- 1State Key Laboratory of Female Fertility Promotion, Center for Reproductive Medicine, Department of Obstetrics and Gynecology, Peking University Third Hospital, Beijing, China
- 2National Clinical Research Center for Obstetrics and Gynecology, Peking University Third Hospital, Beijing, China
- 3Key Laboratory of Assisted Reproduction, Peking University, Ministry of Education, Beijing, China
- 4Beijing Key Laboratory of Reproductive Endocrinology and Assisted Reproductive Technology, Beijing, China
- 5Department of Neurology, Peking University Third Hospital, Beijing, China
- 6Department of Medical Genetics, Center for Medical Genetics, Peking University Health Science Center, Beijing, China
Background: Recurrent pregnancy loss is a distressing event during pregnancy, and understanding its causal factors is crucial. Follistatin, a glycoprotein involved in folliculogenesis and embryogenesis, has been implicated as a potential contributor to the risk of spontaneous abortion. However, establishing a causal relationship requires rigorous investigation using robust methods.
Methods: In this study, we utilized mendelian randomization (MR), a powerful genetic epidemiological approach, to examine the causal relationship between follistatin levels and spontaneous abortion. We obtained instrumental variables strongly associated with follistatin levels from large-scale genome-wide association from the IEU database. The inverse variance weighting (IVW) method was taken as gold standard. We also performed sensitivity test to evaluate the robustness of our result.
Results: MR analysis revealed a significant causal relationship between low follistatin levels and spontaneous abortion (p = 0.03). Sensitivity analyses, including pleiotropy test, heterogeneity test, and leave-one-out analysis, all supported the robustness of our findings.
Conclusion: Our study provides compelling evidence supporting the causal relationship between low follistatin levels and increased risk of spontaneous abortion. These findings underscore the importance of follistatin in the etiology of spontaneous abortion and suggest potential preventive interventions. Modulating follistatin levels or relevant pathways could hold promise for reducing the incidence of spontaneous abortion and improving reproductive outcomes. The utilization of MRs strengthens the validity of our results by mitigating confounding and reverse causality biases. Further research is needed to elucidate the underlying molecular mechanisms and explore therapeutic strategies targeting follistatin levels.
1 Introduction
Spontaneous abortion is a frequently encountered complication during pregnancy, characterized by the loss of pregnancy before 20 weeks of gestation (1). Approximately 9-20% of all recognized pregnancies result in spontaneous abortion. Among these, 3-5% of couples face the challenge of two or more clinically recognized pregnancies ending in failure, known as recurrent pregnancy loss (RPL) (2, 3). RPL can be devastating, bringing great trauma to both the patient and their family (4). Various factors contribute to RPL, with chromosomal abnormality being the most common, responsible for over half of RPL cases (5). Additionally, 10%–15% of women with multiple pregnancy losses exhibit uterine anomalies, such as partial or complete septum (6, 7). Hormonal causes such as luteal phase defect, pregestational diabetes mellitus, and polycystic ovary disease can lead to RPL (8). Moreover, immune disorders may contribute to RPL by dysregulating trophoblast function and endometrial angiogenesis (9). For example, primary antiphospholipid syndrome (APS), present in one-third of RPL patients, is associated with elevated serum anti-phospholipid antibody (aPL) levels. Increased aPL levels reduce placental hormone production, impair trophoblast function, and result in pregnancy loss and other obstetric complications (10). Women with celiac disease often have elevated anti-transglutaminase type 2 (anti-TG2) autoantibodies, leading to reduced blood vessel formation and disrupted endometrial angiogenesis, contributing to RPL (11). Infections, exposure to environmental agents, and elevated homocysteine levels are also implicated in RPL (12). Nevertheless, the understanding of RPL remains significantly limited, as almost 50% of RPL cases are still categorized as unexplained (9).
Follistatin (FST) is a secreted protein that primarily synthesized and secreted by the liver, mainly implicated in suppressing follicle-stimulating hormone (FSH) activity through autocrine or paracrine mechanisms (13–15). Notably, FST serves as a binding protein and regulator in the transforming growth factor-beta (TGF-β) signaling pathway, selectively binding to ligands such as activins and bone morphogenetic proteins (BMP) (13, 16, 17). It restrained granulosa cell proliferation and steroidogenesis by neutralizing the action of activin (18–20). It also enhanced basal estradiol and progesterone production (21, 22), promoting cell invasion via the ALK4-SMAD2/3-SMAD4 signaling pathway (23–26).
Serum FST increased significantly throughout gestation until the first day of parturition and declined afterward (27). Evidence showed its possible role as chemokine to induce trophoblast migration and invasion through the enhanced JNK signaling, contributing to maintain trophoblast function and promote placental development (28, 29). FST was upregulated in the decidua during early pregnancy, and women with RPL were observed to have a lower endometrial expression of FST during the luteal phase (30). A lower FST level in endometrium stromal cells of women with RPL was also observed (30). Conditional knockout of mice uterine Fst can cause severe fertility defects, reduced responsiveness to estrogen and progesterone signals, impaired artificial decidualization, and an unreceptive environment for embryo attachment. These findings suggest that Fst may play a crucial role in facilitating uterine receptivity (31). A decreased FST level was also observed in serum and placenta of women with preeclampsia (PE) (32–34), resulting in impaired trophoblast function through upregulating GDF11 levels in trophoblasts. The dysregulation of the FST-GDF11-Smad2/3 axis may be critical to trophoblast function, which adds more evidence to the essential role of FST on trophoblast during pregnancy (35).
Mendelian randomization (MR) is an epidemiological tool based on genetic variants related to exposure factors, helping to assess the association of these gene variations with outcomes such as disease onset or mortality. Its core relies on using genetic data as a bridge to investigate causal relationships between a particular exposure and a specific outcome (36, 37). Randomized controlled trials (RCTs) have long been recognized as the gold standard for causal inference, yet it is costly and complicated to conduct. Similar to RCTs that randomly assign participants to a trial or control group, MR studies “randomize” participants based on one or more gene alleles that influence risk factors and attempt to determine if carriers of these genetic variations have different disease onset risks compared with non-carriers (38). Traditional observational study designs rely on exposure obtained through questionnaires, biochemical assays, or imaging, whereas genetic variations exist at birth and remain stable throughout life. Notably, information of genetic variation and diseases is easy to acquire through open-dataset, and since it leaves out the complicated implementation process and ethical restriction, it is much easier to conduct compared with RCTs (39). In view of these incomparable advantages of MR, here we performed a two-sample MR analysis of the GWAS summary data from the UK Biobank and EBI database so as to find whether there is a potential causal association between FST level and spontaneous abortion, trying to provide novel evidence in this field of research.
