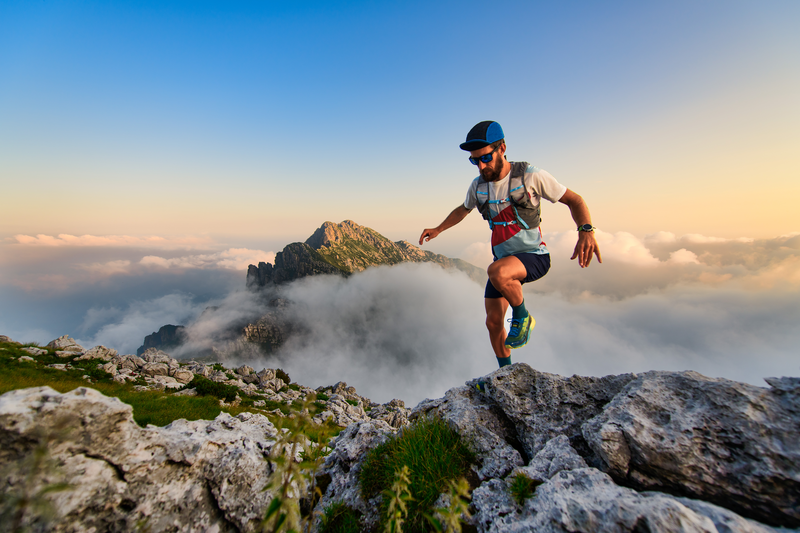
94% of researchers rate our articles as excellent or good
Learn more about the work of our research integrity team to safeguard the quality of each article we publish.
Find out more
ORIGINAL RESEARCH article
Front. Endocrinol. , 06 October 2023
Sec. Obesity
Volume 14 - 2023 | https://doi.org/10.3389/fendo.2023.1243906
This article is part of the Research Topic The Role of Vitamin D in Reducing the Risk of Metabolic Syndromes View all 6 articles
Background: Obesity (OB) is a chronic metabolic disease with important associated comorbidities and mortality. Vitamin D supplementation is frequently administered after bariatric surgery (BS), so as to reduce OB-related complications, maybe including chronic inflammation.
Aim: This study aimed to explore relations between vitamin D metabolites and components of the inflammasome machinery in OB before and after BS and their relations with the improvement of metabolic comorbidities.
Patients and methods: Epidemiological/clinical/anthropometric/biochemical evaluation was performed in patients with OB at baseline and 6 months after BS. Evaluation of i) vitamin-D metabolites in plasma and ii) components of the inflammasome machinery and inflammatory-associated factors [NOD-like-receptors (NLRs), inflammasome-activation-components, cytokines and inflammation/apoptosis-related components, and cell-cycle and DNA-damage regulators] in peripheral blood mononuclear cells (PBMCs) was performed at baseline and 6 months after BS. Clinical and molecular correlations/associations were analyzed.
Results: Significant correlations between vitamin D metabolites and inflammasome-machinery components were observed at baseline, and these correlations were significantly reduced 6 months after BS in parallel to a decrease in inflammation markers, fat mass, and body weight. Treatment with calcifediol remarkably increased 25OHD levels, despite 24,25(OH)2D3 remained stable after BS. Several inflammasome-machinery components were associated with improvement in metabolic comorbidities, especially hypertension and dyslipidemia.
Conclusion: The beneficial effects of vitamin D on OB-related comorbidities after BS patients are associated with significant changes in the molecular expression of key inflammasome-machinery components. The expression profile of these inflammasome components can be dynamically modulated in PBMCs after BS and vitamin D supplementation, suggesting that this profile could likely serve as a sensor and early predictor of the reversal of OB-related complications after BS.
Obesity (OB) is characterized by an excess amount of body fat; it has an important negative impact on health and quality of life (1). OB is a major public health problem due to its growing incidence, high frequency of comorbidities, and mortality (2, 3). Metabolic comorbidities are observed in 10%–30% of patients (4), especially type-2 diabetes (T2DM), hypertension (HT), heart disease, high total cholesterol and triglycerides, stroke, and non-alcoholic fatty liver disease (NAFLD) (5–7).
Bariatric surgery (BS) is a well-recognized treatment for severe OB and generates superior outcomes compared with non-surgical methods (8). BS induces substantial and sustained weight loss through a variety of mechanisms (9–12), which are accompanied by reduction in obesity-related comorbidities and improvement in quality of life (13, 14). In clinical practice, the measurement of the total blood concentration of 25OHD is the marker of the nutritional status of the vitamin D endocrine system (VDES) (15).. Obesity is consistently characterized by lower 25(OH)D blood levels due to several mechanisms including volumetric dilution, adipose tissue (AT) sequestration, impaired hepatic 25-hydroxylation, altered VDES metabolism in AT, and some unknown additional mechanisms (16–20).
Several external factors influence serum/plasma 25(OH)D levels such as season, geographical location, ethnicity, and sunlight exposure (21). Additionally, endogenous factors such as OB, starvation, diabetes, and glucocorticoids have a major influence on cytochrome P450 Family 2 subfamily R member 1 (CYP2R1) activity, affecting the production, and in consequence the circulating levels of 25(OH)-D (22). In this context, total serum 25(OH)-D may incorrectly reflect VDES status. It has been proposed that the ratio of serum 24,25-dihydroxyvitamin D (24,25(OH)2 D to 25(OH)D (vitamin D metabolite ratio [VMR]) could represent a new and more reliable biomarker for assessing vitamin D status in processes such as vitamin D deficiency, idiopathic childhood hypercalcemia, and chronic kidney disease, and even assessing the entire VDES metabolome, idiopathic childhood hypercalcemia, and chronic kidney disease (23), or even to assess the entire VDES metabolome by liquid chromatography coupled to tandem mass spectrometry (SPE–LC–MS/MS) as the technique of choice (24).
Deficiency of 25(OH)-D has been associated with metabolic comorbidities including NAFLD, T2DM, insulin resistance, and metabolic syndrome (25–27). In this context, several studies have reported the influence of vitamin D supplementation on reversion of metabolic comorbidities with contradictory results (28, 29). Recent studies have shown that low levels of 25(OH)-D have been associated with a higher prevalence of infections and autoimmune diseases, while adequate levels have been correlated to protection of these processes (30, 31).
OB is characterized by the presence of low-grade chronic inflammation, due to increased pro-inflammatory cytokine production by macrophages and adipocytes. Multiple studies have shown that in the presence of abdominal adiposity, vitamin D deficiency is linked to inflammation and decreased insulin sensitivity (32).
VDES is a key modulator of immune function and inflammation, the active metabolite of the system reduces adipocyte chemokine and cytokine release, and monocyte chemotaxis (33). Its effects on the systemic and tissue-specific inflammatory response have been attributed to a variety of factors, including suppression of the nuclear factor-κB (NF-κβ pathway), T-helper cell anti-inflammatory activation, reduction in toll-like receptor 4 (TLR-4) expression (which reduces the differentiation of dendritic cells), and modulation of inflammasome activation (34, 35).
Inflammasomes are multimeric protein complexes that detect pathogenic microorganisms and sterile stressors, activate highly pro-inflammatory cytokines, and are, in consequence, responsible for innate immune system response (36, 37). Inflammasome have germline-encoded pattern-recognition receptors (PRRs), which recognize the presence of unique microbial components [pathogen-associated molecular patterns (PAMPs)], including bacterial flagellin or damage-associated molecular patterns (DAMPs), such as uric acid crystals, which are generated by endogenous stress (38); in consequence, inflammasome activates the innate immune system (39) in response to infection and/or to repair damaged tissues (40).
Remarkably, the activity of inflammasome components is regulated by different regulatory proteins, metabolic pathways, and a regulatory mitochondrial hub (37, 41). In consequence, the activation of these inflammasome components leads to the secretion of diverse inflammatory cytokines and key receptors in immune cells, thus inducing the activation of inflammatory cascades, which can lead, in some cases, to cell-cycle alterations and DNA damage (36, 41).
