- 1Chengdu University of Traditional Chinese Medicine, Sichuan, China
- 2Affiliated Hospital of Chengdu University of Traditional Chinese Medicine, Sichuan, China
Diabetic retinopathy (DR) is a microvascular lesion that occurs as a complication of diabetes mellitus. Many studies reveal that retinal neurodegeneration occurs early in its pathogenesis, and abnormal retinal function can occur in patients without any signs of microvascular abnormalities. The gut microbiota is a large, diverse colony of microorganisms that colonize the human intestine. Studies indicated that the gut microbiota is involved in the pathophysiological processes of DR and plays an important role in its development. On the one hand, numerous studies demonstrated the involvement of gut microbiota in retinal neurodegeneration. On the other hand, alterations in gut bacteria in RD patients can cause or exacerbate DR. The present review aims to underline the critical relationship between gut microbiota and DR. After a brief overview of the composition, function, and essential role of the gut microbiota in ocular health, and the review explores the concept of the gut-retina axis and the conditions of the gut-retina axis crosstalk. Because gut dysbiosis has been associated with DR, the review intends to determine changes in the gut microbiome in DR, the hypothesized mechanisms linking to the gut-retina axis, and its predictive potential.
1 Introduction
Diabetic retinopathy (DR) has emerged as a leading cause of visual impairment in working-age people in various regions (1–3). According to the International Diabetes Federation (IDF), more than 500 million people worldwide will have diabetes mellitus (DM) by 2021 (4). The presence and progression of DR are associated with a significant increase in healthcare costs. Diabetes-related direct health expenditures were USD 760 billion in 2019 and are expected to rise to USD 825 billion by 2045 (5). Numerous studies demonstrated that approximately one in every three diabetic patients has DR (6). Given the high incidence of DR and the Global Burden of Disease estimate, it is critical to investigate the predictive potential for DR progression and potential therapeutic targets.
DR has been considered a microvascular complication, a known complication of DM (7–9). Studies suggest that neurodegeneration is an early event in its pathogenesis, and abnormalities in retinal function can occur in patients with no evidence of microvascular abnormalities (10, 11). The American Diabetes Association (ADA) recently defined DR as a precise neurovascular complication (12). Although studies revealed that DR is caused by chronic hyperglycemia, with retinal neovascularization, chronic inflammation, disorders of glucolipid metabolism, and immune response as its hallmarks (13–16), the exact pathogenesis remains unknown. The intestinal microbiota is a large, diverse colony of microorganisms colonizing the human intestine. The intestinal microbiota evolved symbiotically with its host and plays an important role in regulating nutrient absorption (17) and metabolism (18–20), maintaining the intestinal mucosal barrier (21), intestinal immunity and pathogen defense (22, 23). Studies indicated that the intestinal microbiota is involved in the pathophysiological processes of DR and plays an important role in DR development (24, 25).
The gut microbiota is primarily made up of bacteria. However, it also contains other commensals such as archaea, viruses, fungi, and protists (The term “microbiota” refers to consortia of microorganisms living in a specific environment, whereas “commensals” refers to microorganisms that colonize hosts without causing disease) (26). Following their functions, the intestinal microbiota can be classified as commensal, probiotic, or pernicious bacteria. The primary role of probiotics is to improve nutrient digestion and absorption, regulate lipid metabolism, and reduce the inflammatory response (27–29). Simultaneously, pernicious bacteria can activate the inflammatory response in vivo, disrupt the function of the intestinal epithelial barrier, and cause metabolic disorders (30, 31). Dysbiosis of the gut microbiota, also known as gut dysbiosis, is primarily characterized by a reduction in the diversity and abundance of bacteria and fungi, particularly those associated with dysfunction and various pathologies (32).
Moreover, dysbiosis of the gut microbiota can result in various gastrointestinal diseases and processes in distal tissues other than the intestine, such as joints, mucous membranes, and the eyes, which are common sites of invasion (33–35).In addition, new molecular biology-based techniques enable the identification and quantification of microbiota by analyzing DNA and RNA extracted from fecal samples. The studies described in the preceding sections support the notion that gut microbiota has become a hotspot for disease research.
