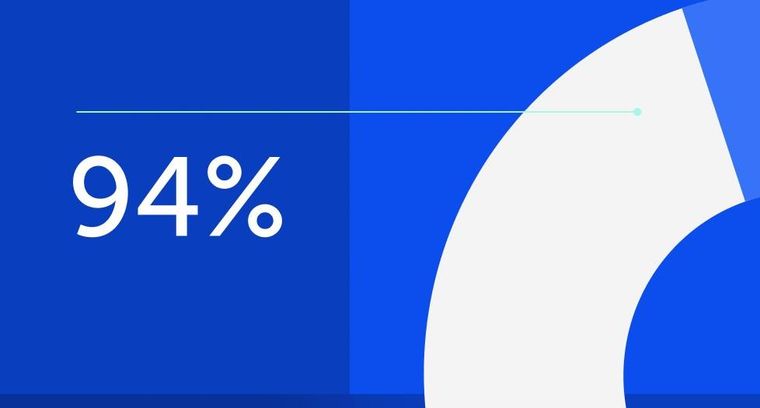
94% of researchers rate our articles as excellent or good
Learn more about the work of our research integrity team to safeguard the quality of each article we publish.
Find out more
ORIGINAL RESEARCH article
Front. Endocrinol., 08 September 2023
Sec. Reproduction
Volume 14 - 2023 | https://doi.org/10.3389/fendo.2023.1213402
This article is part of the Research TopicRecent Advances in Gestational Diabetes MellitusView all 12 articles
Objective: Sex steroid hormones may play a role in insulin resistance and glucose dysregulation. However, evidence regarding associations between early-pregnancy sex steroid hormones and hyperglycemia during pregnancy is limited. The primary objective of this study was to assess the relationships between first trimester sex steroid hormones and the subsequent development of hyperglycemia during pregnancy; with secondary evaluation of sex steroid hormones levels in mid-late pregnancy, concurrent with and subsequent to diagnosis of gestational diabetes.
Methods: Retrospective analysis of a prospective pregnancy cohort study was conducted. Medically low-risk participants with no known major endocrine disorders were recruited in the first trimester of pregnancy (n=319). Sex steroid hormones in each trimester, including total testosterone, free testosterone, estrone, estradiol, and estriol, were assessed using high-performance liquid chromatography and tandem mass spectrometry. Glucose levels of the 1-hour oral glucose tolerance test and gestational diabetes diagnosis were abstracted from medical records. Multivariable linear regression models were fitted to assess the associations of individual first trimester sex steroids and glucose levels.
Results: In adjusted models, first trimester total testosterone (β=5.24, 95% CI: 0.01, 10.46, p=0.05) and free testosterone (β=5.98, 95% CI: 0.97, 10.98, p=0.02) were positively associated with subsequent glucose concentrations and gestational diabetes diagnosis (total testosterone: OR=3.63, 95% CI: 1.50, 8.78; free testosterone: OR=3.69; 95% CI: 1.56, 8.73). First trimester estrone was also positively associated with gestational diabetes (OR=3.66, 95% CI: 1.56, 8.55). In mid-late pregnancy, pregnant people with gestational diabetes had lower total testosterone levels (β=-0.19, 95% CI: -0.36, -0.02) after adjustment for first trimester total testosterone.
Conclusion: Early-pregnancy sex steroid hormones, including total testosterone, free testosterone, and estrone, were positively associated with glucose levels and gestational diabetes in mid-late pregnancy. These hormones may serve as early predictors of gestational diabetes in combination with other risk factors.
Hyperglycemia, mainly caused by gestational diabetes mellitus (GDM), is a common metabolic complication during pregnancy (1, 2). GDM is associated with an increased risk of pregnancy related and neonatal outcomes, such as cesarean delivery, macrosomia, and neonatal hypoglycemia (1, 2). Furthermore, in the longer term, people diagnosed with GDM have a higher risk of progression to type 2 diabetes (T2DM), with around 19% of people with GDM develop T2DM after 5 years or more from delivery (3, 4). Children born to people with GDM have an increased risk of obesity, metabolic diseases and neurodevelopmental disorders (5, 6). Genetic predisposition, age, race/ethnicity, and obesity have been identified as risk factors for GDM (1, 7–9). Yet, the pathogenesis of GDM still is poorly understood.
GDM and T2DM are both characterized by insulin resistance (1, 7). Evidence suggests that endogenous sex steroid hormones (SSH), such as testosterone and estradiol, play important roles in glucose intolerance, insulin resistance and the development of T2DM in non-pregnant people (10–13). Additionally, people with hyperandrogenic conditions, such as polycystic ovary syndrome (PCOS) and congenital adrenal hyperplasia, have a higher risk of insulin resistance and T2DM (14–16). Lowering androgen production in PCOS patients leads improved insulin sensitivity and reduces fasting insulin levels (17, 18). Postmenopausal hormone therapy with estrogen/progestin reduces the incidence of diabetes (19, 20). Therefore, through their impacts on insulin and glucose metabolism, endogenous SSH may be involved in the pathogenesis of T2DM.
Likewise, SSH may play a role in the development of GDM. Nevertheless, pregnancy is a unique period given the rapid hormonal changes and the substantially increased estrogen concentrations (21), which may affect the relationship between SSH and glucose regulation. Evidence from people with PCOS substantiates the link between SSH and the risk of GDM during pregnancy (22, 23). However, to date, very few prospective studies have assessed the involvement of SSH, including testosterone and estriol (E3), in the development of GDM in people without PCOS (24–27). Yet, these previous studies have only examined total testosterone (TT) rather than free testosterone (fT) which represents the biologically active fraction of testosterone. Also, these studies did not concurrently examine multiple estrogens as well as testosterone despite their interrelatedness.
Additionally, the association between SSH and GDM may be bidirectional, operating through adipose tissue and insulin regulation (28, 29). Insulin induces androgen biosynthesis in cultured human ovarian theca and stromal cells (30), which suggests that GDM could in turn alter androgen production. Several small case-control studies have assessed differences in SSH in late pregnancy, subsequent to GDM diagnosis, with inconsistent findings (31–33). Moreover, the previous studies did not consider the potential confounding effect of early-pregnancy SSH on the relationship between GDM and SSH in late pregnancy.
Here, we leverage data and biospecimens from a pregnancy cohort that was medically not greater than normal risk at baseline with no known preexisting hormonal conditions to assess testosterone (fT and TT) and estrogens (estrone, estradiol, E3) in early pregnancy in relation to glucose concentrations and GDM diagnosis assessed in mid-late pregnancy. Secondarily, we evaluated associations between GDM diagnosis and the same set of SSH assessed later in pregnancy with and without adjusting for early-pregnancy SSH levels.
