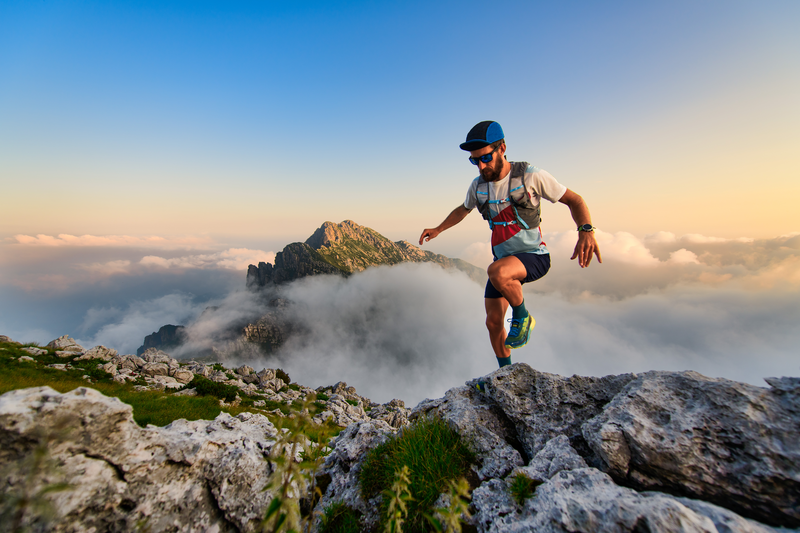
95% of researchers rate our articles as excellent or good
Learn more about the work of our research integrity team to safeguard the quality of each article we publish.
Find out more
MINI REVIEW article
Front. Endocrinol. , 13 September 2023
Sec. Obesity
Volume 14 - 2023 | https://doi.org/10.3389/fendo.2023.1256514
This article is part of the Research Topic Recent Advances in Pediatric Craniopharyngioma View all 5 articles
Hypothalamic obesity (HO) is a complex and rare disorder affecting multiple regulatory pathways of energy intake and expenditure in the brain as well as the regulation of the autonomic nervous system and peripheral hormonal signaling. It can be related to monogenic obesity syndromes which often affect the central leptin-melanocortin pathways or due to injury of the hypothalamus from pituitary and hypothalamic tumors, such as craniopharyngioma, surgery, trauma, or radiation to the hypothalamus. Traditional treatments of obesity, such as lifestyle intervention and specific diets, are still a therapeutic cornerstone, but often fail to result in meaningful and sustained reduction of body mass index. This review will give an update on pharmacotherapies of HO related to hypothalamic injury. Recent obesity drug developments are promising for successful obesity intervention outcomes.
Hypothalamic obesity (HO) is one of the most recalcitrant examples of excessive weight gain and most commonly caused by hypothalamic lesions and tumors such as craniopharyngioma (CP), an embryological tumor located in the hypothalamic and/or pituitary region, and its treatment by surgery and irradiation (1–4). Additional etiologies include other suprasellar brain tumors, trauma, inflammation, and some genetic syndromes (2, 4, 5). CP frequently causes not only hypopituitarism, but also damages the medial hypothalamic nuclei. After surgery, hyperphagia and severe obesity, a major risk factor for craniopharyngioma-related morbidity and mortality, occurs in 50% of survivors even with optimal endocrine management of hypopituitarism (6). Mechanisms leading to the profoundly disturbed energy homeostasis are complex and not well elucidated. Early and effective management of obesity is vital for this population (7), which is more resistant to treatment than alimentary obesity (8–16). There are currently no approved drugs for treatments of HO (17), but drug interventions that tackle both reduction of hyperphagia and stimulation of thermogenesis are promising.
Patients with CP frequently develop obesity following tumor therapy with surgery and/or radiation, and have more features of metabolic syndrome compared to matched controls (18, 19). Furthermore, people with CP have a 3-19-fold higher cardiovascular mortality (20, 21), as well as increased rates of cerebral infarction and type 2 diabetes mellitus compared to the general population (22). In affected patients, both quality of life and survival are substantially decreased due to these metabolic consequences (6, 23). For example, the estimated prevalence of nonalcoholic fatty liver disease in survivors of childhood craniopharyngioma is 47% (24). HO can also occur due to other suprasellar tumors, radiation, trauma, or surgical insult to the hypothalamus. The excessive weight gain following hypothalamic injury occurs irrespective of pituitary deficiency secondary to damage to the hypothalamic-pituitary axis and optimal replacement of hormones. Many, though not all, individuals with HO also experience hyperphagia (25, 26). Recognized risk factors for severe obesity include large hypothalamic tumors or aggressive resections affecting several medial and posterior hypothalamic nuclei reaching the floor of the third ventricle, the area beyond mammillary bodies, and several satiety signaling pathways (10, 27–29). Affected medial hypothalamic nuclei frequently are arcuate (ARC), ventromedial (VMN) and dorsomedial (DMN) nuclei (30). Structural damage of these nuclei often leads to hyperphagia, rapid post-operative weight gain, central insulin and leptin resistance, decreased sympathetic activity, low metabolic rate, and increased energy storage in adipose tissue (11, 30). In particular, VMN damage can lead to disinhibition of vagal tone, resulting in excess stimulation of pancreatic β-cells, hyperinsulinemia, and obesity. Several previous studies showed that the secretion of satiety regulating peptides, such as ghrelin and peptide YY (PYY), may also be altered in CP patients (31, 32).
Clinical features of the full HO syndrome include excessive weight gain leading to morbid obesity with uncontrolled appetite, potentially caused by central leptin resistance and deficient downstream pathways, fatigue, decreased sympathetic activity, low energy expenditure, temperature dysregulation, and increased energy storage in adipose tissue (1–4, 13, 25, 26, 33–37). Similar clinical features are also observed in patients suffering from HO syndrome due to a genetic abnormality (i.e. melanocortin-4 receptor defect, leptin or proopiomelanocortin (POMC) deficiency, Bardet-Biedl Syndrome).
While some patients report hyperphagia, other patients have lower energy intake compared to relevant controls (25, 26). This reduction in energy intake is thought to be offset by greater relative decrease in basal metabolic rate and physical activity that leads to excessive weight gain in HO (26). Alpha-melanocyte stimulating hormone (α-MSH) is one of the key weight-regulating neuropeptides after binding to melanocortin receptor subtypes 3 and 4 in the brain; it is mainly produced in the pituitary and hypothalamus from the cleavage of POMC (38, 39). Individuals with hypothalamic lesions related to craniopharyngioma appear to have significantly decreased serum levels of α-MSH (40, 41), suggesting a melanocortin pathway deficiency. Compared to controls, individuals with HO have significantly higher baseline and post-meal circulating insulin concentrations, potentially indicating insulin hypersecretion through vagus nerve hyperstimulation (11, 31, 32). In HO, biochemically, the degree of hyperleptinemia and hyperinsulinemia are unexpectedly high for the degree of obesity, and catecholamine levels are low, suggestive of decreased sympathetic tone (26, 30, 32, 42–44). Chronic hyperleptinemia, decreased sympathetic tone and deficiency of downstream outputs of leptin signaling are key features contributing to the pathogenesis of HO. Furthermore, disturbed cicadian rhythm and narcolepsy could contribute to decreased energy expenditure (45).
