- 1Department of Pediatric Surgery, Maternal and Child Hospital of Hubei Province, Tongji Medical College, Huazhong University of Science and Technology, Wuhan, Hubei, China
- 2School of Medicine, Wuhan University of Science and Technology, Wuhan, Hubei, China
Objective: Type 2 diabetes mellitus (T2DM) is an endocrine-related disease with an increasing incidence worldwide. Male sexual dysfunction is common in diabetic patients. Therefore, we designed a Mendelian randomization (MR) study to investigate the association of type 2 diabetes and 3 glycemic traits with testosterone levels.
Methods: Uncorrelated single nucleotide polymorphisms (SNPs) associated with T2DM (N = 228), fasting insulin (N = 38), fasting glucose (N = 71), and HbA1c (N = 75) at the genome-wide significance were selected as instrument variables. Genetic associations with testosterone levels (total testosterone, TT, bioavailable testosterone, BT, and sex hormone-binding globulin, SHBG) were obtained from the UK Biobank studies and other large consortia. Two-sample MR analysis was used to minimize the bias caused by confounding factors and response causality. Multivariable MR analysis was performed using Body mass index (BMI), Triglycerides (TG), LDL cholesterol (LDL), and adiponectin to adjust for the effects of potential confounders.
Results: Type 2 diabetes mellitus was associated with the decrease of total testosterone (β: -0.021,95%CI: -0.032, -0.010, p<0.001) and sex hormone binding globulin (β: -0.048,95%CI: -0.065, -0.031, p<0.001). In males, total testosterone (β: 0.058, 95% CI: 0.088, 0.028, p < 0.001) decreased. In females, it was associated with an increase in bioavailable testosterone (β: 0.077,95%CI: 0.058,0.096, p<0.001). Each unit (pmol/L) increase in fasting insulin was associated with 0.283nmol/L decrease in sex hormone-binding globulin (95%CI: -0.464, -0.102, p=0.002) and 0.260nmol/L increase in bioavailable testosterone (95%CI: -0.464, -0.102, p= 0.002). In males, sex hormone binding globulin decreased by 0.507nmol/L (95%CI: -0.960, -0.054, p= 0.028) and bioavailable testosterone increased by 0.216nmol/L (95%CI: 0.087,0.344, p= 0.001). In females, sex hormone binding globulin decreased by 0.714 nmol/L (95%CI: -1.093, -0.335, p<0.001) and bioavailable testosterone increased by 0.467nmol/L (95%CI: 0.286,0.648, p<0.001). Each unit (%) increase in HbA1c was associated with 0.060nmol/L decrease in sex hormone-binding globulin (95%CI: -0.113, -0.007, p= 0.026). In males, total testosterone decreased by 0.171nmol/L (95%CI: -0.288, -0.053, p=0.005) and sex hormone binding globulin decreased by 0.206nmol/L (95%CI: -0.340, -0.072, p=0.003). Total testosterone increased by 0.122nmol/L (95%CI: 0.012,0.233, p=0.029) and bioavailable testosterone increased by 0.163nmol/L (95%CI: 0.042,0.285, p=0.008) in females.
Conclusions: Using MR Analysis, we found independent effects of type 2 diabetes, fasting insulin, and HbA1c on total testosterone and sex hormone-binding globulin after maximum exclusion of the effects of obesity, BMI, TG, LDL and Adiponectin.
Introduction
With the improvement of nutritional status, type 2 diabetes mellitus has gradually become one of the major threats to human health (1). The number of adults with diabetes increased from 108 million to 422 million between 1980 and 2014 (2), with type 2 diabetes accounting for more than 90% of these cases (3). Diabetes has become one of the top three diseases in the world, and the global prevalence is increasing (4). Traditional cross-sectional studies suggest that gonadal function and testosterone levels are decreased in 33%-57% of patients with type 2 diabetes (5). At present, there are clinical studies on the intervention of blood glucose status in type 2 diabetic patients by exogenous testosterone supplementation (6). However, observational studies have limitations such as misclassification and reverse causality. At the same time, type 2 diabetes is a chronic metabolic disorder with a long course and early occult symptoms, so the causal relationship between type 2 diabetes and testosterone level is still unclear (7). There are gender differences in testosterone levels, and the effect of type 2 diabetes on testosterone levels in different genders is still unclear. The effects of various glycemic traits on testosterone levels are still controversial. The effect of different diabetes treatment regimens on altered testosterone levels is unknown. We aimed to investigate these issues by means of Mendelian randomization.
Mendelian randomization (MR) is an epidemiological method that enables causal inference by using single nucleotide polymorphisms (SNPs) as instrumental variables of exposure (8). Compared with observational studies, MR can reduce confounding bias because the genetic alleles are randomly aligned at conception and therefore not correlated with environmental and acquired factors. Because the genotype cannot be altered by disease, the MR design prevents reverse causality (9). Here, we performed a two-sample MR study to comprehensively examine the association of type 2 diabetes and glycemic traits (fasting insulin, fasting glucose, and HbA1c) with testosterone levels.
Method
This two-sample MR analysis was designed to investigate type 2 diabetes and glycemic traits on testosterone levels, including total testosterone (TT), bioavailable testosterone (BT) and sex hormone-binding globulin (SHBG). Additional studies were conducted to investigate the effects of different diabetes treatment regimens on testosterone levels. This study was based on genome-wide association studies (GWAS) and publicly available data from the UK Biobank database and other large consortia, with no overlap between study populations. The included studies were approved by the relevant ethical review boards, and informed consent was obtained from participants (Figure 1).
Selection of instrumental variables and data sources
Type 2 diabetes and glycemic traits (fasting insulin, fasting glucose, and HbA1c).
SNPs associated with type 2 diabetes and glycemic traits (fasting insulin, fasting glucose, and HbA1c) were selected from GWAS meta-analyses. These included 228,499 cases and 1,178,783 controls from the Million Soldier Retirement Program, DIAMANTE, Japan Bank of Biotechnology, and other studies (10). We selected SNPs with P<5*10-8 and calculated linkage disequilibrium between SNPs at each exposure by using the PLINK clustering method based on the 1000 Genomes European panel. SNPs with linkage disequilibrium (defined as R2 < 0.001 and clumping distance = 5000 kb) were excluded. Five SNPs that were also associated with obesity (11) and three SNPs associated with BMI were removed, leaving 228 SNPs to be used as instrumental variables for type 2 diabetes. 38 SNPs were used for fasting insulin, 71 SNPs for fasting glucose, and 75 SNPs for HbA1c.
