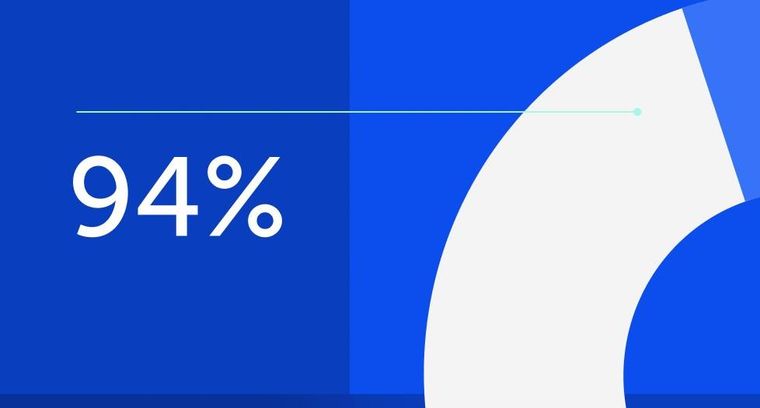
94% of researchers rate our articles as excellent or good
Learn more about the work of our research integrity team to safeguard the quality of each article we publish.
Find out more
REVIEW article
Front. Endocrinol., 19 January 2024
Sec. Clinical Diabetes
Volume 14 - 2023 | https://doi.org/10.3389/fendo.2023.1268619
Diabetes mellitus is a prevalent chronic disease characterized by hyperglycemia. Diabetic peripheral neuropathy (DPN) is one of the complications of diabetes mellitus and is caused by neuron injury induced by hyperglycemic circumstances. The incidence of DPN varies among different countries and regions, ranging from nearly 20% to over 70%. Patients with DPN may encounter symmetric pain or discomfort of the extremes, leading to reduced quality of life and even amputation. The pharmacological management for painful DPN mainly includes antidepressants due to their analgesic effects. Nevertheless, effective therapies to impact the pathogenesis and progression of DPN are lacking. Glucagon-like peptide-1 receptor (GLP-1R) agonists show efficacy in controlling blood glucose and serve as a treatment modality for diabetes mellitus. In recent years, evidence has been proposed that GLP-1R agonists exert neuroprotective effects through modulating inflammation, oxidative stress, and mitochondrial dysfunction. On the other hand, clinical evidence on the potential of GLP-1R agonists for treating DPN is still controversial and limited. This narrative review summarizes the preclinical and clinical studies investigating the capacity of GLP-1R agonists as therapeutic agents for DPN.
Diabetes mellitus (DM) is a chronic disease of the endocrine system characterized by hyperglycemia mainly induced by insufficient insulin secretion or insulin resistance (1). It is estimated that over 500 million individuals are affected by this disease in 2021, and the prevalence of DM will be 1.3 billion by the year 2050 (2). The incidence of DM is dramatically increasing in low- and middle-income countries. In China, it has been reported that 12.4% of adults are affected by DM, which accounts for approximately a quarter of DM patients worldwide; by 2045, it is estimated that there will be 174 million DM patients in China (3). Patients with DM may encounter thirst, frequent urination, weight loss, and other symptoms; moreover, complications of DM may occur, such as diabetic retinopathy, diabetic nephropathy, cardiovascular diseases, and diabetic neuropathy (4–6). These complications put patients with DM at a high risk of low quality of life, increasing treatment cost, disability, and even mortality (7, 8). Diabetic peripheral neuropathy (DPN) is the most common type of diabetic neuropathy (9). It is estimated that in China, approximately half of patients with DM are complicated with DPN (10). Patients with DPN would likely experience symmetric pain or discomfort, typically presenting as numbness, burn-like or sting-like pain, and the feeling of wearing stockings or gloves (4). These symptoms may severely decrease the quality of life and induce anxiety and depression in patients (11). Unfortunately, treatments for DPN are still largely insufficient.
Glucagon-like peptide-1 (GLP-1) is a peptide secreted by the intestinal tract after the stimulation of food intake, which induces the secretion of insulin by β cells, thus modulating blood glucose (12). Based on this mechanism, GLP-1 receptor (GLP-1R) agonists have been developed for the treatment of DM (13). Until now, various clinical trials have demonstrated that GLP-1R agonists show favorable efficacy and tolerable safety in lowering blood glucose in patients with DM (14–16). Apart from the glycemic-lowering effect, GLP-1R agonists are considered pharmacological options for treating obesity and other complications of DM, such as cardiovascular diseases and diabetic nephropathy (17–19). Notably, evidence has been proposed that GLP-1R agonists present neuroprotective effects (20–22). Several clinical studies have also indicated that GLP-1R agonists may serve as a potential therapeutic strategy for DPN (23–25).
This narrative review aimed to summarize the currently available perspective on the potential involvement of GLP-1R agonists in DPN and shed light on the future direction of clinical studies.
