitotenv.2023.168643
11. Xie Q, Kang Y, Zhang C, Xie Y, Wang C, Liu J, et al. The role of kisspeptin in the control of the hypothalamic-pituitary-gonadal axis and reproduction. Front Endocrinol (Lausanne) (2022) 13:925206. doi: 10.3389/fendo.2022.925206
12. Koysombat K, Dhillo WS, Abbara A. Assessing hypothalamic pituitary gonadal function in reproductive disorders. Clin Sci (Lond) (2023) 137:863–79. doi: 10.1042/CS20220146
13. Rubin BS. Bisphenol A: an endocrine disruptor with widespread exposure and multiple effects. J Steroid Biochem Mol Biol (2011) 127:27–34. doi: 10.1016/j.jsbmb.2011.05.002
14. Konieczna A, Rutkowska A, Rachoń D. Health risk of exposure to Bisphenol A (BPA). Rocz Panstw Zakl Hig (2015) 66:5–11.
15. Chen D, Kannan K, Tan H, Zheng Z, Feng YL, Wu Y, et al. Bisphenol analogues other than BPA: environmental occurrence, human exposure, and toxicity-A review. Environ Sci Technol (2016) 50:5438–53. doi: 10.1021/acs.est.5b05387
16. Lehmler HJ, Liu B, Gadogbe M, Bao W. Exposure to bisphenol A, bisphenol F, and bisphenol S in U.S. Adults and children: the national health and nutrition examination survey. 2013-2014. ACS Omega (2018) 3:6523–32. doi: 10.1021/acsomega.8b00824
17. Vandenberg LN, Colborn T, Hayes TB, Heindel JJ, Jacobs DR Jr., Lee DH, et al. Hormones and endocrine-disrupting chemicals: low-dose effects and nonmonotonic dose responses. Endocr Rev (2012) 33:378–455. doi: 10.1210/er.2011-1050
18. Bethea TN, Wesselink AK, Weuve J, Mcclean MD, Hauser R, Williams PL, et al. Correlates of exposure to phenols, parabens, and triclocarban in the Study of Environment, Lifestyle and Fibroids. J Expo Sci Environ Epidemiol (2020) 30:117–36. doi: 10.1038/s41370-019-0114-9
19. Seli DA, Taylor HS. The impact of air pollution and endocrine disruptors on reproduction and assisted reproduction. Curr Opin Obstet Gynecol (2023) 35:210–5. doi: 10.1097/GCO.0000000000000868
20. Yu L, Das P, Vall AJ, Yan Y, Gao X, Sifre MI, et al. Bisphenol A induces human uterine leiomyoma cell proliferation through membrane-associated ERα36 via nongenomic signaling pathways. Mol Cell Endocrinol (2019) 484:59–68. doi: 10.1016/j.mce.2019.01.001
21. Li Z, Lu Q, Ding B, Xu J, Shen Y. Bisphenol A promotes the proliferation of leiomyoma cells by GPR30-EGFR signaling pathway. J Obstet Gynaecol Res (2019) 45:1277–85. doi: 10.1111/jog.13972
22. Shen Y, Lu Q, Zhang P, Wu Y, Ren M. The effect of TGF-β signaling on regulating proliferation of uterine leiomyoma cell via ERα signaling activated by bisphenol A, octylphenol and nonylphenol in vitro. J Cancer Res Ther (2018) 14:S276–s281. doi: 10.4103/0973-1482.235342
23. Li Z, Yin H, Shen Y, Ren M, Xu X. The influence of phenolic environmental estrogen on the transcriptome of uterine leiomyoma cells: A whole transcriptome profiling-based analysis. Ecotoxicol Environ Saf (2021) 211:111945. doi: 10.1016/j.ecoenv.2021.111945
24. Li Z, Yin H, Chen K, Ding B, Xu J, Ren M, et al. Effects of bisphenol A on uterine leiomyoma: In vitro and in vivo evaluation with mechanistic insights related to XBP1. Ecotoxicol Environ Saf (2022) 247:114201. doi: 10.1016/j.ecoenv.2022.114201
25. Wesselink AK, Weuve J, Fruh V, Bethea TN, Claus Henn B, Harmon QE, et al. Urinary concentrations of phenols, parabens, and triclocarban in relation to uterine leiomyomata incidence and growth. Fertil Steril (2021) 116:1590–600. doi: 10.1016/j.fertnstert.2021.07.003
26. Kawakami T, Isama K, Matsuoka A. Analysis of phthalic acid diesters, monoester, and other plasticizers in polyvinyl chloride household products in Japan. J Environ Sci Health A Tox Hazard Subst Environ Eng (2011) 46:855–64. doi: 10.1080/10934529.2011.579870
27. Grindler NM, Vanderlinden L, Karthikraj R, Kannan K, Teal S, Polotsky AJ, et al. Exposure to phthalate, an endocrine disrupting chemical, alters the first trimester placental methylome and transcriptome in women. Sci Rep (2018) 8:6086. doi: 10.1038/s41598-018-24505-w
28. Pacyga DC, Ryva BA, Nowak RA, Bulun SE, Yin P, Li Z, et al. Midlife urinary phthalate metabolite concentrations and prior uterine fibroid diagnosis. Int J Environ Res Public Health (2022) 19(5):2741. doi: 10.3390/ijerph19052741
29. Lee J, Jeong Y, Mok S, Choi K, Park J, Moon HB, et al. Associations of exposure to phthalates and environmental phenols with gynecological disorders. Reprod Toxicol (2020) 95:19–28. doi: 10.1016/j.reprotox.2020.04.076
30. Zota AR, Geller RJ, Calafat AM, Marfori CQ, Baccarelli AA, Moawad GN. Phthalates exposure and uterine fibroid burden among women undergoing surgical treatment for fibroids: a preliminary study. Fertil Steril (2019) 111:112–21. doi: 10.1016/j.fertnstert.2018.09.009
31. Kim HJ, Kim SH, Oh YS, Heo SH, Kim KH, Kim DY, et al. Effects of phthalate esters on human myometrial and fibroid cells: cell culture and NOD-SCID mouse data. Reprod Sci (2021) 28:479–87. doi: 10.1007/s43032-020-00341-0
32. Kim JH. Analysis of the in vitro effects of di-(2-ethylhexyl) phthalate exposure on human uterine leiomyoma cells. Exp Ther Med (2018) 15:4972–8. doi: 10.3892/etm.2018.6040
33. Iizuka T, Yin P, Zuberi A, Kujawa S, Coon JS5, Björvang RD, et al. Mono-(2-ethyl-5-hydroxyhexyl) phthalate promotes uterine leiomyoma cell survival through tryptophan-kynurenine-AHR pathway activation. Proc Natl Acad Sci U.S.A. (2022) 119:e2208886119. doi: 10.1073/pnas.2208886119
34. Zhang M, Liu C, Yuan XQ, Cui FP, Miao Y, Yao W, et al. Oxidatively generated DNA damage mediates the associations of exposure to phthalates with uterine fibroids and endometriosis: Findings from TREE cohort. Free Radic Biol Med (2023) 205:69–76. doi: 10.1016/j.freeradbiomed.2023.05.029
35. Lee G, Kim S, Bastiaensen M, Malarvannan G, Poma G, Caballero Casero N, et al. Exposure to organophosphate esters, phthalates, and alternative plasticizers in association with uterine fibroids. Environ Res (2020) 189:109874. doi: 10.1016/j.envres.2020.109874
36. Fruh V, Claus Henn B, Weuve J, Wesselink AK, Orta OR, Heeren T, et al. Incidence of uterine leiomyoma in relation to urinary concentrations of phthalate and phthalate alternative biomarkers: A prospective ultrasound study. Environ Int (2021) 147:106218. doi: 10.1016/j.envint.2020.106218
37. Zota AR, Vannoy BN. Integrating intersectionality into the exposome paradigm: A novel approach to racial inequities in uterine fibroids. Am J Public Health (2021) 111:104–9. doi: 10.2105/AJPH.2020.305979
38. Ye S, Chung HW, Jeong K, Sung YA, Lee H, Park SY, et al. Blood cadmium and volume of uterine fibroids in premenopausal women. Ann Occup Environ Med (2017) 29:22. doi: 10.1186/s40557-017-0178-8
39. Yan Y, Shi M, Fannin R, Yu L, Liu J, Castro L, et al. Prolonged cadmium exposure alters migration dynamics and increases heterogeneity of human uterine fibroid cells-insights from time lapse analysis. Biomedicines (2022) 10(4):917. doi: 10.3390/biomedicines10040917
40. Yan Y, Liu J, Lawrence A, Dykstra MJ, Fannin R, Gerrish K, et al. Prolonged cadmium exposure alters benign uterine fibroid cell behavior, extracellular matrix components, and TGFB signaling. FASEB J (2021) 35:e21738. doi: 10.1096/fj.202100354R
41. Liu J, Yu L, Castro L, Yan Y, Sifre MI, Bortner CD, et al. A nongenomic mechanism for “metalloestrogenic” effects of cadmium in human uterine leiomyoma cells through G protein-coupled estrogen receptor. Arch Toxicol (2019) 93:2773–85. doi: 10.1007/s00204-019-02544-0
42. Yu L, Liu J, Yan Y, Burwell A, Castro L, Shi M, et al. “Metalloestrogenic” effects of cadmium downstream of G protein-coupled estrogen receptor and mitogen-activated protein kinase pathways in human uterine fibroid cells. Arch Toxicol (2021) 95:1995–2006. doi: 10.1007/s00204-021-03033-z
43. Singh P, Metkari SM, Bhartiya D. Mice uterine stem cells are affected by neonatal endocrine disruption & Initiate uteropathies in adult life independent of circulatory ovarian hormones. Stem Cell Rev Rep (2022) 18:1686–701. doi: 10.1007/s12015-021-10279-8
44. Gaspari L, Soyer-Gobillard MO, Rincheval N, Paris F, Kalfa N, Hamamah S, et al. Birth outcomes in DES children and grandchildren: A multigenerational national cohort study on informative families. Int J Environ Res Public Health (2023) 20(3):2542. doi: 10.3390/ijerph20032542
45. Yang Q, Ali M, Treviño LS, Mas A, Al-Hendy A. Developmental reprogramming of myometrial stem cells by endocrine disruptor linking to risk of uterine fibroids. Cell Mol Life Sci (2023) 80:274. doi: 10.1007/s00018-023-04919-0
46. Yang Q, Ali M, Treviño LS, Mas A, Ismail N, Al-Hendy A. Epigenetic modulation of inflammatory pathways in myometrial stem cells and risk of uterine fibroids. Int J Mol Sci (2023) 24(14):11641. doi: 10.3390/ijms241411641
47. Bariani MV, Cui YH, Ali M, Bai T, Grimm SL, Coarfa C, et al. TGFβ signaling links early life endocrine-disrupting chemicals exposure to suppression of nucleotide excision repair in rat myometrial stem cells. Cell Mol Life Sci (2023) 80:288. doi: 10.1007/s00018-023-04928-z
48. Elkafas H, Ali M, Elmorsy E, Kamel R, Thompson WE, Badary O, et al. Vitamin D3 ameliorates DNA damage caused by developmental exposure to endocrine disruptors in the uterine myometrial stem cells of eker rats. Cells (2020) 9(6):1459. doi: 10.3390/cells9061459
49. Zhang Y, Lu Y, Ma H, Xu Q, Wu X. Combined exposure to multiple endocrine disruptors and uterine leiomyomata and endometriosis in US women. Front Endocrinol (Lausanne) (2021) 12:726876. doi: 10.3389/fendo.2021.726876
50. Yang F, Chen Y. Urinary phytoestrogens and the risk of uterine leiomyomata in US women. BMC Womens Health (2023) 23:261. doi: 10.1186/s12905-023-02381-5
51. Nowak K, Ratajczak-Wrona W, Górska M, Jabłońska E. Parabens and their effects on the endocrine system. Mol Cell Endocrinol (2018) 474:238–51. doi: 10.1016/j.mce.2018.03.014
52. Maske P, Dighe V, Vanage G. n-butylparaben exposure during perinatal period impairs fertility of the F1 generation female rats. Chemosphere (2018) 213:114–23. doi: 10.1016/j.chemosphere.2018.08.130
53. Ogunsina K, Sandler DP, Murphy JD, Harmon QE, D'aloisio AA, Baird DD, et al. Association of genital talc and douche use in early adolescence or adulthood with uterine fibroids diagnoses. Am J Obstet Gynecol (2023) 229(6):665.e1–10. doi: 10.1016/j.ajog.2023.08.014
54. Stephens VR, Rumph JT, Ameli S, Bruner-Tran KL, Osteen KG. The potential relationship between environmental endocrine disruptor exposure and the development of endometriosis and adenomyosis. Front Physiol (2021) 12:807685. doi: 10.3389/fphys.2021.807685
55. Zhang Y, Ma NY. Environmental risk factors for endometriosis: an umbrella review of a meta-analysis of 354 observational studies with over 5 million populations. Front Med (Lausanne) (2021) 8:680833. doi: 10.3389/fmed.2021.680833
56. Basso CG, De Araújo-Ramos AT, Martino-Andrade AJ. Exposure to phthalates and female reproductive health: A literature review. Reprod Toxicol (2022) 109:61–79. doi: 10.1016/j.reprotox.2022.02.006
57. Dutta S, Banu SK, Arosh JA. Endocrine disruptors and endometriosis. Reprod Toxicol (2023) 115:56–73. doi: 10.1016/j.reprotox.2022.11.007
58. Interdonato L, Siracusa R, Fusco R, Cuzzocrea S, Di Paola R. Endocrine disruptor compounds in environment: focus on women's reproductive health and endometriosis. Int J Mol Sci (2023) 24(6):5682. doi: 10.3390/ijms24065682
59. Lee AW, Eata V. Association of environmental phenols with endometriosis and uterine leiomyoma: An analysis of NHANES,. 2003-2006. Reprod Toxicol (2022) 113:30–4. doi: 10.1016/j.reprotox.2022.08.003
60. Peinado FM, Lendínez I, Sotelo R, Iribarne-Durán LM, Fernández-Parra J, Vela-Soria F, et al. Association of urinary levels of bisphenols A, F, and S with endometriosis risk: preliminary results of the endEA study. Int J Environ Res Public Health (2020) 17(4):1194. doi: 10.3390/ijerph17041194
