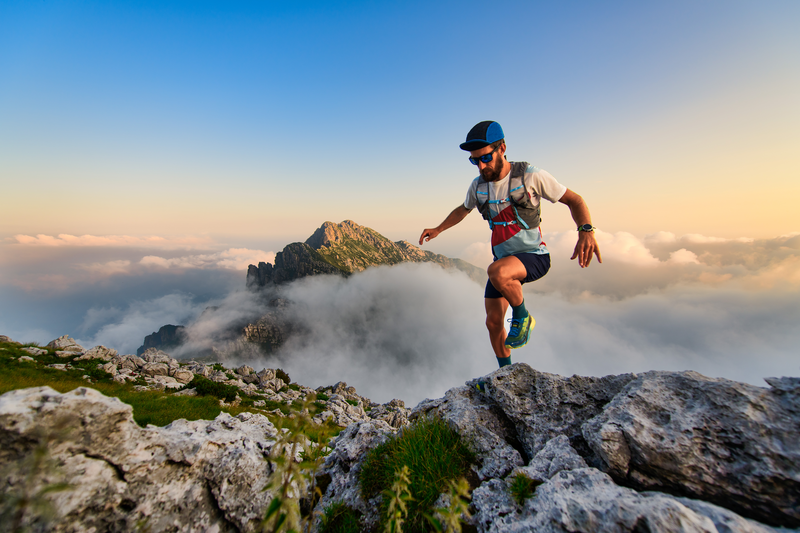
95% of researchers rate our articles as excellent or good
Learn more about the work of our research integrity team to safeguard the quality of each article we publish.
Find out more
ORIGINAL RESEARCH article
Front. Endocrinol. , 31 August 2023
Sec. Clinical Diabetes
Volume 14 - 2023 | https://doi.org/10.3389/fendo.2023.1228153
This article is part of the Research Topic Cystic Fibrosis-Related Diabetes View all 9 articles
Objective: Cystic fibrosis (CF)-related diabetes (CFRD) resulting from partial-to-complete insulin deficiency occurs in 40-50% of adults with CF. In people with CFRD, poor glycemic control leads to a catabolic state that may aggravate CF-induced nutritional impairment and loss of muscle mass. Sensor augmented pump (SAP) therapy may improve glycemic control as compared to multiple daily injection (MDI) therapy.
Research design and methods: This non-randomized clinical trial was aimed at evaluating the effects of insulin therapy optimization with SAP therapy, combined with a structured educational program, on glycemic control and body composition in individuals with insulin-requiring CFRD. Of 46 participants who were offered to switch from MDI to SAP therapy, 20 accepted and 26 continued the MDI therapy. Baseline demographic and clinical characteristics were balanced between groups using a propensity score-based overlap weighting procedure and weighted mixed-effects regression models were used to estimate changes in study outcomes.
Results: After 24 months changes in HbA1c were: -1.1% (-12.1 mmol/mol) (95% CI: -1.5; -0.8) and -0.1% (-1 mmol/mol) (95% CI: -0.5; 0.3) in the SAP and MDI therapy group, respectively, with a between-group difference of -1.0 (-10 mmol/mol) (-1.5; -0.5). SAP therapy was also associated with a decrease in mean glucose (between group difference: -32 mg/dL; 95% CI: -44; -20) and an increase in TIR (between group difference: 19.3%; 95% CI 13.9; 24.7) and in fat-free mass (between group difference: +5.5 Kg, 95% CI: 3.2; 7.8).
Conclusion: Therapy optimization with SAP led to a significant improvement in glycemic control, which was associated with an increase in fat-free mass.
Cystic fibrosis (CF) is a life-threatening genetic disease caused by mutations of the CF transmembrane conductance regulator (CFTR) gene. The gene encodes a protein channel expressed in many organs including the pancreas and the lung. The main clinical manifestations of the disease are in fact pancreatic insufficiency and progressive lung disease. Pancreatic insufficiency is present since early childhood in ~80% of this population and requires high energy-density diet with no restrictions on fat and carbohydrate content and pancreatic enzyme replacement therapy. Lung disease is sustained by recurrent respiratory infections and represents the main cause of death in these individuals. However, the recent availability of highly-effective CFTR modulators is going to radically change the natural history of CF.
