- 1Division of Pediatrics, Department of Health Sciences, University of Piemonte Orientale, Novara, Italy
- 2Endocrinology, Department of Translational Medicine, University of Piemonte Orientale, Novara, Italy
- 3Obesity and Lipodystrophy Center, Endocrinology Unit, University Hospital of Pisa, Pisa, Italy
- 4Chemistry and Endocrinology Laboratory, Department of Laboratory Medicine, University Hospital of Pisa, Pisa, Italy
- 5Interdisciplinary Research Center of Autoimmune and Allergic Diseases, University of Piemonte Orientale, Novara, Italy
- 6Laboratory of Genetics, Struttura Complessa a Direzione Universitaria (SCDU) Biochimica Clinica, Ospedale Maggiore della Carità, Novara, Italy
- 7Physical Medicine and Rehabilitation, Department of Health Sciences, University of Piemonte Orientale, Novara, Italy
- 8Division of Endocrinology and Metabolism, Faculty of Medicine, Dokuz Eylul University, Izmir, Türkiye
- 9Department of Health Sciences, University of Piemonte Orientale, Novara, Italy
Lipodystrophy syndromes are characterized by a progressive metabolic impairment secondary to adipose tissue dysfunction and may have a genetic background. Congenital generalized lipodystrophy type 4 (CGL4) is an extremely rare subtype, caused by mutations in the polymerase I and transcript release factor (PTRF) gene. It encodes for a cytoplasmatic protein called caveolae-associated protein 1 (Cavin-1), which, together with caveolin 1, is responsible for the biogenesis of caveolae, being a master regulator of adipose tissue expandability. Cavin-1 is expressed in several tissues, including muscles, thus resulting, when dysfunctional, in a clinical phenotype characterized by the absence of adipose tissue and muscular dystrophy. We herein describe the clinical phenotypes of two siblings in their early childhood, with a phenotype characterized by a generalized reduction of subcutaneous fat, muscular hypertrophy, distinct facial features, myopathy, and atlantoaxial instability. One of the siblings developed paroxysmal supraventricular tachycardia leading to cardiac arrest at 3 months of age. Height and BMI were normal. Blood tests showed elevated CK, a mild increase in liver enzymes and triglycerides levels, and undetectable leptin and adiponectin concentrations. Fasting glucose and HbA1c were normal, while Homeostatic Model Assessment for Insulin Resistance (HOMA-IR) was mildly elevated. Both patients were hyperphagic and had cravings for foods rich in fats and sugars. Genetic testing revealed a novel pathogenic mutation of the CAVIN1/PTRF gene (NM_012232 exon1:c T21A:p.Y7X) at the homozygous state. The diagnosis of lipodystrophy can be challenging, often requiring a multidisciplinary approach, given the pleiotropic effect, involving several tissues. The coexistence of generalized lack of fat, myopathy with elevated CK levels, arrhythmias, gastrointestinal dysmotility, and skeletal abnormalities should prompt the suspicion for the diagnosis of CGL4, although phenotypic variability may occur.
Introduction
Lipodystrophies are a heterogeneous group of rare disorders characterized by lack or dysfunction of white adipose tissue (WAT) with perturbation in its mass or distribution (1, 2) and consequent alterations in adipokines levels, mainly leptin and adiponectin (3–5). Lipodystrophies can be classified according to their etiology in genetic or acquired syndromes or based on the extension of the lack of subcutaneous adipose tissue as generalized, partial, or localized (6–10). Manifestations of congenital generalized lipodystrophies (CGLs) appear early, typically at birth or immediately after, and are associated with the development of metabolic complications (11, 12). CGL has four main subtypes, all with an autosomal recessive transmission pattern (13, 14). Their estimated prevalence is approximately 0.23 cases/million and 0.96 cases/million, worldwide and in Europe, respectively (15, 16). Several patients with CGL have been diagnosed among consanguineous individuals, particularly in families from Brazil, Lebanon, Scandinavia, and of African ancestry, probably due to the high rate of founder mutations and endogamy in these ethnic groups (17). To date, about 30 patients with congenital generalized lipodystrophy type 4 (CGL4) have been described, most of them in families of Omani, Japanese, Hispanic, Moroccan, and Turkish origin. In Europe, this phenotype is extremely rare, and only two cases have been reported so far in United Kingdom and Russia (18, 19), with patients reporting consanguinity between their parents or being immigrant families from certain regions where consanguineous relationships are common.
CGL4, first described in 2009 (20), is due to mutations in the polymerase I and transcript release factor (PTRF) gene located on chromosome 17q21.2 (21). PTRF gene encodes a cytoplasmatic protein called caveolae-associated protein 1 (Cavin-1), which, together with caveolin 1, is responsible for the biogenesis of caveolae and co-localizes in the adipocytes (22, 23), being a master regulator of cell differentiation and adipose tissue expandability (24). Beside adipocytes, Cavin-1 is expressed in several tissues, including muscles (7). Common findings in CGL4 subjects are the following: a progressive loss of adipose tissue during infancy, emaciated face due to the absence of Bichat fat pads, acromegalic features (mainly face, hands, and feet), prominent superficial subcutaneous veins (pseudo-flebomegaly), accelerated growth, umbilical hernia, hirsutism, clitoromegaly and amenorrhea, osteopenia and distal metaphyseal deformation with joint stiffness, pyloric stenosis, atlantoaxial instability, and percussion-induced muscle mounding (2, 8, 25). Patients display lack of the subcutaneous adipose tissue, while the mechanical adipose tissue (primary in the retro-orbital region and in small amounts in the buccal, masticator, para-pharyngeal, infrapatellar, and popliteal regions) and bone marrow fat are preserved (21, 26, 27). Regarding their metabolic profile, subjects may present with hypertriglyceridemia, hepatomegaly and hepatic steatosis, hyperinsulinemia, and insulin resistance, although clear evidence of type 2 diabetes has not been described (1, 11, 19, 28). A peculiar feature of this CGL subtype is the involvement of the skeletal and cardiac muscles. Patients display congenital myopathy with high creatine kinase serum levels, local protracted muscle contractions (also known as “mounding”), and severe arrhythmias usually exercise induced (such as long QT syndrome, catecholaminergic polymorphic ventricular tachycardia) causing an increased risk for sudden cardiac death (18, 29). Hypertrophic cardiomyopathy, with or without hypertension, associated with an ectopic cardiac fat deposition and/or lipotoxicity has been described (30). Some case reports have described nephrosis and transient immunoglobulin A deficiency (20, 31, 32). Patients affected by this disease are expected to be hyperphagic due to the impairment of the feedback between adipocytes and central feeding mechanisms (10). One of the master regulators of this signaling is leptin, an adipokine produced in the WAT, whose levels are directly proportional to body fat mass and are, therefore, extremely low in subjects with CGL (3). Leptin modulates food intake, satiety, and energy balance through its action on hypothalamic neurons (33); therefore, low levels of leptin in lipodystrophy can trigger hyperphagia (34).
We herein describe the clinical phenotypes of two pediatric siblings with CGL4, carrying a novel mutation in exon 1 of the PTRF gene in the homozygous state.
Methods
Anthropometric measurements and body composition
Birth weight and length were expressed as SD according to WHO growth charts. Height, weight, and waist and hip circumferences (WC and HC, respectively) at our first evaluation were measured by standard procedures, and body mass index (BMI) was calculated. Height, weight, and BMI percentiles and SD were calculated according to the WHO growth charts. Pubertal stages were determined by physical examination, using the criteria of Marshall and Tanner. Skinfold thickness was measured with a Lange caliper (GIMA 27320) at trunk (subscapular) and peripheral (biceps, triceps and calf) sites on the right side of the body. The average of three repeat measurements at each site was calculated. Blood pressure was measured, and systolic (SBP) and diastolic (DBP) values were evaluated according to standard procedure.
