- 1College of Veterinary Medicine, Hunan Agricultural University, Changsha, China
- 2Guizhou Provincial Key Laboratory for Biodiversity Conservation and Utilization in the Fanjing Mountain Region, Tongren University, Tongren, China
- 3College of Animal Science and Technology, Northwest Agriculture and Forestry University, Yangling, China
The objective of the present study was to investigate the potential role of immunization against INH on follicular development, serum reproductive hormone (FSH, E2, and P4) concentrations, and reproductive performance in beef cattle. A total of 196 non-lactating female beef cattle (4-5 years old) with identical calving records (3 records) were immunized with 0.5, 1.0, 1.5, or 2.0 mg [(T1, n = 58), (T2, n = 46), (T3, n = 42) and (T4, n = 36), respectively] of the pcISI plasmid. The control (C) group (n = 14) was immunized with 1.0 mL 0.9% saline. At 21d after primary immunization, all beef cattle were boosted with half of the primary immunization dose. On day 10 after primary immunization, the beef cattle immunized with INH DNA vaccine evidently induced anti-INH antibody except for the T1 group. The T3 group had the greatest P/N value peak among all the groups. The anti-INH antibody positive rates in T2, T3 and T4 groups were significantly higher than that in C and T1 groups. RIA results indicated that serum FSH concentration in T2 group increased markedly on day 45 after booster immunization; the E2 amount in T3 group was significantly increased on day 10 after primary immunization, and the levels of E2 also improved in T2 and T3 groups after booster immunization; the P4 concentration in T2 group was significantly improved on day 21 after primary immunization. Ultrasonography results revealed that the follicles with different diameter sizes were increased, meanwhile, the diameter and growth speed of ovulatory follicle were significantly increased. Furthermore, the rates of estrous, ovulation, conception, and twinning rate were also significantly enhanced. These findings clearly illustrated that INH DNA vaccine was capable of promoting the follicle development, thereby improving the behavioral of estrous and ovulation, eventually leading to an augment in the conception rates and twinning rate of beef cattle.
1 Introduction
In the next decades, beef production needs to increase by 120% to feed a growing world population which was estimated by United Nations (1). The multiple ovulation and embryo transfer (MOET) technique has contributed to accelerate calf production. However, the variability in multiple ovulation response of embryo transfer protocols makes it not easy to obtain a suitable number of viable embryos from donors with high genetic potential at a given time (2). In addition, it has been reported that the repeated use of equine chorionic gonadotropins (eCGs) to induce superovulation is capable of leading to ovarian refractoriness and have clear negative effects on reproduction (3, 4).
Inhibins (INH) plays a vital role on the hypothalamus-pituitary gonadal (HPG) axis, which is secreted by sertoli cells of testis in males and granulosa cells of ovarian follicles in females (5, 6). INH has been proposed as an autocrine/paracrine factor that regulates follicular atresia, growth, steroidogenesis and gonadotropin responsiveness (7, 8). High concentrations of INH can exert negative impact on oocyte quality and embryo development by affecting follicle development (9, 10). Hence, passive and active immunizations against INH were performed to develop alternative methods for superovulation in domestic animals.
In the previous study, neutralization of endogenous INH through active immunization could increase the ovulation rate in several mammalian species, including sheep (11, 12), gilts (13), goats (14), and beef cattle (4). In buffaloes, immunization with AMH-INH-RFRP DNA vaccine could improve follicle development and fertility (15). Yet, the effects of active immunization against INH DNA vaccines on reproductive functions of beef cattle are not completely understood. Here, the current study aimed to investigate the potential role of gene immunization against INH on immune responses, follicle development, serum reproductive hormone (FSH, E2, and P4) concentrations, and evaluate the reproductive effect of this vaccine on estrous, ovulation, and conception rates in beef cattle. Our findings will provide new insights for exploring INH gene immunization technology to induce twins in beef cattle.
2 Materials and methods
2.1 Preparation of pCISI DNA vaccine
pCISI DNA vaccine has been constructed and preserved in our laboratory previously. Briefly, the synthesized porcine inhibin (INH) α (1–32) (NM_214189) fragment with NdeI and HindIII recognition sites at 5’ and 3’ ends was introduced into the S gene 3’ end of HBsAg-S and the resulting plasmid was named pCIS. Subsequently, PCR was performed to amplify the expected INH gene fragment (114 bp) from the pCIS plasmid, and the products were digested using BamHI. The INH fragment with BamHI recognition site at both ends was incorporated between 112 and 113 amino acid in the coding region of S gene of pCIS to construct the recombinant plasmid pCISI encoding HBsAg-S and two copies of INH fragments. Finally, pCISI plasmid was transformed into Escherichia coli (E. coli) DH5α producing pCISI DNA vaccine delivered by E. coli.
E. coli containing pCISI plasmid was inoculated in Luria-Bertani (LB) solid medium with ampicillin and cultured at 37°Cfor 16 h. Then, a single selected colony was randomly picked and added to LB liquid medium (5mL) with ampicillin (60 µg/mL) and cultured in 37°C shaker at 180 rpm/min for 6 h. The E. coli solution was transferred (1: 500) to LB liquid medium in large bottles and cultured in 37°C shaker at 180 rpm/min for 10 h. After harvesting, the DNA vaccines were extracted using EndoFree Maxi Plasmid Kit (Tiangen, Beijing, China) according to the manufacturer’s instructions.
2.2 Animals and vaccination
All animal experimental procedures were performed in accordance with the National Institutes of Health (NIH) Guidelines and the guide for the Chinese Association for Laboratory Animal Science. All cattle were treated according to the Ethical Committee of Animal Experiments, Hunan Agricultural University (No. 432022021). Nanyang cattle used in this study were raised under the same conditions at Tanghe Animal Husbandry Co. LTD. (Tanghe, China). A total of 196 non-lactating female beef cattle (4-5 years old) with identical calving records (3 records) were selected and divided into five groups: groups T1 (0.5 mg, n = 58), T2 (1.0 mg, n = 46), T3 (1.5 mg, n = 42) and T4 (2.0 mg, n = 36) which were intramuscular injection immunized at both the left and right buttock, respectively. The control (C) group (n = 14) was intramuscular injection immunized at both the left and right buttock with 1.0 mL 0.9% saline. At 21d after primary immunization, all beef cattle were boosted with half of the primary immunization dose. Blood was collected from the jugular vein on day 10 and 21 after primary immunization, on day 10 and 45 after booster immunization. Then, the blood samples were centrifuged at 3000 rpm under 4°Cfor 15 min to separate the serum, which were stored at -80°C for further analysis.