The prevalence of DPN varies among different countries and regions. A cross-sectional study conducted in two cities of middle China reported that the overall prevalence of DPN was 71.2% in patients with DM (26). Another study conducted in the southern region of China indicated that the overall prevalence of DPN is 33.1% in patients with type 2 DM who are overweight or obese (27). In Taiwan, the prevalence of DPN is 21.3% (28). A systematic review and meta-analysis involving 29 studies from 8 countries found that in Latin America and the Caribbean region, the incidence of DPN is 46.5% (95% confidence interval: 38.0-55.0%) (29). In the Middle East, a study performed in Saudi Arabia reported that in patients with type 2 DM, the prevalence of DPN assessed by the Michigan Neuropathy Screening Instrument (MNSI) is 27.7% (30). Another study conducted in Jordan showed that the incidence of DPN is 36% in patients with DM (31). In the United States, a study assessed 11.9 million adults with DM and found that a total of 3.9 million (32.7%) patients have symptomatic DPN (32). Another study performed on youth (age < 20 years) reported that the prevalence of DPN assessed by the MNSI is 7% in youth with type 1 DM and 22% in youth with type 2 DM (33). The Diabetes Control and Complications Trial/Epidemiology of Diabetes Interventions and Complications (DCCT/EDIC) study evaluated the incidence of DPN in participants with type 1 DM, followed for over 23 years and illustrated that the incidence of DPN is 33% (34). In Europe, a study randomly assessed 3,250 patients with DM from 31 centers in 16 European countries and suggested that the overall prevalence of DPN is 28% without significant geographical differences (the EURODIAB IDDM Complications Study) (35). The KORA F4/FF4 Study conducted in Germany reported a prevalence of DPN of 25% (36). The ADDITION Demark study reported that the prevalence of DPN was up to 34.8 (37). Generally, the prevalence of DPN in patients with DM ranges from nearly 20% to over 70% in different countries and regions, where studies in China reported the highest prevalence of DPN in patients with DM, suggesting the urgent demands for proper management of DPN in patients with DM, especially patients in China.
On the other hand, the risk factors for DPN have been widely reported. Most studies have recognized that older age, longer DM duration, and worse glucose control are risk factors for DP. Meanwhile, obesity or overweight, worse renal function, unfavorable lipid profile (such as higher low-density lipoprotein cholesterol and lower high-density lipoprotein cholesterol), hypertension, and smoking are also associated with a higher risk of DPN (28, 30, 32, 33, 35, 38). Notably, two studies reported that insulin treatment is associated with a higher risk of DPN (28, 30). Interestingly, a study conducted in Saudi Arabia reported that females are more likely to suffer from DPN (30), while a study performed in the United States suggested that in youth with type 2 DM, male sex is a risk factor for DPN (30). The UK prospective diabetes study (UKPDS) revealed that age, female sex, poor glucose control, weight, alcohol consumption, and current smoking are risk factors for DPN (39). There are also several studies reporting novel factors associated with DPN risk. For instance, lower education level (26), lower vitamin D level or vitamin D deficiency (40), receiving β-blocker treatment (34), the incidence of diabetic retinopathy (41), and lower income (42).
According to the relevant guidelines in China released in 2021 (10), pregabalin and duloxetine are the first-line pharmacological treatments for painful DPN. Meanwhile, considering the economic burden and comorbidities, gabapentin could also serve as an initial therapy. Tricyclic antidepressants such as amitriptyline and imipramine should be carefully administered due to the high incidence of adverse events. Opioids such as tramadol are not recommended due to the high incidence of adverse events and addiction (10). These agents are recommended for the treatment of DPN due to their analgesic effects. Nevertheless, they could not reverse the injury of neurons in patients with DM. Therefore, it is of great significance to search for potential treatment strategies to alleviate the pathogenesis and progression of DPN.
In patients with DM, the metabolism of glucose, lipids, proteins, and nuclear acids is largely dysregulated. Under hyperglycemia, lipids, proteins, and nuclear acids can form advanced glycation end products, interact with the corresponding receptor and finally trigger the activation of inflammation, oxidative stress, and mitochondrial dysfunction, which ultimately induces neuronal injury (43–46).
Qi et al. used methylglyoxal, a byproduct of glucose metabolism, to treat neuroblastoma SH-SY5Y cells to mimic diabetic neuropathy (47). The authors then used GLP-1R agonist liraglutide to treat the methygluoxal-induced SH-SY5Y cells. Liraglutide treatment decreased the levels of superoxide dismutase (SOD) and reactive oxygen species (ROS), suggesting that liraglutide reduced oxidative stress in methygluoxal-induced SH-SY5Y cells. Moreover, the authors also used 1H nuclear magnetic resonance and disclosed that liraglutide altered energy metabolism and elevated gluconeogenesis; it also increased oxidative phosphorylation while suppressing glycolysis in methygluoxal-induced SH-SY5Y cells (47).
Pandey et al. used high-glucose treatment in SH-SY5Y cells to mimic diabetic neuropathy and explored the effect of the GLP-1R agonist exendin-4 on diabetic neuropathy (48). The data showed that exendin-4 treatment elevated the levels of p-protein kinase B (Akt) and B-cell lymphoma 2 (Bcl-2) and suppressed the level of Bax, which indicated a lower level of cell apoptosis. The authors also found that exendin-4 treatment inhibited the level of oxidative stress. In addition, exendin-4 treatment repressed mitochondrial dysfunction, as shown by lower levels of the mitochondrial function-associated genes MCU and UCP3, as well as the mitochondrial fission genes DRP1 and FIS1. More importantly, the authors demonstrated that exendin-4 treatment exerted the abovementioned functions through the Epac/Akt pathway (48).
Liu et al. established a DM rat model by injection of streptozotocin; then, they treated the DM rats with intraperitoneal injection of exendin-4 at 1 nmol/kg for 24 weeks (49). The authors found that compared with placebo injection, the 2000 and 250 Hz current perception threshold values were reduced by exendin-4 injection, indicating a lower level of nerve dysfunction. They also revealed that the reduction in the axon/fibre area ratio of the sciatic nerve and intraepidermal nerve fibre loss were relieved by exendin-4 injection (49).