Body mass index
Pooled data on BMI were obtained from a meta-analysis (12) of 23 studies, which involves 32,161 individuals of European individuals whose populations did not overlap with the UK Biobank.
Triglycerides and LDL cholesterol
Pooled data on TG and LDL were obtained from a meta-analysis (13) of 23 studies involving 94,595 individuals of European individuals, whose populations did not overlap with the UK Biobank.
Adiponectin
The aggregate-level data on adiponectin were derived from a meta-analysis (14) of 16 studies involving 45,891 European individuals whose study populations did not overlap with the UK Biobank data.
Medications for diabetes
A total of four different drugs were selected for analysis, namely insulin, metformin, blood pressure medication, and statin medication. Data on insulin and statin therapy were obtained from FinnGen, which included 218,792 European individuals (15, 16). Data on metformin and blood pressure medication were obtained from the UK Biobank, which included 462,933 and 249,710 European individuals, respectively (17, 18).
Testosterone levels
SNPs for TT, BT, and SHBG levels were obtained from publicly available summary statistics (19). The database uses UK Biobank data, which includes phenotypic and biospecimens collected from approximately 500,000 individuals across the United Kingdom (20). Testosterone and SHBG levels (nmol/L) were measured in 230,454 and 189,473 participants, respectively, using a one-step competition assay and a two-step sandwich immunoassay. BT (nmol/L) was calculated from total testosterone and albumin, which was also measured by BCG analysis on a Beckman Coulter AU5800.
All data sources and instrumental variables are summarized in Supplementary Tables 1, 2.
Statistical analysis
Inverse-variance weighted (IVW) was used as the main MR method to estimate the causal relationship of type 2 diabetes and glycemic traits with testosterone levels. We used a random-effects model for all MR analyses because of the heterogeneity among the databases used. Simultaneously, sensitivity tests were also performed by weighted median, MR-Egger and MR-PRESSO to test the robustness of the results and verify horizontal pleiotropy. If more than 50% of the weights are derived from valid SNPs, the weighted median method can provide valid MR estimates. The embedded intercept of the MR-Egger regression analysis was used to detect horizontal pleiotropy and to provide correct estimates after correction for pleiotropy effects. Using MR-PRESSO to detect and correct for possible outliers, the MR-PRESSO global test can be used to estimate level pleiotropy caused by SNPs heterogeneity (21). Horizontal pleiotropy that could distort causal inferences was assessed by three sensitivity tests and the MR-Egger intercept test. The strength of IVs was assessed by calculating the F-statistic using the formula F= R2× (N −1 −K)/(1−R2) ×K, where R2 represents the proportion of variance in the exposure explained by the genetic variants, N represents sample size, and K represents the number of instruments (22). If the corresponding F-statistic was >10, it was considered that there was no significant weak instrumental bias (22). The power of the MR estimates was calculated using the online calculator tool (23) provided by Stephen Burgess (24).
Heterogeneity of VIs was calculated using Cochran’s Q statistic. At the same time, the potential sources of heterogeneity were identified by “leave-one-out” analysis for each SNPs.
Testosterone levels were defined as continuous variables, and β was used to assess the effect of type 2 diabetes and related glycemic measures on testosterone levels. All analyses were two-tailed. We used the R packages TwoSampleMR and MRPRESSO in R 4.1.3 for the analysis.
Result
The study involved three different populations, i.e., patients with type 2 diabetes regardless of gender, male patients with diabetes and female patients with diabetes.
The relationship between the gene-predicted type 2 diabetes and testosterone level (nmol/L) was as follows (Supplementary Table 3).
Overall, type 2 diabetes was associated with decreased total testosterone (β: -0.021,95%CI: -0.032, -0.010, p<0.001) and sex hormone binding globulin (β: -0.048, 95%CI: -0.065, -0.031, p<0.001).
Similar trends were found in studies that differentiated between genders. In male patients with type 2 diabetes, total testosterone (β: -0.058, 95%CI: -0.088, -0.028, p<0.001) and sex hormone binding globulin (β: -0.097, 95%CI: -0.134, -0.060, p<0.001) decreased. In female patients with type 2 diabetes, sex hormone binding globulin (β: -0.154, 95%CI: -0.193, -0.116, p<0.001) decreased, but bioavailable testosterone (β: 0.077, 95%CI: 0.058, 0.096, p<0.001) showed the opposite trend (Figure 2).
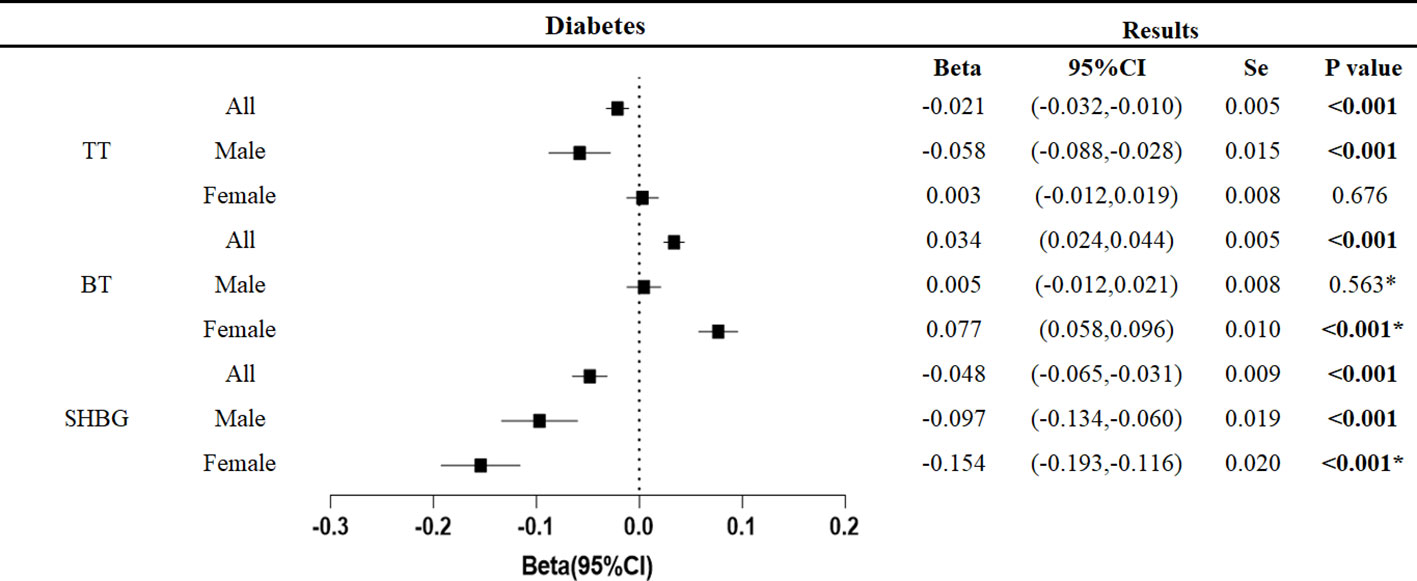
Figure 2 Associations of genetic liability to the type 2 diabetes mellitus with testosterone. P values are for ORs (95% CIs). Se is standard error. * The P value for pleiotropy is less than 0.05.