2.3 Synchronous estrus and ultrasonography detection
Estrus was synchronized in all the experimental Nanyang beef cattle with two injections of prostaglandin F2α (PGF2α; Prostamate; IVX Animal Health, MO, USA) administered 14 days apart, and the follicles with different diameter sizes (large follicles: ≥10mm in diameter; medium follicles: 7-9 mm in diameter; small follicles: 5-7 mm in diameter) were observed and recorded by daily ultrasonography. According to the records of daily ultrasonography, the growth speed of ovulatory follicles was analyzed. Oestrus was determined by the mounting and the obvious vaginal mucous discharge of beef cattle twice a day. Ovulation was confirmed by the abrupt disappearance of the previously detected dominant follicle on daily ultrasonography. Pregnancy diagnosis was performed on day 35 after artificial insemination (AI) to evaluate the number of embryos and twin rate.
2.4 Detection of antibodies against INH in serum
Specific IgG antibodies of serum were determined using an indirect ELISA with synthetic INHα (1-32) antigens (Sigma, USA) as standard antigens on day 10 and 21 after primary immunization, on day 10 and 21 after booster immunization. Briefly, 96-well ELISA plates (Gibco BRL, USA) were coated with 100 ng of standard antigen and kept at 4°C for 12 h. Then, the plate was washed with PBST (PBS with 0.1% Tween-20 (Bioroxx, Germany)) for 6 times, 200 μL of 1% (w/v) BSA in PBST was added into each well and blocked at 37°Cfor 1 h after discarding the reaction reagent. Next, 100 μL of serum samples (diluted to 1:50 with PBST) was added into each well and the plate was incubated at 37°C for 1 h. The bound antibody was detected by adding a second antibody (rabbit anti-bovine) IgG-HRP (diluted to 1:2000 with PBST, Sigma, USA) after washing 6 times with PBST, and then incubated at 37°Cfor 1 h. Finally, 150 μL of tetramethylbenzidine was added into each well and incubated at 37°C. After 30 min, the reaction was stopped with 2 M H2SO4 (50 μL/well), and the optical density was measured at 450 nm using a microplate reader (Bio-Rad, USA). ELISA results were analyzed in terms of P/N ratios, where P was used to denote the OD value of the test blood samples, and N was denoted the OD value of the negative control blood samples. The P/N ratios greater than 2.0, and OD values greater than 0.2 were considered positive responses (16).
2.5 Hormone determination in serum
Serum concentrations of E2, P4 and FSH were measured by radio-immunoassay (RIA) using commercial kits (Furui Bioengineering, China). The assay sensitivity and intra-assay CVs were 0.4 IU/L and 2.9% for FSH, 0.5 pg/mL and 7.3% for E2, 0.02 ng/mL and 8.8% for P4. All samples were measured in thrice.
2.6 Statistical analysis
All data were presented as means ± SEM and analyzed using SPSS Statistics 26.0 software (SPSS Software, Inc., Chicago, USA). Data was analyzed using one-way analysis of variance (ANOVA) test followed by Dunnett’s multiple comparison test and significant difference was inferred for p < 0.05 and extremely significant difference for p < 0.01.
3 Results
3.1 Detection of antibody in serum of beef cattle
As shown in Figure 1, the beef cattle immunized with INH DNA vaccine evidently induced anti-INH antibody, except for the T1 group on day 10 after primary immunization (PM10) compared with the C group. T3 group showed remarkably greater anti-INH antibody amounts than T1 and T2 groups (p < 0.05) on day 10, 21 after primary immunization (PM10, PM21) and on day 10, 45 after booster immunization (BM10, BM45).
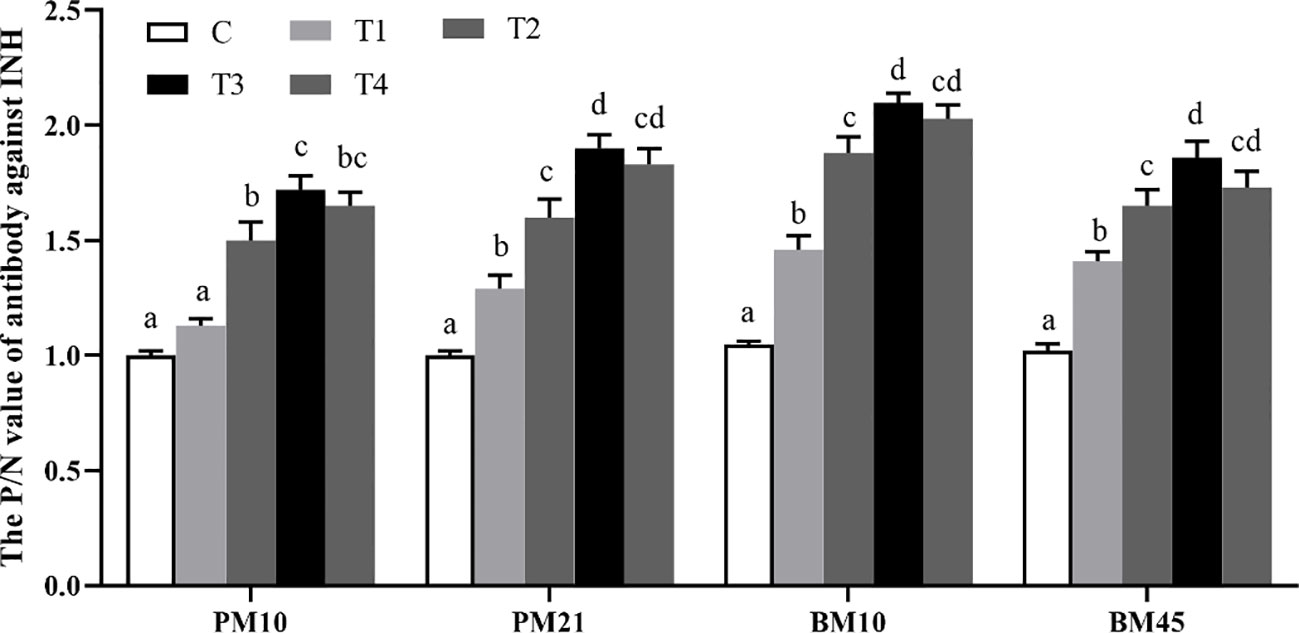
Figure 1 The P/N value of each dosage-group after INH gene immunization. Data are presented as mean ± SEM. The bars with different lower case letters indicate significant differences p < 0.05 among groups.
Furthermore, the positive rates of INH antibodies were calculated in each dosage group after primary immunization and booster immunization (Table 1). The anti-INH antibody positive rates in T2, T3, and T4 groups were significantly higher than those in C and T1 groups (p < 0.01 or p < 0.05) on day 10 and 21 after primary immunization. After booster immunization, the antibody positive rates in T2, T3, and T4 groups significantly increased compared to C and T1 groups (p < 0.01). Especially, there was a sharply increase in T3 group of the antibody positive rates compared to other groups (p < 0.05) after the booster immunization.