Jolivalt et al. treated streptozotocin-induced DM rats with exenatide, a GLP-1R agonist, for 8 weeks and found that exenatide treatment attenuated the reduction in motor nerve conduction velocity and paw intraepidermal fibre density (50). The authors also elucidated that exenatide elevated phosphorylation of extracellular signal-regulated kinases 1/2 (ERK1/2) level, suggesting that exenatide exerted a neuroprotective effect through activating the ERK signaling. Interestingly, the authors also found that exenatide did not vary the blood glucose, insulin level, or body weight in streptozotocin-induced DM rats. The findings of the authors suggested that the neuroprotective effect of exenatide was independent of the glycemic control effect of exenatide (50).
Himeno et al. dissected GLP-1R-expressing lumbar dorsal root ganglion neurons from mice and cultured them with Schwann cell-conditioned media to mimic diabetic conditions (51). The authors then treated dorsal root ganglion neurons with exendin-4. The data revealed that exendin-4 significantly promoted the neurite outgrowth that was previously impaired by Schwann cell-conditioned media. In the in vivo experiments, the authors treated the streptozotocin-induced DM mice with exendin-4 at 10 nmol/kg for 4 weeks and found that exendin-4 promoted the peripheral nerve function as reflected by improved current perception threshold and motor and sensory nerve conduction velocity. Interestingly, the authors revealed that the dose of exendin-4 at 10 nmol/kg for 4 weeks had no effect on blood glucose, body weight, or HbA1c levels in streptozotocin-induced DM mice. These findings indicated that the neuroprotective effect of exendin-4 was independent of its blood glucose-lowering effect (51).
Ma et al. also used streptozotocin injection to establish a DM rat model (22). Subsequently, the daily GLP-1R agonist liraglutide at 200 μg/kg was used to treat the DM rats for 8 weeks. The authors observed that the loss of myelin nerve fibre density was partly restored by liraglutide treatment, and the nerve conduction velocity of motor and sensory nerves was improved by liraglutide treatment. The authors also noticed that the levels of proinflammatory cytokines (including cytokines tumor necrosis factor-α (TNF-α), interleukin-1β (IL-1β), and IL-6), intercellular adhesion molecule 1 (ICAM1), and NADPH oxidase 4 (NOX4)) in the sciatic nerve were all reduced by liraglutide treatment. The authors further explored the relevant signaling pathways and found that mitogen-activated protein kinase (MAPK) and nuclear factor-κB (NF-κB) were repressed by liraglutide treatment (22).
Moustafa et al. established a DM rat model by injecting streptozotocin and nicotinamide (21). Then, they treated DM rats with liraglutide or pregabalin, a therapeutic agent for neurological pain in patients with DPN. The data showed that both liraglutide and pregabalin showed similar effects on the neurological behaviors of DM rats as assessed by motor coordination and latency withdrawal time of tail flick and hind paw cold allodynia tests. Meanwhile, liraglutide ameliorated the dysregulation of malondialdehyde (MDA), nitric oxide (NO), SOD, matrix metalloproteinase (MMP)-2 and -9, as well as IL-6 and IL-10 in the sciatic nerve, while pregabalin had less effect on them. These findings suggested that liraglutide may have similar effects on ameliorating neurological symptoms of DPN as pregabalin, and it also mitigated oxidative stress, inflammation, and matrix remodelling in DPN (21).
Apart from the abovementioned studies, there are also several studies demonstrating the neuroprotective effects of GLP-1R agonists in DM, and we have listed these studies (including the abovementioned studies) in Table 1. Combined with the above-described studies, it is clear that GLP-1R agonists reduced inflammation, oxidative stress, mitochondrial dysfunction, and matrix remodelling to improve neuron functions in DPN (Figure 1). Notably, two studies controlled the dosage of GLP-1R agonists at a level that would not affect the blood glucose of in vivo DM models, and these two studies demonstrated that the neuroprotective effects of GLP-1R agonists are independent of their blood glucose-lowering effects. The molecular mechanisms of the neuroprotective effects of GLP-1R agonists include signaling pathways such as ERK signaling, PI3K signaling, RhoA activity, MAPK/NF-κB signaling, and the Epac/Akt pathway. These studies have laid a solid basis for the clinical application of GLP-1R agonists for treating DPN.
Figure 1 GLP-1R agonists reduce inflammation, oxidative stress, mitochondrial dysfunction, and matrix remodelling to improve neuron functions.
Unlike preclinical evidence, studies investigating the effect of GLP-1R on DPN are quite rare. Most studies reported diabetic neuropathy as one of the endpoints, rather than classifying it into peripheral neuropathy and autonomic neuropathy. A brief description of the studies is listed in Table 2.
Sullivan et al. conducted an analysis of the long-term effectiveness of liraglutide and glimepiride monotherapies in patients with DM from the LEAD-3 trial (56). The LEAD-3 trial assigned patients to randomly receive monotherapies of liraglutide 1.2 mg/day, liraglutide 1.8 mg/day, and glimepiride 8 mg/day (61). The analysis of Sullivan et al. revealed that the rates of neuropathies leading to first/recurrent amputation within 30 years were higher in patients receiving glimepiride 8 mg/day (N=248) than in patients receiving liraglutide 1.2 mg/day (N=251) or liraglutide 1.8 mg/day (n=247), while they were numerically similar between patients receiving liraglutide 1.2 mg/day and liraglutide 1.8 mg/day. Meanwhile, the incidence of minor hypoglycemia was higher in patients receiving glimepiride 8 mg/day than in those receiving liraglutide 1.2 mg/day or liraglutide 1.8 mg/day (56). The findings of this study disclosed that liraglutide showed a better effect on treating DPN. Nevertheless, the main populations of this study are Caucasian, Hispanic, and African-American. Therefore, the findings of this study could not be expanded in Asia. In addition, the sample size of this study is not large enough to drive a solid conclusion. Meanwhile, the evaluation of neuropathy was not mentioned in this article.