Pleiotropy analysis showed pleiotropic effects in male bioavailable testosterone, female bioavailable testosterone, and female sex hormone-binding globulin. This persisted even after exclusion of outliers by MR-PRESSO test and hence making the results of these three studies unreliable. We excluded these indicators in the analyses below. All but the three studies did not detect pleiotropy, and the results were robust with narrowed confidence intervals after exclusion of outliers by MR-PRESSO testing.
The results were robust after adjustment for BMI, TG, LDL, and adiponectin, with no significant change in the overall trend. The results are summarized in the Supplementary Table 4.
Glycemic traits and testosterone levels
The relationship between the gene-predicted increase in fasting insulin level per unit (pmol/L) and testosterone level (nmol/L) was as follows (Figure 3).
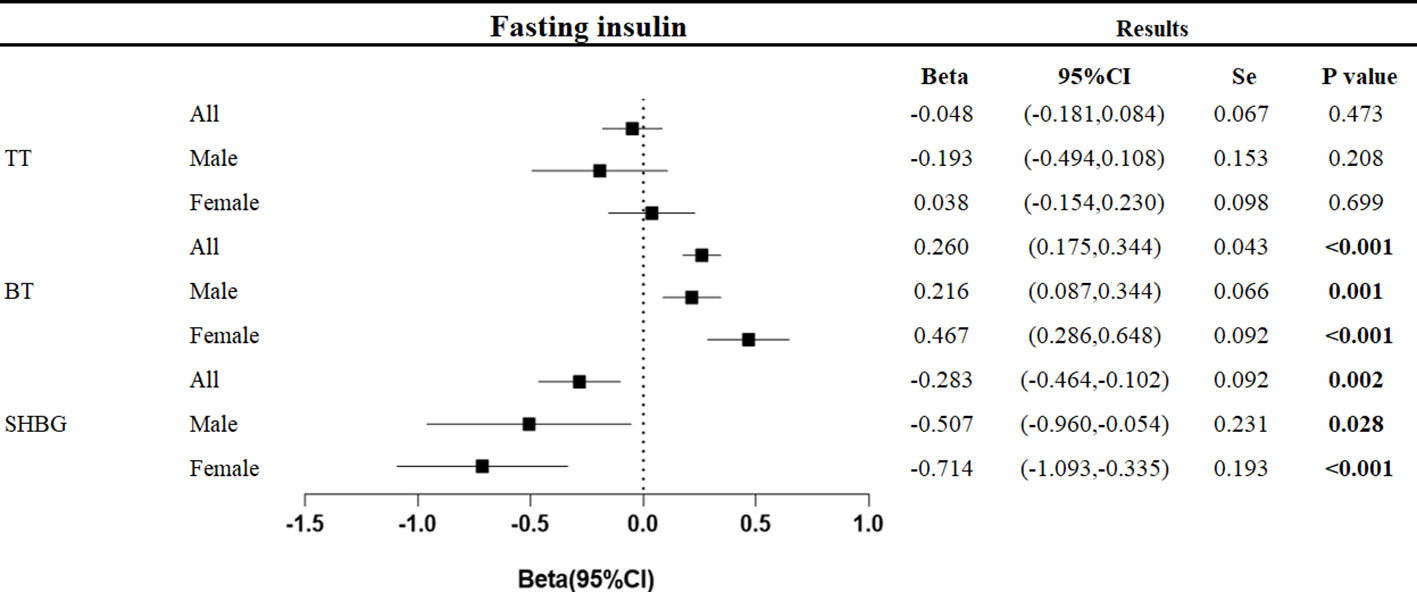
Figure 3 Associations of genetic liability to fasting insulin with testosterone. Beta is the relationship between per unit increase in fasting insulin (pmol/L) and testosterone level (nmol/L). Se is standard error. P values are for ORs (95% CIs). * The P value for pleiotropy is less than 0.05.
At the overall level, each unit increase in fasting insulin was associated with a decrease in sex hormone-binding globulin of 0.283 nmol/L (95%CI: -0.464, -0.102, p=0.002) and an increase in bioavailable testosterone of 0.260 nmol/L (95%CI: 0.175,0.344, p<0.001).
In men, each unit increase in fasting insulin was associated with a decrease in sex hormone-binding globulin of 0.507 nmol/L (95%CI: -0.960, -0.054, p=0.028) and an increase in bioavailable testosterone of 0.216 nmol/L (95%CI: 0.087, 0.344, p<0.001). In women, each unit increase in fasting insulin level was associated with a 0.714 nmol/L decrease in sex hormone-binding globulin (95%CI: -1.093, -0.335, p<0.001) and a 0.467 nmol/L increase in bioavailable testosterone (95%CI: 0.286, 0.648, p<0.001).
The relationship between the genetically predicted increase in HbA1c per unit (%) and testosterone level (nmol/L) was as follows (Figure 4).