61. Kendziorski JA, Belcher SM. Effects of whole life exposure to Bisphenol A or 17α-ethinyl estradiol in uterus of nulligravida CD1 mice. Data Brief (2015) 5:948–53. doi: 10.1016/j.dib.2015.10.034
62. Xue W, Yao X, Ting G, Ling J, Huimin L, Yuan Q, et al. BPA modulates the WDR5/TET2 complex to regulate ERβ expression in eutopic endometrium and drives the development of endometriosis. Environ Pollut (2021) 268:115748. doi: 10.1016/j.envpol.2020.115748
63. Cho YJ, Park SB, Park JW, Oh SR, Han M. Bisphenol A modulates inflammation and proliferation pathway in human endometrial stromal cells by inducing oxidative stress. Reprod Toxicol (2018) 81:41–9. doi: 10.1016/j.reprotox.2018.06.016
64. Wen X, Xiong Y, Jin L, Zhang M, Huang L, Mao Y, et al. Bisphenol A exposure enhances endometrial stromal cell invasion and has a positive association with peritoneal endometriosis. Reprod Sci (2020) 27:704–12. doi: 10.1007/s43032-019-00076-7
65. Jones RL, Lang SA, Kendziorski JA, Greene AD, Burns KA. Use of a mouse model of experimentally induced endometriosis to evaluate and compare the effects of bisphenol A and bisphenol AF exposure. Environ Health Perspect (2018) 126:127004. doi: 10.1289/EHP3802
66. Sirohi D, Al Ramadhani R, Knibbs LD. Environmental exposures to endocrine disrupting chemicals (EDCs) and their role in endometriosis: a systematic literature review. Rev Environ Health (2021) 36:101–15. doi: 10.1515/reveh-2020-0046
67. Cai W, Yang J, Liu Y, Bi Y, Wang H. Association between phthalate metabolites and risk of endometriosis: A meta-analysis. Int J Environ Res Public Health (2019) 16(19):3678. doi: 10.3390/ijerph16193678
68. Yi H, Wu H, Zhu W, Lin Q, Zhao X, Lin R, et al. Phthalate exposure and risk of ovarian dysfunction in endometriosis: human and animal data. Front Cell Dev Biol (2023) 11:1154923. doi: 10.3389/fcell.2023.1154923
69. Kim Y, Kim MR, Kim JH, Cho HH. Aldo-keto reductase activity after diethylhexyl phthalate exposure in eutopic and ectopic endometrial cells. Eur J Obstet Gynecol Reprod Biol (2017) 215:215–9. doi: 10.1016/j.ejogrb.2017.05.018
70. Kim SH, Cho S, Ihm HJ, Oh YS, Heo SH, Chun S, et al. Possible role of phthalate in the pathogenesis of endometriosis: in vitro, animal, and human data. J Clin Endocrinol Metab (2015) 100:E1502–11. doi: 10.1210/jc.2015-2478
71. Gonzalez-Martin R, Palomar A, Medina-Laver Y, Quiñonero A, Domínguez F. Endometrial cells acutely exposed to phthalates in vitro do not phenocopy endometriosis. Int J Mol Sci (2022) 23(19):11041. doi: 10.3390/ijms231911041
72. Monnin N, Fattet AJ, Koscinski I. Endometriosis: update of pathophysiology, (Epi) genetic and environmental involvement. Biomedicines (2023) 11(3):978. doi: 10.3390/biomedicines11030978
73. Chou YC, Chen YC, Chen MJ, Chang CW, Lai GL, Tzeng CR. Exposure to mono-n-butyl phthalate in women with endometriosis and its association with the biological effects on human granulosa cells. Int J Mol Sci (2020) 21(5):1794. doi: 10.3390/ijms21051794
74. Sharma P, Lee JL, Tsai EM, Chang Y, Suen JL. n-butyl benzyl phthalate exposure promotes lesion survival in a murine endometriosis model. Int J Environ Res Public Health (2021) 18(7):3640. doi: 10.3390/ijerph18073640
75. Moreira Fernandez MA, Cardeal ZL, Carneiro MM, André LC. Study of possible association between endometriosis and phthalate and bisphenol A by biomarkers analysis. J Pharm BioMed Anal (2019) 172:238–42. doi: 10.1016/j.jpba.2019.04.048
76. Ottolina J, Schimberni M, Makieva S, Bartiromo L, Fazia T, Bernardinelli L, et al. Early-life factors, in-utero exposures and endometriosis risk: a meta-analysis. Reprod BioMed Online (2020) 41:279–89. doi: 10.1016/j.rbmo.2020.04.005
77. Gaspari L, Soyer-Gobillard MO, Paris F, Kalfa N, Hamamah S, Sultan C. Multigenerational endometriosis : consequence of fetal exposure to diethylstilbestrol? Environ Health (2021) 20:96. doi: 10.1186/s12940-021-00780-5
78. Krishnamoorthy SP, Kalimuthu V, Chandran Manimegalai S, Arulanandu AM, Thiyagarajan R, Balamuthu K. Evaluation of the potential role of diethylstilbestrol on the induction of endometriosis in a rat model - An alternative approach. Biochem Biophys Res Commun (2022) 617:18–24. doi: 10.1016/j.bbrc.2022.05.092
79. Matta K, Lefebvre T, Vigneau E, Cariou V, Marchand P, Guitton Y, et al. Associations between persistent organic pollutants and endometriosis: A multiblock approach integrating metabolic and cytokine profiling. Environ Int (2022) 158:106926. doi: 10.1016/j.envint.2021.106926
80. Guo W, Pan B, Sakkiah S, Yavas G, Ge W, Zou W, et al. Persistent organic pollutants in food: contamination sources, health effects and detection methods. Int J Environ Res Public Health (2019) 16(22):4361. doi: 10.3390/ijerph16224361
81. Pollack AZ, Krall JR, Kannan K, Buck Louis GM. Adipose to serum ratio and mixtures of persistent organic pollutants in relation to endometriosis: Findings from the ENDO Study. Environ Res (2021) 195:110732. doi: 10.1016/j.envres.2021.110732
82. Campbell S, Raza M, Pollack AZ. Perfluoroalkyl substances and endometriosis in US women in NHANES. 2003-2006. Reprod Toxicol (2016) 65:230–5. doi: 10.1016/j.reprotox.2016.08.009
83. Chiappini F, Ceballos L, Olivares C, Bastón JI, Miret N, Pontillo C, et al. Endocrine disruptor hexachlorobenzene induces cell migration and invasion, and enhances aromatase expression levels in human endometrial stromal cells. Food Chem Toxicol (2022) 162:112867. doi: 10.1016/j.fct.2022.112867
84. Chiappini F, Sánchez M, Miret N, Cocca C, Zotta E, Ceballos L, et al. Exposure to environmental concentrations of hexachlorobenzene induces alterations associated with endometriosis progression in a rat model. Food Chem Toxicol (2019) 123:151–61. doi: 10.1016/j.fct.2018.10.056
85. Huang Q, Chen Y, Chen Q, Zhang H, Lin Y, Zhu M, et al. Dioxin-like rather than non-dioxin-like PCBs promote the development of endometriosis through stimulation of endocrine-inflammation interactions. Arch Toxicol (2017) 91:1915–24. doi: 10.1007/s00204-016-1854-0
86. Rumph JT, Stephens VR, Archibong AE, Osteen KG, Bruner-Tran KL. Environmental endocrine disruptors and endometriosis. Adv Anat Embryol Cell Biol (2020) 232:57–78. doi: 10.1007/978-3-030-51856-1_4
87. Kim M, Kim SH, Kim HJ, Whang DH, Yun SC, Lee SR, et al. Plasma levels of polychlorinated biphenyl, genetic polymorphisms, and the risk of advanced stage endometriosis. Gynecol Endocrinol (2020) 36:636–40. doi: 10.1080/09513590.2020.1753183
88. Mukhopadhyay R, Prabhu NB, Kabekkodu SP, Rai PS. Review on bisphenol A and the risk of polycystic ovarian syndrome: an insight from endocrine and gene expression. Environ Sci pollut Res Int (2022) 29:32631–50. doi: 10.1007/s11356-022-19244-5
89. Ghanati K, Jahanbakhsh M, Shakoori A, Aghebat-Bekheir S, Khalili-Rikabadi A, Sadighara P. The association between polycystic ovary syndrome and environmental pollutants based on animal and human study; a systematic review. Rev Environ Health (2023). doi: 10.1515/reveh-2022-0187
90. Hu Y, Wen S, Yuan D, Peng L, Zeng R, Yang Z, et al. The association between the environmental endocrine disruptor bisphenol A and polycystic ovary syndrome: a systematic review and meta-analysis. Gynecol Endocrinol (2018) 34:370–7. doi: 10.1080/09513590.2017.1405931
91. Milanović M, Milošević N, Sudji J, Stojanoski S, Atanacković Krstonošić M, Bjelica A, et al. Can environmental pollutant bisphenol A increase metabolic risk in polycystic ovary syndrome? Clin Chim Acta (2020) 507:257–63. doi: 10.1016/j.cca.2020.05.009
92. Prabhu NB, Vasishta S, Bhat SK, Joshi MB, Kabekkodu SP, Satyamoorthy K, et al. Distinct metabolic signatures in blood plasma of bisphenol A-exposed women with polycystic ovarian syndrome. Environ Sci pollut Res Int (2023) 30:64025–35. doi: 10.1007/s11356-023-26820-w
93. Prabhu NB, Adiga D, Kabekkodu SP, Bhat SK, Satyamoorthy K, Rai PS. Bisphenol A exposure modulates reproductive and endocrine system, mitochondrial function and cellular senescence in female adult rats: A hallmarks of polycystic ovarian syndrome phenotype. Environ Toxicol Pharmacol (2022) 96:104010. doi: 10.1016/j.etap.2022.104010
94. Yang Z, Shi J, Guo Z, Chen M, Wang C, He C, et al. A pilot study on polycystic ovarian syndrome caused by neonatal exposure to tributyltin and bisphenol A in rats. Chemosphere (2019) 231:151–60. doi: 10.1016/j.chemosphere.2019.05.129
95. Shi J, Liu C, Chen M, Yan J, Wang C, Zuo Z, et al. The interference effects of bisphenol A on the synthesis of steroid hormones in human ovarian granulosa cells. Environ Toxicol (2021) 36:665–74. doi: 10.1002/tox.23070
96. Dabeer S, Afjal MA, Ahmad S, Fatima M, Habib H, Parvez S, et al. Transgenerational effect of parental obesity and chronic parental bisphenol A exposure on hormonal profile and reproductive organs of preadolescent Wistar rats of F1 generation: A one-generation study. Hum Exp Toxicol (2020) 39:59–76. doi: 10.1177/0960327119873017
97. Chakraborty S, Anand S, Coe S, Reh B, Bhandari RK. The PCOS-NAFLD multidisease phenotype occurred in medaka fish four generations after the removal of bisphenol A exposure. Environ Sci Technol (2023) 57:12602–19. doi: 10.1021/acs.est.3c01922
98. Jurewicz J, Majewska J, Berg A, Owczarek K, Zajdel R, Kaleta D, et al. Serum bisphenol A analogues in women diagnosed with the polycystic ovary syndrome - is there an association? Environ Pollut (2021) 272:115962. doi: 10.1016/j.envpol.2020.115962
99. Zhan W, Tang W, Shen X, Xu H, Zhang J. Exposure to bisphenol A and its analogs and polycystic ovarian syndrome in women of childbearing age: A multicenter case-control study. Chemosphere (2023) 313:137463. doi: 10.1016/j.chemosphere.2022.137463
100. Jin Y, Zhang Q, Pan JX, Wang FF, Qu F. The effects of di(2-ethylhexyl) phthalate exposure in women with polycystic ovary syndrome undergoing in vitro fertilization. J Int Med Res (2019) 47:6278–93. doi: 10.1177/0300060519876467
101. Al-Saleh I. The relationship between urinary phthalate metabolites and polycystic ovary syndrome in women undergoing in vitro fertilization: Nested case-control study. Chemosphere (2022) 286:131495. doi: 10.1016/j.chemosphere.2021.131495
102. Milankov A, Milanović M, Milošević N, Sudji J, Pejaković S, Milić N, et al. The effects of phthalate exposure on metabolic parameters in polycystic ovary syndrome. Clin Chim Acta (2023) 540:117225. doi: 10.1016/j.cca.2023.117225
103. Zhang M, Liu C, Yuan XQ, Cui FP, Miao Y, Yao W, et al. Individual and joint associations of urinary phthalate metabolites with polycystic ovary and polycystic ovary syndrome: Results from the TREE cohort. Environ Toxicol Pharmacol (2023) 102:104233. doi: 10.1016/j.etap.2023.104233
104. Akın L, Kendirci M, Narin F, Kurtoğlu S, Hatipoğlu N, Elmalı F. Endocrine disruptors and polycystic ovary syndrome: phthalates. J Clin Res Pediatr Endocrinol (2020) 12:393–400. doi: 10.4274/jcrpe.galenos.2020.2020.0037
105. Akgül S, Sur Ü, Düzçeker Y, Balcı A, Kızılkan MP, Kanbur N, et al. Bisphenol A and phthalate levels in adolescents with polycystic ovary syndrome. Gynecol Endocrinol (2019) 35:1084–7. doi: 10.1080/09513590.2019.1630608
106. Guo Z, Qiu H, Wang L, Wang L, Wang C, Chen M, et al. Association of serum organochlorine pesticides concentrations with reproductive hormone levels and polycystic ovary syndrome in a Chinese population. Chemosphere (2017) 171:595–600. doi: 10.1016/j.chemosphere.2016.12.127
107. Merlo E, Silva IV, Cardoso RC, Graceli JB. The obesogen tributyltin induces features of polycystic ovary syndrome (PCOS): a review. J Toxicol Environ Health B Crit Rev (2018) 21:181–206. doi: 10.1080/10937404.2018.1496214