2 Materials and methods
2.1 Study design
Here, we conducted a two-sample MR analysis to examine the possible causal association between FST level and spontaneous abortion. Figure 1 provides an overview of the study’s key factors, including the exposures, outcomes, and genetic instruments. The study was based on previously published materials and public databases and received ethical approval and participant consent from the relevant institutional review committees.
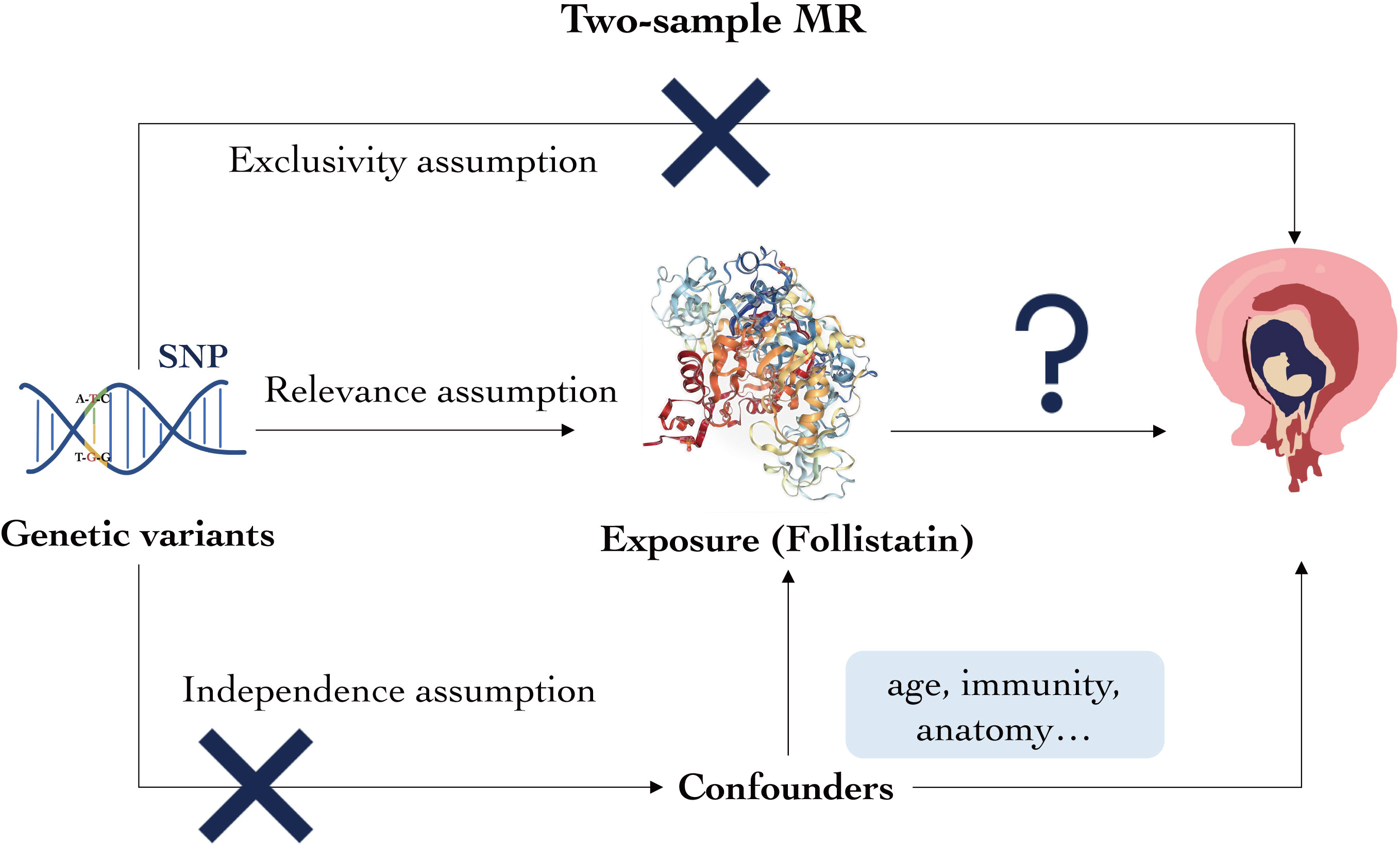
Figure 1 Overview of the study’s key factors. Genetic variant, exposure, and outcomes are as described. Following the three assumptions, genetic variant must be closely correlated with the exposure but cannot be associated with neither the confounders nor the outcomes.
The genetic variants in this study are fully considered based on the three principles below throughout our analysis. First is relevance assumption: the genetic variant must be closely correlated with follistatin levels; second is independence assumption: the genetic variant cannot be associated with any possible confounders of follistatin levels or spontaneous abortion; and third is exclusivity assumption: the genetic variant cannot be related to the relevant outcomes.
2.2 Data sources
2.2.1 Exposure population and data
We extracted exposure data from a previous study (40), downloaded from the website of IEU OPEN GWAS PROJECT (https://gwas.mrcieu.ac.uk/, GWAS ID: ebi-a-GCST90012080).
2.2.2 Outcome population and data
We extracted outcome data from a UK Biobank study (Dataset ID: ukb-d-O03), downloaded from the website of the IEU OPEN GWAS PROJECT (https://gwas.mrcieu.ac.uk/).
2.3 Statistical analysis
All the data processing and statistical analyze are performed using the R4.2.2 software (Lucent Technologies, USA). The MR, heterogeneity test and pleiotropy test were carried by the “Two Sample MR” package (41).
2.4 Extraction of instrumental variables
We performed two-step filtering to eliminate those SNPs that do not satisfy the relevance assumption and obtained the satisfying instrumental variables (IVs). We extracted SNPs that are (1) closely related to the exposure (follistatin level) at a genome-wide significance threshold of p < 5 × 10−8. 2) without linkage disequilibrium (LD) (linkage disequilibrium r2 < 0.05), since we should make sure that no correlation LD between selected IVs and potential confounding factors exist. The R2 value was calculated for these IVs to assess their association with the exposure (42).
