- 1Department of Systems Aging Science and Medicine, Tokyo Metropolitan Institute for Geriatrics and Gerontology (TMIG), Tokyo, Japan
- 2Integrated Research Initiative for Living Well with Dementia (IRIDE), TMIG, Tokyo, Japan
While estrogens are well known for their pivotal role in the female reproductive system, they also play a crucial function in regulating physiological processes associated with learning and memory in the brain. Moreover, they have neuroprotective effects in the pathogenesis of Alzheimer’s disease (AD). Importantly, AD has a higher incidence in older and postmenopausal women than in men, and estrogen treatment might reduce the risk of AD in these women. In general, estrogens bind to and activate estrogen receptors (ERs)-mediated transcriptional machineries, and also stimulate signal transduction through membrane ERs (mERs). Estrogen-related receptors (ERRs), which share homologous sequences with ERs but lack estrogen-binding capabilities, are widely and highly expressed in the human brain and have also been implicated in AD pathogenesis. In this review, we primarily provide a summary of ER and ERR expression patterns in the human brain. In addition, we summarize recent studies on their role in learning and memory. We then review and discuss research that has elucidated the functions and importance of ERs and ERRs in AD pathogenesis, including their role in Aβ clearance and the reduction of phosphorylated tau levels. Elucidation of the mechanisms underlying ER- and ERR-mediated transcriptional machineries and their functions in healthy and diseased brains would provide new perspectives for the diagnosis and treatment of AD. Furthermore, exploring the potential role of estrogens and their receptors, ERs, in AD will facilitate a better understanding of the sex differences observed in AD, and lead to novel sex-specific therapeutic approaches.
1 Introduction
Estrogens, a class of steroid hormones, are one of the major female sex hormones produced primarily in the ovaries and plays a crucial role in the development and maintenance of the female reproductive system and secondary sexual characteristics. Even in non-reproductive tissues and organs, estrogen exerts important effects on various physiological systems in the body, including bone health, cardiovascular health, and brain function in both female and male (1–6). Especially, its decline due to menopause or oophorectomy can lead to several health complications, such as metabolic syndrome, osteoporosis, sarcopenia, frailty, cardiovascular disease, and dementia (6–8).
Of the four major endogenous estrogens in women, estrone (E1), estradiol (E2), estriol (E3), and estetrol (E4), E2 is the most abundant throughout the reproductive lifespan, both in terms of its absolute serum concentration and its potent estrogenic activity (9, 10). Like all steroid hormones, estrogens can readily diffuse across the plasma membrane of cells (11). Inside a cell, they bind to and activate estrogen receptors (ERs), members of the NR3 subgroup of the nuclear receptor superfamily (12–14; Figure 1A). Once activated, ERs modulate the expression of multiple genes at the transcriptional level (15, 16). Humans possess the two primary types of ERs, namely ERα and ERβ, which activate gene transcription by binding to the genomic element known as the estrogen-response element (ERE), typically as a homo- or heterodimer with coactivators, such as steroid receptor coactivator-3 (SRC-3) and p300/CBP (17–19). Both ERα and ERβ are widely expressed in various human tissues, including the reproductive organs, breast tissue, bone, and brain, where they regulate the growth, development, and maintenance of these tissues (20). A subset of ERs associates with the plasma membrane, namely membrane-associated ERα (mERα) and ERβ (mERβ), and belongs to the membrane ERs (mERs) (21–24). These cell surface receptors rapidly activate estrogen signaling through intracellular signaling cascades (Figure 1A). In addition, another mER member, G protein-coupled estrogen receptor 1 (GPER1), also long known as G protein-coupled receptor 30 (GPR30), has been identified in various human tissues, including the reproductive organs, breast tissue, and brain (25–27; Figure 1A).
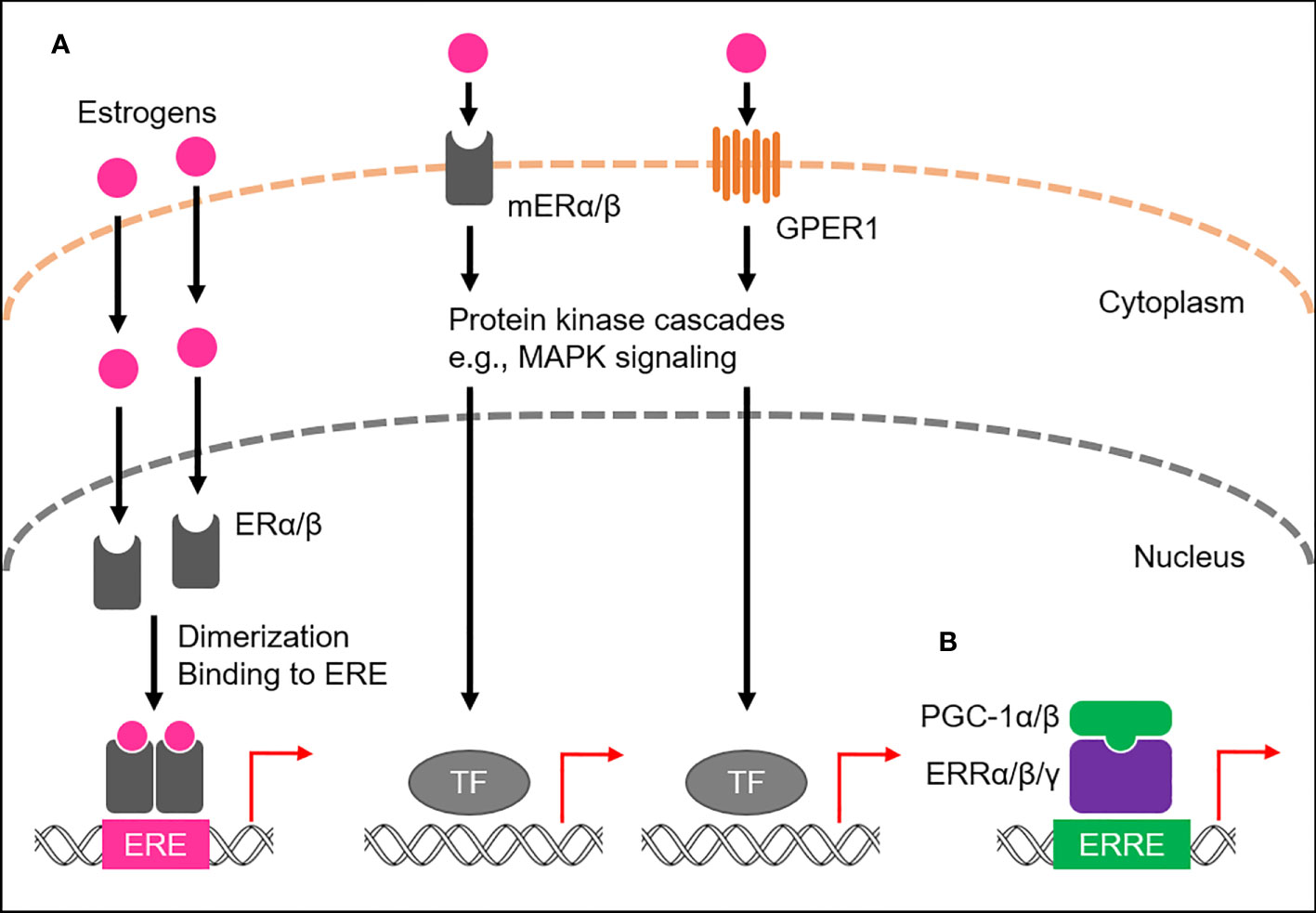
Figure 1 ER- and ERR-mediated transactional regulation. (A) Transactional regulation by ERs, namely ERα and ERβ, and membrane ERs (mERs). Endogenous estrogens bind to ERs or mERs. ERs bind to estrogen responsive elements (EREs) to activate transcription of target genes, while mERs (mERα/β and GPER1) mediate non-genomic effects of estrogens by stimulating activation of the protein kinase cascade, including MAPK signaling, which in turn activates nuclear transcription factors (TFs). (B) Transcriptional regulation by ERRs. ERRs predominantly localize to the nucleus and preferentially bind to estrogen-related receptor responsive elements (ERREs) to transcriptionally regulate the expression of target genes. PGC-1α/β act as co-activators to activate the transcriptional activity of ERRs.