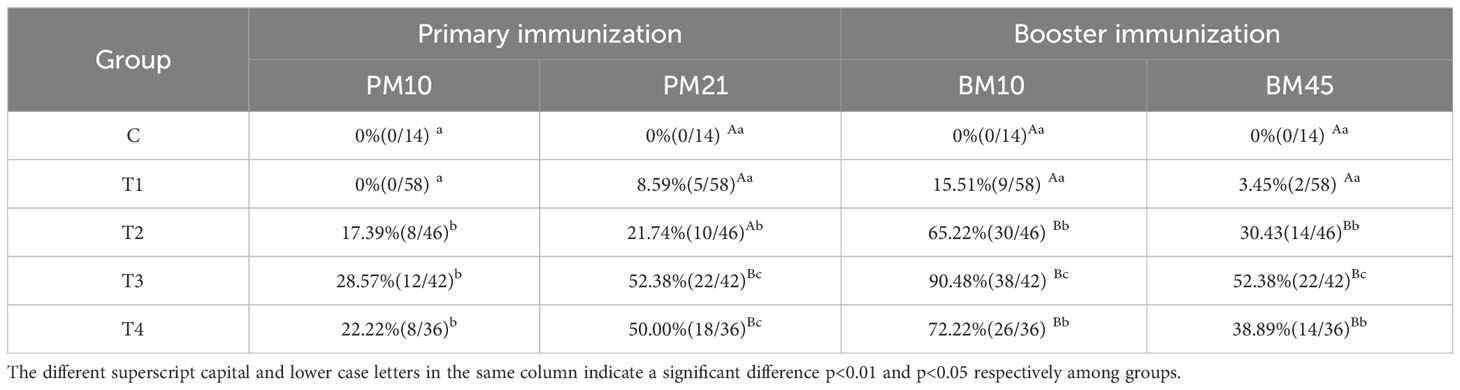
Table 1 Percentage (proportion) of the positive antibody after immunization with different vaccine concentrations.
3.2 Serum hormone concentrations in beef cattle
On day 45 after booster immunization, serum FSH concentration in T2 group increased markedly compared with C group (p<0.05); no significant difference was observed among all groups for FSH amounts on day 10, 21 after primary immunization and on day 10 after booster immunization (p>0.05) (Figure 2A). Compared with the C group, the E2 amount in T3 group was significantly increased by 20.26 pg/mL (p<0.05) on day 10 after primary immunization; levels of E2 also improved in T2 and T3 groups compared with the C group (p<0.05) on day 10 and 45 after booster immunization (Figure 2B). For the P4 concentration in serum, we observed that T2 group was significantly greater than C group (p<0.05) on day 21 after primary immunization (Figure 2C). In addition, we compared the differences of serum FSH, E2, and P4 amounts between the antibody-positive (P) and antibody-negative (N) beef cattle. The results revealed that there was no significant difference in the amount of FSH among groups (p>0.05) (Figure 2D). The concentrations of E2 in P group were significantly increased compared with those in N group of beef cattle after booster immunization (p<0.05) (Figure 2E). The concentrations of P4 in P group were significantly higher than those in N group of beef cattle on day 45 after booster immunization (p<0.05) (Figure 2F).
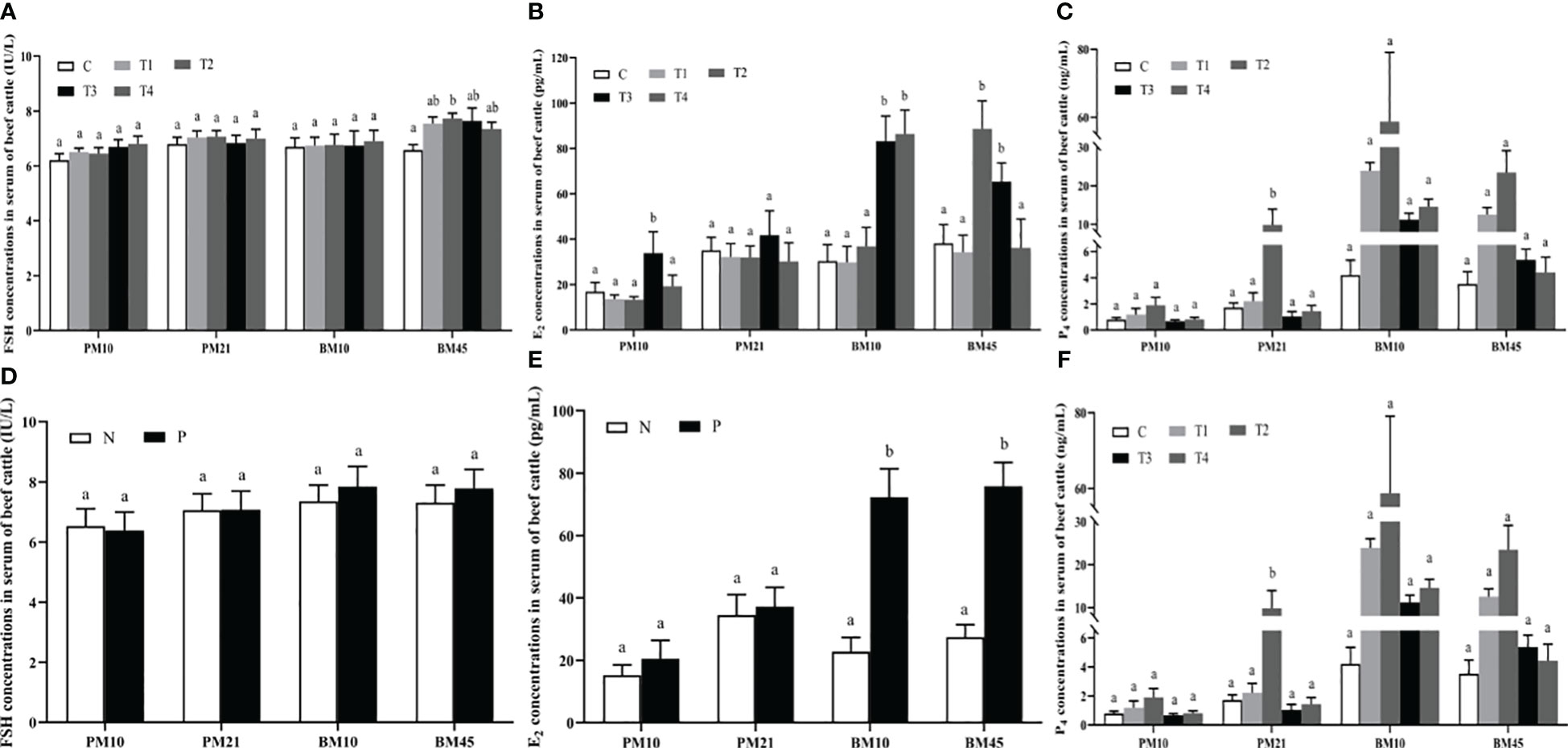
Figure 2 The effect of INH DNA vaccine on FSH (A, D), E2 (B, E), and P4 (C, F) levels in serum of beef cattle. Data are presented as mean ± SEM. The bars with different lower case letters indicate significant differences p < 0.05 among groups.