CF also affects the endocrine function of the pancreas and patients develop a unique form of diabetes, defined as CF-related diabetes (CFRD), which differs from both type 1 and type 2 diabetes mellitus. It is the most common extra-pulmonary CF complication, with a prevalence that is strictly age-related, raising from ~5% in the pediatric population to ~50% in adults, with a further 35% of people having impaired glucose tolerance (IGT) (1, 2).
Dysregulation of glucose metabolism in CF is caused by a progressive decline in insulin secretion, but β-cell loss due to self-digestion of endocrine tissue by trapped pancreatic enzymes is not the only pathological mechanism (3–5). The CFTR protein has been detected also on β-cell surface and its mutations have been associated to alterations in transmembrane potential, accumulation of altered CFTR in the rough endoplasmic reticulum and increased intracellular oxidative stress, leading to premature β-cell apoptosis and insulin deficiency (3).
Conversely, other studies suggest that CFTR may not be expressed in human pancreatic endocrine cells (6) CFRD has been associated with the typical microvascular complications of diabetes mellitus, albeit with different prevalence from type 1 and type 2 diabetes (7–10): retinopathy and microalbuminuria are diagnosed in about 15% of adults with CFRD, while neuropathy and gastroparesis can be detected in more than 50% of them. In terms of long-term clinical outcomes, CFRD has been related to a clinically significant decline in lung function, a decreased survival from lung disease and to a poorer nutritional status (6, 11–15).
Currently, the only recommended treatment for CFRD is insulin therapy, with an early start being associated with a significant improvement in disease prognosis (2, 12, 14–19).
However, insulin treatment may contribute to the multidrug burden of care for people with CF and, our clinical experience has been that, in many cases, multiple daily injection (MDI) regimens may not be optimal for the management of the multifactorial complexity of CFRD. This includes minimal insulin requirements, high risk of hypoglycemia, exacerbations and concomitant steroid therapies, gastroparesis and intestinal and nutritional recommendations.
Sensor augmented pump (SAP) therapy is increasingly accepted as gold standard therapy for people with type 1 diabetes (20–24) and continuous glucose monitoring (CGM) has been validated in people with CFRD (25). CGM-derived parameters of glucose variability have been associated with CF outcomes such as respiratory function and body mass index (BMI) (14, 26–29), but data supporting the benefits of diabetes technology in this populations are still scarce and based on small samples (30–32). Despite its potential advantages, SAP therapy was shown to be less frequently used (4.1% vs 17.7%) and more frequently discontinued (30.0% vs 12.7%) in people with CFRD as compared with those with type 1 diabetes (33).
This study was designed to evaluate the potential impact of insulin therapy optimization with SAP over MDI therapy on glycemic control and body composition in individuals with CFRD requiring insulin treatment.
We conducted a non-randomized clinical trial including adult (>18 years) individuals with CFRD on insulin treatment and with HbA1c ≥ 5% (31 mmol/mol). Exclusion criteria were: pregnancy, advanced renal disease, defined by estimated glomerular filtration rate < 30 mL/min/1.73 m2, and heart failure.
From November 2020 to November 2021, 82 people with CF attending the Diabetes Unit of the Fondazione IRCCS Ca’ Granda Ospedale Maggiore Policlinico in Milano were evaluated for eligibility.
At the screening visit, all subjects were on MDI insulin therapy and, after 2-week run-in period, they underwent an enrollment visit which included clinical, anthropometric and biochemical assessments. Glucose profiles were evaluated with data downloaded from flash glucose monitoring (FGM)/CGM devices. All participants were proposed to participate in a structured preparatory educational program (training to SAP therapy and carbohydrates counting), structured in 4 face-to-face meetings and performed by a multidisciplinary staff (i.e. nutritionist, diabetologist and nurse). After completing the training, all participants were offered to switch from MDI to SAP therapy. Participants who agreed switched to SAP therapy and those who refused continued MDI-scheme insulin therapy. Subjects from both groups had outpatient visits every 3 months according to standard of care. Protocol visits were performed 6, 12 and 24 months after enrollment.
The study complies with the Declaration of Helsinki. The research protocol was approved by the Ethics Committee of the Fondazione Istituto di Ricovero e Cura a Carattere Scientifico (IRCCS) Ca’ Granda Ospedale Maggiore Policlinico (study number 4166, ID 89006) and has been registered on ClinicalTrials.gov (ClinicalTrials.gov Identifier: NCT04379726). A written informed consent was provided by each participant.
