- Beijing Neurosurgical Institute, Capital Medical University, Beijing, China
The role of the gut microbiome has been widely discussed in numerous works of literature. The biggest concern is the association of the gut microbiome with the central nervous system through the microbiome-brain-gut axis in the past ten years. As more and more research has been done on the relationship between the disease of the central nervous system and gut microbes. This fact is being revealed that gut microbes seem to play an important role from the onset and progression of the disease to clinical symptoms, and new treatments. As a special tumor of the central nervous system, pituitary neuroendocrine tumors (PitNETs)are closely related to metabolism, endocrinology, and immunity. These factors are the vectors through which intestinal microbes interact with the central nervous system. However, little is known about the effects of gut microbes on the PitNET. In this review, the relationship of gut microbiota in PitNETs is introduced, the potential effects of the gut-brain axis in this relationship are analyzed, and future research directions are presented.
1 Introduction
A variety of tumors can occur within sella, including meningiomas, germ cell tumors, and metastases, but primary tumors of the adenohypophysial gland are the most common (1). In 2022, the World Health Organization (WHO) adopted PitNET according to tumor cell lineage, cell type, and related characteristics to describe this type of tumor formerly known as a pituitary adenoma (PA) (2). The process of formation of such tumors is generally thought to be caused by the clonal amplification of a single abnormal cell caused by a somatic genetic mutation or chromosomal abnormality, but the exact mechanism remains unclear (3). These tumors are also known as nonfunction pituitary neuroendocrine tumors (NF-PitNETs), prolactinoma, somatotropinoma, corticotropinoma, thyrotropinoma, and gonadotropinoma, depending on whether they cause hormone overproduction (4). Although these tumors are usually non-malignant, their high prevalence, which occurs in 10% of the population, remains a threat to human health (5). Even with the rapid development of neuroendoscopic surgery, the effective treatment of certain subtypes (invasive/functional) of PitNET has been a difficult problem for clinicians (6). In the further study of the pathogenesis and development of PitNETs and the emerging treatment methods including chemotherapy drugs and immunotherapy, the study of intestinal microbiota in patients with PitNETs will naturally become an interesting direction, and indeed some researchers including us have done so (7–10). As the subject of extensive research in the last decade, the gut microbiota is associated with and plays a role in the occurrence or development of numerous diseases (11–13). With further research, the concept of the “gut-brain axis” has been proposed, which is a bidirectional communication system with complex signaling mechanisms, including the vagus nerve, enteric nervous system, immune system, and release of microbial metabolites (14, 15). These studies have shown that the gut microbiome regulates neurobehavioral characteristics and endocrine function and can communicate with the central nervous system. Given the role of PitNETs, a kind of disease of the central nervous system, in the endocrine system, the potential association with gut microbiota is of interest (10). However, to the best of our knowledge, the relationship between gut microbiota and PitNETs, or even the pituitary gland, has not been clearly defined, although the hypothalamic-pituitary-adrenal (HPA) axis has been studied as a hot spot pathway in the brain-gut axis for many years (16, 17). Encouragingly, a large body of research seems to hint at a relationship between PitNETs and gut microbes. In solid tumors such as lung cancer and melanoma, changing the composition of a patient’s gut microbiota based on fecal microbial transplantation or antibiotic administration can enhance the efficacy of immune checkpoint blocking (ICBs) (18). A study showed the antitumor effects of ICB therapy in Cushing’s disease mice (19). The role of gut microbes in the treatment of central nervous system diseases such as depression has been demonstrated (20). These studies have led to the belief that gut microbes may be new therapeutic targets for PitNETs. This review reviews the research progress of the relationship between gut microbiota and PitNETs and summarizes the possible mechanism of gut microbiota involvement in PitNETs.
2 Intestinal flora in patients with PitNETs
We reviewed all the English literature up to October 2023 and found a total of 5 studies exploring the relationship between gut microbiota and PitNETs (7–10, 21). Of these five studies, four of the main studies were in patients with somatotropinoma, while Hu, Et al. described the microbiota of patients with invasive/non-invasive tumors and revealed the difference in microbiome composition between the two groups (Table 1). In these studies, the authors all described the intestinal microbiota composition of patients with PitNETs, revealing that the diversity of intestinal microbiota in PitNETs patients is different from that in the normal population. The richness and diversity of intestinal microbiota species can reflect the health or disease status of the human body and can even be used as predictors of disease prediction (22, 23). Lin et al. used a resume random forest classifier model to distinguish patients with Growth hormone (GH)-secreting pituitary tumor from healthy controls based on gut microbiota composition (9). Serdar et al. successfully distinguished individuals with abnormally high levels of insulin-like growth factor-1 (IGF-1) and accurately identified patients with acromegaly by using a machine learning model using microbiome composition (7). Hu, J. et al. established a classification model and identified 10 species that could be used to predict the occurrence of PitNETs, including Oscillibacter sp. 57_20, Fusobacterium mortiferum, and Clostridium innocuum (10). With the development of metagenomic sequencing and the expansion of sample sizes, for example, as the gut microbiome is an early predictor of disease in other types of diseases, this same effect may be applied to patients with PitNETs (24, 25). This allows for earlier diagnosis and treatment. Regrettably, only one of these studies, involving animal experiments, made a preliminary exploration of the relationship between gut microbiota and PitNETs but was limited to the observation of phenomena that didn’t reveal a possible pathway (8). At the same time, considering all literature reports, the description of intestinal flora composition in patients with different types of PitNETs remains to be explored by researchers. It is worth mentioning that Gavin et al. studied sphenoidal sinus flora in pituitary apoplexy patients and observed differences in the diversity of sphenoidal sinus flora between pituitary apoplexy patients and NF-PitNETs patients (26). Ye et al. recently sequenced 16SrRNA from PitNET tissues and speculated that the pathogenesis and development of tumors may be related to the behavior of bacteria in tumors (27). These studies mean that the microbes associated with PitNETs may not be limited to the gut, and future prospective studies are warranted.
3 Gut microbiota and the gut-brain axis
The known gut microbiota in healthy people is not less than 1000 species, with more than 3 million genes (28). Similar to a fingerprint, each individual has its unique gut microbiota as determined by host genotype, initial colonization by vertical transmission at birth, and dietary habits (29–31). At the same time, age, living environment, and the use of antibiotics can affect the structure of intestinal microbiota (22). For decades, it has been studied scientifically as a key factor in the maintenance of human health and the mechanism of disease occurrence and development and has been confirmed in numerous studies (32, 33). So far, studies on gut microbiota and disease have been divided into several mainstream models (Figure 1). First, researchers sought to characterize changes in the composition and distribution of the gut microbiota in disease states. For example, Birol et al. described differences in gut microbiota composition between patients with idiopathic focal epilepsy and healthy volunteers (34). As sequencing methods have updated (from 16SrRNA to metagenomics), researchers have moved from a genus level of the gut microbiota to a more precise species level providing a basis for further exploration (35). The second model is to conduct animal experiments after fecal microbiota transplantation (FMT) based on describing the differences in intestinal microbiota composition between the disease group and the healthy group, to explore the influence of intestinal microbiota on the body. The study of Li et al. can serve as a typical case (36). First, they described differences in the composition of the gut microbiota between patients with unruptured intracranial aneurysms (UIA) and healthy people, then fed a mixture of fecal bacteria from both groups to mice that had undergone aneurysmal induction surgery, and finally found a significant increase in aneurysm rupture in mice fed stool from patients with UIA. Furthermore, they finally isolated Hungatella hathewayi and introduced taurine as an intermediate substance, suggesting that supplementation with Hungatella hathewayi and taurine could prevent the development of UIAs (36). Such studies of isolating individual strains have gradually become mainstream and are a key step in further exploring the impact of gut microbiota on disease. In addition, the researchers also fed the experimental animals a mix of antibiotics to achieve the consumption of intestinal microbiota and compared the experimental result of intestinal flora consumption and non-consumption, to illustrate the impact of intestinal microbiota on disease or physiological status (37). These three models cover most of the reported studies and are important ways to explore the relationship between gut microbiota and the human body.