In 2003, the only FDA approved weight loss medication for adolescents was orlistat, a pancreatic lipase inhibitor, but it was rarely used due to its low efficacy (46) and unfavorable gastrointestinal side effect profile (46, 47). Several central stimulants have been used off label for the treatment of HO such as methylphenidate, phentermine, dextroamphetamine, mazindol, caffeine and ephedrine (8, 48–51), but data on these drugs came from small studies with mixed results. Other medications such as sibutramine, a norepinephrine and serotonin inhibitor, lorcaserin, a serotonin 2C receptor agonist, and beloranib, a methionine aminopeptidase 2 (MetAP2) inhibitor, were discontinued due to significant adverse events of thromboembolism and other safety concerns (14). Beloranib was initially developed as a cancer drug but its use resulted in progressive weight loss in patients and rodents with HO due to hypothalamic injury (52, 53). Its mechanism for weight loss is not fully understood, but it is believed that it increases fat oxidation and lipolysis resulting in reduced fat mass. Furthermore, it leads to a reduction in caloric intake which is likely centrally mediated. Finally, the combination of bupropion, a norepinephrine-dopamine reuptake inhibitor, and naltrexone, an opioid receptor antagonist (Figure 1), has a black box warning of increased suicidal risk and ideation in young adults (46). There are no studies of bupropion-naltrexone in patients with HO.
Figure 1 Interactions of different anti-obesity agents with orexigenic and anorexigenic pathways of the homeostatic “appetite” center and the “reward” system. Even if mediobasal hypothalamic structures such as the arcuate nucleus (ARC) and para-ventricular nucleus (PVN) are damaged, these drugs can interact with peripheral or brain receptors outside of hypothalamic structures. NPY, neuropeptide Y; AgRP, agouti-related peptide; POMC, proopiomelanocortin; CART, cocaine and amphetamine regulated transcript; NAC, nucleus accumbens; DR, dopamine receptor; DAT, dopamine active transporter; DVC, dorsal vagal complex of brainstem; IML, intermediolateral nucleus along spinal cord containing MC4R–expressing preganglionic cholinergic sympathetic neurons; blue, stimulatory and red, inhibitory receptors; TRH, thyrotropin-releasing hormone; CRH, corticotropin-releasing hormone (created with BioRender.com).
Besides its well described effects on glucose metabolism (54), GLP-1 also functions as a satiety hormone, promoting reduced food intake and meal termination through direct action on the vagus nerve and the brain, including the hypothalamus, hindbrain, hippocampus and mesolimbic brain reward system (55). In addition, GLP1RA treatment activates catecholamine neurons in the area postrema (56). GLP1RAs can be effective weight loss agents in HO due to their effects outside of the hypothalamus. In addition, GLP1RAs may change the balance of sympathetic/parasympathetic tone (57–59), potentially counteracting the abnormalities we have seen in HO (42). GLP1Rs are widely expressed in peripheral tissues that are important for energy regulation, such as adipose tissue, pancreas, liver, and gut (Figure 1). In rodents, GLP1RA semaglutide modulates food preference, reduces food intake, and causes weight loss without decreasing energy expenditure (60). In humans, GLP1RAs, such as semaglutide in the setting of type 2 diabetes (T2D) treatment, reduce the risk of cardiovascular death, nonfatal myocardial infarction and nonfatal stroke (61). Liraglutide is approved in children age 10 years and older for T2D (62), and recently semaglutide was approved for the treatment of obesity in adolescents 12 years and older, with 17% decrease in BMI with semaglutide compared with placebo (63). Liraglutide causes dose-dependent and treatment duration-dependent thyroid C-cell tumors in rodent models, at much higher dose exposures than those used in humans. Therefore, even if the relevance for humans of such tumors has not been determined, GLP1RAs are contraindicated in patients with a family history of medullary thyroid carcinoma or multiple endocrine neoplasia syndrome type 2 (64). Otherwise, gastrointestinal adverse effects are frequent in particular at start of treatment, potentially leading to discontinuation of treatment (65).
Recently, our group tested weekly exenatide in a multicenter, randomized, double-blind, placebo-controlled clinical trial in 10- to 25-year-old children and young adults with HO in the context of hypothalamic injury following intracranial tumor (ECHO trial). In this trial, study participants were randomized to once weekly subcutaneous injections of exenatide 2 mg or placebo for 36 weeks, followed by an 18 week open label once weekly exenatide 2 mg extension for all participants. Modest effects were seen on BMI reduction, while there was a significant reduction of body fat measured by DXA and waist circumference in the exenatide group vs. placebo after 36 weeks (66, 67). Overall, exenatide was well tolerated with the majority of adverse events related to gastrointestinal problems such as nausea and vomiting. In this study, the degree of hypothalamic damage was assessed using a hypothalamic lesion score (HLS). Patients with more extensive hypothalamic damage with involvement of the mammillary bodies showed greater reductions in adiposity following GLP1RA treatment (68). One possible explanation for these study findings is that destruction of endogenous ligand sites of action in the hypothalamus heightens responsiveness of extra-hypothalamic sites of action to exogenous ligands such as GLP1RAs. A clinical trial with newer and more potent GLP1RAs has not yet been performed in patients with HO. Furthermore, incretin-based multiple agonists, such as dual GLP-1/glucose-dependent insulinotropic polypeptide (GIP) receptor agonist tirzepatide or triple GIP/GLP-1, and glucagon receptor agonist retatrutide, are promising for treatment of HO, as multiple redundant weight regulatory pathways are simultaneously targeted (see section 1.7), potentially preventing compensatory weight regain.
Oxytocin (OXT) is a 9-amino acid peptide made in the hypothalamic paraventricular and supraoptic nuclei. Its effect has been tested outside its classical use during labor, and a substantial body of literature supports its favorable safety profile in a wide range of conditions, mostly neuropsychiatric (69).
The neuropeptide OXT induces appetite suppression and acts via brain reward centers and peripheral receptors. OXT-induced weight loss may also be partly due to increased lipolysis and energy expenditure by stimulation of the sympathetic tone and adipose tissue thermogenesis (70). The potential role of OXT as a therapy for obesity has been recently investigated (71). A recent study demonstrated that a single dose of intranasal OXT leads to decreased caloric intake at a subsequent meal in men who are lean, overweight, and with obesity (72). Moreover, in a randomized controlled trial of healthy adults with obesity (N=24), 8 weeks of intranasal OXT (24 IU at mealtimes and bedtime) led to an 8.9 kg weight loss (73).
OXT status has been investigated in individuals with history of brain tumors affecting the hypothalamus and pituitary (70). In a small pilot study, low OXT levels were associated with higher BMI in men with hypopituitarism and diabetes insipidus, however, this finding did not reach statistical significance (74). In a separate study of individuals with craniopharyngioma, the change in salivary OXT in response to meals (75) and exercise (76) appeared to be related to BMI and eating behaviors (77), thereby demonstrating a potential utility of OXT in HO. Furthermore, in a case report of a child with hypopituitarism, diabetes insipidus, and obesity following therapy for a craniopharyngioma, low dose intranasal OXT 6 IU daily combined with carbohydrate restriction, and naltrexone (Figure 1) led to amelioration of hyperphagia, and a sustained decrease in BMI (78). A recent randomized placebo-controlled clinical trial was conducted to determine whether 8 weeks of intranasal OXT (vs 8 weeks of placebo) promotes weight loss in children, adolescents, and young adults with HO using a cross-over design. In this small pilot study with 10 completers, there was no significant effect of intranasal OXT on body weight change. However, OXT was well tolerated, and in exploratory analyses, benefits of OXT for anxiety and impulsivity were noted (79). Preclinical research using labeled OXT administered intranasally to rhesus macaques or rabbits reached the cerebrospinal fluid and brain (80, 81). It is unclear, to which extent weight reduction in response to peripherally or intranasally administered OXT is related to peripheral or central effects. Individuals with hypothalamic injury might lack the ability to respond to intranasal OXT because key hypothalamic nuclei that impact appetite and energy balance are missing. In animal models, however, administration of OXT outside the hypothalamus, in the hindbrain or peripherally, decreases weight gain via decrease in food intake and activation of catecholamine neurons in nucleus tractus solitarius (NTS, see Figure 1) (70, 82–84).