Dysregulation of inflammasome has been associated with several inflammatory disorders including Alzheimer’s disease, autoinflammatory diseases, and OB-related comorbidities, including T2DM, NAFLD, and atherosclerosis (36, 37). A preliminary study of our group has reported dysregulation of several components of inflammasome in patients with OB and their relation with the presence of metabolic comorbidities (42).
Considering the link between vitamin D, inflammation, inflammasome, and OB, the aim of the present study was to investigate the clinical relation between VDES status, inflammasome activation, and reversal of metabolic comorbidities in patients with OB who underwent BS and received oral supplementation with calcifediol (25(OH)3. Peripheral blood mononuclear cells (PBMCs) were used, since gene expression patterns are commonly reflected in these cells and are closely related to the molecular profile of the disease (43). Specifically, the gene expression levels of four groups of components of the inflammasome machinery, namely, 1) NLRs or NOD-like receptors, 2) regulators of inflammasome activation, 3) cytokines and inflammation/apoptosis-related components, and 4) cell-cycle and DNA-damage regulators, were analyzed. Additionally, we measured VDES metabolites using SPE–LC–MS/MS, which has been reported as an accurate and robust method for measuring vitamin D metabolites in human serum (23, 44). The putative relations between gene expression levels of the inflammasome machinery with vitamin D metabolites and the clinical evolution of metabolic and inflammatory comorbidities, associated with OB, after BS were explored.
This study was approved by the Ethics Committee of the Reina Sofia University Hospital (Cordoba, Spain; registration code CB01072018) and was conducted in accordance with the Declaration of Helsinki and according to national and international guidelines. This is a prospective open-label study, wherein a written informed consent was signed by every individual before inclusion into the study. A total of 40 consecutive patients who underwent BS were included. Clinical records were used to collect full medical history of all patients (demographic and clinical characteristics of patients are summarized in Table 1). All patients were managed following available clinical guidelines and recommendations (45–49). They received medical treatment with oral calcifediol in variable dose in order to reach blood levels >30 ng/dL (0.266 mg every 10, 15, 21, or 30 days). Clinical follow-up was performed by the same clinician in all cases at baseline (before surgery) and 6 months after BS. Body composition was evaluated using a multi-frequency bioimpedanciometer (Tanita MC-780MA, Barcelona, Spain), and waist circumference was measured at minimal expiration. Blood samples were obtained at baseline and 6 months after BS from all patients to obtain plasma, serum, and PBMCs. Regular physical exercise and health style education are regularly advised to all patients; specific evaluation of the patient adherence was not performed.
Mass spectrometry grade ammonium formate and formic acid (FA) were acquired from Sigma (Sigma–Aldrich, St. Louis, MO, USA) as ionization and sorbent activation agents; methanol and acetonitrile (ACN) from Scharlab (Barcelona, Spain) and deionized water (18 mΩ cm) from a Millipore Milli-Q water purification system were employed for the preparation of chromatographic mobile phases and SPE solutions.
Analytical standards of vitamin D3, 25-hydroxyvitamin D3 [25(OH)D3], 1,25-dihydroxyvitamin D3 [1,25(OH)2D3], 24,25-dihydroxyvitamin D3 [24,25(OH)2D3] and their deuterated internal standards (ISs), vitamin D3-d3, 25(OH)D3-d3, 1,25(OH)2D3-d3, and 24,25(OH)2D3-d6, were purchased from Sigma-Aldrich. 1,24,25-Trihydroxyvitamin D3 [1,24,25(OH)3D3] analytical standard was obtained from Quimigen S.L. (Madrid, Spain), but no IS for this analyte was available. According to endogenous concentration ranges of these metabolites found in blood samples, a multistandard working solution was prepared: vitamin D3 at 2.5 μg mL–1, 25(OH)D3 at 15 μg mL–1, 24,25(OH)2D3 at 0.5 μg mL–1, 1,25(OH)2D3 at 15 ng mL–1, and 1,24,25(OH)3D3 at 50 ng mL–1. Since the presence of matrix effects has been proven in LC–MS/MS analyses of vitamin D3 and its metabolites in blood samples [2,16], a working solution of ISs was used, in order to correct results variations due to this phenomenon, at the following concentrations: vitamin D3-d3 and 25(OH)D3-d3 at 625 ng mL–1, 24,25(OH)2D3-d6 at 125 ng mL–1, and 1,25(OH)2D3-d3 at 7.5 ng mL–1.
Sample preparation step consisted of thawing plasma samples at room temperature, centrifuging (4°C) at 20,000×g for 10 min and sterilizing the resulting supernatant fraction by filtration. Then, aliquots of 240 μL were pretreated by adding 10 μL of ISs working solution to each one followed by SPE after shaking in vortex for 2 min. Regarding SPE stage, activation of cartridge’s sorbent was achieved by adding 6 mL of methanol and conditioning and equilibration of cartridges by adding 8 mL of the loading sample solvent mix as recommended by the manufacturer. However, different loading sample and interferents clean-up volumes and solvent mixes were evaluated for appropriate process performance. Finally, elution of retained metabolites was achieved with LC mobile phases during 5 min.
The composition of LC mobile phases was 5 mM of ammonium formate in water (phase A) and 5 mM of ammonium formate in methanol (phase B). Chromatographic gradient was programmed as follows with a constant flowrate of 0.5 mL min−1: from 85% of phase B, maintained during the initial 2 min, up to 100% of phase B in the next 5 min, conditions that were kept constant for the final 7 min of the chromatography. Furthermore, a post-run of 10 additional min was set to re-establish and equilibrate the initial conditions for the consequent run.
Chromatograph–detector interface parameters were set to 350°C and 9 L min–1 of drying gas (N2), a nebulizer pressure of 50 psi, and 4,500 V of capillary voltage. Detection was carried out in MRM mode. All metabolites detection parameters were studied by direct infusion of individual standard solutions at a concentration of 1 μg mL−1.
Assessment of analytical features of the proposed method was performed according to the Center for Drug Evaluation and Research (CDER) guidelines. Thus, linearity, sensitivity, accuracy, precision, and recovery were characterized. Applicability of the proposed method was also carried out for further use in clinical studies.
Venous blood from all patients was collected in tubes containing EDTA at baseline and 6 months after BS. PBCMs were isolated as previously described (43, 50).
Total RNA from PBMCs was isolated using Direct-zol RNA kit (Zymo Research, Irvine, CA, USA) following manufacturer’s instructions and as previously described (43, 50, 51). The amount of RNA recovered was determined and its quality assessed by the NanoDrop2000 spectrophotometer (Thermo Fisher). Specifically, all the RNA samples passed the quality controls, being 260/280 and 230/260 absorbance ratios among 1.8–2.0. As previously described (43, 52, 53), 1 μg of RNA was reverse transcribed (RT) to cDNA using random hexamer primers with the First Strand Synthesis Kit (Thermo Fisher).
A 48.48 dynamic array based on microfluidic technology (Fluidigm, San Francisco, CA, USA) was developed and implemented to determine, simultaneously, the expression of 48 transcripts in 48 samples, following the same methods previously described (43, 54). Specific primers for human transcripts of the inflammasome machinery including NLR-/NOD-like receptors (n=7), regulators of inflammasome activation (n=15), cytokines and inflammation/apoptosis-related components (n=18), and cell-cycle and DNA-damage regulators (n=5) were used (42). In addition, three housekeeping genes were used. The selection of this panel of genes was based on two main criteria: 1) the relevance of the given inflammasome components and other cell cycle regulators in the inflammatory and apoptotic process and 2) the demonstrated implication in the inflammatory response in metabolic disorders, especially in OB conditions.
Preamplification, exonuclease treatment, and qPCR dynamic array based on microfluidic technology were implemented following manufacturer’s instructions using the Biomark System and the Real-Time PCR Analysis Software (Fluidigm), as previously described (55, 56). The expression level of each transcript was adjusted by a normalization factor (NF) obtained from the expression levels of two different housekeeping genes [beta actin (ACTB) and glyceraldehyde-3-phosphate dehydrogenase (GAPDH)] using Genorm 3.3. This selection was based on the stability of the housekeeping genes analyzed among the experimental groups to be compared, wherein the expression of these two housekeeping genes was not significantly different among groups.
