- 1Hunan Provincial Key Laboratory of the Research and Development of Novel Pharmaceutical Preparations, Changsha Medical University, Changsha, Hunan, China
- 2Xiangya School of Pharmaceutical Sciences, Central South University, Changsha, Hunan, China
Sarcopenia and diabetes are two age-related diseases that are common in the elderly population, and have a serious effect on their general health and quality of life. Sarcopenia refers to the progressive loss of muscle mass, strength and function, whereas diabetes is a chronic disease characterized by elevated blood sugar levels. The comorbidity of sarcopenia and diabetes is particularly concerning, as people with diabetes have a higher risk of developing sarcopenia due to the combination of insulin resistance, chronic inflammation and reduced physical activity. In contrast, sarcopenia destroyed blood sugar control and exacerbated the development of people with diabetes, leading to the occurrence of a variety of complications. Fortunately, there are a number of effective treatment strategies for sarcopenia in people with diabetes. Physical exercise and a balanced diet with enough protein and nutrients have been proved to enhance the muscular quality and strength of this population. Additionally, pharmacological therapies and lifestyle changes can optimize blood sugar control, which can prevent further muscle loss and improve overall health outcomes. This review aims to summarize the pathogenesis and comprehensive treatment strategies of sarcopenia in elderly patients with type 2 diabetes, which help healthcare professionals recognize their intimate connection and provide a new vision for the treatment of diabetes and its complications in this population. Through early identification and comprehensive treatment, it is possible to improve the muscle function and general quality of life of elderly with diabetes and sarcopenia.
1 Introduction
Sarcopenia is defined as a disease characterized by progressive loss of skeletal muscle mass and function, which usually occurred in the elderly (1, 2). The loss of skeletal muscle mass and function seriously affect the physical health and quality of life of individuals, leading to difficulties in daily activities and increased risk of falls and fractures, even shortened life expectancy of the elderly (3). Risk factors leading to sarcopenia include age, unbalanced diet and physical inactivity. In addition, inflammation and chronic diseases may accelerate its development (4). In 2016, sarcopenia was officially recognized as a disease with distinctive characteristics by the medical community (5). The prevalence of sarcopenia is approximately 10% in individuals aged 60 and above, whereas it reaches 20% to 30% in those aged 80 and above (6, 7). In view of the aging trend of global population, sarcopenia has emerged as an important health concern. The global population aged 65 years or above is expected to increase from 9% in 2019 to nearly 17% in 2050, which increase the risk of sarcopenia (8).
Diabetes mellitus is a chronic metabolic disease, which is mainly characterized by elevated blood sugar levels (9, 10). More than 60% of individuals aged 65 and above have diabetes, while 50% of the elderly people have prediabetes (11). According to the estimation of the International Diabetes Federation, the number of people with diabetes will reach up to 783 million by 2045 (12). Type 2 diabetes mellitus (T2DM) is the most common type, accounting for more than 90% of people with diabetes (13–15). Persistent hyperglycemia can lead to a range of complications, which have a serious impact on the cardiovascular system, kidneys and eyes, thereby posing serious threats to the overall health (16–19). The complications and severity of diabetes lead to a considerable morbidity and mortality rate, make it one of the most urgent worldwide public health problems (20).
Sarcopenia and diabetes in the elderly are intricately linked and have a negative impact on each other (21). Sayani Das examines the relationship between diabetes and sarcopenia in India’s elderly population revealed that individuals with diabetes had a significantly higher risk of sarcopenia (22). Moreover, recent meta-analyses have revealed that the prevalence of sarcopenia is two to three times greater among patients with diabetes in comparison to those without the condition (23, 24). Osaka et al.’s study further underscored the connection by indicating that sarcopenia elevates the risk of nephropathy in people with diabetes (25). According to Feng et al.’ study, the pooled prevalence of sarcopenia in people with diabetes reached up to 18%, which has become an urgent situation to be prevented early (26). The purpose of this paper is to review the pathogenesis and comprehensive treatment strategies of sarcopenia in patients with T2DM, which aims to help clinicians and nutritionists to identify and intervene at an early stage, ultimately enhancing the overall health and well-being of these patients.
2 Sarcopenia
2.1 Definition and prevalence of sarcopenia
In 1989, the term sarcopenia was initially coined by Rosenberg in 1989 (27, 28). The European Working Group on Sarcopenia in the Elderly (EWGSOP) first defined sarcopenia in 2010 as “age-related, generalized decrease in muscle mass and/or decrease in muscle strength or muscle physiology” (29). A similar agreement was subsequently announced in 2014 by the Asian Sarcopenia Working Group, who also altered the pertinent diagnostic cutoff for the Asian population (30). Sarcopenia was recognized as a disease on a global scale and included in the ICD-10 (M62.84) in 2016 (5). The European Sarcopenia Working Group presented a revised definition of sarcopenia in 2018 and an explanation of muscular function. Sarcopenia was defined as the gradual loss of muscle mass, strength, and muscle function (31). It is difficult to determine the prevalence of sarcopenia consistently since different cutoffs and measurement methods result in dramatically different incidences of the disease across studies. According to a meta-analysis of studies, the prevalence in adults over 60 ranges from 10 to 27 percent (32). The number of sarcopenia patients worldwide will rise year after year as the population ages.
2.2 Diagnosis process of sarcopenia
The diagnostic process for sarcopenia was updated by the European Working Group on Sarcopenia in the Elderly as Find-Assess-Confirm-Severity, as illustrates in Figure 1, noting that sarcopenia is considered severe when low muscle strength, low muscle mass and low physical function are all detected (31).
2.2.1 SARC-F questionnaire
The European Working Group on Sarcopenia in the Elderly recommends using the SARC-F questionnaire to assess sarcopenia risk. The SARC-F is a simple five-item self-assessment questionnaire created by Morely (33). SARC-F is a rapid and simple screening method for sarcopenia in the elderly, which has been used widely used worldwide (34). Strength, assisted walking, getting up, climbing stairs, and falling are all assessed. A sarcopenia risk is present if the sum of the scores for each of the five items is greater than or equal to 4 points providing that the maximum total score is 10 points (35). However, due to the high specificity and relatively low to moderate sensitivity of SARC-F in predicting sarcopenia, many patients with sarcopenia may go misjudged (36–39). As a result, the SARC-CalF approach has been proposed, which adds a maximum leg circumference based on SARC-F. The results of several researches indicated that this method significantly improves the sensitivity and accuracy of evaluation (40, 41).
2.2.2 Muscle strength
Muscle strength is the maximal force that one or more muscular groups may generate. The European Working Group on Sarcopenia in the Elderly regards muscle strength as the main reference index for sarcopenia. It is recommended to utilize grip strength as an additional measure when assessing muscular strength because it has a relatively positive correlation in other body sections and is simple to use (42, 43). Upper limb muscle grip strength, which can be measured by a manual ergometer, is typically used to assess grip strength in the upper limbs. The chair stand test measures how quickly patients can stand up from the chair without the aid of their arms, and to assess the muscle strength of their lower limbs (31).
2.2.3 Muscle mass
Muscle mass refers to the total amount of skeletal muscle in the body. Computed tomography (CT), magnetic resonance imaging (MRI), dual-energy X-ray absorptiometry (DXA), and bioelectrical impedance analysis (BIA) are common used to estimate the skeletal muscle mass (31). CT and MRI are the current gold standard for assessing muscle mass with high accuracy and reproducibility. Their use is restricted due to their high price, complexity, and high radiation exposure from CT (44). DXA is considered to be one of the ideal methods for determining muscle mass with its quick operation and minimal radiation exposure (45). However, DXA equipment cannot be widely used in hospitals and communities since it is difficult to carry. Instead, due to its simplicity, portability, and affordability, BIA is better suited for extensive hospitals and communities screening and can be used as a less expensive alternative to assess muscle mass (29, 44, 45).
2.2.4 Muscle function
Muscle function is defined as objectively measured exercise-related systemic function. Its testing methods include daily pace assessment, short-physical performance battery (SPPB), the chair rising test (CRT), and stand-up and go test (Time-up and go test, TUG). Daily Gait speed is a quick, secure and reliable sarcopenia test, which can indicate the health status and is related to their survival possibility of the elderly (46). A walking speed of less than 0.8 m/s is suggested by EWGSOP2 as a sign of severe sarcopenia (31). SPPB is a compound test method of muscle function, which is a standard method in both research and clinical application and is widely used (47, 48). Three tests are included in SPPB, with the highest score of 4 points and the full score of 12 points for each individual test. The risk of poor muscle function is indicated by a score of 9 or less.
2.3 Risk factors of sarcopenia
2.3.1 Intrinsic factors
Sarcopenia is a complicated and multifactorial process, which is influenced by both intrinsic and extrinsic factors, as depicted in Figure 2. Age is considered as a key intrinsic risk factor of sarcopenia. Because age not only directly affects muscle quality and function, but also indirectly increases the risk of sarcopenia by bringing some problems such as hormone disorder, mitochondrial damage and chronic inflammation. With the increase of individual age, the metabolic rate of human body gradually decreases. This metabolic leads to the inhibition of protein synthesis, thereby impairing muscle repair and regeneration capabilities (1). Age-related muscle tissue alterations include serious loss of muscle mass, modifications in muscle fiber morphology, deterioration of muscle function, and reduction of muscle contraction time and strength. Consequently, sarcopenia is widely regarded as an age-related disease (49). Furthermore, age also affects the structure and function of skeletal muscle fibers. As age advances, there is a gradual decrease in the quantity and size of muscle fibers with the skeletal muscle, along with an increase in the presence of fat and connective tissue within the muscle matrix, all of which contribute to the degradation of muscle mass (50). Therefore, it can be seen that age is an important factor of sarcopenia, which affects the health and function of muscles in many ways.
Moreover, the imbalance of the human endocrine system and the fluctuation of hormone levels caused by aging contribute to an increased risk of sarcopenia by affecting skeletal muscle function. An obvious example is that with adult man ages, the decrease of muscle mass and strength is closely related to steady decline testosterone levels (51). As skeletal muscle undergoes the aging process, the level of reactive oxygen species (ROS) gradually increases, disrupting the body’s ability to maintain oxidative balance. This disruption decreases myogenic differentiation and triggers regenerative errors during the skeletal muscle degeneration (52–55). ROS are mainly produced in mitochondria, which also serve as the primary target of intracellular oxidative stress. High levels of ROS can damage mitochondria, resulting in abnormal mitochondrial function, and potentially contributing to the development of sarcopenia (56–58). In addition, the proliferative and differentiation capabilities of satellite cells decline significantly with age, compromising the regenerative potential of muscle cells. These changes result in fibrosis of aging muscle, thereby considered as a pivotal factor in the initiation of sarcopenia (59–61).