Study flow chart is reported in Figure 1.
Figure 1 Study flow chart. CGM, continuous glucose monitoring; CHO, carbohydrates; FGM, flash glucose monitoring; BIA, bio-impedance analysis; SAP, sensor-augmented pump; CHO, carbohydrates.
At screening, participants already wore and actively used a FGM/CGM system (Abbott Freestyle Libre 1, Chicago, IL, USA; Abbott Freestyle Libre 2, Chicago, IL, USA; Dexcom G4, San Diego, CA, USA; Dexcom G5, San Diego, CA, USA; Dexcom G6, San Diego, CA, USA; Medtronic Guardian 3, Minneapolis, MN, USA) according to standard of care, at least 3 months prior to baseline. All participants were trained on the use of FGM/CGM system by our medical staff in order to provide a complete 14-day glucose monitoring at each study visit, when they underwent anthropometric evaluation and blood testing including fasting glycaemia (mg/dL, hexokinase) and HbA1c (% and mmol/mol; HPLC). At enrollment and end-of-study visit, body composition was assessed through a tetrapolar single frequency (50 kHz) bioelectrical impedance analyzer (Akern BIA 101 BIVA, Pontassieve, FI, Italy). Data obtained from bioimpedance were analyzed with the software BodyGram Plus. With this method we could estimate fat-free mass (FFM) and fat mass (FM).
Baseline demographic and clinical characteristics, including sex, age, organ transplantation receipt, percent predicted forced expiratory volume in one second (ppFEV1), assessed at the last pulmonary function tests, and daily insulin requirements (IU/kg of body weight/day) were recorded.
Data from FGM/CGM were downloaded using the dedicated software (Medtronic Carelink, Minneapolis, MN, USA; Abbott Libreview, Chicago, IL, USA, Dexcom Clarity, San Diego, CA, USA). Glucose control was evaluated using the following metrics: mean glucose levels (mg/dL), time in range (TIR, %), assuming a 70-180 mg/dlL range according to the American Diabetes Association Standards of Medical Care 2022 (34), time above range (TAR, %) and time below range (TBR, %).
Insulin pumps (Medtronic MiniMed 640G, Minneapolis, MN, USA; Medtronic MiniMed 670G, Minneapolis, MN, USA; Tandem T:slim X2, San Diego, CA, USA; Roche Accu Chek Insight, Basel, CH; Roche Accu Chek Solo, Basel, CH; Insulet Omnipod, Acton, MA, USA; Ypsomed Ypsopump, Burgdorf, CH) and glucose monitors were chosen among the devices currently available in Italy for SAP therapy according to standard of care and individuals everyday life needs.
The primary endpoint was the change from baseline in HbA1c, whereas secondary endpoints were changes in mean glucose from CGM, TIR, TAR, TBR, weight, BMI, fat-free mass (FFM), fat mass (FM) (in kg or as percent of body weight) and ppFEV1.
Overlap weighting was used to address the imbalance in baseline characteristics between treatment groups (35). This technique uses the propensity score to assign weights to each patient that are proportional to the probability of the patient being assigned to the opposite treatment group. The propensity score was estimated through a logistic regression model including the following predictors: sex, age, lung transplantation, and baseline ppFEV1, BMI, FM (% of body weight), insulin requirement, HbA1c, mean glucose and TBR. Overlap weighting has several advantages compared to other weighting options, such as inclusion of all available participants, handling of extreme propensity scores, and exact balance for the mean of all covariates included in the propensity score model. Balance was evaluated using the standardized mean differences (SMD) and values <0.1 were considered indicative of good balance.
Changes in study outcomes from baseline values, between-group differences and corresponding 95% confidence intervals (CI) were estimated using weighted mixed-effects regression models. The weights were obtained from the procedure described above. These models were also used to plot the estimated means at different time points for both groups. A linear mixed-effects quantile regression model was used to estimate the conditional median of the TBR due to the high degree of asymmetry in the distribution of this outcome (36). Models were also adjusted for baseline variables which showed a residual imbalance after weighting. The corresponding 95% confidence intervals (CI) were obtained by bootstrap resampling (n=1000), using the percentile method.
The statistical significance of the between-group differences was evaluated using the likelihood ratio test, testing the significance of the treatment by time interaction.
To adjust for multiple testing, the Benjamini and Hochberg’s False Discovery Rate procedure was used (37). All statistical tests were two-sided with α=0.05.