The current study is a retrospective analysis of a prospective pregnancy cohort, the Understanding Pregnancy Signals and Infant Development (UPSIDE) study that is a part of the Environmental Influences on Child Health Outcomes (ECHO) program (34). From 2015 to 2019, the UPSIDE study recruited pregnant people (n=326) in their first trimester receiving prenatal care through the University of Rochester Medical Center affiliated obstetric clinics (35). Briefly, the inclusion criteria for the UPSIDE study were (1) <14 weeks of gestation, (2) age 18 or older, (3) a singleton pregnancy, (4) able to communicate in English, (5) no known substance abuse problems or history of psychotic illness, and (6) no greater than normal medical risk. Additionally, women with diagnosed PCOS and T2DM were excluded from the cohort. The study was approved by the institutional review boards at the University of Rochester and Rutgers University. All participants provided written informed consent prior to participation. The current analysis included participants with SSH measured during pregnancy and a 1-hour oral glucose tolerance test (OGTT) or GDM diagnosis (n=319; Figure 1).
Blood samples were collected in each trimester (1st trimester: 12.2 ± 1.3 weeks; 2nd trimester: 21.2 ± 1.8 weeks; 3rd trimester: 31.4 ± 2 weeks) and after processing, serum was stored in a -80°C freezer until overnight shipment to the Endocrine and Metabolic Research Laboratory at Harbor-UCLA Medical Center. SSH, including TT, fT, estrone(E1), estradiol(E2), and E3, were quantified using validated liquid chromatography with tandem mass spectrometry (LC-MS/MS) methods (36). Briefly, LC–MS/MS was used to assess testosterone concentrations using a Shimadzu HPLC system (Columbia, MD) and an Applied Biosystems API5500 LC–MS/MS (Foster City, CA) equipped with a Turbo-Ion-Spray source that used positive mode. Quality control was performed on each assay run using spiked samples. The limit of quantification (LOQ) for TT was 2 ng/dL. Equilibrium dialysis using labeled testosterone was used to measure fT% which is used to calculate fT levels (fT=TT x fT%). fT% was not detected in one sample collected in the 1st trimester. The Shimadzu HPLC system (Columbia, MD) and a triple quadrupole mass spectrometer (API5000 LC–MS/MS, Foster City, CA) were used to measure estrogen concentrations. The LOQ was 2 pg/mL for E1 and E2, and 50 pg/mL for E3. E3 was not detected in 32 samples collected in the 1st trimester was used to replace missing E3 values (n=32) and E3 values less than . We additionally calculated the ratio of TT to E2 as a measure of hormone balance.
As part of routine obstetric care, participants were screened for GDM with 1-hour 50g OGTT at an average gestational age of 27.7 weeks ( ± 2.9 weeks). Participants with a 1-hour OGTT value of more than 135 mg/dL underwent a further diagnostic test with 3-hour 100g OGTT. Per clinical protocols, GDM was diagnosed according to the National Diabetes Data Group (NDDG) criteria: if the 3-hour OGTT values met more than two of the following values: fasting, 105 mg/dL; 1 hour, 190 mg/dL; 2 hours, 165 mg/dL; and 3 hours, 145 mg/dL. Several participants (n=5) were diagnosed with GDM without completing the 3-hour OGTT by either (1) 1-hour OGTT >200 mg/dL, (2) fasting glucose levels >125 mg/dL, or (3) by paneled blood glucose levels due to inability to complete 3-hour OGTT because of intolerance or history of gastric bypass surgery. OGTT values and GDM diagnosis were abstracted from electronic medical records by trained study staff.
For the purpose of this study, we additionally considered the Carpenter-Coustan (CC) criteria which may identify more GDM cases (37, 38). CC criteria use lower threshold values: if the 3-hour OGTT values met more than two of the following values: fasting, 95 mg/dL; 1 hour, 180 mg/dL; 2 hours, 155 mg/dL; and 3 hours, 140 mg/dL. Six additional participants were classified as having GDM based on the CC criteria.
Adipose tissue may be involved in the metabolism of SSH (39–41) and glucose dysregulation (28, 42, 43). We, therefore, included early-pregnancy body mass index (BMI) as a key confounder in the analyses. Early-pregnancy BMI, used as a proxy for pre-pregnancy BMI, was calculated based on weight and height abstracted from medical records from the first clinical visit prior to 14 weeks gestation and the formula (44).
SSH have been linked to adiposity (45) and early excess gestational weight gain (GWG) has been associated with GDM (46). Therefore, early GWG through the end of 2nd trimester was explored as a potential mediator between the associations of first trimester SSH and GDM. GWG through the end of 2nd trimester was calculated as weight at the end of the 2nd trimester minus early-pregnancy weight. Additionally, GWG through the end of 2nd trimester and total GWG until delivery were included as confounders in our secondary analyses of associations between GDM diagnosis and SSH assessed in mid-late pregnancy.
Age, race/ethnicity, parity, gestational age at the time of blood sample collection, fertility treatment, and infant sex, have been associated with SSH levels during pregnancy and in some cases, GDM as well, and were thus included as covariates (8, 47). Race/ethnicity was categorized as non-Hispanic White, non-Hispanic Black, Hispanic, and others. Parity was characterized as nulliparous and parous. Gestational dating was based on crown-rump length at the earliest available ultrasound and last menstrual period was used when an early ultrasound was not available (7%). Fertility treatment (any/none) was classified based on participant self-report. Although participants diagnosed with PCOS were excluded from the UPSIDE study, to address the possibility of undiagnosed cases, participants were evaluated with several questions to address relevant symptoms, including regularity of periods, hirsutism and acne (see Supplementary Materials) (48). Participants (n=13) categorized as potentially undiagnosed PCOS cases and were excluded in the sensitivity analyses. Additionally, four participants reported having a history of GDM in previous pregnancies and were excluded in the sensitivity analyses.
Descriptive statistics were calculated for all variables of interest. SSH were not normally distributed and were thus log-transformed. Early-pregnancy BMI was right skewed and was inverse-transformed. In the primary analyses, a multivariable linear regression model was fitted to assess the association of each first trimester SSH and glucose levels (continuous variable) based on routine 1-hour OGTT. A logistic regression model was fitted to assess the association of each first trimester SSH and GDM diagnosis. Age, race/ethnicity, parity, gestational age at the time of blood sample collection, fertility treatment, early-pregnancy BMI, and infant sex were included as covariates. Fertility treatment was not included in logistic regression models as no positive GDM cases were diagnosed in people reporting fertility treatment for the current pregnancy. GWG through the end of 2nd trimester was further assessed as a potential mediator of the associations between first trimester SSH and GDM diagnosis (Supplementary Figure 1) with bootstrap to estimate bias-corrected confidence intervals (CI). In secondary analyses, linear mixed effects models were fitted to assess the associations of GDM diagnosis and individual SSH in the 2nd and 3rd trimesters. Age, race/ethnicity, parity, gestational age at the time of blood sample collection, fertility treatment, infant sex, early-pregnancy BMI and GWG were included as covariates. First trimester SSH was additionally included as a key confounder. All analyses were conducted using STATA 17.0 (College Station, TX: StataCorp LLC).