3.3 Effect of INH DNA vaccine on follicular development in beef cattle
Daily ultrasonography was performed to scan and record the number of follicles with different sizes, the diameter and growth speed of ovulatory follicles. As depicted in Figure 3A, the large follicles in T2, T3, and T4 groups had a significantly greater number compared to the C group, and greatest count was detected in T3 group (p < 0.05); The number of middle follicles in T1, T2, T3, and T4 groups was greater than that of C group (p < 0.05); The number of small follicles in T1, T2, and T3 groups was greater than that of C and T4 group (p < 0.05). The beef cattle in T2, T3, and T4 groups had a significantly greater diameter of ovulatory follicle compared to the C group (p < 0.05), and T3 group was significant greater than other groups (p < 0.05) (Figure 3B). The growth speed of ovulatory follicle in T1, T2, and T3 groups was much faster compared to C group (p < 0.05), and T2 group was significantly greater than other groups (p < 0.05) (Figure 3C). Furthermore, there was a significant increase of the large and small follicle counts in the P group compared to the N group (p < 0.05) (Figure 3D). The diameter and growth speed of ovulatory follicle in P group were significantly increased compared with those in N group (p < 0.05) (Figures 3E, F).
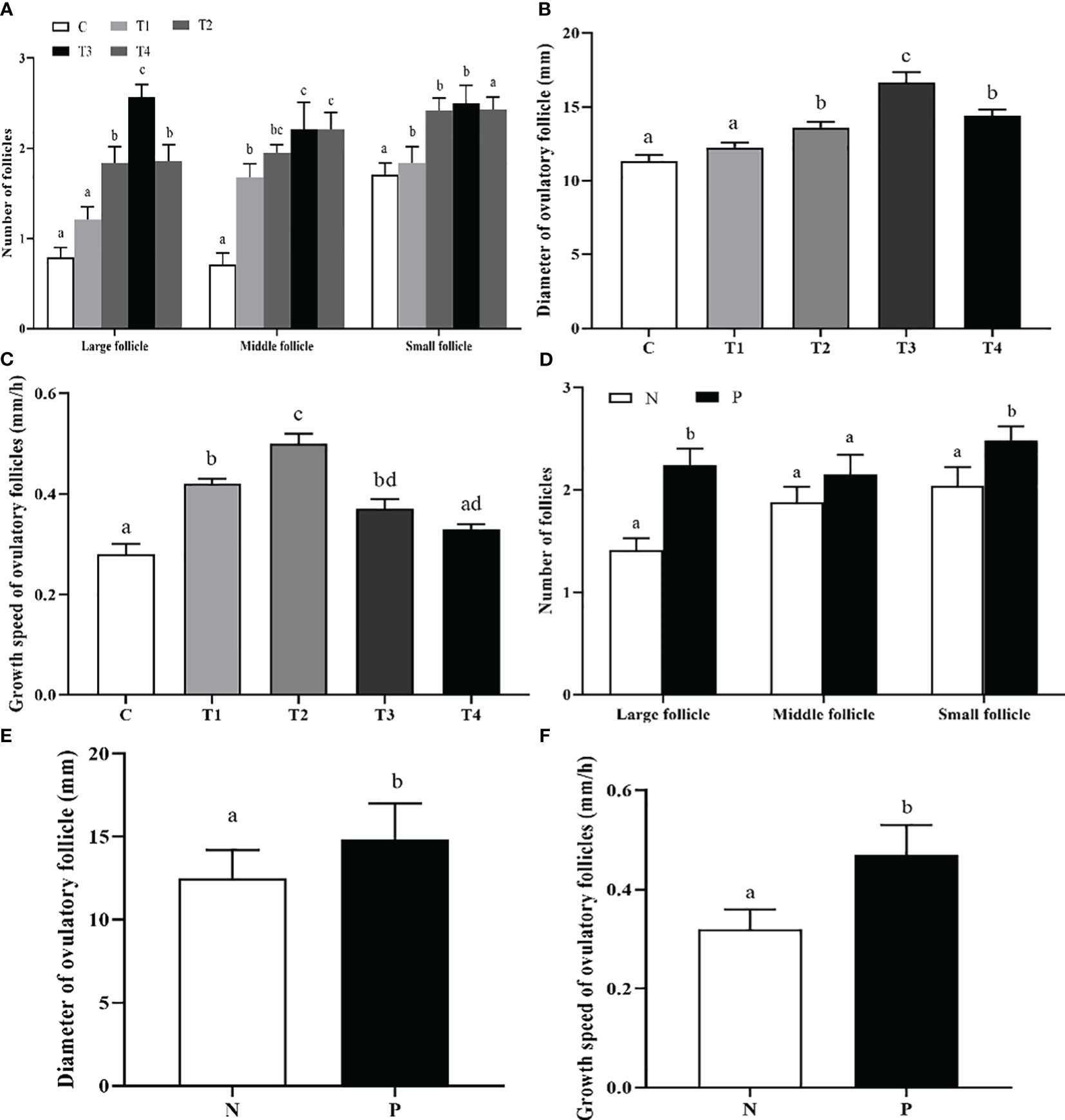
Figure 3 The effect of INH DNA vaccine on follicle counts (A, D), diameter (B, E) and growth speed (C, F) of ovulatory follicles in beef cattle. Data are presented as mean ± SEM. The bars with different lower case letters indicate significant differences p < 0.05 among groups.
Moreover, we also assessed the percentage (proportion) of beef cattle with different ovulatory follicle counts after immunization with different vaccine concentrations. As presented in Table 2, the beef cattle with 2 ovulatory follicles in T2, T3, and T4 groups were significantly increased compared with C group (p < 0.05). The beef cattle with 2 ovulatory follicles in the P group were significantly increased compared to the N group (p < 0.05) (Table 3).