108. Chen M, He C, Zhu K, Chen Z, Meng Z, Jiang X, et al. Resveratrol ameliorates polycystic ovary syndrome via transzonal projections within oocyte-granulosa cell communication. Theranostics (2022) 12:782–95. doi: 10.7150/thno.67167
109. Hammarstrand S, Jakobsson K, Andersson E, Xu Y, Li Y, Olovsson M, et al. Perfluoroalkyl substances (PFAS) in drinking water and risk for polycystic ovarian syndrome, uterine leiomyoma, and endometriosis: A Swedish cohort study. Environ Int (2021) 157:106819. doi: 10.1016/j.envint.2021.106819
110. Zhan W, Qiu W, Ao Y, Zhou W, Sun Y, Zhao H, et al. Environmental exposure to emerging alternatives of per- and polyfluoroalkyl substances and polycystic ovarian syndrome in women diagnosed with infertility: A mixture analysis. Environ Health Perspect (2023) 131:57001. doi: 10.1289/EHP11814
111. Wang W, Zhou W, Wu S, Liang F, Li Y, Zhang J, et al. Perfluoroalkyl substances exposure and risk of polycystic ovarian syndrome related infertility in Chinese women. Environ Pollut (2019) 247:824–31. doi: 10.1016/j.envpol.2019.01.039
112. Domingo JL, Nadal M. Human exposure to per- and polyfluoroalkyl substances (PFAS) through drinking water: A review of the recent scientific literature. Environ Res (2019) 177:108648. doi: 10.1016/j.envres.2019.108648
113. Anderko L, Pennea E. Exposures to per-and polyfluoroalkyl substances (PFAS): Potential risks to reproductive and children's health. Curr Probl Pediatr Adolesc Health Care (2020) 50:100760. doi: 10.1016/j.cppeds.2020.100760
114. Blake BE, Fenton SE. Early life exposure to per- and polyfluoroalkyl substances (PFAS) and latent health outcomes: A review including the placenta as a target tissue and possible driver of peri- and postnatal effects. Toxicology (2020) 443:152565. doi: 10.1016/j.tox.2020.152565
115. Heffernan AL, Cunningham TK, Drage DS, Aylward LL, Thompson K, Vijayasarathy S, et al. Perfluorinated alkyl acids in the serum and follicular fluid of UK women with and without polycystic ovarian syndrome undergoing fertility treatment and associations with hormonal and metabolic parameters. Int J Hyg Environ Health (2018) 221:1068–75. doi: 10.1016/j.ijheh.2018.07.009
116. González-Alvarez ME, Keating AF. Hepatic and ovarian effects of perfluorooctanoic acid exposure differ in lean and obese adult female mice. Toxicol Appl Pharmacol (2023) 474:116614. doi: 10.1016/j.taap.2023.116614
117. Jiao L, Li S, Zhai J, Wang D, Li H, Chu W, et al. Propylparaben concentrations in the urine of women and adverse effects on ovarian function in mice in vivo and ovarian cells in vitro. J Appl Toxicol (2021) 41:1719–31. doi: 10.1002/jat.4225
118. Rafiee A, Hoseini M, Akbari S, Mahabee-Gittens EM. Exposure to Polycyclic Aromatic Hydrocarbons and adverse reproductive outcomes in women: current status and future perspectives. Rev Environ Health (2023). doi: 10.1515/reveh-2022-0182
119. Arab A, Mostafalou S. Pesticides and insulin resistance-related metabolic diseases: Evidences and mechanisms. Pestic Biochem Physiol (2023) 195:105521. doi: 10.1016/j.pestbp.2023.105521
120. Yang Q, Zhao Y, Qiu X, Zhang C, Li R, Qiao J. Association of serum levels of typical organic pollutants with polycystic ovary syndrome (PCOS): a case-control study. Hum Reprod (2015) 30:1964–73. doi: 10.1093/humrep/dev123
121. Srnovršnik T, Virant-Klun I, Pinter B. Polycystic ovary syndrome and endocrine disruptors (Bisphenols, parabens, and triclosan)-A systematic review. Life (Basel) (2023) 13(1):138. doi: 10.3390/life13010138
122. Nevoral J, Kolinko Y, Moravec J, Žalmanová T, Hošková K, Prokešová Š, et al. Long-term exposure to very low doses of bisphenol S affects female reproduction. Reproduction (2018) 156:47–57. doi: 10.1530/REP-18-0092
123. Park SY, Jeon JH, Jeong K, Chung HW, Lee H, Sung YA, et al. The association of ovarian reserve with exposure to bisphenol A and phthalate in reproductive-aged women. J Korean Med Sci (2021) 36:e1. doi: 10.3346/jkms.2021.36.e1
124. Zhang N, Zhao Y, Zhai L, Bai Y, Jia L. Urinary bisphenol A and S are associated with diminished ovarian reserve in women from an infertility clinic in Northern China. Ecotoxicol Environ Saf (2023) 256:114867. doi: 10.1016/j.ecoenv.2023.114867
125. Stavridis K, Triantafyllidou O, Pisimisi M, Vlahos N. Bisphenol-A and female fertility: an update of existing epidemiological studies. J Clin Med (2022) 11(23):7227. doi: 10.3390/jcm11237227
126. Messerlian C, Souter I, Gaskins AJ, Williams PL, Ford JB, Chiu YH, et al. Urinary phthalate metabolites and ovarian reserve among women seeking infertility care. Hum Reprod (2016) 31:75–83. doi: 10.1093/humrep/dev292
127. Li Y, Xiao N, Liu M, Liu Y, He A, Wang L, et al. Dysregulation of steroid metabolome in follicular fluid links phthalate exposure to diminished ovarian reserve of childbearing-age women. Environ Pollut (2023) 330:121730. doi: 10.1016/j.envpol.2023.121730
128. Panagiotou EM, Ojasalo V, Damdimopoulou P. Phthalates, ovarian function and fertility in adulthood. Best Pract Res Clin Endocrinol Metab (2021) 35:101552. doi: 10.1016/j.beem.2021.101552
129. Sun J, Gan L, Lv S, Wang T, Dai C, Sun J. Exposure to Di-(2-Ethylhexyl) phthalate drives ovarian dysfunction by inducing granulosa cell pyroptosis via the SLC39A5/NF-κB/NLRP3 axis. Ecotoxicol Environ Saf (2023) 252:114625. doi: 10.1016/j.ecoenv.2023.114625
130. Ojemaye CY, Onwordi CT, Pampanin DM, Sydnes MO, Petrik L. Presence and risk assessment of herbicides in the marine environment of Camps Bay (Cape Town, South Africa). Sci Total Environ (2020) 738:140346. doi: 10.1016/j.scitotenv.2020.140346
131. Ojemaye CY, Onwordi CT, Pampanin DM, Sydnes MO, Petrik L. Herbicides in camps bay (Cape town, South Africa), supplemented. Sci Total Environ (2021) 778:146057. doi: 10.1016/j.scitotenv.2021.146057
132. Li C, Cao M, Ma L, Ye X, Song Y, Pan W, et al. Pyrethroid pesticide exposure and risk of primary ovarian insufficiency in chinese women. Environ Sci Technol (2018) 52:3240–8. doi: 10.1021/acs.est.7b06689
133. Wang W, Yang LL, Luo SM, Ma JY, Zhao Y, Shen W, et al. Toxic effects and possible mechanisms following malathion exposure in porcine granulosa cells. Environ Toxicol Pharmacol (2018) 64:172–80. doi: 10.1016/j.etap.2018.11.002
134. Ding N, Harlow SD, Randolph JF Jr., Loch-Caruso R, Park SK. Perfluoroalkyl and polyfluoroalkyl substances (PFAS) and their effects on the ovary. Hum Reprod Update (2020) 26:724–52. doi: 10.1093/humupd/dmaa018
135. Shen H, Gao M, Li Q, Sun H, Jiang Y, Liu L, et al. Effect of PFOA exposure on diminished ovarian reserve and its metabolism. Reprod Biol Endocrinol (2023) 21:16. doi: 10.1186/s12958-023-01056-y
136. Mínguez-Alarcón L, Christou G, Messerlian C, Williams PL, Carignan CC, Souter I, et al. Urinary triclosan concentrations and diminished ovarian reserve among women undergoing treatment in a fertility clinic. Fertil Steril (2017) 108:312–9. doi: 10.1016/j.fertnstert.2017.05.020
137. Génard-Walton M, Warembourg C, Duros S, Mercier F, Lefebvre T, Guivarc'h-Levêque A, et al. Serum persistent organic pollutants and diminished ovarian reserve: a single-exposure and mixture exposure approach from a French case-control study. Hum Reprod (2023) 38:701–15. doi: 10.1093/humrep/dead028
138. Tian T, Hao Y, Wang Y, Xu X, Long X, Yan L, et al. Mixed and single effects of endocrine disrupting chemicals in follicular fluid on likelihood of diminished ovarian reserve: A case-control study. Chemosphere (2023) 330:138727. doi: 10.1016/j.chemosphere.2023.138727
139. Jurewicz J, Radwan M, Wielgomas B, Karwacka A, Klimowska A, Kałużny P, et al. Parameters of ovarian reserve in relation to urinary concentrations of parabens. Environ Health (2020) 19:26. doi: 10.1186/s12940-020-00580-3
140. Ara C, Asmatullah, Butt N, Ali S, Batool F, Shakir HA, et al. Abnormal steroidogenesis, oxidative stress, and reprotoxicity following prepubertal exposure to butylparaben in mice and protective effect of Curcuma longa. Environ Sci Pollut Res Int (2021) 28:6111–21. doi: 10.1007/s11356-020-10819-8
141. Li M, Zhou S, Wu Y, Li Y, Yan W, Guo Q, et al. Prenatal exposure to propylparaben at human-relevant doses accelerates ovarian aging in adult mice. Environ Pollut (2021) 285:117254. doi: 10.1016/j.envpol.2021.117254
142. Gal A, Gedye K, Craig ZR, Ziv-Gal A. Propylparaben inhibits mouse cultured antral follicle growth, alters steroidogenesis, and upregulates levels of cell-cycle and apoptosis regulators. Reprod Toxicol (2019) 89:100–6. doi: 10.1016/j.reprotox.2019.07.009
143. Pacyga DC, Chiang C, Li Z, Strakovsky RS, Ziv-Gal A. Parabens and menopause-related health outcomes in midlife women: A pilot study. J Womens Health (Larchmt) (2022) 31:1645–54. doi: 10.1089/jwh.2022.0004
144. Jiang VS, Calafat AM, Williams PL, Chavarro JE, Ford JB, Souter I, et al. Temporal trends in urinary concentrations of phenols, phthalate metabolites and phthalate replacements between. 2000 and. 2017 in Boston, MA. Sci Total Environ (2023) 898:165353. doi: 10.1016/j.scitotenv.2023.165353
145. Génard-Walton M, Mcgee G, Williams PL, Souter I, Ford JB, Chavarro JE, et al. Mixtures of urinary concentrations of phenols and phthalate biomarkers in relation to the ovarian reserve among women attending a fertility clinic. Sci Total Environ (2023) 898:165536. doi: 10.1016/j.scitotenv.2023.165536
146. Özel Ş, Tokmak A, Aykut O, Aktulay A, Hançerlioğulları N, Engin Ustun Y. Serum levels of phthalates and bisphenol-A in patients with primary ovarian insufficiency. Gynecol Endocrinol (2019) 35:364–7. doi: 10.1080/09513590.2018.1534951
147. Chen W EQ, Sun B, Zhang P, Li N, Fei S, Wang Y, et al. PARP1-catalyzed PARylation of YY1 mediates endoplasmic reticulum stress in granulosa cells to determine primordial follicle activation. Cell Death Dis (2023) 14:524. doi: 10.1038/s41419-023-05984-w
148. Li C, Cao M, Qi T, Ye X, Ma L, Pan W, et al. The association of bisphenol A exposure with premature ovarian insufficiency: a case-control study. Climacteric (2021) 24:95–100. doi: 10.1080/13697137.2020.1781078
149. Ding T, Yan W, Zhou T, Shen W, Wang T, Li M, et al. Endocrine disrupting chemicals impact on ovarian aging: Evidence from epidemiological and experimental evidence. Environ Pollut (2022) 305:119269. doi: 10.1016/j.envpol.2022.119269
150. Zhu X, Liu M, Dong R, Gao L, Hu J, Zhang X, et al. Mechanism exploration of environmental pollutants on premature ovarian insufficiency: a systematic review and meta-analysis. Reprod Sci (2023). doi: 10.1007/s43032-023-01326-5
151. Tran DN, Jung EM, Yoo YM, Ahn C, Kang HY, Choi KC, et al. Depletion of follicles accelerated by combined exposure to phthalates and 4-vinylcyclohexene diepoxide, leading to premature ovarian failure in rats. Reprod Toxicol (2018) 80:60–7. doi: 10.1016/j.reprotox.2018.06.071
152. Cao M, Pan W, Shen X, Li C, Zhou J, Liu J. Urinary levels of phthalate metabolites in women associated with risk of premature ovarian failure and reproductive hormones. Chemosphere (2020) 242:125206. doi: 10.1016/j.chemosphere.2019.125206
153. Brehm E, Zhou C, Gao L, Flaws JA. Prenatal exposure to an environmentally relevant phthalate mixture accelerates biomarkers of reproductive aging in a multiple and transgenerational manner in female mice. Reprod Toxicol (2020) 98:260–8. doi: 10.1016/j.reprotox.2020.10.009
154. Craig ZR, Ziv-Gal A. Pretty good or pretty bad? The ovary and chemicals in personal care products. Toxicol Sci (2018) 162:349–60. doi: 10.1093/toxsci/kfx285
155. Zhang FL, Kong L, Zhao AH, Ge W, Yan ZH, Li L, et al. Inflammatory cytokines as key players of apoptosis induced by environmental estrogens in the ovary. Environ Res (2021) 198:111225. doi: 10.1016/j.envres.2021.111225