The majority of participants (n=319) were non-Hispanic White (55.5%), had at least one prior birth (65.2%), had a college education or more (62.0%), and were overweight or obese in early pregnancy (57.6%). Twenty-two participants (6.9%) were classified as having GDM in this study. The characteristics of the participants grouped by GDM diagnosis are described in Table 1. Participants with GDM were slightly older that those without GDM (30.95 ± 0.71 vs 28.66 ± 0.27 years, p=0.005). SSH varied significantly across trimesters except for fT (Supplementary Table 1). Trend tests indicated that E1, E2, and E3 levels increased and TT/E2 ratios decreased across pregnancy (p<0.001). The correlations among first trimester SSH were weak to moderate (r=0.17-0.35) except for the high correlations between TT and fT (r=0.91) and between E1 and E2 (r=0.81). The correlation between TT and E3 was not significant (Supplementary Table 2).
In the primary multivariable regression models, first trimester TT and fT were positively associated with glucose levels measured in mid-late pregnancy after adjusting for maternal age, race/ethnicity, parity, gestational age of blood draw, early-pregnancy BMI, fertility treatment, and infant sex (Table 2). One natural-log unit increases in TT and fT were associated with 5.24 mg/dL (TT: 95% CI: 0.01, 10.46, p=0.05) and 5.98 mg/dL (fT: 95% CI: 0.97, 10.98, p=0.02) higher glucose levels, respectively. Associations between first trimester estrogens and glucose levels were also positive but slightly weaker. Higher first trimester TT and fT was also associated with increased odds of GDM diagnosis (TT: OR=3.63, 95% CI: 1.50, 8.78, p=0.004, Figure 2A; fT: OR=3.69, 95% CI: 1.56, 8.73, p=0.003, Figure 2B). Higher first trimester E1 (OR=3.66, 95% CI: 1.56, 8.55, p=0.003, Figure 2C) and E2 (OR=2.92, 95% CI: 1.00, 8.55, p=0.05), but not E3, were also associated with higher odds of GDM diagnosis. Exclusion of potentially undiagnosed PCOS cases in sensitivity analyses slightly strengthened associations between testosterone and E1 concentrations and glucose levels/GDM diagnosis (Supplementary Table 3). Exclusion of participants with a history of GDM during previous pregnancies had similar results on the associations of fT and E1 with glucose levels and GDM diagnosis (Supplementary Table 4). Associations of TT, fT, and E1 with clinical GDM diagnosis (solely by clinical criteria, not CC criteria) remained significant (Supplementary Table 5). Given the relatively weak correlations between testosterone and estrogens, we explored models including both fT and E1 simultaneously, fT and E1 were still associated with higher odds of GDM diagnosis (fT: OR=3.33, 95% CI: 1.35, 8.23, p=0.009; E1: OR=3.32, 95% CI: 1.38, 8.03 p=0.008); associations with glucose levels were positive but attenuated compared to models assessing the hormones individually (fT: β =5.12, 95% CI: -0.02, 10.26, p=0.05; E1: β =3.31, 95% CI: -1.35, 7.97, p=0.16).
Table 2 Associations of Log-transformed First Trimester Sex Steroid Hormones with Glucose Levels and Gestational Diabetes Diagnosis in Mid-late Pregnancy.
Figure 2 Distribution of first trimester log-transformed sex steroid hormones by gestational diabetes diagnosis. (A) distribution of total testosterone (TT) (B) distribution of free testosterone (FT) (C) distribution of estrone (E1). * indicates significant differences between participants with and without gestational diabetes (GDM) diagnosis.
Early-pregnancy BMI was a key confounding variable in the associations between sex steroids and glucose levels. Early-pregnancy BMI was positively associated with glucose levels (β=0.65, 95% CI: 0.21, 1.08, p=0.004) and first trimester fT and TT/E2 ratio, but was negatively associated with E1 and E2 (Supplementary Table 6). Regression models including early-pregnancy BMI as a covariate (Table 2) showed similar but slightly weakened significant positive associations between testosterone and glucose levels/GDM compared to regression models excluding early-pregnancy BMI (Supplementary Table 7). The relationships among early-pregnancy fT, early-pregnancy BMI and GDM are also illustrated in Supplementary Figure 2. The association between E1 and GDM was attenuated (OR=2.95, 95% CI: 1.31, 6.64, p=0.01) by excluding early-pregnancy BMI in the models (Supplementary Table 7).
GWG might mediate the effect of sex steroids on glucose levels. But GWG through the end of the 2nd trimester was not significantly associated with GDM diagnosis (OR=0.96, p=0.15) and only showed a borderline association with first trimester TT (β=1.61, 95% CI: -0.08, 3.30, p=0.06). The mediation effect of GWG on the relationship between TT and GDM was not significant (indirect effect: β=-0.05, 95% CIbootstrap: -0.22, 0.03).
GDM diagnosis was positively associated with E1 levels (β=0.29, 95% CI: 0.02, 0.56, p=0.03) in the 2nd and 3rd trimesters (Supplementary Table 8). Further adjusting for E1 levels in the 1st trimester, the association between GDM and E1 levels in the 2nd and 3rd trimesters was not significant (β=0.01, 95% CI: -0.18, 0.19, p=0.95). However, GDM diagnosis was inversely associated with TT in the 2nd and 3rd trimesters (β=-0.19, 95% CI: -0.36, -0.02, p=0.03), after adjustment for first trimester TT. But no associations between GDM and fT in the 2nd and 3rd trimesters were observed.
In this prospective pregnancy cohort including pregnant people who were medically not greater than normal risk at enrollment, first trimester TT, fT, and E1 were positively associated with glucose levels and GDM diagnosis in mid-late pregnancy, with similar trends observed for E2. fT and E1 were independently associated with increased odds of subsequent GDM diagnosis, when both were included in the same model. Results were robust to the exclusion of participants with potentially undiagnosed PCOS. GDM diagnosis was associated with lower TT but not fT levels in the 2nd and 3rd trimesters, when first trimester SSH was adjusted, respectively.