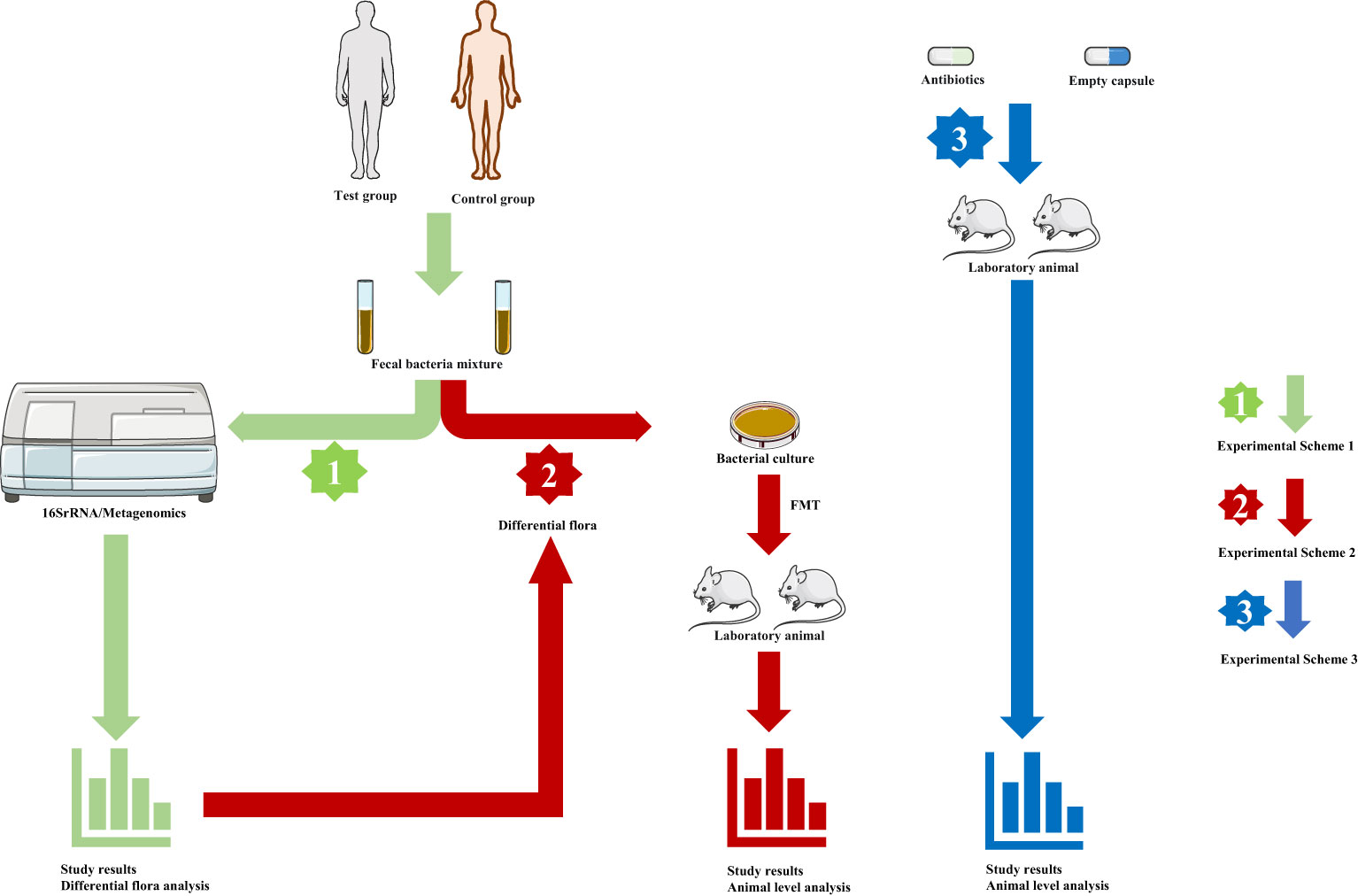
Figure 1 The main models of gut microbiota research. (Green arrow, experimental scheme 1, human fecal samples were collected and sequenced to analyze the characteristics of intestinal microbial composition and metabolism analysis. Red arrow, experimental scheme 2, based on experiment scheme 1, fecal bacteria were transplanted into experimental animals, and the corresponding index changes were observed at the animal level fecal. Blue arrow, experimental scheme 3, Depletion of gut microbes through antibiotics, and studies were conducted in normal and germ-free animals antibiotics.).
As more and more research has been devoted to the gut microbiome, its interaction with the brain has come into focus in neuroscience (38). Robust evidence shows that gut microbes are linked to the central nervous system through at least three mechanisms: neural, endocrine, and immune signaling (39). Recently, Needham et al. reported that the gut microbial metabolite 4-ethylphenol (4EP) can enter the mouse brain after sulfation to 4-ethylphenol (4EPS), disrupting oligodendrocyte and myelination pattern maturation in the brain, and increasing anxiety-like behavior in mice (40). Javier et al. took a different approach, they found that Lactobacillus rhamnosus (JB-1) increased the expression of gamma-aminobutyric acid (GABA) receptors in the cerebral cortex of mice and had beneficial effects in the treatment of depression and anxiety, while subphrenic vagotomy prevented the antianxiety and antidepressant effects of Lactobacillus and GABA changes (41). In studies involving brain tumor diseases, Giuseppina et al. found that changes in gut microbiota caused by antibiotic treatment could affect the growth of glioma in mice, induce early damage to natural killer(NK)cells, and induce changes in microglial phenotype (42). On the other hand, changes in the nervous system can also cause changes in the gut microbiota (34, 43, 44). In conclusion, the most prominent communication pathways in the gut-brain axis are the vagus nerve, tryptophan metabolites, and microbial metabolites (14, 45). The communication network between the gut and the central nervous system is complex and needs to be explored.