156. Pan W, Ye X, Yin S, Ma X, Li C, Zhou J, et al. Selected persistent organic pollutants associated with the risk of primary ovarian insufficiency in women. Environ Int (2019) 129:51–8. doi: 10.1016/j.envint.2019.05.023
157. Ma X, Zhang W, Song J, Li F, Liu J. Lifelong exposure to pyrethroid insecticide cypermethrin at environmentally relevant doses causes primary ovarian insufficiency in female mice. Environ Pollut (2022) 298:118839. doi: 10.1016/j.envpol.2022.118839
158. Ghosh R, Banerjee B, Das T, Jana K, Choudhury SM. Antigonadal and endocrine-disrupting activities of lambda cyhalothrin in female rats and its attenuation by taurine. Toxicol Ind Health (2018) 34:146–57. doi: 10.1177/0748233717742291
159. Cao LB, Leung CK, Law PW, Lv Y, Ng CH, Liu HB, et al. Systemic changes in a mouse model of VCD-induced premature ovarian failure. Life Sci (2020) 262:118543. doi: 10.1016/j.lfs.2020.118543
160. Rattan S, Zhou C, Chiang C, Mahalingam S, Brehm E, Flaws JA. Exposure to endocrine disruptors during adulthood: consequences for female fertility. J Endocrinol (2017) 233:R109–r129. doi: 10.1530/JOE-17-0023
161. Gaspari L, Paris F, Kalfa N, Soyer-Gobillard MO, Sultan C, Hamamah S. Experimental evidence of. 2,3,7,8-tetrachlordibenzo-p-dioxin (TCDD) transgenerational effects on reproductive health. Int J Mol Sci (2021) 22(16):9091. doi: 10.3390/ijms22169091
162. Zhang S, Tan R, Pan R, Xiong J, Tian Y, Wu J, et al. Association of perfluoroalkyl and polyfluoroalkyl substances with premature ovarian insufficiency in Chinese women. J Clin Endocrinol Metab (2018) 103:2543–51. doi: 10.1210/jc.2017-02783
163. Lee JH, Lee M, Ahn C, Kang HY, Tran DN, Jeung EB. Parabens accelerate ovarian dysfunction in a 4-vinylcyclohexene diepoxide-induced ovarian failure model. Int J Environ Res Public Health (2017) 14(2):161. doi: 10.3390/ijerph14020161
164. Zhang Y, Mustieles V, Williams PL, Wylie BJ, Souter I, Calafat AM, et al. Parental preconception exposure to phenol and phthalate mixtures and the risk of preterm birth. Environ Int (2021) 151:106440. doi: 10.1016/j.envint.2021.106440
165. Gaggi G, Di Credico A, Barbagallo F, Ghinassi B, Di Baldassarre A. Bisphenols and perfluoroalkyls alter human stem cells integrity: A possible link with infertility. Environ Res (2023) 235:116487. doi: 10.1016/j.envres.2023.116487
166. Pivonello C, Muscogiuri G, Nardone A, Garifalos F, Provvisiero DP, Verde N, et al. Bisphenol A: an emerging threat to female fertility. Reprod Biol Endocrinol (2020) 18:22. doi: 10.1186/s12958-019-0558-8
167. Catenza CJ, Farooq A, Shubear NS, Donkor KK. A targeted review on fate, occurrence, risk and health implications of bisphenol analogues. Chemosphere (2021) 268:129273. doi: 10.1016/j.chemosphere.2020.129273
168. Campen KA, Kucharczyk KM, Bogin B, Ehrlich JM, Combelles CMH. Spindle abnormalities and chromosome misalignment in bovine oocytes after exposure to low doses of bisphenol A or bisphenol S. Hum Reprod (2018) 33:895–904. doi: 10.1093/humrep/dey050
169. Amir S, Shah STA, Mamoulakis C, Docea AO, Kalantzi OI, Zachariou A, et al. Endocrine disruptors acting on estrogen and androgen pathways cause reproductive disorders through multiple mechanisms: A review. Int J Environ Res Public Health (2021) 18(4):1464. doi: 10.3390/ijerph18041464
170. Lin M, Hua R, Ma J, Zhou Y, Li P, Xu X, et al. Bisphenol A promotes autophagy in ovarian granulosa cells by inducing AMPK/mTOR/ULK1 signalling pathway. Environ Int (2021) 147:106298. doi: 10.1016/j.envint.2020.106298
171. Huang M, Huang M, Li X, Liu S, Fu L, Jiang X, et al. Bisphenol A induces apoptosis through GPER-dependent activation of the ROS/Ca(2+)-ASK1-JNK pathway in human granulosa cell line KGN. Ecotoxicol Environ Saf (2021) 208:111429. doi: 10.1016/j.ecoenv.2020.111429
172. Zhan W, Yang H, Zhang J, Chen Q. Association between co-exposure to phenols and phthalates mixture and infertility risk in women. Environ Res (2022) 215:114244. doi: 10.1016/j.envres.2022.114244
173. Radwan P, Wielgomas B, Radwan M, Krasiński R, Klimowska A, Kaleta D, et al. Urinary bisphenol A concentrations and in vitro fertilization outcomes among women from a fertility clinic. Reprod Toxicol (2020) 96:216–20. doi: 10.1016/j.reprotox.2020.07.009
174. Caporossi L, Viganò P, Paci E, Capanna S, Alteri A, Campo G, et al. Female reproductive health and exposure to phthalates and bisphenol A: A cross sectional study. Toxics (2021) 9(11):299. doi: 10.3390/toxics9110299
175. Trnka B, Polan M, Zigmont VA. Exposure to Di-2-ethylhexyl phthalate (DEHP) and infertility in women, NHANES. 2013-2016. Reprod Toxicol (2021) 103:46–50. doi: 10.1016/j.reprotox.2021.05.010
176. Sacha CR, Souter I, Williams PL, Chavarro JE, Ford J, Mahalingaiah S, et al. Urinary phthalate metabolite concentrations are negatively associated with follicular fluid anti-müllerian hormone concentrations in women undergoing fertility treatment. Environ Int (2021) 157:106809. doi: 10.1016/j.envint.2021.106809
177. Abdo N, Al-Khalaileh H, Alajlouni M, Hamadneh J, Alajlouni AM. Screening for phthalates biomarkers and its potential role in infertility outcomes in Jordan. J Expo Sci Environ Epidemiol (2023) 33:273–82. doi: 10.1038/s41370-022-00517-7
178. Bellavia A, Zou R, Björvang RD, Roos K, Sjunnesson Y, Hallberg I, et al. Association between chemical mixtures and female fertility in women undergoing assisted reproduction in Sweden and Estonia. Environ Res (2023) 216:114447. doi: 10.1016/j.envres.2022.114447
179. Mínguez-Alarcón L, Bellavia A, Gaskins AJ, Chavarro JE, Ford JB, Souter I, et al. Paternal mixtures of urinary concentrations of phthalate metabolites, bisphenol A and parabens in relation to pregnancy outcomes among couples attending a fertility center. Environ Int (2021) 146:106171. doi: 10.1016/j.envint.2020.106171
180. Du Y, Guo N, Wang Y, Teng X, Hua X, Deng T, et al. Follicular fluid concentrations of phthalate metabolites are associated with altered intrafollicular reproductive hormones in women undergoing in vitro fertilization. Fertil Steril (2019) 111:953–61. doi: 10.1016/j.fertnstert.2019.01.021
181. Boberg J, Johansson HKL, Franssen D, Cramer JH, Usai D, Pedersen M, et al. Perinatal exposure to known endocrine disrupters alters ovarian development and systemic steroid hormone profile in rats. Toxicology (2021) 458:152821. doi: 10.1016/j.tox.2021.152821
182. Lin J, Lin X, Qiu J, You X, Xu J. Association between heavy metals exposure and infertility among American women aged. 20-44 years: A cross-sectional analysis from. 2013 to. 2018 NHANES data. Front Public Health (2023) 11:1122183. doi: 10.3389/fpubh.2023.1122183
183. Xu G, Liu S, Huang M, Jiang X, Yang M. Cadmium induces apoptosis of human granulosa cell line KGN via mitochondrial dysfunction-mediated pathways. Ecotoxicol Environ Saf (2021) 220:112341. doi: 10.1016/j.ecoenv.2021.112341
184. Shan D, Wen X, Guan X, Fang H, Liu Y, Qin M, et al. Pubertal lead exposure affects ovary development, folliculogenesis and steroidogenesis by activation of IRE1α-JNK signaling pathway in rat. Ecotoxicol Environ Saf (2023) 257:114919. doi: 10.1016/j.ecoenv.2023.114919
185. Rodríguez-Díaz R, Blanes-Zamora R, Paz-Montelongo S, Gómez-Rodríguez J, Fiestas SR, González-Weller D, et al. The influence of follicular fluid metals on assisted reproduction outcome. Biol Trace Elem Res (2023) 201:5069–82. doi: 10.1007/s12011-023-03578-3
186. Wuri L, Burghardt RC, Arosh JA, Long CR, Banu SK. Hexavalent chromium disrupts oocyte development in rats by elevating oxidative stress, DNA double-strand breaks, microtubule disruption, and aberrant segregation of chromosomes. Int J Mol Sci (2023) 24(12):10003. doi: 10.3390/ijms241210003
187. Palomar A, González-Martín R, Pérez-Debén S, Medina-Laver Y, Quiñonero A, Domínguez F. Mercury impairs human primary endometrial stromal cell function†. Biol Reprod (2022) 106:1022–32. doi: 10.1093/biolre/ioac016
188. Ingaramo P, Alarcón R, Muñoz-De-Toro M, Luque EH. Are glyphosate and glyphosate-based herbicides endocrine disruptors that alter female fertility? Mol Cell Endocrinol (2020) 518:110934. doi: 10.1016/j.mce.2020.110934
189. Sharma RK, Singh P, Setia A, Sharma AK. Insecticides and ovarian functions. Environ Mol Mutagen (2020) 61:369–92. doi: 10.1002/em.22355
190. Ramos Nieto MR, Lasagna M, Cao G, Álvarez G, Santamaria C, Rodriguez Girault ME, et al. Chronic exposure to low concentrations of chlorpyrifos affects normal cyclicity and histology of the uterus in female rats. Food Chem Toxicol (2021) 156:112515. doi: 10.1016/j.fct.2021.112515
191. Sharma A, Kaninathan A, Dahal S, Kumari S, Choudhary B, Raghavan SC. Exposure to endosulfan can cause long term effects on general biology, including the reproductive system of mice. Front Genet (2022) 13:1047746. doi: 10.3389/fgene.2022.1047746
192. Huang J, Hu L, Yang J. Dietary zinc intake and body mass index as modifiers of the association between household pesticide exposure and infertility among US women: a population-level study. Environ Sci pollut Res Int (2023) 30:20327–36. doi: 10.1007/s11356-022-23629-x
193. Zhu W, Zhou W, Huo X, Zhao S, Gan Y, Wang B, et al. Triclosan and female reproductive health: A preconceptional cohort study. Epidemiology (2019) 30 Suppl 1:S24–s31. doi: 10.1097/EDE.0000000000001011
194. Björvang RD, Hallberg I, Pikki A, Berglund L, Pedrelli M, Kiviranta H, et al. Follicular fluid and blood levels of persistent organic pollutants and reproductive outcomes among women undergoing assisted reproductive technologies. Environ Res (2022) 208:112626. doi: 10.1016/j.envres.2021.112626
195. Al-Hussaini TK, Abdelaleem AA, Elnashar I, Shabaan OM, Mostafa R, El-Baz MAH, et al. The effect of follicullar fluid pesticides and polychlorinated biphenyls concentrations on intracytoplasmic sperm injection (ICSI) embryological and clinical outcome. Eur J Obstet Gynecol Reprod Biol (2018) 220:39–43. doi: 10.1016/j.ejogrb.2017.11.003
196. Cohen NJ, Yao M, Midya V, India-Aldana S, Mouzica T, Andra SS, et al. Exposure to perfluoroalkyl substances and women’s fertility outcomes in a Singaporean population-based preconception cohort. Sci Total Environ (2023) 873:162267. doi: 10.1016/j.scitotenv.2023.162267
197. Hallberg I, Björvang RD, Hadziosmanovic N, Koekkoekk J, Pikki A, Van Duursen M, et al. Associations between lifestyle factors and levels of per- and polyfluoroalkyl substances (PFASs), phthalates and parabens in follicular fluid in women undergoing fertility treatment. J Expo Sci Environ Epidemiol. (2023) 33(5):699–709. doi: 10.1038/s41370-023-00579-1
198. Luo K, Liu X, Zhou W, Nian M, Qiu W, Yang Y, et al. Preconception exposure to perfluoroalkyl and polyfluoroalkyl substances and couple fecundity: A couple-based exploration. Environ Int (2022) 170:107567. doi: 10.1016/j.envint.2022.107567
199. Li T, Vazakidou P, Leonards PEG, Damdimopoulos A, Panagiotou EM, Arnelo C, et al. Identification of biomarkers and outcomes of endocrine disruption in human ovarian cortex using In Vitro Models. Toxicology (2023) 485:153425. doi: 10.1016/j.tox.2023.153425
200. Kanimozhi V, Palanivel K, Akbarsha MA, Kadalmani B. Molecular mechanisms of tributyltin-induced alterations in cholesterol homeostasis and steroidogenesis in hamster testis: In vivo and in vitro studies. J Cell Biochem (2018) 119:4021–37. doi: 10.1002/jcb.26564
201. Barbosa KL, Dettogni RS, Da Costa CS, Gastal EL, Raetzman LT, Flaws JA, et al. Tributyltin and the female hypothalamic-pituitary-gonadal disruption. Toxicol Sci (2022) 186:179–89. doi: 10.1093/toxsci/kfab141