Hand-held bioelectric impendence analysis (BIA; TANITA MC-780MA) was used to determine whole-body fat, which was calculated as percentage of body mass. BIA was performed in the male patient, whereas female patients were excluded because age was not comprised in the software formula. BIA percentages were stratified according to the percentile reference value for anthropometric body composition indices in European children from the IDEFICS Study (35).
Imaging assessment
Abdominal ultrasound (Toshiba, Appler 500) and abdominal MRI (Philips 1.5 Tesla) were performed to evaluate hepatomegaly and hepatic steatosis. The extent of fat loss was evaluated by chest and abdominal MRI. Fibroscan (Echosens 502 F00216) was made to assess liver stiffness, expressed in kPa. For neuromuscular assessment, cervical spine X-ray and spinal column X-ray (Samsung AccE GC85A) were performed to evaluate atlantoaxial instability and scoliosis, respectively. Ventricular hypertrophy and arrhythmia were assessed by two-dimensional echocardiography and electrocardiogram (ECG), respectively. The degree of muscular damage was established through musculoskeletal ultrasounds (Samsung SW2F-45EB). Brain and cervical spinal cord MRI (Philips 3 Tesla) were performed to rule out neurological malformations. The presence of bone lytic lesions was assessed by hand and wrist radiography. Skeletal age was established through hand X-ray, according to the Greulich and Pyle method.
Biochemistry, hormonal, and immune evaluations
Biochemical and hormonal evaluations were carried out after 12 h of fasting at baseline. They were assessed using standardized methods in the hospital’s chemistry laboratory, particularly the following: serum glucose (mg/dl), insulin (μUI/ml), C-peptide (ng/ml), glycated hemoglobin (mmol/mol), serum total cholesterol (mg/dl), high-density lipoprotein (HDL)-cholesterol (mg/dl), low-density lipoprotein (LDL)-cholesterol (mg/dl), triglycerides (mg/dl), serum aspartate aminotransferase (AST, U/L), alanine aminotransferase (ALT, U/L), gamma glutamyl transferase (γGT, U/L), serum creatinine (mg/dL), serum 25-hydroxy (OH) vitamin D (ng/ml), calcium (mg/dl), phosphorus (mg/dl), parathyroid hormone (PTH, pg/ml), alkaline phosphatase (ALP, U/L), creatine kinase (CK, U/L), insulin-like growth factor 1 (IGF1, ng/ml), growth hormone (GH, ng/ml), serum complement component 3 (C3, mg/dl), complement component 4 (C4 mg/dl), immunoglobulin A (IgA, mg/dl), immunoglobulin G (IgG, mg/dl), and immunoglobulin M (IgM, mg/dl).
Serum leptin (mcg/L) and adiponectin (mcg/ml) were measured by CLIA from Mediagnost, Reutlingen, Germany.
Genetic analysis
The DNA was extracted from 200 µl of whole blood (anti-coagulated with EDTA) using ReliaPrep™ Blood gDNA Miniprep System (Promega, Fitchburg, WI, USA). For clinical exome sequencing panel, the Agilent SureSelect Custom Constitutional Panel 17 Mb (Agilent, Santa Clara, CA, USA) was used, which includes 5,227 clinically relevant genes. Target enrichment was performed for all designs using the Agilent SureSelectQXT NGS target enrichment kit for Illumina multiplexed sequencing (Agilent Technologies). Sequencing was performed using Illumina MiSeq (Illumina, UK) and MiSeq v3 300 Cycle Reagent Kits. Sequence alignment, annotation, categorization, and variant calling were performed using SureCall v3.5 software (Agilent), and Variant Call Format (VCF) files were annotated with wANNOVAR (http://wannovar.wglab.org) software. The filtering of the variants was based on their frequency in the public databases: 1000 Genome project (http://browser.1000genomes.org), EXAC (http://exac.broadinstitute.org), dbSNP (http://www.ncbi.nlm.nih.gov/projects/SNP/), and gnomAD (https://gnomad.broadinstitute.org/). All the variants that were not present in public databases and variants with a minor allele frequency (MAF) <1% were considered as potentially pathogenic.
The variant (NM_012232 exon1:c T21A:p.Y7X) identified by clinical-exome sequencing was validated by amplifying PTRF exon 1 with primers 5′-AGCCAATCAGCGATCAGACT-3′ (forward) and 5′-GATTTTGTCCAGGAGGCTCA-3′ followed by Sanger sequencing.
Informed consent
Patients and parents gave their informed consent for genetic studies and publication of their clinical details and images.
Results
Patient 1
The oldest of the two siblings was a male Caucasian, born full-term after an uncomplicated pregnancy and delivery in Romania. At birth, his weight was 3.2 kg (−0.69 SD), and length was 50 cm (−0.40 SD). Parents reported not being consanguineous, but they came from the same small Moldavian town. They referred that a male cousin distantly related to both of them, with a phenotype resembling patient 1, died at the age of 40 years from cardiac complications. At 40 days of age, patient 1 was admitted to the hospital for forceful vomiting and underwent surgery for pyloric stenosis. He also suffered from left inguinal hernia, gastroesophageal reflux disease, and lower airway infections, which often required hospitalization (a total of nine admissions for pneumonia in the first 4 years of life occurred). Parents referred that the subcutaneous fat loss became evident during the first months of life, especially in the face and limbs. Motor milestones and mental development were normal. He started walking at 10 months and speaking approximately 15 months of age. His height was always within the target height (TH), weight <3° percentile, and BMI between 3° and 10° percentile. After weaning, parents noticed that he was hyperphagic, mainly for food rich in fats and sugars. The patient’s walking rapidly deteriorated, and at the age of 2 years, he was only able to walk on his toes. Biochemical assessments revealed elevated serum CK levels, IgA deficiency, normal IgG levels, and mildly increased IgM levels. Total cholesterol and fasting glucose levels were within the normal range. Mild hypertriglyceridemia and impaired liver function were detected (Supplementary Table S1). The echocardiogram showed a diastolic dysfunction of the right ventricle, ostium secundum atrial septal defect, tricuspidal reflux (I–II grade), and patent ductus arteriosus (PDA). The abdominal ultrasonography detected hepatomegaly with initial steatosis. Due to the persistently elevated CK levels, he underwent muscle biopsy that showed dystrophic changes (variation in fiber size, necrotic fibers, and fibers with atypical regenerating form). Electromyography and nerve conduction studies were normal.
At our first evaluation, the patient was 5.2 years old, his height was 113.4 cm (58° percentile, +0.21SD) within the target height (TH); weight (19.5 kg, 41° percentile, −0.24 SD) and BMI (15.16 kg/m2, 32° percentile, −0.47 SD) were normal. Waist and hip circumferences were 54 and 55 cm, respectively. Skinfold thickness showed a reduction in subcutaneous fat tissue in the upper and lower limbs (<1° percentile) (Table 1). His body fat percentage at BIA was 11%.
Physical examination was characterized by generalized lack of subcutaneous fat, with preservation in the palms and soles. Acromegaloid appearance, muscular hypertrophy (more evident in the lower limbs), prominent veins, broad nasal bridge, mandibular hypoplasia, and hypertelorism were detected (Figure 1). Liver and spleen were palpable 1 cm below the costal margin, and he had umbilical prominence; no acanthosis nigricans was visible. His genitalia were prepubertal, and testes were approximately 2 ml bilaterally. He could only walk on his toes due to the shortening of the Achilles tendon, keeping a posture of anterior trunk flexion to compensate. Deep tendon reflexes were present. He did not demonstrate percussion-induced muscle mounding or the rippling phenomenon. Mental development was normal.