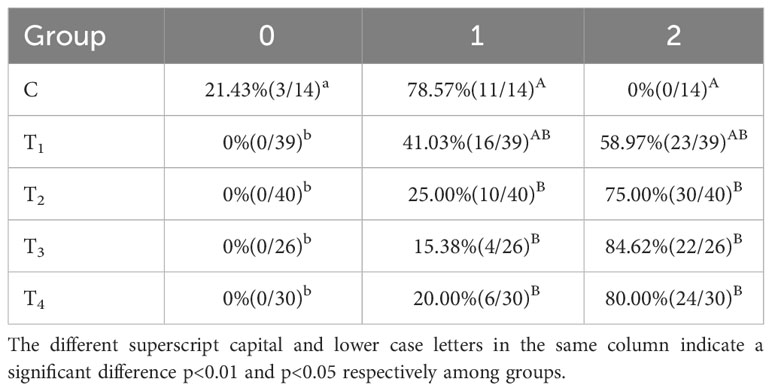
Table 2 Percentage (proportion) of beef cattle with different ovulatory follicle counts after immunization with different vaccine concentrations.
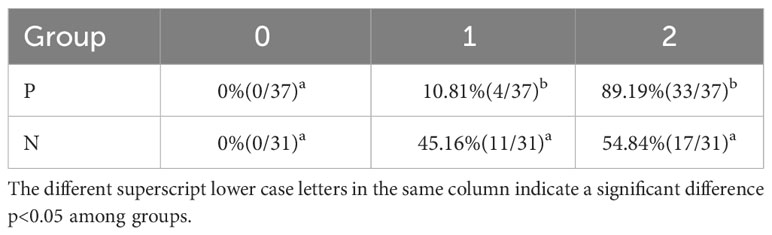
Table 3 Percentage (proportion) of beef cattle with different ovulatory follicle counts in antibody-positive and antibody-negative group.
3.4 Effect of INH DNA vaccine on estrous, ovulation, and conception rates in beef cattle
As presented in Table 4, the proportion of ovulation in T2, T3, and T4 groups was significantly superior to that of the C group by 16.30%, 9.33%, and 6.25%, respectively (p<0.05). In contrast to C group, oestrus and conception rates were remarkably increased (p<0.05) in the immunization groups except for the T3 group. On average, estrous, ovulation, and conception were greater in the antibody-positive beef cattle than those in the negative ones (p<0.05) (Table 5).
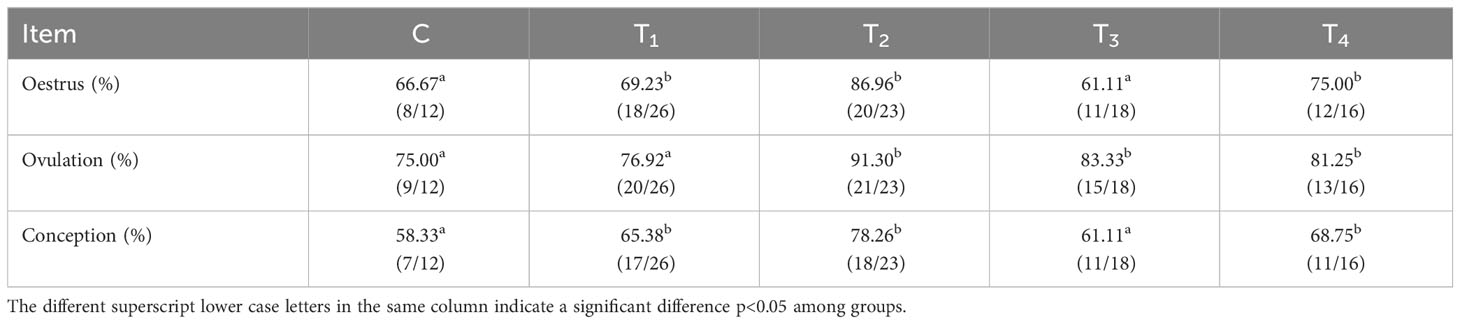
Table 4 Rates of estrous, ovulation, and conception after immunization with different vaccine concentrations.
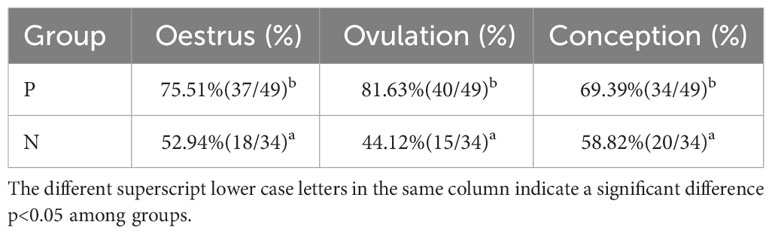
Table 5 Rates of estrous, ovulation, and conception in antibody-positive and antibody-negative group.
3.5 Effect of INH DNA vaccine on embryo counts and twinning rate of beef cattle
After immunization with different vaccine concentrations, the embryo numbers in T3 group were notably increased in contrast to C group (p<0.05) (Figure 4A). The mean twinning rate of beef cattle in T1, T2, T3, and T4 groups was significantly higher than that in C group (p<0.05) (Figure 4B). However, there was no difference in embryo numbers and twinning rate among T1, T2, T3, and T4 groups (p>0.05) (Figures 4A, B). In addition, the embryo numbers in P group tended to be higher than the N group, although the difference didn’t reach significance (p>0.05) (Figure 4C).There was a significant increase of the twinning rate in the P group compared to the N group (p < 0.05) (Figure 4D).