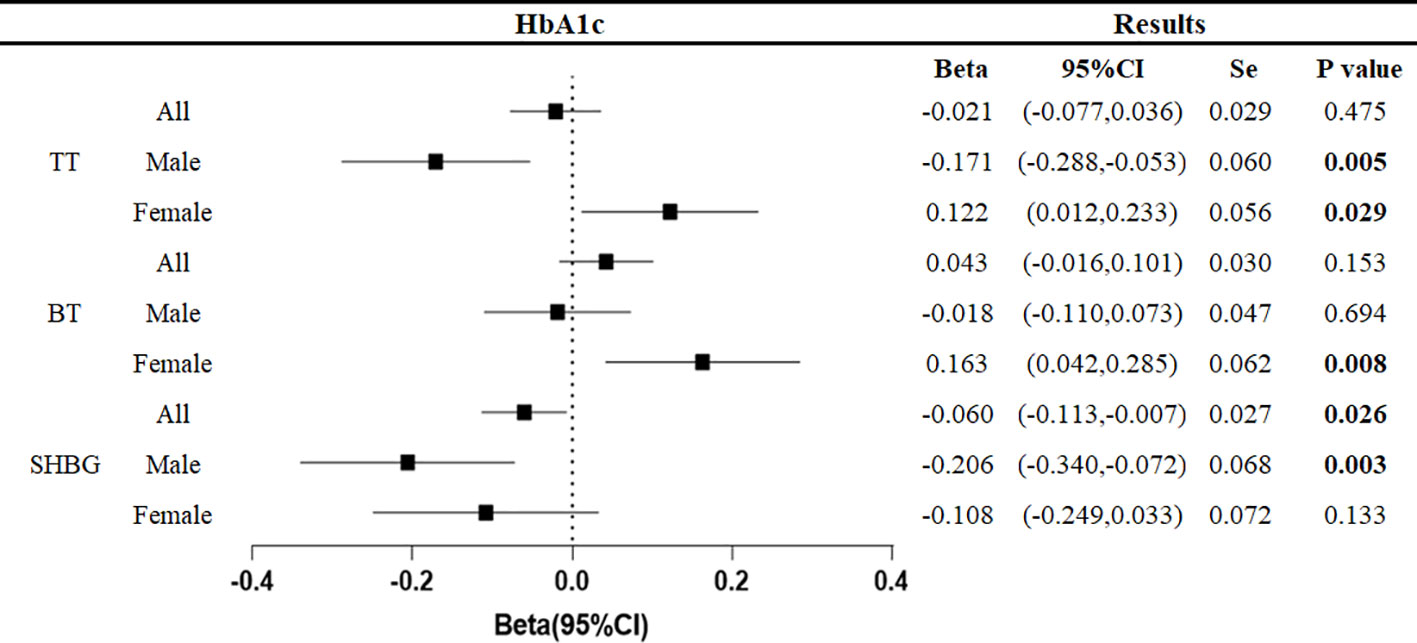
Figure 4 Associations of genetic liability to HbA1c with testosterone. Beta is the relationship between per unit increase in HbA1c (%) and testosterone level (nmol/L). Se is standard error. P values are for ORs (95% CIs). * The P value for pleiotropy is less than 0.05.
At the overall level, each unit increase in HbA1c was associated with a 0.060 nmol/L decrease in sex hormone binding globulin (95%CI: -0.113, -0.007, p=0.026).
In men, each unit increase in HbA1c was associated with a decrease in total testosterone of 0.171 nmol/L (95%CI: -0.055, -0.053, p=0.005) and sex hormone-binding globulin of 0.206 nmol/L (95%CI: -0.340, -0.072, p=0.003). In women, each unit increase in HbA1c was associated with 0.122 nmol/L (95%CI: 0.012,0.233, p=0.029) increase in total testosterone and 0.163 nmol/L (95%CI: 0.042,0.285, p=0.008) increase in bioavailable testosterone.
No effect of fasting glucose on testosterone levels was found in this study (Figure 5).
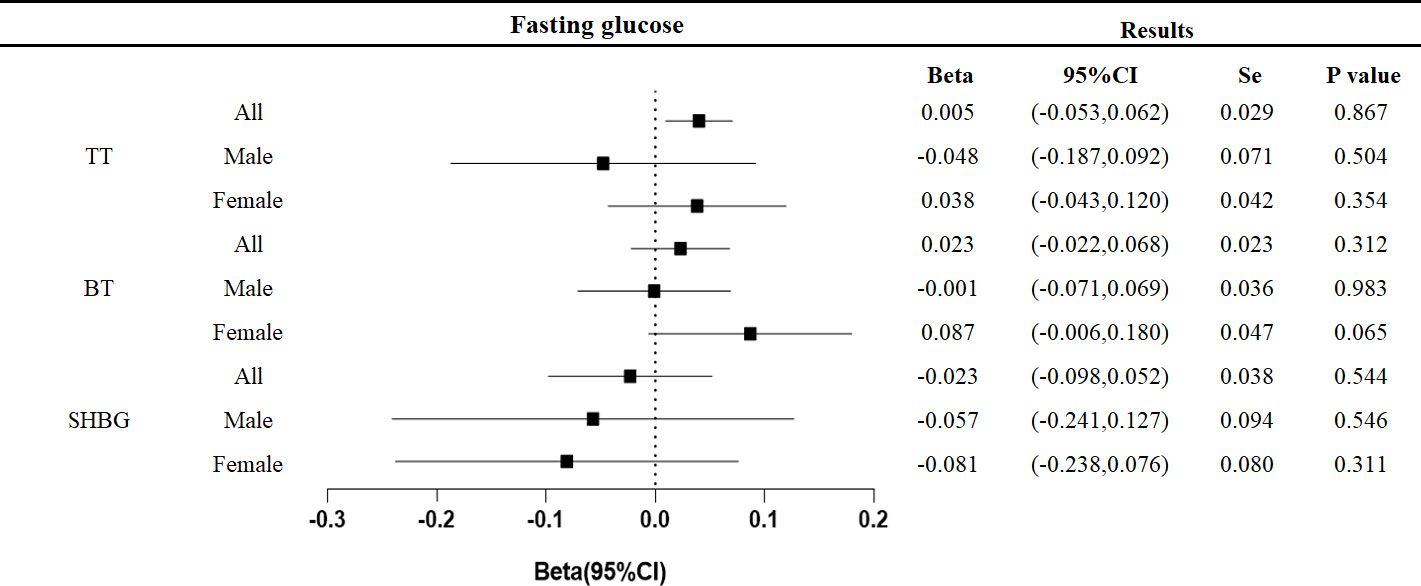
Figure 5 Associations of genetic liability to fasting glucose with testosterone. Beta is the relationship between per unit increase in fasting glucose (nmol/L) and testosterone level (nmol/L). Se is standard error. P values are for ORs (95% CIs). * The P value for pleiotropy is less than 0.05.