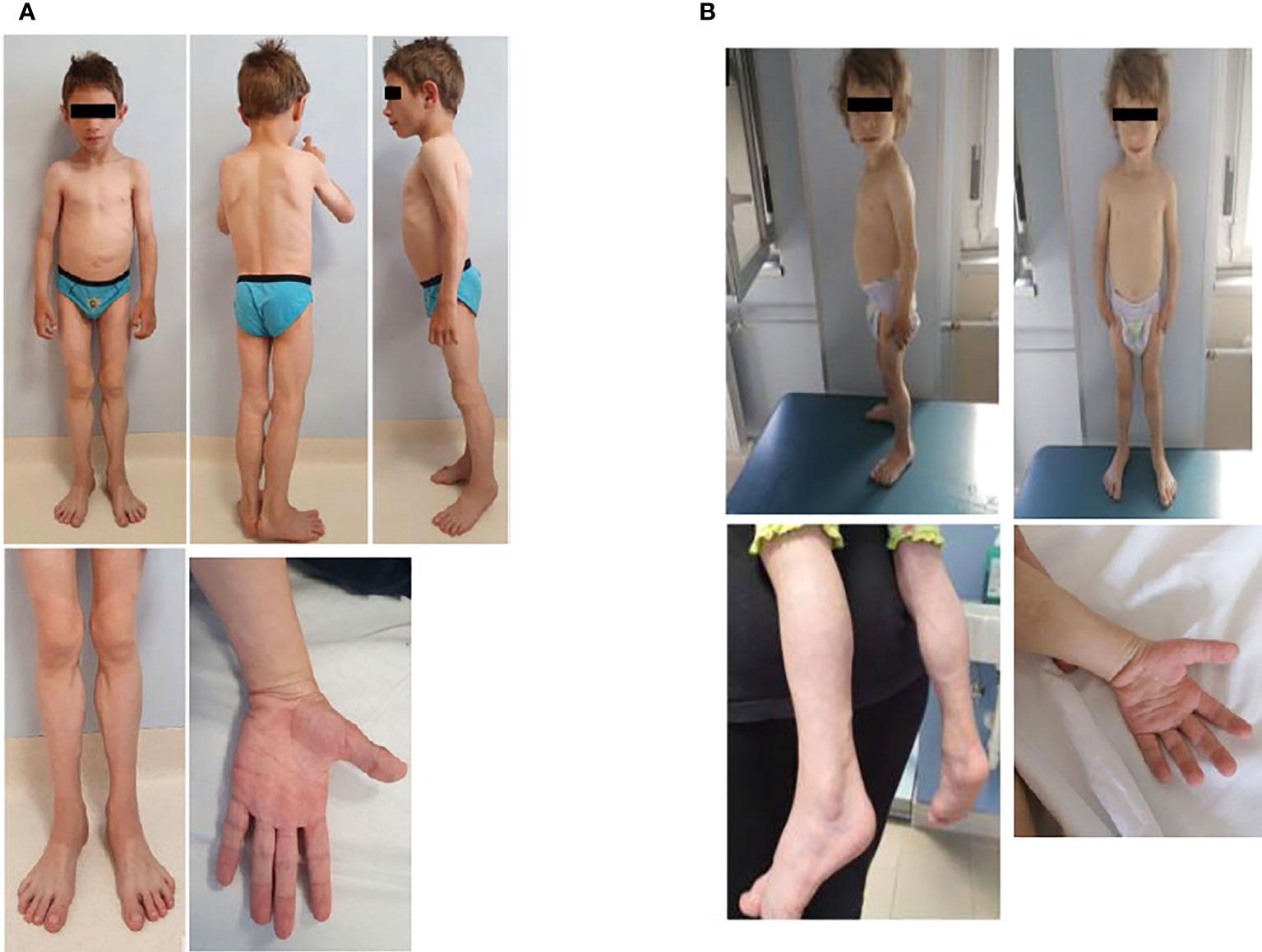
Figure 1 Anterior and side view of the male (A) and the female (B). Pictures show the generalized loss of subcutaneous adipose tissue leading to prominence of muscular hypertrophy (more evident in the lower limbs) and prominent veins. The male patient has also progeroid facial features and an obliged posture on his toes, while the female has an acromegaloid appearance.
Biochemical blood tests (Table 2) showed mild hypertriglyceridemia, normal cholesterol levels, IgA deficiency, mildly increased IgM, impaired liver function, normal thyroid, parathyroid, and adrenal functions. He had a normal fasting glucose profile but had initial signs of insulin resistance. CK levels were very high. Renal function was normal, with no proteinuria. IGF-1 values were in the upper end of the normal range, and GH pulsatility was normal. Serum leptin and adiponectin levels were undetectable.
Cervical X-ray detected atlantoaxial instability with predental space of 3.5 mm in neutral position, which increased to 4 mm during flexion (Supplementary Figure S1A); the spinal column X-ray revealed mild scoliosis (Supplementary Figure S1B). Brain and cervical spinal cord MRI ruled out neurological malformations. He did not report neck pain, and no signs of myelopathy could be observed.
Resting ECG was normal with a regular QT interval, but no exercise test was undertaken on the boy, due to his young age, to search for exercise-induced arrythmias. Echocardiography showed mild mitral regurgitation and patent foramen ovale (PFO), without signs of ventricular hypertrophy.
The hand and wrist radiography showed a bone age more than 2 years advanced, no signs of cystic lesions, but a reduction in the calcium content with alteration of the trabecular bone mass (Supplementary Figure S1C). Abdominal ultrasound and MRI displayed hepatomegaly without hepatic steatosis. No liver stiffness was detected at fibroscan (E, 4.9 kPa). Generalized loss of adipose tissue was evident at T1-weighted head, chest, and abdominal body MRI (Figure 2). Musculoskeletal ultrasound revealed dystrophic changes in muscle fibers (Supplementary Figure S1D).