Therefore, OXT is promising, as it has the potential for reducing energy intake but also increasing energy expenditure by increased brown adipose tissue thermogenesis which is important for avoiding compensatory adaptations to weight loss. However, there are many open questions regarding the mechanisms of action, optimized dosing, and individual factors such as genetics, which might have an impact on responses to OXT treatment.
The combination of oral phentermine and topiramate (Ph/T) is another promising option, which is FDA-approved for people with obesity ≥12y (85, 86). However, this drug has not yet been tested in HO. Ph/T is a sympathomimetic amine combined with a GABA-ergic drug used to treat epilepsy (Figure 1). Children and adolescents with HO have low sympathetic tone (42, 87), thus may benefit from the stimulant-induced decrease in appetite (15, 50). Individuals with HO can also have excess daytime sleepiness (87, 88) which may contribute to impaired regulation of eating behavior and decreased physical activity (26), and may be targetable with stimulants. Reports of other types of stimulant use in individuals with HO have generally demonstrated weight loss or attenuation of weight gain (48, 87, 89). Sibutramine, a compound related to Ph, led to a decrease in BMI in children with HO (14). However, these previous reports demonstrated heterogeneity between participants, and an overall modest effect. Thus, combining Ph with T, may increase the potential maximal weight loss for treatment of HO.
Tesofensine is a centrally acting triple monoamine reuptake inhibitor that tackles low sympathetic tone in patients with HO. Tesofensine results in reduction of caloric intake and weight loss by inhibiting the presynaptic reuptake of dopamine, serotonin, and noradrenaline, and inhibiting the dopamine active transporter (90) (Figure 1). It is combined with the β-blocker metoprolol to reduce potential adverse effects due to adrenergic stimulation. Results from rodent studies suggest that indirect α-adrenergic and D1-dopaminergic stimulation contribute to reduction of food intake and body weight (91). In a recent randomized, double-blind, placebo-controlled Phase 2 trial, Tesomet (tesofensine 0.5 mg + metoprolol 50 mg) or matching placebo was administered daily following a 2:1 randomization for 24 weeks, followed by an open-label extension of another 24 weeks for a total of 48 weeks of treatment. Twenty-one adults with HO (16 females) were randomized, and 18 patients completed 24 weeks of treatment. Tesomet treatment resulted in significant reductions in body weight, waist circumference and glucose levels compared to placebo (92), which were the main efficacy endpoints. The study results also showed that Tesomet was safe and well tolerated and drug-related adverse events were mostly mild sleep disturbances, dry mouth, and headache. There were no significant differences in heart rate or blood pressure between Tesomet and placebo groups (92). A larger clinical trial has not yet been performed testing Tesomet in HO, but a Phase 3 clinical trial is planned.
The MC pathway plays a central role in the regulation of energy homeostasis (93). The MC system consists of two distinct neurons expressed in the arcuate nucleus that express POMC and agouti-related protein (AgRP). These neurons mediate opposing effects on downstream neurons that express the MC-4 receptor (MC4R). POMC neuron activation induces synaptic release of α-melanocyte stimulating hormone (α-MSH - an MC4R agonist), while AgRP is an inverse agonist that binds to and blocks signaling by MC4R (38, 39). The MC4R is one of five human MC receptor subtypes (MC1−5R) that share class A G-protein-coupled receptors (94). The receptors are critically involved in the regulation of energy homeostasis, pigmentation, cardiovascular function, and sexual functions (94). The MC4R plays a key role in energy balance and appetite regulation and is expressed in different brain areas including the paraventricular nucleus of the hypothalamus, areas of the brain reward system, the dorsal motor nucleus of the hindbrain, the preganglionic cholinergic sympathetic neurons of the spinal cord and vagal afferent nerves (Figure 1) (95, 96). When activated, it reduces food intake, increases energy expenditure, and regulates gut hormone secretion such as the potent anorexigenic hormone PYY3-36 via enteroendocrine cells (97). Consequently, administration of pharmaceutical agents that agonize MC4R cause weight loss by suppressing energy intake and stimulating energy expenditure, whereas genetic or pharmacological inhibition of MC4R results in hyperphagia and obesity. While most of the effects of α-MSH are mediated by the brain, it is also released into circulation from the pituitary gland (41, 98, 99), and can act on peripheral tissues, such as the enteric nervous system (100), and brown adipose tissue (101, 102). Systemic α-MSH increases muscle thermogenesis and glucose clearance by increased muscle glucose uptake (41, 97). Moreover, further evidence suggests that the MC pathway is important in the regulation of glucose metabolism, independent of its effects on energy balance. Restoring expression of leptin receptors in POMC neurons normalizes blood glucose and ameliorates hepatic insulin resistance independent of changes in body weight (103).
Synthetic MC receptor agonists are promising for treatment of genetic and surgery-induced HO affecting the MC4R pathway (104) as there is clear evidence that central MC signaling is deficient (40, 41, 44, 105–107) in patients with hypothalamic lesions. Thus, treatment with a MC receptor agonist could present as targeted treatment supplementing a deficiency. For example, setmelanotide, a MC4R agonist is FDA approved for treatment of monogenic obesity related to POMC deficiency, leptin receptor (LEPR) deficiency (108, 109), and Bardet Biedl syndrome (110). Most recently setmelanotide has also been shown to be effective in reducing hunger and BMI in patients with HO due to hypothalamic injury (111, 112), which could mark a breakthrough for the treatment of aquired HO. Side effects included nausea and vomiting. A large international multicenter Phase 3 clinical trial over 1 year investigating the use of setmelanotide in HO is currently underway.
HO is a complex and rare disorder that is characterized by excessive weight gain and difficulty in losing weight due to disruptions in the hypothalamus with resultant dysregulation of appetite and metabolism. Its treatment involves a multidisciplinary approach including dietary modifications, physical activity, and behavioral interventions, but they often fail to result in meaningful and sustained reduction of body mass index. Thus, anti-obesity pharmacotherapy has been increasingly used to promote further weight loss. The anti-obesity drugs for HO that were featured in this review were oxytocin, oral phentermine and topiramate, exenatide, tesofensine, and setmelanotide. Apart from one phase 3 randomized controlled trial that enrolled 42 participants testing weekly exenatide (66), most studies in HO are small case reports or series with fewer than 10 individuals given the rarity of this condition. Thus, current weight loss drugs for HO are being used off label with variable results and require close monitoring. New clinical trials, however, are underway to investigate the use of promising anti-obesity pharmacotherapy for treatment of HO.