In females, androgens are mainly produced by the ovaries, adrenal glands, and adipose tissue (49). The placenta may also contribute to androgen synthesis during pregnancy (50). Prior studies that assessed associations between first trimester androgen levels and subsequent GDM diagnosis are limited. Two studies found a positive relationship between total testosterone levels in early pregnancy and GDM diagnosis in White pregnant people (25, 26), consistent with the results of this study. However, Gözükara, et al. (2015) and Mustaniemi, et al. (2023) measured TT levels using immunoassays and did not directly measure fT, the biologically active form of testosterone (25, 26). Improving upon the limitations of immunoassays, this study used LC-MS/MS, a gold standard method with greater sensitivity and specificity for steroid measurement (51). Similar to TT, first trimester fT showed positive and slightly stronger associations with glucose levels and GDM diagnosis.
Although evidence of the associations of first trimester TT and fT with GDM diagnosis is scarce, in prospective studies of non-pregnant people, TT and/or fT have been positively associated with development of T2DM in pre- and post-menopausal people (10, 12, 52, 53); but other studies have observed either no or attenuated associations after adjusting for adiposity (54–56). Generally, concentrations of TT and fT are higher in pregnant people compared to non-pregnant people (49), so to the extent that androgens play a causal role in glucose dysregulation, pregnancy may be a period of particular vulnerability.
We observed little evidence that adiposity was a confounder or mediator of the relationship between early-pregnancy testosterone and the development of GDM. Gözükara, et al. (2015) and Mustaniemi, et al. (2023) also identified that early pregnancy TT levels were higher among participants who subsequently developed GDM after adjusting for BMI (25, 26), which was consistent with our findings. However, evidence suggests that androgens exert direct and indirect effects on insulin sensitivity in adipose tissue and skeletal muscle (28, 42, 43). In female animal models, testosterone administration increased insulin resistance with or without western diet (57, 58). In subcutaneous adipocytes harvested from healthy non-pregnant people, testosterone treatment induced insulin resistance in vitro and inhibited insulin-stimulated glucose uptake (59). Administration of testosterone to oophorectomized female rodents impaired whole-body insulin-mediated glucose uptake potentially by lessening glycogen synthase expression and GLUT4 transporter expression in skeletal muscle (60–62). Also, anti-androgen treatments improved glucose tolerance in pregnant rat models (63). Given the findings in this study and the research in animal models and human adipocytes, it is postulated that androgens may contribute to the development of GDM by inducing insulin resistance not only in adipose tissue but also in other tissues, such as skeletal muscle.
In pregnant people, estrogens are mainly produced by the ovaries and placenta, with smaller contributions from other tissues, such as adipose tissue and adrenal glands (24). In this study, first trimester E1 levels were positively associated with subsequent GDM diagnosis. Although excessive testosterone could be converted into E1 in adipose tissue (43), in this study E1 was found to be a predictor of GDM independent of fT. We know of no other study that has addressed this association previously, but in a study of non-pregnant premenopausal people, estrone sulfate levels were positively correlated with postprandial glucose levels (56). In non-pregnant premenopausal people with PCOS, higher E1/E2 ratio was associated with increased fasting and postprandial glucose levels and insulin resistance (64). Therefore, E1 is potentially involved in glucose intolerance and GDM.
Research on the mechanisms linking estrogens to glucose regulation has primarily focused on E2 and evidence on E1 is sparse (65). In this study, while all estrogens showed positive associations with glucose levels and GDM, associations were strongest for E1. Borthwick et al. (2001) found that estrone sulfate could normalize hyperglycemia in obese-diabetic mice (both male and female) via the reduction of hepatic glucose-6-phosphatase (66). Although this finding conflicts with our results and findings in premenopausal women (56, 64), it is consistent with findings on E2, which may protect pancreatic β cell functions (67–69), reduce adipocyte hypertrophy and insulin resistance (68, 70), and improve hepatic glucose utilization (71). On the other hand, high concentrations of endogenous E2, particularly seen during pregnancy (21), may reduce insulin sensitivity (72) via decreased GLUT4 transporter expression in skeletal muscle (73) and interfere with insulin binding to insulin receptors (74). Therefore, the effect of endogenous estrogens on glucose regulation may vary in a non-linear manner and high concentrations of E1, similar to E2, potentially induce insulin resistance during pregnancy.
Because GDM may affect the production of SSH via insulin (30, 75), we further assessed the associations of GDM with SSH in mid-late pregnancy. When first-trimester SSH was not considered, the associations between GDM and SSH levels in mid-late pregnancy were consistent with the directions of associations between early-pregnancy SSH and GDM. These results were also similar to previous findings (31–33). When first-trimester estrogen was considered, the associations between GDM and estrogen were greatly attenuated, which indicates that the positive associations in mid-late pregnancy could be accounted by or driven by early-pregnancy estrogen levels. When first-trimester testosterone was considered, the directions of associations between GDM and testosterone were reversed, although the association between GDM and fT was not significant. These findings indicate that other factors changing during mid-late pregnancy, such as insulin levels which may be affected by GDM treatment, sex hormone binding globulin (SHBG) levels which is bound to fT to form TT, placental aromatase, and increasing gestational weight, may affect mid-late pregnancy testosterone levels and thus the relationship between GDM and mid-late pregnancy testosterone levels (30, 75–77).
A strength of this study is the measurement of SSH using the gold standard LC-MS/MS method, which is an advance over prior studies in this field. Furthermore, the prospective design of the study cohort established the temporal relationships between SSH in the 1st trimester and glucose levels and GDM in mid-late pregnancy. In addition, repeat measures of SSH throughout pregnancy enabled us to assess hormone levels both prior to and after GDM diagnosis, while taking early-pregnancy SSH levels into consideration. Several limitations should be considered when interpreting the results of the current analyses. We did not assess insulin resistance or visceral adiposity in our cohort, which are potential key mechanisms linking SSH to GDM (1, 7, 24, 42). Further investigations of the relationship among SSH, adiposity, and insulin resistance during pregnancy are warranted. Also, future studies could assess the effect of insulin and SHGB levels during mid-late pregnancy on the relationship between GDM and mid-late pregnancy testosterone levels. Another limitation is that the limited GDM cases in this study could not provide reliable estimations of the cutoff values of first trimester TT, fT or E1 to predict GDM. Additionally, we did not assess SHBG, which was negatively associated with GDM in a recent meta-analysis (78). SHBG binds both testosterone and E2 during pregnancy (42, 79) and thus, low SHBG levels indicate high serum concentrations of fT and free E2. Therefore, the previous findings of the negative association between SHBG and GDM are consistent with the positive associations between fT and GDM found in this study (78). We assessed the potential undiagnosed PCOS cases by self report using a two-question response to oligomenorrhoea and hirsutism. This self-report approach has been found in longitudinal studies to be associated with clinical biomarkers and measures (48, 80), although additional assessments could confirm the diagnosis.