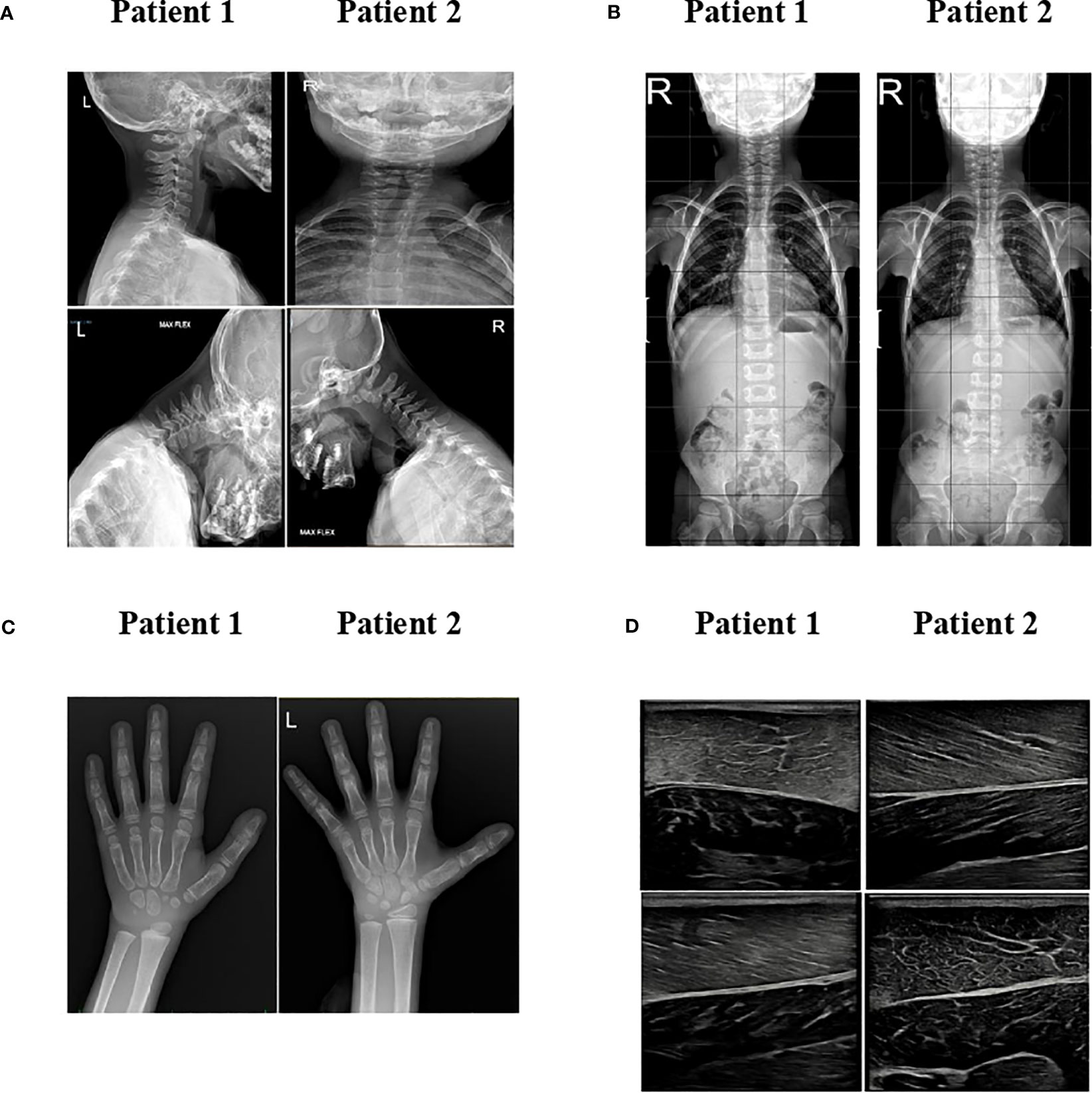
Figure 2 MRI imaging. T1-weighted MR imaging of patients 1 and 2 in comparison with a normal subject at different levels. Adipose tissue has a high signal intensity (brightness) on MRI T1-weighted images. When compared to normal subjects, the two patients have marked loss of subcutaneous fat, except for retro-orbital and bone marrow fat.
Patient 2
The sister of patient 1 was born full-term after an uncomplicated pregnancy and delivery in Romania. At birth, her weight was 3.7 kg (+0.85 SD), and the length was 49 cm (−0.75 SD). At 3 months of age, the patient suffered a cardiac arrest resolved after cardiopulmonary resuscitation. The cardiac assessment revealed a paroxysmal supraventricular tachycardia (PSVT). The child was placed on therapy with beta-blocker and digoxin until she was 1 year old and did not experience further episodes of arrhythmia ever since. Like her brother, a lack of subcutaneous fat was noticed during the first months of life, especially in the face and limbs. She showed normal motor milestones and mental development: she started speaking approximately 15 months of age and walking at 12 months, preferably on her toes. She also presented hyperphagia and preference for food rich in fats and sugars. Her height was always within the target height, weight was 10°–25° percentile, and BMI was between 10° and 25° percentile. Biochemical assessments are shown in Supplementary Table S1. The abdominal ultrasonography showed no signs of hepatomegaly or steatosis.
At our first evaluation, she was 2.2 years old, her height was within the TH (89.1 cm, 50° percentile, +0.0 SD), and weight (11.8 kg, 26° percentile, −0.65 SD) and BMI (14.8 kg/m2, 22° percentile, −0.76 SD) were normal. Waist and hip circumferences were 48 and 45 cm, respectively. Skinfold thickness showed a reduction in subcutaneous fat in the upper and lower limb (<1° percentile) (Table 1). BIA was not performed due to her young age. Physical examination revealed near total of lack of fat with preservation of fat in the palms and soles. She also displayed muscular hypertrophy (more evident in the lower limbs) and prominent veins, typical facial features, a triangular face, broad nasal bridge, mandibular hypoplasia, hypertelorism, periorbital edema, and cutis marmorata. No acanthosis nigricans was visible (Figure 1). Her genitalia were prepubertal with clitoromegaly. She preferred walking on her toes but was able to walk normally, keeping a posture of minimal anterior trunk flexion. Deep tendon reflexes were normal, and percussion-induced muscle mounding or the rippling phenomenon was absent. Mental development was normal.
Biochemical blood tests resembled those of the brother (Table 2). Her fasting glucose was normal with no increase in Homeostatic Model Assessment for Insulin Resistance (HOMA-IR) yet. Her CK levels were increased. IGF-1 concentration was slightly increased, but GH pulsatility was normal. Serum leptin and adiponectin levels were undetectable.
Cervical X-ray detected atlantoaxial instability with predental space of 2.5 mm in neutral position, which increased to 4 mm during flexion (Supplementary Figure S1A); the spinal column X-ray revealed mild scoliosis (Supplementary Figure S1B). Like her brother, she did not have neck pain, and there were no signs of myelopathy. Brain and cervical spinal cord MRI ruled out neurological malformations.
Echocardiography did not show signs of ventricular hypertrophy. The hand and wrist radiography showed a bone age 1 year advanced, with no signs of cystic lesions, but like her brother, a reduction in the calcium content with alteration of the trabecular bone mass was observed (Supplementary Figure S1C).
Abdominal ultrasound and MRI displayed hepatomegaly without hepatic steatosis. Elastography showed increased liver stiffness (5.1 kPA). T1-weighted body MRI was very similar to patient 1, showing generalized loss of adipose tissue (Figure 2). Musculoskeletal ultrasound revealed dystrophic changes in muscle fibers with adipocytes infiltrations (Supplementary Figure S1D).
Genetic analysis
Clinical exome sequencing performed in the male patient revealed the presence of a novel pathogenic variation at the homozygous state in exon 1 of the CAVIN1/PTRF gene (NM_012232.6), namely, c.21T>A that converts the amino acid tyrosine at position 7 of the protein in a stop codon (p.Tyr7Ter), thus causing the premature truncation of the protein. The variant, validated by Sanger sequencing, was inherited from the parents who were both heterozygous for this mutation (Supplementary Figure S2).
Genetic analysis in the sister confirmed the presence of the same pathogenic variation in exon 1 of the PTRF gene (NM_012232.6 c.21T>A;p.Tyr7Ter).
Discussion
This study describes the phenotypic and clinical characteristics of two pediatric siblings affected by CGL type 4 due to a novel homozygous mutation in the PTRF/CAVIN 1 gene.