The analysis was performed using R version 4.3.0 (2023-04-21 ucrt). The “lmer” function from the “lme4” package and the “lqmm” function from the “lqmm” package were used to fit the linear mixed-effects regression models and the quantile mixed-effects regression models, respectively. Figure 1 was created with Biorender.com (38).
Forty-six participants (mean age: 35.6 years, range: 21-56) agreed to participate in the educational program and at the end of the program 26 of them refused to switch to SAP therapy and continued with MDI therapy, while 20 switched to SAP.
No participants in either group experienced severe hypoglycemia or diabetic ketoacidosis.
People who switched to SAP used the following SAP devices: 10 Medtronic 670/780, 6 Omnipod, 3 Tandem t:slim, and 1 Accu-Chek Solo. Additionally, 13 devices had a closed loop system, and 7 had a patch pump. None of the participants were on enteral nutrition or were taking energy supplements.
Table 1 provides a comparison of their baseline demographic and clinical characteristics before and after weighting. Before weighting, participants in the SAP group were more likely females had comparable ages and lung function, while they had a higher BMI and body fatness, lower fasting glucose and insulin requirement as compared to people in the MDI group. Seven participants (35.0%) in the SAP group and 17 participants (65.4%) in the MDI group had received bilateral lung transplantation before enrollment and their steroid therapy dosage remained unchanged for 4 weeks prior to screening visit. Five participants in the SAP group and none in the MDI group were receiving the CFTR modulator therapy lumacaftor/ivacaftor at enrollment. Glycemic control was similar between groups, as indicated by the comparable mean values of HbA1c. After weighting, we obtained two perfectly balanced groups for the measured baseline characteristics, except for a residual imbalance in the frequency of CFTR modulator therapy.
All participants completed the last study visit, though HbA1c was not measured in one patient in the MDI group.
In the SAP group, HbA1c decreased after 6 months from baseline and this reduction persisted over the study period, while it remained substantially unchanged in the MDI group (Figure 2A). Table 2 gives the estimated changes from baseline in HbA1c in both groups. Between-group difference in HbA1c at the end of the study (24 months from enrollment) was -1.0% (-11 mmol/mol) (95% CI: -1.5; -0.5).
Figure 2 Model-based means of HbA1c (A), mean glucose from continuous glucose monitoring (B) and TIR (C) and model-based median TBR (D) from baseline through 24 months among subjects in the MDI versus SAP therapy group. Points estimates (dots) and 95% confidence intervals (bars) were estimated using linear mixed-effects regression models or mixed-effects quantile regression model (for TBR). MDI, Multiple daily injections; SAP, Sensor augmented pump; TBR, Time below range; TIR, Time in range.
Table 2 Changes in study outcomes over 24 months among patients in the MDI group and those undergoing SAP therapy, along with the corresponding between-group differences.
In people who switched to SAP, mean glucose from CGM significantly decreased and TIR increased, while no significant changes were observed in participants in the MDI group (Figures 2B, C). TBR did not significantly change during the study period in both groups (Figure 2D).
People who switched to SAP also had a significant increase in body weight, BMI and fat-free mass and a reduction in fat mass (Figures 3A, B). Changes in ppFEV1 were not significantly different between groups. Estimates of the absolute changes from baseline at each study visit and between-group differences are reported in Table 2.
Figure 3 Model-based means of BMI (A) from baseline through 24 months and 24-month changes in weight, FM and FFM (B) among participants in the MDI versus SAP therapy group. Points estimates (dots) and 95% confidence intervals (bars) were estimated using linear mixed-effects regression models. FM, Fat mass; FFM, Fat-free mass; MDI, Multiple daily injections. SAP, Sensor augmented pump.
In this non-randomized controlled clinical trial, the use of SAP therapy in insulin-requiring adults with CFRD was associated with a significant improvement in glycemic control, expressed as HbA1c, percent of TIR, and mean glucose levels. In addition, SAP therapy induced significant and beneficial changes in body composition with relevant increases in fat-free mass.