2.5 Elimination of confounding factors
We used PhenoScanner (Version 2), a database of human genotype–phenotype associations to figure out whether the confounding factors such as anatomical abnormalities, hormonal imbalances (PCOS, luteal phase defect, etc.), and immune disorders (antiphospholipid syndrome, lupus erythematosus, etc.) may influence our result according to the independent assumption (43, 44). Over 65 billion associations and over 150 million unique genetic variants are recorded in PhenoScanner. We applied the “Phenoscanner” R package to investigated each of the five IVs and their corresponding phenotypes (43, 44). Any IVs exhibiting associations with confounding factors were excluded from the analysis, applying stringent criteria (p < 1 × 10−5, r2 > 0.8).
2.6 Sensitivity test
To make sure that our MR results are robust enough for us to come up with a causal conclusion, we extensively performed three aspects of sensitivity test, namely, heterogeneity test, pleiotropy test, and leave-one-out analysis test. R software was used to visualize results with depicting scatter plot, forest plot, etc.
2.7 Two-sample MR analysis
In two-sample MR analysis, five methods are commonly used: MR-Egger, Weighted Median, Inverse Variance Weighted, Simple Mode, and Weighted Mode. Among these methods, Inverse Variance Weighted is widely accepted and considered the most effective, as it accounts for heterogeneity when assessing causality. However, the other four methods also demonstrate robustness to varying degrees. MR-Egger is particularly useful when there is potential violation of instrumental variable assumptions, such as pleiotropy. It estimates the causal effect while allowing for average pleiotropic bias (45). The Weighted Median method provides a robust estimate by considering the median of all possible instrumental variable estimates, even when up to 50% of the instruments are invalid. This method is advantageous in situations where some instrumental variables may be biased or weak. The Simple Mode and Weighted Mode methods combine the estimates from multiple instruments by either taking the mode or using weighted averages. To evaluate the causal risk of FST levels, we performed all five methods. A positive result from any one or more of these methods would indicate a potential causal risk associated with FST levels.
3 Results
3.1 Study population
The exposure population pertains to follistatin levels, sourced from 39 cohorts of European ancestry. These data were thoroughly cleaned and summarized in a previously published study in 2020 (40), where a genome-wide meta-analysis of 90 cardiovascular-related proteins across 15 studies were identified. We extracted identified genetic variants related to follistatin levels from the recorded ebi-a dataset of IEU open GWAS project (GWAS ID: ebi-a-GCST90012080). In this dataset, 21,758 samples are involved, with 13,022,208 SNPs being reported (40). All of them are of European ancestry. The effect allele (EA), other allele (OA), beta coefficients, p value, and standard error (SE) are also included in this dataset for further investigation.
For the outcomes, summary-level data were obtained from the UK Biobank study (46). In the UK Biobank, pregnancy loss was defined as a history of self-reported spontaneous abortion or termination. We utilized the second round of Neale Lab’s genome-wide association analyses in the UK Biobank, obtaining 1,150 female patients with spontaneous abortion and 360,044 matched controls of European ancestry and 9,543,298 detected SNPs.
3.2 Five SNPs are validated as instrumental variables
MR relies on the idea of random allocation of genetic traits. If the frequency of SNPs harmonizes with the alteration of the exposure, we can tentatively deduce that the SNP is correlated with the exposure. We screen the total of 13,022,208 SNPs in the exposure dataset based on the relevance assumption and independence assumption mentioned in the “study design” and finally achieved five SNPs that satisfied for IVs. We presented some detailed information for these SNPs such as effect allele frequency, standard error, and effect allele (Table 1). In addition, we calculated the R2 value for the IVs, which help to explain the extent of exposure (Table 2).
3.3 MR analysis showed that follistatin level is a causal risk for spontaneous abortion
Here, we adopted five methods to evaluate the follistatin level effect on the risk of spontaneous abortion, and the results are shown in Table 3 and Figure 2. Considering the absence of neither heterogeneity nor pleiotropy (which we would describe in detail on the next part), we selected IVW as the main method as well as the gold standard for determining the causal effect of FST level on the risk of spontaneous abortion (47). We found that the IVW method showed a p value of 0.03517563 (<0.05) and b of −0.001282787 (<0), indicating the causal relationship between low FST level in the European population.
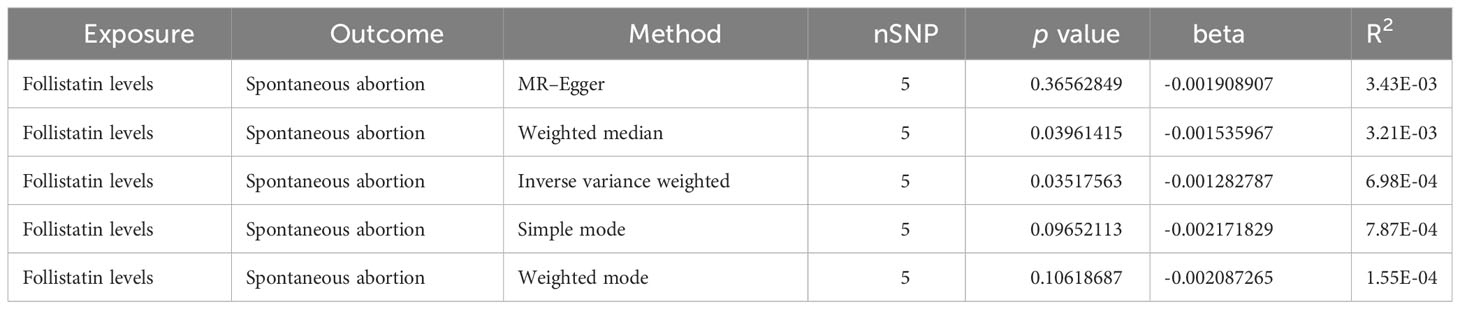
Table 3 Causal effect between follistatin level and spontaneous abortion by different MR analysis methods.
