- 1Department of Urology, The First Affiliated Hospital of Zhengzhou University, Zhengzhou, China
- 2Department of Urology Tongji Hospital, Tongji Medical College, Huazhong University of Science and Technology, Wuhan, Hubei, China
- 3Reproductive Medicine Center, Tongji Hospital, Tongji Medical College, Huazhong University of Science and Technology, Wuhan, Hubei, China
Background: The clinical correlation between erectile dysfunction (ED) and depression has been revealed in cumulative studies. However, the evidence of shared mechanisms between them was insufficient. This study aimed to explore common transcriptomic alterations associated with ED and depression.
Materials and methods: The gene sets associated with ED and depression were collected from the Gene Expression Omnibus (GEO) database. Comparative analysis was conducted to obtain common genes. Using R software and other appropriate tools, we conducted a range of analyses, including function enrichment, interactive network creation, gene cluster analysis, and transcriptional and post-transcriptional signature profiling. Candidate hub crosslinks between ED and depression were selected after external validation and molecular experiments. Furthermore, subpopulation location and disease association of hub genes were explored.
Results: A total of 85 common genes were identified between ED and depression. These genes strongly correlate with cell adhesion, redox homeostasis, reactive oxygen species metabolic process, and neuronal cell body. An interactive network consisting of 80 proteins and 216 interactions was thereby developed. Analysis of the proteomic signature of common genes highlighted eight major shared genes: CLDN5, COL7A1, LDHA, MAP2K2, RETSAT, SEMA3A, TAGLN, and TBC1D1. These genes were involved in blood vessel morphogenesis and muscle cell activity. A subsequent transcription factor (TF)–miRNA network showed 47 TFs and 88 miRNAs relevant to shared genes. Finally, CLDN5 and TBC1D1 were well-validated and identified as the hub crosslinks between ED and depression. These genes had specific subpopulation locations in the corpus cavernosum and brain tissue, respectively.
Conclusion: Our study is the first to investigate common transcriptomic alterations and the shared biological roles of ED and depression. The findings of this study provide insights into the referential molecular mechanisms underlying the co-existence between depression and ED.
1 Introduction
Erectile dysfunction (ED) is a common male sexual dysfunction that increases with age (1), and it could have a negative effect on both physical and psychological health for couples. Data reported that 322 million men would suffer from ED to varying degrees by 2025 (2). There are a multitude of factors that contribute to the pathogenesis of ED, including neurogenic, vascular, endocrinological, and psychological factors. Therefore, it is believed that ED is strongly associated with these diseases. For example, ED could be an early predictor of adverse cardiovascular events (3, 4). Understanding the crosslinks between ED and related diseases is vital for their treatment.
Psychological factors are involved in the process of ED. Therefore, ED may be associated with psychological illness. Depression is a common mental illness regarded as one of the most severe public concerns (5). As a result, major depressive disorder has been deemed the most prevalent mental disorder in the world (6). The interactive relationship between ED and depression has been explored in cumulative studies. Depression occurred in 8.7%–43.1% of patients in ED (7, 8). Liu et al. (3) reported that men with depression were 1.39 times more likely to get impotence. Sexual dysfunction was significantly correlated with the severity of depression (9). Furthermore, ED was 2.92 times more likely to lead to depression. Nocturnal penile tumescence time and rigidity were impaired in depressed men. Preliminarily, biological factors have been proposed as contributing to the co-occurrence of these conditions, except for psychological factors. Goldstein et al. (10) postulated that the hypothalamic–pituitary–adrenocortical axis affected by depression could produce excessive catecholamine, impairing cavernosal muscle relaxation and propelling ED. Moreover, decreased testosterone level has been established as a risk factor for ED (11). Chou et al. (12) found that patients with depression had a lower level of testosterone compared with controls, and testosterone therapy showed a promising effect on depressive symptoms. Furthermore, a significant association between sexual dysfunction including ED and the dosage of antidepressants as well as benzodiazepines was noted (13). Furthermore, men with ED treated with phosphodiesterase-5 inhibitors or penile prostheses had lower depression rates than those who did not receive treatment (14). ED and depression are likely caused by multidimensional mechanisms, and evidence from current studies is insufficient to explain their shared pathogenesis.
Microarray and high-throughput sequencing technologies have advanced pathogenetic research in recent years (15). Mining the common alternations at the molecular level will broaden our understanding of potential mechanisms in ED and depression. Therefore, the study aimed to explore shared transcriptomic profiles between ED and depression. In the present article, we have explored the transcriptome specific to ED and depression using tissue and human samples for the first time. Associated biological markers and signaling pathways were identified and validated adequately. This study would contribute to the theoretical research on coexisting ED and depression as well as promising therapeutic guidance for future studies.
2 Materials and methods
2.1 Data source
The transcriptomic data associated with ED and depression were collected from the Gene Expression Omnibus (GEO) database (https://www.ncbi.nlm.nih.gov/geo/) based on the diagnostic criteria of the diseases in December 2022 (1, 16). The present study focused on major depressive disorder, one of the most prevalent mental disorders worldwide. GSE2457, a dataset of ED, deposited expression profiling of corpus cavernosum by array from 10 diabetes-induced ED in rats and controls (17). GSE54564, a dataset of depression, contained expression data of the human brain amygdala from 42 patients with major depressive disorders and non-psychiatric control subjects pair-matched by age and ethnicity (18, 19). In datasets for validation, GSE206528 incorporated single-cell transcriptome of the corpus cavernosum from eight patients with ED and controls (20). Genes related to depression were retrieved from GSE54570, which involved expression data of brain tissue from an array of 26 patients with major depressive disorders and controls (18).
2.2 Identification of common genes between ED and depression
Gene datasets were filtered and normalized after downloading series matrix files of GSE2457 and GSE54564. After converting the probe name and log2 transformation, the differential expression analysis for the normalized data was performed with the assistance of the “limma” package (21) in R software (version 4.2.1, https://www.r-project.org/) to obtain differentially expressed genes (DEGs) between ED or depression and control groups with the threshold of p-value < 0.05. Common DEGs between GSE2457 and GSE54564 were acquired using the intersection function. The results were visualized with a heatmap and Venn diagram by the “pheatmap” package and EVenn (http://www.ehbio.com/test/venn/) (22).
2.3 Functional analysis
The functional enrichment analysis could interpret the biological roles of determining genes. Gene Ontology (GO), including biological processes, cellular components, and molecular function, and pathways, including Kyoto Encyclopedia of Genes and Genomes (KEGG) and WikiPathway enrichment analyses, were conducted in the Database for Annotation, Visualization and Integrated Discovery (DAVID) online tool (http://david.ncifcrf.gov). The screening criteria included gene number >2, Ease < 1, and p-value < 0.05. The results were visualized by the “circlize”, “ggpubr”, and “ggplot2” packages.
2.4 Interactive network and module analysis
Common DEGs between ED and depression were processed in the Search Tool for the Retrieval of Interacting Genes (STRING) database (http://string-db.org) to explore the relationship among proteins of interest. After disregarding disconnected nodes and setting the interaction score > 0.4, a protein–protein interaction (PPI) network was reintroduced in Cytoscape (version 3.7.1, https://cytoscape.org/) for rearrangement. The ClueGO plugin in Cytoscape was used to demonstrate the internal interconnection of ontologies and pathways involved in common DEGs with medium network specificity and showing pathways with p-value < 0.05 (23).
In the rearranging network, the Molecular Complex Detection (MCODE) plugin was utilized to conduct module analysis for representing specific molecular complexes with the threshold of degree cutoff = 2 and node score cutoff = 0.2 (24). To present the relationship and select enriched terms, the Metascape (http://metascape.org) online tool was employed with a threshold of p-value < 0.05 and enrichment factor >1.5 (25).
2.5 Significant shared gene detection and functional interaction
Since dense interaction existed in the present large-scale network, the CytoHubba plugin in Cytoscape was applied to detect significant shared genes based on topological algorithms consisting of BottleNeck, Cluster coefficient, EPC, MCC, and MNC (26). After selecting five ranking methods, the GeneMANIA online tool (https://genemania.org/) was employed to decipher the information on co-localization, co-expression, and functional characterization among these genes (27).
2.6 Transcriptional and post-transcriptional analysis
Considering the underlying regulations between transcription factor (TF), miRNA, and genes, significant shared gene-related TFs and miRNAs analysis was implemented in the NetworkAnalyst online tool (https://www.networkanalyst.ca/) based on the RegNetwork repository (28). Then, the coregulatory interactions were visualized in Cytoscape for an optimal layout.
2.7 External validation of candidate hub crosslinks
To enhance authority and stringency, the significant shared genes were validated in ED- and depression-related datasets. In GSE206528, after merging and filtering low-expression data, the transcriptome was normalized by NormalizeData, and FindAllMarkers was utilized to analyze featured genes between different groups. These processes were conducted by the “Seurat” package. In GSE54570, DEGs were obtained in similar steps to GSE54564 by the “limma” package. p-value < 0.05 was regarded as statistically significant. Furthermore, expression patterns of the significant shared genes were validated in these gene sets to obtain hub crosslinks between ED and depression.