Scully KJ et al. recently demonstrated a significant improvement in mostly all CGM-derived glucose control parameters in a 3-month follow-up period after switching 13 adolescents and adults with CFRD from MDI to insulin pumps (32). In that study, average glucose decreased from 189 to 159 mg/dL and TIR increased from 54% to 70%. In our larger trial, we were also able to demonstrate a clinically meaningful difference in HbA1c of ∼1% (12.1 mmol/mol) in the group who switched to SAP therapy as compared to people who continued MDI therapy, with a mean HbA1c value in the SAP group that was below the ADA recommended target of <7% (53 mmol/mol). The reduction in HbA1c was mirrored by an improvement in CGM-derived glucose control parameters with mean glucose falling below 140 mg/dL and TIR exceeding the threshold of 70% after 6 months in SAP treatment. These improvements in glycemic control extended to 2 years, thus indicating a persistent superiority of SAP over MDI.
We also demonstrated that an optimized glycemic control, obtained with such a tailored therapy, can be an effective strategy in obtaining a beneficial impact on body composition, as shown by the increased fat-free mass and reduced fat mass in participants who switched to SAP. This effect along with the improved glycemic control is of major relevance in people with CF, and especially in those with CFRD in whom the catabolic state induced by insulin insufficiency increase the risk of poor nutritional status (7). In CF individuals, fat-free mass is an accurate indicator of nutritional status and its depletion is associated with poor lung function and disease-related morbidity and mortality (39, 40).
To our knowledge, this is the first study that provides evidence of the beneficial effects of SAP in terms of both glycemic control and body composition in people with CFRD. The main limitation is the non-randomized study design leading to important differences in the baseline characteristics of the two groups, which, however were addressed by using overlap weighting, an efficient procedure that mimics the attributes of randomized clinical trials. Furthermore, impaired glycemic control is associated with the progression of lung disease and impaired nutritional status (41). Conversely, frequent respiratory infections and exacerbations can also negatively affect insulin sensitivity and body composition (42). However, our study did not collect this information and was not adequately powered to explore the potential role of changes in pulmonary infections as a mechanism for improved glycemic control and body composition. Finally, due to the limited number of subjects enrolled, we were unable to test whether the different SAP devices used by the study participants yielded different results.
In conclusion, this study shows that SAP therapy leads to a significantly better glycemic control than MDI therapy, resulting in increased fat-free mass and suggesting that it may be particularly useful in treating people with CFRD. However, further studies are needed to assess the effect of optimized glucose control with SAP therapy and the associated improvement in nutritional status on long-term outcomes, such as lung function, CF- and CFRD related morbidity, quality of life, and mortality in these individuals.
The data presented in this article are not publicly available due to legal and privacy restrictions, specifically concerning patient confidentiality and participant privacy. Requests to access data should be directed to the corresponding author.
The studies involving humans were approved by the Ethics Committee of the Fondazione IRCCS Ca’ Granda Ospedale Maggiore Policlinico Milano. The studies were conducted in accordance with the local legislation and institutional requirements. Written informed consent for participation in this study was provided by the participants’ legal guardians/next of kin.
VG conceptualized and designed the study, drafted the manuscript and collected data. GA carried out the statistical analyses and critically reviewed the manuscript. LP, LZ, AGr, LCM, VRo, AGa, and VRe collected data. EO, VD, and FB coordinated and supervised data collection, and critically reviewed the manuscript. All authors approved the final manuscript as submitted and agree to be accountable for all aspects of the work. VG is the guarantor of this work and, as such, had full access to all the data in the study and takes responsibility for the integrity of the data and the accuracy of the data analysis.
This study was partly funded by Medtronic. Funding sources had no role in the design or reporting of this study
Preliminary data from this study was presented at the 82nd American Diabetes Association Scientific Sessions, June 3-7, 2022, in New Orleans, LA, and at the 58th “European Association on the Study of Diabetes” Annual Meeting, 19-23 September 2022, in Stockholm.
The authors declare that the research was conducted in the absence of any commercial or financial relationships that could be construed as a potential conflict of interest.
All claims expressed in this article are solely those of the authors and do not necessarily represent those of their affiliated organizations, or those of the publisher, the editors and the reviewers. Any product that may be evaluated in this article, or claim that may be made by its manufacturer, is not guaranteed or endorsed by the publisher.