In this prospective study of pregnant people, higher levels of first-trimester TT, fT and estrone were positively associated with glucose levels and GDM diagnosis in mid-late pregnancy. Our findings suggest that the early-pregnancy hormonal milieu may contribute to and/or predict gestational hyperglycemia. Studies such as the current study that identify early-pregnancy biomarkers may inform future targeted screening and interventions (lifestyle modifications, etc.) aimed at preventing GDM in pregnant people who are at risk.
The datasets presented in this study can be found in online repositories. The names of the repository/repositories and accession number(s) can be found below: https://dash.nichd.nih.gov/study/417122.
The studies involving humans were approved by The University of Rochester Research Subjects Review Board. The studies were conducted in accordance with the local legislation and institutional requirements. Written informed consent for participation in this study was provided by the participants’ legal guardians/next of kin.
All authors contribute to the generation of hypotheses, statistical analyses, manuscript preparation and final approval of the manuscript. All authors contributed to the article and approved the submitted version.
Funding for the UPSIDE-ECHO study is provided by Eunice Kennedy Shriver National Institute of Child Health and Human Development (R01HD083369), NIH Office of the Director grants (UG3OD023349; UH3OD023349), National Institute of Nursing Research (R01NR01760203, K23NR019014) and the Mae Stone Goode Foundation (no grant number). Additional support is provided by the National Institute of Environmental Health Sciences (P30ES005022), and the Wynne Center for Family Research (no grant number).
We appreciated the assistance from Jessica Brunner, BS, who was involved in medical record abstraction of the UPSIDE study, Sally W. Thurston, PhD, who performed estimation of GWG by each trimester, and Lauren Aleksunes, PharmD, PhD, who was involved in the discussion of the mechanisms linking SSH to GDM.
The authors declare that the research was conducted in the absence of any commercial or financial relationships that could be construed as a potential conflict of interest.
All claims expressed in this article are solely those of the authors and do not necessarily represent those of their affiliated organizations, or those of the publisher, the editors and the reviewers. Any product that may be evaluated in this article, or claim that may be made by its manufacturer, is not guaranteed or endorsed by the publisher.
The Supplementary Material for this article can be found online at: https://www.frontiersin.org/articles/10.3389/fendo.2023.1213402/full#supplementary-material
1. Baz B, Riveline J-P, Gautier J-F. ENDOCRINOLOGY OF PREGNANCY: Gestational diabetes mellitus: definition, aetiological and clinical aspects. Eur J Endocrinol (2016) 174(2):R43–51. doi: 10.1530/eje-15-0378
2. Hod M, Kapur A, Sacks DA, Hadar E, Agarwal M, Di Renzo GC, et al. The International Federation of Gynecology and Obstetrics (FIGO) Initiative on gestational diabetes mellitus: A pragmatic guide for diagnosis, management, and care. Int J Gynecology Obstetrics (2015) 131:S173–211. doi: 10.1016/S0020-7292(15)30033-3
3. Juan J, Sun Y, Wei Y, Wang S, Song G, Yan J, et al. Progression to type 2 diabetes mellitus after gestational diabetes mellitus diagnosed by IADPSG criteria: Systematic review and meta-analysis. Front Endocrinol (Lausanne). (2022) 13:1012244. doi: 10.3389/fendo.2022.1012244
4. Bellamy L, Casas JP, Hingorani AD, Williams D. Type 2 diabetes mellitus after gestational diabetes: a systematic review and meta-analysis. Lancet (2009) 373(9677):1773–9. doi: 10.1016/s0140-6736(09)60731-5
5. Bianco ME, Josefson JL. Hyperglycemia during pregnancy and long-term offspring outcomes. Curr Diabetes Rep (2019) 19(12):143. doi: 10.1007/s11892-019-1267-6
6. Rowland J, Wilson CA. The association between gestational diabetes and ASD and ADHD: a systematic review and meta-analysis. Sci Rep (2021) 11(1):5136. doi: 10.1038/s41598-021-84573-3
7. Dalfrà MG, Burlina S, Del Vescovo GG, Lapolla A. Genetics and epigenetics: new insight on gestational diabetes mellitus. Front Endocrinol (Lausanne). (2020) 11:602477. doi: 10.3389/fendo.2020.602477
8. Farrar D, Simmonds M, Bryant M, Lawlor DA, Dunne F, Tuffnell D, et al. Risk factor screening to identify women requiring oral glucose tolerance testing to diagnose gestational diabetes: a systematic review and meta-analysis and analysis of two pregnancy cohorts. PloS One (2017) 12(4):e0175288. doi: 10.1371/journal.pone.0175288
9. Bower JK, Butler BN, Bose-Brill S, Kue J, Wassel CL. Racial/ethnic differences in diabetes screening and hyperglycemia among US women after gestational diabetes. Prev Chronic Dis (2019) 16:E145. doi: 10.5888/pcd16.190144
10. Rasmussen JJ, Selmer C, Frøssing S, Schou M, Faber J, Torp-Pedersen C, et al. Endogenous testosterone levels are associated with risk of type 2 diabetes in women without established comorbidity. J Endocr Soc (2020) 4(6):bvaa050. doi: 10.1210/jendso/bvaa050
11. Ding EL, Song Y, Malik VS, Liu S. Sex differences of endogenous sex hormones and risk of type 2 diabetes: a systematic review and meta-analysis. Jama (2006) 295(11):1288–99. doi: 10.1001/jama.295.11.1288
12. Ding EL, Song Y, Manson JE, Rifai N, Buring JE, Liu S. Plasma sex steroid hormones and risk of developing type 2 diabetes in women: a prospective study. Diabetologia (2007) 50(10):2076–84. doi: 10.1007/s00125-007-0785-y
13. Golden SH, Dobs AS, Vaidya D, Szklo M, Gapstur S, Kopp P, et al. Endogenous sex hormones and glucose tolerance status in postmenopausal women. J Clin Endocrinol Metab (2007) 92(4):1289–95. doi: 10.1210/jc.2006-1895