Estrogen-related receptors (ERRs), ERRα, ERRβ, and ERRγ, have been identified as nuclear receptors with substantial sequence similarity to ERs, thus belonging to the NR3 subgroup, but are orphan nuclear receptors because their endogenous ligands have long been unidentified and estrogens are also not their ligands (13, 14, 28; Figure 1B). Instead, their transcriptional activities are tightly regulated by the interactions of peroxisome proliferator-activated receptor-γ (PPARγ) coactivator 1-α (PGC-1α) and PGC-1β, suggesting the molecular function of both PGC-1α and PGC-1β as protein ligands for ERRs (29). ERRs are highly expressed in almost all human tissues, including skeletal muscle, fat, and brain (20, 30), where they play a role in regulating various physiological processes by transcriptionally activating multiple target genes by binding to the specific genomic element, called the estrogen-related response element (ERRE), as a monomer, homodimer or heterodimer (31–33).
This review focuses on the expression and functions of ERs and ERRs in the brain, mainly in humans. In addition, we discuss the implication of their roles in the pathogenesis of Alzheimer’s disease (AD).
2 ER function in the brain and association with AD
In this section, we summarize multiple neuronal functions of ERs and their association with AD.
2.1 Expression of ERs in the brain
The expression of both ER transcripts is widely observed in almost all cell types, namely neurons and glia, throughout the human brain, but with different expression patterns and levels (34, 35) The transcripts of ESR1, the gene encoding ERα, are predominantly expressed in the hypothalamus, amygdala, cerebellum, and cortex, while the transcripts of ESR2, encoding ERβ, are mainly expressed in the hippocampus and cortex, with lower expression levels than ERα (36). At the protein level, ERα immunopositive cells are first detected in the cortex at 9 weeks’ gestation (GW), especially in the proliferating zone and cortical plate, then gradually decrease during prenatal development, but increase again from birth to adulthood (37–40). ERα protein expression has also been demonstrated in the adult human hippocampus. In the human cortical tissue, ERβ initiates to be detected in the proliferating zones at 15 GW and in the cortical plate at 16-17 GW. Furthermore, ERβ protein expression persists into adulthood with a widespread distribution throughout cortical layers II-VI (37–40). Like ERα protein, ERβ has been detected in the human hippocampus from approximately 15 GW into adulthood, primarily in the pyramidal cells of Ammon’s horn and the dentate gyrus (40). Both ERs are expressed in neurons and glial cells in human cortical and hippocampal tissue during fetal development (40). Notably, higher expression of ERβ than ERα has been observed in the adult human cerebral cortex and hippocampus, suggesting that an important role of ERβ in the human brain (37–40). In the rat brain, besides the nuclear localization of ERs, mERα/β proteins are also found in complementary distributions in multiple regions, including the hippocampus and prefrontal cortex (41). Furthermore, GPER1 is widely distributed, with transcript and protein expression detected in nearly all regions of the adult human brain, particularly in the cerebral cortex, cerebellum, and basal ganglia (36, 42).
2.2 The physiological function of estrogens and ERs in the brain
Several factors, including aging and hormonal status, likely influence the expression of the ERs, ERα and ERβ, in the human brain. In the hippocampus of aged human brains, nuclear-localized ERα protein has been shown to increase in the dentate gyrus (DG) and CA3 region, while decreasing in the CA1 region (43, 44), suggesting changes in ERα-mediated transcriptional gene activation in the human brain during aging. In addition, treatment with a major estrogen, E2, increases nuclear-localized ERα in the human brain and maintains ERα-mediated transcription, compensating for hormonal loss during menopause (45). In contrast, GPER1 expression is unlikely to be affected by aging and surgical menopause (46).
The physiological effects of estrogen and ER expression on learning and memory have been better characterized in rodents, such as rats and mice, compared with in humans (47–51). In the rodent brain, estrogens act on the hippocampus, a complex brain structure that is primarily responsible for learning and forming new memories, where they acutely modulate the electrophysiological properties of hippocampal neurons (47, 49, 50). Through ERβ, E2 induces acute potentiation of excitatory postsynaptic currents (EPSCs) by selectively increasing glutamate release at synapses characterized by low initial release probability, while suppressing inhibitory neurotransmission in hippocampal CA1 neurons through ERα (52, 53). In addition, E2 causes a rapid increase in dendritic spine density in the CA1 region of the hippocampus (54) and can also rapidly enhance kainate-induced currents in hippocampal neurons even in the absence of ERs (55). Furthermore, even the membrane-impermeable estrogen, namely E2 conjugated to bovine serum albumin (E2-BSA), which cannot cross the plasma membranes of living cells, is capable of eliciting rapid estrogen signaling (56, 57). These observations suggest that, in addition to the nuclear ERα/β-mediated pathway, estrogens act through a rapid, membrane-initiated signaling pathway, likely mediated by mERα/β and/or GPER1, that activates multiple protein kinase cascades, including mitogen-activated protein kinase (MAPK) signaling, which in turn modulates synaptic plasticity and neuroprotection by stimulating the expression of multiple genes such as brain-derived neurotrophic factor (Bdnf), a master regulator of neuronal cell survival, synaptic plasticity, hippocampal function, and learning, in hippocampal neurons (58–61). Estrogens also undergo metabolic pathways such as sulfation and glucuronidation to form conjugated metabolites that inactivate E2 (62). The balance between these conjugated and unconjugated forms of estrogens in the brain may contribute to brain health and neuroprotection against the neurodegenerative process (63).
2.3 Estrogens and ERs associated with AD
AD is a progressive neurodegenerative disease that affects the brain and leads to cognitive, memory, and behavioral decline (64–67). The neuropathological hallmarks of AD are senile plaques and neurofibrillary tangles (NFTs) (68, 69). Senile plaques are extracellular structures composed predominantly of insoluble deposits of amyloid β peptide (Aβ) that are known to cause neuronal damage and neuronal cell death, while NFTs are aggregates of hyperphosphorylated tau protein within neurons that cause cell death and cognitive impairment in AD (65, 70–72). Aβ pathology likely precedes and accelerates tau pathology, which together trigger neurodegeneration and cognitive decline during AD development (65, 70, 73).