In a pilot study, Jaiswal et al. enrolled 46 type 2 DM patients with mild to moderate DPN (23). The 46 patients were randomized to receive open-label twice-daily exenatide (N=22) or daily insulin glargine (N=24). The primary outcome was the prevalence of confirmed clinical neuropathy assessed by nerve conduction studies of the median, peroneal motor, and sural sensory nerves with a standard protocol. The secondary outcomes included individual electrophysiology measures, vibration perception, clinical neuropathy changes, etc. After 18 months of follow-up, blood glucose control was similar between the groups. Meanwhile, the prevalence of confirmed clinical neuropathy, intraepidermal nerve fibre density, and nerve conduction were all similar between groups. Nevertheless, limited by the small sample size and the open-label study design, the findings may be biased. In addition, the abnormal nerve conduction study measures at baseline were slightly different (although not statistically significant); this could also affect the findings (23).
Brock et al. conducted a randomized, double-blinded, placebo-controlled trial to explore the effect of liraglutide on neuropathies in patients with type 1 DM (57). The patients in the liraglutide group received 1.2-1.8 mg daily liraglutide for 26 weeks. The primary outcome was the change in latency of early brain evoked potentials. Secondary outcomes included cortical evoked potential, peripheral neurophysiological testing, proinflammatory cytokines, and autonomic function. Peripheral nerve function was assessed by nerve conduction velocities, amplitudes and F‐waves on the motor and sensory nerves. The data showed that liraglutide significantly decreased the level of IL-6 and numerically reduced the levels of other proinflammatory cytokines, including interferon-γ and IL-10. However, the functions of central, autonomic, or peripheral neurons were not affected by liraglutide treatment (57). This study could still be limited by the small sample size. In addition, all patients had type 1 DM, and the neuroprotective effect of liraglutide in patients with type 2 DM was unclear.
Ponirakis et al. conducted a subgroup analysis of the Qatar study, an open-label, randomized controlled trial (62), and compared the peripheral neurological outcomes in patients receiving a combination of exenatide (2 mg/week) and pioglitazone (30 mg/day) and those receiving glargine plus aspart insulin (58). The primary outcome was the findings of corneal confocal microscopy assessing small nerve fibres. The secondary outcomes were vibration perception threshold assessed by the Neurothesiometer, sudomotor function evaluated by electrochemical skin conductance, and neuropathic pain assessed by the Douleur Neuropathique en 4 questionnaire. The authors disclosed that compared with baseline, the combination treatment group (N=21) showed an increase in corneal nerve branch density but worsened vibration perception and unchanged sudomotor function as well as neuropathic pain after 1 year of treatment. Inheriting from the main study, this subgroup analysis has the limitations of small sample size and open-label study design (58).
Issar et al. reported an observational comparative study that compared nerve excitability in patients with type 2 DM who received exenatide (n=32), a dipeptidyl peptidase-4 (DPP-4) inhibitor (n=31), or a sodium-glucose cotransporter-2 (SGLT-2) inhibitor (n=27) (59). Motor nerve excitability was assessed by the function of voltage-gated sodium and potassium ion channels and sodium-potassium pumps. The authors disclosed that in patients who received a DPP-4 inhibitor or an SGLT-2 inhibitor, abnormalities were found in peak threshold reduction, S2 accommodation, and subexcitability and superexcitability, whereas patients who received exenatide showed normal nerve excitability. Moreover, the authors found that exenatide treatment was associated with elevation of nerve function, which was independent of blood glucose control (59). Although these findings were encouraging, further randomized, controlled trials are needed to verify them.
In a retrospective cohort study, Lin et al. (60) reviewed patients with DM who received GLP-1R agonists (n=20,288) and SGLT-2 inhibitors (n=81,152). The primary outcome was the incidence of major adverse limb events (defined as either one of the following events: newly diagnosed critical limb ischemia, percutaneous transluminal angioplasty or peripheral bypass of peripheral artery disease, or nontraumatic amputation). The secondary outcome was major adverse cardiovascular events. The incidence of major adverse limb events was lower in patients who received GLP-1R agonists than in those who received an SGLT-2 inhibitor. In addition, the association of GLP-1R agonists with the low incidence of major adverse limb events was notable in patients with DPN. In fact, DPN is closely associated with adverse limb events. For instance, a post hoc analysis of the Action to Control Cardiovascular Risk in Diabetes (ACCORD) trial illustrated that DPN was a strong predictor of lower-limb amputations (63). Meanwhile, DPN is independently associated with the risk of diabetic foot ulcers, which could lead to nontraumatic lower-limb amputations (64). Although the findings of Lin et al. did not directly point out the treatment potential of GLP-1R agonists for DPN, these results may support the peripheral neuroprotective effect of GLP-1R agonists considering the close association of DPN with adverse limb events (60).
Based on these studies, the treatment efficacy of GLP-1R agonists in patients with DPN is still controversial. In addition, most studies are limited by small sample sizes. On the other hand, only a few studies clearly reported DPN rather than combining it with autonomic neuropathy. Moreover, the assessments of neurological function varied greatly among studies, which may also lead to inconsistent findings among studies. Therefore, further large-scale studies are needed to further explore the therapeutic efficacy of GLP-1R agonists in patients with DPN. Meanwhile, since this study is not a meta-analysis, the association of GLP-1R agonists with outcomes of patients with DPN could not be fully addressed.