Between-group comparisons were analyzed by the Mann–Whitney U test (non-parametric data), or the Kruskal–Wallis test (non-parametric data, when we compared more than two groups). Paired analysis was performed by Student’s t (parametric data) or Wilcoxon test (non-parametric data). Chi-squared test was used to compare categorical data. Statistical analyses were performed using SPSS statistical software version 20 and Graph Pad Prism version 6. Heatmaps and clustering analysis were performed using MetaboAnalyst (57). Data are expressed as mean ± SD and percentages. p-values <0.05 were considered statistically significant.
Forty patients were evaluated, 55% were women, with a mean age of 45 years. As expected, patients underwent BS due to grade 3 OB (Table 1). Gastric bypass was the most common surgical procedure; only 17% received oral treatment with calcifediol before surgery. Six months after BS, patients presented a significant decrease in body weight and fat mass and increase in the percentage of lean mass (Table 2).
Table 2 Clinical characteristic of the evaluated patients (n=40) according to the presence of metabolic comorbidities at diagnosis.
In clinical practice, routine evaluation of serum calcifediol and C-reactive protein (CRP) levels was performed during follow-up of patients with OB who undergo BS. As expected, serum levels of calcifediol (using a chemiluminescence method) increased 6 months after BS due to oral treatment, while CRP levels significantly decreased (Figure 1A). Calcium levels were normal in all patients (median, 8.7 mg/dl).
Figure 1 Change in serum levels of RCP and calcidiol using chemoluminiscence in patients before and 6 months after bariatric surgery (A). Change in vitamin D metabolites using SPE-LC-MD/MS in patients before and 6 months after bariatric surgery (B). Data represent the median ± interquartile range. Asterisks (***p<0.001) indicate significant changes between the clinical variables. ns, non-significant.
Using SPE-LC-MD/MS, 25(OH)-D3 levels also significantly increased, but most of the cases of extreme values (range, 4.96–173.2 ng/ml) were observed 6 months after BS when compared with the chemiluminescence method (range, 0.11–0.52 ng/ml). Remarkably, 24,25OH OH)2-D3 levels do not significantly change (Figure 1B). Additionally, 1,25(OH)2-D3 levels significantly decreased during follow-up. Despite 24,25(OH)2-D3 was not significantly decreased, VMR was markedly decreased 6 months after BS, in parallel with 1,25(OH)2-D3 levels (Figure 1B).
Figure 2 shows that vitamin D3 levels (but not the other vitamin D metabolites) correlated with fat mass at baseline [median Body Mass Index (BMI), 46.7 kg/m2]. Six months after BS, vitamin D3 correlated with lean mass (median BMI, 36.6 kg/m2), after significant decrease in fat mass and increase in the percentage of lean mass (Tables 1, 2).
Figure 2 Clinical correlations between bioelectrical impedance, anthropometric measurements, and vitamin D metabolites in patients before (A) and 6 months after bariatric surgery (B). Only significant correlations (p<0.05) are presented.
Patients with baseline dyslipidemia (DLP) presented with lower 25(OH)-D3 levels compared with patients without such DLP (Figure 3A). Any other correlation between metabolic comorbidities and vitamin D metabolites was observed at baseline. Remarkably, patients in which DLP and T2DM did not reverse presented with lower vitamin D3 levels (Figure 3B).
Figure 3 Significant clinical–molecular associations between vitamin D metabolites and the presence of baseline hypertension, dyslipidemia, and metabolic comorbidities in patients with OB before surgery (A); clinical–molecular associations between vitamin D metabolites and improvement of hypertension and metabolic comorbidities 6 months after surgery (B). Clinical associations between anthropometric measurements and improvement of metabolic comorbidities at baseline (C) and 6 months after surgery (D). Data represent the median ± interquartile range. Asterisks (*p<0.05) indicate significant changes between the clinical variables.
When anthropometric variables were analyzed, improvement of metabolic comorbidities was associated with increased abdominal circumference at baseline and increased fat-mass difference (Figures 3C, D).
At baseline, fat mass was positively correlated with some key component of the inflammasome machinery, especially with inflammasome-activation components (AIM2, CASP5, CASP6, IL1b, IL18R, JNK2, and P2RX7), and also with the chemokines CCL7, CCL8, and with IKKA, and with the NOD-like receptor1 (NLRP1) (Figure 4A). A negative correlation was also observed with IL1a (Figure 4A). In contrast, lean mass was negatively correlated with IL1R, JNK2, and CCL5.
Figure 4 Clinical correlations between bioelectrical impedance, anthropometric measurements, and the mRNA expression of some inflammasome components in patients before (A) and 6 months after bariatric surgery (B). Only significant correlations (p<0.05) are presented.
Six months after BS, fat mass was negatively correlated with IL18, TLR4, NLRC4, NLRP1, and TGFb. Fat mass did not correlate positively with any inflammasome component. In contrast, BMI positively correlated with IL18, JNK2, P2XR7, NLRP7, and TGFb (Figure 4B).
Patients who had improved hypertension 6 months after surgery presented with increased expression levels of the inflammasome activation components (ASC, P2X7, and IL18R), cytokines (CCL8, CXCL2, IKKA, and IL6R), NOD-like receptors (NLRP1), and cell cycle/DNA regulators (TGFb) (Figure 5A). These patients also presented with increased levels of CASP5 and NFKB and tended to express increased levels of IL1RA, NLRP3, and CDKN2A (Figure 5B).
Figure 5 Significant clinical–molecular associations between the improvement of hypertension (6 months after surgery) and the mRNA expression of some inflammasome components at baseline (A) and 6 months after surgery (B). Data represent the median ± interquartile range. Asterisks (*p<0.05) indicate significant changes between the clinical variables.
When metabolic comorbidities were analyzed (hypertension, T2DM, and DLP), their improvement was associated with increased baseline levels of the inflammasome activation component IL18R (Figure 6A; CASP4 and TGFB tended to be also overexpressed in these patients). Additionally, the improvement of these metabolic comorbidities was also associated with increased expression levels of CCL8 and NLRP3 6 months after BS. Remarkably, CASP4, IKKA, and CDKN2A tended to be overexpressed in these patients (Figure 6B).
Figure 6 Significant clinical–molecular associations between the improvement of metabolic comorbidities and the mRNA expression of inflammasome components at baseline (A) and 6 months after surgery (B). Data represent the median ± interquartile range. Asterisks (*p<0.05) indicate significant changes between the clinical variables.
At baseline, 24,25(OH)2-D3 and VMR positively correlated with key inflammasome machinery components, especially with the inflammasome activation components (CASP1, CASP5, IL1b, IL1RA, and P2X7) and with cell cycle and DNA regulators (ATM, CDKN1B, CDKN2D, and SIRT1). In contrast, 25(OH)-D3 correlated with IL1b, IL1RA, CXCR1, IL6R, SIRT1, and TGFb (Figure 7A).
Figure 7 Clinical correlations between vitamin D metabolites and some inflammasome components in patients before (A) and 6 months after bariatric surgery (B). Only significant correlations (p<0.05) are presented.
Six months after BS, 24,25(OH)2-D3 negatively correlated only with AIM2, and 25(OH)-D3 and VMR did not correlate with inflammasome after BS (Figure 7B).
In the present study, changes in VDES metabolites and relevant components of the inflammasome machinery (and inflammatory-associated factors) were comprehensively evaluated in a well-characterized cohort of patients with severe OB, who underwent BS and received treatment with oral calcifediol during 6 months. Additionally, their relation with metabolic comorbidities was studied. To the best of our knowledge, this is the first report that links important inflammasome components, VDES metabolites, and reversal of metabolic comorbidities in the clinical practice.