14. Moran LJ, Misso ML, Wild RA, Norman RJ. Impaired glucose tolerance, type 2 diabetes and metabolic syndrome in polycystic ovary syndrome: a systematic review and meta-analysis. Hum Reprod Update (2010) 16(4):347–63. doi: 10.1093/humupd/dmq001
15. Macut D, Zdravković V, Bjekić-Macut J, Mastorakos G, Pignatelli D. Metabolic perspectives for non-classical congenital adrenal hyperplasia with relation to the classical form of the disease. Front Endocrinol (Lausanne). (2019) 10:681. doi: 10.3389/fendo.2019.00681
16. O’Reilly MW, Taylor AE, Crabtree NJ, Hughes BA, Capper F, Crowley RK, et al. Hyperandrogenemia predicts metabolic phenotype in polycystic ovary syndrome: the utility of serum androstenedione. J Clin Endocrinol Metab (2014) 99(3):1027–36. doi: 10.1210/jc.2013-3399
17. Banaszewska B, Wrotyńska-Barczyńska J, Spaczynski RZ, Pawelczyk L, Duleba AJ. Effects of resveratrol on polycystic ovary syndrome: A double-blind, randomized, placebo-controlled trial. J Clin Endocrinol Metab (2016) 101(11):4322–8. doi: 10.1210/jc.2016-1858
18. Gambineri A, Patton L, Vaccina A, Cacciari M, Morselli-Labate AM, Cavazza C, et al. Treatment with flutamide, metformin, and their combination added to a hypocaloric diet in overweight-obese women with polycystic ovary syndrome: a randomized, 12-month, placebo-controlled study. J Clin Endocrinol Metab (2006) 91(10):3970–80. doi: 10.1210/jc.2005-2250
19. Margolis KL, Bonds DE, Rodabough RJ, Tinker L, Phillips LS, Allen C, et al. Effect of oestrogen plus progestin on the incidence of diabetes in postmenopausal women: results from the Women’s Health Initiative Hormone Trial. Diabetologia (2004) 47(7):1175–87. doi: 10.1007/s00125-004-1448-x
20. Kanaya AM, Herrington D, Vittinghoff E, Lin F, Grady D, Bittner V, et al. Glycemic effects of postmenopausal hormone therapy: the heart and estrogen/progestin replacement study: A randomized, double-blind, placebo-controlled trial. Ann Internal Med (2003) 138(1):1–9. doi: 10.7326/0003-4819-138-1-200301070-00005%m12513038
21. Schock H, Zeleniuch-Jacquotte A, Lundin E, Grankvist K, Lakso H, Idahl A, et al. Hormone concentrations throughout uncomplicated pregnancies: a longitudinal study. BMC Pregnancy Childbirth (2016) 16(1):146. doi: 10.1186/s12884-016-0937-5
22. Toulis KA, Goulis DG, Kolibianakis EM, Venetis CA, Tarlatzis BC, Papadimas I. Risk of gestational diabetes mellitus in women with polycystic ovary syndrome: a systematic review and a meta-analysis. Fertil Steril (2009) 92(2):667–77. doi: 10.1016/j.fertnstert.2008.06.045
23. Joham AE, Ranasinha S, Zoungas S, Moran L, Teede HJ. Gestational diabetes and type 2 diabetes in reproductive-aged women with polycystic ovary syndrome. J Clin Endocrinol Metab (2014) 99(3):E447–52. doi: 10.1210/jc.2013-2007
24. Vejrazkova D, Vcelak J, Vankova M, Lukasova P, Bradnova O, Halkova T, et al. Steroids and insulin resistance in pregnancy. J Steroid Biochem Mol Biol (2014) 139:122–9. doi: 10.1016/j.jsbmb.2012.11.007
25. Gözükara YM, Aytan H, Ertunc D, Tok EC, Demirtürk F, Şahin Ş, et al. Role of first trimester total testosterone in prediction of subsequent gestational diabetes mellitus. J Obstet Gynaecol Res (2015) 41(2):193–8. doi: 10.1111/jog.12525
26. Mustaniemi S, Morin-Papunen L, Keikkala E, Öhman H, Surcel HM, Kaaja R, et al. Associations of low sex hormone-binding globulin and androgen excess in early pregnancy with fasting and post-prandial hyperglycaemia, gestational diabetes, and its severity. Diabetes Metab Res Rev (2023) 39(2):e3599. doi: 10.1002/dmrr.3599
27. Hur J, Cho EH, Baek KH, Lee KJ. Prediction of gestational diabetes mellitus by unconjugated estriol levels in maternal serum. Int J Med Sci (2017) 14(2):123–7. doi: 10.7150/ijms.17321
28. Schiffer L, Kempegowda P, Arlt W, O’Reilly MW. MECHANISMS IN ENDOCRINOLOGY: The sexually dimorphic role of androgens in human metabolic disease. Eur J Endocrinol (2017) 177(3):R125–r143. doi: 10.1530/eje-17-0124
29. Brüning JC, Gautam D, Burks DJ, Gillette J, Schubert M, Orban PC, et al. Role of brain insulin receptor in control of body weight and reproduction. Science (2000) 289(5487):2122–5. doi: 10.1126/science.289.5487.2122
30. Barbieri RL, Makris A, Ryan KJ. Insulin stimulates androgen accumulation in inCubations of human ovarian stroma and theca. Obstet Gynecol (1984) 64(3 Suppl):73s–80s. doi: 10.1097/00006250-198409001-00019
31. Yuan T, Li Y. Steroid profiling and genetic variants in Chinese women with gestational diabetes mellitus. J Steroid Biochem Mol Biol (2021) 214:105999. doi: 10.1016/j.jsbmb.2021.105999
32. Villarroel C, Salinas A, López P, Kohen P, Rencoret G, Devoto L, et al. Pregestational type 2 diabetes and gestational diabetes exhibit different sexual steroid profiles during pregnancy. Gynecol Endocrinol (2017) 33(3):212–7. doi: 10.1080/09513590.2016.1248933
33. Morisset AS, Dubé MC, Drolet R, Pelletier M, Labrie F, Luu-The V, et al. Androgens in the maternal and fetal circulation: association with insulin resistance. J Matern Fetal Neonatal Med (2013) 26(5):513–9. doi: 10.3109/14767058.2012.735725
34. Knapp EA, Kress AM, Parker CB, Page GP, McArthur K, Gachigi KK, et al. The environmental influences on child health outcomes (ECHO)-wide cohort. Am J Epidemiol (2023) 192(8):1249–63. doi: 10.1093/aje/kwad071
35. O’Connor T, Best M, Brunner J, Ciesla AA, Cunning A, Kapula N, et al. Cohort profile: Understanding Pregnancy Signals and Infant Development (UPSIDE): a pregnancy cohort study on prenatal exposure mechanisms for child health. BMJ Open (2021) 11(4):e044798. doi: 10.1136/bmjopen-2020-044798
36. Sathyanarayana S, Butts S, Wang C, Barrett E, Nguyen R, Schwartz SM, et al. Early prenatal phthalate exposure, sex steroid hormones, and birth outcomes. J Clin Endocrinol Metab (2017) 102(6):1870–8. doi: 10.1210/jc.2016-3837