Chronic inflammation related to aging is another important risk factor for sarcopenia (62). The increase of pro-inflammatory cytokine levels and the decrease of anti-inflammatory cytokine levels in vivo are indicators to assess chronic inflammation. Loss of muscle mass and strength is associated with an increase in inflammatory markers in the blood, which reduces lower limb function and physical activity (63, 64). According to several horizontal and longitudinal studies, sarcopenia is associated with high levels of proinflammatory cytokines, including tumor necrosis factor-α (TNF-α), interleukin-6 (IL-6), and C-reactive protein (CRP). These inflammatory factors are secreted by inflammatory and immune cells, which inhibit muscle synthesis and promote muscle degradation, consequently result in sarcopenia (65–67).
2.3.2 Extrinsic factors
The decline of exercise ability are the main reasons for the loss of muscle mass and strength in the elderly (68). Muscle tissue is highly plastic, which needs constant participation and stimulation under load to maintain the proper metabolism and repair functions. Lack of exercise will result in insufficient muscle stimulation, deceleration of protein synthesis, and decrease of muscle mass (69). Moreover, fear of falling causes psychological burden to the elderly, which makes them unwilling to do exercise and increases the risk of muscle loss. At the same time, physical inactivity can easily lead to obesity in the elderly. The weight of the elderly mostly increases in the form of fat rather than thin tissue, and the occurrence of sarcopenia is usually accompanied by the growth of adipose tissue, which promotes each other (70).
In addition to physical inactivity, it is worthing noting that the elderly who have been engaged in a sedentary lifestyle for a long time face a higher risk of sarcopenia. When sedentary time exceeds 2 hours, even physically active elderly people are prone to sarcopenia, which emphasizes that physical activity itself cannot offset the harmful consequences of long-term inactivity (71). Although muscle mass and muscle fibers will naturally decrease with age, the risk of sarcopenia can be effectively reduced by performing moderate to high-intensity physical activities instead of sitting for a long time (72).
Other extrinsic factors such as muscle disuse caused by physical trauma, chronic stress and long sleep will increase the risk of muscle loss (73–75). In addition, long-term exposure to certain pollutants or toxins will adversely affect muscle health and overall health. Extreme weather conditions or geographical location may affect individuals’ ability to engage in outdoor activities and obtain fresh food, thus increasing the risk of sarcopenia. It must be recognized that the progress of sarcopenia is a dynamic process, and the early symptoms are often difficult to find, which leads to the neglect of this disease. In fact, it is very common for most elderly people to take this problem seriously only when pathological conditions and adverse consequences appear. Therefore, it is very important to pay attention to sarcopenia in the elderly at an early stage.
3 Pathogenesis of interaction between sarcopenia and T2DM
3.1 Insulin resistance and muscle mass
Insulin resistance is considered as the main pathophysiological basis of T2DM, which plays an important role in the occurrence and development of sarcopenia (76–78). Insulin is very important to control metabolism, and its main function is to promote protein synthesis, muscle growth and the absorption and utilization of glucose by muscle tissue (79). Insulin resistance occurs when the sensitivity and reactivity of insulin receptors decrease. As a result, blood sugar fails to effectively enter muscle tissue. This leads to insufficient nutrition supply of muscle tissue, which leads to muscle atrophy and reduction (80). Skeletal muscle can absorb 75% of blood sugar through insulin signaling system (81). Therefore, muscle cells with insulin signaling system problems will not be able to effectively use the glucose in the blood stream, leading to accelerated protein decomposition and accelerated muscle loss (82–84). Low muscle mass may make blood sugar control disorder, further aggravating the development of diabetes (78).
3.2 Hyperglycemia
Hyperglycemia is a significant risk factor leading to the loss of muscle mass and function during aging (85), which affect the development and progress of sarcopenia through various mechanisms. Firstly, hyperglycemia leads to an increase in the formation of advanced glycation end products, which accumulate in muscle tissue, destroy muscle contraction and endothelial function, and finally worsen muscle function (86). Secondly, the oxidative stress and inflammatory reaction caused by hyperglycemia will lead to muscle cell damage and cell death (87, 88). In addition, the increase of blood sugar level declines the responsiveness of muscle cells to insulin, which reduces the absorption and utilization of glucose and amino acids by these cells. Muscle tissue cannot get enough nutritional support, leading to the aggravation of sarcopenia (89). Lastly, hyperglycemia affects the energy metabolism of muscle cells by destroying the balance between glycogen production and decomposition. This disorder leads to the decrease of glycogen storage in muscle cells, which leads to the insufficient energy supply of these cells and accelerates the onset of sarcopenia (90). In a word, hyperglycemia poses many threats to muscle health in the process of aging, affecting the structure and function of muscle tissue.
3.3 Oxidative stress and inflammatory reaction
Muscle atrophy in people with diabetes mainly comes from oxidative stress and inflammation. Chronic inflammation, oxidative stress, insulin resistance, the formation of advanced glycation end products and hyperglycemia are all linked to cellular redox imbalance (88, 91). This factors together hinder the metabolism of protein in skeletal muscle, leading to increased oxidative damage of DNA, lipids and protein (92). The increase of lipid metabolites in patients with T2DM can lead to excessive production of ROS. This overproduction can be alleviated by antioxidant enzymes, which play a vital role in regulating muscle regeneration, inducing angiogenesis and reducing muscle fibrosis. Furthermore, the high level of ROS will reduce muscle fiber size and muscle mass, destroy muscle homeostasis, interfere with muscle production, and thus aggravate muscle atrophy (93). Inflammatory response is a complex and important biological mechanism, which responds to pathogens and injury stimuli by activating immune cells and releasing inflammatory mediators, thus protecting the body against injury (94). However, persistent chronic inflammation may represent a potential pathological condition that damages muscle tissue and promote the development and progress of sarcopenia (95).
3.4 Sarcopenic obesity
Sarcopenic obesity (SO) is defined as the co-existence of obesity and sarcopenia in a consensus achieved by the European Society for Clinical Nutrition and Metabolism and the European Association for the Study of Obesity (ESPEN-EASO) in 2022 (96). The prevalence of sarcopenic obesity is high among the elderly, with about one in every ten people suffering from it (97). A large number of studies have shown that SO can increase more negative effects than sarcopenia or obesity alone, including a significant increase in the probability of falling, disability (98). Obesity exists in both people with sarcopenia and diabetes. A study shows that more than half of diabetics are obese at the same time (99). Therefore, the coexistence of SO and T2D has become a common health problem. Studies have shown that the prevalence of SO in diabetic patients is as high as 27%, and it is related to serious adverse consequences (100). Obesity and T2D are one of the main causes of the dysfunction of skeletal muscle stem cell regeneration, which is manifested by the loss of muscle content and the progressive decline of glucose and lipid metabolism of skeletal muscle, thus accelerating the process of diabetes and entering a vicious circle (101). Therefore, we should pay attention to the early screening and identification of SO in people with diabetes, and choose appropriate intervention measures to reduce the incidence and various adverse outcomes in this population.
4 Comprehensive treatment strategies
4.1 Control of blood sugar level
Diabetes is a chronic disease characterized by elevated blood sugar level, which can lead to the occurrence of various complications. Among these, muscle loss, known as sarcopenia, is a common complication influenced by high blood sugar. The decrease of mitochondrial activity induced by hyperglycemia aggravates insulin resistance and impairs energy metabolism (102). Furthermore, the inflammatory responses triggered by hyperglycemia will further lead to insulin resistance and muscle atrophy by inhibiting insulin signaling (103). Therefore, blood sugar control is crucial for people with diabetes and sarcopenia. Effective management of blood sugar level can slow down the rate of muscle loss and reduce protein decomposition. In well-controlled people with diabetes, stable insulin level contributes to the synthesis of muscle protein and promotes muscle quality and strength. This can not only prevent the onset of sarcopenia, but also accelerate muscle growth and repair, and ultimately enhance the overall muscle quality and body function. Skeletal muscle, as the most important exercise organ of the human body, assist in storing protein, regulating glucose metabolism and stabilizing blood sugar level, contributing to overall health of the body (81, 104, 105).
4.2 Nutrition intervention
Compared with the general population, people with diabetes face a higher risk of malnutrition and are more prone to sarcopenia. Nutritional therapies have the potential to prevent or even reverse sarcopenia and frailty (106). Two elderly patients with malignant hematological diseases were studied by Matsunaga et al., and the effectiveness of rehabilitation treatment on sarcopenia was reported (107). Chan et al.’s study noted that expanding nutritional counseling for people with diabetes and effectively reduce the incidence of sarcopenia (108). Several studies demonstrate that diet is no obvious evident benefit for elderly with diabetes and sarcopenia (102, 103, 109). However, the majorities of studies have consistently shown the positive effects of diet and nutrition intervention in the prevention and control of sarcopenia (110–119). Moreover, for many elderly people who cannot exercise, nutritional intervention is still the most promising treatment and prevention strategy (120, 121). Therefore, it is crucial to emphasize the importance of a balanced diet, comprehensive nutrition and vigilant monitoring of diabetes and malnutrition in the elderly. The comprehensive overview of the relationship between nutritional intervention and sarcopenia is shown in Table 1.