Notably, women have a higher lifetime risk of AD than men; the population of women with dementia is estimated to be approximately 1.69 times higher than the population of men with dementia worldwide, and they have approximately three times higher rates of disease progression with a broader range of cognitive symptoms (74–76). Importantly, despite some controversy, early estrogen replacement therapy (ERT), especially when given before menopause, has been shown to reduce the risk of AD in postmenopausal women (77). Estrogens have also been shown to have neuroprotective effects in the brain of rodent models of AD (78, 79). Moreover, increasing evidence suggests that ERs, including nuclear-localized ERα/β, mERα/β, and GPER1, play a role in AD pathogenesis (24, 45, 80, 81).
2.4 The role of ERs in AD pathogenesis
In the brain of AD patients, increased expression of the nuclear-localized ERα proteins has been observed in neurons of the basal forebrain, nucleus basalis of Meynert (NBM), medial mamillary nucleus (MMN), and hypothalamus, while decreased expression has been observed in hippocampal neurons (45, 80–86). Astrocytes are a subtype of glial cells in the brain and spinal cord (87), and increased numbers of nuclear ERα-positive astrocytes have been observed in the CA1 region of the hippocampus in AD patients (88). For Aβ clearance, ERα has been shown to upregulate the transcription of the Aβ degrading enzyme, neprilysin (NEP), in human cellular models of AD (89) (Figure 2A). Furthermore, ERα colocalizes with NFTs in the hippocampus of AD brains, and also physically interacts with tau protein, and this interaction is increased in AD brains (90). In addition, tau overexpression suppresses ERα transcriptional activity, suggesting that tau inhibits beneficial ERα signaling and neuroprotection through interaction with ERα in AD brains (90).
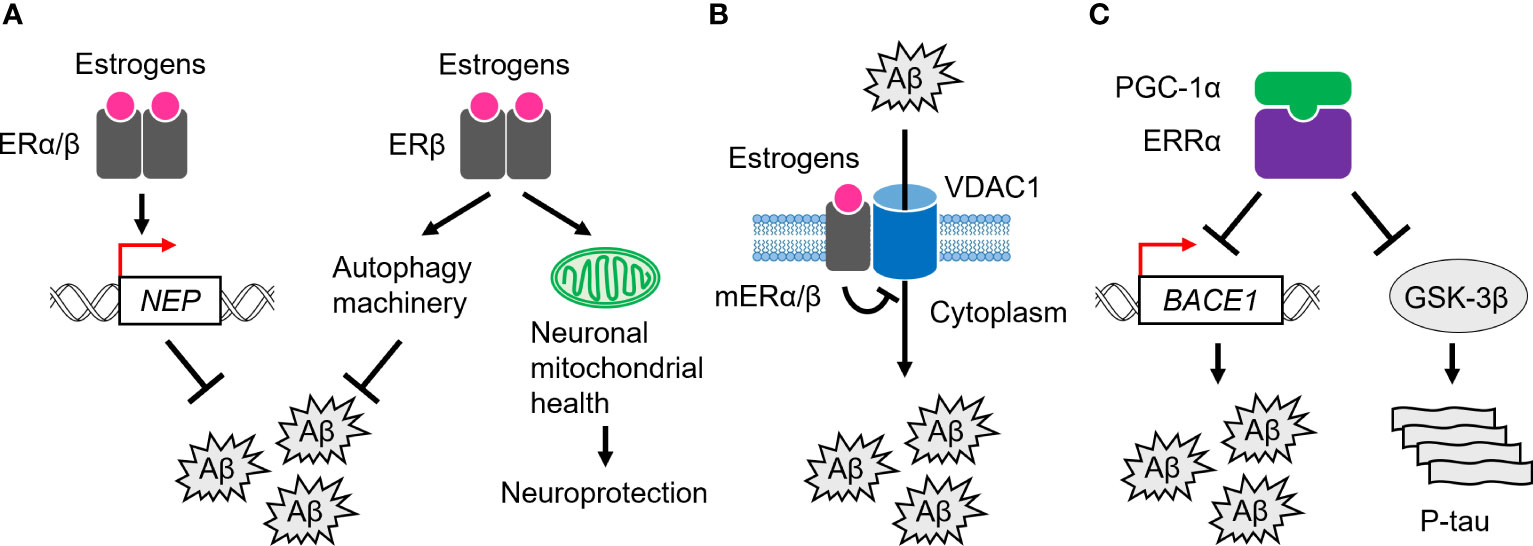
Figure 2 Role of ERs and ERRs in AD pathogenesis. (A) ERα and ERβ are involved in Aβ clearance through transcriptional activation of the neprilysin (NEP) gene. ERβ also activates the autophagy machinery to remove Aβ. In addition, it plays a neuroprotective role by regulating neuronal mitochondrial health. (B) mERα/β inhibit Aβ-induced neurotoxicity by inactivating a channel, VDAC1, at the plasma membrane. (C) ERRα, probably with PGC-1α, suppresses the expression of BACE1 and the kinase activity of GSK-3β, leading to Aβ clearance and tau phosphorylation (P-tau).
Nuclear ERβ proteins were significantly increased in the human NBM and hippocampal neurons in AD patients, whereas less ERβ was expressed in frontal cortex neurons (80, 82, 91). In SH-SY5Y and Swedish mutant (K670N/M671L) amyloid-β precursor protein (APP)-expressing HEK293 cells, which are human cell models of AD, ERβ promotes Aβ degradation by interacting with autophagy related 7 (ATG7) and further enhancing the autophagy machinery (92; Figure 2A). Like ERα, ERβ also upregulates the NEP transcription to promote Aβ clearance in cellular models (89; Figure 2A). These observations suggest that ERβ plays a beneficial role in Aβ clearance in AD. Notably, downregulation of several oxidative phosphorylation (OXPHOS)-related proteins, including ATP synthase subunits and cytochromes, has been demonstrated in the temporal cortex of women with AD and cerebrovascular disease (93). Furthermore, in women, ERβ is associated with the mitochondria in the frontal cortex, and mitochondrial-localized ERβ proteins are decreased in the frontal cortex of women with AD (91). Given that ERβ loss impairs the mitochondrial membrane potential and function, it plays a neuroprotective role in modulating neuronal mitochondrial health modulating mitochondrial health (94–96; Figure 2A).
In the human cortex and hippocampus, mERα associates with voltage-dependent anion-selective channel 1 (VDAC1) at the plasma membrane to inactivate VDAC1 through phosphorylation, which in turn inhibits Aβ cellular entry and Aβ-induced cell death (97–100; Figure 2B). In support of this, activation of mERα/β alone inhibits Aβ-evoked neurotoxicity, oxidative stress, and apoptosis in the mouse primary neurons (101). Indeed, a reduced association of mERα with VDAC1 has been observed in the human cortex of AD brains (100, 102), suggesting the anti-AD capacity of mERα/β.