The landscape of obesity pharmacotherapy is evolving quickly with frequent development of new drugs. Examples are dual and triple agonists that target multiple receptors simultaneously to enhance the effects of various hormones involved in appetite regulation and metabolism to promote weight loss (113). Tirzepatide, a GLP-1 and GIP receptor agonist is an example of a dual agonist that was recently FDA approved for the treatment of adults with type 2 diabetes with its clinical trials showing up to 20% weight loss in participants on the 15 mg dose (114). Retatrutide is an agonist of GIP, GLP-1, and glucagon receptors making it a “triple agonist.” Recent Phase 2 trial results showed 48 weeks of retatrutide treatment resulted in substantial reductions in body weight of -24.2% in the 12-mg group (115). For successful intervention of this treatment-resistant form of obesity, personalized treatment approaches appear to be necessary. Individualized based on pathophysiology and initial treatment responses, Iersel et al. developed algorithms which may be the right direction for the treatment of HO based on phenotypic spectrum (17). If there is no beneficial effect on weight reduction after 3 months of treatment, switching to another antiobesity drug or a combination of drugs might be indicated. Currently, MC4R agonism, i.e. supplementing melanocortin deficiency, could represent a new benchmark for the treatment of HO.
CR: Conceptualization, Writing – original draft, Writing – review & editing. AZ: Writing – original draft, Writing – review & editing.
The authors declare financial support was received for the research, authorship, and/or publication of this article. CR is currently funded by Grants from the NIH (R01s DK98466, DK117623, DK135125, DK 135211) and Dept. of Defense (W81XWH-20-1-0299).
CR has received compensation for consulting, advisory board participation, and speaking engagements from Rhythm Pharmaceuticals.
The remaining author declares that the research was conducted in the absence of any commercial or financial relationships that could be construed as a potential conflict of interest.
All claims expressed in this article are solely those of the authors and do not necessarily represent those of their affiliated organizations, or those of the publisher, the editors and the reviewers. Any product that may be evaluated in this article, or claim that may be made by its manufacturer, is not guaranteed or endorsed by the publisher.
1. Ahmet A, Blaser S, Stephens D, Guger S, JT R, Hamilton J. Weight gain in craniopharyngioma–a model for hypothalamic obesity. J Pediatr Endocrinol Metab (2006) 19(2):121–7. doi: 10.1515/JPEM.2006.19.2.121
2. Kim JH, Choi JH. Pathophysiology and clinical characteristics of hypothalamic obesity in children and adolescents. Ann Pediatr Endocrinol Metab (2013) 18(4):161–7. doi: 10.6065/apem.2013.18.4.161
3. Muller HL. Childhood craniopharyngioma. Recent advances in diagnosis, treatment and follow-up. Horm Res (2008) 69(4):193–202. doi: 10.1159/000113019
4. Rose SR, Horne VE, Bingham N, Jenkins T, Black J, Inge T. Hypothalamic obesity: 4 years of the international registry of hypothalamic obesity disorders. Obes (Silver Spring) (2018) 26(11):1727–32. doi: 10.1002/oby.22315
5. Zegers D, Van Hul W, Van Gaal LF, Beckers S. Monogenic and complex forms of obesity: insights from genetics reveal the leptin-melanocortin signaling pathway as a common player. Crit Rev Eukaryot Gene Expr (2012) 22(4):325–43. doi: 10.1615/CritRevEukarGeneExpr.v22.i4.60
6. Sterkenburg AS, Hoffmann A, Gebhardt U, Warmuth-Metz M, Daubenbuchel AM, Muller HL. Survival, hypothalamic obesity, and neuropsychological/psychosocial status after childhood-onset craniopharyngioma: newly reported long-term outcomes. Neuro Oncol (2015) 17(7):1029–38. doi: 10.1093/neuonc/nov044
7. Van Gaal LF, Mertens IL, De Block CE. Mechanisms linking obesity with cardiovascular disease. Nature (2006) 444(7121):875–80. doi: 10.1038/nature05487
8. Greenway FL, Bray GA. Treatment of hypothalamic obesity with caffeine and ephedrine. Endocr Pract (2008) 14(6):697–703. doi: 10.4158/EP.14.6.697
9. Hamilton JK, Conwell LS, Syme C, Ahmet A, Jeffery A, Daneman D. Hypothalamic obesity following craniopharyngioma surgery: results of a pilot trial of combined diazoxide and metformin therapy. Int J Pediatr Endocrinol (2011) 2011:417949. doi: 10.1155/2011/417949
10. Bereket A, Kiess W, Lustig RH, Muller HL, Goldstone AP, Weiss R, et al. Hypothalamic obesity in children. Obes Rev (2012) 13(9):780–98. doi: 10.1111/j.1467-789X.2012.01004.x
11. Lustig RH. Hypothalamic obesity after craniopharyngioma: mechanisms, diagnosis, and treatment. Front Endocrinol (Lausanne) (2011) 2:60. doi: 10.3389/fendo.2011.00060
12. Lustig RH. Hypothalamic obesity: causes, consequences, treatment. Pediatr Endocrinol Rev (2008) 6(2):220–7.
13. Lustig RH, Hinds PS, Ringwald-Smith K, Christensen RK, Kaste SC, Schreiber RE, et al. Octreotide therapy of pediatric hypothalamic obesity: a double-blind, placebo-controlled trial. J Clin Endocrinol Metab (2003) 88(6):2586–92. doi: 10.1210/jc.2002-030003
14. Danielsson P, Janson A, Norgren S, Marcus C. Impact sibutramine therapy in children with hypothalamic obesity or obesity with aggravating syndromes. J Clin Endocrinol Metab (2007) 92(11):4101–6. doi: 10.1210/jc.2007-0826
15. Mason PW, Krawiecki N, Meacham LR. The use of dextroamphetamine to treat obesity and hyperphagia in children treated for craniopharyngioma. Arch Pediatr Adolesc Med (2002) 156(9):887–92. doi: 10.1001/archpedi.156.9.887
16. Ismail D, O'Connell MA, Zacharin MR. Dexamphetamine use for management of obesity and hypersomnolence following hypothalamic injury. J Pediatr Endocrinol Metab (2006) 19(2):129–34. doi: 10.1515/JPEM.2006.19.2.129
17. van Iersel L, Brokke KE, Adan RAH, Bulthuis LCM, van den Akker ELT, van Santen HM. Pathophysiology and individualized treatment of hypothalamic obesity following craniopharyngioma and other suprasellar tumors: A systematic review. Endocr Rev (2019) 40(1):193–235. doi: 10.1210/er.2018-00017