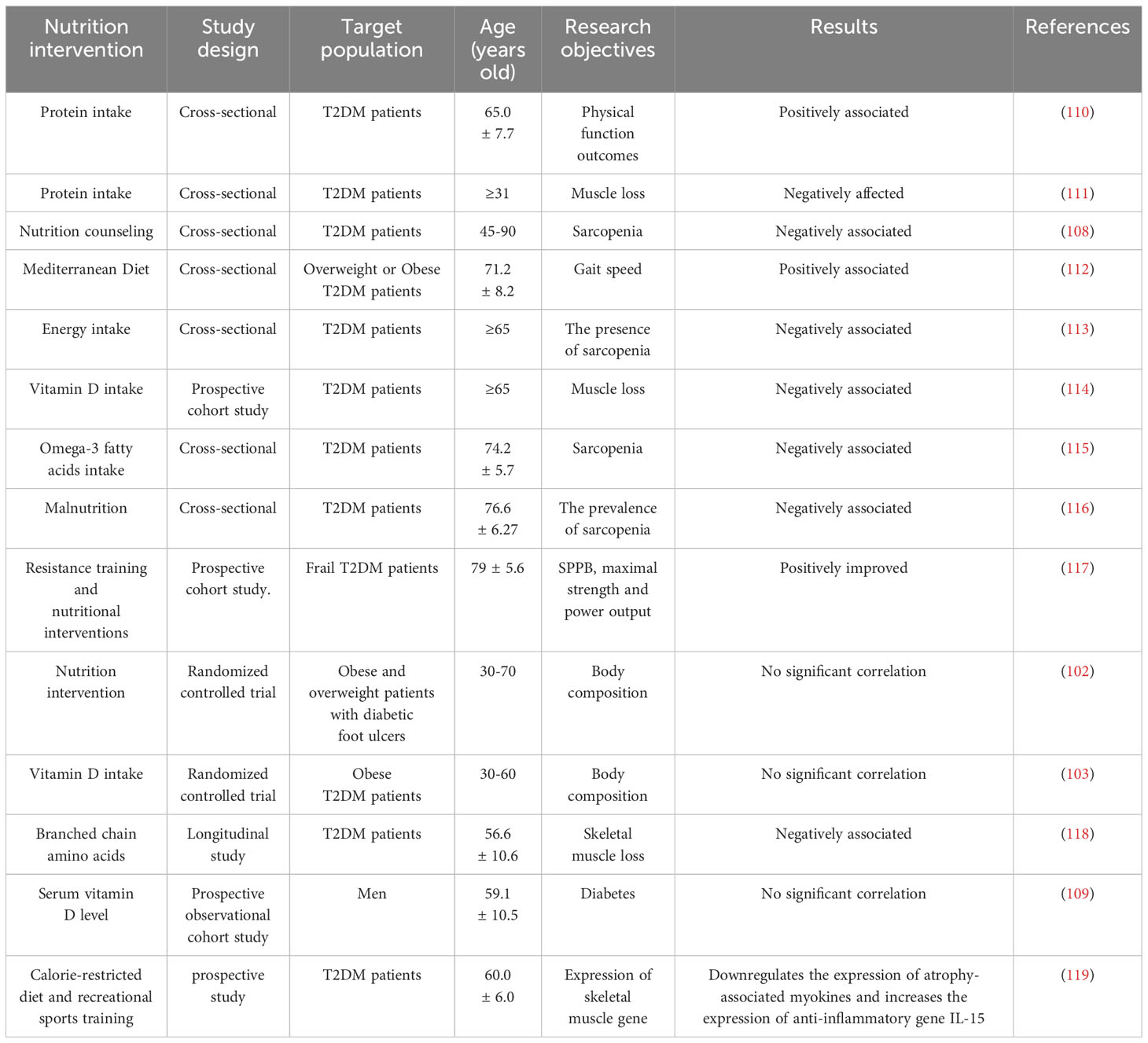
Table 1 Studies on the effect of nutritional intervention on sarcopenia in elderly people with diabetes.
4.2.1 Protein
Protein intake is beneficial to the elderly people with both sarcopenia and T2DM. Increasing protein’s habitual intake can not only effectively improve blood sugar control and maintain muscle mass, but also promote weight control, reduce inflammation and increase insulin sensitivity (122). Protein is a key basic element of muscle function and an indispensable nutrient for muscle synthesis. Wu et al. revealed that the increase of protein intake was related to the increase of grip strength and the decrease of prevalence of sarcopenia (123). Many studies have consistently confirmed that adequate intake of protein can not only stimulate the synthesis of protein in muscle, but also reduce the degradation process of protein and improve the effective utilization of nutrients in muscle (124–126). In addition, proper protein intake has a positive effect on blood sugar regulation, which helps to reduce the risk of diabetes. Protein intake can directly promote postprandial insulin secretion, which may be beneficial to the blood sugar regulation of elderly T2D patients (127). Studies have pointed out that the elderly with sarcopenia should consume 1.6g/kg of protein every day, and the ESPEN expert group and the PROT-AGE research group also suggested that people with chronic diseases (such as diabetes) should consume about 1.2-1.5g/kg/day of protein (128–130). Therefore, higher protein intake should be encouraged for the elderly people with sarcopenia and T2DM.
4.2.2 Essential amino acids
Amino acids are the basic units of muscle protein, and the impact of protein consumption on the rate of muscle synthesis mainly focus on essential amino acids (131, 132). These essential amino acids play a vital role in both muscle synthesis and maintenance, demonstrating a linear relationship with muscle protein synthesis within a specific dosage range (132). In particular, leucine stands out because of its remarkable stimulating effect (133–135). Long-term continuous intake of essential amino acids rich in leucine is related to significant enhancement of skeletal muscle mass, strength, and walking speed in the elderly (136). Rieu et al. studied the completely balanced diet of 20 healthy male subjects with or without leucine, and found that leucine is effective in promoting the synthesis of muscle protein and counteracting the influence of sarcopenia (137). A study emphasizes that leucine can act as insulin secretagogue to effectively improve postprandial blood glucose control and promote the synthesis of muscle protein (138). Therefore, ensuring adequate dietary intake of protein and essential amino acids has become a key factor to maintain overall health and function.
4.2.3 Vitamin D and calcium
Vitamin D play an important role in maintaining muscle health and has a direct impact on muscle development. Vitamin D is a fat-soluble nutrient that strengthens bone health by promoting the absorption of essential minerals such as calcium and phosphorus. A paired case-control study by Yang et al. showed that vitamin D deficiency was associated with an increased risk of sarcopenia (139). Compared with young women with sufficient vitamin D level, young women with insufficient vitamin D level showed a decrease in muscle mass, and at the same time, the fat penetration in muscle increased by about 24%, further highlighting the far-reaching influence of vitamin D on muscle strength (140). In addition, compared with people with sufficient vitamin D, the grip strength of the elderly with low serum vitamin D level is significantly reduced (141). Visser et al. conducted a longitudinal aging study and found that vitamin D deficiency is not only associated with the weakening of muscle function and the onset of sarcopenia, but also increases the risk of osteoporosis and fracture (142). Individuals with sarcopenia consume lesser essential nutrients such as calcium, iron and zinc than the general population, further aggravating the problems related to muscle health (143). Encouragingly, studies involving elderly women show that continuous supplementation of vitamin D and calcium can significantly improve skeletal muscle performance and reduce the incidence of falls (144). These comprehensive studies emphasize the potential benefits of vitamin D and calcium supplements in promoting muscle health, especially in the elderly and individuals with chronic diseases.
4.2.4 Omega-3 fatty acids
A growing body of evidences emphasize the potential role of omega-3 fatty acids in preserving and regulating the quality and function of skeletal muscle. Robinson et al.’ study on diet and its relationship to grip strength in community-dwelling older men and women revealed a positive correlation between eating fatty fish and increasing grip strength (145). Dos Reis et al.’s study revealed that the intake of omega-3 fatty acids is negatively correlated with the incidence of sarcopenia, and a favorable relationship with the skeletal muscle mass index of limbs was also proved (146). A systematic meta-analysis conducted by Bird et al. further supported these findings, emphasizing the positive effects of supplementation of omega-3 long-chain polyunsaturated fatty acids on muscle quality and strength (147). A study showed that omega-3 fatty acid supplements are also expected to reduce inflammation (148). Rats fed with a combination of omega-3 polyunsaturated fatty acids derived from fish oil and wheat oligopeptides showed significant potential to prevent age-related muscle loss and reduced oxidative stress and inflammation in skeletal muscle (149). The comprehensive findings of these studies demonstrate that omega-3 fatty acids have positive and multifaceted potential in muscle protection and inflammation reduction, and are expected to become a potential nutritional support strategy for elderly patients with sarcopenia.
4.3 Physical exercise
Physical exercise is considered as a safe and efficient intervention measures to prevent and treat diabetes mellitus complicated with sarcopenia. Participating in any form of physical activity can significantly improve sarcopenia in the elderly, which has been supported by a lot of research (116, 150–157), as shown in Table 2. On a global scale, physical exercise occupies a central position as the main prevention and treatment method of sarcopenia (31). Studies from different countries have consistently emphasized the far-reaching advantages of regular physical activity in maintaining muscle quality and function (158–162). Studies have emphasized the dual benefits of aerobic exercise and anaerobic exercise in reducing insulin resistance. Aerobic exercise can especially enhance insulin sensitivity, while resistance training and strength training can also significantly improve insulin resistance (163, 164). For those elderly who struggle with sarcopenia, physical exercise, with special emphasis on resistance training, is considered as an effective strategy to combat the decline of activity ability related to age-related chronic diseases (165–168). When combined with nutritional supplements, resistance training can maximize its intervention (169, 170). According to international guidelines, it is recommended that patients with T2DM participate in resistance training at least twice a week (171). Therefore, it is imperative to incorporate resistance training into the exercise guide tailored for the elderly.
4.4 Selection of antidiabetic drugs
The effect of antidiabetic drugs on sarcopenia is still ongoing research topic, with certain drugs demonstrating unclear effects. Some antidiabetic drugs, particularly long-term use of sulfonylurea drugs, such as glinide, gliclazide and glibedi, have been associated with muscle loss or injury. These drugs play a role by inhibiting KATP channel to stimulate insulin secretion (172). According to a large number of clinical reports, this mechanism is related to rapid skeletal muscle atrophy (173–175). On the contrary, some antidiabetic drugs show promise in maintaining muscle quality. In a study by Lee et al., it was observed that elderly men with diabetes experienced rapid skeletal muscle loss, but those who used insulin sensitizers such as metformin or thiazolidinedione lost significantly less weight than those who did not receive such treatment. This indicates that insulin sensitizer may weaken muscle loss (176). Some other studies have also shown that insulin administration can increase the skeletal muscle mass or gait speed of patients with diabetes, which may slow down sarcopenia in patients with T2DM (177–179). However, it is impossible to overlook the weight gain caused by insulin use (180). In conclusion, the effect of antidiabetic drugs on sarcopenia varies with specific drugs and individual conditions. Further clinical research is needed to fully clarify the complex relationship between antidiabetic drugs and sarcopenia.
5 Conclusion
In this study, the pathogenesis of sarcopenia in people with diabetes and comprehensive treatment strategies were summarized. There is a complex interrelationship between the two diseases. Type 2 diabetes leads to the progress of sarcopenia, and conversely, sarcopenia exacerbates the severity of diabetes. Lifestyle choice, age-related metabolic changes, elevated blood sugar levels and insulin resistance all contribute to sarcopenia in patients with T2DM. Therefore, healthcare professionals need to pay more attention to the early prevention of muscle loss in elderly diabetes patients.
Effective prevention and treatment of sarcopenia complicated by diabetes requires dietary intervention rich in protein, essential amino acids, vitamin D, calcium and other important nutrients (including omega-3 fatty acids). In addition, it is very necessary to advocate moderate exercise and physical exercise among patients with T2DM and sarcopenia. Considering all kinds of exercise types and intensities, it is very important to customize these activities according to the needs of the elderly. Equally important is the management of blood sugar level and the improvement of insulin resistance. When prescribing antidiabetic drugs, clinicians should include sarcopenia in the evaluation of its pharmacological effects. However, there is no specific drug for sarcopenia at present, which emphasizes the importance of active prevention.
Age-related sarcopenia has become an urgent global health challenge as the population ages. Diabetes is still one of the most common chronic diseases among the elderly. The complicated interaction and common pathogenic mechanism between these two diseases have a far-reaching impact on the health of the elderly. Health care professionals must give priority to the early detection, screening and diagnosis of sarcopenia in people with diabetes, and formulate comprehensive and reasonable preventive measures in time, and ultimately promote the well-being of the elderly population.