4 Possible mechanisms linking gut microbiota to PitNETs
4.1 Endocrine system
The HPA axis is the most interesting part of the gut-brain axis when we want to explore the relationship between gut microbiota (46). Proper functioning of the HPA axis is essential for maintaining mental and physical health (47). When the function of the HPA axis is abnormal, it will affect the health status of the human body, and a large number of previous studies have proved that it is closely related to depression, bipolar disorder, depression, and other mental diseases (47, 48). In the initial stage of life, microorganisms have the function of regulating the stress response of the HPA axis (49). Social bias in germ-free (GF) and antibiotic-treated (ABX) mice have been reported to be associated with increased levels of the stress hormone corticosterone, which is produced primarily by activation of the HPA axis, and these changes are primarily through changes in neural activity rather than neural circuitry, whereas Enterococcus faecalis promotes social activity and reduces corticosterone levels in mice after social stress (16). Patients with Cushing’s disease and acromegalyhave a varying prevalence of psychiatric disorders, according to a systematic literature review of neuropsychological conditions in patients with PitNETs (50, 51). In addition, prolactinoma patients had a higher frequency of depressive symptoms, anxiety, and feelings of hostility (52, 53). This is due to the pituitary tumor itself, treatment, and/or long-term effects of hormonal changes on the hypothalamic-pituitary terminal organ axis (54). Especially in Cushing’s disease, the onset of psychiatric symptoms may be caused by changes in cortisol levels in the body (55). Excessive cortisol secretion, as an indicator of abnormal HPA axis function, can aggravate the symptoms of mental disorders (56). Numerous preclinical studies have demonstrated that colonization of certain types of bacteria can improve psychiatric symptoms (57–59). For example, Bifidobacterium infantile has been shown to improve behavioral deficits and restore basal norepinephrine (NA) concentrations (60). Messaoudi et al. showed that treatment with Lactobacillus helveticus R0052 and Bifidobacterium longum R0175 improved mental status and reduced cortisol levels (61). Therefore, whether gut microbes can improve the mental status of patients with PitNETs and improve hormone secretion in patients with functional PitNETs is an interesting entry point, and the HPA axis may be the key. At the same time, whether abnormal hormone secretion of functional PitNETs and gut microbiota can be linked through the HPA axis is the next research focus.
Somatotropinoma is characterized by excessive secretion of GH and increased circulatingIGF-1 concentration (62). The studies mentioned above have confirmed that the gut microbiota composition of patients with somatotropinoma is specific and correlated with GH/IGF-1 levels (7, 9). In animal studies, the researchers found whether GH or IGF-1 levels were reduced in GF mice (63–65). In turn, supplementation of GF mice or suckling piglets with gut microbiota caused corresponding increases in GH and IGF-1 levels (66, 67). Either the change of GH/IGF-1 and intestinal microbiota will lead to the change on the other side (68). It is reasonable to believe that the association between somatotropinoma and intestinal microbiota is related to the change in GH/IGF-1 level.
Intestinal microbiota performs various physiological functions in thyroxine metabolism (69, 70). Gut microbiota can directly affect thyroid hormone levels through its deiodinase activity and thyroid-stimulating hormone inhibition (71). It can also indirectly affect thyroid hormone synthesis by affecting iodine absorption (71). Therefore, it is worth exploring whether there is a relationship between the inappropriate secretion of thyroid-stimulating hormone in thyrotropinoma patients and intestinal flora.
The use of Ames mice provides an animal model lacking several pituitary hormones, including GH, thyroid-stimulating hormone, and prolactin(PRL) (72). The study by Denise et al. showed a change in gut microbiota composition in Ames mice compared to controls (72). Patients with PitNETs are likely to have endocrine dysfunction before or after surgery, manifested by the disturbance of one or more hormones (1, 73). What is the effect of this on the gut microbiota of these patients?
4.2 Immune system
The presence of gut microbiota can promote the development of the innate immune system (74). For instance, the gut microbiota is involved in the maturation of innate lymphocytes (ILCs) (75). Furthermore, a large number of literature has shown the presence of intestinal flora in both humoral and cellular immunity (76, 77). Takeshi et al. reported that 11 microbiota, including Parabacteroides distasonis and Parabacteroides Johnsonii, could co-induce CD8+T cell expression in mice and effectively inhibit tumor growth (78). Intestinal microbiota can promote the induction of immunoglobulin A (IgA) B cells and plasma cell differentiation (79). Reliable studies have also demonstrated that gut microbiota can affect the levels of immune effectors such as interleukin (IL), interferon (IFN), and tumor necrosis factor (TNF), and participate in the process of disease occurrence or development (80–84). In PitNETs, the distribution of immune cells varies among subtypes. Studies showed that macrophages, T lymphocytes, and other immune cells were more infiltrated in functional PitNETs than in NF-PitNETs (85, 86). In a study of pituitary tumors, researchers found that IFN-α significantly inhibited the secretion of functional pituitary adenoma hormones (87). TNF-α in invasive pituitary adenoma can promote pathological osteoclast formation by directly inducing osteoclast differentiation, leading to inflammatory bone destruction (88). Qiu et al. reported that serum levels of IL-4, IL-5, and IL-17 were significantly increased in patients with pituitary adenoma, and IL-17 may be an important marker related to tumor invasiveness (89). In our previous study, it was demonstrated that the gut microbiota of patients with somatotropinoma can affect the immune indexes of tumor mouse models, which is that intestinal microbes from patients with somatotropinoma promoted the growth of subcutaneous tumors in mice and up-regulated the number of programmed cell death-ligand 1 (PD-L1) positive cells in tumor tissue (8). In other types of brain tumors, gut microbiota can also affect tumors through the immune system (42). In addition, in a hot area of research called immune checkpoints, Kemeny et al. used anti-PD-L1 treatment to successfully reduce plasma adrenoceptor ticotropic hormore (ACTH) levels, delay tumor growth, and improve mouse survival in a model of Cushing’s disease (19). A large body of research evidence also suggests that there is a close relationship between gut microbiota and the response to immune checkpoint therapy. For example, bifidobacterium can enhance the therapeutic effect ofPD-L1 inhibitors on mouse melanoma, while enhancing dendritic cell function and CD8+T cell-mediated anti-tumor mechanisms (90).
4.3 Metabolism and metabolites
The gut microbiota is capable of producing and releasing active metabolites that act as signaling molecules in the brain-gut axis (91). Bacterial metabolites such as dopamine, serotonin/norepinephrine GABA, acetylcholine, and histamine can also act as neurotransmitters in the central nervous system (91, 92). Specifically, dopamine acts as both a neurotransmitter and a hormone in the hypothalamic-pituitary axis (93). First, chronic dopamine deficiency is associated with the formation of pituitary tumors and many sites in the dopamine-D2 –second receptor-second messenger pathway may be involved (94, 95). Second, dopamine binds to dopamine receptor type 2 (D2DR) in the anterior pituitary gland, thereby inhibiting hormone secretion and cell division in the anterior pituitary gland (93). Finally, dopamine agonists (DAs), by binding to D2DR, lead to the inhibition of hormone secretion and tumor shrinkage in different pituitary tumor tissue types (96). As another important substance, short-chain fatty acids (SCFAs) are produced by the gut microbiota during the fermentation of partial and non-digested polysaccharides (97). Wang et al. showed that SCFAs decreased cyclic adenosine monophosphate (cAMP) levels and subsequently protein kinase A(PKA) activity in the anterior pituitary cells of dairy cows. Inhibition of PKA activity decreased cyclic-AMP response binding protein (CREB) phosphorylation, which inhibited GH and PRL gene transcription (98). Furthermore, patients with PitNETs often have metabolic disorders associated with impaired glucose tolerance including insulin sensitivity (99–101). A variety of gut microbes regulate insulin sensitivity, like Firmicutes, which can produce butyrate and increases insulin sensitivity (102, 103). At the same time, microbial metabolites are usually associated with immunity, hormone levels, and so on, which is a complex mechanism of joint action (104–107).