202. Arya S, Dwivedi AK, Alvarado L, Kupesic-Plavsic S. Exposure of U.S. population to endocrine disruptive chemicals (Parabens, Benzophenone-3, Bisphenol-A and Triclosan) and their associations with female infertility. Environ Pollut (2020) 265:114763. doi: 10.1016/j.envpol.2020.114763
203. Ao J, Qiu W, Huo X, Wang Y, Wang W, Zhang Q, et al. Paraben exposure and couple fecundity: a preconception cohort study. Hum Reprod (2023) 38:726–38. doi: 10.1093/humrep/dead016
204. Siegel RL, Miller KD, Jemal A. Cancer statistics,. 2020. CA Cancer J Clin (2020) 70:7–30. doi: 10.3322/caac.21590
205. Sung H, Ferlay J, Siegel RL, Laversanne M, Soerjomataram I, Jemal A, et al. Global cancer statistics. 2020: GLOBOCAN estimates of incidence and mortality worldwide for 36 cancers in 185 countries. CA Cancer J Clin (2021) 71:209–49. doi: 10.3322/caac.21660
206. Fuentes N, Silveyra P. Estrogen receptor signaling mechanisms. Adv Protein Chem Struct Biol (2019) 116:135–70. doi: 10.1016/bs.apcsb.2019.01.001
207. Keshavarz-Maleki R, Kaviani A, Omranipour R, Gholami M, Khoshayand MR, Ostad SN, et al. Bisphenol-A in biological samples of breast cancer mastectomy and mammoplasty patients and correlation with levels measured in urine and tissue. Sci Rep (2021) 11:18411. doi: 10.1038/s41598-021-97864-6
208. Ansari MI, Bano N, Kainat KM, Singh VK, Sharma PK. Bisphenol A exposure induces metastatic aggression in low metastatic MCF-7 cells via PGC-1α mediated mitochondrial biogenesis and epithelial-mesenchymal plasticity. Life Sci (2022) 302:120649. doi: 10.1016/j.lfs.2022.120649
209. Zhang X, Cheng C, Zhang G, Xiao M, Li L, Wu S, et al. Co-exposure to BPA and DEHP enhances susceptibility of mammary tumors via up-regulating Esr1/HDAC6 pathway in female rats. Ecotoxicol Environ Saf (2021) 221:112453. doi: 10.1016/j.ecoenv.2021.112453
210. Liu G, Cai W, Liu H, Jiang H, Bi Y, Wang H. The association of bisphenol A and phthalates with risk of breast cancer: A meta-analysis. Int J Environ Res Public Health (2021) 18(5):2375. doi: 10.3390/ijerph18052375
211. Parada H Jr., Gammon MD, Chen J, Calafat AM, Neugut AI, Santella RM, et al. Urinary phthalate metabolite concentrations and breast cancer incidence and survival following breast cancer: the long island breast cancer study project. Environ Health Perspect (2018) 126:047013. doi: 10.1289/EHP2083
212. Segovia-Mendoza M, Palacios-Arreola MI, Monroy-Escamilla LM, Soto-Piña AE, Nava-Castro KE, Becerril-Alarcón Y, et al. Association of serum levels of plasticizers compounds, phthalates and bisphenols, in patients and survivors of breast cancer: A real connection? Int J Environ Res Public Health (2022) 19(13):8040. doi: 10.3390/ijerph19138040
213. Fiocchetti M, Bastari G, Cipolletti M, Leone S, Acconcia F, Marino M. The peculiar estrogenicity of diethyl phthalate: modulation of estrogen receptor α Activities in the proliferation of breast cancer cells. Toxics (2021) 9(10):237. doi: 10.3390/toxics9100237
214. Shan A, Leng L, Li J, Luo XM, Fan YJ, Yang Q, et al. TCDD-induced antagonism of MEHP-mediated migration and invasion partly involves aryl hydrocarbon receptor in MCF7 breast cancer cells. J Hazard Mater (2020) 398:122869. doi: 10.1016/j.jhazmat.2020.122869
215. Yang PJ, Hou MF, Tsai EM, Liang SS, Chiu CC, Ou-Yang F, et al. Breast cancer is associated with methylation and expression of the a disintegrin and metalloproteinase domain 33 (ADAM33) gene affected by endocrine−disrupting chemicals. Oncol Rep (2018) 40:2766–77. doi: 10.3892/or.2018.6675
216. Kanaya N, Bernal L, Chang G, Yamamoto T, Nguyen D, Wang YZ, et al. Molecular mechanisms of polybrominated diphenyl ethers (BDE-47, BDE-100, and BDE-153) in human breast cancer cells and patient-derived xenografts. Toxicol Sci (2019) 169:380–98. doi: 10.1093/toxsci/kfz054
217. He Y, Peng L, Zhang W, Liu C, Yang Q, Zheng S, et al. Adipose tissue levels of polybrominated diphenyl ethers and breast cancer risk in Chinese women: A case-control study. Environ Res (2018) 167:160–8. doi: 10.1016/j.envres.2018.07.009
218. Wei J, Li X, Xiang L, Song Y, Liu Y, Jiang Y, et al. Metabolomics and lipidomics study unveils the impact of polybrominated diphenyl ether-47 on breast cancer mice. J Hazard Mater (2020) 390:121451. doi: 10.1016/j.jhazmat.2019.121451
219. Hurley S, Goldberg D, Park JS, Petreas M, Bernstein L, Anton-Culver H, et al. A breast cancer case-control study of polybrominated diphenyl ether (PBDE) serum levels among California women. Environ Int (2019) 127:412–9. doi: 10.1016/j.envint.2019.03.043
220. Mancini FR, Cano-Sancho G, Gambaretti J, Marchand P, Boutron-Ruault MC, Severi G, et al. Perfluorinated alkylated substances serum concentration and breast cancer risk: Evidence from a nested case-control study in the French E3N cohort. Int J Cancer (2020) 146:917–28. doi: 10.1002/ijc.32357
221. Li AJ, Feldman SM, Mcnally RK, Kannan K. Distribution of organohalogen and synthetic musk compounds in breast adipose tissue of breast cancer patients in ulster county, new york, USA. Arch Environ Contam Toxicol (2019) 77:68–78. doi: 10.1007/s00244-019-00621-0
222. Kass L, Gomez AL, Altamirano GA. Relationship between agrochemical compounds and mammary gland development and breast cancer. Mol Cell Endocrinol (2020) 508:110789. doi: 10.1016/j.mce.2020.110789
223. Liu H, Sun Y, Ran L, Li J, Shi Y, Mu C, et al. Endocrine-disrupting chemicals and breast cancer: a meta-analysis. Front Oncol (2023) 13:1282651. doi: 10.3389/fonc.2023.1282651
224. Parada H Jr., Gammon MD, Ettore HL, Chen J, Calafat AM, Neugut AI, et al. Urinary concentrations of environmental phenols and their associations with breast cancer incidence and mortality following breast cancer. Environ Int (2019) 130:104890. doi: 10.1016/j.envint.2019.05.084
225. Shen X, Liang J, Zheng L, Wang H, Wang Z, Ji Q, et al. Ultrasound-assisted dispersive liquid-liquid microextraction followed by gas chromatography-mass spectrometry for determination of parabens in human breast tumor and peripheral adipose tissue. J Chromatogr B Analyt Technol BioMed Life Sci (2018) 1096:48–55. doi: 10.1016/j.jchromb.2018.08.004
226. Amin MM, Tabatabaeian M, Chavoshani A, Amjadi E, Hashemi M, Ebrahimpour K, et al. Paraben content in adjacent normal-malignant breast tissues from women with breast cancer. BioMed Environ Sci (2019) 32:893–904. doi: 10.3967/bes2019.112
227. Majhi PD, Sharma A, Roberts AL, Daniele E, Majewski AR, Chuong LM, et al. Effects of benzophenone-3 and propylparaben on estrogen receptor-dependent R-loops and DNA damage in breast epithelial cells and mice. Environ Health Perspect (2020) 128:17002. doi: 10.1289/EHP5221
228. Mogus JP, Laplante CD, Bansal R, Matouskova K, Schneider BR, Daniele E, et al. Exposure to propylparaben during pregnancy and lactation induces long-term alterations to the mammary gland in mice. Endocrinology (2021) 162(6):bqab041. doi: 10.1210/endocr/bqab041
229. Wu AH, Franke AA, Wilkens LR, Tseng C, Conroy SM, Li Y, et al. Risk of breast cancer and prediagnostic urinary excretion of bisphenol A, triclosan and parabens: The Multiethnic Cohort Study. Int J Cancer (2021) 149:1426–34. doi: 10.1002/ijc.33692
230. Rylander C, Veierød MB, Weiderpass E, Lund E, Sandanger TM. Use of skincare products and risk of cancer of the breast and endometrium: a prospective cohort study. Environ Health (2019) 18:105. doi: 10.1186/s12940-019-0547-6
231. Taylor KW, Troester MA, Herring AH, Engel LS, Nichols HB, Sandler DP, et al. Associations between personal care product use patterns and breast cancer risk among white and black women in the sister study. Environ Health Perspect (2018) 126:027011. doi: 10.1289/EHP1480
232. Rodgers KM, Udesky JO, Rudel RA, Brody JG. Environmental chemicals and breast cancer: An updated review of epidemiological literature informed by biological mechanisms. Environ Res (2018) 160:152–82. doi: 10.1016/j.envres.2017.08.045
233. Hager E, Chen J, Zhao L. Minireview: parabens exposure and breast cancer. Int J Environ Res Public Health (2022) 19(3):1873. doi: 10.3390/ijerph19031873
234. Vopham T, Bertrand KA, Jones RR, Deziel NC, Dupré NC, James P, et al. Dioxin exposure and breast cancer risk in a prospective cohort study. Environ Res (2020) 186:109516. doi: 10.1016/j.envres.2020.109516
235. Danjou AMN, Coudon T, Praud D, Lévêque E, Faure E, Salizzoni P, et al. Long-term airborne dioxin exposure and breast cancer risk in a case-control study nested within the French E3N prospective cohort. Environ Int (2019) 124:236–48. doi: 10.1016/j.envint.2019.01.001
236. Pierozan P, Jerneren F, Karlsson O. Perfluorooctanoic acid (PFOA) exposure promotes proliferation, migration and invasion potential in human breast epithelial cells. Arch Toxicol (2018) 92:1729–39. doi: 10.1007/s00204-018-2181-4
237. Hurley S, Goldberg D, Wang M, Park JS, Petreas M, Bernstein L, et al. Breast cancer risk and serum levels of per- and poly-fluoroalkyl substances: a case-control study nested in the California Teachers Study. Environ Health (2018) 17:83. doi: 10.1186/s12940-018-0426-6
238. Su Y, Santucci-Pereira J, Dang NM, Kanefsky J, Rahulkannan V, Hillegass M, et al. Effects of pubertal exposure to butyl benzyl phthalate, perfluorooctanoic acid, and zeranol on mammary gland development and tumorigenesis in rats. Int J Mol Sci (2022) 23(3):1398. doi: 10.3390/ijms23031398
239. Parada H Jr., Sun X, Tse CK, Engel LS, Olshan AF, Troester MA. Plasma levels of dichlorodiphenyldichloroethene (DDE) and dichlorodiphenyltrichloroethane (DDT) and survival following breast cancer in the Carolina Breast Cancer Study. Environ Int (2019) 125:161–71. doi: 10.1016/j.envint.2019.01.032
240. Cohn BA, Cirillo PM, Terry MB. DDT and breast cancer: prospective study of induction time and susceptibility windows. J Natl Cancer Inst (2019) 111:803–10. doi: 10.1093/jnci/djy198
241. Rusiecki JA, Denic-Roberts H, Byrne C, Cash J, Raines CF, Brinton LA, et al. Serum concentrations of DDE, PCBs, and other persistent organic pollutants and mammographic breast density in Triana, Alabama, a highly exposed population. Environ Res (2020) 182:109068. doi: 10.1016/j.envres.2019.109068
242. Huang W, He Y, Xiao J, Huang Y, Li A, He M, et al. Risk of breast cancer and adipose tissue concentrations of polychlorinated biphenyls and organochlorine pesticides: a hospital-based case-control study in Chinese women. Environ Sci pollut Res Int (2019) 26:32128–36. doi: 10.1007/s11356-019-06404-3
243. Filippini T, Torres D, Lopes C, Carvalho C, Moreira P, Naska A, et al. Cadmium exposure and risk of breast cancer: A dose-response meta-analysis of cohort studies. Environ Int (2020) 142:105879. doi: 10.1016/j.envint.2020.105879
244. Tarhonska K, Lesicka M, Janasik B, Roszak J, Reszka E, Braun M, et al. Cadmium and breast cancer - Current state and research gaps in the underlying mechanisms. Toxicol Lett (2022) 361:29–42. doi: 10.1016/j.toxlet.2022.03.003
245. Shan Z, Wei Z, Shaikh ZA. Suppression of ferroportin expression by cadmium stimulates proliferation, EMT, and migration in triple-negative breast cancer cells. Toxicol Appl Pharmacol (2018) 356:36–43. doi: 10.1016/j.taap.2018.07.017
246. Wei Z, Shan Z, Shaikh ZA. Epithelial-mesenchymal transition in breast epithelial cells treated with cadmium and the role of Snail. Toxicol Appl Pharmacol (2018) 344:46–55. doi: 10.1016/j.taap.2018.02.022
247. Kehm RD, Oskar S, Tehranifar P, Zeinomar N, Rundle AG, Herbstman JB, et al. Associations of prenatal exposure to polycyclic aromatic hydrocarbons with pubertal timing and body composition in adolescent girls: Implications for breast cancer risk. Environ Res (2021) 196:110369. doi: 10.1016/j.envres.2020.110369