CGL4 is a recently identified subtype of CGL (CGL4, MIM 613327) characterized by distinctive clinical features such as myopathy, gastrointestinal abnormalities, cardiac arrythmias, and cervical spine instability (36). Initially, CGL4 was phenotypically described in two Mexican American siblings as a novel subtype of CGL (37). Afterwards, mutations in the polymerase I and transcript release factor (PTRF) gene, encoding a cytoplasmatic protein called caveolae-associated protein 1 (Cavin-1), essential for caveolae formation, were described as the etiology (20). To date, 15 mutations (involving 30 patients) of this gene have been described in HGMD (http://www.hgmd.cf.ac.uk/ac/index.php) and diagnosed in several countries (Japan, Saudi Arabia, Oman, Egyptian, Turkey, the United Kingdom, Germany, Morocco, and Mexico). Previously, five missense/nonsense mutations, two splice sites, five small deletions, and three small insertions were discovered in the CAVIN1/PTRF gene (18–20, 28, 30, 36–46). We herein describe a novel homozygous mutation (c.21T>A; p.Tyr7Ter) that causes a change in the reading frame to stop codon, resulting in a premature protein truncation with a likely loss of function effect. Interestingly, the majority of patients with CGL4 reported so far had null mutations in the CAVIN1/PTRF gene, exhibiting classical characteristics of the disease similar to our cases. Although limited, milder phenotypes have been described in rare cases, suggesting phenotypic variability in CGL4. A single base deletion (c.947delA) resulting in a frameshift, depletion of the normal stop codon, and extension of the 3′ untranslated region (UTR) reading frame of the CAVIN1/PTRF gene, producing a predicted protein of higher molecular weight, was described in a Moroccan child who was reported to have a subtle phenotype of CGL4 (41). This prompted the question of whether less severe mutations, such as missense mutations, could be associated with CGL4 of less severe phenotypes. Mild metabolic disorders in heterozygous carriers were reported, suggesting that these individuals may be at risk for mild metabolic disturbances even though they do not exhibit overt lipodystrophy or myopathy (18, 25).
Both of our patients showed early metabolic complications such as hypertriglyceridemia, low HDL cholesterol levels, hepatomegaly, and hepatic steatosis with elevated levels of liver enzymes. A slight increase in HOMA-IR was observed only in one patient. The metabolic phenotype was similar to other previously described CGL4 cases (19, 38, 39, 45, 47). Dyslipidemia is a common finding among these patients, even in the first years of life, whereas diabetes tends to develop during puberty, but subjects can express elevated fasting insulin levels and mild insulin resistance at a younger age. In line with previous reports, none of our siblings had proteinuria or impaired renal function (31, 34). This could be explained by the younger age of our cases compared to the expected age of the onset of this comorbidity (31, 34). The relationship between generalized lipodystrophy and metabolic derangements is well known. Looking specifically at CGL4, PTRF-CAVIN protein is a master regulator in the process of caveolae stabilization (22, 23). The lack of caveolae can affect the regulation of lipolysis, fatty acid flux, triglyceride synthesis, and alteration of insulin signaling in adipocytes, as caveolae are known to concentrate insulin receptors along their margins (14, 39). Caveolae function is extremely complex, including the compartmentalization of multiple signaling pathways not related to the metabolism. A recent case report has shown how the immune system of a subject with CGL4 did not mount a normal response to a single dose of lipid nanoparticle encapsulated mRNA anti-SARS-CoV-2 vaccine but responded to a single dose of the Adenovirus-based vaccine (48). The authors hypothesized that the poor patient’s immune response could be related to the reduced import of the vaccine due to the lack of caveolin, since the lipid nanoparticle encapsulated mRNA is internalized by caveolae-mediated endocytosis.
Patients with CGL4 have been described to present a milder metabolic profile compared to the other subtypes of CGL (20, 28, 36, 38), but several biases may intervene to confound the clinical picture, including the age at diagnosis, the dietary regimen, and lifestyle of various affected subjects. In the past, authors hypothesized that the missense mutations frequently observed in patients with CGL4 could cause a milder metabolic phenotype (28). Another possible explanation could be related to a relatively later loss of secretory function of adipocytes, mainly leptin and adiponectin than other CGLs (10). However, both our patients showed very low/undetectable levels of leptin and adiponectin at very young ages, similarly to most children with CGL4 described by others (31–33, 40). In addition, patients with CGL1 or CGL2, the subtype associated with the most severe metabolic phenotypes, had not only low leptin and adiponectin levels but also very low circulating free leptin and high-soluble leptin receptor levels (49). Since the circulating free leptin isoform is the biologically active subtype, this form could determine the clinically divergent metabolic phenotype. We could therefore speculate that patients with CGL4 have higher circulating free leptin levels and lower protein-bound forms than other CGLs, causing the milder phenotype. Further studies on these patients are necessary to confirm this hypothesis. Other possible explanations for the milder phenotype could be related to the younger age of our patients, preventing the natural disease progression, which typically occurs after early childhood, or related to the gastrointestinal disease potentially limiting food intake or the hypertrophic muscles potentially consuming a portion of surplus energy.
Body composition studies highlighted a reduction in whole subcutaneous adipose tissue, especially over the extremities. MRI scans revealed the characteristic pattern of fat distribution, with generalized loss of SAT but preserved mechanical fat in the retroorbital and periarticular regions, over the palms and soles and in the bone marrow fat. These results were similar to those demonstrated in several studies regarding CGL4 (19, 29, 37, 38), except for the presence of adipose tissue in the palms and soles that was not described in older patients (38). We could hypothesize that the adipose tissue loss in the hands and feet could manifest later in life, typically when the children are in puberty, contrary to the metabolic adipose tissue loss that is already evident from the first months of life.
However, despite the decreased body fat, children had normal height and growth rate was accelerated, likely a result of the relatively high IGF-1 values detected in the patients despite normal GH pulsatility. Since caveolae constitute one pathway for the internalization of GH in vitro (18, 46), the elevated growth velocity and IGF-1 levels, observed in our children with CGL4, could be associated with the reducing caveolae formations, typical of this subtype of lipodystrophy, since GH pulsatility was normal.
The role of leptin as a regulator of bone mass is controversial (50, 51). However, children and adults affected by CGL had no alterations in bone metabolism markers, despite leptin deficiency (52, 53). In our patients, hand and wrist X-ray of our siblings showed a reduction in the calcium content with trabecular bone mass alteration, while baseline serum markers of bone metabolism were within the normal range, except for hypovitaminosis D, and no past fractures were reported. The low calcium content could be related to hypovitaminosis D and the increased growth rate at the time of diagnosis. The follow-up will help clarify bone metabolism during growth.
Apart from the bone, CGL4 importantly affects skeletal, cardiac, and gastrointestinal muscles (21, 23). Bioptic, electron microscopy, and US studies have shown chronic dystrophic changes, including marked variation in muscle fiber size, internal nucleation, a few necrotic and regenerating fibers, increased interstitial fibrosis with reduced caveolae formation, and accumulation of lipid droplets (20, 29, 39–41). Although the exact pathogenesis of myopathy remains unclear, it is common thought that the lack of caveolae has a crucial role in the pathogenesis of the muscle phenotype (30). In our patients, muscle abnormality was denounced during the first years of life, as demonstrated by rapidly deteriorating walking ability and extremely elevated serum CK levels. The muscle biopsy performed on the boy showed variation in fiber size, necrotic fibers, and atypical regenerating forms. At ultrasound, muscles appeared hyperechogenic due to replacement of the hypoechogenic muscle bundles with adipocytes. Tight Achilles tendon is another hallmark of CGL4 caused by hypertrophic calves. Patients are not able to walk on their feet but are forced to stay on their toes, which dramatically increases forefoot pressures (29). In agreement with the literature, both of our patients suffered from Achilles tendon retraction from the first years of life, with the boy presenting a more severe phenotype. Other interesting features in both children were scoliosis and atlantoaxial dislocation, whereas we did not observe percussion-induced muscle mounding, a peculiar feature of CGL4 and of muscular dystrophy due to mutations in the CAV3 gene (19, 29, 40). In the latter disease, preservation of sarcolemmal caveolin-3 staining pattern was observed in young patients compared to older subjects who showed a complete deficiency of caveolin-3, associated with percussion-induced muscle mounding (20, 40, 41). We may hypothesize that in patients with CGL4, secondary reduction in caveolin-3 might arise with age and/or disease progression, and only when deficiency of both proteins (caveolin-3 and cavin-1) develops that muscle mounding can be observed.