2.8 Experimental validation of candidate hub crosslinks
To experimentally validate the candidate hub crosslinks between ED and depression, the corpus cavernosum samples were harvested from control and ED rats. For ED rats, 8-week-old Sprague-Dawley rats were injected with streptozotocin (60 mg/kg) intraperitoneally. Then, rats with fasting blood glucose levels greater than 16.7 mmol/L 3 and 7 days after the injection were considered to be diabetic. After the administration for 10 weeks, an apomorphine test was conducted to assess erectile function based on our previous study (29), and those rats with negative results were treated as ED rats. Electrostimulation was used later to evaluate ED and control rats’ erectile function (29). The study was approved by the Experimental Animal Administration Committee of Wuhan Servicebio Biotechnology in China.
Quantitative real-time PCR was conducted to determine the mRNA levels of control and ED rats. Based on the corresponding protocols, total RNA from six control and ED rats was extracted using the RNA-easyTM Isolation Reagent (G3013, Servicebio, China). Then, cDNA was synthesized from the RNA samples by the Servicebio®RT First Strand cDNA Synthesis Kit (G3330, Servicebio, China). Quantitative real-time PCR was operated based on 2×SYBR Green qPCR Master Mix (G3320, Servicebio, China). Detailed information on primer sequences is shown in Supplementary Table 1. The mRNA levels of genes were presented as mean ± standard deviation. The comparisons were performed by Student’s t-test and shown in the GraphPad software (LLC, San Diego, California, USA).
2.9 Subpopulation distribution
The Male Health Atlas database (MHA, http://www.malehealthatlas.cn/) (20) was used to excavate the subpopulation distribution of hub genes in cell clusters of the corpus cavernosum. The database of Human Protein Atlas was used to analyze hub gene localization in brain tissue (HPA, https://www.proteinatlas.org/).
3 Results
3.1 Identification of common genes between ED and depression
The research approach of the present study is shown in Figure 1. Differential expression analysis in GSE2457 revealed a total of 1,570 DEGs between the ED and control groups (Figure 2A). Similarly, a total of 1,587 DEGs were excavated between the depression and control groups (Figure 2B). Common genes between ED and depression were obtained by the intersection of these two DEGs. Finally, 85 genes were identified for further analysis.
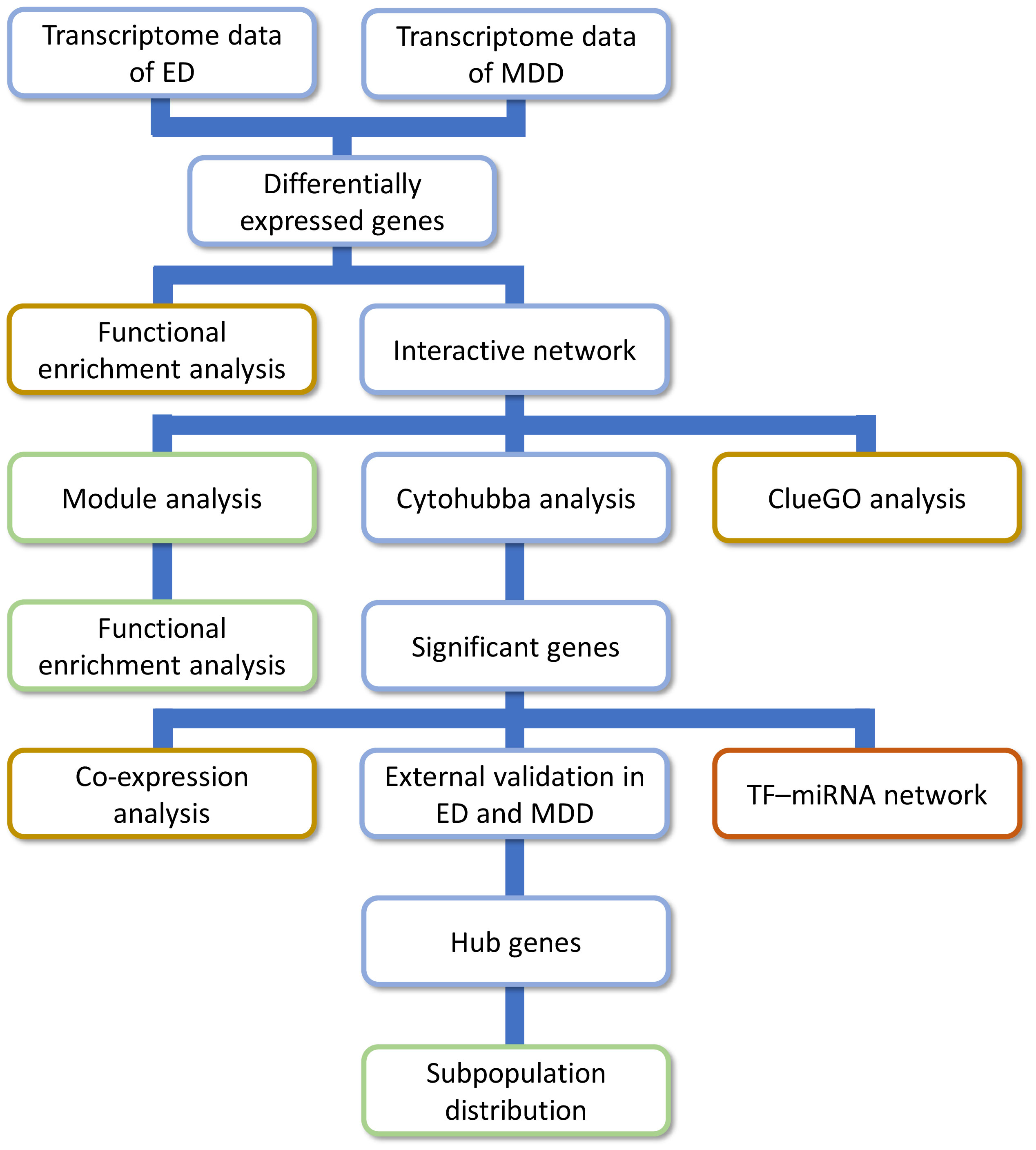
Figure 1 The research approach of the present study. ED, erectile dysfunction; MDD, major depressive disorder.
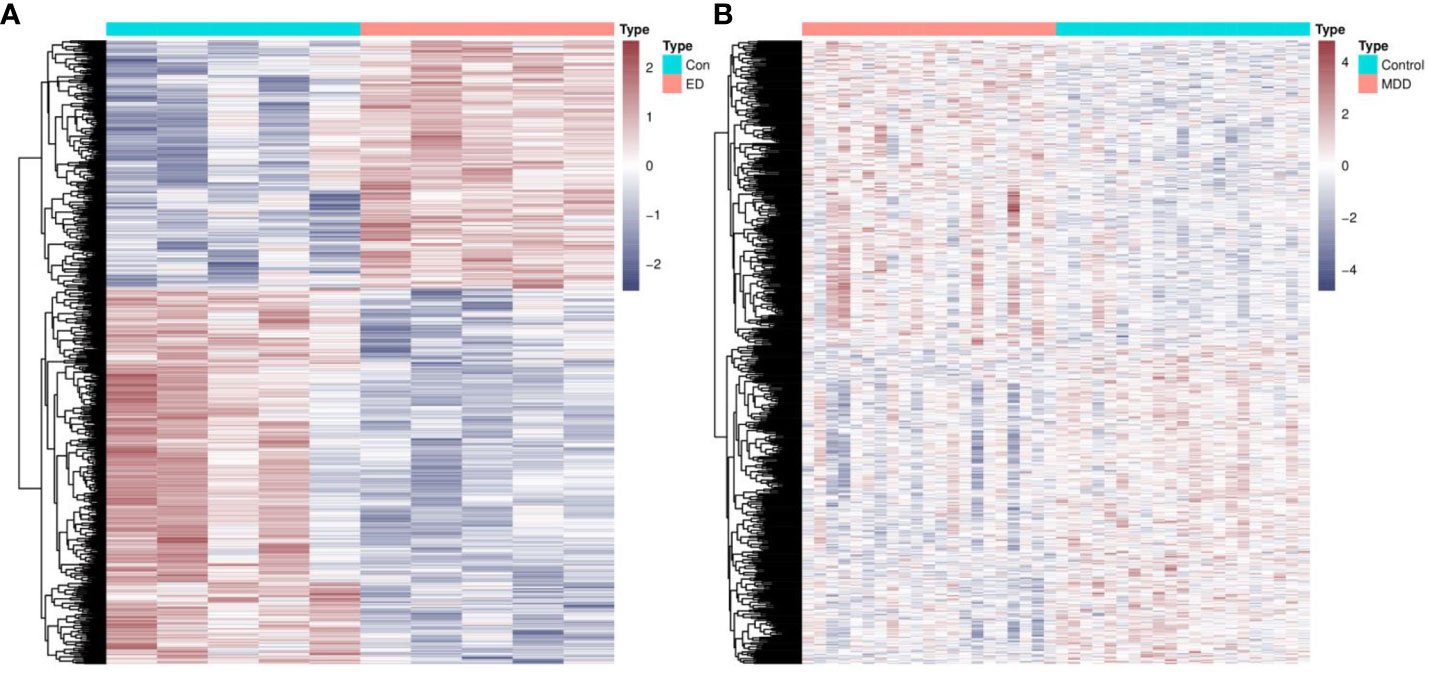
Figure 2 Identification of common genes between ED and depression. (A) The heatmap of DEGs between ED and control groups. (B) The heatmap of DEGs between depression and control groups. ED, erectile dysfunction; DEGs, differentially expressed genes.