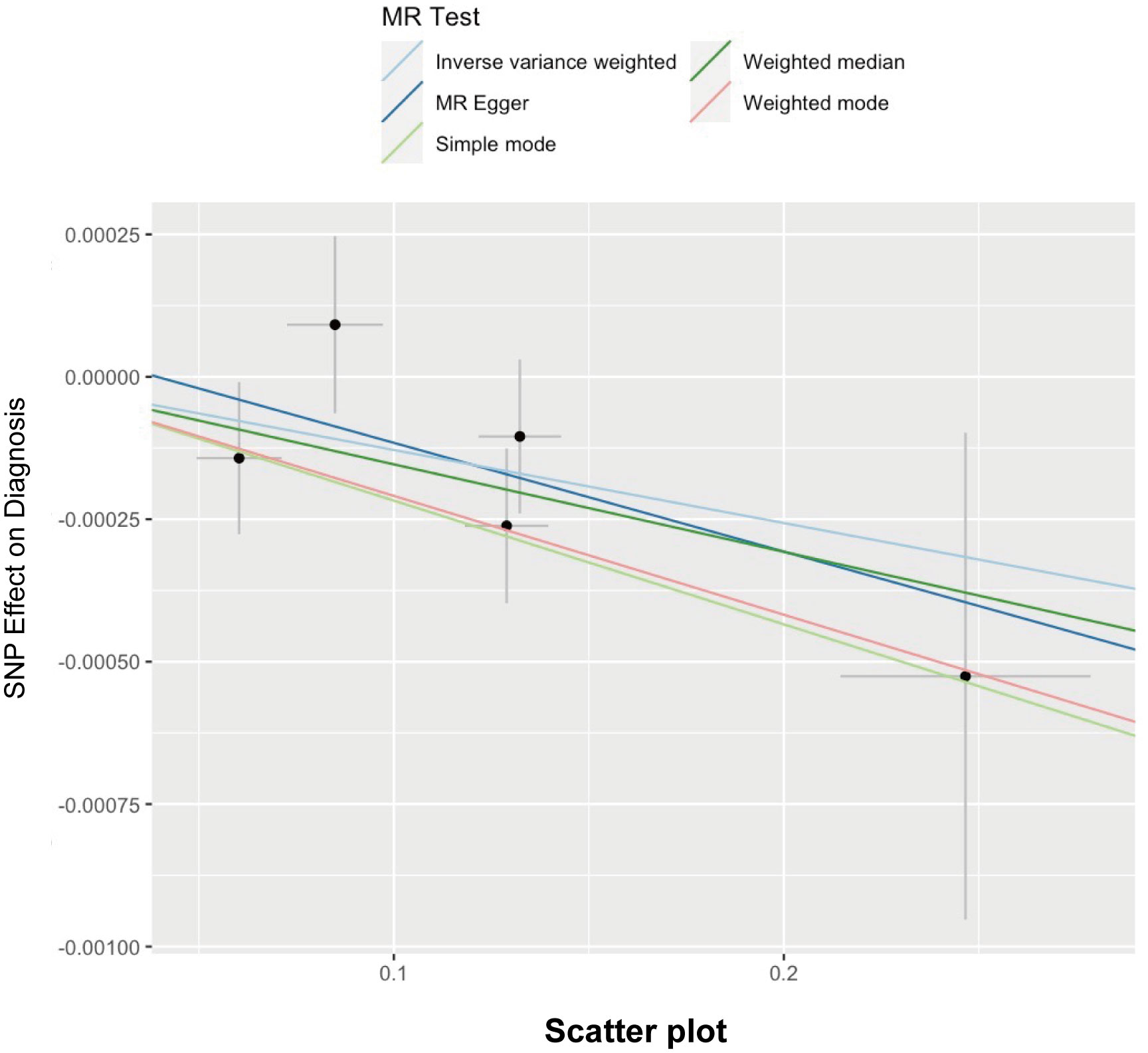
Figure 2 Scatter plot illustrating the distribution of individual ratio estimates of follistatin levels with spontaneous abortion as the outcome. Trend lines derived from five different 2SMR methods are also included in each scatter plot to indicate cause and effect.
3.4 Sensitivity analysis
In this work, sensitivity analysis is performed to (1) evaluate whether the results are robust and the conclusions are reliable; (2) assess whether the results have potential biases (such as genetic pleiotropy and data heterogeneity); and (3) evaluate whether there is a certain instrumental variable that significantly affects the outcome variable.
We detected no heterogeneity in the five IVs that we chose for the spontaneous abortion (MR–Egger Q statistics = 2.738052; Qdf = 3; Qpval = 0.4337995; IVW Q statistics = 2.875518; Qdf = 4; Qpval = 0.5788685) (Table 4). We then focused on the pleiotropy using the MR–Egger method. The intercept with the Y-axis represents the horizontal pleiotropy. Zero horizontal pleiotropy is one of the prerequisites of applying the MR method according to the exclusive assumption. Here, we noticed no horizontal pleiotropy existed in our MR analysis results (Egger_intercept = 7.505441e-05; se = 0.0002024312; p value = 0.7354456) (Table 5). In the “leave-one-out” analysis, we sequentially removed each SNP and calculated the MR effect of the remaining SNPs. We noticed that the removal of any of these individual SNPs did not result in significant changes of the overall causal estimation effect (Figure 3). Taken together, these results suggest that our findings were robust and the exception of single IV exert no difference on the overall estimated causal effect.