18. Srinivasan S, Ogle GD, Garnett SP, Briody JN, Lee JW, Cowell CT. Features of the metabolic syndrome after childhood craniopharyngioma. J Clin Endocrinol Metab (2004) 89(1):81–6. doi: 10.1210/jc.2003-030442
19. Simoneau-Roy J, O'Gorman C, Pencharz P, Adeli K, Daneman D, Hamilton J. Insulin sensitivity and secretion in children and adolescents with hypothalamic obesity following treatment for craniopharyngioma. Clin Endocrinol (Oxf) (2010) 72(3):364–70. doi: 10.1111/j.1365-2265.2009.03639.x
20. Mong S, Pomeroy SL, Cecchin F, Juraszek A, Alexander ME. Cardiac risk after craniopharyngioma therapy. Pediatr Neurol (2008) 38(4):256–60. doi: 10.1016/j.pediatrneurol.2007.11.007
21. Erfurth EM. Endocrine aspects and sequel in patients with craniopharyngioma. J Pediatr Endocrinol Metab (2015) 28(1-2):19–26. doi: 10.1515/jpem-2014-0419
22. Olsson DS, Andersson E, Bryngelsson IL, Nilsson AG, Johannsson G. Excess mortality and morbidity in patients with craniopharyngioma, especially in patients with childhood onset: a population-based study in Sweden. J Clin Endocrinol Metab (2015) 100(2):467–74. doi: 10.1210/jc.2014-3525
23. Muller HL, Faldum A, Etavard-Gorris N, Gebhardt U, Oeverink R, Kolb R, et al. Functional capacity, obesity and hypothalamic involvement: cross-sectional study on 212 patients with childhood craniopharyngioma. Klin Padiatr (2003) 215(6):310–4. doi: 10.1159/000083671
24. Jung SY, Lee YJ, Lee HJ, Lee YA, Moon JS, Ko JS, et al. Nonalcoholic fatty liver disease in long-term survivors of childhood-onset craniopharyngioma. Ann Pediatr Endocrinol Metab (2017) 22(3):189–96. doi: 10.6065/apem.2017.22.3.189
25. Hoffmann A, Postma FP, Sterkenburg AS, Gebhardt U, Muller HL. Eating behavior, weight problems and eating disorders in 101 long-term survivors of childhood-onset craniopharyngioma. J Pediatr Endocrinol Metab (2015) 28(1-2):35–43. doi: 10.1515/jpem-2014-0415
26. Harz KJ, Muller HL, Waldeck E, Pudel V, Roth C. Obesity in patients with craniopharyngioma: assessment of food intake and movement counts indicating physical activity. J Clin Endocrinol Metab (2003) 88(11):5227–31. doi: 10.1210/jc.2002-021797
27. Roth CL, Eslamy H, Werny D, Elfers C, Shaffer ML, Pihoker C, et al. Semi-quantitative analysis of hypothalamic damage on MRI predicts risk for hypothalamic obesity. Obes (Silver Spring) (2015) 23(6):1226–33. doi: 10.1002/oby.21067
28. Elowe-Gruau E, Beltrand J, Brauner R, Pinto G, Samara-Boustani D, Thalassinos C, et al. Childhood craniopharyngioma: hypothalamus-sparing surgery decreases the risk of obesity. J Clin Endocrinol Metab (2013) 98(6):2376–82. doi: 10.1210/jc.2012-3928
29. Muller HL, Gebhardt U, Teske C, Faldum A, Zwiener I, Warmuth-Metz M, et al. Post-operative hypothalamic lesions and obesity in childhood craniopharyngioma: results of the multinational prospective trial KRANIOPHARYNGEOM 2000 after 3-year follow-up. Eur J Endocrinol (2011) 165(1):17–24. doi: 10.1530/EJE-11-0158
30. Roth CL. Hypothalamic obesity in patients with craniopharyngioma: profound changes of several weight regulatory circuits. Front Endocrinol (Lausanne) (2011) 2:49. doi: 10.3389/fendo.2011.00049
31. O'Gorman CS, Simoneau-Roy J, Pencharz Mb P, Adeli K, Hamilton J. Delayed ghrelin suppression following oral glucose tolerance test in children and adolescents with hypothalamic injury secondary to craniopharyngioma compared with obese controls. Int J Pediatr Obes (2011) 6(3-4):285–8. doi: 10.3109/17477166.2010.519388
32. Roth CL, Gebhardt U, Muller HL. Appetite-regulating hormone changes in patients with craniopharyngioma. Obes (Silver Spring) (2011) 19(1):36–42. doi: 10.1038/oby.2010.80
33. Muller HL. Increased daytime sleepiness in patients with childhood craniopharyngioma and hypothalamic tumor involvement: review of the literature and perspectives. Int J Endocrinol (2010) 2010:519607. doi: 10.1155/2010/519607
34. Muller HL, Handwerker G, Wollny B, Faldum A, Sorensen N. Melatonin secretion and increased daytime sleepiness in childhood craniopharyngioma patients. J Clin Endocrinol Metab (2002) 87(8):3993–6. doi: 10.1210/jcem.87.8.8751
35. Muller HL, Emser A, Faldum A, Bruhnken G, Etavard-Gorris N, Gebhardt U, et al. Longitudinal study on growth and body mass index before and after diagnosis of childhood craniopharyngioma. J Clin Endocrinol Metab (2004) 89(7):3298–305. doi: 10.1210/jc.2003-031751
36. Erfurth EM. Diagnosis, background, and treatment of hypothalamic damage in craniopharyngioma. Neuroendocrinology (2020) 110(9-10):767–79. doi: 10.1159/000509616
37. Abuzzahab MJ, Roth CL, Shoemaker AH. Hypothalamic obesity: prologue and promise. Horm Res Paediatr (2019) 91(2):128–36. doi: 10.1159/000496564
38. Wilkinson CW. Roles of acetylation and other post-translational modifications in melanocortin function and interactions with endorphins. Peptides (2006) 27(2):453–71. doi: 10.1016/j.peptides.2005.05.029
39. Perroud B, Alvarado RJ, Espinal GM, Morado AR, Phinney BS, Warden CH. In vivo multiplex quantitative analysis of 3 forms of alpha melanocyte stimulating hormone in pituitary of prolyl endopeptidase deficient mice. Mol Brain (2009) 2:14. doi: 10.1186/1756-6606-2-14
40. Roth CL, Enriori PJ, Gebhardt U, Hinney A, Muller HL, Hebebrand J, et al. Changes of peripheral alpha-melanocyte-stimulating hormone in childhood obesity. Metabolism (2010) 59(2):186–94. doi: 10.1016/j.metabol.2009.06.031
41. Enriori PJ, Chen W, Garcia-Rudaz MC, Grayson BE, Evans AE, Comstock SM, et al. alpha-Melanocyte stimulating hormone promotes muscle glucose uptake via melanocortin 5 receptors. Mol Metab (2016) 5(10):807–22. doi: 10.1016/j.molmet.2016.07.009
42. Roth CL, Hunneman DH, Gebhardt U, Stoffel-Wagner B, Reinehr T, Muller HL. Reduced sympathetic metabolites in urine of obese patients with craniopharyngioma. Pediatr Res (2007) 61(4):496–501. doi: 10.1203/pdr.0b013e3180332cd6
43. Muller HL, Bueb K, Bartels U, Roth C, Harz K, Graf N, et al. Obesity after childhood craniopharyngioma–German multicenter study on pre-operative risk factors and quality of life. Klin Padiatr (2001) 213(4):244–9. doi: 10.1055/s-2001-16855