3.2 Functional enrichment analysis of common genes
GO analysis revealed the biological functions of common genes between ED and depression (Figure 3A). Biological processes enriched cellular adhesion, redox homeostasis, glutathione metabolism, and oxidant detoxification. The neuronal cell body, dendrite cytoplasm, axon, and lateral plasma membrane were also enriched in cellular component significantly. Furthermore, these genes were associated with glutathione-disulfide reductase activity, actin binding, and structural constituent of muscle in molecular function (Figure 3B). In pathway enrichment analysis, shared pathways contained cell adhesion molecules, one-carbon metabolism, and NRP1-triggered signaling pathways in KEGG and WikiPathway (Figure 3C).
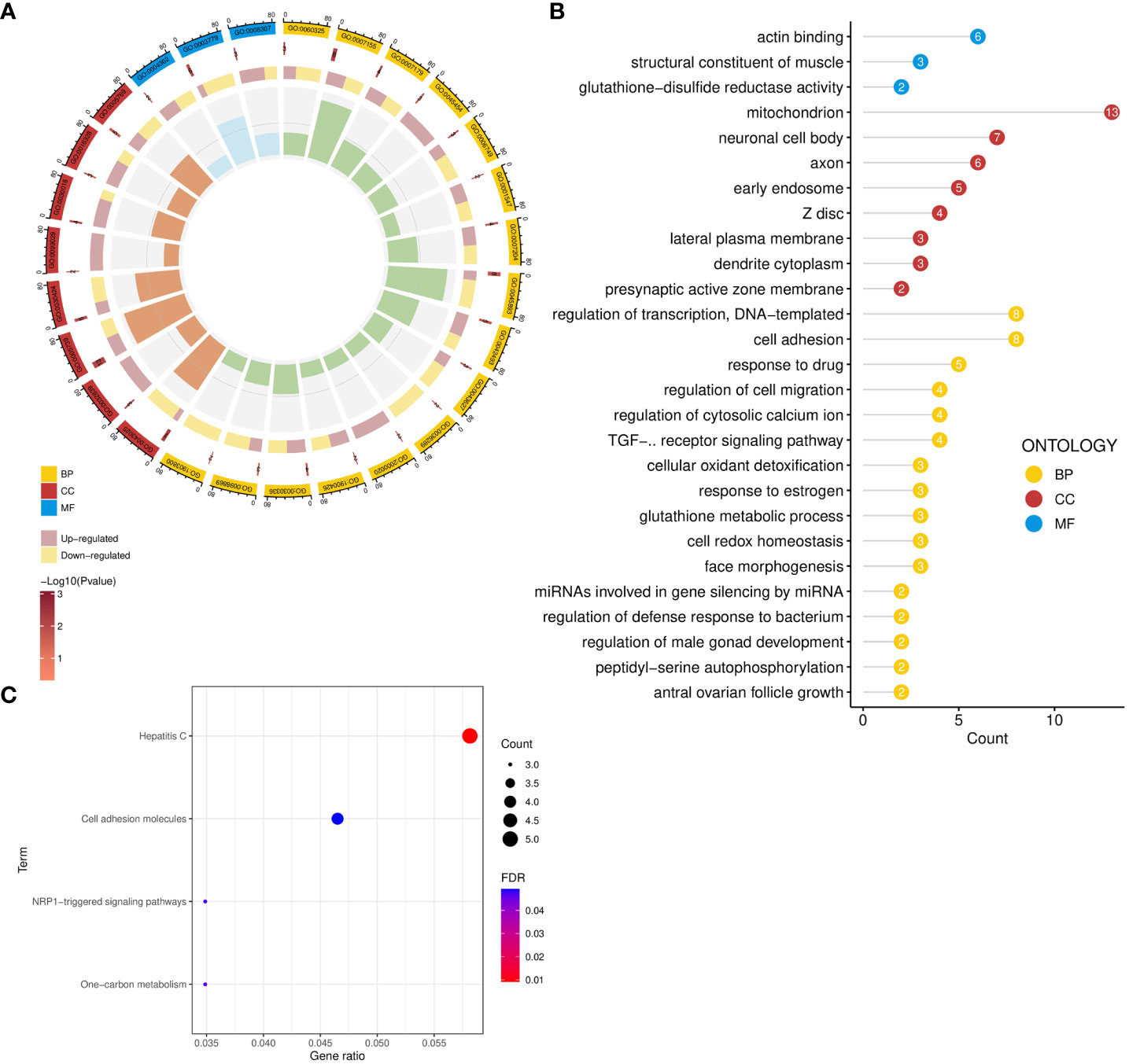
Figure 3 Functional enrichment analysis of common genes. (A, B) GO enrichment analysis of common genes. (C) Pathway enrichment analysis of common genes. GO, Gene Ontology; BP, biological process; CC, cellular component; MF, molecular function.
3.3 Construction of an interactive network and module analysis
A total of 80 protein nodes and 216 interactions constitute an interactive network (Figure 4A). In addition to the functions associated with GO and pathway enrichment analysis, these genes also took part in the regulation of reactive oxygen species metabolism, JNK cascade, cell–cell contact zone, and Wnt signaling pathway. Furthermore, long-chain fatty acid and amino acid metabolic processes were observed in the internal interconnection of ontologies and pathways (Figure 4B).
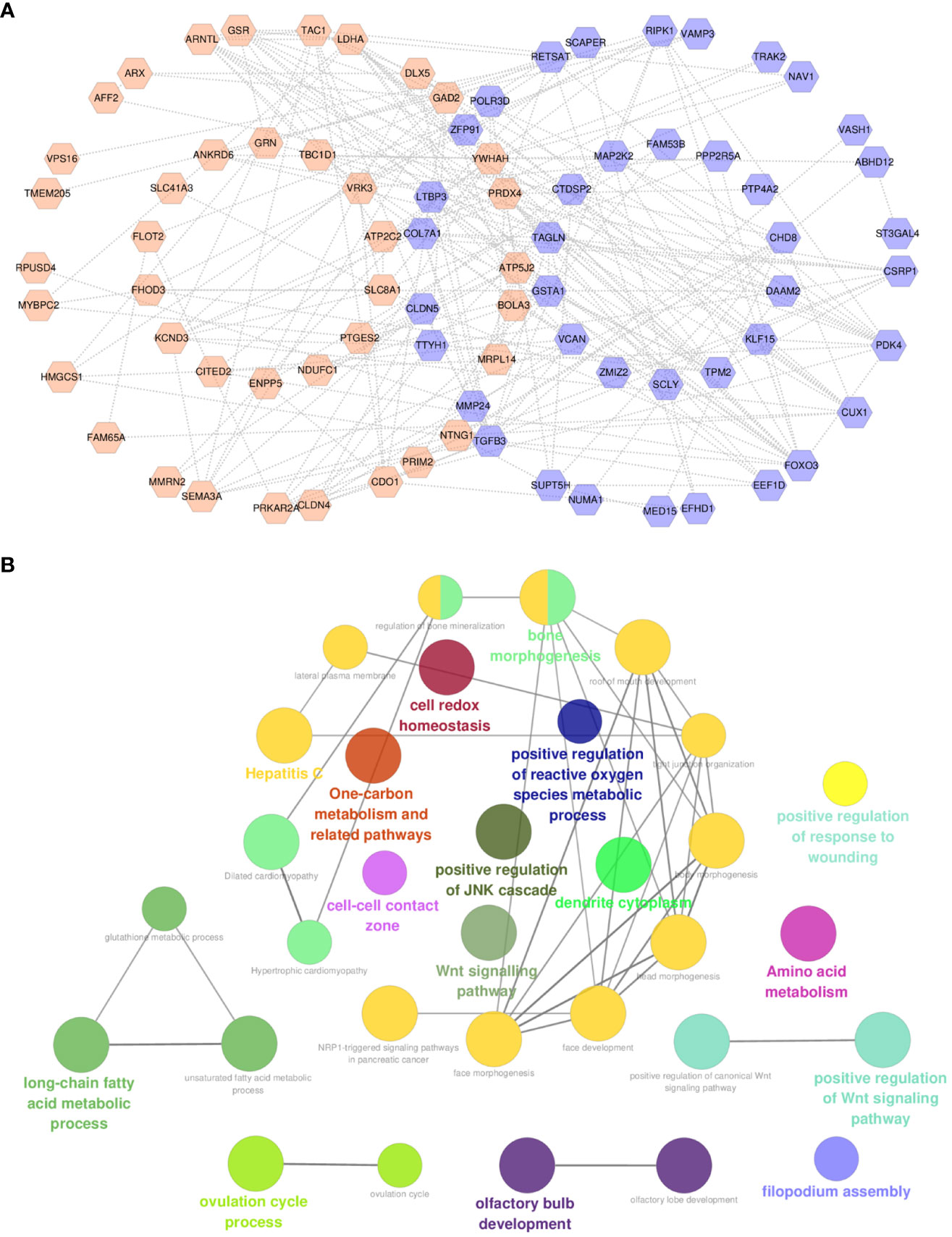
Figure 4 Construction of interactive network and functional analysis. (A) Protein–protein interactive network of common genes. (B) ClueGO functional analysis of common genes. For (A), orange labels represent upregulated CPRGs and purple labels denote downregulated genes.