The neuroprotective contribution of GPER1 in AD has been highlighted in the rodent AD models (103). GPER1 inhibits Aβ-induced oxidative stress and neuronal cell death in rat neuronal cells (104). Moreover, GPER1 has been observed to stimulate extracellular signal-regulated kinase (ERK) signaling in rat hippocampal neurons, leading to activation of synaptic NMDA receptors and trafficking of AMPA receptors into hippocampal synapses, which in turn causes a persistent increase in synaptic efficacy, suggesting a role for GPER1 in modulating neuronal plasticity in neurodegenerative diseases, including AD (105).
3 ERR function in the brain and association with AD
We have reviewed the association between ERRs and AD pathogenesis.
3.1 ERR expression and function in the brain
In humans, the ERRα transcripts, ESRRA, and proteins are widely and highly expressed in almost all regions of the brain, including the hippocampus, cerebral cortex, and cerebellum (36). The ERRγ transcripts, ESRRG, are also detected throughout the human brain, but protein expression was detected at low levels in the cerebral cortex and cerebellum, and undetectable in the hippocampus. Notably, these two ERR proteins localize to neuronal cells, but not to glial cells, within their expressed regions in the human brain. In contrast, the ERRβ transcript, ESRRB, and protein expression are observed at no or very low levels in the human brain (36).
The roles of ERRα and ERRγ in memory and learning have been more extensively studied in rodents compared with in humans (59, 106, 107). The RNA expression pattern of all three ERRs, Esrra, Esrrb, and Esrrg, in the rodent brain is similar to that in the human brain, and the ERRα and ERRγ proteins are abundantly expressed throughout the mouse brain, including the cortex and hippocampus (36, 106, 108, 109), wheras ERRβ is primarily expressed in the developing mouse brain (110). Loss of neuronal ERRγ in the cortex and hippocampus impairs spatial learning and memory in mice (107). Long-term potentiation (LTP) is further impaired in ERRγ-deficient hippocampal neurons, which are rescued by supplementation of the mitochondrial substrate for ATP generation, pyruvate, suggesting a role for ERRγ in regulating neuronal cell metabolism (107). In contrast, the cognitive abilities of ERRα knockout mice were comparable to those of wild-type littermates (106). Meanwhile, endurance exercise increases hippocampal fibronectin type III domain containing 5 (Fndc5) gene expression in mice through an ERRα/PGC-1α transcriptional complex, which in turn stimulates Bdnf gene expression, suggesting that ERRα may act as a mediator of exercise-induced beneficial effects that enhance cognitive function, including learning and memory.
3.2 Role of ERRs in AD
Accumulating evidence suggests a role for the ERR-mediated transcriptional machinery in AD pathogenesis. In APP-expressing HEK293 cells, ERRα inhibits Aβ production (111; Figure 2C). ERRα also downregulates the protein expression level of β-site amyloid precursor protein cleaving enzyme 1 (BACE1), the major β-secretase for Aβ production in neurons (112). However, it has not been demonstrated whether BACE1 gene expression is directly regulated by ERRα at the transcriptional level. Furthermore, ERRα attenuates phosphorylated tau levels with a concomitant reduction in the phosphorylation of glycogen synthase kinase 3β (GSK-3β), an active form of the potent kinase for tau hyperphosphorylation (111; Figure 2C). In the APP/PS1 mice, a mouse model of AD that harbors human transgenes for both APP with the Swedish mutation and presenilin-1 (PSEN1) with the L166P mutation, ERRα RNA and protein expression levels are reduced in the cortex and hippocampus (111). In addition, PGC-1α RNA and protein expression levels are decreased in the AD brains with disease severity (113). Hippocampal PGC-1α protein content is inversely correlated with total Aβ content (113). Although the role of ERRγ in the pathogenesis of AD remains largely unexplored, despite its abundant expression in the human brain, these observations suggest that the ERR/PGC-1α transcriptional complex plays an important role in suppressing both Aβ and tau pathology throughout the progression of AD.
4 Conclusion
Strong evidences suggest that ERs and ERRs play important roles in human brain function, including learning and memory, as well as in the pathogenesis of AD, including the protection against Aβ-induced neurotoxicity and reduction of tau phosphorylation. Overall, however, the molecular mechanisms underlying the neuroprotective effects of ERs and ERRs in AD remain to be elucidated. Further studies are required to fully understand their roles in brain function and AD pathogenesis, which may lead to the development of novel therapeutic targets for the treatment of AD. In addition, exploring the potential roles of sex hormones, including estrogens, and their receptors in AD will help to better understand the sex differences observed in AD, and further lead to new sex-specific therapeutic approaches.
Author contributions
All authors listed have made a substantial, direct, and intellectual contribution to the work and approved it for publication.
Funding
This work was supported by grants from the Japan Society for the Promotion of Science (JSPS) to KS (20K06596), KT (20K07350), and SI (21H04829), and grants from the Kowa Life Science Foundation (KS), Kanzawa Medical Research Foundation (KS), Takeda Science Foundation (KT and SI), Naito Foundation (KT), The Japan Geriatric Society (Research Grant Award in Geriatrics and Gerontology) (KT), and IRIDE in TMIG (KS).
Conflict of interest
The authors declare that the research was conducted in the absence of any commercial or financial relationships that could be construed as a potential conflict of interest.
Publisher’s note
All claims expressed in this article are solely those of the authors and do not necessarily represent those of their affiliated organizations, or those of the publisher, the editors and the reviewers. Any product that may be evaluated in this article, or claim that may be made by its manufacturer, is not guaranteed or endorsed by the publisher.
References
1. Chou CH, Chen MJ. The effect of steroid hormones on ovarian follicle development. Vitam Horm (2018) 107:155–75. doi: 10.1016/bs.vh.2018.01.013
2. Hilton HN, Clarke CL, Graham JD. Estrogen and progesterone signalling in the normal breast and its implications for cancer development. Mol Cell Endocrinol (2018) 466:2–14. doi: 10.1016/j.mce.2017.08.011
3. Noyola-Martínez N, Halhali A, Barrera D. Steroid hormones and pregnancy. Gynecol. Endocrinol (2019) 35:376–84. doi: 10.1080/09513590.2018.1564742
4. Barton BE, Herrera GG, Anamthathmakula P, Rock JK, Willie A, Harris EA, et al. Roles of steroid hormones in oviductal function. Reproduction (2020) 159:R125–37. doi: 10.1530/REP-19-0189
5. Xu XL, Huang ZY, Yu K, Li J, Fu XW, Deng SL. Estrogen biosynthesis and signal transduction in ovarian disease. Front Endocrinol (Lausanne) (2022) 13:827032. doi: 10.3389/fendo.2022.827032
6. Yoh K, Ikeda K, Horie K, Inoue S. Roles of estrogen, estrogen receptors, and estrogen-related receptors in skeletal muscle: regulation of mitochondrial function. Int J Mol Sci (2023) 24:1853. doi: 10.3390/ijms24031853
7. De Paoli M, Zakharia A, Werstuck GH. The role of estrogen in insulin resistance: a review of clinical and preclinical data. Am J Pathol (2021) 191:1490–8. doi: 10.1016/j.ajpath.2021.05.011
8. Geraci A, Calvani R, Ferri E, Marzetti E, Arosio B, Cesari M. Sarcopenia and menopause: the role of estradiol. Front Endocrinol (Lausanne) (2021) 12:682012. doi: 10.3389/fendo.2021.682012
11. Nussey S, Whitehead S. Endocrinology: an integrated approach. Oxford: BIOS Scientific Publishers BIOS Scientific Publishers Limited (2001).