Since Rowan (36) et al. introduced the concept of the “gut-retina axis” and demonstrated the existence of gut-retina crosstalk, the significance of gut microbes as important modulators of ocular disease have grown (37). Scientists identified that diet, probiotics, and antibiotics could influence the gut microbiota and, thus, the development of retinal disease (38). Increased intraocular pressure, glucose accumulation in vessels, and neovascularization can affect the health of the eye in poorly controlled diabetes (39). These processes are associated with microvascular complications in the eye, such as cataracts, glaucoma, and DR (40). DR, a complication of poorly controlled diabetes, can eventually lead to blindness (41). Dysbiosis of the gut microbiota is closely linked to the occurrence, development, and prognosis of DR.
On the one hand, an increasing number of studies have demonstrated that gut microbiota plays a role in retinal neurodegeneration (42, 43), in retinal inflammatory processes (44), and affect glucose metabolism, insulin resistance, and entero-insulin secretion (45). Conversely, alterations in the gut bacterial microbiome in people with RD, and thus dysbiosis of gut microbiota, can also cause or aggravate DR (46). For example, carnosine was depleted in DR patients compared to healthy controls. Carnosine is an endogenous dipeptide composed of β-alanine and L-histidine with significant antioxidant and anti-inflammatory properties (47). These findings suggest that gut microbiota mediates gut-retinal communication, which is important in DR.
The present review aims to highlight the importance of gut microbiota in DR. The critical role of gut dysbiosis in the development and progression of DR is discussed briefly. Subsequently, the concept of the gut-retina axis and the mediators and conditions that allow gut-retina crosstalk will be investigated, focusing on the mechanisms involved in regulating DR by the intestinal microbiota. Finally, diet and antibiotics strategies for treating DR via the regulated intestinal microbiota and, thus, the treatment of DR will be described.
2 Microbiota and ocular diseases
2.1 Gut microbiota and ocular diseases
Recent studies have confirmed the presence of many microorganisms, such as bacteria, viruses, and fungi, on the human body surface and within the body (48). These microorganisms are ten times higher than the body’s cells and have 100 times more genes than the body’s genome, with 1000 to 1150 bacteria colonizing the intestine (49, 50). Although many microorganisms exist in the human gut, only about 160 species belong to the advantage bacterium group (51). The human gut microbiota is primarily composed of two dominant bacterial phyla (human microorganisms are classified by phylum, order, family, genus, and species), Firmicutes and Bacteroidetes, which account for more than 90% of the entire community, and other subdominant phyla such as Proteobacteria, Aspergillus, Actinomycetes, and Clostridium (52). Different intestinal flora interacts in the intestinal micro-ecosystem, and the intestinal flora and their host have a mutually beneficial commensal relationship (53, 54). It maintains a complex dynamic balance in healthy populations that can help the body with various physiological functions, mainly limiting the colonization of pathogenic intestinal bacteria and maintaining the integrity of the intestinal epithelial barrier and immune homeostasis (55, 56). In addition, the intestinal flora decomposes and utilizes food residues to provide humans with essential vitamins, amino acids, and other nutrients through the mediation of a series of digestive enzymes. It can also metabolize harmful substances like nitrosamines and lactic acid. Therefore, intestinal microorganisms play an important role in the human micro-ecosystem. When there is gut dysbiosis, the intestinal micro-ecosystem is disrupted, resulting in chronic inflammatory responses and immune diseases in the eye (Table 1), such as fungal keratitis (61), DR (62), age-related macular degeneration (AMD) (63), and uveitis (UVT) (64). In addition, there is a link between inflammatory bowel diseases and ocular diseases; 10% of subjects with inflammatory bowel disease have ocular diseases (such as episcleritis, uveitis, and conjunctivitis) (65). In humans, patients with DR have a significantly lower proportion of Bacteroidetes and Actinobacteria than healthy individuals (45, 46).