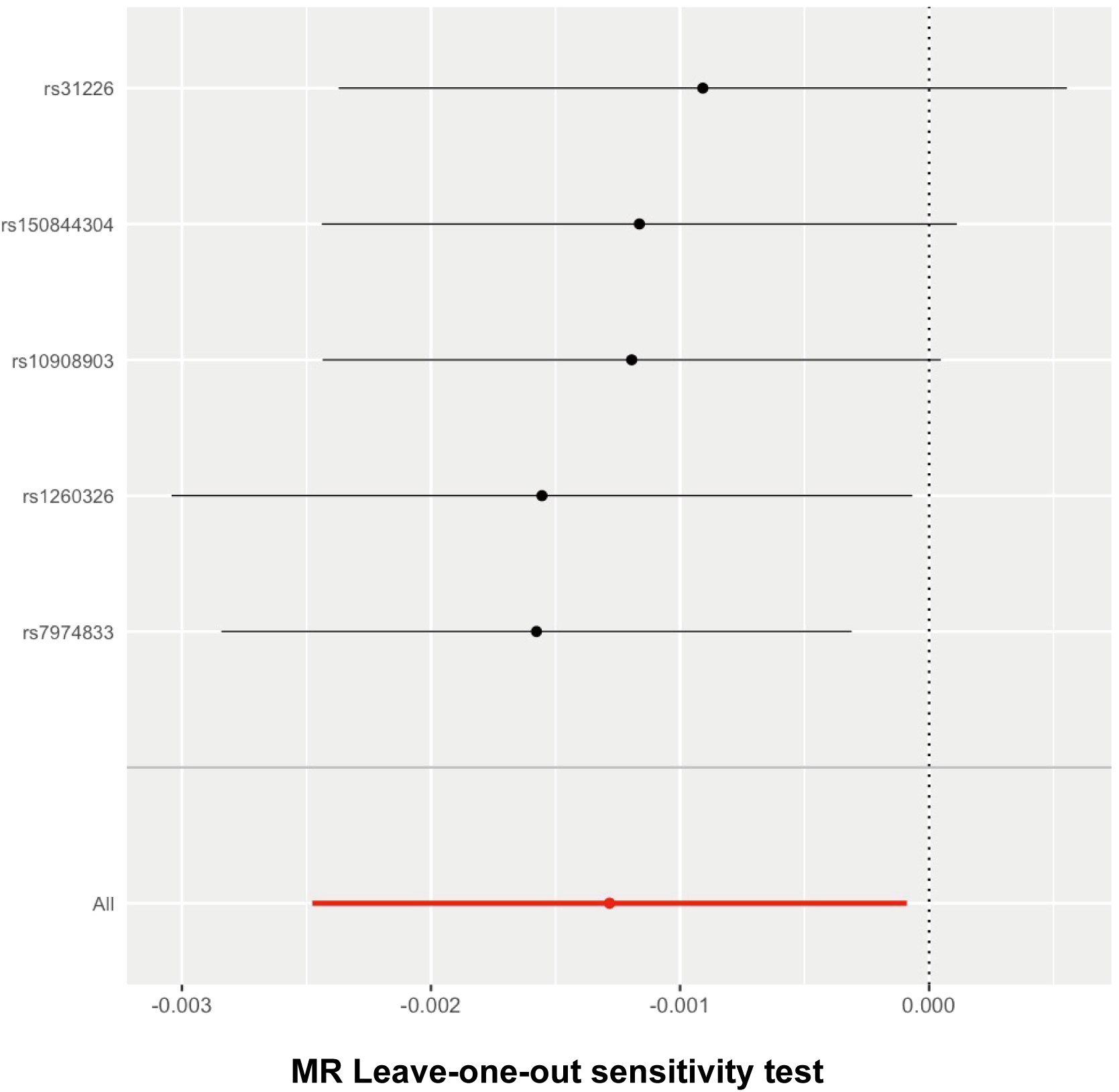
Figure 3 Leave-one-out analysis for follistatin levels on spontaneous abortion. The given dark dots indicate effect measures from IVW-MR analysis excluding specific SNPs. Red line indicates pooled analysis including all SNPs by the IVW-MR method (plotted for comparison).
The Wald ratio method was used to estimate the causal effect of each individual SNP on the risk of spontaneous abortion. The findings have been presented in a forest plot to provide a visual representation (Figure 4). The threshold of significance for the forest plot remained controversial. It can be defined as either p < 0.05 or p < 0.05/n (n refers to the number of SNPs). Here, comprehensively regarding the p values for each single SNP on the outcome (Table 1), the leave-one-out analysis test (Figure 3), and all the SNPs combined (Figure 4), it was quite clear that a causal association existed between follistatin level and the risk of spontaneous abortion.
4 Discussion
In this study, we employed two-sample MR to investigate the causal relationship between FST levels and spontaneous abortion. The evidence from MR analysis indicates that low follistatin level was a causal risk factor for spontaneous abortion and these results were generally reliable as the sensitivity analysis strongly supports. These findings have important implications for understanding the pathogenesis of spontaneous abortion and may contribute to the development of potential preventive and therapeutic strategies.
It is widely acknowledged that establishing proper placentation involves sequential processes, notably trophoblast invasion and angiogenesis (48). The orchestrated interplay of angiogenic processes and steroid hormones induces transformative changes in the endometrium, facilitating its receptivity to the blastocyst and initiating the implantation process (49). Successful placentation and the commencement of pregnancy hinge on prerequisites such as endometrial angiogenesis, decidualization, and trophoblast invasion (48, 50, 51). Any dysregulation during the above processes may lead to pregnancy failure and RPL. High levels of anti-annexin V antibody can bind to trophoblast cells, affecting trophoblast invasiveness and causing defective placentation (52). Also, lots of proteins and related pathways have been recognized to be involved in regulating trophoblast function, such as TGF-β which governs the differentiation program of extravillous trophoblasts in the developing human placenta (53).
The underlying biological mechanisms linking low follistatin levels with spontaneous abortion warrant further investigation. Follistatin not only has an inhibitory effect on FSH secretion from cultured anterior pituitary cells (54) but also is involved in trophoblast invasion and embryonic development. FST acts as an antagonist to the TGF-β superfamily and thereby modulates important signaling pathways such as JNK signaling, ALK4-SMAD2/3-SMAD4 signaling, and FST-GDF11-Smad2/3, further affecting trophoblast function (55). It is hypothesized that reduced follistatin levels may disrupt multiple pathways such as JNK signaling, ALK4-SMAD2/3-SMAD4 signaling, and FST-GDF11-Smad2/3, leading to trophoblast dysfunction and causing impaired implantation and defective placental development, ultimately resulting in abortion. Future research should focus on elucidating the specific molecular mechanisms through which follistatin influences pregnancy outcomes.
Our results are consistent with previous studies that have reported a probable association between low follistatin levels and adverse pregnancy outcomes (30, 56–58). For instance, Prakash et al. (30) observed a dramatic decrease of FST expression in endometrial stromal cells of women with spontaneous abortion. However, it should be noted that some studies reported no significant decrease of follistatin in the serum of women with spontaneous abortion (59, 60). These discrepancies may arise from variations in sample size and characteristics, since it only includes around 10 abortion samples and 10 control samples.
Although previous studies have pointed out a possible association between FST and spontaneous abortion, there is no absolute evidence on a genetic aspect. Establishing a direct causal link between follistatin and spontaneous abortion presents challenges due to confounding effects from factors such as lifestyle and environmental influences. To overcome this challenge, a promising approach is the utilization of MR, which leverages genetic variants as IVs to infer causal relationships. In our study, we utilized MR to present novel evidence that demonstrates a causal relationship between low FST levels and spontaneous abortion. This finding holds significant implications for the field of reproductive health. Our results suggest that interventions targeting increased FST levels may have the potential to reduce the incidence of spontaneous abortion, which is a devastating outcome for numerous couples. However, despite the valuable insights gained from this study, several limitations should be acknowledged. We recognize that our analysis only included the European population, introducing the possibility of potential bias associated with differing ancestries.