5 Gut microbes and other neuroendocrine tumors
Neuroendocrine tumor (NET) is a heterogeneous group of tumors originating from different neuroendocrine organs or cells (108). In addition to PitNETs, the NETs in other organs have also been linked to gut microbes (109). By studying stool samples from 18 patients with rectal neuroendocrine tumor (RNET) and 40 controls, Hu et al. found that patients with RNET had aberrant depletion and attenuated connection. Finally, they suggest that this disordered ecological structure may contribute to the disease-causing process of this tumor (110). In gastroenteropancreatic neuroendocrine tumors (GEP-NETs), the researchers found a significant decrease in bacterial species and an increase in fungi in the patients’ gut microbes. These changes may participate in the disease process by influencing the tumor microenvironment (111). Similar to the study of PitNETs, although little has been reported so far, the relationship between gut microbes and NETs has gradually become a focus of researchers and the tumor microenvironment may be a key factor.
6 Conclusion
Patients with different types of PitNETs have their characteristics of intestinal microbial composition and can be distinguished by this characteristic. As a specific type of brain tumor, PitNETs are closely related to hormone secretion, metabolism, and the immune system. These factors are the mediators of the connection between the gut microbiota and the central nervous system, so, the link between PitNETs and gut microbes may be mediated by metabolites, hormones, and immune molecules. Thus, the future exploration of the relationship between the two parties is promising, such as whether intestinal microbes are involved in the occurrence and development of PitNETs, whether intestinal microbes can affect the clinical symptoms of PitNETs, and the influence of intestinal microbes on the immunotherapy of PitNETs, but it also means more work and challenges (Figure 2). According to current research, immunity and metabolism are perhaps the most important areas of concern. Expanding the number of cases and further studying the effect of intestinal microbes on tumors and its mechanism based on animal models may be the direction of further research.
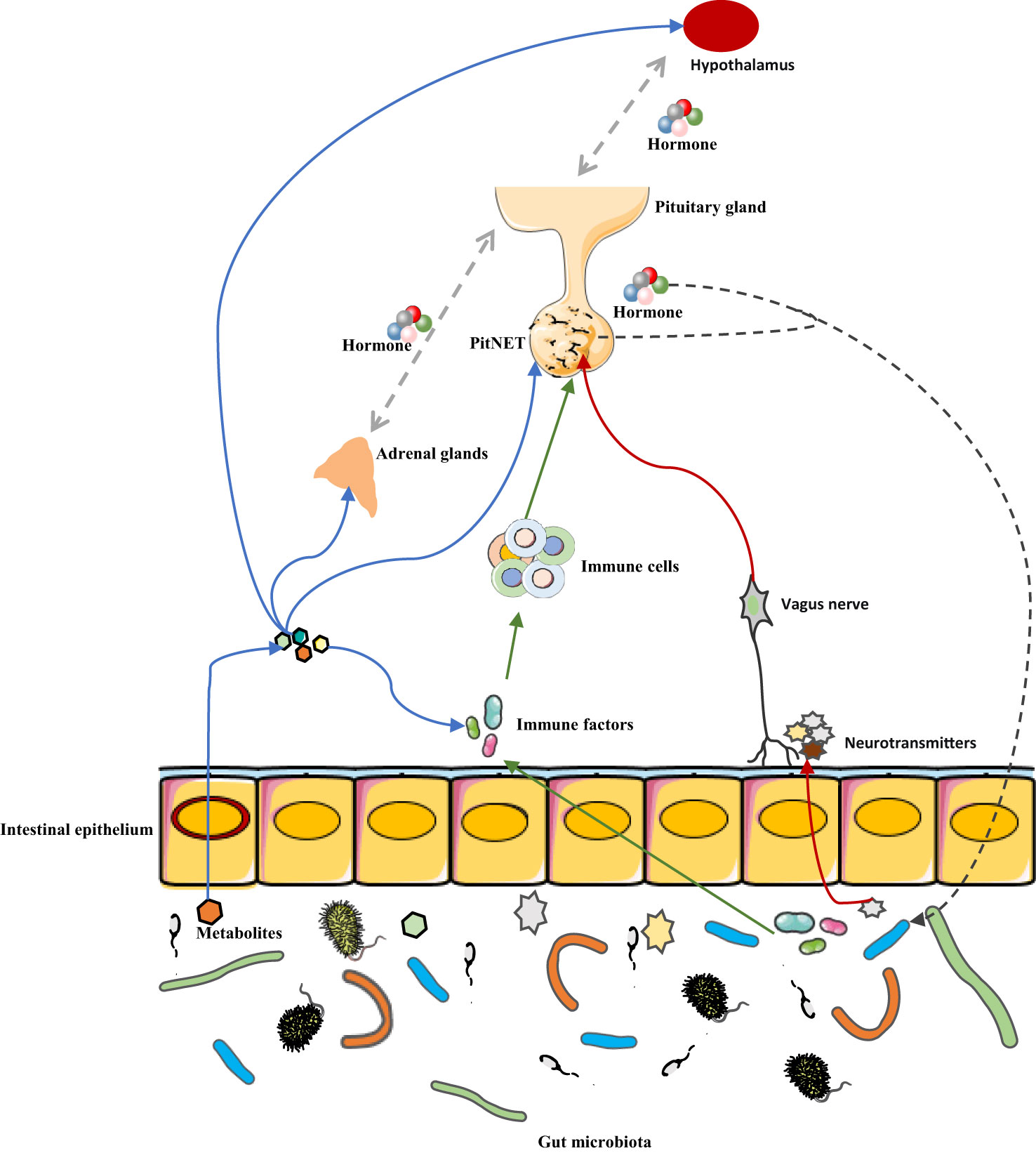
Figure 2 Potential mechanism of association between gut microbiota and PitNET. The interaction between gut microbes and PitNET may be mediated by immune factors, metabolites, hormone secretion, and neurotransmitters. Blue arrow, metabolites of gut microbes can not only affect PitNET by affecting immune factors, HPA axis hormone secretion, but also directly affect PitNET. Green arrow, gut microbes affect immune factors, which in turn affect immune cells in the tumor microenvironment, and ultimately PitNET. Red arrow, gut microbes release neurotransmitters that affect PitNET through the vagus pathway. Black arrow, PitNET, and the hormones it secretes can affect the composition or metabolism of gut microbes.
Author contributions
DN: Writing – original draft. CL: Resources, Writing – review & editing. YZ: Project administration, Writing – review & editing.
Conflict of interest
The authors declare that the research was conducted in the absence of any commercial or financial relationships that could be construed as a potential conflict of interest.
Publisher’s note
All claims expressed in this article are solely those of the authors and do not necessarily represent those of their affiliated organizations, or those of the publisher, the editors and the reviewers. Any product that may be evaluated in this article, or claim that may be made by its manufacturer, is not guaranteed or endorsed by the publisher.