12. Muramatsu M, Inoue S. Estrogen receptors: how do they control reproductive and nonreproductive functions? Biochem Biophys Res Commun (2000) 270:1–10. doi: 10.1006/bbrc.2000.2214
13. Olivares AM, Moreno-Ramos OA, Haider NB. Role of nuclear receptors in central nervous system development and associated diseases. J Exp Neurosci (2015) 9:93–121. doi: 10.4137/JEN.S25480
14. Kobayashi A, Azuma K, Ikeda K, Inoue S. Mechanisms underlying the regulation of mitochondrial respiratory chain complexes by nuclear steroid receptors. Int J Mol Sci (2020) 21:6683. doi: 10.3390/ijms21186683
15. Ikeda K, Horie-Inoue K, Inoue S. Identification of estrogen-responsive genes based on the DNA binding properties of estrogen receptors using high-throughput sequencing technology. Acta Pharmacol Sin (2015) 36:24–31. doi: 10.1038/aps.2014.123
16. Yaşar P, Ayaz G, User SD, Güpür G, Muyan M. Molecular mechanism of estrogen-estrogen receptor signaling. reprod. Med Biol (2017) 16:4–20. doi: 10.1002/rmb2.12006
17. Pettersson K, Svensson K, Mattsson R, Carlsson B, Ohlsson R, Berkenstam A. Expression of a novel member of estrogen response element-binding nuclear receptors is restricted to the early stages of chorion formation during mouse embryogenesis. Mech Dev (1996) 54:211–23. doi: 10.1016/0925-4773(95)00479-3
18. Klinge CM. Estrogen receptor interaction with co-activators and co-repressors. Steroids (2000) 65:227–51. doi: 10.1016/s0039-128x(99)00107-5
19. Yi P, Wang Z, Feng Q, Chou CK, Pintilie GD, Shen H, et al. Structural and functional impacts of ER coactivator sequential recruitment. Mol Cell (2017) 67:733–743.e4. doi: 10.1016/j.molcel.2017.07.026
20. Bookout AL, Jeong Y, Downes M, Yu RT, Evans RM, Mangelsdorf DJ. Anatomical profiling of nuclear receptor expression reveals a hierarchical transcriptional network. Cell (2006) 126:789–99. doi: 10.1016/j.cell.2006.06.049
21. Micevych PE, Kelly MJ. Membrane estrogen receptor regulation of hypothalamic function. Neuroendocrinology (2012) 96:103–10. doi: 10.1159/000338400
22. Soltysik K, Czekaj P. Membrane estrogen receptors - is it an alternative way of estrogen action. J Physiol Pharmacol (2013) 64:129–42.
23. Arnal JF, Lenfant F, Metivier R, Flouriot G, Henrion D, Adlanmerini M, et al. Membrane and nuclear estrogen receptor alpha actions: from tissue specificity to medical implications. Physiol Rev (2017) 97:1045–87. doi: 10.1152/physrev.00024.2016
24. Wnuk A, Przepiórska K, Pietrzak BA, Kajta M. Emerging evidence on membrane estrogen receptors as novel therapeutic targets for central nervous system pathologies. Int J Mol Sci (2023) 24:4043. doi: 10.3390/ijms24044043
25. Prossnitz ER, Arterburn JB, Sklar LA. GPR30: a G protein-coupled receptor for estrogen. Mol Cell Endocrinol (2007) 265–266:138–42. doi: 10.1016/j.mce.2006.12.010
26. Hazell GG, Yao ST, Roper JA, Prossnitz ER, O'Carroll AM, Lolait SJ. Localisation of GPR30, a novel G protein-coupled oestrogen receptor, suggests multiple functions in rodent brain and peripheral tissues. J Endocrinol (2009) 202:223–36. doi: 10.1677/JOE-09-0066
27. Warfvinge K, Krause DN, Maddahi A, Edvinsson JCA, Edvinsson L, Haanes KA. Estrogen receptors α, β and GPER in the CNS and trigeminal system - molecular and functional aspects. J Headache. Pain (2020) 21:131. doi: 10.1186/s10194-020-01197-0
28. Divekar SD, Tiek DM, Fernandez A, Riggins RB. Estrogen-related receptor β (ERRβ)-renaissance receptor or receptor renaissance. Nucl Recept Signal (2016) 14:e002. doi: 10.1621/nrs.14002
29. Di W, Lv J, Jiang S, Lu C, Yang Z, Ma Z, et al. PGC-1: the energetic regulator in cardiac metabolism. Curr Issues Mol Biol (2018) 28:29–46. doi: 10.21775/cimb.028.029
30. Giguère V. Transcriptional control of energy homeostasis by the estrogen-related receptors. Endocr Rev (2008) 29:677–96. doi: 10.1210/er.2008-0017
31. Gearhart MD, Holmbeck SM, Evans RM, Dyson HJ, Wright PE. Monomeric complex of human orphan estrogen related receptor-2 with DNA: a pseudo-dimer interface mediates extended half-site recognition. J Mol Biol (2003) 327:819–32. doi: 10.1016/s0022-2836(03)00183-9
32. Huppunen J, Aarnisalo P. Dimerization modulates the activity of the orphan nuclear receptor ERRgamma. Biochem Biophys Res Commun (2004) 314:964–70. doi: 10.1016/j.bbrc.2003.12.194
33. Barry JB, Laganière J, Giguère V. A single nucleotide in an estrogen-related receptor alpha site can dictate mode of binding and peroxisome proliferator-activated receptor gamma coactivator 1alpha activation of target promoters. Mol Endocrinol (2006) 20:302–10. doi: 10.1210/me.2005-0313
34. Donahue JE, Stopa EG, Chorsky RL, King JC, Schipper HM, Tobet SA, et al. Cells containing immunoreactive estrogen receptor-alpha in the human basal forebrain. Brain Res (2000) 856:142–51. doi: 10.1016/s0006-8993(99)02413-0
35. Chaban VV, Lakhter AJ, Micevych P. A membrane estrogen receptor mediates intracellular calcium release in astrocytes. Endocrinology (2004) 145:3788–95. doi: 10.1210/en.2004-0149
36. Sjöstedt E, Zhong W, Fagerberg L, Karlsson M, Mitsios N, Adori C, et al. An atlas of the protein-coding genes in the human, pig, and mouse brain. Science (2020) 367:eaay5947. doi: 10.1126/science.aay5947
37. Pau CY, Pau KY, Spies HG. Putative estrogen receptor beta and alpha mRNA expression in male and female rhesus macaques. Mol Cell Endocrinol (1998) 146:59–68. doi: 10.1016/s0303-7207(98)00197-x
38. Register TC, Shively CA, Lewis CE. Expression of estrogen receptor alpha and beta transcripts in female monkey hippocampus and hypothalamus. Brain Res (1998) 788:320–2. doi: 10.1016/s0006-8993(98)00036-5