Our study yields meaningful clinical implications. First, it indicates that FST levels might be integrated into routine antenatal assessments to evaluate the risk of pregnancy failure. This is particularly crucial for individuals with a history of spontaneous abortion, where assessing their FST levels may serve as a predictive indicator for the occurrence of RPL. Concurrently, FST may function as a prospective biomarker for targeted interventions like hormonal therapies or lifestyle adjustments and the development of personalized medical strategies. Future studies could explore interventions aimed at modulating follistatin levels to potentially prevent or mitigate the risk of spontaneous abortion. Moreover, investigations into the long-term effects of low follistatin levels on maternal and offspring health outcomes would be valuable for a comprehensive understanding of the implications.
In conclusion, our study contributes to the growing body of reliable evidence supporting the critical role of FST in successful pregnancy outcomes and highlights it as a promising therapeutic target. We remain hopeful that further research, conducted with larger sample sizes based on our observations, will provide additional insights into the underlying mechanisms that link FST and pregnancy outcomes.
Data availability statement
This study analyzed the exposure data acquired from EBI database and the outcome data sourced from the UK Biobank. Both datasets are publicly accessible and can be found on the IEU Open GWAS Project website (https://gwas.mrcieu.ac.uk/).
Author contributions
CG: Formal analysis, Investigation, Methodology, Writing – original draft, Writing – review & editing. WY: Methodology, Writing – review & editing. XL: Writing – review & editing. XLL: Writing – review & editing. YW: Validation, Writing – review & editing. CT: Conceptualization, Methodology, Supervision, Writing – review & editing.
Funding
The author(s) declare financial support was received for the research, authorship, and/or publication of this article. This project was supported by the Natural Science Foundation of China (82171654) and the Clinical Medicine Plus X-Young Scholars Project of Peking University (Grant PKU2022LCXQ025.
Acknowledgments
We would like to express our sincere gratitude to our team members for their invaluable support and contributions to this research project. Their dedication and expertise have been instrumental in the successful completion of this work.
Conflict of interest
The authors declare that the research was conducted in the absence of any commercial or financial relationships that could be construed as a potential conflict of interest.
Publisher’s note
All claims expressed in this article are solely those of the authors and do not necessarily represent those of their affiliated organizations, or those of the publisher, the editors and the reviewers. Any product that may be evaluated in this article, or claim that may be made by its manufacturer, is not guaranteed or endorsed by the publisher.
References
1. Griebel CP, Halvorsen J, Golemon TB, Day AA. Management of spontaneous abortion. Am Fam Physician. (2005) 72(7):1243–50.
2. Macklon NS, Geraedts JP, Fauser BC. Conception to ongoing pregnancy: the 'black box' of early pregnancy loss. Hum Reprod Update. (2002) 8(4):333–43. doi: 10.1093/humupd/8.4.333
3. Hendzel MJ, Wei Y, Mancini MA, Van Hooser A, Ranalli T, Brinkley BR, et al. Mitosis-specific phosphorylation of histone H3 initiates primarily within pericentromeric heterochromatin during G2 and spreads in an ordered fashion coincident with mitotic chromosome condensation. Chromosoma. (1997) 106(6):348–60. doi: 10.1007/s004120050256
4. Ruderman RS, Yilmaz BD, McQueen DB. Treating the couple: how recurrent pregnancy loss impacts the mental health of both partners. Fertil Steril. (2020) 114(6):1182. doi: 10.1016/j.fertnstert.2020.09.165
5. Kiwi R. Recurrent pregnancy loss: evaluation and discussion of the causes and their management. Cleve Clin J Med (2006) 73(10):913–21. doi: 10.3949/ccjm.73.10.913
6. Propst AM, Hill JA 3rd. Anatomic factors associated with recurrent pregnancy loss. Semin Reprod Med (2000) 18(4):341–50. doi: 10.1055/s-2000-13723
7. Rock JA, Murphy AA. Anatomic abnormalities. Clin Obstet Gynecol. (1986) 29(4):886–911. doi: 10.1097/00003081-198612000-00015
8. Amrane S, McConnell R. Endocrine causes of recurrent pregnancy loss. Semin Perinatol. (2019) 43(2):80–3. doi: 10.1053/j.semperi.2018.12.004
9. Ticconi C, Pietropolli A, Di Simone N, Piccione E, Fazleabas A. Endometrial immune dysfunction in recurrent pregnancy loss. Int J Mol Sci (2019) 20(21):5332. doi: 10.3390/ijms20215332
10. Caruso A, De Carolis S, Di Simone N. Antiphospholipid antibodies in obstetrics: new complexities and sites of action. Hum Reprod Update. (1999) 5(3):267–76. doi: 10.1093/humupd/5.3.267
11. Di Simone N, De Spirito M, Di Nicuolo F, Tersigni C, Castellani R, Silano M, et al. Potential new mechanisms of placental damage in celiac disease: anti-transglutaminase antibodies impair human endometrial angiogenesis. Biol Reprod (2013) 89(4):88. doi: 10.1095/biolreprod.113.109637
12. Di Simone N, Riccardi P, Maggiano N, Piacentani A, D'Asta M, Capelli A, et al. Effect of folic acid on homocysteine-induced trophoblast apoptosis. Mol Hum Reprod (2004) 10(9):665–9. doi: 10.1093/molehr/gah091
13. Lin SY, Morrison JR, Phillips DJ, de Kretser DM. Regulation of ovarian function by the TGF-beta superfamily and follistatin. Reproduction. (2003) 126(2):133–48. doi: 10.1530/rep.0.1260133
14. Perakakis N, Upadhyay J, Ghaly W, Chen J, Chrysafi P, Anastasilakis AD, et al. Regulation of the activins-follistatins-inhibins axis by energy status: Impact on reproductive function. Metabolism. (2018) 85:240–9. doi: 10.1016/j.metabol.2018.05.003
15. Rajput SK, Lee K, Zhenhua G, Di L, Folger JK, Smith GW. Embryotropic actions of follistatin: paracrine and autocrine mediators of oocyte competence and embryo developmental progression. Reprod Fertil Dev (2013) 26(1):37–47.