References
1. Lake MG, Krook LS, Cruz SV. Pituitary adenomas: an overview. Am Fam Physician (2013) 88(5):319–27.
2. Asa SL, Mete O, Perry A, Osamura RY. Overview of the 2022 WHO classification of pituitary tumors. Endocr Pathol (2022) 33(1):6–26. doi: 10.1007/s12022-022-09703-7
3. Daly AF, Beckers A. The epidemiology of pituitary adenomas. Endocrinol Metab Clin North Am (2020) 49(3):347–55. doi: 10.1016/j.ecl.2020.04.002
4. Asa SL, Mete O, Cusimano MD, McCutcheon IE, Perry A, Yamada S, et al. Pituitary neuroendocrine tumors: a model for neuroendocrine tumor classification. Mod Pathol (2021) 34(9):1634–50. doi: 10.1038/s41379-021-00820-y
5. Melmed S, Kaiser UB, Lopes MB, Bertherat J, Syro LV, Raverot G, et al. Clinical biology of the pituitary adenoma. Endocr Rev (2022) 43(6):1003–37. doi: 10.1210/endrev/bnac010
6. Ilie MD, Lasolle H, Raverot G. Emerging and novel treatments for pituitary tumors. J Clin Med (2019) 8(8):1107. doi: 10.3390/jcm8081107
7. Sahin S, Gundogdu A, Nalbantoglu U, Kadioglu P, Karaca Z, Hacioglu A, et al. Acromegaly is associated with a distinct oral and gut microbiota. Pituitary (2022) 25(3):520–30. doi: 10.1007/s11102-022-01223-1
8. Nie D, Fang Q, Cheng J, Li B, Li M, Wang H, et al. The intestinal flora of patients with GHPA affects the growth and the expression of PD-L1 of tumor. Cancer Immunol Immunother (2022) 71(5):1233–45. doi: 10.1007/s00262-021-03080-6
9. Lin B, Wang M, Gao R, Ye Z, Yu Y, He W, et al. Characteristics of gut microbiota in patients with GH-secreting pituitary adenoma. Microbiol Spectr (2022) 10(1):e0042521. doi: 10.1128/spectrum.00425-21
10. Hu J, Yang J, Chen L, Meng X, Zhang X, Li W, et al. Alterations of the gut microbiome in patients with pituitary adenoma. Pathol Oncol Res (2022) 28:1610402. doi: 10.3389/pore.2022.1610402
11. Ma Q, Li Y, Li P, Wang M, Wang J, Tang Z, et al. Research progress in the relationship between type 2 diabetes mellitus and intestinal flora. BioMed Pharmacother (2019) 117:109138. doi: 10.1016/j.biopha.2019.109138
12. Bercik P, Collins SM, Verdu EF. Microbes and the gut-brain axis. Neurogastroenterol Motil (2012) 24(5):405–13. doi: 10.1111/j.1365-2982.2012.01906.x
13. Jin M, Qian Z, Yin J, Xu W, Zhou X. The role of intestinal microbiota in cardiovascular disease. J Cell Mol Med (2019) 23(4):2343–50. doi: 10.1111/jcmm.14195
14. Socała K, Doboszewska U, Szopa A, Serefko A, Włodarczyk M, Zielińska A, et al. The role of microbiota-gut-brain axis in neuropsychiatric and neurological disorders. Pharmacol Res (2021) 172:105840. doi: 10.1016/j.phrs.2021.105840
15. Cryan JF, ORiordan KJ, Cowan CSM, Sandhu KV, Bastiaanssen TFS, Boehme M, et al. The microbiota-gut-brain axis. Physiol Rev (2019) 99(4):1877–2013. doi: 10.1152/physrev.00018.2018
16. Wu WL, Adame MD, Liou CW, Barlow JT, Lai TT, Sharon G, et al. Microbiota regulate social behaviour via stress response neurons in the brain. Nature (2021) 595(7867):409–14. doi: 10.1038/s41586-021-03669-y
17. Makris AP, Karianaki M, Tsamis KI, Paschou SA. The role of the gut-brain axis in depression: endocrine, neural, and immune pathways. Hormones (Athens) (2021) 20(1):1–12. doi: 10.1007/s42000-020-00236-4
18. Park EM, Chelvanambi M, Bhutiani N, Kroemer G, Zitvogel L, Wargo JA. Targeting the gut and tumor microbiota in cancer. Nat Med (2022) 28(4):690–703. doi: 10.1038/s41591-022-01779-2
19. Kemeny HR, Elsamadicy AA, Farber SH, Champion CD, Lorrey SJ, Chongsathidkiet P, et al. Targeting PD-L1 initiates effective antitumor immunity in a murine model of cushing disease. Clin Cancer Res (2020) 26(5):1141–51. doi: 10.1158/1078-0432.CCR-18-3486
20. Liu RT, Walsh RFL, Sheehan AE. Prebiotics and probiotics for depression and anxiety: A systematic review and meta-analysis of controlled clinical trials. Neurosci Biobehav Rev (2019) 102:13–23. doi: 10.1016/j.neubiorev.2019.03.023
21. Hacioglu A, Gundogdu A, Nalbantoglu U, Karaca Z, Urhan ME, Sahin S, et al. Gut microbiota in patients with newly diagnosed acromegaly: a pilot cross-sectional study. Pituitary (2021) 24(4):600–10. doi: 10.1007/s11102-021-01137-4
22. Hollister EB, Gao C, Versalovic J. Compositional and functional features of the gastrointestinal microbiome and their effects on human health. Gastroenterology (2014) 146(6):1449–58. doi: 10.1053/j.gastro.2014.01.052
23. Duan M, Han Z, Huang N. Changes of intestinal microflora in neonatal necrotizing enterocolitis: a single-center study. J Int Med Res (2020) 48(9):300060520957804. doi: 10.1177/0300060520957804
24. Liu Y, Méric G, Havulinna AS, Teo SM, Åberg F, Ruuskanen M, et al. Early prediction of incident liver disease using conventional risk factors and gut-microbiome-augmented gradient boosting. Cell Metab (2022) 34(5):719–730.e4. doi: 10.1016/j.cmet.2022.03.002
25. Loomba R, Seguritan V, Li W, Long T, Klitgord N, Bhatt A, et al. Gut microbiome-based metagenomic signature for non-invasive detection of advanced fibrosis in human nonalcoholic fatty liver disease. Cell Metab (2017) 25(5):1054–1062.e5. doi: 10.1016/j.cmet.2017.04.001
26. Humphreys GJ, Waqar M, McBain AJ, Gnanalingham KK. Sphenoid sinus microbiota in pituitary apoplexy: a preliminary study. Pituitary (2017) 20(6):619–23. doi: 10.1007/s11102-017-0823-9
27. Ye L, Wu BS, Xu ZA, Ji XF, Guan L, Li PP, et al. Evidence for an intra-tumoral microbiome in pituitary neuroendocrine tumors with different clinical phenotypes. J Neurooncol (2023) 163(1):133–42. doi: 10.1007/s11060-023-04318-2
28. Clemente JC, Ursell LK, Parfrey LW, Knight R. The impact of the gut microbiota on human health: an integrative view. Cell (2012) 148(6):1258–70. doi: 10.1016/j.cell.2012.01.035
29. Conlon MA, Bird AR. The impact of diet and lifestyle on gut microbiota and human health. Nutrients (2014) 7(1):17–44. doi: 10.3390/nu7010017
30. Spor A, Koren O, Ley R. Unravelling the effects of the environment and host genotype on the gut microbiome. Nat Rev Microbiol (2011) 9(4):279–90. doi: 10.1038/nrmicro2540
31. Chu DM, Ma J, Prince AL, Antony KM, Seferovic MD, Aagaard KM. Maturation of the infant microbiome community structure and function across multiple body sites and in relation to mode of delivery. Nat Med (2017) 23(3):314–26. doi: 10.1038/nm.4272
32. Lavelle A, Sokol H. Gut microbiota-derived metabolites as key actors in inflammatory bowel disease. Nat Rev Gastroenterol Hepatol (2020) 17(4):223–37. doi: 10.1038/s41575-019-0258-z