Author contributions
YH: Writing – original draft, Conceptualization, Visualization, Writing – review & editing. JX: Writing – review & editing. BW: Writing – review & editing. SD: Writing – review & editing. RS: Writing – review & editing. WZ: Supervision, Writing – review & editing. ST: Supervision, Writing – review & editing. BH: Supervision, Writing – review & editing.
Funding
The author(s) declare financial support was received for the research, authorship, and/or publication of this article. The work is supported by “The 14th Five-Year Plan” Application Characteristic Discipline of Hunan Province (Pharmaceutical Science) with Changsha Medical University, China.
Conflict of interest
The authors declare that the research was conducted in the absence of any commercial or financial relationships that could be construed as a potential conflict of interest.
Publisher’s note
All claims expressed in this article are solely those of the authors and do not necessarily represent those of their affiliated organizations, or those of the publisher, the editors and the reviewers. Any product that may be evaluated in this article, or claim that may be made by its manufacturer, is not guaranteed or endorsed by the publisher.
References
1. Cruz-Jentoft AJ, Sayer AA. Sarcopenia. Lancet (2019) 393(10191):2636–46. doi: 10.1016/s0140-6736(19)31138-9
2. Cruz-Jentoft AJ, Landi F, Topinková E, Michel JP. Understanding sarcopenia as a geriatric syndrome. Curr Opin Clin Nutr Metab Care (2010) 13(1):1–7. doi: 10.1097/MCO.0b013e328333c1c1
3. Wiedmer P, Jung T, Castro JP, Pomatto LCD, Sun PY, Davies KJA, et al. Sarcopenia - Molecular mechanisms and open questions. Ageing Res Rev (2021) 65:101200. doi: 10.1016/j.arr.2020.101200
4. Yuan S, Larsson SC. Epidemiology of sarcopenia: Prevalence, risk factors, and consequences. Metabolism (2023) 144:155533. doi: 10.1016/j.metabol.2023.155533
5. Anker SD, Morley JE, von Haehling S. Welcome to the ICD-10 code for sarcopenia. J Cachexia Sarcopenia Muscle (2016) 7(5):512–4. doi: 10.1002/jcsm.12147
6. Shafiee G, Keshtkar A, Soltani A, Ahadi Z, Larijani B, Heshmat R. Prevalence of sarcopenia in the world: a systematic review and meta- analysis of general population studies. J Diabetes Metab Disord (2017) 16:21. doi: 10.1186/s40200-017-0302-x
7. Volpato S, Bianchi L, Cherubini A, Landi F, Maggio M, Savino E, et al. Prevalence and clinical correlates of sarcopenia in community-dwelling older people: application of the EWGSOP definition and diagnostic algorithm. J Gerontol A Biol Sci Med Sci (2014) 69(4):438–46. doi: 10.1093/gerona/glt149
8. Almohaisen N, Gittins M, Todd C, Sremanakova J, Sowerbutts AM, Aldossari A, et al. Prevalence of undernutrition, frailty and sarcopenia in community-dwelling people aged 50 years and above: systematic review and meta-analysis. Nutrients (2022) 14(8):1537. doi: 10.3390/nu14081537
9. Guariguata L, Whiting DR, Hambleton I, Beagley J, Linnenkamp U, Shaw JE. Global estimates of diabetes prevalence for 2013 and projections for 2035. Diabetes Res Clin Pract (2014) 103(2):137–49. doi: 10.1016/j.diabres.2013.11.002
10. Yang YY, Chen Z, Yang XD, Deng RR, Shi LX, Yao LY, et al. Piperazine ferulate prevents high-glucose-induced filtration barrier injury of glomerular endothelial cells. Exp Ther Med (2021) 22(4):1175. doi: 10.3892/etm.2021.10607
11. American Diabetes Association Professional Practice Committee. 13. Older Adults: Standards of Medical Care in Diabetes-2022. Diabetes Care. Diabetes Care (2022) 45(Suppl 1):S195–S207. doi: 10.2337/dc22-S013
12. Magliano DJ, Boyko EJ, committee IDFDAtes. IDF Diabetes Atlas. Idf diabetes atlas Vol. 2021. . Brussels: International Diabetes Federation© International Diabetes Federation (2021).
13. Zheng Y, Ley SH, Hu FB. Global aetiology and epidemiology of type 2 diabetes mellitus and its complications. Nat Rev Endocrinol (2018) 14(2):88–98. doi: 10.1038/nrendo.2017.151
14. Cho NH, Shaw JE, Karuranga S, Huang Y, da Rocha Fernandes JD, Ohlrogge AW, et al. IDF Diabetes Atlas: Global estimates of diabetes prevalence for 2017 and projections for 2045. Diabetes Res Clin Pract (2018) 138:271–81. doi: 10.1016/j.diabres.2018.02.023
15. Kerner W, Brückel J. Definition, classification and diagnosis of diabetes mellitus. Exp Clin Endocrinol Diabetes (2014) 122(7):384–6. doi: 10.1055/s-0034-1366278
16. Stratton IM, Adler AI, Neil HA, Matthews DR, Manley SE, Cull CA, et al. Association of glycaemia with macrovascular and microvascular complications of type 2 diabetes (UKPDS 35): prospective observational study. Bmj (2000) 321(7258):405–12. doi: 10.1136/bmj.321.7258.405
17. Yang YY, Shi LX, Li JH, Yao LY, Xiang DX. Piperazine ferulate ameliorates the development of diabetic nephropathy by regulating endothelial nitric oxide synthase. Mol Med Rep (2019) 19(3):2245–53. doi: 10.3892/mmr.2019.9875
18. Mi W, Xia Y, Bian Y. Meta-analysis of the association between aldose reductase gene (CA)n microsatellite variants and risk of diabetic retinopathy. Exp Ther Med (2019) 18(6):4499–509. doi: 10.3892/etm.2019.8086
19. Chen Y, Tan S, Liu M, Li J. LncRNA TINCR is downregulated in diabetic cardiomyopathy and relates to cardiomyocyte apoptosis. Scand Cardiovasc J (2018) 52(6):335–9. doi: 10.1080/14017431.2018.1546896
20. Jiang H, Xia C, Lin J, Garalleh HAL, Alalawi A, Pugazhendhi A. Carbon nanomaterials: A growing tool for the diagnosis and treatment of diabetes mellitus. Environ Res (2023) 221:115250. doi: 10.1016/j.envres.2023.115250
21. Mesinovic J, Zengin A, De Courten B, Ebeling PR, Scott D. Sarcopenia and type 2 diabetes mellitus: a bidirectional relationship. Diabetes Metab Syndr Obes (2019) 12:1057–72. doi: 10.2147/dmso.S186600
22. Das S. The impact of diabetes on sarcopenia in community-dwelling older adults in India: key findings from the longitudinal ageing study in India (LASI). Diabetes Epidemiol Manage (2023) 12:100158. doi: 10.1016/j.deman.2023.100158
23. Chung SM, Moon JS, Chang MC. Prevalence of sarcopenia and its association with diabetes: A meta-analysis of community-dwelling Asian population. Front Med (Lausanne) (2021) 8:681232. doi: 10.3389/fmed.2021.681232
24. Qiao YS, Chai YH, Gong HJ, Zhuldyz Z, Stehouwer CDA, Zhou JB, et al. The association between diabetes mellitus and risk of Sarcopenia: accumulated evidences from observational studies. Front Endocrinol (Lausanne) (2021) 12:782391. doi: 10.3389/fendo.2021.782391
25. Osaka T, Fukui M. IDF2022-0754 Sarcopenia is a risk factor for progression of diabetic nephropathy in type 2 diabetes. Diabetes Res Clin Pract (2023) 197:110390. doi: 10.1016/j.diabres.2023.110390
26. Feng L, Gao Q, Hu K, Wu M, Wang Z, Chen F, et al. Prevalence and risk factors of sarcopenia in patients with diabetes: A meta-analysis. J Clin Endocrinol Metab (2022) 107(5):1470–83. doi: 10.1210/clinem/dgab884
28. Rosenberg IH. Sarcopenia: origins and clinical relevance. J Nutr (1997) 127(5 Suppl):990s–1s. doi: 10.1093/jn/127.5.990S
29. Cruz-Jentoft AJ, Baeyens JP, Bauer JM, Boirie Y, Cederholm T, Landi F, et al. Sarcopenia: European consensus on definition and diagnosis: Report of the European Working Group on Sarcopenia in Older People. Age Ageing (2010) 39(4):412–23. doi: 10.1093/ageing/afq034
30. Chen LK, Liu LK, Woo J, Assantachai P, Auyeung TW, Bahyah KS, et al. Sarcopenia in Asia: consensus report of the Asian Working Group for Sarcopenia. J Am Med Dir Assoc (2014) 15(2):95–101. doi: 10.1016/j.jamda.2013.11.025
31. Cruz-Jentoft AJ, Bahat G, Bauer J, Boirie Y, Bruyère O, Cederholm T, et al. Sarcopenia: revised European consensus on definition and diagnosis. Age Ageing (2019) 48(4):601. doi: 10.1093/ageing/afz046
32. Petermann-Rocha F, Balntzi V, Gray SR, Lara J, Ho FK, Pell JP, et al. Global prevalence of sarcopenia and severe sarcopenia: a systematic review and meta-analysis. J Cachexia Sarcopenia Muscle (2022) 13(1):86–99. doi: 10.1002/jcsm.12783
33. Malmstrom TK, Morley JE. SARC-F: a simple questionnaire to rapidly diagnose sarcopenia. J Am Med Dir Assoc (2013) 14(8):531–2. doi: 10.1016/j.jamda.2013.05.018
34. Bauer J, Morley JE, Schols A, Ferrucci L, Cruz-Jentoft AJ, Dent E, et al. Sarcopenia: A time for action. An SCWD position paper. J Cachexia Sarcopenia Muscle (2019) 10(5):956–61. doi: 10.1002/jcsm.12483
35. Malmstrom TK, Miller DK, Simonsick EM, Ferrucci L, Morley JE. SARC-F: a symptom score to predict persons with sarcopenia at risk for poor functional outcomes. J Cachexia Sarcopenia Muscle (2016) 7(1):28–36. doi: 10.1002/jcsm.12048
36. Woo J, Leung J, Morley JE. Validating the SARC-F: a suitable community screening tool for sarcopenia? J Am Med Dir Assoc (2014) 15(9):630–4. doi: 10.1016/j.jamda.2014.04.021
37. Bahat G, Yilmaz O, Kılıç C, Oren MM, Karan MA. Performance of SARC-F in regard to sarcopenia definitions, muscle mass and functional measures. J Nutr Health Aging (2018) 22(8):898–903. doi: 10.1007/s12603-018-1067-8