Different gene clusters were mined based on functional annotation modules in the following steps. A total of three gene clusters were identified (Figure 5A). There were 13 nodes and 24 interactions in cluster 1, focusing on biological regulation, including growth factor stimulus, cellular response to stress, glucose homeostasis, and the PI3K-Akt-mTOR signaling pathway (Figure 5B). Four genes in cluster 2 were associated with response to stimulus and oxidative stress (Figure 5C). In addition, the cellular process and actin cytoskeleton organization were notable in cluster 3 (Figure 5D).
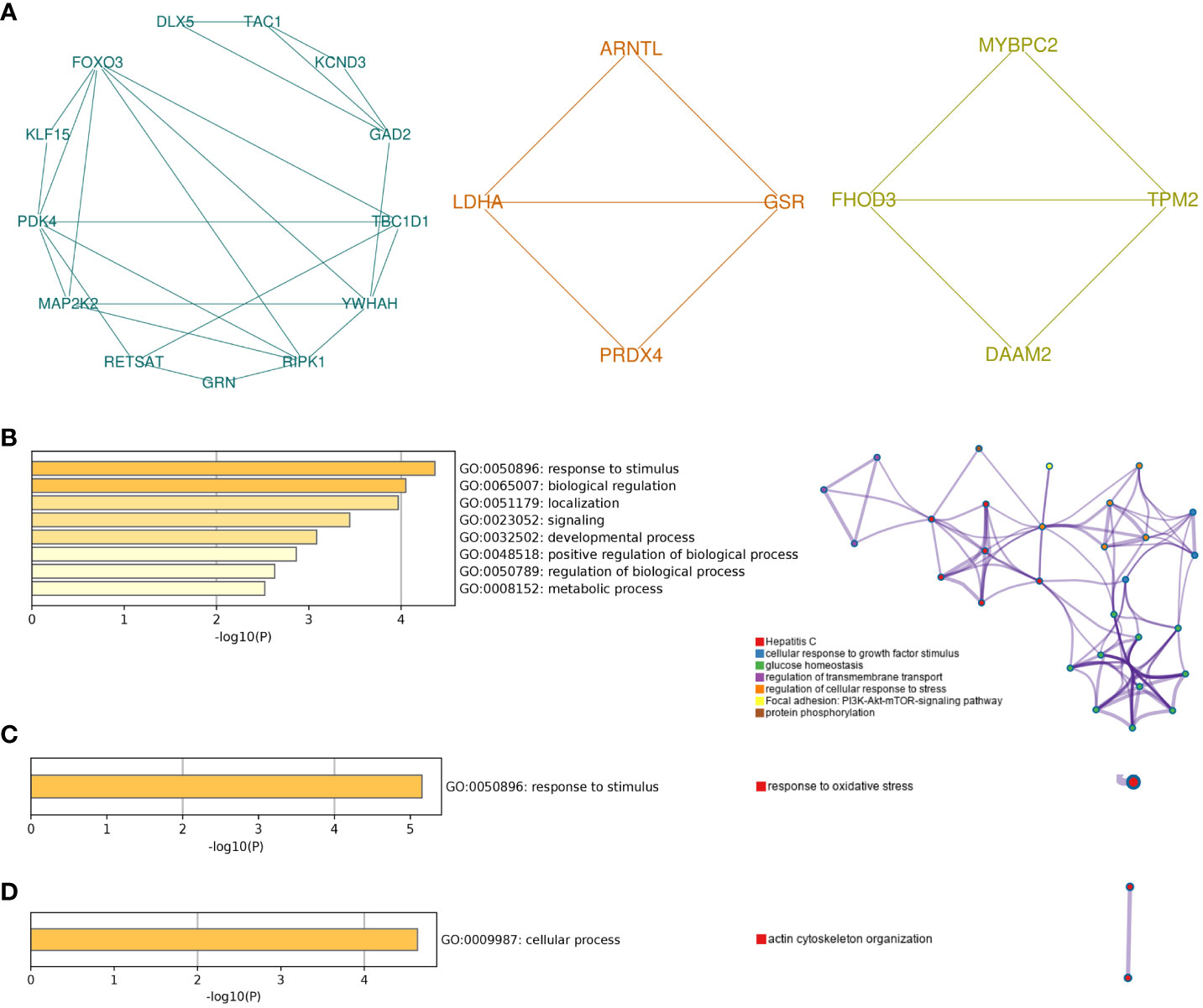
Figure 5 Module analysis of common genes. (A) The top three gene clusters based on module analysis. GO biological process and pathway and process enrichment analysis of cluster 1 (B), cluster 2 (C), and cluster 3 (D). For pathway and process enrichment analysis in (B–D), each node represents an enriched term and is colored by cluster ID. GO, Gene Ontology.
3.4 Analysis of the comprehensive proteomic signature of significant shared genes
To further narrow the gene information and maximize the roles of important genes, comparative analysis based on topological algorithms was conducted, and a total of eight significant shared genes were obtained, namely, CLDN5, COL7A1, LDHA, MAP2K2, RETSAT, SEMA3A, TAGLN, and TBC1D1 (Figure 6A). The interaction between significant shared genes and other genes in the protein–protein network is shown in Figure 6B. Furthermore, based on co-expression and genetic interactions, GeneMANIA indicated that these genes had a close relationship with blood vessel morphogenesis, muscle cell activity, and actin cytoskeleton (Figure 6C). Their biological functions and roles in relevant diseases are listed in Supplementary Table 2.
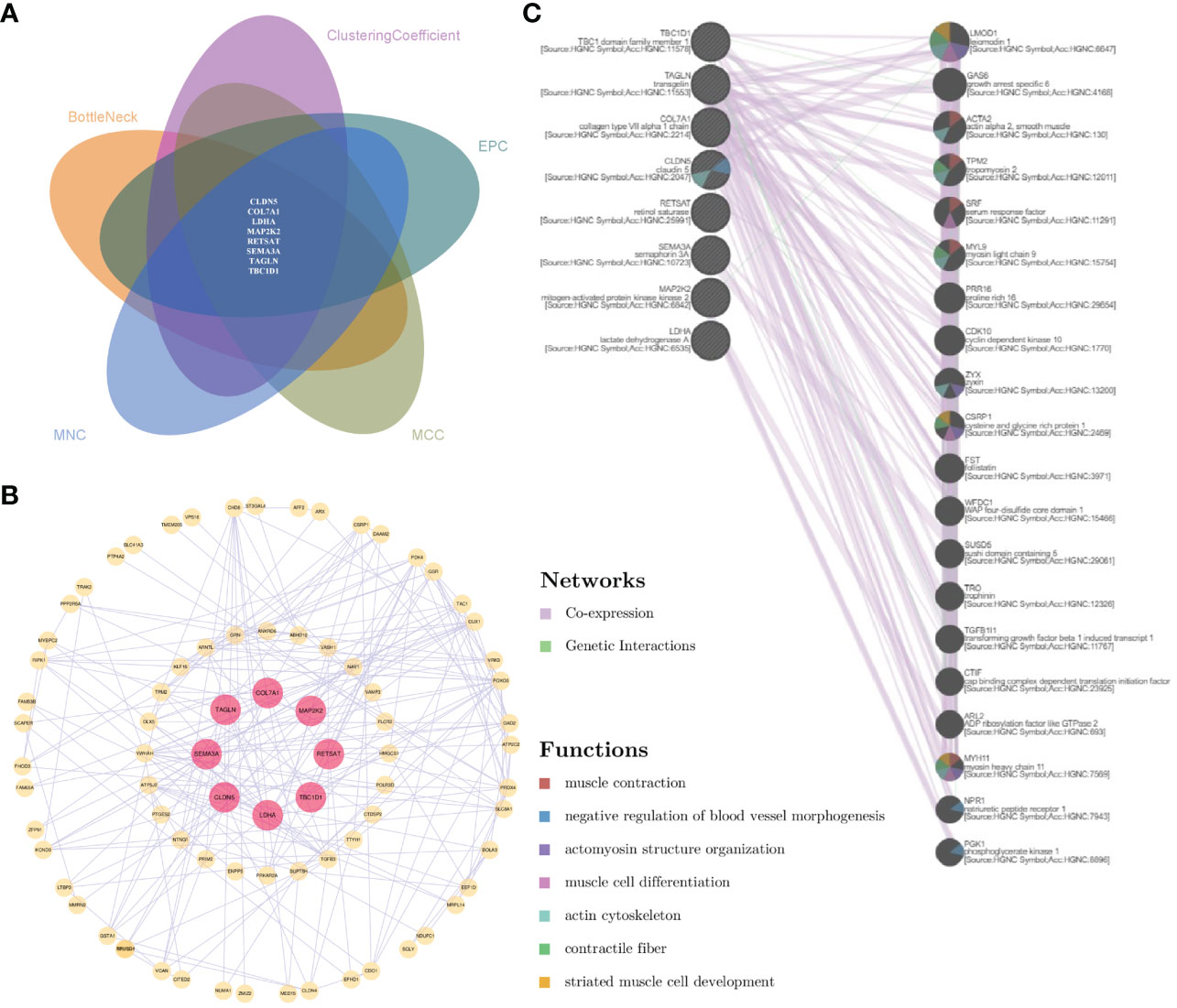
Figure 6 Analysis of the comprehensive proteomic signature of significant shared genes. (A) Comparative analysis via topological algorithms in Cytohubba. (B) Protein–protein interactive network of significant shared genes. (C) The functional interaction in GeneMANIA. For (C), genes on the right side showed the 20 most frequently changed neighboring genes.