Decreased serum levels of 25(OH)-D3 have been widely reported in patients (of all ages) with OB (58, 59). In this cohort, decreased 25(OH)-D3 and vitamin D3 serum levels were associated with the presence of DLP at baseline and 6 months after BS, respectively. These results are in concordance with previous publications, in which vitamin D has been described as a significant independent inverse determinant of total cholesterol and low-density lipoprotein (LDL) and triglycerides (60). Despite this, no correlation or association was observed with lipoprotein (HDL) cholesterol levels, which have been previously described in the literature (61, 62). Remarkably, we did not observe differences in patients who underwent sleeve gastrectomy or gastric bypass, probably due to the fact that all patients received calcifediol at different dosage in order to achieve a sufficiency level (>30 ng/dl).
Additionally, some studies have suggested a link between medical treatment with vitamin D and changes in body composition. Specifically, a 3-month double-blind, randomized clinical trial in women demonstrated that vitamin D supplementation (25 μg/day as vitamin D3) reduced body fat mass regardless of BMI (63). In the same line, a meta-analysis described improvement in BMI and waist circumference following vitamin D supplementation (64). In contrast, we did not observe changes in BMI or body composition in patients according to the circulating 25(OH)-D3 levels at baseline. Similar results have been reported in intervention studies and meta-analyses in healthy population (65) and in patients with OB and after BS (66–68).
It is remarkable that despite a clear association between 25(OH)-D3 deficiency and OB, medical treatment has not been clearly associated with clinical improvement (69). This discordance might be explained by the metabolites measured. Indeed, it is well-known that 25(OH)-D3 is the most commonly measured metabolite (70), but it is frequently decreased in patients with OB due to several mechanisms. Several hypotheses have tried to explain this situation, including the 25(OH)-D3 sequestration by the adipose tissue, changes in vitamin D metabolism, volumetric dilution, or vitamin D receptor polymorphism (71). In this context, it would be valuable to determine another metabolite that could not be influenced by internal or external factors. A previous study of our group has demonstrated that the VMR was an appropriate marker of vitamin D status in patients with severe OB (grade 3 or higher) compared with patients without OB (23). In the present study, 6 months after surgery, serum levels of 25(OH)D significantly increased after oral supplementation with calcifediol, but 24,25(OH)2D3 levels did not change; these findings might be explained by the fact that patients received supplementation with calcifediol, which is a potent supplement with a higher rate of intestinal absorption (when compared with cholecalciferol) (72).
It has been previously described that the enlarged pool of visceral and subcutaneous adipose tissue probably impounds vitamin D and its metabolites, reducing their bioavailability (34). As expected, total levels of vitamin D3 were inversely correlated with fat mass at baseline in our cohort of patients, but this correlation disappeared 6 months after BS.
Curiously, vitamin D3 negatively correlated with lean mass after BS. Similar to this result, previous studies have suggested that there was no effect of vitamin D supplementation on lean mass and muscle strength in elderly subjects (73). Additionally, a double-blind, placebo-controlled randomized clinical trial that compared the administration of 2,000 IU/day oral vitamin D3 or placebo during a lifestyle-based weight loss intervention reported that vitamin D3 supplementation during weight loss decreased leg strength, without affecting lean mass, among postmenopausal women (74). Based on this, we suggest that an ideal study for evaluating the effect of vitamin D supplementation in patients with OB should include the measurement of VDES metabolites (or metabolome) and body composition evaluation in order to avoid confounding results.
As expected, our results demonstrated that inflammation status significantly decreased (reflected in decreased CRP levels) 6 months after BS and calcifediol treatment with normalization of 25(OH)D3 serum levels (in parallel to a significant decrease in body weight, fat mass, and reduction in metabolic comorbidities, as previously reported (75). In this context, improvement in metabolic comorbidities has been associated with fat mass loss after BS (66, 76, 77). In a previous study, we analyzed the effect of vitamin D supplementation on the reversal of metabolic comorbidities in a large cohort of patients after BS (n=346). In that cohort, improvement of comorbidities was independent of the serum levels of 25OHD (66). In contrast, in this study, patients that still presented with DLP or T2DM 6 months after surgery had lower circulating levels of vitamin D3 but no other metabolites. Differences might be explained by the different body composition of the evaluated patients (increased fat mass levels).
Previous studies have reported an association between inflammasome, OB, and fat mass (42, 78). In this cohort, we clearly observe positive correlations between fat mass and key inflammasome machinery components, and negative correlations between lean mass and inflammasome components, in concordance with previous publications that suggest a significant dysregulation of inflammasome components in patients with severe OB (37). For example, it has been described that visceral adipose tissue from patients with metabolic comorbidities show increased expression of IL1β (79), and IL18 has been associated with atherosclerosis and T2DM (80). These interleukins are clearly dysregulated and positively correlated with fat mass at baseline, but IL18 was negatively correlated with fat mass 6 months after BS and calcifediol treatment.
Additionally, the increased expression of inflammasome components in patients with severe OB has been associated with improvement of metabolic comorbidities, suggesting that there is a different inflammasome profile in patients that would significantly improve after BS. Particularly, according to previous studies, patients with essential HT exhibit elevated levels of circulating IL1β. In line with this, pyroptosis and inflammasome have been associated with the development of HT (42, 81). In our cohort, we observed that improvement of HT was associated with increased baseline levels of several key inflammasome components including IL6R, IL18R, and NLRP1.
Probably, NLRP3 is the most studied inflammasome. In fact, NLP3 has been associated with metabolic comorbidities including DLP and T2DM (82–84), and its role and regulation are a matter of debate for improving OB-related comorbidities (37). Remarkably, in our study, the patients with increased expression levels of NLRP3 improved HT and metabolic comorbidities 6 months after BS.
Finally, previous studies have demonstrated that NLRP3 can interact with vitamin D receptor (VDR) (35). Specifically, 1,25(OH)2 D and Vitamin D receptor signaling mechanisms increase the phagocytic ability of monocytes to modulate the innate immune system (85) and promote the ability of dendritic cells to modulate regulatory T-cell differentiation (86, 87). Furthermore, VDR deficiency is associated with increased inflammation and deregulation in several inflammatory diseases, such as inflammatory bowel disease, sepsis, diabetes, and asthma (87). It has been demonstrated that VDR inhibits the deubiquitination of NLRP3 by BRCC3 (35), which is a deubiquitinase with a crucial role for the post-transcriptional activation of NLRP3 (88). Similar results have been observed in peripheral blood monocytes from pregnant women with preeclampsia and in human monocyte cell lines (89). In addition, a preclinical model of OB and asthma showed a significant increase in airway hyper-responsiveness, airway inflammation, pro-inflammatory cytokine levels, mRNA expression of NLRP3 and IL1β in mice with OB, asthma, and lower 25(OH)D levels (90). In our cohort, IL1β and IL6R mRNA expression correlated with 25(OH)-D3 and 24,25(OH)2-D3 circulating levels in patients before BS.
In vitro studies have revealed that treatment with 1,25(OH)2D3 suppressed the expression of NLRP3 inflammasome-related genes and the production of IL1β in human corneal epithelial cells (91). 1,25(OH)2D3 treatment also inhibited caspase-1 activation and IL1β secretion in an ulcerative colitis model (92). Furthermore, treatment with 1,25(OH)2D3- and 25(OH)D3 also induced IL1β release from THP-1 cells in vitro (93). Additionally, it has been demonstrated that 1,25(OH)2VD3 inhibited the nigericin-induced activation step of NLRP1 inflammasome in unprimed keratinocytes (94).
Animal models have shown that treatment with 1,25(OH)2 D3 inactivates the NLRP3 inflammasome; in consequence, treatment with the hormonally active metabolite of VDES decreased surgery-induced neuroinflammation and memory and cognitive dysfunctions in aged mice (95). Treatment with 1,25(OH)2 D3 also exerted protective effects against retinal vascular damage and cell apoptosis especially in mice with type 1 diabetes (96). Furthermore, a strong correlation between increased NLRP3 inflammasome pathway and decreased 1,25(OH)2 D3 concentrations in the vitreous of proliferative diabetic retinopathy patients has been observed, suggesting that vitamin D supplementation may play a key role in preventing, treating, and improving the prognosis of this disease (97). In the present study, a significant reduction in the correlation between vitamin D metabolites and the molecular expression of inflammasome has been shown. Due to confounding factors, this change cannot be attributed to the medical treatment with calcifediol or the bariatric surgery-induced weight loss, exclusively.