38. Yang M, Hu X, Xie L, Zhang L, Zhou J, Lin J, et al. SARC-F for sarcopenia screening in community-dwelling older adults: Are 3 items enough? Med (Baltimore) (2018) 97(30):e11726. doi: 10.1097/md.0000000000011726
39. Ida S, Murata K, Nakadachi D, Ishihara Y, Imataka K, Uchida A, et al. Development of a Japanese version of the SARC-F for diabetic patients: an examination of reliability and validity. Aging Clin Exp Res (2017) 29(5):935–42. doi: 10.1007/s40520-016-0668-5
40. Xu Z, Zhang P, Chen Y, Jiang J, Zhou Z, Zhu H. Comparing SARC-CalF with SARC-F for screening sarcopenia in adults with type 2 diabetes mellitus. Front Nutr (2022) 9:803924. doi: 10.3389/fnut.2022.803924
41. da Luz MCL, Pinho CPS, Bezerra GKA, da Conceição Chaves de Lemos M, da Silva Diniz A, Cabral PC. SARC-F and SARC-CalF in screening for sarcopenia in older adults with Parkinson's disease. Exp Gerontol (2021) 144:111183. doi: 10.1016/j.exger.2020.111183
42. Cederholm T, Jensen GL, Correia M, Gonzalez MC, Fukushima R, Higashiguchi T, et al. GLIM criteria for the diagnosis of malnutrition – A consensus report from the global clinical nutrition community. J Cachexia Sarcopenia Muscle (2019) 10(1):207–17. doi: 10.1002/jpen.1440
43. Papadopoulos E, Jin R, Monginot S, Alibhai SMH. Performance of the SARC-F in identifying low grip strength and physical performance in older adults with cancer. J Geriatr Oncol (2023) 14(2):101424. doi: 10.1016/j.jgo.2022.10142
44. Tosato M, Marzetti E, Cesari M, Savera G, Miller RR, Bernabei R, et al. Measurement of muscle mass in sarcopenia: from imaging to biochemical markers. Aging Clin Exp Res (2017) 29(1):19–27. doi: 10.1007/s40520-016-0717-0
45. Cesari M, Fielding RA, Pahor M, Goodpaster B, Hellerstein M, van Kan GA, et al. Biomarkers of sarcopenia in clinical trials-recommendations from the International Working Group on Sarcopenia. J Cachexia Sarcopenia Muscle (2012) 3(3):181–90. doi: 10.1007/s13539-012-0078-2
46. Ackermans LLGC, Rabou J, Basrai M, Schweinlin A, Bischoff SC, Cussenot O, et al. Screening, diagnosis and monitoring of sarcopenia: When to use which tool? Clin Nutr ESPEN (2022) 48:36–44. doi: 10.1016/j.clnesp.2022.01.027
47. Lee SY, Choo PL, Pang BWJ, Lau LK, Jabbar KA, Seah WT, et al. SPPB reference values and performance in assessing sarcopenia in community-dwelling Singaporeans - Yishun study. BMC Geriatr (2021) 21(1):213. doi: 10.1186/s12877-021-02147-4
48. Bergland A, Strand BH. Norwegian reference values for the Short Physical Performance Battery (SPPB): the Tromsø Study. BMC Geriatr (2019) 19(1):216. doi: 10.1186/s12877-019-1234-8
49. He P, Du G, Qin X, Li Z. Reduced energy metabolism contributing to aging of skeletal muscle by serum metabolomics and gut microbiota analysis. Life Sci (2023) 323:121619. doi: 10.1016/j.lfs.2023.121619
50. Lim J-Y, Frontera WR. Skeletal muscle aging and sarcopenia: Perspectives from mechanical studies of single permeabilized muscle fibers. J Biomechanics (2023) 152:111559. doi: 10.1016/j.jbiomech.2023.111559
51. Dos Santos MR, Storer TW. Testosterone treatment as a function-promoting therapy in sarcopenia associated with aging and chronic disease. Endocrinol Metab Clinics North America (2022) 51(1):187–204. doi: 10.1016/j.ecl.2021.11.012
52. Scicchitano BM, Pelosi L, Sica G, Musarò A. The physiopathologic role of oxidative stress in skeletal muscle. Mech Ageing Dev (2018) 170:37–44. doi: 10.1016/j.mad.2017.08.009
53. Sandiford SD, Kennedy KA, Xie X, Pickering JG, Li SS. Dual oxidase maturation factor 1 (DUOXA1) overexpression increases reactive oxygen species production and inhibits murine muscle satellite cell differentiation. Cell Commun Signal (2014) 12:5. doi: 10.1186/1478-811x-12-5
54. Sestili P, Barbieri E, Martinelli C, Battistelli M, Guescini M, Vallorani L, et al. Creatine supplementation prevents the inhibition of myogenic differentiation in oxidatively injured C2C12 murine myoblasts. Mol Nutr Food Res (2009) 53(9):1187–204. doi: 10.1002/mnfr.200800504
55. Vasilaki A, Jackson MJ. Role of reactive oxygen species in the defective regeneration seen in aging muscle. Free Radic Biol Med (2013) 65:317–23. doi: 10.1016/j.freeradbiomed.2013.07.008
56. Fealy CE, Grevendonk L, Hoeks J, Hesselink MKC. Skeletal muscle mitochondrial network dynamics in metabolic disorders and aging. Trends Mol Med (2021) 27(11):1033–44. doi: 10.1016/j.molmed.2021.07.013
57. Gouspillou G, Bourdel-Marchasson I, Rouland R, Calmettes G, Biran M, Deschodt-Arsac V, et al. Mitochondrial energetics is impaired in vivo in aged skeletal muscle. Aging Cell (2014) 13(1):39–48. doi: 10.1111/acel.12147
58. Gonzalez-Freire M, Scalzo P, D'Agostino J, Moore ZA, Diaz-Ruiz A, Fabbri E, et al. Skeletal muscle ex vivo mitochondrial respiration parallels decline in vivo oxidative capacity, cardiorespiratory fitness, and muscle strength: The Baltimore Longitudinal Study of Aging. Aging Cell (2018) 17(2):e12725. doi: 10.1111/acel.12725
59. Sousa-Victor P, García-Prat L, Muñoz-Cánoves P. Control of satellite cell function in muscle regeneration and its disruption in ageing. Nat Rev Mol Cell Biol (2022) 23(3):204–26. doi: 10.1038/s41580-021-00421-2
60. Day K, Shefer G, Shearer A, Yablonka-Reuveni Z. The depletion of skeletal muscle satellite cells with age is concomitant with reduced capacity of single progenitors to produce reserve progeny. Dev Biol (2010) 340(2):330–43. doi: 10.1016/j.ydbio.2010.01.006
61. Verdijk LB, Dirks ML, Snijders T, Prompers JJ, Beelen M, Jonkers RA, et al. Reduced satellite cell numbers with spinal cord injury and aging in humans. Med Sci Sports Exerc (2012) 44(12):2322–30. doi: 10.1249/MSS.0b013e3182667c2e
62. Dalle S, Rossmeislova L, Koppo K. The role of inflammation in age-related sarcopenia. Front Physiol (2017) 8:1045. doi: 10.3389/fphys.2017.01045
63. Ferrucci L, Penninx BW, Volpato S, Harris TB, Bandeen-Roche K, Balfour J, et al. Change in muscle strength explains accelerated decline of physical function in older women with high interleukin-6 serum levels. J Am Geriatr Soc (2002) 50(12):1947–54. doi: 10.1046/j.1532-5415.2002.50605.x
64. Cesari M, Penninx BW, Pahor M, Lauretani F, Corsi AM, Rhys Williams G, et al. Inflammatory markers and physical performance in older persons: the InCHIANTI study. J Gerontol A Biol Sci Med Sci (2004) 59(3):242–8. doi: 10.1093/gerona/59.3.m242
65. Haren MT, Malmstrom TK, Miller DK, Patrick P, Perry HM 3rd, Herning MM, et al. Higher C-reactive protein and soluble tumor necrosis factor receptor levels are associated with poor physical function and disability: a cross-sectional analysis of a cohort of late middle-aged African Americans. J Gerontol A Biol Sci Med Sci (2010) 65(3):274–81. doi: 10.1093/gerona/glp148
66. Bautmans I, Onyema O, Van Puyvelde K, Pleck S, Mets T. Grip work estimation during sustained maximal contraction: validity and relationship with dependency and inflammation in elderly persons. J Nutr Health Aging (2011) 15(8):731–6. doi: 10.1007/s12603-010-0317-1
67. Alemán H, Esparza J, Ramirez FA, Astiazaran H, Payette H. Longitudinal evidence on the association between interleukin-6 and C-reactive protein with the loss of total appendicular skeletal muscle in free-living older men and women. Age Ageing (2011) 40(4):469–75. doi: 10.1093/ageing/afr040
68. Giallauria F, Cittadini A, Smart NA, Vigorito C. Resistance training and sarcopenia. Monaldi Arch Chest Dis (2016) 84(1-2):738. doi: 10.4081/monaldi.2015.738
69. Ibata N, Terentjev EM. Why exercise builds muscles: titin mechanosensing controls skeletal muscle growth under load. Biophys J (2021) 120(17):3649–63. doi: 10.1016/j.bpj.2021.07.023
70. Kalinkovich A, Livshits G. Sarcopenic obesity or obese sarcopenia: A cross talk between age-associated adipose tissue and skeletal muscle inflammation as a main mechanism of the pathogenesis. Ageing Res Rev (2017) 35:200–21. doi: 10.1016/j.arr.2016.09.008
71. de Souza LF, Danielewicz AL, Rech CR, d'Orsi E, Mendonça VA, Lacerda ACR, et al. How much time in sedentary behavior is associated with probable sarcopenia in older adults? Geriatric Nurs (2022) 48:127–31. doi: 10.1016/j.gerinurse.2022.09.007
72. Nagai K, Matsuzawa R, Wada Y, Tsuji S, Itoh M, Sano K, et al. Impact of isotemporal substitution of sedentary time with physical activity on sarcopenia in older Japanese adults. J Am Med Directors Assoc (2021) 22(4):876–8. doi: 10.1016/j.jamda.2021.02.007
73. Wahlen BM, Mekkodathil A, Al-Thani H, El-Menyar A. Impact of sarcopenia in trauma and surgical patient population: A literature review. Asian J Surg (2020) 43(6):647–53. doi: 10.1016/j.asjsur.2019.10.010
74. Stefanaki C, Pervanidou P, Boschiero D, Chrousos GP. Chronic stress and body composition disorders: implications for health and disease. Hormones (Athens) (2018) 17(1):33–43. doi: 10.1007/s42000-018-0023-7
75. Li X, He J, Sun Q. Sleep duration and sarcopenia: an updated systematic review and meta-analysis. J Am Med Directors Assoc (2023) 24(8):1193–206.e5. doi: 10.1016/j.jamda.2023.04.032