2.2 Ocular surface microbiota in patients with DR
Several studies have used traditional microbial cultures and 16S rRNA gene sequencing to describe the commensal microbiota on the ocular surface. Under normal physiological conditions, the microbiota is relatively stable, with low diversity and abundance, while still playing an important role in maintaining ocular surface homeostasis (66, 67). However, Suwajanakorn et al. (68) used next-generation sequencing analysis to demonstrate the importance of DR and glycemic control status in influencing changes in the ocular surface microbiome. Subsequent studies identified that microbes could be transferred to the retina of type 1 diabetic mice with retinopathy through gut and plasma microbiota (69). Furthermore, the microbiota composition varies throughout the body, including the eye. Although the overall gut microbiota comprises Firmicutes and Bacteroidetes (70), the ocular surface microbiota primarily comprises Proteobacteria and Actinobacteria (71, 72). Proteobacteria, Actinobacteria, and Firmicutes account for over 87% of all microorganisms present in the eye (73). With further investigation, the doctrine that active microbiota is present in the eye has been broken. For example, the internal eye compartment is sterile, Whereas the external compartment is exposed to environmental microorganisms (74).
2.3 Prevalence of gut dysbiosis in DR patients
As a metabolic disease caused by multiple factors, the gut microbiota composition differs between T2DM patients and healthy individuals. For example, significant reductions in the proportion of Firmicutes and Clostridium appear in the microbiota of diabetic patients compared to healthy controls (75). Subsequent studies have confirmed the role of gut flora in systemic metabolism and T2DM. Qin et al. (76) and Karlsson et al. (77) performed metagenomic sequencing in Chinese and Swedish diabetic patients, respectively, demonstrating that T2DM was characterized by gut dysbiosis. Further research has linked dysbiosis of the gut flora to insulin resistance (IR) and abnormal lipid metabolism, which are important factors in the pathogenesis of T2DM (78). In addition, Bacteroidetes to Firmicutes ratio (B/F ratio) is a potential diagnostic biomarker for DM (79, 80).
Previous studies have demonstrated that the pathogenesis of T2DM is commonly associated with altered gut microbiota. However, it is unclear whether diabetic patients with or without retinopathy have different gut microbial dysbiosis. Scientists investigated this and identified that DR patients have intestinal dysbiosis similar to T2DM patients, with the main differences being a decrease in microbial diversity (81), changes in microbial composition and structure (37, 46), low levels of beneficial microflora and higher levels of pathogenic bacteria (46, 82). Huang et al. (83) found increased Bifidobacterium and Lactobacillus levels and decreased Escherichia-Shigella, Faecalibacterium, Eubacterium_hallii_group, and Clostridium genera in DM and DR patients compared to the healthy population. Furthermore, patients with DR have a different gut microbiota than those with diabetes, but little variability exists among them. Moreover, Prasad et al. examined the retinas of diabetic mice and determined that gut microbial dysbiosis aggravated retinal impairment and inflammation (69). All these studies confirm that dysbiotic gut microbiota characterized DM and DR.
3 Overview of gut-retina axis
For decades, scientists have studied the relationship between the gastrointestinal (GI) tract and the brain, and numerous studies have confirmed the existence of the brain-gut axis. The “gut-brain axis” refers to the specific linkage between the GI tract and the central nervous system (CNS), which consists of a bidirectional exchange between the two (84). The presence of the brain-gut axis suggests that CNS regulates and governed gut metabolic activity, and there is growing evidence that not only the brain (CNS) can influence GI tract function, but the gut flora can also influence the development of CNS diseases (85, 86). For example, several studies demonstrated that amyloid deposits and neuronal fiber tangle deposits in the enteric nervous system (ENS) of patients with Alzheimer’s disease are similar to those found in the brain parenchyma (87, 88). Lewy vesicles, which appear in the brain of patients with Parkinson’s disease, have also been identified in enteric neurons (89).
The possibility of an interconnection between the eye and CNS has long been debated because the retina is an extension of the brain (90). The retina is the light-sensitive neural tissue that lines the back of the eye. In anatomical and developmental terms, the retina is a brain extension known as the ‘peripheral brain.’ Both organs consist of neurons derived from a neural tube with a multilayered cellular structure and synaptic connections. Moreover, the retina transmits information to the brain’s visual cortex via the optic nerve, which converts optical signals into nerve impulses. In addition, the retina has the advantages of clear structural stratification, visualization, ease of observation, and relative ease of functional testing compared to the brain, making it an ideal model for observing and studying neurological diseases (91).