This study has some limitations, including the cohort size, the short follow-up, and the absence of a control group for comparing the treatment with calcifediol; additionally, a specific evaluation of physical activity and lifestyle changes was not performed. Despite this, several strengths include the well-characterization of the cohort, the complete evaluation of the metabolites, and the clinical management of the patients according to the current clinical guidelines in BS.
Taken together, the results of the present study reveal new conceptual and functional information in the OB/BS field, with potential clinical implications, by demonstrating a clear dysregulation pattern of key components of the inflammasome machinery and VDES metabolism in patients with OB and in those patients who had improved metabolic OB-related comorbidities after BS and calcifediol treatment. This study provides solid evidence demonstrating that VDES metabolism in combination with some components of the inflammasome machinery might play a critical pathophysiological role in the improvement of OB-related comorbidities, offering a clinically relevant opportunity for novel targets that should be tested in humans. Importantly, these data demonstrated that the expression profile of inflammasome-machinery components can be dynamically modulated in PBMCs after BS and calcifediol treatment, suggesting that this profile could likely serve as a sensor and early predictor of the reversal of OB-related complications after BS. Interventional studies that evaluate vitamin D supplementation, vitamin D metabolites determination, and inflammasome expression should be performed in patients of different races and BMI.
The original contributions presented in the study are included in the article/supplementary material, further inquiries can be directed to the corresponding author/s.
The studies involving humans were approved by Reina Sofia University Hospital Ethics Committee. The studies were conducted in accordance with the local legislation and institutional requirements. The participants provided their written informed consent to participate in this study.
ADH-M and RML. contributed to the design of the study; ADH-M contributed with the analysis and interpretation of data; FP-C, JMQG and RB performed a comprehensive analysis of the results and the manuscript; ADH-M. wrote the draft version of the article; LSC-P, MJM-P, AC-C, AM, JC, MCC, AC-D, MAG-M and MDG, contributed to the data acquisition and approved the final version of the article. All authors have read and agreed to the published version of the manuscript.
This work was funded by Junta de Andalucía (PI-0038-2019, BIO-0139), Instituto de Salud Carlos III, co-funded by European Union (ERDF/ESF, “Investing in your future”: ISCIII-AES-2019/002525; JR/00050), FEIOMM Translational Research Grant (2019), and CIBERobn. CIBER is an initiative of Instituto de Salud Carlos III, Ministerio de Sanidad, Servicios Sociales e Igualdad, Spain.
The authors declare that the research was conducted in the absence of any commercial or financial relationships that could be construed as a potential conflict of interest.
All claims expressed in this article are solely those of the authors and do not necessarily represent those of their affiliated organizations, or those of the publisher, the editors and the reviewers. Any product that may be evaluated in this article, or claim that may be made by its manufacturer, is not guaranteed or endorsed by the publisher.
1. Seidell JC, Halberstadt J. The global burden of obesity and the challenges of prevention. Ann Nutr Metab (2015) 66 Suppl 2:7–12. doi: 10.1159/000375143
2. Gallagher D, Heymsfield SB, Heo M, Jebb SA, Murgatroyd PR, Sakamoto Y. Healthy percentage body fat ranges: an approach for developing guidelines based on body mass index. Am J Clin Nutr (2000) 72:694–701. doi: 10.1093/ajcn/72.3.694
3. Tsai AG, Williamson DF, Glick HA. Direct medical cost of overweight and obesity in the USA: a quantitative systematic review. Obes Rev (2011) 12:50–61. doi: 10.1111/j.1467-789X.2009.00708.x
4. Denis GV, Obin MS. ‘Metabolically healthy obesity’: origins and implications. Mol Aspects Med (2013) 34:59–70. doi: 10.1016/j.mam.2012.10.004
5. Bastien M, Poirier P, Lemieux I, Despres JP. Overview of epidemiology and contribution of obesity to cardiovascular disease. Prog Cardiovasc Dis (2014) 56:369–81. doi: 10.1016/j.pcad.2013.10.016
6. Ligibel JA, Alfano CM, Courneya KS, Demark-Wahnefried W, Burger RA, Chlebowski RT, et al. American Society of Clinical Oncology position statement on obesity and cancer. J Clin Oncol (2014) 32:3568–74. doi: 10.1200/JCO.2014.58.4680
7. Scherer PE, Hill JA. Obesity, diabetes, and cardiovascular diseases: A compendium. Circ Res (2016) 118:1703–5. doi: 10.1161/CIRCRESAHA.116.308999
8. Wolfe BM, Kvach E, Eckel RH. Treatment of obesity: weight loss and bariatric surgery. Circ Res (2016) 118:1844–55. doi: 10.1161/CIRCRESAHA.116.307591
9. Chondronikola M, Harris LL, Klein S. Bariatric surgery and type 2 diabetes: are there weight loss-independent therapeutic effects of upper gastrointestinal bypass? J Intern Med (2016) 280:476–86. doi: 10.1111/joim.12527
10. Abdeen G, le Roux CW. Mechanism underlying the weight loss and complications of roux-en-Y gastric bypass. Review Obes Surg (2016) 26:410–21. doi: 10.1007/s11695-015-1945-7
11. Rubino F, Schauer PR, Kaplan LM, Cummings DE. Metabolic surgery to treat type 2 diabetes: clinical outcomes and mechanisms of action. Annu Rev Med (2010) 61:393–411. doi: 10.1146/annurev.med.051308.105148
12. Catoi AF, Parvu A, Muresan A, Busetto L. Metabolic mechanisms in obesity and type 2 diabetes: insights from bariatric/metabolic surgery. Obes Facts (2015) 8:350–63. doi: 10.1159/000441259
13. Koliaki C, Liatis S, le Roux CW, Kokkinos A. The role of bariatric surgery to treat diabetes: current challenges and perspectives. BMC Endocr Disord (2017) 17:50. doi: 10.1186/s12902-017-0202-6
14. Hafida S, Mirshahi T, Nikolajczyk BS. The impact of bariatric surgery on inflammation: quenching the fire of obesity? Curr Opin Endocrinol Diabetes Obes (2016) 23:373–8. doi: 10.1097/MED.0000000000000277
15. Vranic L, Mikolasevic I, Milic S. Vitamin D deficiency: consequence or cause of obesity? Medicina (Kaunas) (2019) 55(9):541. doi: 10.3390/medicina55090541