33. Mitrea L, Nemeş SA, Szabo K, Teleky BE, Vodnar DC. Guts imbalance imbalances the brain: A review of gut microbiota association with neurological and psychiatric disorders. Front Med (Lausanne) (2022) 9:813204. doi: 10.3389/fmed.2022.813204
34. Şafak B, Altunan B, Topçu B, Eren Topkaya A. The gut microbiome in epilepsy. Microbial Pathogenesis (2020) 139:103853. doi: 10.1016/j.micpath.2019.103853
35. Shahab M, Shahab N. Coevolution of the human host and gut microbiome: metagenomics of microbiota. Cureus (2022) 14(6):e26310. doi: 10.7759/cureus.26310
36. Li H, Xu H, Li Y, Jiang Y, Hu Y, Liu T, et al. Alterations of gut microbiota contribute to the progression of unruptured intracranial aneurysms. Nat Commun (2020) 11(1):3218. doi: 10.1038/s41467-020-16990-3
37. Shikata F, Shimada K, Sato H, Ikedo T, Kuwabara A, Furukawa H, et al. Potential influences of gut microbiota on the formation of intracranial aneurysm. Hypertension (2019) 73(2):491–6. doi: 10.1161/HYPERTENSIONAHA.118.11804
38. Wang HX, Wang YP. Gut microbiota-brain axis. Chin Med J (Engl) (2016) 129(19):2373–80. doi: 10.4103/0366-6999.190667
39. Martin CR, Osadchiy V, Kalani A, Mayer EA. The brain-gut-microbiome axis. Cell Mol Gastroenterol Hepatol (2018) 6(2):133–48. doi: 10.1016/j.jcmgh.2018.04.003
40. Needham BD, Funabashi M, Adame MD, Wang Z, Boktor JC, Haney J, et al. A gut-derived metabolite alters brain activity and anxiety behaviour in mice. Nature (2022) 602(7898):647–53. doi: 10.1038/s41586-022-04396-8
41. Bravo JA, Forsythe P, Chew MV, Escaravage E, Savignac HM, Dinan TG, et al. Ingestion of Lactobacillus strain regulates emotional behavior and central GABA receptor expression in a mouse via the vagus nerve. Proc Natl Acad Sci U.S.A. (2011) 108(38):16050–5. doi: 10.1073/pnas.1102999108
42. D’Alessandro G, Antonangeli F, Marrocco F, Porzia A, Lauro C, Santoni A, et al. Gut microbiota alterations affect glioma growth and innate immune cells involved in tumor immunosurveillance in mice. Eur J Immunol (2020) 50(5):705–11. doi: 10.1002/eji.201948354
43. Kang Y, Yang Y, Wang J, Ma Y, Cheng H, Wan D. Correlation between intestinal flora and serum inflammatory factors in post-stroke depression in ischemic stroke. J Coll Physicians Surg Pak (2021) 31(10):1224–7. doi: 10.29271/jcpsp.2021.10.1224
44. Geng H, Shu S, Dong J, Li H, Xu C, Han Y, et al. Association study of gut flora in Wilson’s disease through high-throughput sequencing. Med (Baltimore) (2018) 97(31):e11743.
45. Forsythe P, Bienenstock J, Kunze WA. Vagal pathways for microbiome-brain-gut axis communication. In: Lyte M, Cryan JF, editors. Microbial endocrinology: the microbiota-gut-brain axis in health and disease. New York, NY: Springer New York (2014). p. 115–33.
46. Misiak B, Łoniewski I, Marlicz W, Frydecka D, Szulc A, Rudzki L, et al. The HPA axis dysregulation in severe mental illness: Can we shift the blame to gut microbiota? Prog Neuropsychopharmacol Biol Psychiatry (2020) 102:109951.
47. Leistner C, Menke A. Hypothalamic-pituitary-adrenal axis and stress. Handb Clin Neurol (2020) 175:55–64. doi: 10.1016/B978-0-444-64123-6.00004-7
48. Tafet GE, Nemeroff CB. Pharmacological treatment of anxiety disorders: the role of the HPA axis. Front Psychiatry (2020) 11:443.
49. Sudo N, Chida Y, Aiba Y, Sonoda J, Oyama N, Yu XN, et al. Postnatal microbial colonization programs the hypothalamic-pituitary-adrenal system for stress response in mice. J Physiol (2004) 558(Pt 1):263–75. doi: 10.3389/fpsyt.2020.00443
50. Pertichetti M, Serioli S, Belotti F, Mattavelli D, Schreiber A, Cappelli C, et al. Pituitary adenomas and neuropsychological status: a systematic literature review. Neurosurgical Rev (2020) 43(4):1065–78. doi: 10.1113/jphysiol.2004.063388
51. Gareau MG, Jury J, MacQueen G, Sherman PM, Perdue MH. Probiotic treatment of rat pups normalises corticosterone release and ameliorates colonic dysfunction induced by maternal separation. Gut (2007) 56(11):1522–8. doi: 10.1007/s10143-019-01134-z
52. Ezzat S. Living with acromegaly. Endocrinol Metab Clin North Am (1992) 21(3):753–60. doi: 10.1136/gut.2006.117176
53. Vale JM. Hyperprolactinemia and major depression. Am J Psychiatry (1991) 148(4):547–8. doi: 10.1016/S0889-8529(18)30212-3
54. Weitzner MA, Kanfer S, Booth-Jones M. Apathy and pituitary disease: it has nothing to do with depression. J Neuropsychiatry Clin Neurosci (2005) 17(2):159–66. doi: 10.1176/ajp.148.4.547
55. Marsh L, Guinan E, Shah E, Powell M, Lowy C, Kopelman MD. A prospective study of the cognitive and psychiatric effects of pituitary tumours and their treatments. J Clin Neurosci (2020) 75:122–7. doi: 10.1176/jnp.17.2.159
56. Yang F, Cao X, Sun X, Wen H, Qiu J, Xiao H, et al. Hair cortisol is associated with social support and symptoms in schizophrenia. Front Psychiatry (2020) 11:572656. doi: 10.1016/j.jocn.2020.03.007
57. Huang L, Lv X, Ze X, Ma Z, Zhang X, He R, et al. Combined probiotics attenuate chronic unpredictable mild stress-induced depressive-like and anxiety-like behaviors in rats. Front Psychiatry (2022) 13:990465. doi: 10.3389/fpsyt.2020.572656
58. Zheng P, Zeng B, Zhou C, Liu M, Fang Z, Xu X, et al. Gut microbiome remodeling induces depressive-like behaviors through a pathway mediated by the host’s metabolism. Mol Psychiatry (2016) 21(6):786–96. doi: 10.3389/fpsyt.2022.990465
59. Mika A, Day HE, Martinez A, Rumian NL, Greenwood BN, Chichlowski M, et al. Early life diets with prebiotics and bioactive milk fractions attenuate the impact of stress on learned helplessness behaviours and alter gene expression within neural circuits important for stress resistance. Eur J Neurosci (2017) 45(3):342–57. doi: 10.1038/mp.2016.44
60. Desbonnet L, Garrett L, Clarke G, Kiely B, Cryan JF, Dinan TG. Effects of the probiotic Bifidobacterium infantis in the maternal separation model of depression. Neuroscience (2010) 170(4):1179–88. doi: 10.1111/ejn.13444
61. Messaoudi M, Lalonde R, Violle N, Javelot H, Desor D, Nejdi A, et al. Assessment of psychotropic-like properties of a probiotic formulation (Lactobacillus helveticus R0052 and Bifidobacterium longum R0175) in rats and human subjects. Br J Nutr (2011) 105(5):755–64. doi: 10.1016/j.neuroscience.2010.08.005
62. Dineen R, Stewart PM, Sherlock M. Acromegaly. Qjm (2017) 110(7):411–20. doi: 10.1017/S0007114510004319
63. Weger BD, Gobet C, Yeung J, Martin E, Jimenez S, Betrisey B, et al. The mouse microbiome is required for sex-specific diurnal rhythms of gene expression and metabolism. Cell Metab (2019) 29(2):362–382.e8.