1. Alves C, Della-Manna T, Albuquerque CTM. Cystic fibrosis-related diabetes: an update on pathophysiology, diagnosis, and treatment. J Pediatr Endocrinol Metab (2020) 33:835–43. doi: 10.1515/jpem-2019-0484
2. Cystic Fibrosis Foundation Patient Registry 2020 Annual Data Report Bethesda, Patient Registry 2019 Annual Data Report. Maryland (2021) Cystic Fibrosis Foundation. Available at: https://www.cff.org/sites/default/files/2021-10/2019-Patient-Registry-Annual-Data-Report.pdf (Access date: February 21, 2023)
3. Blackman SM, Tangpricha V. Endocrine disorders in cystic fibrosis. Pediatr Clin North Am (2016) 6:699–708. doi: 10.1016/j.pcl.2016.04.009
4. Moran A, Diem P, Klein DJ, Levitt MD, Robertson RP. Pancreatic endocrine function in cystic fibrosis. J Pediatr (1991) 118:715–23. doi: 10.1016/S0022-3476(05)80032-0
5. Yoon JC. Evolving mechanistic views and emerging therapeutic strategies for cystic fibrosis-related diabetes. J Endocr Soc (2017) 1:1386–400. doi: 10.1210/js.2017-00362
6. White MG, Maheshwari RR, Anderson SJ, Berlinguer-Palmini R, Jones C, Richardson SJ, et al. In situ analysis reveals that CFTR is expressed in only a small minority of β-cells in normal adult human pancreas. J Clin Endocrinol Metab (2020) 105(5):1366–74. doi: 10.1210/clinem/dgz209
7. Sandouk Z, Khan F, Khare S, Moran A. Cystic fibrosis related diabetes (CFRD) prognosis. J Clin Transl Endocrinol (2021) 26:100278. doi: 10.1016/j.jcte.2021.100278
8. Konrad K, Scheuing N, Badenhoop K, Borkenstein MH, Gohlke B, Schöfl C, et al. Cystic fibrosis-related diabetes compared with type 1 and type 2 diabetes in adults. Diabetes Metab Res Rev (2013) 29:568–75. doi: 10.1002/dmrr.2429
9. Van den Berg JM, Morton AM, Kok SW, Pijl H, Conway SP, Heijerman HG. Microvascular complications in patients with cystic fibrosis-related diabetes (CFRD). J Cyst Fibros (2008) 7:515–9. doi: 10.1016/j.jcf.2008.05.008
10. Schwarzenberg SJ, Thomas W, Olsen TW, Grover T, Walk D, Milla C, et al. Microvascular complications in cystic fibrosis-related diabetes. Diabetes Care (2007) 30:1056–61. doi: 10.2337/dc06-1576
11. Moran A, Dunitz J, Nathan B, Saeed A, Holme B, Thomas W. Cystic fibrosis-related diabetes: current trends in prevalence, incidence, and mortality. Diabetes Care (2009) 32:1626–31. doi: 10.2337/dc09-0586
12. Moran A, Pekow P, Grover P, Zorn M, Slovis B, Pilewski J, et al. Insulin therapy to improve BMI in cystic fibrosis-related diabetes without fasting hyperglycemia: results of the cystic fibrosis related diabetes therapy trial. Diabetes Care (2009) 32:1783–8. doi: 10.2337/dc09-0585
13. Norris AW, Ode KL, Merjaneh L, Sanda S, Yi Y, Sun X, et al. Survival in a bad neighborhood: pancreatic islets in cystic fibrosis. J Endocrinol (2019), JOE–18-0468.R1. doi: 10.1530/JOE-18-0468
14. Prentice BJ, Ooi CY, Strachan RE, Hameed S, Ebrahimkhani S, Waters SA, et al. Early glucose abnorMalities are associated with pulmonary inflammation in young children with cystic fibrosis. J Cyst Fibros (2019) 18:869–73. doi: 10.1016/j.jcf.2019.03.010
15. Ode KL, Frohnert B, Laguna T, Phillips J, Holme B, Regelmann W, et al. Oral glucose tolerance testing in children with cystic fibrosis. Pediatr Diabetes (2010) 11:487–92. doi: 10.1111/j.1399-5448.2009.00632.x
16. Milla CE, Warwick WJ, Moran A. Trends in pulmonary function in patients with cystic fibrosis correlate with the degree of glucose intolerance at baseline. Am J Respir Crit Care Med (2000) 162:891–5. doi: 10.1164/ajrccm.162.3.9904075
17. Dobson L, Hattersley AT, Tiley S, Elworthy S, Oades PJ, Sheldon CD. Clinical improvement in cystic fibrosis with early insulin treatment. Arch Dis Child (2002) 87:430–1. doi: 10.1136/adc.87.5.430
18. Mozzillo E, Franzese A, Valerio G, Sepe A, De Simone I, Mazzarella G, et al. One-year glargine treatment can improve the course of lung disease in children and adolescents with cystic fibrosis and early glucose derangements. Pediatr Diabetes (2009) 10:162–7. doi: 10.1111/j.1399-5448.2008.00451.x
19. Nousia-Arvanitakis S, Galli-Tsinopoulou A, Karamouzis M. Insulin improves clinical status of patients with cystic-fibrosis-related diabetes mellitus. Acta Paediatr (2001) 90:515–9. doi: 10.1080/080352501750197647