76. Gallagher EJ, Leroith D, Karnieli E. Insulin resistance in obesity as the underlying cause for the metabolic syndrome. Mt Sinai J Med (2010) 77(5):511–23. doi: 10.1002/msj.20212
77. Johnson AM, Olefsky JM. The origins and drivers of insulin resistance. Cell (2013) 152(4):673–84. doi: 10.1016/j.cell.2013.01.041
78. DeFronzo RA, Tripathy D. Skeletal muscle insulin resistance is the primary defect in type 2 diabetes. Diabetes Care (2009) 32 Suppl 2(Suppl 2):S157–63. doi: 10.2337/dc09-S302
79. Abdulla H, Smith K, Atherton PJ, Idris I. Role of insulin in the regulation of human skeletal muscle protein synthesis and breakdown: a systematic review and meta-analysis. Diabetologia (2016) 59(1):44–55. doi: 10.1007/s00125-015-3751-0
80. Guillet C, Boirie Y. Insulin resistance: a contributing factor to age-related muscle mass loss? Diabetes Metab (2005) 31:5S20–5S6. doi: 10.1016/S1262-3636(05)73648-X
81. Gareev I, Beylerli O, Aliev G, Pavlov V, Izmailov A, Zhang Y, et al. The role of long non-coding RNAs in intracranial aneurysms and subarachnoid hemorrhage. Life (Basel) (2020) 10(9):155. doi: 10.3390/life10090155
82. Lee CG, Boyko EJ, Strotmeyer ES, Lewis CE, Cawthon PM, Hoffman AR, et al. Association between insulin resistance and lean mass loss and fat mass gain in older men without diabetes mellitus. J Am Geriatr Soc (2011) 59(7):1217–24. doi: 10.1111/j.1532-5415.2011.03472.x
83. Reaven GM. The insulin resistance syndrome: definition and dietary approaches to treatment. Annu Rev Nutr (2005) 25:391–406. doi: 10.1146/annurev.nutr.24.012003.132155
84. Beilerli A, Kudriashov V, Sufianov A, Kostin A, Begliarzade S, Ilyasova T, et al. Regulation and mechanism of action of miRNAs on insulin resistance in skeletal muscles. Non-coding RNA Res (2023) 8(2):218–23. doi: 10.1016/j.ncrna.2023.02.005
85. Umegaki H. Sarcopenia and diabetes: Hyperglycemia is a risk factor for age-associated muscle mass and functional reduction. J Diabetes Investig (2015) 6(6):623–4. doi: 10.1111/jdi.12365
86. Lee H, Kim SY, Lim Y. Lespedeza bicolor extract supplementation reduced hyperglycemia-induced skeletal muscle damage by regulation of AMPK/SIRT/PGC1α–related energy metabolism in type 2 diabetic mice. Nutr Res (2023) 110:1–13. doi: 10.1016/j.nutres.2022.12.007
87. Meng SJ, Yu LJ. Oxidative stress, molecular inflammation and sarcopenia. Int J Mol Sci (2010) 11(4):1509–26. doi: 10.3390/ijms11041509
88. Mori H, Kuroda A, Ishizu M, Ohishi M, Takashi Y, Otsuka Y, et al. Association of accumulated advanced glycation end-products with a high prevalence of sarcopenia and dynapenia in patients with type 2 diabetes. J Diabetes Investig (2019) 10(5):1332–40. doi: 10.1111/jdi.13014
89. Rato L, Duarte AI, Tomás GD, Santos MS, Moreira PI, Socorro S, et al. Pre-diabetes alters testicular PGC1-α/SIRT3 axis modulating mitochondrial bioenergetics and oxidative stress. Biochim Biophys Acta (2014) 1837(3):335–44. doi: 10.1016/j.bbabio.2013.12.00
90. Li W, Liang X, Zeng Z, Yu K, Zhan S, Su Q, et al. Simvastatin inhibits glucose uptake activity and GLUT4 translocation through suppression of the IR/IRS-1/Akt signaling in C2C12 myotubes. BioMed Pharmacother (2016) 83:194–200. doi: 10.1016/j.biopha.2016.06.029
91. Yoo JI, Ha YC, Choi H, Kim KH, Lee YK, Koo KH, et al. Malnutrition and chronic inflammation as risk factors for sarcopenia in elderly patients with hip fracture. Asia Pac J Clin Nutr (2018) 27(3):527–32. doi: 10.6133/apjcn.082017.02
92. Brioche T, Lemoine-Morel S. Oxidative stress, sarcopenia, antioxidant strategies and exercise: molecular aspects. Curr Pharm Des (2016) 22(18):2664–78. doi: 10.2174/1381612822666160219120531
93. Kozakowska M, Pietraszek-Gremplewicz K, Jozkowicz A, Dulak J. The role of oxidative stress in skeletal muscle injury and regeneration: focus on antioxidant enzymes. J Muscle Res Cell Motil (2015) 36(6):377–93. doi: 10.1007/s10974-015-9438-9
94. Gabay C, Kushner I. Acute-phase proteins and other systemic responses to inflammation. N Engl J Med (1999) 340(6):448–54. doi: 10.1056/nejm199902113400607
95. Suzuki K. Chronic inflammation as an immunological abnormality and effectiveness of exercise. Biomolecules (2019) 9(6):223. doi: 10.3390/biom9060223
96. Donini LM, Busetto L, Bischoff SC, Cederholm T, Ballesteros-Pomar MD, Batsis JA, et al. Definition and diagnostic criteria for sarcopenic obesity: ESPEN and EASO consensus statement. Obes Facts (2022) 15(3):321–35. doi: 10.1159/000521241
97. Gao Q, Mei F, Shang Y, Hu K, Chen F, Zhao L, et al. Global prevalence of sarcopenic obesity in older adults: A systematic review and meta-analysis. Clin Nutr (2021) 40(7):4633–41. doi: 10.1016/j.clnu.2021.06.009
98. Gandham A, Mesinovic J, Jansons P, Zengin A, Bonham MP, Ebeling PR, et al. Falls, fractures, and areal bone mineral density in older adults with sarcopenic obesity: A systematic review and meta-analysis. Obes Rev (2021) 22(5):e13187. doi: 10.1111/obr.13187
99. Khan RMM, Chua ZJY, Tan JC, Yang Y, Liao Z, Zhao Y. From pre-diabetes to diabetes: diagnosis, treatments and translational research. Medicina (Kaunas) (2019) 55(9):546. doi: 10.3390/medicina55090546
100. Zhou Y-y, Wang J-f, Yao Q, Jian Q-f, Luo Z-p. Prevalence of sarcopenic obesity in patients with diabetes and adverse outcomes: A systematic review and meta-analysis. Clin Nutr ESPEN (2023) 58:128–35. doi: 10.1016/j.clnesp.2023.09.920
101. Xu J, Li X, Chen W, Zhang Z, Zhou Y, Gou Y, et al. Myofiber Baf60c controls muscle regeneration by modulating Dkk3-mediated paracrine signaling. J Exp Med (2023) 220(9):e20221123. doi: 10.1084/jem.20221123
102. Basiri R, Spicer MT, Ledermann T, Arjmandi BH. Effects of nutrition intervention on blood glucose, body composition, and phase angle in obese and overweight patients with diabetic foot ulcers. Nutrients (2022) 14(17):3564. doi: 10.3390/nu14173564
103. Sadiya A, Ahmed SM, Carlsson M, Tesfa Y, George M, Ali SH, et al. Vitamin D3 supplementation and body composition in persons with obesity and type 2 diabetes in the UAE: A randomized controlled double-blinded clinical trial. Clin Nutr (2016) 35(1):77–82. doi: 10.1016/j.clnu.2015.02.017
104. Yoshimoto Y, Oishi Y. Mechanisms of skeletal muscle-tendon development and regeneration/healing as potential therapeutic targets. Pharmacol Ther (2023) 243:108357. doi: 10.1016/j.pharmthera.2023.108357
105. Wu J, Egusa A, Nishimura T. Carnosine synthase deficiency in mice affects protein metabolism in skeletal muscle. Biochem Biophys Res Commun (2022) 612:22–9. doi: 10.1016/j.bbrc.2022.04.075
106. Cruz-Jentoft AJ, Kiesswetter E, Drey M, Sieber CC. Nutrition, frailty, and sarcopenia. Aging Clin Exp Res (2017) 29(1):43–8. doi: 10.1007/s40520-016-0709-0
107. Matsunaga T, Furuya T, Deto T, Masuda H, Nakanishi K. Two older hematological Malignancy patients for whom nutrition rehabilitation was effective against global leadership initiative on malnutrition-defined malnutrition and sarcopenia. Cureus (2023) 15(8):e43069. doi: 10.7759/cureus.43069
108. Chan L-C, Yang Y-C, Lin H-C, Wahlqvist ML, Hung Y-J, Lee M-S. Nutrition counseling is associated with less sarcopenia in diabetes: A cross-sectional and retrospective cohort study. Nutrition (2021) 91-92:111269. doi: 10.1016/j.nut.2021.111269
109. Hwang J, Zmuda JM, Kuipers AL, Bunker CH, Santanasto AJ, Wheeler VW, et al. Serum vitamin D and age-related muscle loss in Afro-Caribbean men: the importance of age and diabetic status. J Frailty Aging (2019) 8(3):131–7. doi: 10.14283/jfa.2018.40
110. Henson J, Arsenyadis F, Redman E, Brady EM, Coull NA, Edwardson CL, et al. Relative protein intake and associations with markers of physical function in those with type 2 diabetes. Diabetes Med (2022) 39(8):e14851. doi: 10.1111/dme.14851
111. Fanelli SM, Kelly OJ, Krok-Schoen JL, Taylor CA. Low protein intakes and poor diet quality associate with functional limitations in US adults with diabetes: A 2005-2016 NHANES analysis. Nutrients (2021) 13(8):2582. doi: 10.3390/nu13082582
112. Buchanan A, Villani A. Association of adherence to a Mediterranean diet with excess body mass, muscle strength and physical performance in overweight or obese adults with or without type 2 diabetes: two cross-sectional studies. Healthcare (Basel) (2021) 9(10):1255. doi: 10.3390/healthcare9101255
113. Okamura T, Miki A, Hashimoto Y, Kaji A, Sakai R, Osaka T, et al. Shortage of energy intake rather than protein intake is associated with sarcopenia in elderly patients with type 2 diabetes: A cross-sectional study of the KAMOGAWA-DM cohort. J Diabetes (2019) 11(6):477–83. doi: 10.1111/1753-0407.12874
114. Takahashi F, Hashimoto Y, Kaji A, Sakai R, Kawate Y, Okamura T, et al. Vitamin intake and loss of muscle mass in older people with type 2 diabetes: A prospective study of the KAMOGAWA-DM cohort. Nutrients (2021) 13(7):2335. doi: 10.3390/nu13072335
115. Okamura T, Hashimoto Y, Miki A, Kaji A, Sakai R, Iwai K, et al. Reduced dietary omega-3 fatty acids intake is associated with sarcopenia in elderly patients with type 2 diabetes: a cross-sectional study of KAMOGAWA-DM cohort study. J Clin Biochem Nutr (2020) 66(3):233–7. doi: 10.3164/jcbn.19-85