64. Schwarzer M, Makki K, Storelli G, Machuca-Gayet I, Srutkova D, Hermanova P, et al. Lactobacillus plantarum strain maintains growth of infant mice during chronic undernutrition. Science (2016) 351(6275):854–7. doi: 10.1016/j.cmet.2018.09.023
65. Yan J, Herzog JW, Tsang K, Brennan CA, Bower MA, Garrett WS, et al. Gut microbiota induce IGF-1 and promote bone formation and growth. Proc Natl Acad Sci U.S.A. (2016) 113(47):E7554–e7563. doi: 10.1126/science.aad8588
66. Cheng CS, Wei HK, Wang P, Yu HC, Zhang XM, Jiang SW, et al. Early intervention with faecal microbiota transplantation: an effective means to improve growth performance and the intestinal development of suckling piglets. Animal (2019) 13(3):533–41. doi: 10.1073/pnas.1607235113
67. Lu J, Lu L, Yu Y, Cluette-Brown J, Martin CR and Claud EC L, Yu Y, Cluette-Brown J, Martin CR, Claud EC. Effects of intestinal microbiota on brain development in humanized gnotobiotic mice. Sci Rep (2018) 8(1):5443. doi: 10.1017/S1751731118001611
68. Jensen EA, Young JA, Mathes SC, List EO, Carroll RK, Kuhn J, et al. Crosstalk between the growth hormone/insulin-like growth factor-1 axis and the gut microbiome: A new frontier for microbial endocrinology. Growth Horm IGF Res (2020) 53-54:101333. doi: 10.1038/s41598-018-23692-w
69. Yao Z, Zhao M, Gong Y, Chen W, Wang Q, Fu Y, et al. Relation of gut microbes and L-thyroxine through altered thyroxine metabolism in subclinical hypothyroidism subjects. Front Cell Infect Microbiol (2020) 10:495. doi: 10.1016/j.ghir.2020.101333
70. Hazenberg MP, de Herder WW, Visser TJ. Hydrolysis of iodothyronine conjugates by intestinal bacteria. FEMS Microbiol Rev (1988) 4(1):9–16. doi: 10.3389/fcimb.2020.00495
71. Knezevic J, Starchl C, Tmava Berisha A, Amrein K. Thyroid-gut-axis: how does the microbiota influence thyroid function? Nutrients (2020) 12(6):1769. doi: 10.3390/nu12061769
72. Wiesenborn DS, Gálvez EJC, Spinel L, Victoria B, Allen B, Schneider A, et al. The role of ames dwarfism and calorie restriction on gut microbiota. J Gerontol A Biol Sci Med Sci (2020) 75(7):e1–8. doi: 10.3390/nu12061769
73. Melmed S. Pituitary-tumor endocrinopathies. N Engl J Med (2020) 382(10):937–50. doi: 10.1093/gerona/glz236
74. Ganal-Vonarburg SC, Duerr CU. The interaction of intestinal microbiota and innate lymphoid cells in health and disease throughout life. Immunology (2020) 159(1):39–51. doi: 10.1056/NEJMra1810772
75. Gury-BenAri M, Thaiss CA, Serafini N, Winter DR, Giladi A, Lara-Astiaso D, et al. The spectrum and regulatory landscape of intestinal innate lymphoid cells are shaped by the microbiome. Cell (2016) 166(5):1231–1246.e13. doi: 10.1111/imm.13138
76. Yu B, Wang L, Chu Y. Gut microbiota shape B cell in health and disease settings. J Leukoc Biol (2021) 110(2):271–81. doi: 10.1016/j.cell.2016.07.043
77. Honda K, Littman DR. The microbiota in adaptive immune homeostasis and disease. Nature (2016) 535(7610):75–84. doi: 10.1002/JLB.1MR0321-660R
78. Tanoue T, Morita S, Plichta DR, Skelly AN, Suda W, Sugiura Y, et al. A defined commensal consortium elicits CD8 T cells and anti-cancer immunity. Nature (2019) 565(7741):600–5. doi: 10.1038/nature18848
79. Lo BC, Chen GY, Núñez G, Caruso R. Gut microbiota and systemic immunity in health and disease. Int Immunol (2021) 33(4):197–209. doi: 10.1038/s41586-019-0878-z
80. Sanz Y, Moya-Pérez A. Microbiota, inflammation and obesity. Adv Exp Med Biol (2014) 817:291–317. doi: 10.1093/intimm/dxaa079
81. Qi X, Yun C, Sun L, Xia J, Wu Q, Wang Y, et al. Gut microbiota-bile acid-interleukin-22 axis orchestrates polycystic ovary syndrome. Nat Med (2019) 25(8):1225–33. doi: 10.1007/978-1-4939-0897-4_14
82. Martínez-López M, Iborra S, Conde-Garrosa R, Mastrangelo A, Danne C, Mann ER, et al. Microbiota sensing by mincle-syk axis in dendritic cells regulates interleukin-17 and -22 production and promotes intestinal barrier integrity. Immunity (2019) 50(2):446–461.e9. doi: 10.1038/s41591-019-0509-0
83. Aden K, Rehman A, Waschina S, Pan WH, Walker A, Lucio M, et al. Metabolic functions of gut microbes associate with efficacy of tumor necrosis factor antagonists in patients with inflammatory bowel diseases. Gastroenterology (2019) 157(5):1279–1292.e11. doi: 10.1016/j.immuni.2018.12.020
84. Sun MF, Zhu YL, Zhou ZL, Jia XB, Xu YD, Yang Q, et al. Neuroprotective effects of fecal microbiota transplantation on MPTP-induced Parkinson’s disease mice: Gut microbiota, glial reaction and TLR4/TNF-α signaling pathway. Brain Behav Immun (2018) 70:48–60. doi: 10.1053/j.gastro.2019.07.025
85. Iacovazzo D, Chiloiro S, Carlsen E, Bianchi A, Giampietro A, Tartaglione T, et al. Tumour-infiltrating cytotoxic T lymphocytes in somatotroph pituitary neuroendocrine tumours. Endocrine (2020) 67(3):651–8. doi: 10.1016/j.bbi.2018.02.005
86. Zhou W, Zhang C, Zhang D, Peng J, Ma S, Wang X, et al. Comprehensive analysis of the immunological landscape of pituitary adenomas: implications of immunotherapy for pituitary adenomas. J Neurooncol (2020) 149(3):473–87. doi: 10.1007/s12020-019-02145-y
87. Hofland LJ, de Herder WW, Waaijers M, Zuijderwijk J, Uitterlinden P, van Koetsveld PM, et al. Interferon-alpha-2a is a potent inhibitor of hormone secretion by cultured human pituitary adenomas. J Clin Endocrinol Metab (1999) 84(9):3336–43. doi: 10.1007/s11060-020-03636-z
88. Zhu H, Guo J, Shen Y, Dong W, Gao H, Miao Y, et al. Functions and mechanisms of tumor necrosis factor-α and noncoding RNAs in bone-invasive pituitary adenomas. Clin Cancer Res (2018) 24(22):5757–66.