39. Osterlund MK, Gustafsson JA, Keller E, Hurd YL. Estrogen receptor beta (ERbeta) messenger ribonucleic acid (mRNA) expression within the human forebrain: distinct distribution pattern to ERalpha mRNA. J Clin Endocrinol Metab (2000) 85:3840–6. doi: 10.1210/jcem.85.10.6913
40. González M, Cabrera-Socorro A, Pérez-García CG, Fraser JD, López FJ, Alonso R, et al. Distribution patterns of estrogen receptor alpha and beta in the human cortex and hippocampus during development and adulthood. J Comp Neurol (2007) 503:790–802. doi: 10.1002/cne.21419
41. Almey A, Milner TA, Brake WG. Estrogen receptors in the central nervous system and their implication for dopamine-dependent cognition in females. Horm. Behav (2015) 74:125–38. doi: 10.1016/j.yhbeh.2015.06.010
42. Hadjimarkou MM, Vasudevan N. GPER1/GPR30 in the brain: crosstalk with classical estrogen receptors and implications for behavior. J Steroid Biochem Mol Biol (2018) 176:57–64. doi: 10.1016/j.jsbmb.2017.04.012
43. Tohgi H, Utsugisawa K, Yamagata M, Yoshimura M. Effects of age on messenger RNA expression of glucocorticoid, thyroid hormone, androgen, and estrogen receptors in postmortem human hippocampus. Brain Res (1995) 700:245–53. doi: 10.1016/0006-8993(95)00971-r
44. Ishunina TA, Swaab DF. Alterations in the human brain in menopause. Maturitas (2007) 57:20–2. doi: 10.1016/j.maturitas.2007.02.009
45. Ishunina TA, Fischer DF, Swaab DF. Estrogen receptor alpha and its splice variants in the hippocampus in aging and alzheimer's disease. Neurobiol Aging (2007) 28:1670–81. doi: 10.1016/j.neurobiolaging.2006.07.024
46. Prossnitz ER, Barton M. The G-protein-coupled estrogen receptor GPER in health and disease. Nat Rev Endocrinol (2011) 7:715–26. doi: 10.1038/nrendo.2011.122
47. Wong M, Moss RL. Long-term and short-term electrophysiological effects of estrogen on the synaptic properties of hippocampal CA1 neurons. J Neurosci (1992) 12:3217–25. doi: 10.1523/JNEUROSCI.12-08-03217.1992
48. Luine VN, Richards ST, Wu VY, Beck KD. Estradiol enhances learning and memory in a spatial memory task and effects levels of monoaminergic neurotransmitters. Horm. Behav (1998) 34:149–62. doi: 10.1006/hbeh.1998.1473
49. Rudick CN, Gibbs RB, Woolley CS. A role for the basal forebrain cholinergic system in estrogen-induced disinhibition of hippocampal pyramidal cells. J Neurosci (2003) 23:4479–90. doi: 10.1523/JNEUROSCI.23-11-04479.2003
50. Kramár EA, Chen LY, Brandon NJ, Rex CS, Liu F, Gall CM, et al. Cytoskeletal changes underlie estrogen's acute effects on synaptic transmission and plasticity. J Neurosci (2009) 29:12982–93. doi: 10.1523/JNEUROSCI.3059-09.2009
51. Talebi A, Naghdi N, Sepehri H, Rezayof A. The role of estrogen receptors on spatial learning and memory in CA1 region of adult Male rat hippocampus. Iran J Pharm Res (2010) 9:183–91.
52. Smejkalova T, Woolley CS. Estradiol acutely potentiates hippocampal excitatory synaptic transmission through a presynaptic mechanism. J Neurosci (2010) 30:16137–48. doi: 10.1523/JNEUROSCI.4161-10.2010
53. Huang GZ, Woolley CS. Estradiol acutely suppresses inhibition in the hippocampus through a sex-specific endocannabinoid and mGluR-dependent mechanism. Neuron (2012) 74:801–8. doi: 10.1016/j.neuron.2012.03.035
54. Phan A, Gabor CS, Favaro KJ, Kaschack S, Armstrong JN, MacLusky NJ, et al. Low doses of 17β-estradiol rapidly improve learning and increase hippocampal dendritic spines. Neuropsychopharmacology (2012) 37:2299–309. doi: 10.1038/npp.2012.82
55. Gu Q, Korach KS, Moss RL. Rapid action of 17beta-estradiol on kainate-induced currents in hippocampal neurons lacking intracellular estrogen receptors. Endocrinology (1999) 140:660–6. doi: 10.1210/endo.140.2.6500
56. Woolley CS. Acute effects of estrogen on neuronal physiology. Annu Rev Pharmacol Toxicol (2007) 47:657–80. doi: 10.1146/annurev.pharmtox.47.120505.105219
57. Kelly MJ, Rønnekleiv OK. A selective membrane estrogen receptor agonist maintains autonomic functions in hypoestrogenic states. Brain Res (2013) 1514:75–82. doi: 10.1016/j.brainres.2013.03.020
58. Roepke TA, Ronnekleiv OK, Kelly MJ. Physiological consequences of membrane-initiated estrogen signaling in the brain. Front Biosci (Landmark Ed) (2011) 16:1560–73. doi: 10.2741/3805
59. Wrann CD, White JP, Salogiannnis J, Laznik-Bogoslavski D, Wu J, Ma D, et al. Exercise induces hippocampal BDNF through a PGC-1α/FNDC5 pathway. Cell Metab (2013) 18:649–59. doi: 10.1016/j.cmet.2013.09.008
60. Briz V, Liu Y, Zhu G, Bi X, Baudry M. A novel form of synaptic plasticity in field CA3 of hippocampus requires GPER1 activation and BDNF release. J Cell Biol (2015) 210:1225–37. doi: 10.1083/jcb.201504092
61. Xu J, Bai J, Gao F, Xu C, Huang Y, Li D, et al. Anti-inflammatory actions of G-Protein-Coupled estrogen receptor 1 (GPER) and brain-derived estrogen following cerebral ischemia in ovariectomized rats. Biol (Basel) (2023) 12:99. doi: 10.3390/biology12010099
62. Raftogianis R, Creveling C, Weinshilboum R, Weisz J. Estrogen metabolism by conjugation. J Natl Cancer Inst Monogr (2000) 27:113–24. doi: 10.1093/oxfordjournals.jncimonographs.a024234
63. Vitku J, Hill M, Kolatorova L, Kubala Havrdova E, Kancheva R. Steroid sulfation in neurodegenerative diseases. Front Mol Biosci (2022) 9:839887. doi: 10.3389/fmolb.2022.839887
64. Masters CL, Bateman R, Blennow K, Rowe CC, Sperling RA, Cummings JL. Alzheimer's disease. Nat Rev Dis Primers (2015) 1:15056. doi: 10.1038/nrdp.2015.56
65. Knopman DS, Amieva H, Petersen RC, Chételat G, Holtzman DM, Hyman BT, et al. Alzheimer Disease. Nat Rev Dis Primers (2021) 7:33. doi: 10.1038/s41572-021-00269-y
66. Sato K, Takayama KI, Hashimoto M, Inoue S. Transcriptional and post-transcriptional regulations of amyloid-β precursor protein (APP) mRNA. Front Aging (2021) 2:721579. doi: 10.3389/fragi.2021.721579