248. Gearhart-Serna LM, Davis JB, Jolly MK, Jayasundara N, Sauer SJ, Di Giulio RT, et al. A polycyclic aromatic hydrocarbon-enriched environmental chemical mixture enhances AhR, antiapoptotic signaling and a proliferative phenotype in breast cancer cells. Carcinogenesis (2020) 41:1648–59. doi: 10.1093/carcin/bgaa047
249. Neff AM, Blanco SC, Flaws JA, Bagchi IC, Bagchi MK. Chronic exposure of mice to bisphenol-A alters uterine fibroblast growth factor signaling and leads to aberrant epithelial proliferation. Endocrinology (2019) 160:1234–46. doi: 10.1210/en.2018-00872
250. Caserta D, De Marco MP, Besharat AR, Costanzi F. Endocrine disruptors and endometrial cancer: molecular mechanisms of action and clinical implications, a systematic review. Int J Mol Sci (2022) 23(6):2956. doi: 10.3390/ijms23062956
251. Leung YK, Biesiada J, Govindarajah V, Ying J, Kendler A, Medvedovic M, et al. Low-dose bisphenol A in a rat model of endometrial cancer: A CLARITY-BPA study. Environ Health Perspect (2020) 128:127005. doi: 10.1289/EHP6875
252. Michalczyk K, Kapczuk P, Witczak G, Bosiacki M, Kurzawski M, Chlubek D, et al. The associations between metalloestrogens, GSTP1, and SLC11A2 polymorphism and the risk of endometrial cancer. Nutrients (2022) 14(15):3079. doi: 10.3390/nu14153079
253. Michalczyk K, Kupnicka P, Witczak G, Tousty P, Bosiacki M, Kurzawski M, et al. Assessment of cadmium (Cd) and lead (Pb) blood concentration on the risk of endometrial cancer. Biol (Basel) (2023) 12(5):717. doi: 10.3390/biology12050717
254. Razumova Z, Govorov I, Östensson E, Mints M. Cadmium intake as a prognostic factor in endometrial cancer: A swedish cohort-based study. Nutr Cancer (2022) 74:175–84. doi: 10.1080/01635581.2021.1883681
255. Atakul T, Altinkaya SO, Abas BI, Yenisey C. Serum copper and zinc levels in patients with endometrial cancer. Biol Trace Elem Res (2020) 195:46–54. doi: 10.1007/s12011-019-01844-x
256. Michalczyk K, Kapczuk P, Kupnicka P, Witczak G, Michalczyk B, Bosiacki M, et al. Assessment of serum zn, cu, mn, and fe concentration in women with endometrial cancer and different endometrial pathologies. Nutrients (2023) 15(16):3605. doi: 10.3390/nu15163605
257. Sarink D, Franke AA, White KK, Wu AH, Cheng I, Quon B, et al. BPA, parabens, and phthalates in relation to endometrial cancer risk: A case-control study nested in the multiethnic cohort. Environ Health Perspect (2021) 129:57702. doi: 10.1289/EHP8998
258. Wen HJ, Chang TC, Ding WH, Tsai SF, Hsiung CA, Wang SL. Exposure to endocrine disruptor alkylphenols and the occurrence of endometrial cancer. Environ Pollut (2020) 267:115475. doi: 10.1016/j.envpol.2020.115475
259. Ali AT, Al-Ani O, Al-Ani F. Epidemiology and risk factors for ovarian cancer. Prz Menopauzalny (2023) 22:93–104. doi: 10.5114/pm.2023.128661
260. Zahra A, Kerslake R, Kyrou I, Randeva HS, Sisu C, Karteris E. Impact of environmentally relevant concentrations of bisphenol A (BPA) on the gene expression profile in an in vitro model of the normal human ovary. Int J Mol Sci (2022) 23(10):5334. doi: 10.3390/ijms23105334
261. Zahra A, Dong Q, Hall M, Jeyaneethi J, Silva E, Karteris E, et al. Identification of potential bisphenol A (BPA) exposure biomarkers in ovarian cancer. J Clin Med (2021) 10(9):1979. doi: 10.3390/jcm10091979
262. Sawicka E, Saczko J, Kulbacka J, Szydełko M, Szymańska B, Piwowar A. The influence of interaction between cadmium with 17β-estradiol,. 2-methoxyestradiol and 16α-hydroxyestrone on viability and p-glycoprotein in ovarian cancer cell line. Int J Mol Sci (2022) 23(5):2628. doi: 10.3390/ijms23052628
263. Liu X, Zhan T, Gao Y, Cui S, Liu W, Zhang C, et al. Benzophenone-1 induced aberrant proliferation and metastasis of ovarian cancer cells via activated ERα and Wnt/β-catenin signaling pathways. Environ Pollut (2022) 292:118370. doi: 10.1016/j.envpol.2021.118370
264. Medellín-Garibay SE, Alcántara-Quintana LE, Rodríguez-Báez AS, Sagahón-Azúa J, Rodríguez-Aguilar M, Hernández Cueto MLA, et al. Urinary phthalate metabolite and BPA concentrations in women with cervical cancer. Environ Sci pollut Res Int (2023) 30:21033–42. doi: 10.1007/s11356-022-23654-w
265. Vander Borght M, Wyns C. Fertility and infertility: Definition and epidemiology. Clin Biochem (2018) 62:2–10. doi: 10.1016/j.clinbiochem.2018.03.012
266. Babakhanzadeh E, Nazari M, Ghasemifar S, Khodadadian A. Some of the factors involved in male infertility: A prospective review. Int J Gen Med (2020) 13:29–41. doi: 10.2147/IJGM.S241099
267. Murshidi MM, Choy JT, Eisenberg ML. Male infertility and somatic health. Urol Clin North Am (2020) 47:211–7. doi: 10.1016/j.ucl.2019.12.008
268. Caporossi L, Viganò P, Paci E, Capanna S, Alteri A, Pigini D, et al. A case-control study on the effects of plasticizers exposure on male fertility. Int J Environ Res Public Health (2022) 20(1):235. doi: 10.3390/ijerph20010235
269. Zhang L, Dong L, Ding S, Qiao P, Wang C, Zhang M, et al. Effects of n-butylparaben on steroidogenesis and spermatogenesis through changed E2 levels in male rat offspring. Environ Toxicol Pharmacol (2014) 37:705–17. doi: 10.1016/j.etap.2014.01.016
270. Gaspari L, Tessier B, Paris F, Bergougnoux A, Hamamah S, Sultan C, et al. Endocrine-disrupting chemicals and disorders of penile development in humans. Sex Dev (2021) 15:213–28. doi: 10.1159/000517157
271. Cannarella R, Gül M, Rambhatla A, Agarwal A. Temporal decline of sperm concentration: role of endocrine disruptors. Endocrine (2023) 79:1–16. doi: 10.1007/s12020-022-03136-2
272. Palak E, Lebiedzińska W, Anisimowicz S, Sztachelska M, Pierzyński P, Wiczkowski W, et al. The association between bisphenol A, steroid hormones, and selected microRNAs levels in seminal plasma of men with infertility. J Clin Med (2021) 10(24):5945. doi: 10.3390/jcm10245945
273. Jiang X, Yin L, Zhang N, Han F, Liu WB, Zhang X, et al. Bisphenol A induced male germ cell apoptosis via IFNβ-XAF1-XIAP pathway in adult mice. Toxicol Appl Pharmacol (2018) 355:247–56. doi: 10.1016/j.taap.2018.07.009
274. Liu X, Wang Z, Liu F. Chronic exposure of BPA impairs male germ cell proliferation and induces lower sperm quality in male mice. Chemosphere (2021) 262:127880. doi: 10.1016/j.chemosphere.2020.127880
275. Mostari MH, Rahaman MM, Akhter MA, Ali MH, Sasanami T, Tokumoto T. Transgenerational effects of bisphenol A on zebrafish reproductive tissues and sperm motility. Reprod Toxicol (2022) 109:31–8. doi: 10.1016/j.reprotox.2022.02.005
276. Song X, Miao M, Zhou X, Li D, Tian Y, Liang H, et al. Bisphenol A exposure and sperm ACHE hydroxymethylation in men. Int J Environ Res Public Health (2019) 16(1):152. doi: 10.3390/ijerph16010152
277. Ryu DY, Pang WK, Adegoke EO, Rahman MS, Park YJ, Pang MG. Abnormal histone replacement following BPA exposure affects spermatogenesis and fertility sequentially. Environ Int (2022) 170:107617. doi: 10.1016/j.envint.2022.107617
278. Zeng JY, Chen PP, Liu C, Deng YL, Miao Y, Zhang M, et al. Bisphenol A analogues in associations with serum hormone levels among reproductive-aged Chinese men. Environ Int (2022) 167:107446. doi: 10.1016/j.envint.2022.107446
279. Kim HK, Ko DH, Lee W, Kim KR, Chun S, Song J, et al. Body fluid concentrations of bisphenol A and their association with in vitro fertilization outcomes. Hum Fertil (Camb) (2021) 24:199–207. doi: 10.1080/14647273.2019.1612104
280. Caporossi L, Alteri A, Campo G, Paci E, Tranfo G, Capanna S, et al. Cross sectional study on exposure to BPA and phthalates and semen parameters in men attending a fertility center. Int J Environ Res Public Health (2020) 17(2):489. doi: 10.3390/ijerph18041742
281. Benson TE, Gaml-Sørensen A, Ernst A, Brix N, Hougaard KS, Hærvig KK, et al. Urinary bisphenol A, F and S levels and semen quality in young adult danish men. Int J Environ Res Public Health (2021) 18(4):1742. doi: 10.1038/s41598-018-35787-5
282. Ji H, Miao M, Liang H, Shi H, Ruan D, Li Y, et al. Exposure of environmental Bisphenol A in relation to routine sperm parameters and sperm movement characteristics among fertile men. Sci Rep (2018) 8:17548. doi: 10.1016/j.envint.2018.09.020
283. Joensen UN, Jørgensen N, Thyssen JP, Szecsi PB, Stender S, Petersen JH, et al. Urinary excretion of phenols, parabens and benzophenones in young men: Associations to reproductive hormones and semen quality are modified by mutations in the Filaggrin gene. Environ Int (2018) 121:365–74. doi: 10.1016/j.envres.2021.111289
284. Kiwitt-Cárdenas J, Adoamnei E, Arense-Gonzalo JJ, Sarabia-Cos L, Vela-Soria F, Fernández MF, et al. Associations between urinary concentrations of bisphenol A and sperm DNA fragmentation in young men. Environ Res (2021) 199:111289. doi: 10.1016/j.fct.2019.02.016
285. Mantzouki C, Bliatka D, Iliadou PK, Margeli A, Papassotiriou I, Mastorakos G, et al. Serum Bisphenol A concentrations in men with idiopathic infertility. Food Chem Toxicol (2019) 125:562–5. doi: 10.3390/ijerph15081770
286. Tian Y, Zhou X, Miao M, Li DK, Wang Z, Li R, et al. Association of bisphenol A exposure with LINE-1 hydroxymethylation in human semen. Int J Environ Res Public Health (2018) 15(8):1770. doi: 10.3389/fgene.2019.01001
287. Zhang W, Li M, Sun F, Xu X, Zhang Z, Liu J, et al. Association of sperm methylation at LINE-1, four candidate genes, and nicotine/alcohol exposure with the risk of infertility. Front Genet (2019) 10:1001. doi: 10.1016/j.scitotenv.2020.140673
288. Wang B, Qin X, Xiao N, Yao Y, Duan Y, Cui X, et al. Phthalate exposure and semen quality in infertile male population from Tianjin, China: Associations and potential mediation by reproductive hormones. Sci Total Environ (2020) 744:140673. doi: 10.3390/antiox11081617
289. Virant-Klun I, Imamovic-Kumalic S, Pinter B. From oxidative stress to male infertility: review of the associations of endocrine-disrupting chemicals (Bisphenols, phthalates, and parabens) with human semen quality. Antioxidants (Basel) (2022) 11(8):1617. doi: 10.1016/j.envres.2018.07.004
290. Smarr MM, Kannan K, Sun L, Honda M, Wang W, Karthikraj R, et al. Preconception seminal plasma concentrations of endocrine disrupting chemicals in relation to semen quality parameters among male partners planning for pregnancy. Environ Res (2018) 167:78–86. doi: 10.1016/j.envpol.2022.118969
291. Xiong F, Zhou B, Wu NX, Deng LJ, Xie JY, Li XJ, et al. The association of certain seminal phthalate metabolites on spermatozoa apoptosis: an exploratory mediation analysis via sperm protamine. Environ pollut (2022) 300:118969. doi: 10.1016/j.bcp.2022.115249
292. Cosci I, Garolla A, Cabrelle A, Sut S, Dall'acqua S, Ferlin A, et al. Lipophilic phthalic acid esters impair human sperm acrosomal reaction through the likely inhibition of phospholipase A(2)-signaling pathway. Biochem Pharmacol (2022) 205:115249. doi: 10.3390/toxics11050448
293. Zhou F, Guo C, Wang L, Zhang G, Wang J, Chen W, et al. Mono-(2-ethylhexyl) Phthalate (MEHP)-Induced Telomere Structure and Function Disorder Mediates Cell Cycle Dysregulation and Apoptosis via c-Myc and Its Upstream Transcription Factors in a Mouse Spermatogonia-Derived (GC-1) Cell Line. Toxics (2023) 11(5):448. doi: 10.1016/j.chemosphere.2019.05.017
294. Mondal S, Ghosh S, Bhattacharya S, Mukherjee S. Chronic dietary administration of lower levels of diethyl phthalate induces murine testicular germ cell inflammation and sperm pathologies: Involvement of oxidative stress. Chemosphere (2019) 229:443–51. doi: 10.1016/j.chemosphere.2022.134469
295. Zeng JY, Miao Y, Liu C, Deng YL, Chen PP, Zhang M, et al. Serum multiple organochlorine pesticides in relation to testosterone concentrations among Chinese men from an infertility clinic. Chemosphere (2022) 299:134469. doi: 10.1016/j.tox.2021.153017
296. Knapke ET, Magalhaes DP, Dalvie MA, Mandrioli D, Perry MJ. Environmental and occupational pesticide exposure and human sperm parameters: A Navigation Guide review. Toxicology (2022) 465:153017. doi: 10.1016/j.toxlet.2018.08.015