In conclusion, the incidence of DPN varies from nearly 20% to over 70% in patients with DM, which severely hampers quality of life and even leads to amputation. The currently recommended pharmacological treatments for DPN mainly include antidepressants due to their analgesic effects, while a strategy for the pathogenesis and progression of DPN is lacking. GLP-1R agonists exert neuroprotective effects in DPN by reducing neuronal apoptosis, inflammation, oxidative stress, mitochondrial dysfunction, and matrix remodelling. Nevertheless, the available clinical evidence on the neuroprotective effects of GLP-1R agonists in DPN is still controversial and limited. Therefore, it is urgent to validate the therapeutic effect of GLP-1R agonists with large-scale studies and well-designed randomized, controlled trials. Additionally, systematic review and meta-analysis would be helpful to address the role of GLP-1R agonists in treating DPN.
CL: Conceptualization, Formal Analysis, Writing – original draft, Writing – review & editing. TW: Formal Analysis, Writing – review & editing. NR: Conceptualization, Funding acquisition, Resources, Writing – review & editing.
The author(s) declare financial support was received for the research, authorship, and/or publication of this article. This study was supported by the Heilongjiang Research Project on Traditional Chinese Medicine (ZHY2022-139), (ZYW2022-003).
The authors declare that the research was conducted in the absence of any commercial or financial relationships that could be construed as a potential conflict of interest.
All claims expressed in this article are solely those of the authors and do not necessarily represent those of their affiliated organizations, or those of the publisher, the editors and the reviewers. Any product that may be evaluated in this article, or claim that may be made by its manufacturer, is not guaranteed or endorsed by the publisher.
1. Gregg EW, Buckley J, Ali MK, Davies J, Flood D, Mehta R, et al. Improving health outcomes of people with diabetes: target setting for the WHO Global Diabetes Compact. Lancet (2023) 401(10384):1302–12. doi: 10.1016/S0140-6736(23)00001-6
2. Collaborators GBDD. Global, regional, and national burden of diabetes from 1990 to 2021, with projections of prevalence to 2050: a systematic analysis for the Global Burden of Disease Study 2021. Lancet (2023) 402(10397):203–34. doi: 10.1016/S0140-6736(23)01301-6
3. Wang L, Peng W, Zhao Z, Zhang M, Shi Z, Song Z, et al. Prevalence and treatment of diabetes in China, 2013-2018. JAMA (2021) 326(24):2498–506. doi: 10.1001/jama.2021.22208
4. Galiero R, Caturano A, Vetrano E, Beccia D, Brin C, Alfano M, et al. Peripheral neuropathy in diabetes mellitus: pathogenetic mechanisms and diagnostic options. Int J Mol Sci (2023) 24(4):3554. doi: 10.3390/ijms24043554
5. Cao Z, Huang D, Tang C, Lu Y, Huang S, Peng C, et al. Pyroptosis in diabetes and diabetic nephropathy. Clin Chim Acta (2022) 531:188–96. doi: 10.1016/j.cca.2022.04.011
6. Morales J, Handelsman Y. Cardiovascular outcomes in patients with diabetes and kidney disease: JACC review topic of the week. J Am Coll Cardiol (2023) 82(2):161–70. doi: 10.1016/j.jacc.2023.04.052
7. Malik RA, Tesfaye S, Ziegler D. Medical strategies to reduce amputation in patients with type 2 diabetes. Diabetes Med (2013) 30(8):893–900. doi: 10.1111/dme.12169
8. Bell DS. Heart failure: the frequent, forgotten, and often fatal complication of diabetes. Diabetes Care (2003) 26(8):2433–41. doi: 10.2337/diacare.26.8.2433
9. Preston FG, Riley DR, Azmi S, Alam U. Painful diabetic peripheral neuropathy: practical guidance and challenges for clinical management. Diabetes Metab Syndr Obes (2023) 16:1595–612. doi: 10.2147/DMSO.S370050
10. CDS BGONC. Expert consensus on diagnosis and treatment of diabetic neuropathy (2021 edition). Chin J Diabetes Mellitus (2021) 13(6):540–57. doi: 10.3760/cma.j.cn311282-20210608-00357
11. Bai Y, Ma JH, Yu Y, Wang ZW. Effect of cognitive-behavioral therapy or mindfulness therapy on pain and quality of life in patients with diabetic neuropathy: A systematic review and meta-analysis. Pain Manag Nurs (2022) 23(6):861–70. doi: 10.1016/j.pmn.2022.05.005
12. Scheen AJ, Lefebvre PJ. Glucagon, from past to present: a century of intensive research and controversies. Lancet Diabetes Endocrinol (2023) 11(2):129–38. doi: 10.1016/S2213-8587(22)00349-7
13. Li S, Vandvik PO, Lytvyn L, Guyatt GH, Palmer SC, Rodriguez-Gutierrez R, et al. SGLT-2 inhibitors or GLP-1 receptor agonists for adults with type 2 diabetes: a clinical practice guideline. BMJ (2021) 373:n1091. doi: 10.1136/bmj.n1091