Gastrointestinal tract disorders have been described in СGL4 to underline the role of cavin-1 in muscle functionality (18, 20, 28, 38, 42, 45). Massive smooth muscle hypertrophy and thick muscularis mucosa have been shown in CGL4 patients (18, 30). Our male patient had a history of pyloric stenosis, while his sister did not show signs of gastrointestinal involvement, suggesting a phenotypic variability in the presence of the same mutation. The ultrasound study of the gut was normal, but tight dysmotility could be hypothesized since both children, mainly the male one, frequently suffer from abdominal pain not responsive to classic gastrointestinal care advice.
Cardiac arrhythmia have been reported in many CGL4 patients, with an increased risk of sudden death, likely secondary to ventricular arrhythmia also during adolescence (18, 20, 28, 30, 38, 41, 45, 54). The underlying mechanism of paroxysmal ventricular tachycardia (PVT) in patients with CGL4 is unclear. Autoptic studies showed adipocytes interdigitating muscle fibers and mild fibro-fatty infiltration close to the coronary sinus area and atrioventricular node (30). Therefore, the novel cardiac pathology could promote the development of progressive cardiomyopathy, with left ventricular hypertrophy and interstitial/perivascular fibrosis around cardiomyocytes with an alteration of membrane polarization. Mutations in CAV3 gene, also involved in the caveolae formation, induce an increase in late sodium current by affecting the sodium channels located in the caveolae (28); we could hypothesize that PTRF/CAVIN1 mutations in patients affect the sodium channel or the other cardiac channels. In resting conditions, no arrhythmia episodes are described in patients with CGL4. However, during exercise when the heart is subjected to a more significant physical effort, due to the possible mechanism previously described, arrhythmias could develop. Since cardiac arrhythmia is a severe and potentially life-threatening condition, occurring frequently after emotions or exercise, patients with CGL4 should be closely monitored by ECG and, if necessary, fitted with an implanted pacemaker and cardioverter defibrillator (ICD) device. The girl, but not the boy, suffered from paroxysmal supraventricular tachycardia (PSVT) at 3 months of age, causing a cardiac arrest, suggesting again a significant degree of phenotypic variability. After cardiopulmonary resuscitation, the child started therapy with beta-blocker and digoxin until she was 1 year old. No other episodes have been described since then. Echocardiography did not show signs of ventricular hypertrophy in both children. However, they are under tight control, and physical activity has been scheduled after cardiologist and physiatrist indications and monitoring.
Conclusion
We have herein described the phenotype of two pediatric siblings affected by CGL4 due to a novel homozygous mutation of the CAVIN1/PTRF gene. The coexistence of generalized lack of fat, myopathy with elevated CK levels, arrhythmias, gastrointestinal dysmotility, and skeletal abnormalities should prompt a diagnosis of CGL4, although phenotypic variability could be present.
CGL4 in Europe is extremely rare, and only two other cases have been described so far, while the majority of patients come from families of Omani, Japanese, Hispanic, Moroccan, and Turkish origin.
Most patients from these countries, described so far, have identical point mutations, suggesting that they have all the same origin, while in Europe, the genetic etiology of CGL4 previously reported is more complex due to a variability in patients with different ethnic backgrounds, and the presence of immigrant families form different parts of the world. The diagnosis of patients with CGL4 with a heterogeneous molecular etiology is fundamental to detail variable phenotypic expressions, as was observed in our patients.
Data availability statement
The datasets presented in this article are not readily available because of ethical and privacy restrictions. Requests to access the datasets should be directed to the corresponding author/s.
Ethics statement
The studies involving human participants were reviewed and approved by the Ethics Committee of Maggiore Hospital Novara (protocol code 69/19 and date of approval 2019). Written informed consent to participate in this study was provided by the participants' legal guardian/next of kin. Written informed consent was obtained from the participants/patients' legal guardian/next of keen for the publication of this case report.
Author contributions
VM, TD, MR, and FP collected the anamnestic and the biochemical data for the patients. VM, AB, and TD performed the literature search, reviewed, and extracted data from the papers. MG, SM, and CP performed the genetic and hormones analysis. VM and SB performed the figures and table designing. VM, FP, SM, and MG wrote the manuscript. FP, BA, GA, GC, FS, and IR verified the analytical methods and supervised the manuscript drafting. All authors discussed the results and contributed to the final manuscript. All authors contributed to the article and approved the submitted version.
Funding
This publication is part of the project NODES, which has received funding from the MUR-M4C2 1.5 of PNRR with grant agreement no. ECS00000036.
Acknowledgments
We would like to thank the patients and their parents for their participation in this work. The Obesity and Lipodystrophy Center at the University Hospital of Pisa and the SCDU Endocrinology of University of Piemonte Orientale are part of the European Consortium of Lipodystrophies. The Obesity and Lipodystrophy Center at the University Hospital of Pisa is also part of the European Reference Network for Rare Endocrine Conditions (EndoERN) and for Rare Hereditary Metabolic Disorders (MetabERNProject ID No 739543).
Conflict of interest
FP worked as a consultant for the following companies, which are involved with lipodystrophy and/or diabetes: Aegerion/Amryt Pharmaceuticals, Novo Nordisk. BA attended advisory board meetings organized by Amryt Pharmaceuticals and Regeneron Pharmaceuticals and has served as a consultant and/or received honoraria as a speaker from Third Rock Ventures, Amryt, Regeneron, AstraZeneca, Lilly, MSD, Novartis, Novo Nordisk, Boehringer-Ingelheim, Servier, and Sanofi-Aventis. GC has received fees for consulting and/or received travel funds or participated in studies from the following companies, which are involved with lipodystrophy and/or diabetes: Aegerion/Amryt Pharmaceuticals, Novo-Nordisk, and Rhythm Pharmaceuticals. SM received travel funds from the following company, which was involved with lipodystrophy: Amryt Pharmaceuticals. FS has worked as a consultant, participated in studies, and/or received travel funds from the following companies, which are involved with lipodystrophy and/or diabetes: Aegerion/Amryt, Novo Nordisk, Lilly, Bruno Pharma, and Pfizer.
The remaining authors declare that the research was conducted in the absence of any commercial or financial relationships that could be construed as a potential conflict of interest.
Publisher’s note
All claims expressed in this article are solely those of the authors and do not necessarily represent those of their affiliated organizations, or those of the publisher, the editors and the reviewers. Any product that may be evaluated in this article, or claim that may be made by its manufacturer, is not guaranteed or endorsed by the publisher.