No pleiotropic effects were found in any of the above studies, and the confidence intervals of the results were narrowed after exclusion of outliers by the MR-PRESSO test. The results are summarized in the Supplementary Tables 5–7.
Medications for diabetes
Multivariate Mendelian randomization analysis was performed for the four drugs (insulin, metformin, blood pressure medication, and statin medication). After adjustment for insulin therapy, the difference in testosterone levels (including total testosterone, bioavailable testosterone, and sex hormone-binding globulin) disappeared in all patients with diabetes, regardless of gender. At the same time, the downward trend of testosterone level was curbed, but showed an upward trend. Although this difference was not statistically significant, we can at least assume that the hypo-testosterone state was improved with insulin treatment. After adjusting for metformin treatment, the overall difference in testosterone levels in diabetic patients disappeared, except for bioavailable testosterone and sex hormone binding globulin levels in female patients. However, the results were not significantly altered after adjustment for statin therapy and antihypertensive medication alone. We summarized the relevant results in the Table 8.
Discussion
Testosterone, an endogenous hormone, is the main male hormone produced by the testis and the precursor of estrogen synthesized by the female ovary (25). In males, it is mainly responsible for promoting the development and maintenance of male sexual characteristics (26) and plays a significant role in the development and maintenance of the male skeletal system (27). It is responsible for the maintenance of sexual desire in females (28). In general, low testosterone is thought to be closely related to sexual dysfunction (29). Testosterone not only has a major effect on sexual function, but also has effects on metabolism, mood, and cognition (30, 31).
Many observational studies have shown that testosterone levels in type 2 diabetic patients are lower than those in normal subjects (32). Due to the limitations of observational studies, causality cannot be fully determined, and confounding factors cannot be well excluded from studies. The study is the first to examine this issue using genetic tools. We investigated this issue using a large sample of diabetes GWAS data and three glycemic traits as exposure factors.
Fasting glucose represents short-term blood glucose, and HbA1c represents blood glucose up to three months (33). We also used the fasting insulin level as an exposure factor, which provides a proxy for the degree of insulin resistance (34). Testosterone levels vary greatly between men and women (35), so we also conducted sex-specific studies. In addition, we also adjusted for common diabetes drugs and obtained the improvement of testosterone decline with various treatment regimens.
Testosterone levels represent the sum of unbound as well as bound testosterone in the circulation. Most circulating testosterone is bound to SHBG and albumin, and only 2.0% to 4.0% of circulating testosterone is unbound or free (36). Bioavailable testosterone was defined as the sum of testosterone bound to albumin and free testosterone. Total testosterone was numerically equivalent to the sum of bioavailable testosterone and SHBG-bound testosterone. At the same time, the free hormone hypothesis states that the intracellular concentration and biological activity of hormones depend on the concentration of free hormones and not on the hormones in plasma bound to proteins (37).
The association between type 2 diabetes and testosterone levels has previously been questioned for two reasons. First, cross-sectional studies suggest a strong inverse association between testosterone and obesity (38), which is often accompanied by abnormal lipid metabolism in patients with diabetes. Therefore, it is not clear whether the low testosterone status in diabetic patients is affected by abnormal lipid metabolism. Second, patients with type 2 diabetes always have lower SHBG levels (39). Testosterone levels are largely affected by SHBG, especially as some studies have found no significant difference in bioavailable testosterone levels in patients with diabetes (40), which contrasts with the prevalence of sexual dysfunction in patients with diabetes. These problems are due to the nature of observational studies, which cannot well rule out confounding.
To address the first question, we found that the effect of diabetes on testosterone was still significant after excluding obesity-related genes and adjusting for BMI, lipid parameters (TG and LDL) and serum adiponectin. Especially for male patients, it can be determined that their total testosterone and sex hormone binding globulin showed a statistically significant decrease. Clear results were obtained after minimizing the effect of lipids and obesity. This effect is probably due to hyperglycemia and insulin resistance (41) due to diabetes itself and is independent of diabetic dyslipidemia. The analysis of three glucose traits also provided better support for this conclusion.
To address the second issue, in this study, three different indices of testosterone level were analyzed separately. Although a statistically significant increase in bioavailable testosterone was found in total and in women, this conclusion was not robust due to pleiotropy. Therefore, our study cannot support an increase in bioavailable testosterone levels in patients with diabetes, especially in studies that distinguish between sex. We suspect that the combination of hyperglycemia and insulin resistance is responsible for complex changes in bioavailable testosterone levels that require further elucidation. But in any case, there is no doubt that total testosterone and SHBG levels are significantly decreased in diabetic patients.
It is important that we obtain more valuable results after adjustment for conventional medications in the treatment of diabetes. Blood pressure medication and statin medication did not affect testosterone levels. However, our results were significantly changed after adjustment for exogenous insulin injection and oral metformin treatment. Especially after insulin injection treatment, the three indicators of testosterone level showed an opposite upward trend. In this study, we found that an increase in fasting insulin measures was associated with a decrease in testosterone levels, particularly sex hormone-binding globulin. Therefore, it can be concluded that injection of exogenous insulin after the appearance of insulin resistance can still have a positive effect on the low testosterone status caused by diabetes. This is also consistent with the clinical observation (42).
For the treatment of diabetes, the current guidelines of most countries do not recommend direct injection of exogenous insulin. Our results suggest that oral administration of metformin and injection of exogenous insulin may be a good way to improve low testosterone status in diabetic patients.