16. Wortsman J, Matsuoka LY, Chen TC, Lu Z, Holick MF. Decreased bioavailability of vitamin D in obesity. Am J Clin Nutr (2000) 72:690–3. doi: 10.1093/ajcn/72.3.690
17. Targher G, Bertolini L, Scala L, Cigolini M, Zenari L, Falezza G, et al. Associations between serum 25-hydroxyvitamin D3 concentrations and liver histology in patients with non-alcoholic fatty liver disease. Nutr Metab Cardiovasc Dis (2007) 17:517–24. doi: 10.1016/j.numecd.2006.04.002
18. Walsh JS, Bowles S, Evans AL. Vitamin D in obesity. Curr Opin Endocrinol Diabetes Obes (2017) 24:389–94. doi: 10.1097/MED.0000000000000371
19. Gangloff A, Bergeron J, Lemieux I, Despres JP. Changes in circulating vitamin D levels with loss of adipose tissue. Curr Opin Clin Nutr Metab Care (2016) 19:464–70. doi: 10.1097/MCO.0000000000000315
20. Wamberg L, Christiansen T, Paulsen SK, Fisker S, Rask P, Rejnmark L, et al. Expression of vitamin D-metabolizing enzymes in human adipose tissue – the effect of obesity and diet-induced weight loss. Int J Obes (Lond) (2013) 37:651–7. doi: 10.1038/ijo.2012.112
21. Makris K, Sempos C, Cavalier E. The measurement of vitamin D metabolites part II-the measurement of the various vitamin D metabolites. Hormones (Athens) (2020) 19:97–107. doi: 10.1007/s42000-020-00188-9
22. Bouillon R, Bikle D. Vitamin D metabolism revised: fall of dogmas. J Bone Miner Res (2019) 34:1985–92. doi: 10.1002/jbmr.3884
23. de los Santos Castillo-Peinado L, Calderón-Santiago M, Herrera-Martínez AD, León-Idougourram S, Gálvez-Moreno MÁ, Sánchez-Cano RL, et al. Measuring vitamin D3 metabolic status, comparison between vitamin D deficient and sufficient individuals. Separations (2022) 9(6):141. doi: 10.3390/separations9060141
24. Jones G, Kaufmann M. Diagnostic aspects of vitamin D: clinical utility of vitamin D metabolite profiling. JBMR Plus (2021) 5:e10581. doi: 10.1002/jbm4.10581
25. Botella-Carretero JI, Alvarez-Blasco F, Villafruela JJ, Balsa JA, Vazquez C, Escobar-Morreale HF. Vitamin D deficiency is associated with the metabolic syndrome in morbid obesity. Clin Nutr (2007) 26:573–80. doi: 10.1016/j.clnu.2007.05.009
26. Kheiri B, Abdalla A, Osman M, Ahmed S, Hassan M, Bachuwa G. Vitamin D deficiency and risk of cardiovascular diseases: a narrative review. Clin Hypertens (2018) 24:9. doi: 10.1186/s40885-018-0094-4
27. Melguizo-Rodriguez L, Costela-Ruiz VJ, Garcia-Recio E, De Luna-Bertos E, Ruiz C, Illescas-Montes R. Role of vitamin D in the metabolic syndrome. Nutrients (2021) 13(3):830. doi: 10.3390/nu13030830
28. Lee K, Kim J. Serum vitamin D status and metabolic syndrome: a systematic review and dose-response meta-analysis. Nutr Res Pract (2021) 15:329–45. doi: 10.4162/nrp.2021.15.3.329
29. Weldegiorgis TZ, Hidru TH, Yang XL, Xia YL, Ma L, Li HH. Association between serum 25-hydroxyvitamin D concentrations and metabolic syndrome in the middle-aged and elderly Chinese population in Dalian, northeast China: A cross-sectional study. J Diabetes Investig (2020) 11:184–91. doi: 10.1111/jdi.13086
30. Pludowski P, Holick MF, Pilz S, Wagner CL, Hollis BW, Grant WB, et al. Vitamin D effects on musculoskeletal health, immunity, autoimmunity, cardiovascular disease, cancer, fertility, pregnancy, dementia and mortality-a review of recent evidence. Autoimmun Rev (2013) 12:976–89. doi: 10.1016/j.autrev.2013.02.004
31. Sosa Henriquez M, Gomez de Tejada Romero MJ. La suplementación de calcio y vitamina D en el manejo de la osteoporosis. ¿Cuál es la dosis aconsejable de vitamina D? Rev Osteoporos Metab Miner (2021) 13(2). doi: 10.4321/s1889-836x2021000200006
32. Miao Z, Wang S, Wang Y, Guo L, Zhang J, Liu Y, et al. A potential linking between vitamin D and adipose metabolic disorders. Can J Gastroenterol Hepatol (2020) 2020:2656321. doi: 10.1155/2020/2656321
33. Karkeni E, Marcotorchino J, Tourniaire F, Astier J, Peiretti F, Darmon P, et al. Vitamin D limits chemokine expression in adipocytes and macrophage migration in vitro and in male mice. Endocrinology (2015) 156:1782–93. doi: 10.1210/en.2014-1647
34. Greco EA, Lenzi A, Migliaccio S. Role of hypovitaminosis D in the pathogenesis of obesity-induced insulin resistance. Nutrients (2019) 11(7):1506. doi: 10.3390/nu11071506
35. Rao Z, Chen X, Wu J, Xiao M, Zhang J, Wang B, et al. Vitamin D receptor inhibits NLRP3 activation by impeding its BRCC3-mediated deubiquitination. Front Immunol (2019) 10:2783. doi: 10.3389/fimmu.2019.02783
36. Guo H, Callaway JB, Ting JP. Inflammasomes: mechanism of action, role in disease, and therapeutics. Nat Med (2015) 21:677–87. doi: 10.1038/nm.3893
37. Aura D, Herrera-Martinez VH-A, Pérez-Gómez Jesús, Gahete MD, Luque RaúlM. Inflammasomes: Cause or consequence of obesity-associated comorbidities in humans. Obesity (2022) 30:2351–62. doi: 10.1002/oby.23581
38. Rider P, Carmi Y, Guttman O, Braiman A, Cohen I, Voronov E, et al. IL-1alpha and IL-1beta recruit different myeloid cells and promote different stages of sterile inflammation. J Immunol (2011) 187:4835–43. doi: 10.4049/jimmunol.1102048
39. Takeuchi O, Akira S. Pattern recognition receptors and inflammation. Cell (2010) 140:805–20. doi: 10.1016/j.cell.2010.01.022
40. Vanaja SK, Rathinam VA, Fitzgerald KA. Mechanisms of inflammasome activation: recent advances and novel insights. Trends Cell Biol (2015) 25:308–15. doi: 10.1016/j.tcb.2014.12.009
41. Swanson KV, Deng M, Ting JP. The NLRP3 inflammasome: molecular activation and regulation to therapeutics. Nat Rev Immunol (2019) 19:477–89. doi: 10.1038/s41577-019-0165-0
42. Herrero-Aguayo V, Saez-Martinez P, Lopez-Canovas JL, Prados-Carmona JJ, Alcantara-Laguna MD, Lopez FL, et al. Dysregulation of components of the inflammasome machinery after bariatric surgery: novel targets for a chronic disease. J Clin Endocrinol Metab (2021) 106(12):e4917–34. doi: 10.1210/clinem/dgab586
43. Gahete MD, Del Rio-Moreno M, Camargo A, Alcala-Diaz JF, Alors-Perez E, Delgado-Lista J, et al. Changes in splicing machinery components influence, precede, and early predict the development of type 2 diabetes: from the CORDIOPREV study. EBioMedicine (2018) 37:356–65. doi: 10.1016/j.ebiom.2018.10.056
44. Mata-Granados cF-V JM, Luque de Castro MD, Quesada Gómez JM. Determining the principal metabolites of vitamin D in the blood through on-line solid phase extraction with liquidchromatography-mass spectrometry in tandem. Rev Osteoporos Metab Miner (2010) 2:55–60.