37. Berggren EK, Boggess KA, Stuebe AM, Jonsson Funk M. National Diabetes Data Group vs Carpenter-Coustan criteria to diagnose gestational diabetes. Am J Obstet Gynecol (2011) 205(3):253.e1–7. doi: 10.1016/j.ajog.2011.06.026
38. Harper LM, Mele L, Landon MB, Carpenter MW, Ramin SM, Reddy UM, et al. Carpenter-coustan compared with national diabetes data group criteria for diagnosing gestational diabetes. Obstet Gynecol. May (2016) 127(5):893–8. doi: 10.1097/aog.0000000000001383
39. Quinkler M, Sinha B, Tomlinson JW, Bujalska IJ, Stewart PM, Arlt W. Androgen generation in adipose tissue in women with simple obesity–a site-specific role for 17beta-hydroxysteroid dehydrogenase type 5. J Endocrinol Nov (2004) 183(2):331–42. doi: 10.1677/joe.1.05762
40. Hetemäki N, Mikkola TS, Tikkanen MJ, Wang F, Hämäläinen E, Turpeinen U, et al. Adipose tissue estrogen production and metabolism in premenopausal women. J Steroid Biochem Mol Biol (2021) 209:105849. doi: 10.1016/j.jsbmb.2021.105849
41. Maliqueo M, Cruz G, Espina C, Contreras I, García M, Echiburú B, et al. Obesity during pregnancy affects sex steroid concentrations depending on fetal gender. Int J Obes (Lond) (2017) 41(11):1636–45. doi: 10.1038/ijo.2017.159
42. Gambineri A, Pelusi C. Sex hormones, obesity and type 2 diabetes: is there a link? Endocr Connect (2019) 8(1):R1–r9. doi: 10.1530/ec-18-0450
43. Li Y, Chen C, Ma Y, Xiao J, Luo G, Li Y, et al. Multi-system reproductive metabolic disorder: significance for the pathogenesis and therapy of polycystic ovary syndrome (PCOS). Life Sci (2019) 228:167–75. doi: 10.1016/j.lfs.2019.04.046
44. Groth SW, Fernandez ID, Block RC, Thurston SW, Wong E, Brunner J, et al. Biological changes in the pregnancy-postpartum period and subsequent cardiometabolic risk-UPSIDE MOMS: A research protocol. Res Nurs Health (2021) 44(4):608–19. doi: 10.1002/nur.22141
45. Pasquali R, Oriolo C. Obesity and androgens in women. Front Horm Res (2019) 53:120–34. doi: 10.1159/000494908
46. Robitaille J. Excessive gestational weight gain and gestational diabetes: importance of the first weeks of pregnancy. Diabetologia (2015) 58(10):2203–5. doi: 10.1007/s00125-015-3725-2
47. Barrett ES, Mbowe O, Thurston SW, Butts S, Wang C, Nguyen R, et al. Predictors of steroid hormone concentrations in early pregnancy: results from a multi-center cohort. Matern Child Health J (2019) 23(3):397–407. doi: 10.1007/s10995-018-02705-0
48. Taponen S, Martikainen H, Järvelin MR, Laitinen J, Pouta A, Hartikainen AL, et al. Hormonal profile of women with self-reported symptoms of oligomenorrhea and/or hirsutism: Northern Finland birth cohort 1966 study. J Clin Endocrinol Metab (2003) 88(1):141–7. doi: 10.1210/jc.2002-020982
49. Makieva S, Saunders PT, Norman JE. Androgens in pregnancy: roles in parturition. Hum Reprod Update (2014) 20(4):542–59. doi: 10.1093/humupd/dmu008
50. Escobar JC, Patel SS, Beshay VE, Suzuki T, Carr BR. The human placenta expresses CYP17 and generates androgens de novo. J Clin Endocrinol Metab (2011) 96(5):1385–92. doi: 10.1210/jc.2010-2504
51. French D. Advances in bioanalytical techniques to measure steroid hormones in serum. Bioanalysis (2016) 8(11):1203–19. doi: 10.4155/bio-2015-0025
52. Oh JY, Barrett-Connor E, Wedick NM, Wingard DL. Endogenous sex hormones and the development of type 2 diabetes in older men and women: the Rancho Bernardo study. Diabetes Care (2002) 25(1):55–60. doi: 10.2337/diacare.25.1.55
53. Kalyani RR, Franco M, Dobs AS, Ouyang P, Vaidya D, Bertoni A, et al. The association of endogenous sex hormones, adiposity, and insulin resistance with incident diabetes in postmenopausal women. J Clin Endocrinol Metab (2009) 94(11):4127–35. doi: 10.1210/jc.2009-0910
54. Muka T, Nano J, Jaspers L, Meun C, Bramer WM, Hofman A, et al. Associations of steroid sex hormones and sex hormone-binding globulin with the risk of type 2 diabetes in women: A population-based cohort study and meta-analysis. Diabetes (2017) 66(3):577–86. doi: 10.2337/db16-0473
55. Fenske B, Kische H, Gross S, Wallaschofski H, Völzke H, Dörr M, et al. Endogenous androgens and sex hormone-binding globulin in women and risk of metabolic syndrome and type 2 diabetes. J Clin Endocrinol Metab (2015) 100(12):4595–603. doi: 10.1210/jc.2015-2546
56. Mather KJ, Kim C, Christophi CA, Aroda VR, Knowler WC, Edelstein SE, et al. Steroid sex hormones, sex hormone-binding globulin, and diabetes incidence in the diabetes prevention program. J Clin Endocrinol Metab (2015) 100(10):3778–86. doi: 10.1210/jc.2015-2328
57. Beloosesky R, Gold R, Almog B, Sasson R, Dantes A, Land-Bracha A, et al. Induction of polycystic ovary by testosterone in immature female rats: Modulation of apoptosis and attenuation of glucose/insulin ratio. Int J Mol Med (2004) 14(2):207–15. doi: 10.3892/ijmm.14.2.207
58. Bishop CV, Takahashi D, Mishler E, Slayden OD, Roberts CT, Hennebold J, et al. Individual and combined effects of 5-year exposure to hyperandrogenemia and Western-style diet on metabolism and reproduction in female rhesus macaques. Hum Reprod (2021) 36(2):444–54. doi: 10.1093/humrep/deaa321
59. Corbould A. Chronic testosterone treatment induces selective insulin resistance in subcutaneous adipocytes of women. J Endocrinol (2007) 192(3):585–94. doi: 10.1677/joe.1.07070
60. Rincon J, Holmäng A, Wahlström EO, Lönnroth P, Björntorp P, Zierath JR, et al. Mechanisms behind insulin resistance in rat skeletal muscle after oophorectomy and additional testosterone treatment. Diabetes (1996) 45(5):615–21. doi: 10.2337/diab.45.5.615
61. Holmäng A, Larsson BM, Brzezinska Z, Björntorp P. Effects of short-term testosterone exposure on insulin sensitivity of muscles in female rats. Am J Physiol (1992) 262(6 Pt 1):E851–5. doi: 10.1152/ajpendo.1992.262.6.E851