3.5 Determination of the transcriptional and post-transcriptional signature
In functional enrichment analysis, miRNA activity was found to be significant in biological processes. Therefore, transcriptional, and post-transcriptional interactions may play an important role in modulating the shared transcriptome. The TF–gene–miRNA network showed 47 TFs and 88 miRNAs (Figure 7A). SEMA3A possessed the most miRNA interactions, and most TFs focused on LDHA. Moreover, TBP and MEF2A were common transcription factors involved in three shared genes, which may play a significant role in ED and depression.
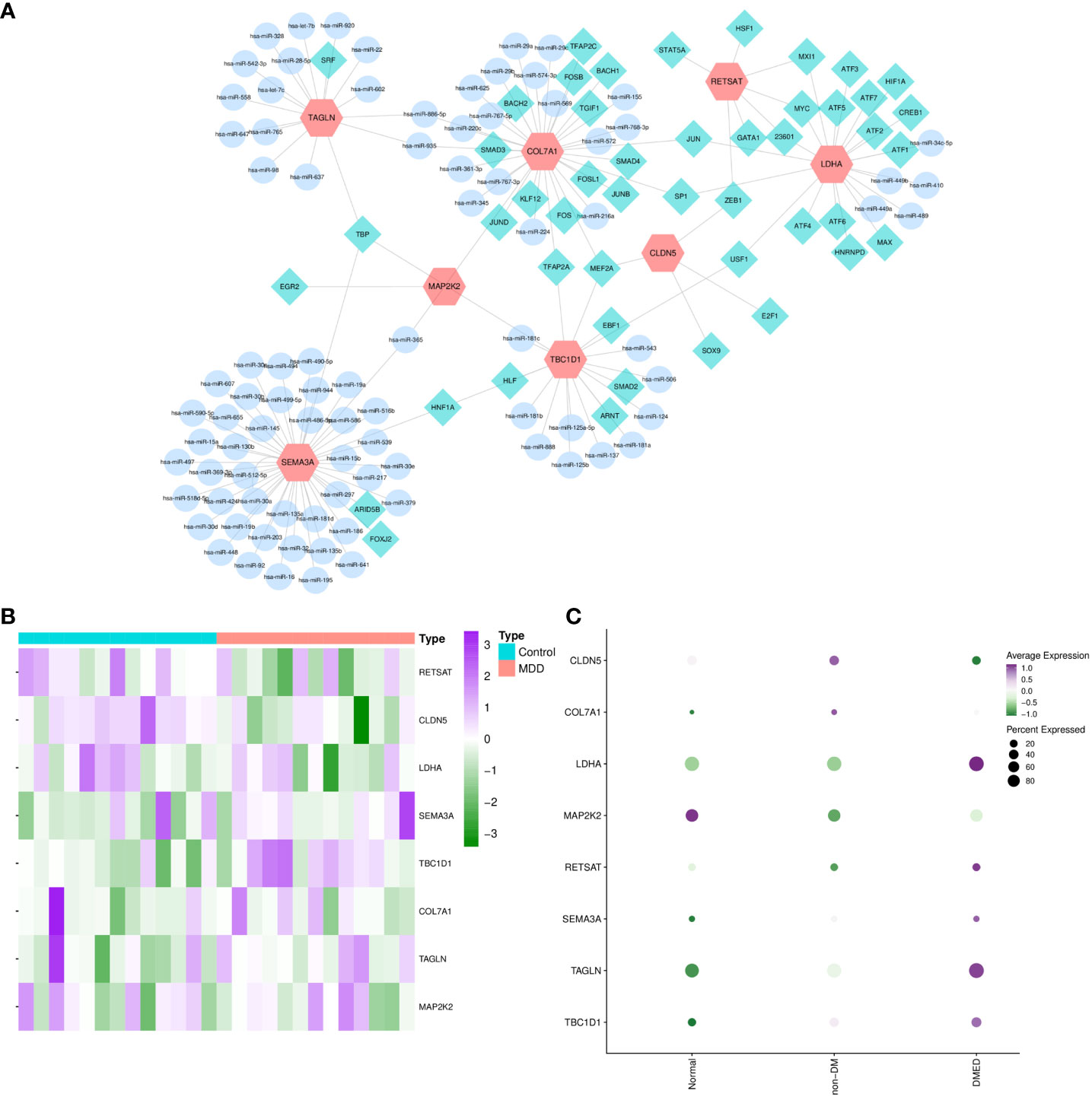
Figure 7 TF–gene–miRNA network and external validation of candidate hub crosslinks. MiRNA–CPRGs network construction and functional enrichment analysis. (A) TF–gene–miRNA co-regulatory network. The profiling of significant shared genes in validated depression (B) and ED-related gene sets (C). MDD, major depressive disorder; ED, erectile dysfunction; DM, diabetes mellitus; DMED, diabetes mellitus-related erectile dysfunction.
3.6 External validation of candidate hub crosslinks
After screening and functional analysis step by step, significant shared genes were validated in ED- and depression-related datasets simultaneously to obtain hub crosslinks. After performing differential expression analysis in GSE206528 and GSE54570, respectively, overlapped genes in the two conditions were compared with shared genes (Figures 7B,C). It was validated and determined that CLDN5 and TBC1D1 were the hubs of genetic links between ED and depression. Later, the expression profiles of CLDN5 and TBC1D1 were validated in ED rats and controls by quantitative real-time PCR. In Supplementary Figure 1, the results of quantitative real-time PCR suggest that notable decreases and increases were observed in the expressions of CLDN5 and TBC1D1 in the ED group compared with the control group (p-value < 0.05), emphasizing the critical genetic links of CLDN5 and TBC1D1 between ED and depression.
3.7 Subpopulation distribution of hub genes between ED and depression
Subpopulation distribution in specific tissues is vital for performing the functions of genes. In MHA, the human corpus cavernosum included 11 types of cells (Figure 8A). TBC1D1 was predominantly localized in vessel endothelial cells, corpus cavernosum, and vessel smooth muscle cells (Figures 8B,C). CLDN5 was found to be enriched in the corpus cavernosum and vessel endothelial cells (Figures 8D, E). In brain tissue, TBC1D1 had a higher distribution in choroid plexus and hippocampal formation. CLDN5 targeted the choroid plexus, thalamus, pons, and cerebral cortex (Figure 8F).
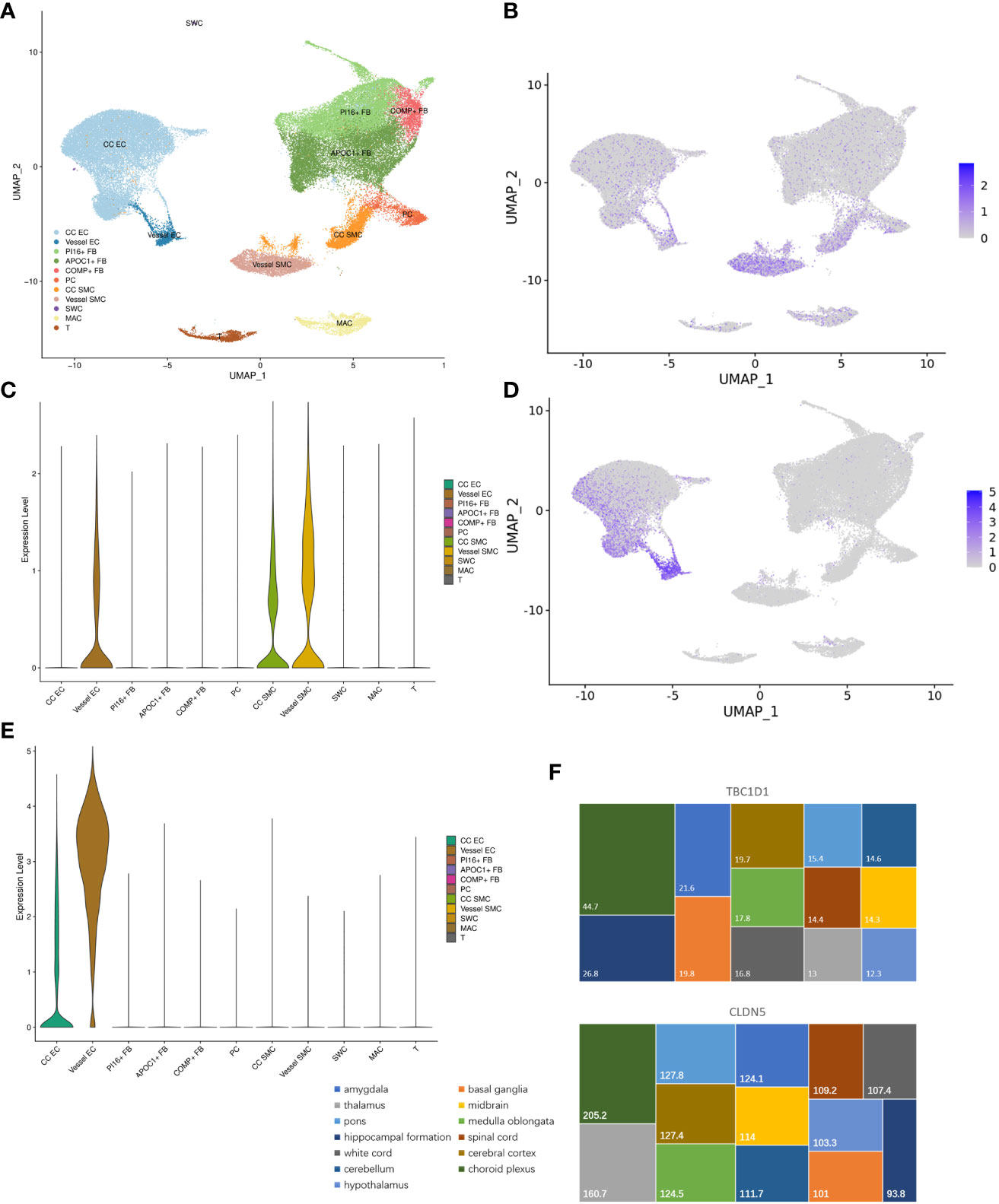
Figure 8 Subpopulation distribution of hub genes between ED and depression. (A) The whole cellular distribution in human corpus cavernosum. (B, C) Expression distribution of TBC1D1 in human corpus cavernosum. (D, E) Expression distribution of CLDN5 in human corpus cavernosum. (F) Expression distribution of TBC1D1 and CLDN5 in brain tissue. ED, erectile dysfunction.