14. Saxena AR, Frias JP, Brown LS, Gorman DN, Vasas S, Tsamandouras N, et al. Efficacy and safety of oral small molecule glucagon-like peptide 1 receptor agonist danuglipron for glycemic control among patients with type 2 diabetes: A randomized clinical trial. JAMA Netw Open (2023) 6(5):e2314493. doi: 10.1001/jamanetworkopen.2023.14493
15. Urva S, Coskun T, Loh MT, Du Y, Thomas MK, Gurbuz S, et al. LY3437943, a novel triple GIP, GLP-1, and glucagon receptor agonist in people with type 2 diabetes: a phase 1b, multicentre, double-blind, placebo-controlled, randomised, multiple-ascending dose trial. Lancet (2022) 400(10366):1869–81. doi: 10.1016/S0140-6736(22)02033-5
16. Group GSR, Nathan DM, Lachin JM, Balasubramanyam A, Burch HB, Buse JB, et al. Glycemia reduction in type 2 diabetes - glycemic outcomes. N Engl J Med (2022) 387(12):1063–74. doi: 10.1056/nejmoa2200433
17. Kelly AS. Current and future pharmacotherapies for obesity in children and adolescents. Nat Rev Endocrinol (2023) 19:534–41. doi: 10.1038/s41574-023-00858-9
18. Ussher JR, Drucker DJ. Glucagon-like peptide 1 receptor agonists: cardiovascular benefits and mechanisms of action. Nat Rev Cardiol (2023) 20(7):463–74. doi: 10.1038/s41569-023-00849-3
19. Alicic RZ, Cox EJ, Neumiller JJ, Tuttle KR. Incretin drugs in diabetic kidney disease: biological mechanisms and clinical evidence. Nat Rev Nephrol (2021) 17(4):227–44. doi: 10.1038/s41581-020-00367-2
20. Mohiuddin MS, Himeno T, Inoue R, Miura-Yura E, Yamada Y, Nakai-Shimoda H, et al. Glucagon-like peptide-1 receptor agonist protects dorsal root ganglion neurons against oxidative insult. J Diabetes Res (2019) 2019:9426014. doi: 10.1155/2019/9426014
21. Moustafa PE, Abdelkader NF, El Awdan SA, El-Shabrawy OA, Zaki HF. Liraglutide ameliorated peripheral neuropathy in diabetic rats: Involvement of oxidative stress, inflammation and extracellular matrix remodeling. J Neurochem (2018) 146(2):173–85. doi: 10.1111/jnc.14336
22. Ma J, Shi M, Zhang X, Liu X, Chen J, Zhang R, et al. GLP−1R agonists ameliorate peripheral nerve dysfunction and inflammation via p38 MAPK/NF−kappaB signaling pathways in streptozotocin−induced diabetic rats. Int J Mol Med (2018) 41(5):2977–85. doi: 10.3892/ijmm.2018.3509
23. Jaiswal M, Martin CL, Brown MB, Callaghan B, Albers JW, Feldman EL, et al. Effects of exenatide on measures of diabetic neuropathy in subjects with type 2 diabetes: results from an 18-month proof-of-concept open-label randomized study. J Diabetes Complications (2015) 29(8):1287–94. doi: 10.1016/j.jdiacomp.2015.07.013
24. Peskin BR, Shcheprov AV, Boye KS, Bruce S, Maggs DG, Gaebler JA. Cardiovascular outcomes associated with a new once-weekly GLP-1 receptor agonist vs. traditional therapies for type 2 diabetes: a simulation analysis. Diabetes Obes Metab (2011) 13(10):921–7. doi: 10.1111/j.1463-1326.2011.01430.x
25. Strokov IA, Trakhtenberg YA, Kovalenko AL. Efficacy and safety of Cytoflavin in the treatment of diabetic polyneuropathy: results of a multicenter, double-blind, placebo-controlled, randomized CYLINDER study. Zh Nevrol Psikhiatr Im S S Korsakova (2023) 123(5):100–7. doi: 10.17116/jnevro2023123051100
26. Qin L, Niu JY, Zhou JY, Zhang QJ, Zhou F, Zhang N, et al. Prevalence and risk factors of diabetic peripheral neuropathy in Chinese communities. Zhonghua Liu Xing Bing Xue Za Zhi. (2019) 40(12):1578–84. doi: 10.3760/cma.j.issn.0254-6450.2019.12.014
27. Li L, Chen J, Wang J, Cai D. Prevalence and risk factors of diabetic peripheral neuropathy in Type 2 diabetes mellitus patients with overweight/obese in Guangdong province, China. Prim Care Diab (2015) 9(3):191–5. doi: 10.1016/j.pcd.2014.07.006
28. Pai YW, Lin CH, Lee IT, Chang MH. Prevalence and biochemical risk factors of diabetic peripheral neuropathy with or without neuropathic pain in Taiwanese adults with type 2 diabetes mellitus. Diabetes Metab Syndr (2018) 12(2):111–6. doi: 10.1016/j.dsx.2017.09.013
29. Yovera-Aldana M, Velasquez-Rimachi V, Huerta-Rosario A, More-Yupanqui MD, Osores-Flores M, Espinoza R, et al. Prevalence and incidence of diabetic peripheral neuropathy in Latin America and the Caribbean: A systematic review and meta-analysis. PloS One (2021) 16(5):e0251642. doi: 10.1371/journal.pone.0251642
30. Al Ayed MY, Ababneh M, Robert AA, Al-Musalum M, Sabrery D, Amer M, et al. Prevalence and risk factors for diabetic peripheral neuropathy among patients with type 2 diabetes in Saudi Arabia: A cross-sectional study. Curr Diabetes Rev (2023) 19(7):e141122210875. doi: 10.2174/1573399819666221114105817
31. Atallah SM, Al-Jaghbir MT, Zayed AA. The prevalence of diabetic peripheral neuropathy among diabetic Palestinian refugees in the Nuzha area, Jordan: a cross-sectional study. Lancet (2021) 398 Suppl 1:S15. doi: 10.1016/S0140-6736(21)01501-4
32. Candrilli SD, Davis KL, Kan HJ, Lucero MA, Rousculp MD. Prevalence and the associated burden of illness of symptoms of diabetic peripheral neuropathy and diabetic retinopathy. J Diabetes Complications (2007) 21(5):306–14. doi: 10.1016/j.jdiacomp.2006.08.002