16. Thompson TB, Lerch TF, Cook RW, Woodruff TK, Jardetzky TS. The structure of the follistatin:activin complex reveals antagonism of both type I and type II receptor binding. Dev Cell (2005) 9(4):535–43. doi: 10.1016/j.devcel.2005.09.008
17. Nakamura T, Takio K, Eto Y, Shibai H, Titani K, Sugino H. Activin-binding protein from rat ovary is follistatin. Science. (1990) 247(4944):836–8. doi: 10.1126/science.2106159
18. Rabinovici J, Spencer SJ, Doldi N, Goldsmith PC, Schwall R, Jaffe RB. Activin-A as an intraovarian modulator: actions, localization, and regulation of the intact dimer in human ovarian cells. J Clin Invest. (1992) 89(5):1528–36. doi: 10.1172/JCI115745
19. Rabinovici J, Spencer SJ, Jaffe RB. Recombinant human activin-A promotes proliferation of human luteinized preovulatory granulosa cells in vitro. J Clin Endocrinol Metab (1990) 71(5):1396–8. doi: 10.1210/jcem-71-5-1396
20. Li W, Yuen BH, Leung PC. Inhibition of progestin accumulation by activin-A in human granulosa cells. J Clin Endocrinol Metab (1992) 75(1):285–9. doi: 10.1210/jc.75.1.285
21. Li W, Khorasheh S, Yuen BH, Ling N, Leung PC. Stimulation of progesterone secretion by recombinant follistatin-288 in human granulosa cells. Endocrinology. (1993) 132(4):1750–6. doi: 10.1210/endo.132.4.8384994
22. Peng C, Ohno T, Khorasheh S, Leung PCK. Activin and follistatin as local regulators in the human ovary. Neurosignals. (1996) 5(2):81–9. doi: 10.1159/000109177
23. Li Y, Klausen C, Zhu H, Leung PC. Activin A increases human trophoblast invasion by inducing SNAIL-mediated MMP2 up-regulation through ALK4. J Clin Endocrinol Metab (2015) 100(11):E1415–27. doi: 10.1210/jc.2015-2134
24. Sun F, Cheng L, Guo L, Su S, Li Y, Yan J. Activin A promotes human trophoblast invasion by upregulating integrin beta3 via ALK4-SMAD4 signaling. Placenta. (2022) 129:62–9. doi: 10.1016/j.placenta.2022.10.004
25. Zhu S, Li Z, Cui L, Ban Y, Leung PCK, Li Y, et al. Activin A increases human trophoblast invasion by upregulating integrin beta1 through ALK4. FASEB J (2021) 35(2):e21220. doi: 10.1096/fj.202001604R
26. Li Y, Klausen C, Cheng JC, Zhu H, Leung PC. Activin A, B, and AB increase human trophoblast cell invasion by up-regulating N-cadherin. J Clin Endocrinol Metab (2014) 99(11):E2216–25. doi: 10.1210/jc.2014-2118
27. Koninger A, Schmidt B, Damaske D, Birdir C, Enekwe A, Kimmig R, et al. Follistatin during pregnancy and its potential role as an ovarian suppressing agent. Eur J Obstet Gynecol Reprod Biol (2017) 212:150–4. doi: 10.1016/j.ejogrb.2017.03.001
28. Li J, Qi Y, Yang K, Zhu L, Cui X, Liu Z. Follistatin is a novel chemoattractant for migration and invasion of placental trophoblasts of mice. Cells. (2022) 11(23):3816. doi: 10.3390/cells11233816
29. Liu G, Qi Y, Wu J, Lin F, Liu Z, Cui X. Follistatin is a crucial chemoattractant for mouse decidualized endometrial stromal cell migration by JNK signalling. J Cell Mol Med (2023) 27(1):127–40. doi: 10.1111/jcmm.17648
30. Prakash A, Li TC, Tuckerman E, Laird S, Wells M, Ledger WL. A study of luteal phase expression of inhibin, activin, and follistatin subunits in the endometrium of women with recurrent miscarriage. Fertil Steril. (2006) 86(6):1723–30. doi: 10.1016/j.fertnstert.2006.05.040
31. Fullerton PT Jr., Monsivais D, Kommagani R, Matzuk MM. Follistatin is critical for mouse uterine receptivity and decidualization. Proc Natl Acad Sci U S A. (2017) 114(24):E4772–E81. doi: 10.1073/pnas.1620903114
32. Mdlalose S, Moodley J, Naicker T. The role of follistatin and granulocyte-colony stimulating factor in HIV-associated pre-eclampsia. Pregnancy Hypertens (2020) 19:81–6. doi: 10.1016/j.preghy.2019.12.012
33. Zhao L, Shang T, Wang YL, Tang S, Li H, Liu ZH. [Study on the relationship between activin A, follistatin and preeclampsia]. Zhonghua Fu Chan Ke Za Zhi. (2003) 38(11):676–9.
34. Garces MF, Vallejo SA, Sanchez E, Palomino-Palomino MA, Leal LG, Angel-Muller E, et al. Longitudinal analysis of maternal serum Follistatin concentration in normal pregnancy and preeclampsia. Clin Endocrinol (Oxf). (2015) 83(2):229–35. doi: 10.1111/cen.12715
35. Li H, Zhou L, Zhang C, Xi Q, Lv J, Huo W, et al. Follistatin dysregulation impaired trophoblast biological functions by GDF11-Smad2/3 axis in preeclampsia placentas. Placenta. (2022) 121:145–54. doi: 10.1016/j.placenta.2022.03.015
36. Smith GD, Ebrahim S. 'Mendelian randomization': can genetic epidemiology contribute to understanding environmental determinants of disease? Int J Epidemiol (2003) 32(1):1–22. doi: 10.1093/ije/dyg070
37. Davies NM, Holmes MV, Davey Smith G. Reading Mendelian randomisation studies: a guide, glossary, and checklist for clinicians. BMJ (2018) 362:k601. doi: 10.1136/bmj.k601
38. Zheng J, Baird D, Borges MC, Bowden J, Hemani G, Haycock P, et al. Recent developments in mendelian randomization studies. Curr Epidemiol Rep (2017) 4(4):330–45. doi: 10.1007/s40471-017-0128-6
39. Emdin CA, Khera AV, Kathiresan S. Mendelian randomization. JAMA. (2017) 318(19):1925–6. doi: 10.1001/jama.2017.17219
40. Folkersen L, Gustafsson S, Wang Q, Hansen DH, Hedman AK, Schork A, et al. Genomic and drug target evaluation of 90 cardiovascular proteins in 30,931 individuals. Nat Metab (2020) 2(10):1135–48. doi: 10.1038/s42255-020-00287-2
41. Hemani G, Zheng J, Elsworth B, Wade KH, Haberland V, Baird D, et al. The MR-Base platform supports systematic causal inference across the human phenome. Elife (2018) 7:e34408. doi: 10.7554/eLife.34408