Many features of neurodegenerative processes in the CNS are similar to those observed in the retina, and some CNS neurodegenerative diseases can affect the retina and vice versa (92). Retinal lesions, such as ganglion cell layer thinning, can occur early in Alzheimer’s disease (93). Retinal chronic progressive neurodegeneration, which can happen in the elderly, can result in eye disorders like glaucoma, AMD, and DR (94). Therefore, scholars have questioned whether the concept of a brain-gut axis applies to the retina independently of the brain, that is, whether the gut-retina axis can be distinguished from the gut-brain axis (64).
Subsequent studies have confirmed the existence of the gut-retina axis and demonstrated that dysregulation of the gut microbiota contributes to the development of ocular diseases (95–97). Moreover, the concept of “gut-retina axis” was formally proposed (98, 99), demonstrating that the gut-retina axis is closely related to ocular immune system homeostasis and plays an important role in various ocular diseases, such as AMD (36, 63), UVT (100), and glaucoma (101). The intestinal-ocular axis has emerged as a new area of basic and clinical research in ophthalmology. However, more in-depth research is needed to confirm and support the existence of the gut-retinal axis.
4 How the intestinal microbiota achieves mutual communication between the gut-retina
The gut-retina axis is an emerging concept that describes a strong interaction between the gut host-microbiota interface and the retina. Because the retina is immune-privileged, a critical question is how this gut-retina crosstalk can be validated. The retina is a ten-layer complex composed of numerous cells, including glial cells (Müller cells, astrocytes, and microglia), retinal microvascular endothelial cells (RMECs), retinal pigment epithelium (RPE), and all types of neurons (102, 103). RPEs, RMECs, and tight junctures form the outer and inner blood-retinal barrier (BRB). The integrity of the BRB is crucial for the function of various cells within the retina because it prevents the entry of peripheral pathogens, pathogen-associated molecular patterns (PAMPs), and leukocytes, rendering the retina an immune-privileged tissue (104). Therefore, scientists have conducted numerous studies that have revealed that the crosstalk between the gut and retina is primarily achieved through the following pathways.
The interaction between microbes, gut-derived products, and the retina can be explained by the disruption of the BRB, which is common in retinal diseases (105, 106). The GI epithelium serves as a broad interface with the external environment. Single epithelial cells, also called intestinal cells, are tightly connected and cover the inner surface of our intestinal mucosa. These cells provide a barrier by using transcellular and paracellular transport mechanisms to selectively regulate the exchanges of luminal toxins, antigens, nutrients, and water absorption between the inner and outer environments (107). Conversely, the GI epithelial barrier must maintain rapid cell renewal and barrier integrity while being exposed to continuous environmental assaults. Dysbiosis of the intestinal microbiota and inflammatory response in the presence of specific eye diseases (such as DR and UVT) can lead to intestinal barrier impairment, which increases permeability (43). Consequently, impaired gut barrier function leads to the excessive translocation of gut-derived products (such as LTA, PGN, and LPS) and even live gut bacteria into the bloodstream (108). A recent study found microbiota in the intraocular environment of healthy populations and patients with ocular diseases, breaching the dogma that the intraocular environment is sterile (109).
Short-chain fatty acids (SCFAs) are beneficial microbial metabolites produced only in the gut. Chen et al. (110) demonstrate that SCFAs can cross the BRB via systemic circulation and reach the retina, triggering an innate immune response. Consistent with this, data indicate that increased intestinal permeability caused by altered gut microbiota may allow for more significant translocation of gut metabolites and products, which may modulate retina-specific immune cells (111). In addition, studies have confirmed that SCFAs entering the systemic circulation are transported via the monocarboxylate transporter (MCT-1) across the blood-brain barrier and function in the CNS (112). SCFAs may be able to enter the retina and exert regulatory effects because MCT-1 is also present in BRB (113). All these studies suggest the presence of intraocular crosstalk.