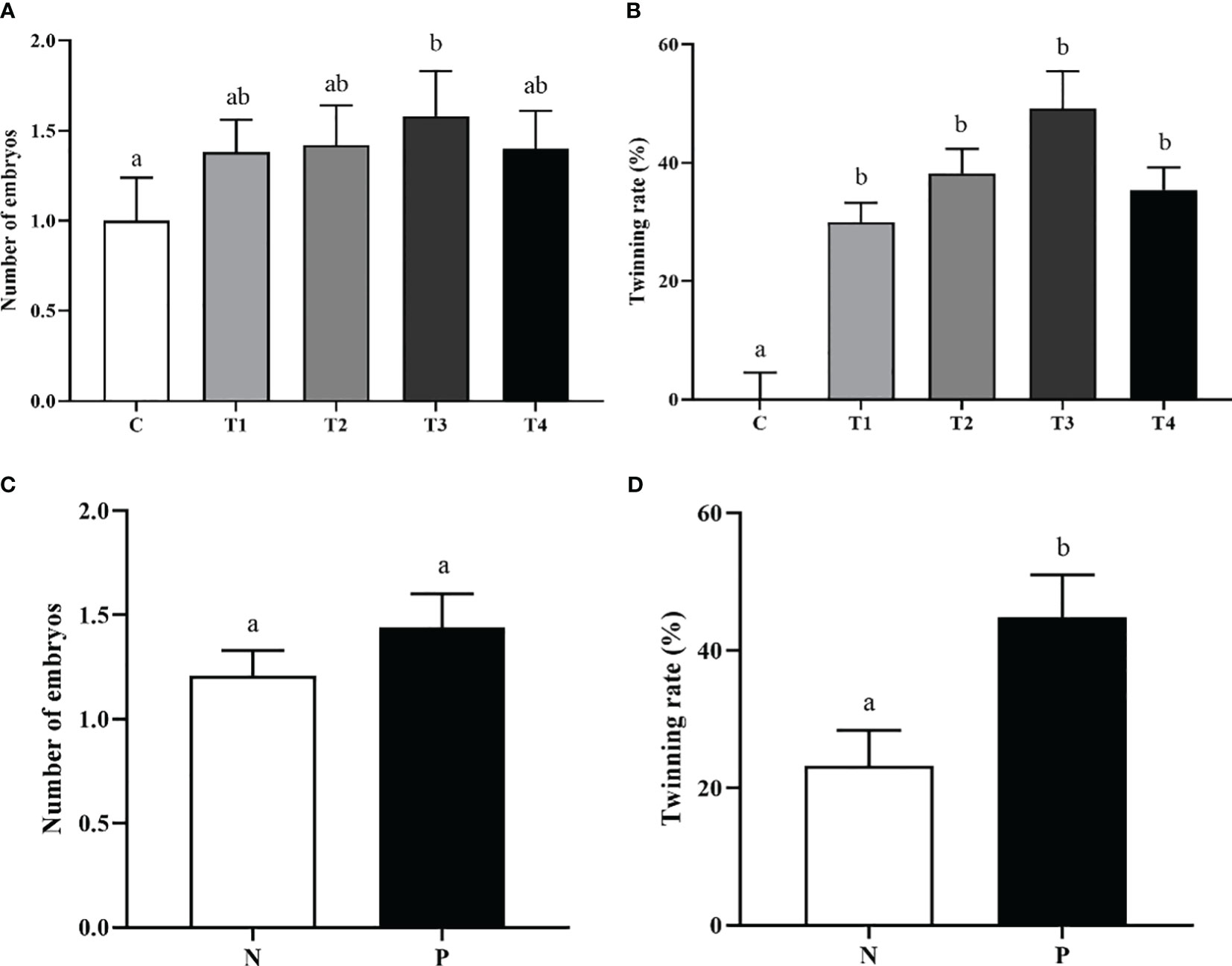
Figure 4 The effect of INH DNA vaccine on embryo counts (A, C) and twinning rate (B, D) of beef cattle. Data are presented as mean ± SEM. The bars with different lower case letters indicate significant differences p < 0.05 among groups.
4 Discussion
There is a wealth of evidence that INH recombinant DNA vaccines can improve reproductive function in various animals, but the effect of these DNA vaccines in improving fertility was still inadequate. Both humoral and cellular immune responses could be induced by DNA vaccines (17). Previous studies have demonstrated that some DNA vaccines expressing INH gene have been elicited better immune responses (18). In the current study, the pCISI vaccines evoked the production of anti-INH antibody after both primary and booster immunizations, except for the T1 group on day 10 after primary immunization in beef cattle. In rats, pcISI plasmid containing recombinant statin a subunit (1-32) fragments increased the antibody titers in a dose-dependent manner (19). However, our data showed that the increase in P/N values was not dose dependently varied among treatment groups. We hypothesized that the species differences and dose optimization may have contributed to the bias in the results. Of particular interest was INH immunization, which is generated anti-INH antibody to neutralize the endogenous INH and increase FSH contents, eventually leading to an improvement in reproductive performance (18, 20).
Previous studies about the effect of active immunization with INH on FSH secretion have been inconsistent. Some reports demonstrated that FSH secretions were increased (14, 21), while others found an increase in ovulation rate without an increase in FSH concentrations after active immunization against INH (22, 23). In the present study, we found that the immunized and control groups didn’t show any change in FSH concentration, with the exception of a marked increase of FSH concentration in T2 group on day 45 after booster immunization. These findings suggest that INH may have local autocrine/paracrine effects, one of which was neutralized may augment FSH in enhancing follicular growth in the ovary. Conceivably, the increased number of large follicles observed in treatment groups are responsible for the elevated E2 level measured in serum (23, 24).
It has been reported that INH is able to inhibit FSH-induced E2 production in granulosa cells (25). E2 produced by ovarian follicles plays an important role in governing the estrous cycle and has a direct effect on the reproductive capability of cattle (26). Elevated level of E2 and low concentration of P4 can promote the estrus expression, follicle maturation and ovulation (27, 28). In our data, the increased estrus and ovulation rate were consistent with higher plasma E2 concentrations in the immunization groups. The elevated plasma P4 levels in INH immunized heifers reflects the increased ovulation rate and increased number of corpora lutea (4). Similarly, we observed that the concentrations of P4 didn’t show any change between N and P groups, except on day 45 after booster immunization. In a practical perspective, our results suggest that the follicles didn’t ovulate and the corpus luteum (CL) formation didn’t occur (14), however the follicle ovulation occurred and the CL was formed on day 45 after booster immunization.
The number of large follicles showed significant increases when mice and rats were immunized with DNA vaccine expressing the INHα (1-32) fragment (18, 29). Furthermore, immunization with INH DNA vaccine enhanced the number of follicles with different diameter sizes, growth speed, and the diameter of the ovulatory follicle, which agree with earlier reports (15). Therefore, the biological activity of endogenous INH is neutralized by active immunization against INH, resulting in increased FSH-induced E2 production, thereby stimulating additional or new follicle growth.
Evidence showed that active immunization against INH increases twin-calving and lambing rate (30). Immunization against INH increased the percentage (proportion) of beef cattle with multiple ovulatory follicles in our study. The cattle with multiple ovulatory follicles have an increased probability of multiple ovulations and a higher probability of twinning rate. Previous studies reported that immunization against INH enhanced embryo quality after superovulation and insemination in Holstein heifers (31, 32). The oocyte maturation rate and parthenogenic embryo development potency were increased after treatment with anti-INH α subunit antibodies in vitro (33). In the present study, the embryo numbers in T3 group were notably increased, but the DNA vaccines encoding INH genes did not significantly increased in other groups, and there was no significant difference in embryo numbers between antibody-positive and antibody-negative cattle. The reason may be that vaccine-induced antibodies were not enough to completely neutralize endogenous INH, or the number of experiment animals was not sufficient to detect significant increase in embryo numbers of cattle. As well as in other study, beside the increases in total number of embryos, embryo quality was also improved following immunization against INH (34). The mean twinning rate of beef cattle in treatment groups was significantly increased. Such beneficial effects on early embryo qualities and twinning rate following the nullification of INH bioactivities lend us a new hypothesis of improving cattle fertility by immunization against INH.