42. Bowden J, Del Greco MF, Minelli C, Davey Smith G, Sheehan NA, Thompson JR. Assessing the suitability of summary data for two-sample Mendelian randomization analyses using MR-Egger regression: the role of the I2 statistic. Int J Epidemiol. (2016) 45(6):1961–74. doi: 10.1093/ije/dyw220
43. Staley JR, Blackshaw J, Kamat MA, Ellis S, Surendran P, Sun BB, et al. PhenoScanner: a database of human genotype-phenotype associations. Bioinformatics. (2016) 32(20):3207–9. doi: 10.1093/bioinformatics/btw373
44. Kamat MA, Blackshaw JA, Young R, Surendran P, Burgess S, Danesh J, et al. PhenoScanner V2: an expanded tool for searching human genotype-phenotype associations. Bioinformatics. (2019) 35(22):4851–3. doi: 10.1093/bioinformatics/btz469
45. Hemani G, Bowden J, Davey Smith G. Evaluating the potential role of pleiotropy in Mendelian randomization studies. Hum Mol Genet (2018) 27(R2):R195–208. doi: 10.1093/hmg/ddy163
46. Sudlow C, Gallacher J, Allen N, Beral V, Burton P, Danesh J, et al. UK biobank: an open access resource for identifying the causes of a wide range of complex diseases of middle and old age. PLoS Med (2015) 12(3):e1001779. doi: 10.1371/journal.pmed.1001779
47. Sproviero W, Winchester L, Newby D, Fernandes M, Shi L, Goodday SM, et al. High blood pressure and risk of dementia: A two-sample mendelian randomization study in the UK biobank. Biol Psychiatry (2021) 89(8):817–24. doi: 10.1016/j.biopsych.2020.12.015
48. Huppertz B, Peeters LL. Vascular biology in implantation and placentation. Angiogenesis. (2005) 8(2):157–67. doi: 10.1007/s10456-005-9007-8
49. Di Simone N, D'Ippolito S, Marana R, Di Nicuolo F, Castellani R, Pierangeli SS, et al. Antiphospholipid antibodies affect human endometrial angiogenesis: protective effect of a synthetic peptide (TIFI) mimicking the phospholipid binding site of beta(2) glycoprotein I. Am J Reprod Immunol (2013) 70(4):299–308. doi: 10.1111/aji.12130
50. Cullinan-Bove K, Koos RD. Vascular endothelial growth factor/vascular permeability factor expression in the rat uterus: rapid stimulation by estrogen correlates with estrogen-induced increases in uterine capillary permeability and growth. Endocrinology. (1993) 133(2):829–37. doi: 10.1210/endo.133.2.8344219
51. Reynolds LP, Borowicz PP, Caton JS, Vonnahme KA, Luther JS, Buchanan DS, et al. Uteroplacental vascular development and placental function: an update. Int J Dev Biol (2010) 54(2-3):355–66. doi: 10.1387/ijdb.082799lr
52. Di Simone N, Castellani R, Caliandro D, Caruso A. Monoclonal anti-annexin V antibody inhibits trophoblast gonadotropin secretion and induces syncytiotrophoblast apoptosis. Biol Reprod (2001) 65(6):1766–70. doi: 10.1095/biolreprod65.6.1766
53. Haider S, Lackner AI, Dietrich B, Kunihs V, Haslinger P, Meinhardt G, et al. Transforming growth factor-beta signaling governs the differentiation program of extravillous trophoblasts in the developing human placenta. Proc Natl Acad Sci U S A. (2022) 119(28):e2120667119. doi: 10.1073/pnas.2120667119
54. Yokoyama Y, Nakamura T, Nakamura R, Irahara M, Aono T, Sugino H. Identification of activins and follistatin proteins in human follicular fluid and placenta. J Clin Endocrinol Metab (1995) 80(3):915–21. doi: 10.1210/jcem.80.3.7883850
55. Goldman-Wohl D, Yagel S. Regulation of trophoblast invasion: from normal implantation to pre-eclampsia. Mol Cell Endocrinol (2002) 187(1-2):233–8. doi: 10.1016/S0303-7207(01)00687-6
56. Prakash A, Laird S, Tuckerman E, Li TC, Ledger WL. Inhibin A and activin A may be used to predict pregnancy outcome in women with recurrent miscarriage. Fertil Steril. (2005) 83(6):1758–63. doi: 10.1016/j.fertnstert.2004.11.072
57. Daponte A, Deligeoroglou E, Garas A, Pournaras S, Hadjichristodoulou C, Messinis IE. Activin A and follistatin as biomarkers for ectopic pregnancy and missed abortion. Dis Markers. (2013) 35(5):497–503. doi: 10.1155/2013/969473
58. Lin SY, Craythorn RG, O'Connor AE, Matzuk MM, Girling JE, Morrison JR, et al. Female infertility and disrupted angiogenesis are actions of specific follistatin isoforms. Mol Endocrinol (2008) 22(2):415–29. doi: 10.1210/me.2006-0529
59. Muttukrishna S, Jauniaux E, Greenwold N, McGarrigle H, Jivraj S, Carter S, et al. Circulating levels of inhibin A, activin A and follistatin in missed and recurrent miscarriages. Hum Reprod (2002) 17(12):3072–8. doi: 10.1093/humrep/17.12.3072
Keywords: follistatin, spontaneous abortion, mendelian randomization, abortion, recurrent pregnancy loss
Citation: Gong C, Yang W, Liu X, Li X, Wang Y and Tian C (2024) Low follistatin level is a causal risk factor for spontaneous abortion: a two-sample mendelian randomization study. Front. Endocrinol. 14:1255591. doi: 10.3389/fendo.2023.1255591
Received: 09 July 2023; Accepted: 30 November 2023;
Published: 03 January 2024.
Edited by:
Zitao Liu, New Hope Fertility Center, United StatesCopyright © 2024 Gong, Yang, Liu, Li, Wang and Tian. This is an open-access article distributed under the terms of the Creative Commons Attribution License (CC BY). The use, distribution or reproduction in other forums is permitted, provided the original author(s) and the copyright owner(s) are credited and that the original publication in this journal is cited, in accordance with accepted academic practice. No use, distribution or reproduction is permitted which does not comply with these terms.
*Correspondence: Chan Tian, dGlhbmNoYW5AYmptdS5lZHUuY24=