116. Casals-Vázquez C, Suárez-Cadenas E, Estébanez Carvajal FM, Aguilar Trujillo MP, Jiménez Arcos MM, Vázquez Sáchez M. [Relationship between quality of life, physical activity, nutrition, glycemic control and sarcopenia in older adults with type 2 diabetes mellitus]. Nutr Hosp (2017) 34(5):1198–204. doi: 10.20960/nh.1070
117. Izquierdo M, Laosa O, Cadore EL, Abizanda P, Garcia-Garcia FJ, Hornillos M, et al. Two-year follow-up of a multimodal intervention on functional capacity and muscle power in frail patients with type 2 diabetes. J Am Med Dir Assoc (2021) 22(9):1906–11. doi: 10.1016/j.jamda.2021.06.022
118. Low S, Wang J, Moh A, Ang SF, Ang K, Shao YM, et al. Amino acid profile of skeletal muscle loss in type 2 diabetes: Results from a 7-year longitudinal study in asians. Diabetes Res Clin Pract (2022) 186:109803. doi: 10.1016/j.diabres.2022.109803
119. da Silva Soares DB, Shinjo SK, Santos AS, de Cassia Rosa de Jesus J, Schenk S, de Castro GS, et al. Skeletal muscle gene expression in older adults with type 2 diabetes mellitus undergoing calorie-restricted diet and recreational sports training - a randomized clinical trial. Exp Gerontology (2022) 164:111831. doi: 10.1016/j.exger.2022.111831
120. Martínez-Arnau FM, Fonfría-Vivas R, Buigues C, Castillo Y, Molina P, Hoogland AJ, et al. Effects of leucine administration in sarcopenia: A randomized and placebo-controlled clinical trial. Nutrients (2020) 12(4):932. doi: 10.3390/nu12040932
121. Lee HX, Yeo A, Tan CN, Yew S, Tay L, Ding YY, et al. Combined impact of positive screen for sarcopenia and frailty on physical function, cognition and nutrition in the community dwelling older adult. Ann Geriatr Med Res (2021) 25(3):210–6. doi: 10.4235/agmr.21.0068
122. Millward DJ, Layman DK, Tomé D, Schaafsma G. Protein quality assessment: impact of expanding understanding of protein and amino acid needs for optimal health. Am J Clin Nutr (2008) 87(5):1576s–81s. doi: 10.1093/ajcn/87.5.1576S
123. Wu Y, de Crom TO, Schaft NVD, Chen Z, Rivadeneira F, Boer CG, et al. Dietary protein intake with body composition, hand grip strength, and sarcopenia in older adults: A population-based study. Clin Nutr ESPEN (2023) 54:476. doi: 10.1016/j.clnesp.2022.09.062
124. Fuchs CJ, Hermans WJH, Holwerda AM, Smeets JSJ, Senden JM, van Kranenburg J, et al. Branched-chain amino acid and branched-chain ketoacid ingestion increases muscle protein synthesis rates in vivo in older adults: a double-blind, randomized trial. Am J Clin Nutr (2019) 110(4):862–72. doi: 10.1093/ajcn/nqz120
125. Paddon-Jones D, Sheffield-Moore M, Katsanos CS, Zhang XJ, Wolfe RR. Differential stimulation of muscle protein synthesis in elderly humans following isocaloric ingestion of amino acids or whey protein. Exp Gerontol (2006) 41(2):215–9. doi: 10.1016/j.exger.2005.10.006
126. Hou Y, Yoon Y, Oh E, Sung B, Kim Y. Effects of soy protein hydrolysates on antioxidant activity and inhibition of muscle loss. Int Food Res J (2022) 29(6):1458–67. doi: 10.47836/ifrj.29.6.22
127. Lewgood J, Oliveira B, Korzepa M, Forbes SC, Little JP, Breen L, et al. Efficacy of dietary and supplementation interventions for individuals with type 2 diabetes. Nutrients (2021) 13(7):2378. doi: 10.3390/nu13072378
128. Deutz NE, Bauer JM, Barazzoni R, Biolo G, Boirie Y, Bosy-Westphal A, et al. Protein intake and exercise for optimal muscle function with aging: recommendations from the ESPEN Expert Group. Clin Nutr (2014) 33(6):929–36. doi: 10.1016/j.clnu.2014.04.007
129. Bauer J, Biolo G, Cederholm T, Cesari M, Cruz-Jentoft AJ, Morley JE, et al. Evidence-based recommendations for optimal dietary protein intake in older people: a position paper from the PROT-AGE Study Group. J Am Med Dir Assoc (2013) 14(8):542–59. doi: 10.1016/j.jamda.2013.05.021
130. Coletta G, Phillips SM. An elusive consensus definition of sarcopenia impedes research and clinical treatment: A narrative review. Ageing Res Rev (2023) 86:101883. doi: 10.1016/j.arr.2023.101883
131. Pennings B, Boirie Y, Senden JM, Gijsen AP, Kuipers H, van Loon LJ. Whey protein stimulates postprandial muscle protein accretion more effectively than do casein and casein hydrolysate in older men. Am J Clin Nutr (2011) 93(5):997–1005. doi: 10.3945/ajcn.110.008102
132. Rennie MJ, Bohé J, Wolfe RR. Latency, duration and dose response relationships of amino acid effects on human muscle protein synthesis. J Nutr (2002) 132(10):3225s–7s. doi: 10.1093/jn/131.10.3225S
133. Koopman R, Wagenmakers AJ, Manders RJ, Zorenc AH, Senden JM, Gorselink M, et al. Combined ingestion of protein and free leucine with carbohydrate increases postexercise muscle protein synthesis in vivo in male subjects. Am J Physiol Endocrinol Metab (2005) 288(4):E645–53. doi: 10.1152/ajpendo.00413.2004
134. Dardevet D, Sornet C, Bayle G, Prugnaud J, Pouyet C, Grizard J. Postprandial stimulation of muscle protein synthesis in old rats can be restored by a leucine-supplemented meal. J Nutr (2002) 132(1):95–100. doi: 10.1093/jn/132.1.95
135. Frexes-Steed M, Lacy DB, Collins J, Abumrad NN. Role of leucine and other amino acids in regulating protein metabolism in vivo. Am J Physiol (1992) 262(6 Pt 1):E925–35. doi: 10.1152/ajpendo.1992.262.6.E925
136. Kobayashi H. [Amino acid nutrition in the prevention and treatment of sarcopenia]. Yakugaku Zasshi (2018) 138(10):1277–83. doi: 10.1248/yakushi.18-00091-4
137. Rieu I, Balage M, Sornet C, Giraudet C, Pujos E, Grizard J, et al. Leucine supplementation improves muscle protein synthesis in elderly men independently of hyperaminoacidaemia. J Physiol (2006) 575(Pt 1):305–15. doi: 10.1113/jphysiol.2006.110742
138. van Loon LJ. Leucine as a pharmaconutrient in health and disease. Curr Opin Clin Nutr Metab Care (2012) 15(1):71–7. doi: 10.1097/MCO.0b013e32834d617a
139. Yang C, Dai Y, Li Z, Peng Y, Zhang L, Jia H. Relationship of serum 25-hydroxyvitamin D levels with sarcopenia and body composition in community-dwelling older adults: A paired case-control study. J Am Med Dir Assoc (2023) 24(8):1213–9. doi: 10.1016/j.jamda.2023.06.004
140. Gilsanz V, Kremer A, Mo AO, Wren TA, Kremer R. Vitamin D status and its relation to muscle mass and muscle fat in young women. J Clin Endocrinol Metab (2010) 95(4):1595–601. doi: 10.1210/jc.2009-2309
141. Visser M, Deeg DJ, Lips P. Low vitamin D and high parathyroid hormone levels as determinants of loss of muscle strength and muscle mass (sarcopenia): the Longitudinal Aging Study Amsterdam. J Clin Endocrinol Metab (2003) 88(12):5766–72. doi: 10.1210/jc.2003-030604
142. Bhattacharya S, Bhadra R, Schols AMWJ, van Helvoort A, Sambashivaiah S. Nutrition in the prevention and management of sarcopenia - A special focus on Asian Indians. Osteoporosis Sarcopenia (2022) 8(4):135–44. doi: 10.1016/j.afos.2022.12.002
143. Seo MH, Kim MK, Park SE, Rhee EJ, Park CY, Lee WY, et al. The association between daily calcium intake and sarcopenia in older, non-obese Korean adults: the fourth Korea National Health and Nutrition Examination Survey (KNHANES IV) 2009. Endocr J (2013) 60(5):679–86. doi: 10.1507/endocrj.ej12-0395
144. Bischoff HA, Stähelin HB, Dick W, Akos R, Knecht M, Salis C, et al. Effects of vitamin D and calcium supplementation on falls: a randomized controlled trial. J Bone Miner Res (2003) 18(2):343–51. doi: 10.1359/jbmr.2003.18.2.343
145. Robinson SM, Jameson KA, Batelaan SF, Martin HJ, Syddall HE, Dennison EM, et al. Diet and its relationship with grip strength in community-dwelling older men and women: the Hertfordshire cohort study. J Am Geriatr Soc (2008) 56(1):84–90. doi: 10.1111/j.1532-5415.2007.01478.x
146. Dos Reis AS, Limirio LS, Santos HO, de Oliveira EP. Intake of polyunsaturated fatty acids and ω-3 are protective factors for sarcopenia in kidney transplant patients. Nutrition (2021) 81:110929. doi: 10.1016/j.nut.2020.110929
147. Bird JK, Troesch B, Warnke I, Calder PC. The effect of long chain omega-3 polyunsaturated fatty acids on muscle mass and function in sarcopenia: A scoping systematic review and meta-analysis. Clin Nutr ESPEN (2021) 46:73–86. doi: 10.1016/j.clnesp.2021.10.011
148. Haghiac M, Yang XH, Presley L, Smith S, Dettelback S, Minium J, et al. Dietary omega-3 fatty acid supplementation reduces inflammation in obese pregnant women: A randomized double-blind controlled clinical trial. PLoS One (2015) 10(9):e0137309. doi: 10.1371/journal.pone.0137309
149. Pan D, Yang L, Yang X, Xu D, Wang S, Gao H, et al. Potential nutritional strategies to prevent and reverse sarcopenia in aging process: Role of fish oil-derived ω-3 polyunsaturated fatty acids, wheat oligopeptide and their combined intervention. J Advanced Res (2023) S2090-1232(23)00111-X. doi: 10.1016/j.jare.2023.04.005
150. Zhao D, Shi W, Bi L, Qi Y, Hu S, Li C, et al. Effect of short-term acute moderate-intensity resistance exercise on blood glucose in older patients with type 2 diabetes mellitus and sarcopenia. Geriatr Gerontol Int (2022) 22(8):653–9. doi: 10.1111/ggi.14437