44. Roth C, Wilken B, Hanefeld F, Schroter W, Leonhardt U. Hyperphagia in children with craniopharyngioma is associated with hyperleptinaemia and a failure in the downregulation of appetite. Eur J Endocrinol (1998) 138(1):89–91. doi: 10.1530/eje.0.1380089
45. Muller HL, Tauber M, Lawson EA, Ozyurt J, Bison B, Martinez-Barbera JP, et al. Hypothalamic syndrome. Nat Rev Dis Primers (2022) 8(1):24. doi: 10.1038/s41572-022-00351-z
46. Singhal V, Sella AC, Malhotra S. Pharmacotherapy in pediatric obesity: current evidence and landscape. Curr Opin Endocrinol Diabetes Obes (2021) 28(1):55–63. doi: 10.1097/MED.0000000000000587
47. Bray GA. Medical treatment of obesity: the past, the present and the future. Best Pract Res Clin Gastroenterol (2014) 28(4):665–84. doi: 10.1016/j.bpg.2014.07.015
48. Denzer C, Denzer F, Lennerz BS, Vollbach H, Lustig RH, Wabitsch M. Treatment of hypothalamic obesity with dextroamphetamine: A case series. Obes Facts (2019) 12(1):91–102. doi: 10.1159/000495851
49. van Schaik J, Welling MS, de Groot CJ, van Eck JP, Juriaans A, Burghard M, et al. Dextroamphetamine treatment in children with hypothalamic obesity. Front Endocrinol (Lausanne) (2022) 13:845937. doi: 10.3389/fendo.2022.845937
50. Elfers CT, Roth CL. Effects of methylphenidate on weight gain and food intake in hypothalamic obesity. Front Endocrinol (Lausanne) (2011) 2:78. doi: 10.3389/fendo.2011.00078
51. Sadatomo T, Sakoda K, Yamanaka M, Kutsuna M, Kurisu K. Mazindol administration improved hyperphagia after surgery for craniopharyngioma–case report. Neurol Med Chir (Tokyo) (2001) 41(4):210–2. doi: 10.2176/nmc.41.210
52. Elfers CT, Roth CL. Robust reductions of excess weight and hyperphagia by beloranib in rat models of genetic and hypothalamic obesity. Endocrinology (2017) 158(1):41–55. doi: 10.1210/en.2016-1665
53. Shoemaker A, Proietto J, Abuzzahab MJ, Markovic T, Malloy J, Kim DD. A randomized, placebo-controlled trial of beloranib for the treatment of hypothalamic injury-associated obesity. Diabetes Obes Metab (2017) 19(8):1165–70. doi: 10.1111/dom.12928
54. Drucker DJ. Mechanisms of action and therapeutic application of glucagon-like peptide-1. Cell Metab (2018) 27(4):740–56. doi: 10.1016/j.cmet.2018.03.001
55. Kanoski SE, Hayes MR, Skibicka KP. GLP-1 and weight loss: unraveling the diverse neural circuitry. Am J Physiol Regul Integr Comp Physiol (2016) 310(10):R885–95. doi: 10.1152/ajpregu.00520.2015
56. Yamamoto H, Lee CE, Marcus JN, Williams TD, Overton JM, Lopez ME, et al. Glucagon-like peptide-1 receptor stimulation increases blood pressure and heart rate and activates autonomic regulatory neurons. J Clin Invest (2002) 110(1):43–52. doi: 10.1172/JCI0215595
57. Nakatani Y, Kawabe A, Matsumura M, Aso Y, Yasu T, Banba N, et al. Effects of GLP-1 receptor agonists on heart rate and the autonomic nervous system using holter electrocardiography and power spectrum analysis of heart rate variability. Diabetes Care (2016) 39(2):e22–3. doi: 10.2337/dc15-1437
58. Kumarathurai P, Anholm C, Larsen BS, Olsen RH, Madsbad S, Kristiansen O, et al. Effects of liraglutide on heart rate and heart rate variability: A randomized, double-blind, placebo-controlled crossover study. Diabetes Care (2017) 40(1):117–24. doi: 10.2337/dc16-1580
59. Cacciatori V, Zoppini G, Bellavere F, Rigolon R, Thomaseth K, Pichiri I, et al. Long-acting GLP-1 receptor agonist exenatide influence on the autonomic cardiac sympatho-vagal balance. J Endocr Soc (2018) 2(1):53–62. doi: 10.1210/js.2017-00300
60. Gabery S, Salinas CG, Paulsen SJ, Ahnfelt-Ronne J, Alanentalo T, Baquero AF, et al. Semaglutide lowers body weight in rodents via distributed neural pathways. JCI Insight (2020) 5(6). doi: 10.1172/jci.insight.133429
61. Marso SP, Bain SC, Consoli A, Eliaschewitz FG, Jodar E, Leiter LA, et al. Semaglutide and cardiovascular outcomes in patients with type 2 diabetes. N Engl J Med (2016) 375(19):1834–44. doi: 10.1056/NEJMoa1607141
62. Tamborlane WV, Barrientos-Perez M, Fainberg U, Frimer-Larsen H, Hafez M, Hale PM, et al. Liraglutide in children and adolescents with type 2 diabetes. New Engl J Med (2019) 381(7):637–46. doi: 10.1056/NEJMoa1903822
63. Weghuber D, Boberg K, Hesse D, Jeppesen OK, Sorrig R, Kelly AS, et al. Semaglutide treatment for obesity in teenagers: a plain language summary of the STEP TEENS research study. J Comp Eff Res (2023) 12(2):e220187. doi: 10.2217/cer-2022-0187
64. Kuhnen P, Biebermann H, Wiegand S. Pharmacotherapy in childhood obesity. Horm Res Paediatr (2022) 95(2):177–92. doi: 10.1159/000518432
65. Hirsch IB. The future of the GLP-1 receptor agonists. JAMA (2019) 321(15):1457–8. doi: 10.1001/jama.2019.2941
66. Roth CL, Perez FA, Whitlock KB, Elfers C, Yanovski JA, Shoemaker AH, et al. A phase 3 randomized clinical trial using a once-weekly glucagon-like peptide-1 receptor agonist in adolescents and young adults with hypothalamic obesity. Diabetes Obes Metab (2021) 23(2):363–73. doi: 10.1111/dom.14224
67. Shoemaker AH, Silver HJ, Buchowski M, Slaughter JC, Yanovski JA, Elfers C, et al. Energy balance in hypothalamic obesity in response to treatment with a once-weekly GLP-1 receptor agonist. Int J Obes (Lond) (2022) 46(3):623–9. doi: 10.1038/s41366-021-01043-6
68. Perez FA, Elfers C, Yanovski JA, Shoemaker AH, Abuzzahab MJ, Roth CL. MRI measures of hypothalamic injury are associated with glucagon-like peptide-1 receptor agonist treatment response in people with hypothalamic obesity. Diabetes Obes Metab (2021) 23(7):1532–41. doi: 10.1111/dom.14366
69. MacDonald E, Dadds MR, Brennan JL, Williams K, Levy F, Cauchi AJ. A review of safety, side-effects and subjective reactions to intranasal oxytocin in human research. Psychoneuroendocrinology (2011) 36(8):1114–26. doi: 10.1016/j.psyneuen.2011.02.015
70. McCormack SE, Blevins JE, Lawson EA. Metabolic effects of oxytocin. Endocr Rev (2020) 41(2):121–45. doi: 10.1210/endrev/bnz012
71. Blevins JE, Baskin DG. Translational and therapeutic potential of oxytocin as an anti-obesity strategy: Insights from rodents, nonhuman primates and humans. Physiol Behav (2015) 152:438–49. doi: 10.1016/j.physbeh.2015.05.023