45. Busetto L, Dicker D, Azran C, Batterham RL, Farpour-Lambert N, Fried M, et al. Practical recommendations of the obesity management task force of the european association for the study of obesity for the post-bariatric surgery medical management. Obes Facts (2017) 10:597–632. doi: 10.1159/000481825
46. Di Lorenzo N, Antoniou SA, Batterham RL, Busetto L, Godoroja D, Iossa A, et al. Clinical practice guidelines of the European Association for Endoscopic Surgery (EAES) on bariatric surgery: update 2020 endorsed by IFSO-EC, EASO and ESPCOP. Surg Endosc (2020) 34:2332–58. doi: 10.1007/s00464-020-07555-y
47. Fried M, Yumuk V, Oppert JM, Scopinaro N, Torres A, Weiner R, et al. International Federation for Surgery of O, Metabolic Disorders-European C, et al. Interdisciplinary European guidelines on metabolic and bariatric surgery. Obes Surg (2014) 24:42–55. doi: 10.1007/s11695-013-1079-8
48. O’Kane M, Parretti HM, Pinkney J, Welbourn R, Hughes CA, Mok J, et al. British Obesity and Metabolic Surgery Society Guidelines on perioperative and postoperative biochemical monitoring and micronutrient replacement for patients undergoing bariatric surgery-2020 update. Obes Rev (2020) 21:e13087. doi: 10.1111/obr.13087
49. Eisenberg D, Shikora SA, Aarts E, Aminian A, Angrisani L, Cohen RV, et al. 2022 American society of metabolic and bariatric surgery (ASMBS) and international federation for the surgery of obesity and metabolic disorders (IFSO) indications for metabolic and bariatric surgery. Obes Surg (2022) 33(1):3–14. doi: 10.1007/s11695-022-06332-1
50. Del Rio-Moreno M, Alors-Perez E, Gonzalez-Rubio S, Ferrin G, Reyes O, Rodriguez-Peralvarez M, et al. Dysregulation of the splicing machinery is associated to the development of nonalcoholic fatty liver disease. J Clin Endocrinol Metab (2019) 104:3389–402. doi: 10.1210/jc.2019-00021
51. Del Rio-Moreno M, Luque RM, Rangel-Zuniga OA, Alors-Perez E, Alcala-Diaz JF, Roncero-Ramos I, et al. Dietary intervention modulates the expression of splicing machinery in cardiovascular patients at high risk of type 2 diabetes development: from the CORDIOPREV study. Nutrients (2020) 12(11):3528. doi: 10.3390/nu12113528
52. Vazquez-Borrego MC, Fuentes-Fayos AC, Herrera Martinez AD, LL F, Ibanez-Costa A, Moreno Moreno P, et al. Biguanides exert antitumoral actions in pituitary tumor cells through AMPK-dependent and -independent mechanisms. J Clin Endocrinol Metab (2019) 104(8):3501–13. doi: 10.1210/jc.2019-00056
53. Herrera-Martinez AD, Pedraza-Arevalo S, L-López F, Gahete MD, Galvez-Moreno MA, Castano JP, et al. Type 2 diabetes in neuroendocrine tumors: are biguanides and statins part of the solution? J Clin Endocrinol Metab (2018) 104(1):57–73. doi: 10.1210/jc.2018-01455
54. Jimenez-Vacas JM, Herrero-Aguayo V, Montero-Hidalgo AJ, Gomez-Gomez E, Fuentes-Fayos AC, Leon-Gonzalez AJ, et al. Dysregulation of the splicing machinery is directly associated to aggressiveness of prostate cancer. EBioMedicine (2020) 51:102547. doi: 10.1016/j.ebiom.2019.11.008
55. Jimenez-Vacas JM, Herrero-Aguayo V, Montero-Hidalgo AJ, Saez-Martinez P, Gomez-Gomez E, Leon-Gonzalez AJ, et al. Clinical, cellular, and molecular evidence of the additive antitumor effects of biguanides and statins in prostate cancer. J Clin Endocrinol Metab (2021) 106:e696–710. doi: 10.1210/clinem/dgaa877
56. Fuentes-Fayos AC, Vazquez-Borrego MC, Jimenez-Vacas JM, Bejarano L, Pedraza-Arevalo S, LL F, et al. Splicing machinery dysregulation drives glioblastoma development/aggressiveness: oncogenic role of SRSF3. Brain (2020) 143:3273–93. doi: 10.1093/brain/awaa273
57. Xia J, Wishart DS. Using metaboAnalyst 3.0 for comprehensive metabolomics data analysis. Curr Protoc Bioinf (2016) 55:14 10 11–14 10 91. doi: 10.1002/cpbi.11
58. Bindayel IA. Effect of age and body mass index on vitamin D level in children with asthma in Riyadh. Sci Rep (2021) 11:11522. doi: 10.1038/s41598-021-91108-3
59. Lagunova Z, Porojnicu AC, Lindberg F, Hexeberg S, Moan J. The dependency of vitamin D status on body mass index, gender, age and season. Anticancer Res (2009) 29:3713–20. doi: 10.14341/2071-8713-4886
60. Glueck CJ, Jetty V, Rothschild M, Duhon G, Shah P, Prince M, et al. Associations between serum 25-hydroxyvitamin D and lipids, lipoprotein cholesterols, and homocysteine. N Am J Med Sci (2016) 8:284–90. doi: 10.4103/1947-2714.187137
61. Jorde R, Grimnes G. Exploring the association between serum 25-hydroxyvitamin D and serum lipids-more than confounding? Eur J Clin Nutr (2018) 72:526–33. doi: 10.1038/s41430-018-0088-z
62. Mai XM, Videm V, Sheehan NA, Chen Y, Langhammer A, Sun YQ. Potential causal associations of serum 25-hydroxyvitamin D with lipids: a Mendelian randomization approach of the HUNT study. Eur J Epidemiol (2019) 34:57–66. doi: 10.1007/s10654-018-0465-x
63. Salehpour A, Hosseinpanah F, Shidfar F, Vafa M, Razaghi M, Dehghani S, et al. A 12-week double-blind randomized clinical trial of vitamin D(3) supplementation on body fat mass in healthy overweight and obese women. Nutr J (2012) 11:78. doi: 10.1186/1475-2891-11-78
64. Perna S. Is vitamin D supplementation useful for weight loss programs? A systematic review and meta-analysis of randomized controlled trials. Medicina (Kaunas) (2019) 55(7):368. doi: 10.3390/medicina55070368
65. Duan L, Han L, Liu Q, Zhao Y, Wang L, Wang Y. Effects of vitamin D supplementation on general and central obesity: results from 20 randomized controlled trials involving apparently healthy populations. Ann Nutr Metab (2020) 76:153–64. doi: 10.1159/000507418
66. León S, Alcántara Laguna M, Molina Puerta MJ, Gálvez Morenos MA, Herrera-Martínez AD. 25-OH-vitamin D and reversal of metabolic comorbidities associated with obesity after bariatric surgery. Rev Osteoporos Metab Miner (2022) 14:42–7. doi: 10.4321/s1889-836x2022000100005
67. Golzarand M, Hollis BW, MirmIran P, Wagner CL, Shab-Bidar S. Vitamin D supplementation and body fat mass: a systematic review and meta-analysis. Eur J Clin Nutr (2018) 72:1345–57. doi: 10.1038/s41430-018-0132-z
68. Pathak K, Soares MJ, Calton EK, Zhao Y, Hallett J. Vitamin D supplementation and body weight status: a systematic review and meta-analysis of randomized controlled trials. Obes Rev (2014) 15:528–37. doi: 10.1111/obr.12162
69. Park JE, Pichiah PBT, Cha YS. Vitamin D and metabolic diseases: growing roles of vitamin D. J Obes Metab Syndr (2018) 27:223–32. doi: 10.7570/jomes.2018.27.4.223
70. Holick MF. Vitamin D status: measurement, interpretation, and clinical application. Ann Epidemiol (2009) 19:73–8. doi: 10.1016/j.annepidem.2007.12.001
71. Bennour I, Haroun N, Sicard F, Mounien L, Landrier JF. Vitamin D and obesity/adiposity-A brief overview of recent studies. Nutrients (2022) 14(10):2049. doi: 10.3390/nu14102049
72. Quesada-Gomez JM, Bouillon R. Is calcifediol better than cholecalciferol for vitamin D supplementation? Osteoporos Int (2018) 29:1697–711. doi: 10.1007/s00198-018-4520-y
73. Marantes I, Achenbach SJ, Atkinson EJ, Khosla S, Melton LJ 3rd, Amin S. Is vitamin D a determinant of muscle mass and strength? J Bone Miner Res (2011) 26:2860–71. doi: 10.1002/jbmr.510