Supplementary material
The Supplementary Material for this article can be found online at: https://www.frontiersin.org/articles/10.3389/fendo.2023.1212729/full#supplementary-material
References
1. Araújo-Vilar D, Santini F. Diagnosis and treatment of lipodystrophy: a step-by-step approach. J Endocrinol Invest (2019) 42:61–73. doi: 10.1007/S40618-018-0887-Z
2. Brown RJ, Araujo-Vilar D, Cheung PT, Dunger D, Garg A, Jack M, et al. The diagnosis and management of lipodystrophy syndromes: a multi-society practice guideline. J Clin Endocrinol Metab (2016) 101:4500–11. doi: 10.1210/JC.2016-2466
3. Ren Y, Zhao H, Yin C, Lan X, Wu L, Du X, et al. Adipokines, hepatokines and myokines: focus on their role and molecular mechanisms in adipose tissue inflammation. Front Endocrinol (Lausanne) (2022) 13:873699. doi: 10.3389/FENDO.2022.873699
4. Friedman JM. Leptin and the endocrine control of energy balance. Nat Metab (2019) 1:754–64. doi: 10.1038/S42255-019-0095-Y
5. Francisco V, Pino J, Campos-Cabaleiro V, Ruiz-Fernández C, Mera A, Gonzalez-Gay MA, et al. Obesity, fat mass and immune system: role for leptin. Front Physiol (2018) 9:640. doi: 10.3389/FPHYS.2018.00640
6. Ceccarini G, Magno S, Pelosini C, Ferrari F, Sessa MR, Scabia G, et al. Congenital generalized lipoatrophy (Berardinelli-seip syndrome) type 1: description of novel AGPAT2 homozygous variants showing the highly heterogeneous presentation of the disease. Front Endocrinol (Lausanne) (2020) 11:39/BIBTEX. doi: 10.3389/FENDO.2020.00039/BIBTEX
7. Patni N, Hegele RA, Garg A. Caveolar dysfunction and lipodystrophies. Eur J Endocrinol (2022) 186:C1–4. doi: 10.1530/EJE-21-1243
8. Özen S, Akıncı B, Oral EA. Current diagnosis, treatment and clinical challenges in the management of lipodystrophy syndromes in children and young people. J Clin Res Pediatr Endocrinol (2020) 12:17–28. doi: 10.4274/JCRPE.GALENOS.2019.2019.0124
9. Ceccarini G, Magno S, Gilio D, Pelosini C, Santini F. Autoimmunity in lipodystrophy syndromes. Presse Med (2021) 50(3):104073. doi: 10.1016/J.LPM.2021.104073
10. Lim K, Haider A, Adams C, Sleigh A, Savage DB. Lipodistrophy: a paradigm for understanding the consequences of “overloading” adipose tissue. Physiol Rev (2021) 101:907–93. doi: 10.1152/PHYSREV.00032.2020
11. Akinci B, Meral R, Oral EA. Phenotypic and genetic characteristics of lipodystrophy: pathophysiology, metabolic abnormalities, and comorbidities. Curr Diabetes Rep (2018) 18(12):143. doi: 10.1007/S11892-018-1099-9
12. Foss-Freitas MC, Akinci B, Luo Y, Stratton A, Oral EA. Diagnostic strategies and clinical management of lipodystrophy. Expert Rev Endocrinol Metab (2020) 15:95–114. doi: 10.1080/17446651.2020.1735360
13. Rochford JJ. When adipose tissue lets you down: understanding the functions of genes disrupted in lipodystrophy. Diabetes (2022) 71:589–98. doi: 10.2337/DBI21-0006
14. Garg A. Clinical review#: lipodystrophies: genetic and acquired body fat disorders. J Clin Endocrinol Metab (2011) 96:3313–25. doi: 10.1210/JC.2011-1159
15. Chiquette E, Oral EA, Garg A, Araújo-Vilar D, Dhankhar P. Estimating the prevalence of generalized and partial lipodystrophy: findings and challenges. Diabetes Metab Syndr Obes (2017) 10:375–83. doi: 10.2147/DMSO.S130810
16. Hoa Chung L, Qi Y. Lipodystrophy - a rare condition with serious metabolic abnormalities. Rare Dis (2020). doi: 10.5772/INTECHOPEN.88667
17. Nolis T. Exploring the pathophysiology behind the more common genetic and acquired lipodystrophies. J Hum Genet (2014) 59:16–23. doi: 10.1038/JHG.2013.107
18. Rajab A, Straub V, McCann LJ, Seelow D, Varon R, Barresi R, et al. Fatal cardiac arrhythmia and long-QT syndrome in a new form of congenital generalized lipodystrophy with muscle rippling (CGL4) due to PTRF-CAVIN mutations. PloS Genet (2010) 6(3):e1000874. doi: 10.1371/JOURNAL.PGEN.1000874
19. Sorkina E, Makarova P, Bolotskaya L, Ulyanova I, Chernova T, Tiulpakov A. Unusual clinical features associated with congenital generalized lipodystrophy type 4 in a patient with a novel E211X CAVIN1 gene variant. Clin Diabetes Endocrinol (2020) 6:7. doi: 10.1186/s40842-020-00095-3
20. Hayashi YK, Matsuda C, Ogawa M, Goto K, Tominaga K, Mitsuhashi S, et al. Human PTRF mutations cause secondary deficiency of caveolins resulting in muscular dystrophy with generalized lipodystrophy. J Clin Invest (2009) 119:2623–33. doi: 10.1172/JCI38660
21. Gomes Lima J, Catão Ferreira dos Santos M, Tamara Araújo de Melo Campos J. Congenital generalized lipodystrophy. J Rare Dis Res Treat (2018) 3:1–6. doi: 10.29245/2572-9411/2018/2.1147
22. Liu L, Pilch PF. A critical role of cavin (polymerase I and transcript release factor) in caveolae formation and organization. J Biol Chem (2008) 283:4314–22. doi: 10.1074/JBC.M707890200
23. Liu L. Lessons from cavin-1 deficiency. Biochem Soc Trans (2020) 48:147–54. doi: 10.1042/BST20190380
24. Perez-Diaz S, Johnson LA, DeKroon RM, Moreno-Navarrete JM, Alzate O, Fernandez-Real JM, et al. Polymerase I and transcript release factor (PTRF) regulates adipocyte differentiation and determines adipose tissue expandability. FASEB J (2014) 28:3769–79. doi: 10.1096/FJ.14-251165
25. Zammouri J, Vatier C, Capel E, Auclair M, Storey-London C, Bismuth E, et al. Molecular and cellular bases of lipodystrophy syndromes. Front Endocrinol (Lausanne) (2022) 12:803189. doi: 10.3389/FENDO.2021.803189
26. Patni N, Garg A. Congenital generalized lipodystrophies–new insights into metabolic dysfunction. Nat Rev Endocrinol (2015) 11:522–34. doi: 10.1038/NRENDO.2015.123
27. Altay C, Seçil M, Demir T, Atik T, Akıncı G, Kutbay NÖ, et al. Determining residual adipose tissue characteristics with MRI in patients with various subtypes of lipodystrophy. Diagn Interv Radiol (2017) 23:428–34. doi: 10.5152/DIR.2017.17019
28. Shastry S, Delgado MR, Dirik E, Turkmen M, Agarwal AK, Garg A. Congenital generalized lipodystrophy, type 4 (CGL4) associated with myopathy due to novel PTRF mutations. Am J Med Genet A (2010) 152A:2245–53. doi: 10.1002/AJMG.A.33578
29. Akinci G, Topaloglu H, Demir T, Danyeli AE, Talim B, Keskin FE, et al. Clinical spectra of neuromuscular manifestations in patients with lipodystrophy: a multicenter study. Neuromuscular Disord (2017) 27:923–30. doi: 10.1016/j.nmd.2017.05.015
30. Patni N, Vuitch F, Garg A. Postmortem findings in a young man with congenital generalized lipodystrophy, type 4 due to CAVIN1 mutations. J Clin Endocrinol Metab (2019) 104:957–60. doi: 10.1210/jc.2018-01331
31. Javor ED, Moran SA, Young JR, Cochran EK, Depaoli AM, Oral EA, et al. Proteinuric nephropathy in acquired and congenital generalized lipodystrophy: baseline characteristics and course during recombinant leptin therapy. J Clin Endocrinol Metab (2004) 89:3199–207. doi: 10.1210/JC.2003-032140
32. Simha V, Garg A. Inherited lipodystrophies and hypertriglyceridemia. Curr Opin Lipidol (2009) 20:300–8. doi: 10.1097/MOL.0B013E32832D4A33
33. Obradovic M, Sudar-Milovanovic E, Soskic S, Essack M, Arya S, Stewart AJ, et al. Leptin and obesity: role and clinical implication. Front Endocrinol (Lausanne) (2021) 12:585887. doi: 10.3389/FENDO.2021.585887
34. Akinci B, Sahinoz M, Oral E. Lipodystrophy syndromes: presentation and treatment (2018). Endotext (Accessed October 15, 2022).