5 Mechanism of gut-retina axis regulation in DR
The studies described in the preceding sections support a causative role of microbiota in triggering DR, but the specific mechanisms involved remain elusive. Gut dysbiosis has been associated with DM and DR. The gut-retina axis could be a potential target for preventing DR, a well-known complication of DM. A critical question now is how the gut microbiota influences the development and treatment of DR through the gut-retina axis. We reviewed the literature and identified that the hypothesized mechanisms relating to the gut-retina axis include disruption of intestinal barrier function, activation of the stimulator of interferon genes (STING) signaling pathway, production of lipopolysaccharide (LPS), angiotensin-converting enzyme 2 (ACE2) deficiency, and affecting gut microbiota metabolites (Figure 1).
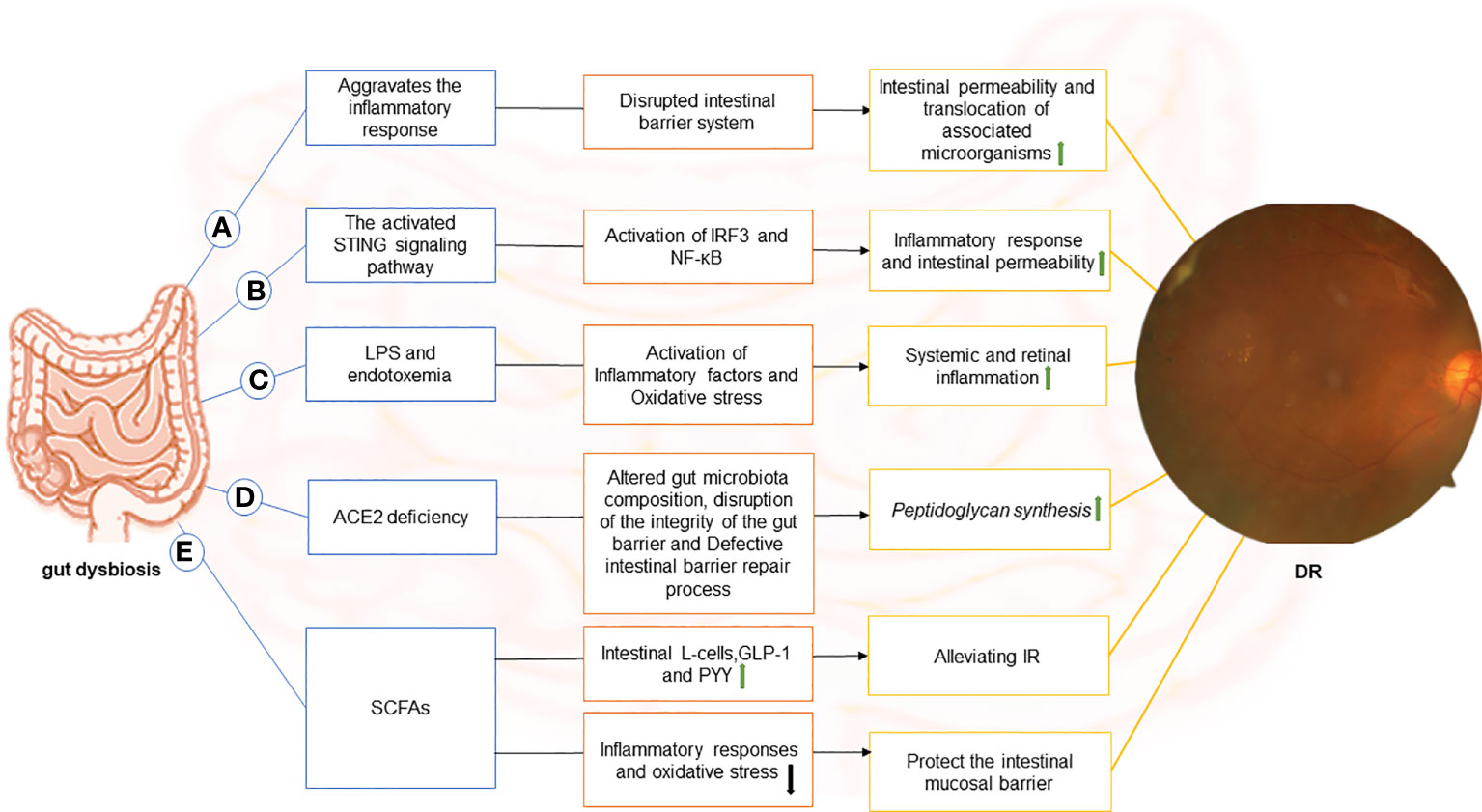
Figure 1 The image depicts the various possible mechanisms that connect the gut to the retina in DR and the components of each hypothetical gut-retina axis. (A) dysbiosis of the gut microbiota in diabetic patients causes local (including gut) and systemic inflammation. Subsequently, with inflammation, the intestinal barrier system is compromised. In this context, intestinal permeability increases, and associated microorganisms translocate. (B) stimulator of interferon genes (STING) pathway-mediated inflammatory signaling is activated, leading to activation of interferon regulatory factor 3 (IRF3) and nuclear factor-κB (NF-κB), which exacerbates intestinal barrier disruption. In this case, gut microbial products translocate into the blood and reach the retina through the damaged blood-retinal barrier, causing chronic activation of the STING pathway in the retina and contributing to the progression of DR disease. (C) endotoxemia can result from the release of lipopolysaccharide (LPS) in the gut and its entry into the blood. LPS can diffuse directly into the circulatory system and promote the release of inflammatory factors through receptor-ligand binding (primarily to CD14 and TLR4) in DM due to increased intestinal permeability or absorption via enterocytes. Moreover, binding to TLR4 increases oxidative stress, leading to systemic and retinal inflammation.(D) Angiotensin-converting enzyme 2 (ACE2) deficiency alters the gut microbiome composition in diabetic mice, disrupts intestinal barrier integrity, and results in intestinal barrier repair defects. The disruption of the intestinal vascular barrier causes peptidoglycan synthesis in mice, which enters the plasma and promotes DR. (E)Short-chain fatty acids (SCFAs) inhibit inflammatory responses and oxidative stress, suppress endotoxin-induced inflammation, and protect the intestinal mucosal barrier. It also stimulates intestinal L-cells and promotes the secretion of glucagon-like peptide-1 (GLP-1) and endocrine-regulating peptide (PYY) to alleviate IR. .