4 Discussion
The presence of both ED and depression significantly impacts the psychosocial wellbeing and quality of life of couples (30). Although high comorbidity between the two conditions is apparent, there remains considerable uncertainty around the pathogenetic nature. There is no clinical, epidemiological association between ED and depression that is sufficient to explain the co-occurrence of the diseases. In this study, we comprehensively analyzed the shared transcriptome between ED and depression using multi-omics methods for the first time. Hub crosslinked genes and correlative signaling pathways were identified and validated based on multi-dimension data.
Considering the heterogeneity in ED and depression-related tissues, the differential expression and comparative analysis identified only 85 common genes. Functional enrichment analysis revealed that these genes were involved in cell redox homeostasis, glutathione metabolic process, glutathione-disulfide reductase activity, and cellular oxidant activities. The results indicate that oxidative stress is crucial in ED and depression. Cumulative reactive oxygen species during ED inhibit the synthesis and bioavailability of NO, which is an important mediator of endothelium-dependent vasodilation in the corpus cavernosum (31). NADPH oxidases are the main source of endogenous reactive oxygen species, which have been noted in ED tissues (29). The use of antioxidants has shown promising results in the treatment of ED. Owing to high oxygen consumption and weak defenses, the brain is more susceptible to oxidative injury, and oxidative stress is believed to be the main cause of major depressive disorder (32). The activity of glutathione peroxidases is lowered and contributes to reactive oxygen species in patients with depression (33). The maintenance of cellular redox homeostasis was crucial in ED and depression, indicating the important shared mechanism.
Since neurologic disorders may play a significant role in linking ED and depression, cellular component analysis was expected to enrich neuronal cell body, dendrite cytoplasm, and axon. Pathway enrichment analysis revealed changes in NRP1-triggered signaling pathways and cell adhesion molecules. In a model of mouse depression, NRP1 was found to be involved in hippocampal neurogenesis and neuroplasticity via miR-30 (34). Neuronal cell adhesion could also be considered a potential marker in antidepressant response (35). Dysfunction in endothelial cell-to-cell junctions was found to be significant in the pathogenesis of hypercholesterolemia-induced ED (36). Subsequent functional enrichment in gene clusters focused on the reactive oxygen species metabolic process, the cell–cell contact zone, and the nutrient metabolic process. It is important to consider these factors in relation to the shared association between ED and depression in future studies.
After a comprehensive analysis of the proteomic signature, a total of eight significant shared genes, namely, CLDN5, COL7A1, LDHA, MAP2K2, RETSAT, SEMA3A, TAGLN, and TBC1D1, were identified. COL7A1 encodes the alpha chain of type VII collagen. Available research concentrated on COL7A1 in all forms of dystrophic epidermolysis bullosa (37, 38). As an important component of the tumor microenvironment, COL7A1 demonstrated prognostic value in patients with gastric cancer and pancreatic cancer (39, 40). Further study of COL7A1 was warranted due to its promising function. LDHA belongs to the lactate dehydrogenase family. It is associated with pyruvate metabolism, glycolysis, and oxidoreductase activity (41). Significant change in LDHA was observed in cerebral gluconeogenesis in chronic stress-related depression (42). Gong et al. (43) reported that Angelicae Sinensis Radix modulated energy metabolism in depression by inhibiting the expression of LDHA. Since oxidoreductase activity was predominant in the process of ED, it was plausible to explore LDHA in the corpus cavernosum. MAP2K2 plays a critical role in mitogen growth factor signal transduction, which is enriched in the central nervous system. It is lower in individuals with psychiatric disorders (44). Similarly, it is also involved in the process of ED (45). RETSAT, known as retinol saturase, is upregulated in ED and diabetic rats (46). It is also a potent modulator of the cellular response to oxidative stress and the generation of reactive oxygen species in vivo and in vitro (47). SEMA3A is a member of the semaphorin family with an Ig-like C2-type domain and is involved in axon guidance and neuronal connectivity. Existing evidence indicates that SEMA3A alleles are associated with genetic disorders in the central nervous system, including autism spectrum disorders and neuronal migration (48). Zhou et al. (49) demonstrated that rs139438618 at the SEMA3A locus is significantly associated with the comorbidity of alcohol dependence and major depressive disorder. Whether SEMA3A is related to ED is unknown, and this may be explored in the future. TAGLN is ubiquitously expressed in vascular and visceral smooth muscle as a marker of smooth muscle differentiation. In a model of myostatin homozygous mutant knockout pig, increased expression of TAGLN was noted in the penile corpus cavernosum, which could be a target for treating ED (50). Moreover, angiogenesis may be a possible link between TAGLN and depression (51–53).
After identification and validation, CLDN5 and TBC1D1 were regarded as the hub genetic links of ED and depression. CLDN5, also known as Claudin 5, belongs to endothelium-specific cellular junction proteins. Its decrease could reduce endothelial content in the corpus cavernosum and deteriorate ED (36). Furthermore, its family may serve as an endothelial barrier to reflect hemodynamic changes in the corpus cavernosum (54). On the other hand, CLDN5 was associated with blood–brain barrier permeability. Menard et al. (55) found that social stress would inhibit the expression of CLDN5, destroy blood–brain barrier integrity, and induce depression ultimately (56). Furthermore, TNF-α could affect blood–brain barrier permeability and CLDN5 expression of endothelial cells in major depressive episodes (57). Stress-related brain entry of inflammatory molecules and IL-6–CLDN5 interaction are also involved in the development of depression (58). TBC1D1 plays a role in regulating cell growth and differentiation. In the available studies, TBC1D1 was not reported to be involved in the pathogenesis of ED or depression, but it is one of the suicide attempt polygenes that may have a link to depression (59). Therefore, it is significant to explore the specific mechanisms of CLDN5 and TBC1D1 in the crosslink of ED and depression.
Some limitations were found in this study. Although comprehensive excavation and validation were conducted based on tissue and human samples, biases could exist due to limited sample size, diabetic state, and heterogeneity across different species. A total of 85 genes generated from comparative analysis lacked appropriate statistical correction. Furthermore, these findings could not establish a causal relationship between the shared transcriptomic profiles of the identified genes and such disorders. Further prospective studies are needed. Moreover, psychosocial factors play an important role in the progress of depression. Chiu et al. (60) reported that social trauma and dissociation contributed to psychotic symptoms in patients with major depressive disorder. The psychosocial contents were insufficient due to the genetic basis of this research. The molecular mechanisms underlying the shared pathogenesis in ED and depression have not been explored enough, and we will investigate these mechanisms in future research.
5 Conclusions
Our multi-omics analysis revealed the shared biological roles and transcriptomic profiles of ED and depression for the first time. After identification and validation, CLDN5 and TBC1D1 were selected as the hub genetic links between the two conditions. These results provided referential molecular mechanisms in concurrent depression and ED.
Data availability statement
The original contributions presented in the study are included in the article/Supplementary Files, further inquiries can be directed to the corresponding author/s.
Author contributions
Conceptualization: PY, YC, and QM; Formal analysis: PY, TS, LC, and YW; Writing-original draft: PY; Funding acquisition: PY and YC; Data collection and analysis: PY, TS, and TL; Review & editing: PY, YC, TL, and QM. All authors contributed to the article and approved the submitted version.
Funding
This work was supported by a grant from the National Natural Science Foundation of China (No. 82201775 and 82201758) and the Medical Science and Technology Research-related joint construction project of Henan Province (No. LHGJ20220343).
Acknowledgments
The authors would like to thank the associated databases for the availability of the data and Home for Researchers editorial team (www.home-for-researchers.com) for language editing service.
Conflict of interest
The authors declare that the research was conducted in the absence of any commercial or financial relationships that could be construed as a potential conflict of interest.
Publisher’s note
All claims expressed in this article are solely those of the authors and do not necessarily represent those of their affiliated organizations, or those of the publisher, the editors and the reviewers. Any product that may be evaluated in this article, or claim that may be made by its manufacturer, is not guaranteed or endorsed by the publisher.