89. Qiu L, Yang J, Wang H, Zhu Y, Wang Y, Wu Q. Expression of T-helper-associated cytokines in the serum of pituitary adenoma patients preoperatively and postperatively. Med Hypotheses (2013) 80(6):781–6. doi: 10.1158/1078-0432.CCR-18-0472
90. Sivan A, Corrales L, Hubert N, Williams JB, Aquino-Michaels K, Earley ZM, et al. Commensal Bifidobacterium promotes antitumor immunity and facilitates anti-PD-L1 efficacy. Science (2015) 350(6264):1084–9. doi: 10.1016/j.mehy.2013.03.011
91. Doroszkiewicz J, Groblewska M, Mroczko B. The role of gut microbiota and gut-brain interplay in selected diseases of the central nervous system. Int J Mol Sci (2021) 22(18):10028. doi: 10.3390/ijms221810028
92. Strandwitz P. Neurotransmitter modulation by the gut microbiota. Brain Res (2018) 1693(Pt B):128–33. doi: 10.3390/ijms221810028
93. Wood DF, Johnston JM, Johnston DG. Dopamine, the dopamine D2 receptor and pituitary tumours. Clin Endocrinol (Oxf) (1991) 35(6):455–66. doi: 10.1016/j.brainres.2018.03.015
94. Hofland LJ, Feelders RA, de Herder WW, Lamberts SW. Pituitary tumours: the sst/D2 receptors as molecular targets. Mol Cell Endocrinol (2010) 326(1-2):89–98. doi: 10.1111/j.1365-2265.1991.tb00928.x
95. Liu YT, Liu F, Cao L, Xue L, Gu WT, Zheng YZ, et al. The KBTBD6/7-DRD2 axis regulates pituitary adenoma sensitivity to dopamine agonist treatment. Acta Neuropathol (2020) 140(3):377–96. doi: 10.1016/j.mce.2010.04.020
96. Pivonello C, Patalano R, Negri M, Pirchio R, Colao A, Pivonello R, et al. Resistance to dopamine agonists in pituitary tumors: molecular mechanisms. Front Endocrinol (Lausanne) (2021) 12:791633. doi: 10.1007/s00401-020-02180-4
97. Tan J, McKenzie C, Potamitis M, Thorburn AN, Mackay CR, Macia L, et al. The role of short-chain fatty acids in health and disease. Adv Immunol (2014) 121:91–119. doi: 10.3389/fendo.2021.791633
98. Wang JF, Fu SP, Li SN, Hu ZM, Xue WJ, Li ZQ, et al. Short-chain fatty acids inhibit growth hormone and prolactin gene transcription via cAMP/PKA/CREB signaling pathway in dairy cow anterior pituitary cells. Int J Mol Sci (2013) 14(11):21474–88. doi: 10.1016/B978-0-12-800100-4.00003-9
99. Baba N, Kameda H, Nakamura A, Yong Cho K, Nomoto H, Mitsuhashi T, et al. Silent pituitary adenoma and metabolic disorders: obesity, abnormal glucose tolerance, hypertension and dyslipidemia. Endocr J (2021) 68(2):195–200. doi: 10.3390/ijms141121474
100. Frederic C, Isabelle M, Bernard CD, Thierry B. Cushing’s disease. Orphanet J Rare Dis (2012) 7:41. doi: 10.1507/endocrj.EJ20-0185
101. Raverot G, Jouanneau E, Trouillas J. Management of endocrine disease: clinicopathological classification and molecular markers of pituitary tumours for personalized therapeutic strategies. Eur J Endocrinol (2014) 170(4):R121–32. doi: 10.1186/1750-1172-7-41
102. Fei N, Zhao L. An opportunistic pathogen isolated from the gut of an obese human causes obesity in germfree mice. Isme J (2013) 7(4):880–4. doi: 10.1530/EJE-13-1031
103. Säemann MD, Böhmig GA, Osterreicher CH, Burtscher H, Parolini O, Diakos C, et al. Anti-inflammatory effects of sodium butyrate on human monocytes: potent inhibition of IL-12 and up-regulation of IL-10 production. FASEB J (2000) 14(15):2380–2. doi: 10.1038/ismej.2012.153
104. Cani PD, Amar J, Iglesias MA, Poggi M, Knauf C, Bastelica D, et al. Metabolic endotoxemia initiates obesity and insulin resistance. Diabetes (2007) 56(7):1761–72. doi: 10.1096/fj.00-0359fje
105. Jia X, Xu W, Zhang L, Li X, Wang R, Wu S. Impact of gut microbiota and microbiota-related metabolites on hyperlipidemia. Front Cell Infect Microbiol (2021) 11:634780. doi: 10.2337/db06-1491
106. Debnath N, Kumar R, Kumar A, Mehta PK, Yadav AK. Gut-microbiota derived bioactive metabolites and their functions in host physiology. Biotechnol Genet Eng Rev (2021) 37(2):105–53. doi: 10.3389/fcimb.2021.634780
107. Westfall S, Caracci F, Zhao D, Wu QL, Frolinger T, Simon J, et al. Microbiota metabolites modulate the T helper 17 to regulatory T cell (Th17/Treg) imbalance promoting resilience to stress-induced anxiety- and depressive-like behaviors. Brain Behav Immun (2021) 91:350–68. doi: 10.1080/02648725.2021.1989847
108. Vitale G, Dicitore A, Barrea L, Sbardella E, Razzore P, Campione S, et al. From microbiota toward gastro-enteropancreatic neuroendocrine neoplasms: Are we on the highway to hell? Rev Endocr Metab Disord (2021) 22(3):511–25. doi: 10.1016/j.bbi.2020.10.013
109. Mohamed A, Asa SL, McCormick T, Al-Shakhshir H, Dasari A, Mauricio R, et al. The role of the microbiome in gastroentero-pancreatic neuroendocrine neoplasms (GEP-NENs). Curr Issues Mol Biol (2022) 44(5):2015–28. doi: 10.1007/s11154-021-09639-z
110. Hu W, Chen ZM, Li XX, Lu L, Yang GH, Lei ZX, et al. Faecal microbiome and metabolic signatures in rectal neuroendocrine tumors. Theranostics (2022) 12(5):2015–27. doi: 10.3390/cimb44050136
Keywords: intestinal flora, gut microbiota, pituitary neuroendocrine tumor (PitNET), gut-brain axis, pituitary tumor
Citation: Nie D, Li C and Zhang Y (2023) PitNETs and the gut microbiota: potential connections, future directions. Front. Endocrinol. 14:1255911. doi: 10.3389/fendo.2023.1255911
Received: 10 July 2023; Accepted: 20 October 2023;
Published: 06 November 2023.
Edited by:
Mian Guo, The Second Affiliated Hospital of Harbin Medical University, ChinaReviewed by:
Natalia Zeber-Lubecka, Medical Centre for Postgraduate Education, PolandTeleky Bernadette-Emoke, University of Agricultural Sciences and Veterinary Medicine of Cluj-Napoca, Romania
Copyright © 2023 Nie, Li and Zhang. This is an open-access article distributed under the terms of the Creative Commons Attribution License (CC BY). The use, distribution or reproduction in other forums is permitted, provided the original author(s) and the copyright owner(s) are credited and that the original publication in this journal is cited, in accordance with accepted academic practice. No use, distribution or reproduction is permitted which does not comply with these terms.
*Correspondence: Yazhuo Zhang, zyztxzz@126.com