20. Foster NC, Beck RW, Miller KM, Clements MA, Rickels MR, DiMeglio LA, et al. State of type 1 diabetes management and outcomes from the T1D exchange in 2016-2018. Diabetes Technol Ther (2019) 2:66–72. doi: 10.1089/dia.2018.0384
21. Prahalad P, Tanenbaum M, Hood K, Maahs DM. Diabetes technology: improving care, improving patient-reported outcomes and preventing complications in young people with Type 1 diabetes. Diabetes Med (2018) 35:419–29. doi: 10.1111/dme.13588
22. Polonsky WH, Hessler D, Ruedy KJ, Beck RW, DIAMOND Study Group. The impact of continuous glucose monitoring on markers of quality of life in adults with type 1 diabetes: further findings from the DIAMOND randomized clinical trial. Diabetes Care (2017) 40:736–41. doi: 10.2337/dc17-0133
23. Bergenstal RM, Garg S, Weinzimer SA, Buckingham BA, Bode BW, Tamborlane WV, et al. Safety of a hybrid closed-loop insulin delivery system in patients with type 1 diabetes. JAMA (2016) 316:1407–8. doi: 10.1001/jama.2016.11708
24. Fernandes N. A randomized trial of closed-loop control in children with type 1 diabetes. N Engl J Med (2020) 383:2484. doi: 10.1056/NEJMc2030417
25. O’Riordan SM, Hindmarsh P, Hill NR, Matthews DR, George S, Greally P, et al. Validation of continuous glucose monitoring in children and adolescents with cystic fibrosis: a prospective cohort study. Diabetes Care (2009) 32:1020–2. doi: 10.2337/dc08-1925
26. Dobson L, Sheldon CD, Hattersley AT. Conventional measures underestimate glycaemia in cystic fibrosis patients. Diabetes Med (2004) 21:691–6. doi: 10.1111/j.1464-5491.2004.01219.x
27. Leclercq A, Gauthier B, Rosner V, Weiss L, Moreau F, Constantinescu AA, et al. Early assessment of glucose abnormalities during continuous glucose monitoring associated with lung function impairment in cystic fibrosis patients. J Cyst Fibros (2014) 13:478–84. doi: 10.1016/j.jcf.2013.11.005
28. Frost F, Dyce P, Nazareth D, Malone V, Walshaw MJ. Continuous glucose monitoring guided insulin therapy is associated with improved clinical outcomes in cystic fibrosis-related diabetes. J Cyst Fibros (2018) 17:798–803. doi: 10.1016/j.jcf.2018.05.005
29. Scully KJ, Sherwood JS, Martin K, Ruazol M, Marchetti P, Larkin M, et al. Continuous glucose monitoring and hbA1c in cystic fibrosis: clinical correlations and implications for CFRD diagnosis. J Clin Endocrinol Metab (2022) 107:e1444–54. doi: 10.1210/clinem/dgab857
30. Hardin DS, Rice J, Rice M, Rosenblatt R. Use of the insulin pump in treat cystic fibrosis related diabetes. J Cyst Fibros (2009) 8:174–8. doi: 10.1016/j.jcf.2008.12.001
31. Sulli N, Bertasi S, Zullo S, Shashaj B. Use of continuous subcutaneous insulin infusion in patients with cystic fibrosis related diabetes: three case reports. J Cyst Fibros (2007) 6:237–40. doi: 10.1016/j.jcf.2006.07.005
32. Scully KJ, Palani G, Zheng H, Moheet A, Putman MS. The effect of control IQ hybrid closed loop technology on glycemic control in adolescents and adults with cystic fibrosis-related diabetes. Diabetes Technol Ther (2022) 24:446–52. doi: 10.1089/dia.2021.0354
33. Scheuing N, Badenhoop K, Borkenstein M, Konrad K, Lilienthal E, Laubner K, et al. Why is insulin pump treatment rarely used in adolescents and young adults with cystic fibrosis-related diabetes? Pediatr Diabetes (2015) 16:10–5. doi: 10.1111/pedi.12158
34. American Diabetes Association Professional Practice Committee. 6. Glycemic targets: standards of medical care in diabetes-2022. Diabetes Care (2022) 45(Suppl 1):S83–96. doi: 10.2337/dc22-S006
35. Li F, Thomas LE, Li F. Addressing extreme propensity scores via the overlap weights. Am J Epidemiol (2019) 188:250–7. doi: 10.1093/aje/kwy201
36. Geraci M, Bottai M. Quantile regression for longitudinal data using the asymmetric Laplace distribution. Biostatistics (2007) 8:140–54. doi: 10.1093/biostatistics/kxj039
37. Benjamini Y, Hochberg Y. Controlling the false discovery rate: A practical and powerful approach to multiple testing. J R Stat Society Ser B (Methodological) (1995) 57:289–300. doi: 10.1111/j.2517-6161.1995.tb02031.x