Supplementary material
The Supplementary Material for this article can be found online at: https://www.frontiersin.org/articles/10.3389/fendo.2023.1221043/full#supplementary-material
References
1. Shamloul R, Ghanem H. Erectile dysfunction. Lancet (London England) (9861) 2013:153–65:381. doi: 10.1016/S0140-6736(12)60520-0
2. Ayta IA, McKinlay JB, Krane RJ. The likely worldwide increase in erectile dysfunction between 1995 and 2025 and some possible policy consequences. BJU Int (1999) 84(1):50–6. doi: 10.1046/j.1464-410x.1999.00142.x
3. Liu Q, Zhang Y, Wang J, Li S, Cheng Y, Guo J, et al. Erectile dysfunction and depression: A systematic review and meta-analysis. J Sexual Med (2018) 15(8):1073–82. doi: 10.1016/j.jsxm.2018.05.016
4. Yuan P, Ma D, Zhang Y, Gao X, Wang J, Li R, et al. Analysis of cardiovascular risks for erectile dysfunction in Chinese patients with type 2 diabetes mellitus lacking clinical symptoms of cardiovascular diseases. Trans Androl Urol (2020) 9(6):2500–9. doi: 10.21037/tau-20-999
5. Hao Y, Ge H, Sun M, Gao Y. Selecting an appropriate animal model of depression. Int J Mol Sci (2019) 20(19):4827. doi: 10.3390/ijms20194827
6. Moussavi S, Chatterji S, Verdes E, Tandon A, Patel V, Ustun B. Depression, chronic diseases, and decrements in health: results from the World Health Surveys. Lancet (London England) (2007) 370(9590):851–8. doi: 10.1016/S0140-6736(07)61415-9
7. Weber MF, Smith DP, O'Connell DL, Patel MI, de Souza PL, Sitas F, et al. Risk factors for erectile dysfunction in a cohort of 108 477 Australian men. Med J Australia (2013) 199(2):107–11. doi: 10.5694/mja12.11548
8. Pietrzyk B, Olszanecka-Glinianowicz M, Owczarek A, Gabryelewicz T, Almgren-Rachtan A, Prajsner A, et al. Depressive symptoms in patients diagnosed with benign prostatic hyperplasia. Int Urol Nephrol (2015) 47(3):431–40. doi: 10.1007/s11255-015-0920-5
9. El Yazidi FE, Boualame A, Akammar S, Zahrae Elfahiri F, Aitbenlaassel O, Adali I, et al. [Prevalence and characteristics of sexual dysfunction among Moroccan patients consulting for a first depressive episode]. L'Encephale (2019) 45(6):501–5. doi: 10.1016/j.encep.2019.06.003
10. Goldstein I. The mutually reinforcing triad of depressive symptoms, cardiovascular disease, and erectile dysfunction. Am J Cardiol (2000) 86(2a):41f–5f. doi: 10.1016/S0002-9149(00)00892-4
11. Tsujimura A. The relationship between testosterone deficiency and men's health. World J Men's Health (2013) 31(2):126–35. doi: 10.5534/wjmh.2013.31.2.126
12. Chou PS, Chou WP, Chen MC, Lai CL, Wen YC, Yeh KC, et al. Newly diagnosed erectile dysfunction and risk of depression: a population-based 5-year follow-up study in Taiwan. J Sexual Med (2015) 12(3):804–12. doi: 10.1111/jsm.12792
13. Wainipitapong S, Chiddaycha M, Charoenmakpol N. Sexual dysfunction and associated factors in Thai patients with psychiatric disorders. Gen Psychiatry (2023) 36(2):e100989. doi: 10.1136/gpsych-2022-100989
14. Nackeeran S, Havanur A, Ory J, Althof S, Ramasamy R. Erectile dysfunction is a modifiable risk factor for major depressive disorder: analysis of a federated research network. J Sexual Med (2021) 18(12):2005–11. doi: 10.1016/j.jsxm.2021.09.016
15. Wang Z, Gerstein M, Snyder M. RNA-Seq: a revolutionary tool for transcriptomics. Nat Rev Genet (2009) 10(1):57–63. doi: 10.1038/nrg2484
16. Raison CL, Sanacora G, Woolley J, Heinzerling K, Dunlop BW, Brown RT, et al. Single-dose psilocybin treatment for major depressive disorder: A randomized clinical trial. Jama (2023) 330(9):843–53. doi: 10.1001/jama.2023.14530
17. Sullivan CJ, Teal TH, Luttrell IP, Tran KB, Peters MA, Wessells H. Microarray analysis reveals novel gene expression changes associated with erectile dysfunction in diabetic rats. Physiol Genomics (2005) 23(2):192–205. doi: 10.1152/physiolgenomics.00112.2005
18. Chang LC, Jamain S, Lin CW, Rujescu D, Tseng GC, Sibille E. A conserved BDNF, glutamate- and GABA-enriched gene module related to human depression identified by coexpression meta-analysis and DNA variant genome-wide association studies. PloS One (2014) 9(3):e90980. doi: 10.1371/journal.pone.0090980
19. Wang X, Lin Y, Song C, Sibille E, Tseng GC. Detecting disease-associated genes with confounding variable adjustment and the impact on genomic meta-analysis: with application to major depressive disorder. BMC Bioinf (2012) 13:52. doi: 10.1186/1471-2105-13-52
20. Zhao L, Han S, Su H, Li J, Zhi E, Li P, et al. Single-cell transcriptome atlas of the human corpus cavernosum. Nat Commun (2022) 13(1):4302. doi: 10.1038/s41467-022-31950-9
21. Ritchie ME, Phipson B, Wu D, Hu Y, Law CW, Shi W, et al. limma powers differential expression analyses for RNA-sequencing and microarray studies. Nucleic Acids Res (2015) 43(7):e47. doi: 10.1093/nar/gkv007
22. Chen T, Zhang H, Liu Y, Liu YX, Huang L. EVenn: Easy to create repeatable and editable Venn diagrams and Venn networks online. J Genet Genomics = Yi Chuan xue bao (2021) 48(9):863–6. doi: 10.1016/j.jgg.2021.07.007
23. Bindea G, Mlecnik B, Hackl H, Charoentong P, Tosolini M, Kirilovsky A, et al. ClueGO: a Cytoscape plug-in to decipher functionally grouped gene ontology and pathway annotation networks. Bioinf (Oxford England) (2009) 25(8):1091–3. doi: 10.1093/bioinformatics/btp101
24. Bader GD, Hogue CW. An automated method for finding molecular complexes in large protein interaction networks. BMC Bioinf (2003) 4:2. doi: 10.1186/1471-2105-4-2
25. Zhou Y, Zhou B, Pache L, Chang M, Khodabakhshi AH, Tanaseichuk O, et al. Metascape provides a biologist-oriented resource for the analysis of systems-level datasets. Nat Commun (2019) 10(1):1523. doi: 10.1038/s41467-019-09234-6
26. Chin CH, Chen SH, Wu HH, Ho CW, Ko MT, Lin CY. cytoHubba: identifying hub objects and sub-networks from complex interactome. BMC Syst Biol (2014) 8 Suppl 4(Suppl 4):S11. doi: 10.1186/1752-0509-8-S4-S11
27. Warde-Farley D, Donaldson SL, Comes O, Zuberi K, Badrawi R, Chao P, et al. The GeneMANIA prediction server: biological network integration for gene prioritization and predicting gene function. Nucleic Acids Res (2010) 38:W214–20. doi: 10.1093/nar/gkq537
28. Xia J, Gill EE, Hancock RE. NetworkAnalyst for statistical, visual and network-based meta-analysis of gene expression data. Nat Protoc (2015) 10(6):823–44. doi: 10.1038/nprot.2015.052
29. Yuan P, Ma D, Gao X, Wang J, Li R, Liu Z, et al. Liraglutide ameliorates erectile dysfunction via regulating oxidative stress, the rhoA/ROCK pathway and autophagy in diabetes mellitus. Front Pharmacol (2020) 11:1257. doi: 10.3389/fphar.2020.01257
30. Manalo TA, Biermann HD, Patil DH, Mehta A. The temporal association of depression and anxiety in young men with erectile dysfunction. J Sexual Med (2022) 19(2):201–6. doi: 10.1016/j.jsxm.2021.11.011
31. Ryu JK, Kim DJ, Lee T, Kang YS, Yoon SM, Suh JK. The role of free radical in the pathogenesis of impotence in streptozotocin-induced diabetic rats. Yonsei Med J (2003) 44(2):236–41. doi: 10.3349/ymj.2003.44.2.236
32. Bhatt S, Nagappa AN, Patil CR. Role of oxidative stress in depression. Drug Discovery Today (2020) 25(7):1270–6. doi: 10.1016/j.drudis.2020.05.001
33. Robaczewska J, Kędziora-Kornatowska K, Kucharski R, Nowak M, Muszalik M, Kornatowski M, et al. Decreased expression of heme oxygenase is associated with depressive symptoms and may contribute to depressive and hypertensive comorbidity. Redox Rep Commun Free Radical Res (2016) 21(5):209–18. doi: 10.1080/13510002.2015.1101889
34. Khandelwal N, Dey SK, Chakravarty S, Kumar A. miR-30 family miRNAs mediate the effect of chronic social defeat stress on hippocampal neurogenesis in mouse depression model. Front Mol Neurosci (2019) 12:188. doi: 10.3389/fnmol.2019.00188