35. Nagy P, Kovacs E, Moreno LA, Veidebaum T, Tornaritis M, Kourides Y, et al. Percentile reference values for anthropometric body composition indices in European children from the IDEFICS study. Int J Obes (Lond) (2014) 38 Suppl 2:S15–25. doi: 10.1038/IJO.2014.131
36. Jelani M, Ahmed S, Almramhi MM, Mohamoud HSA, Bakur K, Anshasi W, et al. Novel nonsense mutation in the PTRF gene underlies congenital generalized lipodystrophy in a consanguineous Saudi family. Eur J Med Genet (2015) 58:216–21. doi: 10.1016/J.EJMG.2015.02.002
37. Simha V, Agarwal AK, Aronin PA, Iannaccone ST, Garg A. Novel subtype of congenital generalized lipodystrophy associated with muscular weakness and cervical spine instability. Am J Med Genet A (2008) 146A:2318–26. doi: 10.1002/AJMG.A.32457
38. Akinci G, Topaloglu H, Akinci B, Onay H, Karadeniz C, Ergul Y, et al. Spectrum of clinical manifestations in two young Turkish patients with congenital generalized lipodystrophy type 4. Eur J Med Genet (2016) 59:320–4. doi: 10.1016/J.EJMG.2016.05.001
39. Dwianingsih EK, Takeshima Y, Itoh K, Yamauchi Y, Awano H, Malueka RG, et al. A Japanese child with asymptomatic elevation of serum creatine kinase shows PTRF-CAVIN mutation matching with congenital generalized lipodystrophy type 4. Mol Genet Metab (2010) 101:233–7. doi: 10.1016/j.ymgme.2010.06.016
40. Murakami N, Hayashi YK, Oto Y, Shiraishi M, Itabashi H, Kudo K, et al. Congenital generalized lipodystrophy type 4 with muscular dystrophy: clinical and pathological manifestations in early childhood. Neuromuscul Disord (2013) 23:441–4. doi: 10.1016/J.NMD.2013.02.005
41. Ardissone A, Bragato C, Caffi L, Blasevich F, Maestrini S, Bianchi ML, et al. Novel PTRF mutation in a child with mild myopathy and very mild congenital lipodystrophy. BMC Med Genet (2013) 14:89. doi: 10.1186/1471-2350-14-89
42. van der Pol RJ, Benninga MA, Magré J, van Maldergem L, Rotteveel J, van der Knaap MS, et al. Berardinelli-seip syndrome and achalasia: a shared pathomechanism? Eur J Pediatr (2015) 174:975. doi: 10.1007/S00431-015-2556-Y
43. Salle-Teyssières L, Auclair M, Terro F, Nemani M, Elsayed SM, Elsobky E, et al. Maladaptative autophagy impairs adipose function in congenital generalized lipodystrophy due to cavin-1 deficiency. J Clin Endocrinol Metab (2016) 101:2892–904. doi: 10.1210/JC.2016-1086
44. Takeyari S, Takakuwa S, Miyata K, Yamamoto K, Nakayama H, Ohata Y, et al. Metreleptin treatment for congenital generalized lipodystrophy type 4 (CGL4): a case report. Clin Pediatr Endocrinol (2019) 28:1–7. doi: 10.1297/CPE.28.1
45. Güneş N, Kutlu T, Tekant GT, Eroğlu AG, Üstündağ NÇ, Öztürk B, et al. Congenital generalized lipodystrophy: the evaluation of clinical follow-up findings in a series of five patients with type 1 and two patients with type 4. Eur J Med Genet (2020) 63:103819. doi: 10.1016/j.ejmg.2019.103819
46. Adiyaman SC, v.Schnurbein J, de Laffolie J, Hahn A, Siebert R, Wabitsch M, et al. Congenital generalized lipodystrophy type 4 due to a novel PTRF/CAVIN1 pathogenic variant in a child: effects of metreleptin substitution. J Pediatr Endocrinol Metab (2022) 35:946–52. doi: 10.1515/jpem-2022-0022
47. Brown RJ, Meehan CA, Cochran E, Rother KI, Kleiner DE, Walter M, et al. Effects of metreleptin in pediatric patients with lipodystrophy. J Clin Endocrinol Metab (2017) 102:1511–9. doi: 10.1210/JC.2016-3628
48. Zaffina S, Piano Mortari E, Di Prinzio RR, Cappa M, Novelli A, Agolini E, et al. Case report: precision COVID-19 immunization strategy to overcome individual fragility: a case of generalized lipodystrophy type 4. Front Immunol (2022) 13:869042/FULL. doi: 10.3389/FIMMU.2022.869042/FULL
49. Cortés VA, Smalley S v., Goldenberg D, Lagos CF, Hodgson MI, Santos JL. Divergent metabolic phenotype between two sisters with congenital generalized lipodystrophy due to double AGPAT2 homozygous mutations. a clinical, genetic and in silico study. PloS One (2014) 9:869042. doi: 10.1371/JOURNAL.PONE.0087173
50. Reid IR, Baldock PA, Cornish J. Effects of leptin on the skeleton. Endocr Rev (2018) 39:938–59. doi: 10.1210/ER.2017-00226
51. Karsenty G, Oury F. The central regulation of bone mass, the first link between bone remodeling and energy metabolism. J Clin Endocrinol Metab (2010) 95:4795–801. doi: 10.1210/JC.2010-1030
52. Moran SA, Patten N, Young JR, Cochran E, Sebring N, Reynolds J, et al. Changes in body composition in patients with severe lipodystrophy after leptin replacement therapy. Metabolism (2004) 53:513–9. doi: 10.1016/j.metabol.2003.10.019
53. Christensen JD, Lungu AO, Cochran E, Collins MT, Gafni RI, Reynolds JC, et al. Bone mineral content in patients with congenital generalized lipodystrophy is unaffected by metreleptin replacement therapy. J Clin Endocrinol Metab (2014) 53(4):513–9. doi: 10.1210/JC.2014-1353
Keywords: lipodystrophy, CGL4, PTRF, adipose tissue, leptin, muscular dystrophy
Citation: Mancioppi V, Daffara T, Romanisio M, Ceccarini G, Pelosini C, Santini F, Bellone S, Mellone S, Baricich A, Rabbone I, Aimaretti G, Akinci B, Giordano M and Prodam F (2023) A new mutation in the CAVIN1/PTRF gene in two siblings with congenital generalized lipodystrophy type 4: case reports and review of the literature. Front. Endocrinol. 14:1212729. doi: 10.3389/fendo.2023.1212729
Received: 26 April 2023; Accepted: 26 June 2023;
Published: 12 July 2023.
Edited by:
Ronald Cohen, The University of Chicago, United StatesReviewed by:
Marco Cappa, Bambino Gesù Children’s Hospital (IRCCS), ItalyGiulia Rodari, IRCCS Ca ‘Granda Foundation Maggiore Policlinico Hospital, Italy
Copyright © 2023 Mancioppi, Daffara, Romanisio, Ceccarini, Pelosini, Santini, Bellone, Mellone, Baricich, Rabbone, Aimaretti, Akinci, Giordano and Prodam. This is an open-access article distributed under the terms of the Creative Commons Attribution License (CC BY). The use, distribution or reproduction in other forums is permitted, provided the original author(s) and the copyright owner(s) are credited and that the original publication in this journal is cited, in accordance with accepted academic practice. No use, distribution or reproduction is permitted which does not comply with these terms.
*Correspondence: Flavia Prodam, ZmxhdmlhLnByb2RhbUBtZWQudW5pdXBvLml0