5 Conclusions
This study characterized that neutralization of endogenous INH through intramuscular injection immunization with INH DNA vaccine, successfully elicited a humoral immune response and increased the plasma E2 concentration. These findings clearly illustrated that INH DNA vaccine was capable of promoting the follicle development, thereby improving the behavioral of estrous and ovulation, eventually leading to an augment in the conception rates and twinning rate of beef cattle.
Data availability statement
The original contributions presented in the study are included in the article/supplementary material. Further inquiries can be directed to the corresponding authors.
Ethics statement
All cattle were treated according to the Ethical Committee of Animal Experiments, Hunan Agricultural University (No. 432022021). The studies were conducted in accordance with the local legislation and institutional requirements. Written informed consent was obtained from the owners for the participation of their animals in this study.
Author contributions
JM: Formal analysis, Visualization, Writing – original draft. QL: Methodology, Writing – review & editing. LX: Software, Writing – review & editing. WL: Methodology, Writing – review & editing. ZG: Resources, Writing – review & editing. LG: Resources, Writing – review & editing. XL: Visualization, Writing – review & editing. SW: Funding acquisition, Validation, Writing – review & editing.
Funding
The author(s) declare financial support was received for the research, authorship, and/or publication of this article. This study was supported by National Natural Science Foundation of China (3110774 and 31672507), Natural Science Foundation of Hunan province (2020JJ4359), Hunan Agricultural University Double First- Class Construction Project (SYL201802017).
Conflict of interest
The authors declare that the research was conducted in the absence of any commercial or financial relationships that could be construed as a potential conflict of interest.
Publisher’s note
All claims expressed in this article are solely those of the authors and do not necessarily represent those of their affiliated organizations, or those of the publisher, the editors and the reviewers. Any product that may be evaluated in this article, or claim that may be made by its manufacturer, is not guaranteed or endorsed by the publisher.
References
1. Cappellozza BI, Cooke RF, Harvey KM. Omega-6 fatty acids: a sustainable alternative to improve beef production efficiency. Anim (Basel) (2021) 11(6):1764. doi: 10.3390/ani11061764
2. Takedomi T, Kishi H, Medan MS, Aoyagi Y, Konishi M, Itoh T, et al. Active immunization against inhibin improves superovulatory response to exogenous FSH in cattle. J Reprod Dev (2005) 51:341–6. doi: 10.1262/jrd.16055
3. Roy F, Maurel MC, Combes B, Vaiman D, Cribiu EP, Lantier I, et al. The negative effect of repeated equine chorionic gonadotropin treatment on subsequent fertility in Alpine goats is due to a humoral immune response involving the major histocompatibility complex. Biol Reprod (1999) 60:805–13. doi: 10.1095/biolreprod60.4.805
4. Medan MS, Akagi S, Kaneko H, Watanabe G, Tsonis CG, Taya K. Effects of re-immunization of heifers against inhibin on hormonal profiles and ovulation rate. Reproduction (2004) 128:475–82. doi: 10.1530/rep.1.00232
5. Ying SY. Inhibins, activins, and follistatins: gonadal proteins modulating the secretion of follicle-stimulating hormone. Endocrine Rev (1988) 9:267–93. doi: 10.1210/edrv-9-2-267
6. Rehman A, Ahmad E, Arshad U, Riaz A, Akhtar MS, Ahmad T, et al. Effects of immunization against inhibin α-subunit on ovarian structures, pregnancy rate, embryonic and fetal losses, and prolificacy rate in goats where estrus was induced during the non-breeding season. Anim Reprod Sci (2021) 224:106654. doi: 10.1016/j.anireprosci.2020.106654
7. Knight P. Roles of inhibins, activins, and follistatin in the female reproductive system. Front Neuroendocrinol (1996) 17:476–509. doi: 10.1006/frne.1996.0013
8. Akhtar MF, Ahmad E, Ali I, Shafiq M, Chen Z. The effect of inhibin immunization in seminiferous epithelium of Yangzhou goose ganders: a histological study. Anim (Basel) (2021) 11(10):2801. doi: 10.3390/ani11102801
9. Li Y, Fortin J, Ongaro L, Zhou X, Boehm U, Schneyer A, et al. Betaglycan (TGFBR3) functions as an inhibin A, but not inhibin B, coreceptor in pituitary gonadotrope cells in mice. Endocrinology (2018) 159:4077–91. doi: 10.1210/en.2018-00770
10. Ma L, Li Z, Ma Z, Ma J, Zhao F. Immunization against inhibin promotes fertility in cattle: A meta-analysis and quality assessment. Front Vet Sci (2021) 8:687923. doi: 10.3389/fvets.2021.687923
11. Mizumachi M, Voglmayr J, Washington D, Chen C-L, Bardin C. Superovulation of ewes immunized against the human recombinant inhibin αSubunit associated with increased pre-and postovulatory follicle-stimulating hormone levels. Endocrinology (1990) 126:1058–63. doi: 10.1210/endo-126-2-1058
12. Dan X, Liu X, Han Y, Liu Q, Yang L. Effect of the novel DNA vaccine fusing inhibin alpha (1-32) and the RF-amide related peptide-3 genes on immune response, hormone levels and fertility in Tan sheep. Anim Reprod Sci (2016) 164:105–10. doi: 10.1016/j.anireprosci.2015.11.018
13. Brown R, Hungerford J, Greenwood P, Bloor R, Evans D, Tsonis C, et al. Immunization against recombinant bovine inhibin α subunit causes increased ovulation rates in gilts. Reproduction (1990) 90:199–205. doi: 10.1530/jrf.0.0900199
14. Medan M, Watanabe G, Sasaki k, Nagura Y, Sakaime H, Fujita M, et al. Ovarian and hormonal response of female goats to active immunization against inhibin. J Endocrinol (2003) 177:287–94. doi: 10.1677/joe.0.1770287
15. Chen C, Zhao X, An Z, Ahmad MJ, Niu K, Zhang X, et al. Nasal immunization with AMH-INH-RFRP DNA vaccine for improving follicle development and fertility in buffaloes. Front Endocrinol (2023) 14:1076404. doi: 10.3389/fendo.2023.1076404