151. Chien YH, Tsai CJ, Wang DC, Chuang PH, Lin HT. Effects of 12-week progressive sandbag exercise training on glycemic control and muscle strength in patients with type 2 diabetes mellitus combined with possible sarcopenia. Int J Environ Res Public Health (2022) 19(22):228. doi: 10.3390/ijerph192215009
152. Buch A, Eldor R, Kis O, Keinan-Boker L, Dunsky A, Rubin A, et al. The effect of circuit resistance training, empagliflozin or "vegeterranean diet" on physical and metabolic function in older subjects with type 2 diabetes: a study protocol for a randomized control trial (CEV-65 trial). BMC Geriatr (2019) 19(1):228. doi: 10.1186/s12877-019-1219-7
153. Pfeifer LO, Botton CE, Diefenthaeler F, Umpierre D, Pinto RS. Effects of a power training program in the functional capacity, on body balance and lower limb muscle strength of elderly with type 2 diabetes mellitus. J Sports Med Phys Fitness (2021) 61(11):1529–37. doi: 10.23736/s0022-4707.21.11880-8
154. Botton CE, Umpierre D, Rech A, Pfeifer LO, MaChado CLF, Teodoro JL, et al. Effects of resistance training on neuromuscular parameters in elderly with type 2 diabetes mellitus: A randomized clinical trial. Exp Gerontol (2018) 113:141–9. doi: 10.1016/j.exger.2018.10.001
155. Hsieh PL, Tseng CH, Tseng YJ, Yang WS. Resistance training improves muscle function and cardiometabolic risks but not quality of life in older people with type 2 diabetes mellitus: A randomized controlled trial. J Geriatr Phys Ther (2018) 41(2):65–76. doi: 10.1519/jpt.0000000000000107
156. Marcotte-Chénard A, Tremblay D, Mony MM, Brochu M, Dionne IJ, Langlois MF, et al. Low-volume walking HIIT: Efficient strategy to improve physical capacity and reduce the risk of cardiovascular disease in older women with type 2 diabetes. Diabetes Metab Syndr (2021) 15(5):102233. doi: 10.1016/j.dsx.2021.102233
157. Ferreira LF, Scariot EL, da Rosa LHT. The effect of different exercise programs on sarcopenia criteria in older people: A systematic review of systematic reviews with meta-analysis. Arch Gerontol Geriatr (2023) 105:104868. doi: 10.1016/j.archger.2022.104868
158. Park S. Association between polygenetic risk scores related to sarcopenia risk and their interactions with regular exercise in a large cohort of Korean adults. Clin Nutr (2021) 40(10):5355–64. doi: 10.1016/j.clnu.2021.09.003
159. Li L, He Y, Jin N, Li H, Liu X. Effects of protein supplementation and exercise on delaying sarcopenia in healthy older individuals in Asian and non-Asian countries: A systematic review and meta-analysis. Food Chemistry: X (2022) 13:100210. doi: 10.1016/j.fochx.2022.100210
160. Kim H, Suzuki T, Saito K, Yoshida H, Kojima N, Kim M, et al. Effects of exercise and tea catechins on muscle mass, strength and walking ability in community-dwelling elderly Japanese sarcopenic women: a randomized controlled trial. Geriatr Gerontol Int (2013) 13(2):458–65. doi: 10.1111/j.1447-0594.2012.00923.x
161. Scott D, Blizzard L, Fell J, Giles G, Jones G. Associations between dietary nutrient intake and muscle mass and strength in community-dwelling older adults: the Tasmanian Older Adult Cohort Study. J Am Geriatr Soc (2010) 58(11):2129–34. doi: 10.1111/j.1532-5415.2010.03147.x
162. Hashemi R, Motlagh AD, Heshmat R, Esmaillzadeh A, Payab M, Yousefinia M, et al. Diet and its relationship to sarcopenia in community dwelling Iranian elderly: a cross sectional study. Nutrition (2015) 31(1):97–104. doi: 10.1016/j.nut.2014.05.003
163. Bird SR, Hawley JA. Update on the effects of physical activity on insulin sensitivity in humans. BMJ Open Sport Exerc Med (2016) 2(1):e000143. doi: 10.1136/bmjsem-2016-000143
164. Codella R, Ialacqua M, Terruzzi I, Luzi L. May the force be with you: why resistance training is essential for subjects with type 2 diabetes mellitus without complications. Endocrine (2018) 62(1):14–25. doi: 10.1007/s12020-018-1603-7
165. Leenders M, Verdijk LB, van der Hoeven L, van Kranenburg J, Nilwik R, van Loon LJ. Elderly men and women benefit equally from prolonged resistance-type exercise training. J Gerontol A Biol Sci Med Sci (2013) 68(7):769–79. doi: 10.1093/gerona/gls241
166. Fragala MS, Fukuda DH, Stout JR, Townsend JR, Emerson NS, Boone CH, et al. Muscle quality index improves with resistance exercise training in older adults. Exp Gerontol (2014) 53:1–6. doi: 10.1016/j.exger.2014.01.027
167. McLeod JC, Stokes T, Phillips SM. Resistance exercise training as a primary countermeasure to age-related chronic disease. Front Physiol (2019) 10:645. doi: 10.3389/fphys.2019.00645
168. Liu Y, Ye W, Chen Q, Zhang Y, Kuo CH, Korivi M. Resistance exercise intensity is correlated with attenuation of hbA1c and insulin in patients with type 2 diabetes: A systematic review and meta-analysis. Int J Environ Res Public Health (2019) 16(1):140. doi: 10.3390/ijerph16010140
169. Yoshimura Y, Bise T, Shimazu S, Tanoue M, Tomioka Y, Araki M, et al. Effects of a leucine-enriched amino acid supplement on muscle mass, muscle strength, and physical function in post-stroke patients with sarcopenia: A randomized controlled trial. Nutrition (2019) 58:1–6. doi: 10.1016/j.nut.2018.05.028
170. Yoshimura Y, Wakabayashi H, Yamada M, Kim H, Harada A, Arai H. Interventions for treating sarcopenia: A systematic review and meta-analysis of randomized controlled studies. J Am Med Dir Assoc (2017) 18(6):553.e1–.e16. doi: 10.1016/j.jamda.2017.03.019
171. Hordern MD, Dunstan DW, Prins JB, Baker MK, Singh MA, Coombes JS. Exercise prescription for patients with type 2 diabetes and pre-diabetes: a position statement from Exercise and Sport Science Australia. J Sci Med Sport (2012) 15(1):25–31. doi: 10.1016/j.jsams.2011.04.005
172. Zhang X, Zhao Y, Chen S, Shao H. Anti-diabetic drugs and sarcopenia: emerging links, mechanistic insights, and clinical implications. J Cachexia Sarcopenia Muscle (2021) 12(6):1368–79. doi: 10.1002/jcsm.12838
173. Tricarico D, Mele A, Lundquist AL, Desai RR, George AL Jr., Conte Camerino D. Hybrid assemblies of ATP-sensitive K+ channels determine their muscle-type-dependent biophysical and pharmacological properties. Proc Natl Acad Sci U.S.A. (2006) 103(4):1118–23. doi: 10.1073/pnas.0505974103
174. Tricarico D, Mele A, Camerino GM, Bottinelli R, Brocca L, Frigeri A, et al. The KATP channel is a molecular sensor of atrophy in skeletal muscle. J Physiol (2010) 588(Pt 5):773–84. doi: 10.1113/jphysiol.2009.185835
175. Mele A, Buttiglione M, Cannone G, Vitiello F, Camerino DC, Tricarico D. Opening/blocking actions of pyruvate kinase antibodies on neuronal and muscular KATP channels. Pharmacol Res (2012) 66(5):401–8. doi: 10.1016/j.phrs.2012.07.007
176. Lee CG, Boyko EJ, Barrett-Connor E, Miljkovic I, Hoffman AR, Everson-Rose SA, et al. Insulin sensitizers may attenuate lean mass loss in older men with diabetes. Diabetes Care (2011) 34(11):2381–6. doi: 10.2337/dc11-1032
177. Sugimoto K, Ikegami H, Takata Y, Katsuya T, Fukuda M, Akasaka H, et al. Glycemic control and insulin improve muscle mass and gait speed in type 2 diabetes: the MUSCLES-DM study. J Am Med Directors Assoc (2021) 22(4):834–8.e1. doi: 10.1016/j.jamda.2020.11.003
178. Bouchi R, Fukuda T, Takeuchi T, Nakano Y, Murakami M, Minami I, et al. Insulin treatment attenuates decline of muscle mass in Japanese patients with type 2 diabetes. Calcif Tissue Int (2017) 101(1):1–8. doi: 10.1007/s00223-017-0251-x
179. Ferrari U, Then C, Rottenkolber M, Selte C, Seissler J, Conzade R, et al. Longitudinal association of type 2 diabetes and insulin therapy with muscle parameters in the KORA-Age study. Acta Diabetol (2020) 57(9):1057–63. doi: 10.1007/s00592-020-01523-7
Keywords: sarcopenia, type 2 diabetes, elderly, pathogenesis, treatment
Citation: Hou Y, Xiang J, Wang B, Duan S, Song R, Zhou W, Tan S and He B (2024) Pathogenesis and comprehensive treatment strategies of sarcopenia in elderly patients with type 2 diabetes mellitus. Front. Endocrinol. 14:1263650. doi: 10.3389/fendo.2023.1263650
Received: 20 July 2023; Accepted: 19 December 2023;
Published: 08 January 2024.
Edited by:
Chunjie Jiang, University of Texas MD Anderson Cancer Center, United StatesReviewed by:
Jingyuan Dai, The Ohio State University, United StatesJin Zhang, University of Mississippi Medical Center, United States
Owen Kelly, Sam Houston State University, United States
Copyright © 2024 Hou, Xiang, Wang, Duan, Song, Zhou, Tan and He. This is an open-access article distributed under the terms of the Creative Commons Attribution License (CC BY). The use, distribution or reproduction in other forums is permitted, provided the original author(s) and the copyright owner(s) are credited and that the original publication in this journal is cited, in accordance with accepted academic practice. No use, distribution or reproduction is permitted which does not comply with these terms.
*Correspondence: Wenhu Zhou, emhvdXdlbmh1eWFvamlAMTYzLmNvbQ==; Songwen Tan, c29uZ3dlbi50YW5AY3N1LmVkdS5jbg==; Binsheng He, aG5haW9zQDE2My5jb20=