297. Song Y, Yang L. Transgenerational impaired spermatogenesis with sperm H19 and Gtl2 hypomethylation induced by the endocrine disruptor p,p’-DDE. Toxicol Lett (2018) 297:34–41. doi: 10.1016/j.chemosphere.2022.135010
298. Miao Y, Zeng JY, Rong M, Li M, Zhang L, Liu C, et al. Organochlorine pesticide exposures, metabolic enzyme genetic polymorphisms and semen quality parameters among men attending an infertility clinic. Chemosphere (2022) 303:135010. doi: 10.1016/j.fct.2023.113991
299. Yang L, Mei G, Yang Y, Cui J, Peng S, Peng Z, et al. Hexachlorocyclohexane impairs human sperm motility by affecting lysine glutarylation and mitochondrial functions. Food Chem Toxicol (2023) 179:113991. doi: 10.1016/j.ijheh.2019.07.001
300. Figueroa ZI, Young HA, Mumford SL, Meeker JD, Barr DB, Gray GM, et al. Pesticide interactions and risks of sperm chromosomal abnormalities. Int J Hyg Environ Health (2019) 222:1021–9. doi: 10.1016/j.envres.2023.116825
301. Thacharodi A, Hassan S, Acharya G, Vithlani A, Hoang Le Q, Pugazhendhi A. Endocrine disrupting chemicals and their effects on the reproductive health in men. Environ Research (2023) 236:116825. doi: 10.3389/fendo.2023.1227836
302. Hamed MA, Akhigbe TM, Adeogun AE, Adesoye OB, Akhigbe RE. Impact of organophosphate pesticides exposure on human semen parameters and testosterone: a systematic review and meta-analysis. Front Endocrinol (Lausanne) (2023) 14:1227836. doi: 10.3390/metabo11120799
303. Moreira S, Pereira SC, Seco-Rovira V, Oliveira PF, Alves MG, Pereira ML. Pesticides and male fertility: A dangerous crosstalk. Metabolites (2021) 11(12):799. doi: 10.3390/ijms23158206
304. Moreira S, Silva R, Carrageta DF, Alves MG, Seco-Rovira V, Oliveira PF, et al. Carbamate pesticides: shedding light on their impact on the male reproductive system. Int J Mol Sci (2022) 23(15):8206. doi: 10.1111/and.14580
305. Ali Abd El-Rahman H, Omar AR. Ameliorative effect of avocado oil against lufenuron induced testicular damage and infertility in male rats. Andrologia (2022) 54:e14580. doi: 10.5455/OVJ.2023.v13.i7.9
306. Sasi SM, Alghoul NM, Awayn N, Elghoul A, Prastiya RA. Sperm abnormality and infertility in male mice treated with the recommended dose of dimethoate and its double. Open Vet J (2023) 13:873–8. doi: 10.1016/j.theriogenology.2023.01.019
307. Carranza-Martin AC, Fabra MC, Urrutia Luna N, Farnetano N, Anchordoquy JP, Anchordoquy JM, et al. In vitro adverse effects of amitraz on semen quality: Consequences in bovine embryo development. Theriogenology (2023) 199:106–13. doi: 10.1177/0748233718778665
308. Harchegani AB, Rahmani A, Tahmasbpour E, Kabootaraki HB, Rostami H, Shahriary A. Mechanisms of diazinon effects on impaired spermatogenesis and male infertility. Toxicol Ind Health (2018) 34:653–64. doi: 10.1016/j.pestbp.2020.104731
309. Ham J, You S, Lim W, Song G. Pyridaben induces mitochondrial dysfunction and leads to latent male reproductive abnormalities. Pestic Biochem Physiol (2021) 171:104731. doi: 10.1016/j.reprotox.2021.03.002
310. Bae JW, Kwon WS. The deleterious toxic effects of bifenthrin on male fertility. Reprod Toxicol (2021) 101:74–80. doi: 10.1016/j.ecoenv.2022.114163
311. Sun W, Tian F, Pan H, Chang X, Xia M, Hu J, et al. Flurochloridone induced abnormal spermatogenesis by damaging testicular Sertoli cells in mice. Ecotoxicol Environ Saf (2022) 246:114163. doi: 10.1016/j.reprotox.2020.04.002
312. Bae JW, Kwon WS. Investigating the effects of fipronil on male fertility: Insight into the mechanism of capacitation. Reprod Toxicol (2020) 94:1–7. doi: 10.1016/j.envpol.2020.114573
313. Ham J, You S, Lim W, Song G. Etoxazole induces testicular malfunction in mice by dysregulating mitochondrial function and calcium homeostasis. Environ Pollut (2020) 263:114573. doi: 10.1016/j.etap.2021.103724
314. Abdel-Razik RK, Mosallam EM, Hamed NA, Badawy MEI, Abo-El-Saad MM. Testicular deficiency associated with exposure to cypermethrin, imidacloprid, and chlorpyrifos in adult rats. Environ Toxicol Pharmacol (2021) 87:103724. doi: 10.1016/j.sjbs.2022.103541
315. Alamgir Kobir M, Akter L, Sultana N, Pervin M, Abdul Awal M, Rabiul Karim M. Effects of imidacloprid-contaminated feed exposure on spermatogenic cells and Leydig cells in testes of adult male rabbits (Oryctolagus cuniculus). Saudi J Biol Sci (2023) 30:103541. doi: 10.1016/j.fct.2021.112586
316. Pandey N, Maske P, Mote C, Dighe V. Exposure to Atrazine through gestation and lactation period led to impaired sexual maturation and subfertility in F1 male rats with congenital deformities in F2 progeny. Food Chem Toxicol (2021) 157:112586. doi: 10.3390/toxics11040330
317. Marcu D, Keyser S, Petrik L, Fuhrimann S, Maree L. Contaminants of emerging concern (CECs) and male reproductive health: challenging the future with a double-edged sword. Toxics (2023) 11(4):330. doi: 10.3389/fcell.2023.1173575
318. Sheikh IA, Beg MA, Hamoda TA, Mandourah HMS, Memili E. Androgen receptor signaling and pyrethroids: Potential male infertility consequences. Front Cell Dev Biol (2023) 11:1173575. doi: 10.1021/acs.jafc.8b03407
319. Chen R, Cui Y, Zhang X, Zhang Y, Chen M, Zhou T, et al. Chlorpyrifos induction of testicular-cell apoptosis through generation of reactive oxygen species and phosphorylation of AMPK. J Agric Food Chem (2018) 66:12455–70. doi: 10.1002/jat.3789
320. Hazarika J, Ganguly M, Mahanta R. Molecular interactions of chlorpyrifos and its environmental degradation products with human sex hormone-binding globulin: an in silico study. J Appl Toxicol (2019) 39:1002–11. doi: 10.1002/em.22242
321. Pallotta MM, Barbato V, Pinton A, Acloque H, Gualtieri R, Talevi R, et al. In vitro exposure to CPF affects bovine sperm epigenetic gene methylation pattern and the ability of sperm to support fertilization and embryo development. Environ Mol Mutagen (2019) 60:85–95. doi: 10.1080/07391102.2021.1875885
322. Hazarika J, Ganguly M, Borgohain G, Sarma S, Bhuyan P, Mahanta R. Disruption of androgen receptor signaling by chlorpyrifos (CPF) and its environmental degradation products: a structural insight. J Biomol Struct Dyn (2022) 40:6027–38. doi: 10.2147/RMHP.S175230
323. Lwin TZ, Than AA, Min AZ, Robson MG, Siriwong W. Effects of pesticide exposure on reproductivity of male groundnut farmers in Kyauk Kan village, Nyaung-U, Mandalay region, Myanmar. Risk Manag Healthc Policy (2018) 11:235–41. doi: 10.1016/j.chemosphere.2019.05.020
324. Li J, Pang G, Ren F, Fang B. Chlorpyrifos-induced reproductive toxicity in rats could be partly relieved under high-fat diet. Chemosphere (2019) 229:94–102. doi: 10.4236/jep.2018.91002
325. Eshete M, Bowleg J, Perales SG, Okunrobo M, Watkins D, Spencer H. Adsorption of propazine, simazine and bisphenol A on the surface of nanoparticles of iron oxide nanoparticles of carbon and metallic oxides. J Environ Prot (2018) 09:13–24. doi: 10.1002/jbt.22026
326. Girish BP, Reddy PS. Forskolin ameliorates mancozeb-induced testicular and epididymal toxicity in Wistar rats by reducing oxidative toxicity and by stimulating steroidogenesis. J Biochem Mol Toxicol (2018) 32(2). doi: 10.1016/j.reprotox.2021.01.010
327. Bae JW, Kwon WS. Piperonyl butoxide, a synergist of pesticides can elicit male-mediated reproductive toxicity. Reprod Toxicol (2021) 100:120–5. doi: 10.1016/j.chemosphere.2019.125580
328. Hu Y, Zhang Y, Vinturache A, Wang Y, Shi R, Chen L, et al. Effects of environmental pyrethroids exposure on semen quality in reproductive-age men in Shanghai, China. Chemosphere (2020) 245:125580. doi: 10.1177/07482337231209356
329. Alagöz M, Yazar S, Secilmis Canbay H, Acıkgöz A, Corba BS. Associations of semen quality with pyrethroids in semen and urine samples of men with oligozoospermia: A cross-sectional study in Samsun, Turkey. Toxicol Ind Health (2023) 39:754–61. doi: 10.1111/andr.13228
330. Giulioni C, Maurizi V, Castellani D, Scarcella S, Skrami E, Balercia G, et al. The environmental and occupational influence of pesticides on male fertility: A systematic review of human studies. Andrology (2022) 10:1250–71. doi: 10.1111/andr.12499
331. Samarasinghe S, Krishnan K, Naidu R, Megharaj M, Miller K, Fraser B, et al. Parabens generate reactive oxygen species in human spermatozoa. Andrology (2018) 6:532–41. doi: 10.3390/antiox9121302
332. Oliveira MM, Martins F, Silva MG, Correia E, Videira R, Peixoto F. Use of parabens (Methyl and butyl) during the gestation period: mitochondrial bioenergetics of the testes and antioxidant capacity alterations in testes and other vital organs of the F1 generation. Antioxidants (Basel) (2020) 9(12):1302. doi: 10.1080/01480545.2023.2176512
333. Martins FC, Oliveira MM, Gaivão I, Videira RA, Peixoto F. The administration of methyl and butyl parabens interferes with the enzymatic antioxidant system and induces genotoxicity in rat testis: possible relation to male infertility. Drug Chem Toxicol (2023) 8:1–8. doi: 10.1016/j.reprotox.2019.02.012
334. Henriques MC, Loureiro S, Fardilha M, Herdeiro MT. Exposure to mercury and human reproductive health: A systematic review. Reprod Toxicol (2019) 85:93–103. doi: 10.3390/antiox12081572
335. Moreira R, Martins AD, Alves MG, De Lourdes Pereira M, Oliveira PF. A comprehensive review of the impact of chromium picolinate on testicular steroidogenesis and antioxidant balance. Antioxidants (Basel) (2023) 12(8):1572. doi: 10.1097/JOM.0000000000001422
336. Ianos O, Sari-Minodier I, Villes V, Lehucher-Michel MP, Loundou A, Perrin J. Meta-analysis reveals the association between male occupational exposure to solvents and impairment of semen parameters. J Occup Environ Med (2018) 60:e533–42. doi: 10.1093/aje/kwy235
337. Petersen KU, Hansen J, Ebbehoej NE, Bonde JP. Infertility in a cohort of male danish firefighters: A register-based study. Am J Epidemiol (2019) 188:339–46. doi: 10.1016/j.scitotenv.2018.04.380
338. Jian JM, Chen D, Han FJ, Guo Y, Zeng L, Lu X, et al. A short review on human exposure to and tissue distribution of per- and polyfluoroalkyl substances (PFASs). Sci Total Environ (2018) 636:1058–69. doi: 10.1080/19396368.2018.1481465
339. Steves AN, Turry A, Gill B, Clarkson-Townsend D, Bradner JM, Bachli I, et al. Per- and polyfluoroalkyl substances impact human spermatogenesis in a stem-cell-derived model. Syst Biol Reprod Med (2018) 64:225–39. doi: 10.1080/01480545.2017.1320406
340. Matsuyama T, Yabe K, Kuwata C, Ito K, Ando Y, Iida H, et al. Transcriptional profile of ethylene glycol monomethyl ether-induced testicular toxicity in rats. Drug Chem Toxicol (2018) 41:105–12. doi: 10.1016/j.metabol.2018.03.022
341. Lymperi S, Giwercman A. Endocrine disruptors and testicular function. Metabolism (2018) 86:79–90. doi: 10.1016/j.beem.2021.101567
342. Stukenborg JB, Mitchell RT, Söder O. Endocrine disruptors and the male reproductive system. Best Pract Res Clin Endocrinol Metab (2021) 35:101567. doi: 10.1093/humrep/deaa045
343. Fisher BG, Thankamony A, Mendiola J, Petry CJ, Frederiksen H, Andersson AM, et al. Maternal serum concentrations of bisphenol A and propyl paraben in early pregnancy are associated with male infant genital development. Hum Reprod (2020) 35:913–28. doi: 10.3389/fendo.2021.694669
344. Komarowska MD, Grubczak K, Czerniecki J, Hermanowicz A, Hermanowicz JM, Debek W, et al. Identification of the bisphenol A (BPA) and the two analogues BPS and BPF in cryptorchidism. Front Endocrinol (Lausanne) (2021) 12:694669. doi: 10.1016/j.therap.2018.02.007
345. Tournaire M, Devouche E, Epelboin S, Cabau A, Dunbavand A, Levadou A. Birth defects in children of men exposed in utero to diethylstilbestrol (DES). Therapie (2018) 73:399–407. doi: 10.1016/j.jhazmat.2021.127547