33. Jaiswal M, Divers J, Dabelea D, Isom S, Bell RA, Martin CL, et al. Prevalence of and risk factors for diabetic peripheral neuropathy in youth with type 1 and type 2 diabetes: SEARCH for diabetes in youth study. Diabetes Care (2017) 40(9):1226–32. doi: 10.2337/dc17-0179
34. Braffett BH, Gubitosi-Klug RA, Albers JW, Feldman EL, Martin CL, White NH, et al. Risk factors for diabetic peripheral neuropathy and cardiovascular autonomic neuropathy in the diabetes control and complications trial/epidemiology of diabetes interventions and complications (DCCT/EDIC) study. Diabetes (2020) 69(5):1000–10. doi: 10.2337/db19-1046
35. Tesfaye S, Stevens LK, Stephenson JM, Fuller JH, Plater M, Ionescu-Tirgoviste C, et al. Prevalence of diabetic peripheral neuropathy and its relation to glycaemic control and potential risk factors: the EURODIAB IDDM Complications Study. Diabetologia (1996) 39(11):1377–84. doi: 10.1007/s001250050586
36. Herder C, Kannenberg JM, Huth C, Carstensen-Kirberg M, Rathmann W, Koenig W, et al. Proinflammatory cytokines predict the incidence and progression of distal sensorimotor polyneuropathy: KORA F4/FF4 study. Diabetes Care (2017) 40(4):569–76. doi: 10.2337/dc16-2259
37. Charles M, Ejskjaer N, Witte DR, Borch-Johnsen K, Lauritzen T, Sandbaek A. Prevalence of neuropathy and peripheral arterial disease and the impact of treatment in people with screen-detected type 2 diabetes: the ADDITION-Denmark study. Diabetes Care (2011) 34(10):2244–9. doi: 10.2337/dc11-0903
38. Liu J, Yuan X, Liu J, Yuan G, Sun Y, Zhang D, et al. Risk factors for diabetic peripheral neuropathy, peripheral artery disease, and foot deformity among the population with diabetes in Beijing, China: A multicenter, cross-sectional study. Front Endocrinol (Lausanne) (2022) 13:824215. doi: 10.3389/fendo.2022.824215
39. Stratton IM, Holman RR, Boulton AJ. Risk factors for neuropathy in UKPDS. Diabetologia (2004) 47(Suppl 1):A47.
40. Pang C, Yu H, Cai Y, Song M, Feng F, Gao L, et al. Vitamin D and diabetic peripheral neuropathy: A multi-centre nerve conduction study among Chinese patients with type 2 diabetes. Diabetes Metab Res Rev (2023):e3679. doi: 10.1002/dmrr.3679
41. Nie C, Bao HP. Analysis of the related risk factors of diabetic peripheral neuropathy. Zhonghua Shi Yan He Lin Chuang Bing Du Xue Za Zhi. (2012) 26(6):467–9.
42. Morkrid K, Ali L, Hussain A. Risk factors and prevalence of diabetic peripheral neuropathy: A study of type 2 diabetic outpatients in Bangladesh. Int J Diabetes Dev Ctries. (2010) 30(1):11–7. doi: 10.4103/0973-3930.60004
43. Wada R, Yagihashi S. Role of advanced glycation end products and their receptors in development of diabetic neuropathy. Ann N Y Acad Sci (2005) 1043:598–604. doi: 10.1196/annals.1338.067
44. Vincent AM, Perrone L, Sullivan KA, Backus C, Sastry AM, Lastoskie C, et al. Receptor for advanced glycation end products activation injures primary sensory neurons via oxidative stress. Endocrinology (2007) 148(2):548–58. doi: 10.1210/en.2006-0073
45. Bikbova G, Oshitari T, Bikbov M. Diabetic neuropathy of the retina and inflammation: perspectives. Int J Mol Sci (2023) 24(11):9166. doi: 10.3390/ijms24119166
46. Lin Q, Li K, Chen Y, Xie J, Wu C, Cui C, et al. Oxidative stress in diabetic peripheral neuropathy: pathway and mechanism-based treatment. Mol Neurobiol (2023) 60(8):4574–94. doi: 10.1007/s12035-023-03342-7
47. Qi L, Gao R, Chen Z, Lin D, Liu Z, Wang L, et al. Liraglutide reduces oxidative stress and improves energy metabolism in methylglyoxal-induced SH-SY5Y cells. Neurotoxicology (2022) 92:166–79. doi: 10.1016/j.neuro.2022.08.007
48. Pandey S, Mangmool S, Madreiter-Sokolowski CT, Wichaiyo S, Luangmonkong T, Parichatikanond W. Exendin-4 protects against high glucose-induced mitochondrial dysfunction and oxidative stress in SH-SY5Y neuroblastoma cells through GLP-1 receptor/Epac/Akt signaling. Eur J Pharmacol (2023) 954:175896. doi: 10.1016/j.ejphar.2023.175896
49. Liu WJ, Jin HY, Lee KA, Xie SH, Baek HS, Park TS. Neuroprotective effect of the glucagon-like peptide-1 receptor agonist, synthetic exendin-4, in streptozotocin-induced diabetic rats. Br J Pharmacol (2011) 164(5):1410–20. doi: 10.1111/j.1476-5381.2011.01272.x
50. Jolivalt CG, Fineman M, Deacon CF, Carr RD, Calcutt NA. GLP-1 signals via ERK in peripheral nerve and prevents nerve dysfunction in diabetic mice. Diabetes Obes Metab (2011) 13(11):990–1000. doi: 10.1111/j.1463-1326.2011.01431.x
51. Himeno T, Kamiya H, Naruse K, Harada N, Ozaki N, Seino Y, et al. Beneficial effects of exendin-4 on experimental polyneuropathy in diabetic mice. Diabetes (2011) 60(9):2397–406. doi: 10.2337/db10-1462