67. Sato K, Takayama KI, Inoue S. Role of piRNA biogenesis and its neuronal function in the development of neurodegenerative diseases. Front Aging Neurosci (2023) 15:1157818. doi: 10.3389/fnagi.2023.1157818
69. Markesbery WR. Neuropathological criteria for the diagnosis of alzheimer's disease. Neurobiol Aging (1997) 18:S13–9. doi: 10.1016/s0197-4580(97)00064-x
70. Long JM, Holtzman DM. Alzheimer Disease: an update on pathobiology and treatment strategies. Cell (2019) 179:312–39. doi: 10.1016/j.cell.2019.09.001
71. Zhang Z, Yang X, Song YQ, Tu J. ). autophagy in alzheimer's disease pathogenesis: therapeutic potential and future perspectives. Ageing Res Rev (2021) 72:101464. doi: 10.1016/j.arr.2021.101464
72. DeTure MA, Dickson DW. The neuropathological diagnosis of alzheimer's disease. Mol Neurodegener (2019) 14:32. doi: 10.1186/s13024-019-0333-5
73. Nelson PT, Alafuzoff I, Bigio EH, Bouras C, Braak H, Cairns NJ, et al. Correlation of Alzheimer disease neuropathologic changes with cognitive status: a review of the literature. J Neuropathol. Exp Neurol (2012) 71:362–81. doi: 10.1097/NEN.0b013e31825018f7
74. Nichols E, Steinmetz JD, Vollset SE, Fukutaki K, Chalek J, Abd-Allah F, et al. Estimation of the global prevalence of dementia in 2019 and forecasted prevalence in 2050: an analysis for the global burden of disease study 2019. Lancet Public Health (2022) 7:e105–25. doi: 10.1016/S2468-2667(21)00249-8
75. Andersen K, Launer LJ, Dewey ME, Letenneur L, Ott A, Copeland JR, et al. Gender differences in the incidence of AD and vascular dementia: the EURODEM studies. EURODEM incidence research group. Neurology (1999) 53:1992–7. doi: 10.1212/wnl.53.9.1992
76. Marongiu R. Accelerated ovarian failure as a unique model to study peri-menopause influence on alzheimer's disease. Front Aging Neurosci (2019) 11:242. doi: 10.3389/fnagi.2019.00242
77. Rocca WA, Grossardt BR, Shuster LT. Oophorectomy, estrogen, and dementia: a 2014 update. Mol Cell Endocrinol (2014) 389:7–12. doi: 10.1016/j.mce.2014.01.020
78. Xu H, Wang R, Zhang YW, Zhang X. Estrogen, beta-amyloid metabolism/trafficking, and alzheimer's disease. Ann N Y Acad Sci (2006) 1089:324–42. doi: 10.1196/annals.1386.036
79. Li Y, Meeran SM, Patel SN, Chen H, Hardy TM, Tollefsbol TO. Epigenetic reactivation of estrogen receptor-α (ERα) by genistein enhances hormonal therapy sensitivity in ERα-negative breast cancer. Mol Cancer (2013) 12:9. doi: 10.1186/1476-4598-12-9
80. Savaskan E, Olivieri G, Meier F, Ravid R, Müller-Spahn F. Hippocampal estrogen beta-receptor immunoreactivity is increased in alzheimer's disease. Brain Res (2001) 908:113–9. doi: 10.1016/s0006-8993(01)02610-5
81. Perlman WR, Tomaskovic-Crook E, Montague DM, Webster MJ, Rubinow DR, Kleinman JE, et al. Alteration in estrogen receptor alpha mRNA levels in frontal cortex and hippocampus of patients with major mental illness. Biol Psychiatry (2005) 58:812–24. doi: 10.1016/j.biopsych.2005.04.047
82. Ishunina TA, Swaab DF. Increased expression of estrogen receptor alpha and beta in the nucleus basalis of meynert in alzheimer's disease. Neurobiol Aging (2001) 22:417–26. doi: 10.1016/s0197-4580(00)00255-4
83. Hu XY, Qin S, Lu YP, Ravid R, Swaab DF, Zhou JN. Decreased estrogen receptor-alpha expression in hippocampal neurons in relation to hyperphosphorylated tau in Alzheimer patients. Acta Neuropathol (2003) 106:213–20. doi: 10.1007/s00401-003-0720-3
84. Ishunina TA, Swaab DF. Increased neuronal metabolic activity and estrogen receptors in the vertical limb of the diagonal band of broca in alzheimer's disease: relation to sex and aging. Exp Neurol (2003) 183:159–72. doi: 10.1016/s0014-4886(03)00138-9
85. Ishunina TA, Kamphorst W, Swaab DF. Changes in metabolic activity and estrogen receptors in the human medial mamillary nucleus: relation to sex, aging and alzheimer's disease. Neurobiol Aging (2003) 24:817–28. doi: 10.1016/s0197-4580(03)00009-5
86. Hestiantoro A, Swaab DF. Changes in estrogen receptor-alpha and -beta in the infundibular nucleus of the human hypothalamus are related to the occurrence of alzheimer's disease neuropathology. J Clin Endocrinol Metab (2004) 89:1912–25. doi: 10.1210/jc.2003-030862
87. Fields RD, Araque A, Johansen-Berg H, Lim SS, Lynch G, Nave KA, et al. Glial biology in learning and cognition. Neuroscientist (2014) 20:426–31. doi: 10.1177/1073858413504465
88. Lu YP, Zeng M, Hu XY, Xu H, Swaab DF, Ravid R, et al. Estrogen receptor alpha-immunoreactive astrocytes are increased in the hippocampus in alzheimer's disease. Exp Neurol (2003) 183:482–8. doi: 10.1016/s0014-4886(03)00205-x
89. Liang K, Yang L, Yin C, Xiao Z, Zhang J, Liu Y, et al. Estrogen stimulates degradation of beta-amyloid peptide by up-regulating neprilysin. J Biol Chem (2010) 285:935–42. doi: 10.1074/jbc.M109.051664
90. Wang C, Zhang F, Jiang S, Siedlak SL, Shen L, Perry G, et al. Estrogen receptor-α is localized to neurofibrillary tangles in alzheimer's disease. Sci Rep (2016) 6:20352. doi: 10.1038/srep20352
91. Long J, He P, Shen Y, Li R. New evidence of mitochondria dysfunction in the female alzheimer's disease brain: deficiency of estrogen receptor-β. J Alzheimers Dis (2012) 30:545–58. doi: 10.3233/JAD-2012-120283
92. Wei Y, Huang J. Role of estrogen and its receptors mediated-autophagy in cell fate and human diseases. J Steroid Biochem Mol Biol (2019) 191:105380. doi: 10.1016/j.jsbmb.2019.105380
93. Gallart-Palau X, Lee BS, Adav SS, Qian J, Serra A, Park JE, et al. Gender differences in white matter pathology and mitochondrial dysfunction in alzheimer's disease with cerebrovascular disease. Mol Brain (2016) 9:27. doi: 10.1186/s13041-016-0205-7