35. Fabbri C, Crisafulli C, Gurwitz D, Stingl J, Calati R, Albani D, et al. Neuronal cell adhesion genes and antidepressant response in three independent samples. Pharmacogenom J (2015) 15(6):538–48. doi: 10.1038/tpj.2015.15
36. Ryu JK, Zhang LW, Jin HR, Piao S, Choi MJ, Tuvshintur B, et al. Derangements in endothelial cell-to-cell junctions involved in the pathogenesis of hypercholesterolemia-induced erectile dysfunction. J Sexual Med (2009) 6(7):1893–907. doi: 10.1111/j.1743-6109.2009.01275.x
37. Atanasova VS, Jiang Q, Prisco M, Gruber C, Piñón Hofbauer J, Chen M, et al. Amlexanox enhances premature termination codon read-through in COL7A1 and expression of full length type VII collagen: potential therapy for recessive dystrophic epidermolysis bullosa. J Invest Dermatol (2017) 137(9):1842–9. doi: 10.1016/j.jid.2017.05.011
38. Baardman R, Bremer J, Diercks GFH, Jan SZ, Lemmink HH, Bolling MC, et al. Single glycine deletion in COL7A1 acting as glycine substitution in dystrophic epidermolysis bullosa. J Eur Acad Dermatol Venereol JEADV (2021) 35(9):e597–600. doi: 10.1111/jdv.17328
39. Oh SE, Oh MY, An JY, Lee JH, Sohn TS, Bae JM, et al. Prognostic value of highly expressed type VII collagen (COL7A1) in patients with gastric cancer. Pathol Oncol Res POR (2021) 27:1609860. doi: 10.3389/pore.2021.1609860
40. Geng Y, Guan R, Hong W, Huang B, Liu P, Guo X, et al. Identification of m6A-related genes and m6A RNA methylation regulators in pancreatic cancer and their association with survival. Ann Trans Med (2020) 8(6):387. doi: 10.21037/atm.2020.03.98
41. Huo N, Cong R, Sun ZJ, Li WC, Zhu X, Xue CY, et al. STAT3/LINC00671 axis regulates papillary thyroid tumor growth and metastasis via LDHA-mediated glycolysis. Cell Death Dis (2021) 12(9):799. doi: 10.1038/s41419-021-04081-0
42. Herbet M, Piątkowska-Chmiel I, Motylska M, Gawrońska-Grzywacz M, Nieradko-Iwanicka B, Dudka J. Alteration in the expression of genes involved in cerebral glucose metabolism as a process of adaptation to stressful conditions. Brain Sci (2022) 12(4):498. doi: 10.3390/brainsci12040498
43. Gong W, Zhu S, Chen C, Yin Q, Li X, Du G, et al. The anti-depression effect of angelicae sinensis radix is related to the pharmacological activity of modulating the hematological anomalies. Front Pharmacol (2019) 10:192. doi: 10.3389/fphar.2019.00192
44. Yuan P, Zhou R, Wang Y, Li X, Li J, Chen G, et al. Altered levels of extracellular signal-regulated kinase signaling proteins in postmortem frontal cortex of individuals with mood disorders and schizophrenia. J Affect Disord (2010) 124(1-2):164–9. doi: 10.1016/j.jad.2009.10.017
45. Labazi H, Wynne BM, Tostes R, Webb RC. Metformin treatment improves erectile function in an angiotensin II model of erectile dysfunction. J Sexual Med (2013) 10(9):2154–64. doi: 10.1111/jsm.12245
46. Kazemi E, Zargooshi J, Kaboudi M, Izadi F, Mohammadi Motlagh HR, Kahrizi D, et al. Investigation of gene expression and genetic simultaneous control associated with erectile dysfunction and diabetes. Cell Mol Biol (Noisy-le-Grand France) (2021) 67(3):195–200. doi: 10.14715/cmb/2021.67.3.31
47. Pang XY, Wang S, Jurczak MJ, Shulman GI, Moise AR. Retinol saturase modulates lipid metabolism and the production of reactive oxygen species. Arch Biochem Biophys (2017) 633:93–102. doi: 10.1016/j.abb.2017.09.009
48. Van Battum EY, Brignani S, Pasterkamp RJ. Axon guidance proteins in neurological disorders. Lancet Neurol (2015) 14(5):532–46. doi: 10.1016/S1474-4422(14)70257-1
49. Zhou H, Polimanti R, Yang BZ, Wang Q, Han S, Sherva R, et al. Genetic risk variants associated with comorbid alcohol dependence and major depression. JAMA Psychiatry (2017) 74(12):1234–41. doi: 10.1001/jamapsychiatry.2017.3275
50. Choe HM, Gao K, Paek HJ, Liu XY, Li ZY, Quan BH, et al. Silencing myostatin increases area fraction of smooth muscle in the corpus cavernosum of pigs. Anim Reprod Sci (2022) 247:107077. doi: 10.1016/j.anireprosci.2022.107077
51. Tsuji-Tamura K, Morino-Koga S, Suzuki S, Ogawa M. The canonical smooth muscle cell marker TAGLN is present in endothelial cells and is involved in angiogenesis. J Cell Sci (2021) 134(15):jcs254920. doi: 10.1242/jcs.254920
52. Matsuno H, Tsuchimine S, O'Hashi K, Sakai K, Hattori K, Hidese S, et al. Association between vascular endothelial growth factor-mediated blood-brain barrier dysfunction and stress-induced depression. Mol Psychiatry (2022) 27(9):3822–32. doi: 10.1038/s41380-022-01618-3
53. Almeida OP, Ford AH, Flicker L, Hankey GJ, Yeap BB, Clancy P, et al. Angiogenesis inhibition and depression in older men. J Psychiatry Neurosci Jpn (2014) 39(3):200–5. doi: 10.1503/jpn.130158
54. Wessells H, Sullivan CJ, Tsubota Y, Engel KL, Kim B, Olson NE, et al. Transcriptional profiling of human cavernosal endothelial cells reveals distinctive cell adhesion phenotype and role for claudin 11 in vascular barrier function. Physiol Genomics (2009) 39(2):100–8. doi: 10.1152/physiolgenomics.90354.2008
55. Menard C, Pfau ML, Hodes GE, Kana V, Wang VX, Bouchard S, et al. Social stress induces neurovascular pathology promoting depression. Nat Neurosci (2017) 20(12):1752–60. doi: 10.1038/s41593-017-0010-3
56. Dudek KA, Dion-Albert L, Lebel M, LeClair K, Labrecque S, Tuck E, et al. Molecular adaptations of the blood-brain barrier promote stress resilience vs. Depression Proc Natl Acad Sci U S A (2020) 117(6):3326–36. doi: 10.1073/pnas.1914655117
57. Hochman E, Taler M, Flug R, Gur S, Dar S, Bormant G, et al. Serum claudin-5 levels among patients with unipolar and bipolar depression in relation to the pro-inflammatory cytokine tumor necrosis factor-alpha levels. Brain Behav Immunity (2023) 109:162–7. doi: 10.1016/j.bbi.2023.01.015
58. Gal Z, Torok D, Gonda X, Eszlari N, Anderson IM, Deakin B, et al. Inflammation and blood-brain barrier in depression: interaction of CLDN5 and IL6 gene variants in stress-induced depression. Int J Neuropsychopharmacol (2023) 26(3):189–97. doi: 10.1093/ijnp/pyac079
59. Sokolowski M, Wasserman J, Wasserman D. Polygenic associations of neurodevelopmental genes in suicide attempt. Mol Psychiatry (2016) 21(10):1381–90. doi: 10.1038/mp.2015.187
60. Chiu CD, Li DJ, Hsieh YC, Chou LS, Au JS, Chen YL, et al. Linking childhood trauma and dissociation to psychotic symptoms in major depressive disorder, bipolar disorders, and schizophrenia: A transdiagnostic examination using patient and clinician ratings. psychol Trauma Theory Res Pract Policy (2023). doi: 10.1037/tra0001584
Keywords: erectile dysfunction, depression, biomarker, shared transcriptome, bioinformatics
Citation: Yuan P, Chen Y, Sun T, Cui L, Wei Y, Li T and Meng Q (2023) Exploring potential genes and mechanisms linking erectile dysfunction and depression. Front. Endocrinol. 14:1221043. doi: 10.3389/fendo.2023.1221043
Received: 11 May 2023; Accepted: 20 November 2023;
Published: 04 December 2023.
Edited by:
Hubert Vaudry, Université de Rouen, FranceReviewed by:
Sorawit Wainipitapong, Chulalongkorn University, ThailandRavi Philip Rajkumar, Jawaharlal Institute of Postgraduate Medical Education and Research (JIPMER), India
Copyright © 2023 Yuan, Chen, Sun, Cui, Wei, Li and Meng. This is an open-access article distributed under the terms of the Creative Commons Attribution License (CC BY). The use, distribution or reproduction in other forums is permitted, provided the original author(s) and the copyright owner(s) are credited and that the original publication in this journal is cited, in accordance with accepted academic practice. No use, distribution or reproduction is permitted which does not comply with these terms.
*Correspondence: Penghui Yuan, yuanph2018@126.com; Qingjun Meng, mengqj11@163.com; Yinwei Chen, ywchening@outlook.com