52. Tsukamoto M, Niimi N, Sango K, Takaku S, Kanazawa Y, Utsunomiya K. Neurotrophic and neuroprotective properties of exendin-4 in adult rat dorsal root ganglion neurons: involvement of insulin and RhoA. Histochem Cell Biol (2015) 144(3):249–59. doi: 10.1007/s00418-015-1333-3
53. Kornelius E, Tsou SH, Chang CC, Ho YJ, Lin SC, Chen WL, et al. Liraglutide attenuates glucolipotoxicity-induced RSC96 schwann cells’ Inflammation and dysfunction. Biomolecules (2022) 12(10):1338. doi: 10.3390/biom12101338
54. Gumuslu E, Cine N, Ertan M, Mutlu O, Komsuoglu Celikyurt I, Ulak G. Exenatide upregulates gene expression of glucagon-like peptide-1 receptor and nerve growth factor in streptozotocin/nicotinamide-induced diabetic mice. Fundam Clin Pharmacol (2018) 32(2):174–80. doi: 10.1111/fcp.12329
55. Zhang Y, Zhang Q, Bai Y, Zheng H, Ji L, Zhu X, et al. Glycogen synthesis kinase-3beta involves in the analgesic effect of liraglutide on diabetic neuropathic pain. J Diabetes Complications (2023) 37(3):108416. doi: 10.1016/j.jdiacomp.2023.108416
56. Sullivan SD, Alfonso-Cristancho R, Conner C, Hammer M, Blonde L. A simulation of the comparative long-term effectiveness of liraglutide and glimepiride monotherapies in patients with type 2 diabetes mellitus. Pharmacotherapy (2009) 29(11):1280–8. doi: 10.1592/phco.29.11.1280
57. Brock C, Hansen CS, Karmisholt J, Moller HJ, Juhl A, Farmer AD, et al. Liraglutide treatment reduced interleukin-6 in adults with type 1 diabetes but did not improve established autonomic or polyneuropathy. Br J Clin Pharmacol (2019) 85(11):2512–23. doi: 10.1111/bcp.14063
58. Ponirakis G, Abdul-Ghani MA, Jayyousi A, Almuhannadi H, Petropoulos IN, Khan A, et al. Effect of treatment with exenatide and pioglitazone or basal-bolus insulin on diabetic neuropathy: a substudy of the Qatar Study. BMJ Open Diabetes Res Care (2020) 8(1):e001420. doi: 10.1136/bmjdrc-2020-001420
59. Issar T, Kwai NCG, Poynten AM, Arnold R, Milner KL, Krishnan AV. Effect of exenatide on peripheral nerve excitability in type 2 diabetes. Clin Neurophysiol (2021) 132(10):2532–9. doi: 10.1016/j.clinph.2021.05.033
60. Lin DS, Yu AL, Lo HY, Lien CW, Lee JK, Chen WJ. Major adverse cardiovascular and limb events in people with diabetes treated with GLP-1 receptor agonists vs SGLT2 inhibitors. Diabetologia (2022) 65(12):2032–43. doi: 10.1007/s00125-022-05772-9
61. Garcia-Hernandez P, Arechavaleta-Granell Mdel R, Yamamoto J, Falahati A, Gonzalez-Galvez G, Grupo de Investigadores de L-eM. Liraglutide and glimepiride on glycaemic control in type 2 diabetes in the Mexican cohort (LEAD 3). Rev Med Inst Mex Seguro Soc (2010) 48(5):543–8.
62. Abdul-Ghani M, Migahid O, Megahed A, Adams J, Triplitt C, DeFronzo RA, et al. Combination therapy with exenatide plus pioglitazone versus basal/bolus insulin in patients with poorly controlled type 2 diabetes on sulfonylurea plus metformin: the Qatar study. Diabetes Care (2017) 40(3):325–31. doi: 10.2337/dc16-1738
63. Goldman MP, Corriere MA, Craven T, Davis RP, Sheehan M, Hurie JB, et al. Evaluation of neuropathy, glycemic control, and revascularization as risk factors for future lower extremity amputation among diabetic patients. Ann Vasc surg (2021) 73:254–63. doi: 10.1016/j.avsg.2020.10.022
Keywords: diabetic peripheral neuropathy, glucagon-like peptide-1 receptor agonists, neuroprotection, inflammation, oxidative stress
Citation: Liu C, Wu T and Ren N (2024) Glucagon-like peptide-1 receptor agonists for the management of diabetic peripheral neuropathy. Front. Endocrinol. 14:1268619. doi: 10.3389/fendo.2023.1268619
Received: 28 July 2023; Accepted: 27 December 2023;
Published: 19 January 2024.
Edited by:
Habib Yaribeygi, Semnan University of Medical Sciences, IranReviewed by:
Athanasia K. Papazafiropoulou, Tzaneio Hospital, GreeceCopyright © 2024 Liu, Wu and Ren. This is an open-access article distributed under the terms of the Creative Commons Attribution License (CC BY). The use, distribution or reproduction in other forums is permitted, provided the original author(s) and the copyright owner(s) are credited and that the original publication in this journal is cited, in accordance with accepted academic practice. No use, distribution or reproduction is permitted which does not comply with these terms.
*Correspondence: Na Ren, bmFmYW43NDU0OTY1N0AxNjMuY29t
Disclaimer: All claims expressed in this article are solely those of the authors and do not necessarily represent those of their affiliated organizations, or those of the publisher, the editors and the reviewers. Any product that may be evaluated in this article or claim that may be made by its manufacturer is not guaranteed or endorsed by the publisher.
Research integrity at Frontiers
Learn more about the work of our research integrity team to safeguard the quality of each article we publish.