72. Lawson EA, Marengi DA, DeSanti RL, Holmes TM, Schoenfeld DA, Tolley CJ. Oxytocin reduces caloric intake in men. Obes (Silver Spring) (2015) 23(5):950–6. doi: 10.1002/oby.21069
73. Zhang H, Wu C, Chen Q, Chen X, Xu Z, Wu J, et al. Treatment of obesity and diabetes using oxytocin or analogs in patients and mouse models. PloS One (2013) 8(5):e61477. doi: 10.1371/journal.pone.0061477
74. Aulinas A, Plessow F, Asanza E, Silva L, Marengi DA, Fan W, et al. Low plasma oxytocin levels and increased psychopathology in hypopituitary men with diabetes insipidus. J Clin Endocrinol Metab (2019) 134(8):3181–91. doi: 10.1210/jc.2018-02608
75. Daubenbuchel AM, Hoffmann A, Eveslage M, Ozyurt J, Lohle K, Reichel J, et al. Oxytocin in survivors of childhood-onset craniopharyngioma. Endocrine (2016) 54(2):524–31. doi: 10.1007/s12020-016-1084-5
76. Gebert D, Auer MK, Stieg MR, Freitag MT, Lahne M, Fuss J, et al. De-masking oxytocin-deficiency in craniopharyngioma and assessing its link with affective function. Psychoneuroendocrinology (2018) 88:61–9. doi: 10.1016/j.psyneuen.2017.11.006
77. Daubenbuchel AM, Ozyurt J, Boekhoff S, Warmuth-Metz M, Eveslage M, Muller HL. Eating behaviour and oxytocin in patients with childhood-onset craniopharyngioma and different grades of hypothalamic involvement. Pediatr Obes (2019) 14(9):e12527. doi: 10.1111/ijpo.12527
78. Hsu EA, Miller JL, Perez FA, Roth CL. Oxytocin and Naltrexone successfully treat hypothalamic obesity in a boy post-craniopharyngioma resection. J Clin Endocrinol Metab (2017) 103(2):370–5. doi: 10.1210/jc.2017-02080
79. McCormack SE, Wang Z, Wade KL, Dedio A, Cilenti N, Crowley J, et al. A pilot randomized clinical trial of intranasal oxytocin to promote weight loss in individuals with hypothalamic obesity. J Endocr Soc (2023) 7(5):bvad037. doi: 10.1210/jendso/bvad037
80. Lee MR, Scheidweiler KB, Diao XX, Akhlaghi F, Cummins A, Huestis MA, et al. Oxytocin by intranasal and intravenous routes reaches the cerebrospinal fluid in rhesus macaques: determination using a novel oxytocin assay. Mol Psychiatry (2018) 23(1):115–22. doi: 10.1038/mp.2017.27
81. Ishii D, Kageyama M, Umeda S. Cerebral and extracerebral distribution of radioactivity associated with oxytocin in rabbits after intranasal administration: Comparison of TTA-121, a newly developed oxytocin formulation, with Syntocinon. PloS One (2021) 16(12):e0261451. doi: 10.1371/journal.pone.0261451
82. Anekonda VT, Thompson BW, Ho JM, Roberts ZS, Edwards MM, Nguyen HK, et al. Hindbrain administration of oxytocin reduces food intake, weight gain and activates catecholamine neurons in the hindbrain nucleus of the solitary tract in rats. J Clin Med (2021) 10(21):5078. doi: 10.3390/jcm10215078
83. Elfers CT, Blevins JE, Lawson EA, Pittner R, Silva D, Kiselyov A, et al. Robust reductions of body weight and food intake by an oxytocin analog in rats. Front Physiol (2021) 12:726411. doi: 10.3389/fphys.2021.726411
84. Asker M, Krieger JP, Liles A, Tinsley IC, Borner T, Maric I, et al. Peripherally restricted oxytocin is sufficient to reduce food intake and motivation, while CNS entry is required for locomotor and taste avoidance effects. Diabetes Obes Metab (2023) 25(3):856–77. doi: 10.1111/dom.14937
85. Allison DB, Gadde KM, Garvey WT, Peterson CA, Schwiers ML, Najarian T, et al. Controlled-release phentermine/topiramate in severely obese adults: a randomized controlled trial (EQUIP). Obes (Silver Spring) (2012) 20(2):330–42. doi: 10.1038/oby.2011.330
86. Hsia DS, Gosselin NH, Williams J, Farhat N, Marier JF, Shih W, et al. A randomized, double-blind, placebo-controlled, pharmacokinetic and pharmacodynamic study of a fixed-dose combination of phentermine/topiramate in adolescents with obesity. Diabetes Obes Metab (2020) 22(4):480–91. doi: 10.1111/dom.13910
87. Son JW, Kim S. Comprehensive review of current and upcoming anti-obesity drugs. Diabetes Metab J (2020) 44(6):802–18. doi: 10.4093/dmj.2020.0258
88. Mandrell BN, LaRosa K, Hancock D, Caples M, Sykes A, Lu Z, et al. Predictors of narcolepsy and hypersomnia due to medical disorder in pediatric craniopharyngioma. J Neurooncol (2020) 148(2):307–16. doi: 10.1007/s11060-020-03519-3
89. Horne VE, Bielamowicz K, Nguyen J, Hilsenbeck S, Lindsay H, Sonabend R, et al. Methylphenidate improves weight control in childhood brain tumor survivors with hypothalamic obesity. Pediatr Blood Cancer (2020) 67(7):e28379. doi: 10.1002/pbc.28379
90. Hansen HH, Hansen G, Tang-Christensen M, Larsen PJ, Axel AM, Raben A, et al. The novel triple monoamine reuptake inhibitor tesofensine induces sustained weight loss and improves glycemic control in the diet-induced obese rat: comparison to sibutramine and rimonabant. Eur J Pharmacol (2010) 636(1-3):88–95. doi: 10.1016/j.ejphar.2010.03.026
91. Axel AM, Mikkelsen JD, Hansen HH. Tesofensine, a novel triple monoamine reuptake inhibitor, induces appetite suppression by indirect stimulation of alpha1 adrenoceptor and dopamine D1 receptor pathways in the diet-induced obese rat. Neuropsychopharmacology (2010) 35(7):1464–76. doi: 10.1038/npp.2010.16
92. Huynh K, Klose M, Krogsgaard K, Drejer J, Byberg S, Madsbad S, et al. Randomized controlled trial of Tesomet for weight loss in hypothalamic obesity. Eur J Endocrinol (2022) 186(6):687–700. doi: 10.1530/EJE-21-0972
93. Holland J, Sorrell J, Yates E, Smith K, Arbabi S, Arnold M, et al. A brain-melanocortin-vagus axis mediates adipose tissue expansion independently of energy intake. Cell Rep (2019) 27(8):2399–410 e6. doi: 10.1016/j.celrep.2019.04.089
94. Heyder NA, Kleinau G, Speck D, Schmidt A, Paisdzior S, Szczepek M, et al. Structures of active melanocortin-4 receptor-Gs-protein complexes with NDP-alpha-MSH and setmelanotide. Cell Res (2021) 31(11):1176–89. doi: 10.1038/s41422-021-00569-8
95. Sohn JW, Harris LE, Berglund ED, Liu T, Vong L, Lowell BB, et al. Melanocortin 4 receptors reciprocally regulate sympathetic and parasympathetic preganglionic neurons. Cell (2013) 152(3):612–9. doi: 10.1016/j.cell.2012.12.022
96. Kishi T, Aschkenasi CJ, Lee CE, Mountjoy KG, Saper CB, Elmquist JK. Expression of melanocortin 4 receptor mRNA in the central nervous system of the rat. J Comp Neurol (2003) 457(3):213–35. doi: 10.1002/cne.10454