16. Xue CL, Liang AX, Mao DG, Han L, Liu XB, Zhang J, et al. Effect of genetic adjuvants on immune respondance, growth and hormone levels in somatostatin DNA vaccination-induced Hu lambs. Vaccine (2010) 28:1541–6. doi: 10.1016/j.vaccine.2009.11.058
17. Kobiyama K, Jounai N, Aoshi T, Tozuka M, Takeshita F, Coban C, et al. Innate immune signaling by and genetic adjuvants for DNA vaccination. Vaccines (2013) 1:278–92. doi: 10.3390/vaccines1030278
18. Wang SL, Han L, Ahmad S, Cao SX, Xue LQ, Xing ZF, et al. Effect of a DNA vaccine harboring two copies of inhibin alpha (1-32) fragments on immune response, hormone concentrations and reproductive performance in rats. Theriogenology (2012) 78:393–401. doi: 10.1016/j.theriogenology.2012.02.019
19. Han L, Mao DG, Zhang DK, Liang AX, Fang M, Moaeen-ud-Din M, et al. Development and evaluation of a novel DNA vaccine expressing inhibin α(1-32) fragment for improving the fertility in rats and sheep. Anim Reprod Sci (2008) 109:251–65. doi: 10.1016/j.anireprosci.2007.12.012
20. Gordon A, Aguilar R, Garrido-gracia JC, Guil-luna S, Sanchez cespedes R, Millán Y, et al. Immunoneutralization of inhibin in cycling rats increases follicle-stimulating hormone secretion, stimulates the ovary and attenuates progesterone receptor-dependent preovulatory luteinizing hormone secretion. Neuroendocrinology (2010) 91:291–301. doi: 10.1159/000299791
21. Wrathall J, Mcleod B, Glencross R, Knight P. Effects of active immunization against a synthetic peptide sequence of the inhibin α-subunit on plasma gonadotrophin concentrations, ovulation rate and lambing rate in ewes. Reproduction (1992) 95:175–82. doi: 10.1530/jrf.0.0950175
22. Tannetta D, Feist S, Bleach E, Groome N, Evans L, Knight P. Effects of active immunization of sheep against an amino terminal peptide of the inhibin alpha C subunit on intrafollicular levels of activin A, inhibin A and follistatin. J Endocrinol (1998) 157:157–68. doi: 10.1677/joe.0.1570157
23. Hennies M, Voglmayr J, Dietrich E, Stollmann M, Moeller R, Holtz W. Hormonal response of female goats to active immunization against a recombinant human inhibin α-subunit, and establishment of an enzyme-linked immunosorbent assay for caprine follicle-stimulating hormone. Reprod Domest Anim (2001) 36:65–71. doi: 10.1046/j.1439-0531.2001.00250.x
24. Li P, Meng J, Liu W, Smith GW, Yao J, Lyu L. Transcriptome analysis of bovine ovarian follicles at predeviation and onset of deviation stages of a follicular wave. Int J Genomics (2016) 2016:3472748. doi: 10.1155/2016/3472748
25. Yu X, Qiao T, Hua L, Liu S, Zhao X, LV C, et al. Synergistic regulatory effect of inhibin and anti-Müllerian hormone on fertility of mice. Front Veterinary Sci (2021) 8:747619. doi: 10.3389/fvets.2021.747619
26. Mcevoy MJ, Mcafee M, Hession JA, Creedon L. A mathematical model of estradiol production from ultrasound data for bovine ovarian follicles. Cells (2022) 11(23):3908. doi: 10.3390/cells11233908
27. Czyk S, Janowski T, Ras M. Current views on the phenomenon of silent heat in cows. Medycyna Weterynaryina (2005) 61:729–9.
28. Emmen JM, Couse JF, Elmore SA, Yates MM, Kissling GE, Korach KS. In vitro growth and ovulation of follicles from ovaries of estrogen receptor (ER) α and ERβ null mice indicate a role for ERβ in follicular maturation. Endocrinology (2005) 146:2817–26. doi: 10.1210/en.2004-1108
29. Han L, Zhen YH, Liang AX, Zhang J, Riaz H, Xiong JJ, et al. Oral vaccination with inhibin DNA delivered using attenuated Salmonella choleraesuis for improving reproductive traits in mice. J Basic Microbiol (2014) 54:962–8. doi: 10.1002/jobm.201300052
30. Morris D, Mcdermott M, Diskin M, Morrison C, Swift P, Sreenan J. Effect of immunization against synthetic peptide sequences of bovine inhibin α-subunit on ovulation rate and twin-calving rate in heifers. Reproduction (1993) 97:255–61. doi: 10.1530/jrf.0.0970255
31. Liu YP, Mao XB, Wei YM, Yu JN, Li H, Chen RA, et al. Studies on enhancing embryo quantity and quality by immunization against inhibin in repeatedly superovulated Holstein heifers and the associated endocrine mechanisms. Anim Reprod Sci (2013) 142:10–8. doi: 10.1016/j.anireprosci.2013.08.005
32. Chen F, Lu J, Guo R, Mei C, Guo B, Li W, et al. Rectifying cow infertility under heat stress by immunization against inhibin and supplementation of progesterone. Domest Anim Endocrinol (2022) 80:106726. doi: 10.1016/j.domaniend.2022.106726
33. Li D, Qin G, Wei Y, Lu F, Huang Q, Jiang H, et al. Immunisation against inhibin enhances follicular development, oocyte maturation and superovulatory response in water buffaloes. Reproduction Fertility Dev (2011) 23:788–97. doi: 10.1071/RD10279
Keywords: inhibin, vaccine, immunization, reproductive performance, beef cattle
Citation: Meng J, Li Q, Xiao L, Liu W, Gao Z, Gong L, Lan X and Wang S (2024) Immunization against inhibin DNA vaccine as an alternative therapeutic for improving follicle development and reproductive performance in beef cattle. Front. Endocrinol. 14:1275022. doi: 10.3389/fendo.2023.1275022
Received: 09 August 2023; Accepted: 04 December 2023;
Published: 15 February 2024.
Edited by:
Claus Yding Andersen, University of Copenhagen, DenmarkReviewed by:
Xiangguo Wang, Beijing University of Agriculture, ChinaChunjin Li, Jilin University, China
Copyright © 2024 Meng, Li, Xiao, Liu, Gao, Gong, Lan and Wang. This is an open-access article distributed under the terms of the Creative Commons Attribution License (CC BY). The use, distribution or reproduction in other forums is permitted, provided the original author(s) and the copyright owner(s) are credited and that the original publication in this journal is cited, in accordance with accepted academic practice. No use, distribution or reproduction is permitted which does not comply with these terms.
*Correspondence: Shuilian Wang, d2FuZ3NodWlsaWFuMTIzNEAxMjYuY29t; Xianyong Lan, TGFueGlhbnlvbmc3OUBud3N1YWYuZWR1LmNu