74. Mason C, Tapsoba JD, Duggan C, Imayama I, Wang CY, Korde L, et al. Effects of vitamin D3 supplementation on lean mass, muscle strength, and bone mineral density during weight loss: A double-blind randomized controlled trial. J Am Geriatr Soc (2016) 64:769–78. doi: 10.1111/jgs.14049
75. Nedeljkovic-Arsenovic O, Banovic M, Radenkovic D, Rancic N, Polovina S, Micic D, et al. The amount of weight loss six months after bariatric surgery: it makes a difference. Obes Facts (2019) 12:281–90. doi: 10.1159/000499387
76. Toghaw P, Matone A, Lenbury Y, De Gaetano A. Bariatric surgery and T2DM improvement mechanisms: a mathematical model. Theor Biol Med Model (2012) 9:16. doi: 10.1186/1742-4682-9-16
77. Jurowich C, Thalheimer A, Hartmann D, Bender G, Seyfried F, Germer CT, et al. Improvement of type 2 diabetes mellitus (T2DM) after bariatric surgery–who fails in the early postoperative course? Obes Surg (2012) 22:1521–6. doi: 10.1007/s11695-012-0676-2
78. Barra NG, Henriksbo BD, Anhe FF, Schertzer JD. The NLRP3 inflammasome regulates adipose tissue metabolism. Biochem J (2020) 477:1089–107. doi: 10.1042/BCJ20190472
79. Esser N, L’Homme L, De Roover A, Kohnen L, Scheen AJ, Moutschen M, et al. Obesity phenotype is related to NLRP3 inflammasome activity and immunological profile of visceral adipose tissue. Diabetologia (2013) 56:2487–97. doi: 10.1007/s00125-013-3023-9
80. Thorand B, Kolb H, Baumert J, Koenig W, Chambless L, Meisinger C, et al. Elevated levels of interleukin-18 predict the development of type 2 diabetes: results from the MONICA/KORA Augsburg Study, 1984-2002. Diabetes (2005) 54:2932–8. doi: 10.2337/diabetes.54.10.2932
81. De Miguel C, Pelegrin P, Baroja-Mazo A, Cuevas S. Emerging role of the inflammasome and pyroptosis in hypertension. Int J Mol Sci (2021) 22(3):1064. doi: 10.3390/ijms22031064
82. Bando S, Fukuda D, Soeki T, Nishimoto S, Uematsu E, Matsuura T, et al. Expression of NLRP3 in subcutaneous adipose tissue is associated with coronary atherosclerosis. Atherosclerosis (2015) 242:407–14. doi: 10.1016/j.atherosclerosis.2015.07.043
83. Yin Z, Deng T, Peterson LE, Yu R, Lin J, Hamilton DJ, et al. Transcriptome analysis of human adipocytes implicates the NOD-like receptor pathway in obesity-induced adipose inflammation. Mol Cell Endocrinol (2014) 394:80–7. doi: 10.1016/j.mce.2014.06.018
84. Serena C, KeIran N, CePeruelo-Mallafre V, Ejarque M, Fradera R, Roche K, et al. Obesity and type 2 diabetes alters the immune properties of human adipose derived stem cells. Stem Cells (2016) 34:2559–73. doi: 10.1002/stem.2429
85. Shin DM, Yuk JM, Lee HM, Lee SH, Son JW, Harding CV, et al. Mycobacterial lipoprotein activates autophagy via TLR2/1/CD14 and a functional vitamin D receptor signalling. Cell Microbiol (2010) 12:1648–65. doi: 10.1111/j.1462-5822.2010.01497.x
86. Carlberg C, Campbell MJ. Vitamin D receptor signaling mechanisms: integrated actions of a well-defined transcription factor. Steroids (2013) 78:127–36. doi: 10.1016/j.steroids.2012.10.019
87. Dimitrov V, White JH. Vitamin D signaling in intestinal innate immunity and homeostasis. Mol Cell Endocrinol (2017) 453:68–78. doi: 10.1016/j.mce.2017.04.010
88. Juliana C, Fernandes-Alnemri T, Kang S, Farias A, Qin F, Alnemri ES. Non-transcriptional priming and deubiquitination regulate NLRP3 inflammasome activation. J Biol Chem (2012) 287:36617–22. doi: 10.1074/jbc.M112.407130
89. Matias ML, Romao-Veiga M, Ribeiro VR, Nunes PR, Gomes VJ, Devides AC, et al. Progesterone and vitamin D downregulate the activation of the NLRP1/NLRP3 inflammasomes and TLR4-MyD88-NF-kappaB pathway in monocytes from pregnant women with preeclampsia. J Reprod Immunol (2021) 144:103286. doi: 10.1016/j.jri.2021.103286
90. Zhang JH, Chen YP, Yang X, Li CQ. Vitamin D3 levels and NLRP3 expression in murine models of obese asthma: association with asthma outcomes. Braz J Med Biol Res (2017) 51:e6841. doi: 10.1590/1414-431X20176841
91. Dai Y, Zhang J, Xiang J, Li Y, Wu D, Xu J. Calcitriol inhibits ROS-NLRP3-IL-1beta signaling axis via activation of Nrf2-antioxidant signaling in hyperosmotic stress stimulated human corneal epithelial cells. Redox Biol (2019) 21:101093. doi: 10.1016/j.redox.2018.101093
92. Cao R, Ma Y, Li S, Shen D, Yang S, Wang X, et al. 1,25(OH)(2) D(3) alleviates DSS-induced ulcerative colitis via inhibiting NLRP3 inflammasome activation. J Leukoc Biol (2020) 108:283–95. doi: 10.1002/JLB.3MA0320-406RR
93. Tulk SE, Liao KC, Muruve DA, Li Y, Beck PL, MacDonald JA. Vitamin D(3) metabolites enhance the NLRP3-dependent secretion of IL-1beta from human THP-1 monocytic cells. J Cell Biochem (2015) 116:711–20. doi: 10.1002/jcb.24985
94. Nakajo T, Katayoshi T, Kitajima N, Tsuji-Naito K. 1,25-Dihydroxyvitamin D(3) attenuates IL-1beta secretion by suppressing NLRP1 inflammasome activation by upregulating the NRF2-HO-1 pathway in epidermal keratinocytes. Redox Biol (2021) 48:102203. doi: 10.1016/j.redox.2021.102203
95. Jingyan Jiang XH, Gao X, Shenghui Yu. Vitamin D3 supplementation attenuates surgery-induced neuroinflammation and cognitive impairment by regulating NLRP3 inflammasome in mice. Mediators Inflamm (2022) 2022:4696415. doi: 10.1155/2022/4696415
96. Lu L, Lu Q, Chen W, Li J, Li C, Zheng Z. Vitamin D(3) protects against diabetic retinopathy by inhibiting high-glucose-induced activation of the ROS/TXNIP/NLRP3 inflammasome pathway. J Diabetes Res (2018) 2018:8193523. doi: 10.1155/2018/8193523
Keywords: obesity, bariatric surgery, inflammasome, vitamin D, comorbidities
Citation: Herrera-Martínez AD, Castillo-Peinado LLS, Molina-Puerta MJ, Calañas-Continente A, Membrives A, Castilla J, Camacho Cardenosa M, Casado-Díaz A, Gálvez-Moreno MA, Gahete MD, Quesada Gómez JM, Bouillon R, Priego-Capote F and Luque RM (2023) Bariatric surgery and calcifediol treatment, Gordian knot of severe-obesity-related comorbidities treatment. Front. Endocrinol. 14:1243906. doi: 10.3389/fendo.2023.1243906
Received: 21 June 2023; Accepted: 11 August 2023;
Published: 06 October 2023.
Edited by:
Ziad H. Al-Oanzi, Clinical Laboratory Science, Saudi ArabiaReviewed by:
Saeed Alsareii, Najran University, Saudi ArabiaCopyright © 2023 Herrera-Martínez, Castillo-Peinado, Molina-Puerta, Calañas-Continente, Membrives, Castilla, Camacho Cardenosa, Casado-Díaz, Gálvez-Moreno, Gahete, Quesada Gómez, Bouillon, Priego-Capote and Luque. This is an open-access article distributed under the terms of the Creative Commons Attribution License (CC BY). The use, distribution or reproduction in other forums is permitted, provided the original author(s) and the copyright owner(s) are credited and that the original publication in this journal is cited, in accordance with accepted academic practice. No use, distribution or reproduction is permitted which does not comply with these terms.
*Correspondence: Aura D. Herrera-Martínez, YXVyaXRhLmRobUBnbWFpbC5jb20=; Raúl M. Luque, YmMybHVodXJAdWNvLmVz
Disclaimer: All claims expressed in this article are solely those of the authors and do not necessarily represent those of their affiliated organizations, or those of the publisher, the editors and the reviewers. Any product that may be evaluated in this article or claim that may be made by its manufacturer is not guaranteed or endorsed by the publisher.
Research integrity at Frontiers
Learn more about the work of our research integrity team to safeguard the quality of each article we publish.