97. Rossi J, Balthasar N, Olson D, Scott M, Berglund E, Lee CE, et al. Melanocortin-4 receptors expressed by cholinergic neurons regulate energy balance and glucose homeostasis. Cell Metab (2011) 13(2):195–204. doi: 10.1016/j.cmet.2011.01.010
98. Panaro BL, Tough IR, Engelstoft MS, Matthews RT, Digby GJ, Moller CL, et al. The melanocortin-4 receptor is expressed in enteroendocrine L cells and regulates the release of peptide YY and glucagon-like peptide 1 in vivo. Cell Metab (2014) 20(6):1018–29. doi: 10.1016/j.cmet.2014.10.004
99. Cone RD. Anatomy and regulation of the central melanocortin system. Nat Neurosci (2005) 8(5):571–8. doi: 10.1038/nn1455
100. Gautron L, Lee C, Funahashi H, Friedman J, Lee S, Elmquist J. Melanocortin-4 receptor expression in a vago-vagal circuitry involved in postprandial functions. J Comp Neurol (2010) 518(1):6–24. doi: 10.1002/cne.22221
101. Voss-Andreae A, Murphy JG, Ellacott KL, Stuart RC, Nillni EA, Cone RD, et al. Role of the central melanocortin circuitry in adaptive thermogenesis of brown adipose tissue. Endocrinology (2007) 148(4):1550–60. doi: 10.1210/en.2006-1389
102. Glavas MM, Joachim SE, Draper SJ, Smith MS, Grove KL. Melanocortinergic activation by melanotan II inhibits feeding and increases uncoupling protein 1 messenger ribonucleic acid in the developing rat. Endocrinology (2007) 148(7):3279–87. doi: 10.1210/en.2007-0184
103. Berglund ED, Vianna CR, Donato J Jr., Kim MH, Chuang JC, Lee CE, et al. Direct leptin action on POMC neurons regulates glucose homeostasis and hepatic insulin sensitivity in mice. J Clin Invest (2012) 122(3):1000–9. doi: 10.1172/JCI59816
104. Yeo GSH, Chao DHM, Siegert AM, Koerperich ZM, Ericson MD, Simonds SE, et al. The melanocortin pathway and energy homeostasis: From discovery to obesity therapy. Mol Metab (2021) 48:101206. doi: 10.1016/j.molmet.2021.101206
105. Patel L, Cooper CD, Quinton ND, Butler GE, Gill MS, Jefferson IG, et al. Serum leptin and leptin binding activity in children and adolescents with hypothalamic dysfunction. J Pediatr Endocrinol Metab (2002) 15(7):963–71. doi: 10.1515/JPEM.2002.15.7.963
106. Shaikh MG, Grundy RG, Kirk JM. Hyperleptinaemia rather than fasting hyperinsulinaemia is associated with obesity following hypothalamic damage in children. Eur J Endocrinol (2008) 159(6):791–7. doi: 10.1530/EJE-08-0533
107. Roth CL. Hypothalamic obesity in craniopharyngioma patients: disturbed energy homeostasis related to extent of hypothalamic damage and its implication for obesity intervention. J Clin Med (2015) 4(9):1774–97. doi: 10.3390/jcm4091774
108. Kuhnen P, Clement K, Wiegand S, Blankenstein O, Gottesdiener K, Martini LL, et al. Proopiomelanocortin deficiency treated with a melanocortin-4 receptor agonist. N Engl J Med (2016) 375(3):240–6. doi: 10.1056/NEJMoa1512693
109. Clement K, van den Akker E, Argente J, Bahm A, Chung WK, Connors H, et al. Efficacy and safety of setmelanotide, an MC4R agonist, in individuals with severe obesity due to LEPR or POMC deficiency: single-arm, open-label, multicentre, phase 3 trials. Lancet Diabetes Endocrinol (2020) 8(12):960–70. doi: 10.1016/S2213-8587(20)30364-8
110. Haws R, Brady S, Davis E, Fletty K, Yuan G, Gordon G, et al. Effect of setmelanotide, a melanocortin-4 receptor agonist, on obesity in Bardet-Biedl syndrome. Diabetes Obes Metab (2020) 22(11):2133–40. doi: 10.1111/dom.14133
111. Roth CL, Shoemaker A, Gottschalk M, Miller JL, Yuan G, Chen E, et al. Interim Efficacy and Safety Analysis of Setmelanotide As a Novel Treatment for Hypothalamic Obesity. Special Issue: Abstracts from the 40th Annual Meeting of the Obesity Society at Obesityweek® (2022), (Obesity Week San Diego Nov 2022) 30(S1):125.
112. Roth CL, Shoemaker AH, Gottschalk M, Miller JL, Yuan G, Malhotra S, et al. Impact of Setmelanotide Treatment on Reducing Hyperphagia in Pediatric and Adult Patients With Hypothalamic Obesity. Istanbul, Turkey: Bioscientifica 25th European Congress of Endocrinology. (2023). doi: 10.1530/endoabs.90.P680
113. Chichura KS, Elfers CT, Salameh TS, Kamat V, Chepurny OG, McGivney A, et al. A peptide triple agonist of GLP-1, neuropeptide Y1, and neuropeptide Y2 receptors promotes glycemic control and weight loss. Sci Rep (2023) 13(1):9554. doi: 10.1038/s41598-023-36178-1
114. Jastreboff AM, Aronne LJ, Ahmad NN, Wharton S, Connery L, Alves B, et al. Tirzepatide once weekly for the treatment of obesity. N Engl J Med (2022) 387(3):205–16. doi: 10.1056/NEJMoa2206038
Keywords: obesity, brain tumor, hypothalamic injury, energy homeostasis, drug intervention
Citation: Roth CL and Zenno A (2023) Treatment of hypothalamic obesity in people with hypothalamic injury: new drugs are on the horizon. Front. Endocrinol. 14:1256514. doi: 10.3389/fendo.2023.1256514
Received: 10 July 2023; Accepted: 22 August 2023;
Published: 13 September 2023.
Edited by:
Hermann Lothar Mueller, Klinikum Oldenburg, GermanyReviewed by:
Daisuke Kohno, Gunma University, JapanCopyright © 2023 Roth and Zenno. This is an open-access article distributed under the terms of the Creative Commons Attribution License (CC BY). The use, distribution or reproduction in other forums is permitted, provided the original author(s) and the copyright owner(s) are credited and that the original publication in this journal is cited, in accordance with accepted academic practice. No use, distribution or reproduction is permitted which does not comply with these terms.
*Correspondence: Christian L. Roth, Y2hyaXN0aWFuLnJvdGhAc2VhdHRsZWNoaWxkcmVucy5vcmc=
Disclaimer: All claims expressed in this article are solely those of the authors and do not necessarily represent those of their affiliated organizations, or those of the publisher, the editors and the reviewers. Any product that may be evaluated in this article or claim that may be made by its manufacturer is not guaranteed or endorsed by the publisher.
Research integrity at Frontiers
Learn more about the work of our research integrity team to safeguard the quality of each article we publish.