62. Inada A, Fujii NL, Inada O, Higaki Y, Furuichi Y, Nabeshima YI. Effects of 17β-estradiol and androgen on glucose metabolism in skeletal muscle. Endocrinology (2016) 157(12):4691–705. doi: 10.1210/en.2016-1261
63. Zhang Y, Hu M, Yang F, Zhang Y, Ma S, Zhang D, et al. Increased uterine androgen receptor protein abundance results in implantation and mitochondrial defects in pregnant rats with hyperandrogenism and insulin resistance. J Mol Med (Berl) (2021) 99(10):1427–46. doi: 10.1007/s00109-021-02104-z
64. Kim N, Chun S. Association between the serum estrone-to-estradiol ratio and parameters related to glucose metabolism and insulin resistance in women with polycystic ovary syndrome. Clin Exp Reprod Med (2021) 48(4):374–9. doi: 10.5653/cerm.2021.04553
65. Alemany M. Estrogens and the regulation of glucose metabolism. World J Diabetes (2021) 12(10):1622–54. doi: 10.4239/wjd.v12.i10.1622
66. Borthwick EB, Houston MP, Coughtrie MW, Burchell A. The antihyperglycemic effect of estrone sulfate in genetically obese-diabetic (ob/ob) mice is associated with reduced hepatic glucose-6-phosphatase. Horm Metab Res (2001) 33(12):721–6. doi: 10.1055/s-2001-19136
67. Liu S, Mauvais-Jarvis F. Rapid, nongenomic estrogen actions protect pancreatic islet survival. Islets (2009) 1(3):273–5. doi: 10.4161/isl.1.3.9781
68. Mauvais-Jarvis F, Clegg DJ, Hevener AL. The role of estrogens in control of energy balance and glucose homeostasis. Endocr Rev (2013) 34(3):309–38. doi: 10.1210/er.2012-1055
69. Nadal A, Alonso-Magdalena P, Soriano S, Ropero AB, Quesada I. The role of oestrogens in the adaptation of islets to insulin resistance. J Physiol (2009) 587(Pt 21):5031–7. doi: 10.1113/jphysiol.2009.177188
70. Stubbins RE, Najjar K, Holcomb VB, Hong J, Núñez NP. Oestrogen alters adipocyte biology and protects female mice from adipocyte inflammation and insulin resistance. Diabetes Obes Metab Jan (2012) 14(1):58–66. doi: 10.1111/j.1463-1326.2011.01488.x
71. Liebmann M, Asuaje Pfeifer M, Grupe K, Scherneck S. Estradiol (E2) improves glucose-stimulated insulin secretion and stabilizes GDM progression in a prediabetic mouse model. Int J Mol Sci (2022) 23(12):6693. doi: 10.3390/ijms23126693
72. González C, Alonso A, Alvarez N, Díaz F, Martínez M, Fernández S, et al. Role of 17beta-estradiol and/or progesterone on insulin sensitivity in the rat: implications during pregnancy. J Endocrinol (2000) 166(2):283–91. doi: 10.1677/joe.0.1660283
73. Barros RP, Morani A, Moriscot A, MaChado UF. Insulin resistance of pregnancy involves estrogen-induced repression of muscle GLUT4. Mol Cell Endocrinol (2008) 295(1-2):24–31. doi: 10.1016/j.mce.2008.07.008
74. Root-Bernstein R, Podufaly A, Dillon PF. Estradiol binds to insulin and insulin receptor decreasing insulin binding in vitro. Original research. Front Endocrinol (2014) 5:118. doi: 10.3389/fendo.2014.00118
75. Uzelac PS, Li X, Lin J, Neese LD, Lin L, Nakajima ST, et al. Dysregulation of leptin and testosterone production and their receptor expression in the human placenta with gestational diabetes mellitus. Placenta (2010) 31(7):581–8. doi: 10.1016/j.placenta.2010.04.002
76. Kallak TK, Hellgren C, Skalkidou A, Sandelin-Francke L, Ubhayasekhera K, Bergquist J, et al. Maternal and female fetal testosterone levels are associated with maternal age and gestational weight gain. Eur J Endocrinol (2017) 177(4):379–88. doi: 10.1530/eje-17-0207
77. O’Leary P, Boyne P, Flett P, Beilby J, James I. Longitudinal assessment of changes in reproductive hormones during normal pregnancy. Clin Chem (1991) 37(5):667–72. doi: 10.1093/clinchem/37.5.667
78. Li MY, Rawal S, Hinkle SN, Zhu YY, Tekola-Ayele F, Tsai MY, et al. Sex hormone-binding globulin, cardiometabolic biomarkers, and gestational diabetes: A longitudinal study and meta-analysis. Matern Fetal Med (2020) 2(1):2–9. doi: 10.1097/fm9.0000000000000037
79. Vermeulen A, Verdonck L, Kaufman JM. A critical evaluation of simple methods for the estimation of free testosterone in serum. J Clin Endocrinol Metab (1999) 84(10):3666–72. doi: 10.1210/jcem.84.10.6079
Keywords: sex steroid hormone, hyperglycemia, gestational diabetes, testosterone, estrogen
Citation: Meng Y, Thornburg LL, Hoeger KM, Núñez ZR, Kautz A, Evans AT, Wang C, Miller RK, Groth SW, O’Connor TG and Barrett ES (2023) Association between sex steroid hormones and subsequent hyperglycemia during pregnancy. Front. Endocrinol. 14:1213402. doi: 10.3389/fendo.2023.1213402
Received: 27 April 2023; Accepted: 08 August 2023;
Published: 08 September 2023.
Edited by:
Yanhui Lu, Food and Drug Administration, United StatesReviewed by:
Nicolas Crisosto, University of Chile, ChileCopyright © 2023 Meng, Thornburg, Hoeger, Núñez, Kautz, Evans, Wang, Miller, Groth, O’Connor and Barrett. This is an open-access article distributed under the terms of the Creative Commons Attribution License (CC BY). The use, distribution or reproduction in other forums is permitted, provided the original author(s) and the copyright owner(s) are credited and that the original publication in this journal is cited, in accordance with accepted academic practice. No use, distribution or reproduction is permitted which does not comply with these terms.
*Correspondence: Ying Meng, WWluZ19NZW5nQHVybWMucm9jaGVzdGVyLmVkdQ==
Disclaimer: All claims expressed in this article are solely those of the authors and do not necessarily represent those of their affiliated organizations, or those of the publisher, the editors and the reviewers. Any product that may be evaluated in this article or claim that may be made by its manufacturer is not guaranteed or endorsed by the publisher.
Research integrity at Frontiers
Learn more about the work of our research integrity team to safeguard the quality of each article we publish.