Previously, animal models of diabetes and metabolic syndrome have shown that hyperglycemia and insulin resistance play an important role in causing hypogonadism (43, 44). Animal studies confirm that mice with insulin receptor knockout exhibit hypogonadotropic hypogonadism (45, 46). Cellular studies in rat hypothalamic neurons have found that insulin plays a key role in promoting GnRH secretion (47). In addition to its action on the hypothalamus, insulin also acts directly on the liver to stimulate SHBG production (48, 49).
It has also been noted that lifestyle or pharmacologic management that improves insulin resistance increases testosterone levels (5) It has also been suggested that testosterone deficiency is associated with impaired gonadotropin response (50). It has been found that long-term hyperglycemia may lead to metabolic imbalance, inflammation, and oxidative stress, which may lead to a decrease in testosterone level (51).
Our analysis was performed according to gender in our study, and the changes in indicators of female patients often showed opposite trends compared with those of male patients, especially in total testosterone and bioavailable testosterone. This is consistent with what we have observed clinically (52). We speculate that it may be related to the fact that physiological amounts of estrogen in women reduce insulin resistance (53).
In conclusion, we used Mendelian randomization to determine the effects of T2DM, fasting insulin, and HbA1c on the three testosterone levels after controlling for SNPs associated with obesity and BMI and adjusting for confounding factors such as BMI, TG, LDL, and serum adiponectin. Compared with fasting plasma glucose, fasting insulin and Hb1Ac were more powerful predictors of SHBG and total testosterone. Previously, the causal relationship between the changes of sex hormones in diabetic patients has been controversial. Combined with the characteristics of Mendelian randomization studies, we believe that our study can solve this problem to a certain extent. We also found that oral metformin and injection of exogenous insulin improved the low testosterone status in diabetic patients after adjusting the common treatment methods.
Limitations of our study
First, although we excluded the currently known FTO gene and performed reanalysis with adjustment for BMI, TC, LDL, and serum adiponectin, we could not completely rule out the effect of obesity on testosterone levels. Second, limited by GWAS, we decided to use fasting insulin levels as a proxy for insulin resistance. Although this indicator can represent insulin resistance to a certain extent, it is not the gold standard of insulin resistance (54), and it will also introduce some bias. Third, we used SNPS pooled from the meta-analysis, which makes it difficult to calculate f-values, so we cannot completely rule out the effect of weak instrumental variables. Fourth, different measures of testosterone levels can yield different results (55). Fifth, the pleiotropic effect found in the analysis of biologically available testosterone levels is fatal to the reliability of the results of the Mendelian randomization analysis. We subsequently added analyses of different glycemic measures, but more reliable methods are needed.
Data availability statement
The original contributions presented in the study are included in the article/Supplementary Material. Further inquiries can be directed to the corresponding author.
Author contributions
CJ: Conceptualization, Methodology, Software, Investigation, Formal Analysis, Writing - original draft. YW: Data Curation, Writing - original draft. WY: Visualization, Investigation. XY: Conceptualization, Funding acquisition, Resources, Supervision, Writing - review & editing. All authors contributed to the article and approved the submitted version.
Conflict of interest
The authors declare that the research was conducted in the absence of any commercial or financial relationships that could be construed as a potential conflict of interest.
Publisher’s note
All claims expressed in this article are solely those of the authors and do not necessarily represent those of their affiliated organizations, or those of the publisher, the editors and the reviewers. Any product that may be evaluated in this article, or claim that may be made by its manufacturer, is not guaranteed or endorsed by the publisher.
Supplementary material
The Supplementary Material for this article can be found online at: https://www.frontiersin.org/articles/10.3389/fendo.2023.1238090/full#supplementary-material
References
1. Zimmet P, Alberti KG, Magliano DJ, Bennett PH. Diabetes mellitus statistics on prevalence and mortality: facts and fallacies. Nat Rev Endocrinol (2016) 12:616–22. doi: 10.1038/nrendo.2016.105
2. NCD Risk Factor Collaboration (NCD-RisC). Worldwide trends in diabetes since 1980: a pooled analysis of 751 population-based studies with 4.4 million participants. Lancet (2016) 387(10027):1513–30. doi: 10.1016/S0140-6736(16)00618-8
3. Howarth FC, Shafiullah M, Qureshi MA. Chronic effects of type 2 diabetes mellitus on cardiac muscle contraction in the Goto-Kakizaki rat. Exp Physiol (2007) 92(6):1029–36. doi: 10.1113/expphysiol.2007.038703
4. Li G, Zhang P, Wang J, An Y, Gong Q, Gregg EW, et al. Cardiovascular mortality, all-cause mortality, and diabetes incidence after lifestyle intervention for people with impaired glucose tolerance in the Da Qing diabetes prevention study: a 23-year follow-up study. Lancet Diabetes Endocrinol (2014) 2(6):474–80. doi: 10.1016/S2213-8587(14)70057-9
5. Grossmann M, Thomas MC, Panagiotopoulos S, Sharpe K, Macisaac RJ, Clarke S, et al. Low testosterone levels are common and associated with insulin resistance in men with diabetes. J Clin Endocrinol Metab (2008) 93(5):1834–40. doi: 10.1210/jc.2007-2177
6. Wittert G, Bracken K, Robledo KP, Grossmann M, Yeap BB, Handelsman DJ, et al. Testosterone treatment to prevent or revert type 2 diabetes in men enrolled in a lifestyle programme (T4DM): a randomised, double-blind, placebo-controlled, 2-year, phase 3b trial. Lancet Diabetes Endocrinol (2021) 9(1):32–45. doi: 10.1016/S2213-8587(20)30367-3PMID:33338415
7. American Diabetes Association Diagnosis and classification of diabetes mellitus. Diabetes Care (2014) 37(Suppl. S1):S81–90. doi: 10.2337/dc14-S081
8. Davies NM, Holmes MV, Davey Smith G. Reading Mendelian randomisation studies: a guide, glossary, and checklist for clinicians. BMJ (2018) 362:k601. doi: 10.1136/bmj.k601
9. Pingault JB, O’Reilly PF, Schoeler T, Ploubidis GB, Rijsdijk F, Dudbridge F. Using genetic data to strengthen causal inference in observational research. Nat Rev Genet (2018) 19:566–80. doi: 10.1038/s41576-018-0020-3
10. Vujkovic M, Keaton JM, Lynch JA, Miller DR, Zhou J, Tcheandjieu C, et al. HPAP Consortium; Regeneron Genetics Center; VA Million Veteran Program. Discovery of 318 new risk loci for type 2 diabetes and related vascular outcomes among 1.4 million participants in a multi-ancestry meta-analysis. Nat Genet (2020) 52(7):680–91. doi: 10.1038/s41588-020-0637-y
11. Frayling TM, Timpson NJ, Weedon MN, Zeggini E, Freathy RM, Lindgren CM, et al. A common variant in the FTO gene is associated with body mass index and predisposes to childhood and adult obesity. Science (2007) 316(5826):889–94. doi: 10.1126/science.1141634
12. Kilpeläinen TO, Carli JF, Skowronski AA. Genome-wide meta-analysis uncovers novel loci influencing circulating leptin levels. Nat Commun (2016) 7:10494. doi: 10.1038/ncomms10494
13. Willer CJ, Schmidt EM, Sengupta S, Global Lipids Genetics Consortium. Discovery and refinement of loci associated with lipid levels. Nat Genet (2013) 45(11):1274–83. doi: 10.1038/ng.2797
14. Dastani Z, Hivert MF, Timpson N, Perry JR, Yuan X, Scott RA, et al. Novel loci for adiponectin levels and their influence on type 2 diabetes and metabolic traits: a multi-ethnic meta-analysis of 45,891 individuals. PloS Genet (2012) 8(3):e1002607. doi: 10.1371/journal.pgen.1002607