346. Zhao T, Tang X, Li D, Zhao J, Zhou R, Shu F, et al. Prenatal exposure to environmentally relevant levels of PBDE-99 leads to testicular dysgenesis with steroidogenesis disorders. J Hazard Mater (2022) 424:127547. doi: 10.1002/bdr2.1865
347. Tan H, Wu G, Wang S, Lawless J, Sinn A, Chen D, et al. Prenatal exposure to atrazine induces cryptorchidism and hypospadias in F1 male mouse offspring. Birth Defects Res (2021) 113:469–84. doi: 10.1016/j.envint.2021.106815
348. Desalegn AA, Iszatt N, Stigum H, Jensen TK, Eggesbø M. A case-cohort study of perinatal exposure to potential endocrine disrupters and the risk of cryptorchidism in the Norwegian HUMIS study. Environ Int (2021) 157:106815. doi: 10.1007/s11356-019-06031-y
349. Rouget F, Kadhel P, Monfort C, Viel JF, Thome JP, Cordier S, et al. Chlordecone exposure and risk of congenital anomalies: the Timoun Mother-Child Cohort Study in Guadeloupe (French West Indies). Environ Sci pollut Res Int (2020) 27:40992–8. doi: 10.1093/humrep/deaa378
350. Le Moal J, Goria S, Guillet A, Rigou A, Chesneau J. Time and spatial trends of operated cryptorchidism in France and environmental hypotheses: a nationwide study from. 2002 to. 2014. Hum Reprod (2021) 36:1383–94. doi: 10.1371/journal.pone.0236394
351. Axelsson J, Scott K, Dillner J, Lindh CH, Zhang H, Rylander L, et al. Exposure to polychlorinated compounds and cryptorchidism; A nested case-control study. PloS One (2020) 15:e0236394. doi: 10.1016/j.taap.2022.116262
352. Li Q, Zhu Q, Tian F, Li J, Shi L, Yu Y, et al. In utero di-(2-ethylhexyl) phthalate-induced testicular dysgenesis syndrome in male newborn rats is rescued by taxifolin through reducing oxidative stress. Toxicol Appl Pharmacol (2022) 456:116262. doi: 10.1016/j.taap.2022.116262
353. Bougnères P, Porcher R, Esterle L, Baker D, de la Vaissière A, Meurisse S, et al. Exploring the risk of hypospadias in children born from mothers living close to a vineyard. PloS One (2021) 16:e0249800. doi: 10.1136/oemed-2022-108594
354. Das D, Dutta HK, Borbora D, Brahma RC, Das JM. Assessing the relationship between hypospadias risk and parental occupational exposure to potential endocrine-disrupting chemicals. Occup Environ Med (2023) 80:93–6. doi: 10.1016/j.scitotenv.2022.157084
355. Bornman R, Acerini CL, Chevrier J, Rauch S, Crause M, Obida M, et al. Maternal exposure to DDT, DDE, and pyrethroid insecticides for malaria vector control and hypospadias in the VHEMBE birth cohort study, Limpopo, South Africa. Sci Total Environ (2022) 845:157084. doi: 10.1093/toxsci/kfac059
356. Gray LE, Conley JM, Lambright CS, Furr JR. In utero exposure to a mixture of the perfluoroalkyl-isopropyl pesticide pyrifluquinazon with dibutyl phthalate cumulatively disrupts male rat reproductive development via different mechanisms of action. Toxicol Sci (2022) 188:234–47. doi: 10.1136/oemed-2019-105801
357. Cognez N, Warembourg C, Zaros C, Metten MA, Bouvier G, Garlantézec R, et al. Residential sources of pesticide exposure during pregnancy and the risks of hypospadias and cryptorchidism: the French ELFE birth cohort. Occup Environ Med (2019) 76:672–9. doi: 10.1016/j.chemosphere.2022.137343
358. Tysman M, Toppari J, Main KM, Adamsson A, Wohlfahrt-Veje C, Antignac JP, et al. Levels of persistent organic pollutants in breast milk samples representing Finnish and Danish boys with and without hypospadias. Chemosphere (2023) 313:137343. doi: 10.1056/NEJMcp2209151
359. Pinsky PF, Parnes H. Screening for prostate cancer. N Engl J Med (2023) 388:1405–14. doi: 10.1007/s40618-022-01983-4
360. Modica R, Benevento E, Colao A. Endocrine-disrupting chemicals (EDCs) and cancer: new perspectives on an old relationship. J Endocrinol Invest (2023) 46:667–77. doi: 10.1186/s12940-021-00779-y
361. Salamanca-Fernández E, Rodríguez-Barranco M, Amiano P, Delfrade J, Chirlaque MD, Colorado S, et al. Bisphenol-A exposure and risk of breast and prostate cancer in the Spanish European Prospective Investigation into Cancer and Nutrition study. Environ Health (2021) 20:88. doi: 10.1016/j.envres.2022.113142
362. Sánchez P, Castro B, Martínez-Rodríguez S, Ríos-Pelegrina R, Del Moral RG, Torres JM, et al. Impact of chronic exposure of rats to bisphenol A from perinatal period to adulthood on intraprostatic levels of 5α-reductase isozymes, aromatase, and genes implicated in prostate cancer development. Environ Res (2022) 212:113142. doi: 10.1016/j.envres.2022.113142
363. Cortés-Ramírez SA, Salazar AM, Sordo M, Ostrosky-Wegman P, Morales-Pacheco M, Cruz-Burgos M, et al. Transcriptome-wide analysis of low-concentration exposure to bisphenol A, S, and F in prostate cancer cells. Int J Mol Sci (2023) 24(11):9462. doi: 10.1016/j.envpol.2019.113318
364. Fatma Karaman E, Caglayan M, Sancar-Bas S, Ozal-Coskun C, Arda-Pirincci P, Ozden S. Global and region-specific post-transcriptional and post-translational modifications of bisphenol A in human prostate cancer cells. Environ Pollut (2019) 255:113318. doi: 10.1016/j.tox.2022.153178
365. Liu S, He B, Li H. Bisphenol S promotes the progression of prostate cancer by regulating the expression of COL1A1 and COL1A2. Toxicology (2022) 472:153178. doi: 10.1097/EE9.0000000000000231
366. Goldberg MS, Zapata-Marin S, Labrèche F, Ho V, Lavigne E, Valois MF, et al. Ambient exposures to selected volatile organic compounds and the risk of prostate cancer in Montreal. Environ Epidemiol (2022) 6:e231. doi: 10.1016/j.envres.2022.114869
367. Macedo S, Teixeira E, Gaspar TB, Boaventura P, Soares MA, Miranda-Alves L, et al. Endocrine-disrupting chemicals and endocrine neoplasia: A forty-year systematic review. Environ Res (2023) 218:114869. doi: 10.1016/j.envres.2019.108902
368. Chuang SC, Chen HC, Sun CW, Chen YA, Wang YH, Chiang CJ, et al. Phthalate exposure and prostate cancer in a population-based nested case-control study. Environ Res (2020) 181:108902. doi: 10.1016/j.urolonc.2023.04.022
369. Singh V, Madeshiya AK, Ansari NG, Singh MK, Abhishek A. CYP1A1 gene polymorphism and heavy metal analyses in benign prostatic hyperplasia and prostate cancer: An explorative case-control study. Urol Oncol (2023) 41:355.e9–355.e17. doi: 10.1007/s11356-023-26828-2
370. Cao HM, Yang YZ, Huang BY, Zhang Y, Wu Y, Wan Z, et al. A cross-sectional study of the association between heavy metals and pan-cancers associated with sex hormones in NHANES 1999-2018. Environ Sci pollut Res Int (2023) 30:61005–17. doi: 10.1016/j.envint.2019.105109
371. Lim JT, Tan YQ, Valeri L, Lee J, Geok PP, Chia SE, et al. Association between serum heavy metals and prostate cancer risk - A multiple metal analysis. Environ Int (2019) 132:105109. doi: 10.1016/j.envres.2023.117063
372. Hurwitz LM, Beane Freeman LE, Andreotti G, Hofmann JN, Parks CG, Sandler DP, et al. Joint associations between established genetic susceptibility loci, pesticide exposures, and risk of prostate cancer. Environ Res (2023) 237:117063. doi: 10.1016/j.envres.2023.115718
373. Rhee J, Barry KH, Huang WY, Sampson JN, Hofmann JN, Silverman DT, et al. A prospective nested case-control study of serum concentrations of per- and polyfluoroalkyl substances and aggressive prostate cancer risk. Environ Res (2023) 228:115718. doi: 10.1016/j.ecoenv.2023.115267
374. Wei Y, He H, Han T, Wang B, Ji P, Wu X, et al. Environmental explanation of prostate cancer progression based on the comprehensive analysis of perfluorinated compounds. Ecotoxicol Environ Saf (2023) 263:115267. doi: 10.3390/ijms23147900
375. Charazac A, Hinault C, Dolfi B, Hautier S, Decondé Le Butor C, Bost F, et al. Low doses of PFOA promote prostate and breast cancer cells growth through different pathways. Int J Mol Sci (2022) 23(14):7900. doi: 10.1016/j.fertnstert.2016.05.021
376. Anderson RE, Hanson HA, Patel DP, Johnstone E, Aston KI, Carrell DT, et al. Cancer risk in first- and second-degree relatives of men with poor semen quality. Fertil Steril (2016) 106:731–8. doi: 10.3389/fphys.2018.00406
377. Anand-Ivell R, Cohen A, Nørgaard-Pedersen B, Jönsson BAG, Bonde JP, Hougaard DM, et al. Amniotic fluid INSL3 measured during the critical time window in human pregnancy relates to cryptorchidism, hypospadias, and phthalate load: A large case-control study. Front Physiol (2018) 9:406. doi: 10.1002/vms3.443
378. Mutwedu VB, Nyongesa AW, Azine PC, Chiregereza DK, Ngoumtsop VH, Mugumaarhahama Y, et al. Growth performance and reproductive function impairment of glyphosate-based herbicide in male Guinea pig (Cavia porcellus). Vet Med Sci (2021) 7:1047–55. doi: 10.1002/vms3.443
379. Franco APS, Lima Figueiredo ER, Melo GS, Souza JSE, Gonçalves NV, Gomes FC, et al. Predictors of testicular cancer mortality in Brazil: A. 20-year ecological study. Cancers (Basel) (2023) 15(16):4149. doi: 10.1002/jbt.23058
380. Mitra S, Patra T, Saha D, Ghosh P, Mustafi SM, Varghese AC, et al. Sub-chronic cadmium and lead compound exposure induces reproductive toxicity and development of testicular germ cell neoplasia in situ in murine model: Attenuative effects of resveratrol. J Biochem Mol Toxicol (2022) 36:e23058. doi: 10.1016/j.chemosphere.2023.138698
381. Anđelković M, Djordjevic AB, Vukelić D, Đukić-Ćosić D, Aćimović M, Bojanić N, et al. Cadmium and lead implication in testis cancer; is there a connection? Chemosphere (2023) 330:138698. doi: 10.3892/ol.2019.10418
382. Ren Y, Shao W, Zuo L, Zhao W, Qin H, Hua Y, et al. Mechanism of cadmium poisoning on testicular injury in mice. Oncol Lett (2019) 18:1035–42. doi: 10.1007/s12015-023-10601-6
383. Kaushik A, Metkari SM, Ali S, Bhartiya D. Preventing/reversing adverse effects of endocrine disruption on mouse testes by normalizing tissue resident VSELs. Stem Cell Rev Rep (2023) 19(7):2525–40. doi: 10.1016/j.fct.2022.113004
384. Yawer A, Sychrová E, Raška J, Babica P, Sovadinová I. Endocrine-disrupting chemicals affect Sertoli TM4 cell functionality through dysregulation of gap junctional intercellular communication in vitro. Food Chem Toxicol (2022) 164:113004. doi: 10.1016/j.envres.2020.109787
385. Fernández-Martínez NF, Ching-López A, Olry De Labry Lima A, Salamanca-Fernández E, Pérez-Gómez B, Jiménez-Moleón JJ, et al. Relationship between exposure to mixtures of persistent, bioaccumulative, and toxic chemicals and cancer risk: A systematic review. Environ Res (2020) 188:109787. doi: 10.3390/ijerph17020489
Keywords: endocrine disrupting chemicals, reproductive system, hypothalamic-pituitary-gonadal axis, hormone-like effects, infertility
Citation: Pan J, Liu P, Yu X, Zhang Z and Liu J (2024) The adverse role of endocrine disrupting chemicals in the reproductive system. Front. Endocrinol. 14:1324993. doi: 10.3389/fendo.2023.1324993
Received: 20 October 2023; Accepted: 26 December 2023;
Published: 17 January 2024.
Edited by:
Spyridon N. Karras, Aristotle University of Thessaloniki, GreeceReviewed by:
Luís Pedro Rato, Instituto Politécnico da Guarda, PortugalParikipandla Sridevi, Indira Gandhi National Tribal University, India
Copyright © 2024 Pan, Liu, Yu, Zhang and Liu. This is an open-access article distributed under the terms of the Creative Commons Attribution License (CC BY). The use, distribution or reproduction in other forums is permitted, provided the original author(s) and the copyright owner(s) are credited and that the original publication in this journal is cited, in accordance with accepted academic practice. No use, distribution or reproduction is permitted which does not comply with these terms.
*Correspondence: Jinxing Liu, bGp4Mjc2QHNpbmEuY29t; Zhongming Zhang, emhvbmdtaW5nemhhbmdAaG90bWFpbC5jb20=
†These authors have contributed equally to this work and share last authorship