94. Yang SH, Sarkar SN, Liu R, Perez EJ, Wang X, Wen Y, et al. Estrogen receptor beta as a mitochondrial vulnerability factor. J Biol Chem (2009) 284:9540–8. doi: 10.1074/jbc.M808246200
95. Irwin RW, Yao J, To J, Hamilton RT, Cadenas E, Brinton RD. Selective oestrogen receptor modulators differentially potentiate brain mitochondrial function. J Neuroendocrinol (2012) 24:236–48. doi: 10.1111/j.1365-2826.2011.02251.x
96. Burstein SR, Kim HJ, Fels JA, Qian L, Zhang S, Zhou P, et al. Estrogen receptor beta modulates permeability transition in brain mitochondria. Biochim Biophys Acta Bioenerg (2018) 1859:423–33. doi: 10.1016/j.bbabio.2018.03.006
97. Herrera JL, Fernandez C, Diaz M, Cury D, Marin R. Estradiol and tamoxifen differentially regulate a plasmalemmal voltage-dependent anion channel involved in amyloid-beta induced neurotoxicity. Steroids (2011) 76:840–4. doi: 10.1016/j.steroids.2011.02.014
98. Marin R. Signalosomes in the brain: relevance in the development of certain neuropathologies such as alzheimer's disease. Front Physiol (2011) 2:23. doi: 10.3389/fphys.2011.00023
99. Smilansky A, Dangoor L, Nakdimon I, Ben-Hail D, Mizrachi D, Shoshan-Barmatz V. The voltage-dependent anion channel 1 mediates amyloid β toxicity and represents a potential target for Alzheimer disease therapy. J Biol Chem (2015) 290:30670–3083. doi: 10.1074/jbc.M115.691493
100. Canerina-Amaro A, Hernandez-Abad LG, Ferrer I, Quinto-Alemany D, Mesa-Herrera F, Ferri C, et al. Lipid raft ER signalosome malfunctions in menopause and alzheimer's disease. Front Biosci (Schol. Ed) (2017) 9:111–26. doi: 10.2741/s476
101. Wnuk A, Przepiórska K, Rzemieniec J, Pietrzak B, Kajta M. Selective targeting of non-nuclear estrogen receptors with PaPE-1 as a new treatment strategy for alzheimer's disease. Neurotox Res (2020) 38:957–66. doi: 10.1007/s12640-020-00289-8
102. Ramírez CM, González M, Díaz M, Alonso R, Ferrer I, Santpere G, et al. VDAC and ERalpha interaction in caveolae from human cortex is altered in alzheimer's disease. Mol Cell Neurosci (2009) 42:172–83. doi: 10.1016/j.mcn.2009.07.001
103. Roque C, Baltazar G. G Protein-coupled estrogen receptor 1 (GPER) activation triggers different signaling pathways on neurons and astrocytes. Neural. Regen. Res (2019) 14:2069–70. doi: 10.4103/1673-5374.262577
104. Kurt AH, Yuksel KZ, Uremis N, Uremis MM, Altun I, Bosnak M, et al. Protective effects of G protein-coupled estrogen receptor 1 (GPER1) on β-amyloid-induced neurotoxicity: implications for alzheimer’s disease. Neurochem J (2019) 13:99–104. doi: 10.1134/S1819712419010148
105. Clements L, Alexander A, Hamilton K, Irving A, Harvey J. G-Protein coupled estrogen receptor (GPER1) activation promotes synaptic insertion of AMPA receptors and induction of chemical LTP at hippocampal temporoammonic-CA1 synapses. Mol Brain (2023) 16:16. doi: 10.1186/s13041-023-01003-3
106. Cui H, Lu Y, Khan MZ, Anderson RM, McDaniel L, Wilson HE, et al. Behavioral disturbances in estrogen-related receptor alpha-null mice. Cell Rep (2015) 11:344–50. doi: 10.1016/j.celrep.2015.03.032
107. Pei L, Mu Y, Leblanc M, Alaynick W, Barish GD, Pankratz M, et al. Dependence of hippocampal function on ERRγ-regulated mitochondrial metabolism. Cell Metab (2015) 21:628–36. doi: 10.1016/j.cmet.2015.03.004
108. Tanida T, Matsuda KI, Yamada S, Kawata M, Tanaka M. Immunohistochemical profiling of estrogen-related receptor gamma in rat brain and colocalization with estrogen receptor alpha in the preoptic area. Brain Res (2017) 1659:71–80. doi: 10.1016/j.brainres.2017.01.024
109. Lorke DE, Süsens U, Borgmeyer U, Hermans-Borgmeyer I. Differential expression of the estrogen receptor-related receptor gamma in the mouse brain. Brain Res Mol Brain Res (2000) 77:277–80. doi: 10.1016/s0169-328x(00)00063-2
110. Real MA, Heredia R, Dávila JC, Guirado S. Efferent retinal projections visualized by immunohistochemical detection of the estrogen-related receptor beta in the postnatal and adult mouse brain. Neurosci Lett (2008) 438:48–53. doi: 10.1016/j.neulet.2008.04.044
111. Tang Y, Min Z, Xiang XJ, Liu L, Ma YL, Zhu BL, et al. Estrogen-related receptor alpha is involved in alzheimer's disease-like pathology. Exp Neurol (2018) 305:89–96. doi: 10.1016/j.expneurol.2018.04.003
112. Cai H, Wang Y, McCarthy D, Wen H, Borchelt DR, Price DL, et al. BACE1 is the major beta-secretase for generation of abeta peptides by neurons. Nat Neurosci (2001) 4:233–4. doi: 10.1038/85064
Keywords: estrogen, estrogen receptor, estrogen-related receptor, brain, Alzheimer’s disease
Citation: Sato K, Takayama K-i and Inoue S (2023) Expression and function of estrogen receptors and estrogen-related receptors in the brain and their association with Alzheimer’s disease. Front. Endocrinol. 14:1220150. doi: 10.3389/fendo.2023.1220150
Received: 10 May 2023; Accepted: 20 June 2023;
Published: 04 July 2023.
Edited by:
Ivan Nalvarte, Karolinska Institutet (KI), SwedenReviewed by:
Jonathan Wolf Mueller, University of Birmingham, United KingdomCopyright © 2023 Sato, Takayama and Inoue. This is an open-access article distributed under the terms of the Creative Commons Attribution License (CC BY). The use, distribution or reproduction in other forums is permitted, provided the original author(s) and the copyright owner(s) are credited and that the original publication in this journal is cited, in accordance with accepted academic practice. No use, distribution or reproduction is permitted which does not comply with these terms.
*Correspondence: Satoshi Inoue, c2lub3VlQHRtaWcub3IuanA=