38. Available at: https://www.biorender.com/.
39. Alicandro G, Bisogno A, Battezzati A, Bianchi ML, Corti F, Colombo C. Recurrent pulmonary exacerbations are associated with low fat free mass and low bone mineral density in young adults with cystic fibrosis. J Cyst Fibros (2014) 13:328–34. doi: 10.1016/j.jcf.2013.11.001
40. Gomes A, Hutcheon D, Ziegler J. Association between fat-free mass and pulmonary function in patients with cystic fibrosis: A narrative review. Nutr Clin Pract (2019) 34:715–27. doi: 10.1002/ncp.10251
41. Prentice BJ, Jaffe A, Hameed S, Verge CF, Waters S, Widger J. Cystic fibrosis-related diabetes and lung disease: an update. Eur Respir Rev (2021) 30(159):200293. doi: 10.1183/16000617.0293-2020
42. Mozzillo E, Franceschi R, Piona C, Passanisi S, Casertano A, Pjetraj D, et al. Diabetes and prediabetes in children with cystic fibrosis: A systematic review of the literature and recommendations of the Italian Society for Pediatric Endocrinology and Diabetes (ISPED). Front Endocrinol (Lausanne) (2021) 12:673539. doi: 10.3389/fendo.2021.673539
Keywords: cystic fibrosis, cystic fibrosis related diabetes, sensor augmented pumps, insulin therapy optimization, insulin pumps, insulin
Citation: Grancini V, Alicandro G, Porcaro LL, Zazzeron L, Gramegna A, Morlacchi LC, Rossetti V, Gaglio A, Resi V, Daccò V, Blasi F and Orsi E (2023) Effects of insulin therapy optimization with sensor augmented pumps on glycemic control and body composition in people with cystic fibrosis-related diabetes. Front. Endocrinol. 14:1228153. doi: 10.3389/fendo.2023.1228153
Received: 24 May 2023; Accepted: 11 August 2023;
Published: 31 August 2023.
Edited by:
James Shaw, Newcastle University, United KingdomReviewed by:
Claudia Piona, University City Hospital of Verona, ItalyCopyright © 2023 Grancini, Alicandro, Porcaro, Zazzeron, Gramegna, Morlacchi, Rossetti, Gaglio, Resi, Daccò, Blasi and Orsi. This is an open-access article distributed under the terms of the Creative Commons Attribution License (CC BY). The use, distribution or reproduction in other forums is permitted, provided the original author(s) and the copyright owner(s) are credited and that the original publication in this journal is cited, in accordance with accepted academic practice. No use, distribution or reproduction is permitted which does not comply with these terms.
*Correspondence: V. Grancini, dmFsZXJpYS5ncmFuY2luaUBwb2xpY2xpbmljby5taS5pdA==
Disclaimer: All claims expressed in this article are solely those of the authors and do not necessarily represent those of their affiliated organizations, or those of the publisher, the editors and the reviewers. Any product that may be evaluated in this article or claim that may be made by its manufacturer is not guaranteed or endorsed by the publisher.
Research integrity at Frontiers
Learn more about the work of our research integrity team to safeguard the quality of each article we publish.