15. Available at: https://gwas.mrcieu.ac.uk/datasets/finn-b-KELA_DIAB_INSUL/.
16. Available at: https://gwas.mrcieu.ac.uk/datasets/finn-b-RX_STATIN/.
17. Available at: https://gwas.mrcieu.ac.uk/datasets/ukb-b-18009/.
18. Available at: https://gwas.mrcieu.ac.uk/datasets/ukb-b-14609/.
19. Ruth KS, Day FR, Tyrrell J, Thompson DJ, Wood AR, Mahajan A, et al. Using human genetics to understand the disease impacts of testosterone in men and women. Nat Med (2020) 26(2):252–8. doi: 10.1038/s41591-020-0751-5
20. Bycroft C, Freeman C, Petkova D, Band G, Elliott LT, Sharp K, et al. The UK Biobank resource with deep phenotyping and genomic data. Nature (2018) 562(7726):203–9. doi: 10.1038/s41586-018-0579-z
21. Bowden J, Davey Smith G, Haycock PC, Burgess S. Consistent estimation in Mendelian randomization with some invalid instruments using a weighted median estimator. Genet Epidemiol (2016) 40:304–14. doi: 10.1002/gepi.21965
22. Staiger D. Instrumental variables regression with weak instruments. Econometrica (1997) 65:557–86. doi: 10.2307/2171753
23. Stephen Burgess S. Online sample size and power calculator for Mendelian randomization with a binary outcome (2022). Available at: https://sb452.shinyapps.io/power/ (Accessed 30 Sep 2022).
24. Burgess S. Sample size and power calculations in Mendelian randomization with a single instrumental variable and a binary outcome. Int J Epidemiol (2014) 43:922–9. doi: 10.1093/ije/dyu005
25. Bachmann G, Bancroft J, Braunstein G, Burger H, Davis S, Dennerstein L, et al. Female androgen insufficiency: the Princeton consensus statement on definition, classification, and assessment. Fertil Steril (2002) 77(4):660–5. doi: 10.1016/S0015-0282(02)02969-2
26. American College of Sports Medicine Position Statement on Proper and Improper Weight Loss Program. Medicine Science Sports Exercise (1983). doi: 10.1249/00005768-198315010-00016
27. Yarrow JF, Wronski TJ, Borst SE. Testosterone and adult male bone: actions independent of 5α-reductase and aromatase. Exerc Sport Sci Rev (2015) 43(4):222–30. doi: 10.1249/JES.0000000000000056
28. Smith T, Batur P. Prescribing testosterone and DHEA: The role of androgens in women. Cleve Clin J Med (2021) 88(1):35–43. doi: 10.3949/ccjm.88a.20030
29. Mikhail N. Does testosterone have a role in erectile function? Am J Med (2006) 119(5):373–82. doi: 10.1016/j.amjmed.2005.07.042
30. Dos Santos RL, Da Silva FB, Ribeiro RF, Stefanon I. Sex hormones in the cardiovascular system. Horm Mol Biol Clin Invest (2014) 18(2):89–103. doi: 10.1515/hmbci-2013-0048
31. Pike CJ, Carroll JC, Rosario ER, Barron AM. Protective actions of sex steroid hormones in Alzheimer’s disease. Front Neuroendocrinol (2009) 30(2):239–58. doi: 10.1016/j.yfrne.2009.04.015
32. Holman RR, Paul SK, Bethel MA, Matthews DR, Neil HA. 10-year follow-up of intensive glucose control in type 2 diabetes. N Engl J Med (2008) 359:1577–89. doi: 10.1056/NEJMoa0806470
33. Wang Z, Du Z, Zhu F. Glycosylated hemoglobin is associated with systemic inflammation, hypercoagulability, and prognosis of COVID-19 patients. Diabetes Res Clin Pract (2020) 164:108214. doi: 10.1016/j.diabres.2020.108214
34. Katz A, Nambi SS, Mather K, Baron AD, Follmann DA, Sullivan G, et al. Quantitative insulin sensitivity check index: A simple, accurate method for assessing insulin sensitivity in humans. J Clin Endocrinol Metab (2000) 85(7):2402–10. doi: 10.1210/jcem.85.7.6661
35. Clark RV, Wald JA, Swerdloff RS, Wang C, Wu FCW, Bowers LD, et al. Large divergence in testosterone concentrations between men and women: Frame of reference for elite athletes in sex-specific competition in sports, a narrative review. Clin Endocrinol (Oxf) (2019) 90(1):15–22. doi: 10.1111/cen.13840
36. Goldman AL, Bhasin S, Wu FCW, Krishna M, Matsumoto AM, Jasuja R. A Reappraisal of testosterone’s binding in circulation: physiological and clinical implications. Endocr Rev (2017) 38(4):302–24. doi: 10.1210/er.2017-00025
37. Mendel CM. The free hormone hypothesis: a physiologically based mathematical model. Endocrine Rev (1989) 10:232–74. doi: 10.1210/edrv-10-3-232
38. Dhindsa S, Miller MG, McWhirter CL, Mager DE, Ghanim H, Chaudhuri A, et al. Testosterone concentrations in diabetic and nondiabetic obese men. Diabetes Care (2010) 33:1186–92. doi: 10.2337/dc09-1649
39. Simon D, Charles MA, Nahoul K, Orssaud G, Kremski J, Hully V, et al. Association between plasma total testosterone and cardiovascular risk factors in healthy adult men: the Telecom Study. J Clin Endocrinol Metab (1997) 82:682–5. doi: 10.1210/jc.82.2.682
40. Stanworth RD, Kapoor D, Channer KS, Jones TH. Statin therapy is associated with lower total but not bioavailable or free testosterone in men with type 2 diabetes. Diabetes Care (2009) 32(4):541–6. doi: 10.2337/dc08-1183
41. Kaur G, Thompson LA, Babcock RL, Mueller K, Dufour JM. Sertoli cells engineered to express insulin to lower blood glucose in diabetic mice. DNA Cell Biol (2018) 37(8):680–90. doi: 10.1089/dna.2017.3937
42. Hu Y, Ding B, Shen Y, Yan RN, Li FF, Sun R, et al. Rapid changes in serum testosterone in men with newly diagnosed type 2 diabetes with intensive insulin and metformin. Diabetes Care (2021) 44(4):1059–61. doi: 10.2337/dc20-1558
43. Vignozzi L, Morelli A, Filippi S, Ambrosini S, Mancina R, Luconi M, et al. Testosterone regulates RhoA/Rho-kinase signaling in two distinct animal models of chemical diabetes. J Sex Med (2007) 4:620–30. doi: 10.1111/j.1743-6109.2007.00440.x
44. Filippi S, Vignozzi L, Morelli A, Chavalmane AK, Sarchielli E, Fibbi B, et al. Testosterone partially ameliorates metabolic profile and erectile responsiveness to PDE5 inhibitors in an animal model of male metabolic syndrome. J Sex Med (2009) 6:3274–88. doi: 10.1111/j.1743-6109.2009.01467.x
45. Bruning JC, Gautam D, Burks DJ, Gillette J, Schubert M, Orban PC, et al. Role of brain insulin receptor in control of body weight and reproduction. Science (2000) 289(5487):2122–5. doi: 10.1126/science.289.5487.2122
46. Gamba M, Pralong FP. Control of GnRH neuronal activity by metabolic factors: the role of leptin and insulin. Mol Cell Endocrinol (2006) 254:133–9. doi: 10.1016/j.mce.2006.04.023
47. Salvi R, Castillo E, Voirol MJ, Glauser M, Rey JP, Gaillard RC, et al. Gonadotropin-releasing hormone-expressing neurons immortalized conditionally are activated by insulin: implication of the mitogen-activated protein kinase pathway. Endocrinology (2006) 147(2):816–26. doi: 10.1210/en.2005-0728
48. Vallerie SN, Furuhashi M, Fucho R, Hotamisligil GS. A predominant role for parenchymal c-Jun amino terminal kinase (JNK) in the regulation of systemic insulin sensitivity. PloS One (2008) 3:e3151. doi: 10.1371/journal.pone.0003151
49. Dandona P, Aljada A, Bandyopadhyay A. Inflammation: the link between insulin resistance obesity and diabetes. Trends Immunol (2004) 25:4–7. doi: 10.1016/j.it.2003.10.013
50. Dhindsa S, Prabhakar S, Sethi M, Bandyopadhyay A, Chaudhuri A, Dandona P. Frequent occurrence of hypogonadotropic hypog-onadism in type 2 diabetes. J Clin Endocrinol Metab (2004) 89:5462–8. doi: 10.1210/jc.2004-0804
51. Derkach KV, Bakhtyukov AA, Bayunova LV, Zorina II, Shpakov AO. Normalization of testicular steroidogenesis and spermatogenesis in male rats with type 2 diabetes mellitus under the conditions of metformin therapy. Dokl Biol Sci (2020) 493(1):110–3. doi: 10.1134/S0012496620040031
52. Ding EL, Song Y, Malik VS, Liu S. Sex differences of endogenous sex hormones and risk of type 2 diabetes: a systematic review and meta-analysis. JAMA (2006) 295:1288–99. doi: 10.1001/jama.295.11.1288
53. Cagnacci A, Soldani R, Carriero PL, Paoletti AM, Fioretti P, Melis GB. Effects of low doses of transdermal 17 beta-estradiol on carbohydrate metabolism in postmenopausal women. J Clin Endocrinol Metab (1992) 74(6):1396–400. doi: 10.1210/jcem.74.6.1317387
54. DeFronzo RA, Tobin JD, Andres R. Glucose clamp technique: a method for quantifying insulin secretion and resistance. Am J Physiol (1979) 237:E214–23. doi: 10.1152/ajpendo.1979.237.3.E214
Keywords: Mendelian randomization, testosterone, diabetes, glycemic traits, sexual dysfunction
Citation: Jiang C, Wang Y, Yang W and Yang X (2023) New evidence for the effect of type 2 diabetes and glycemic traits on testosterone levels: a two-sample Mendelian randomization study. Front. Endocrinol. 14:1238090. doi: 10.3389/fendo.2023.1238090
Received: 10 June 2023; Accepted: 25 September 2023;
Published: 11 October 2023.
Edited by:
Carmine Gazzaruso, University of Milan, ItalyReviewed by:
Swayamsidha Mangaraj, Siksha O Anusandhan University, IndiaMayank Choubey, New York University, United States
Huating Li, Shanghai Jiao Tong University, China
Copyright © 2023 Jiang, Wang, Yang and Yang. This is an open-access article distributed under the terms of the Creative Commons Attribution License (CC BY). The use, distribution or reproduction in other forums is permitted, provided the original author(s) and the copyright owner(s) are credited and that the original publication in this journal is cited, in accordance with accepted academic practice. No use, distribution or reproduction is permitted which does not comply with these terms.
*Correspondence: Xinghai Yang, NzU0OTM2NTRAcXEuY29t