5.1 Affecting intestinal barrier system function
The intestinal mechanical and biological barriers are formed by the hierarchical and regular distribution of intrinsic intestinal bacteria in the intestinal epithelial cells, mucus on the mucosal surface, and the tight connection between cells. Both contribute to the human intestinal barrier system, which protects the organism from harmful or foreign pathogenic bacteria. The intestinal biological barrier primarily consists of Bifidobacterium and other bacteria found in the deep layer and Peptostreptococcus in the intermediate layer, which accounts for more than 99% of intestinal bacteria. These intrinsic intestinal bacteria act as a biological barrier by pre-empting colonization sites, competing for nutrients, producing organic acids and SCFAs to lower intestinal pH, producing bacterins, and inducing a moderate inflammatory response (114).
Gut microbiota is being extensively investigated for its role in DM and its complications. Changes in the gut-microbiome cause pathological inflammation and accelerate DR progression. Consequently, it influences the immune system and homeostasis locally (within the gut) and systemically (115). In this context, increased intestinal permeability and associated microbial translocation are important in the pathogenesis of DR (116). Furthermore, this contributes to the chronic systemic inflammatory process and further disrupts the intestinal barrier system. However, it has been demonstrated that even in the absence of ocular infection, the eye is susceptible to inflammatory disease, which is influenced by intraocular microbiota dysbiosis (103). In contrast, the relationship between the initiating factors of intraocular microbiota dysbiosis and DM leading to inflammation requires further investigation.
5.2 Stimulator of interferon genes signaling pathway-mediated inflammation
In various inflammatory diseases, aberrant regulation of the STING pathway has emerged as a critical pathogenic mechanism (117). STING is an endoplasmic reticulum (ER) adaptor protein commonly expressed in the ER. STING activation by the cytoplasmic DNA sensor cycle GMP-AMP synthase (cGAS) causes the activation of the nuclear factor-κB (NF-κB) and the transcription factor interferon regulatory factor 3 (IRF3) (118). A positive feedback loop between dysbiosis and abnormal activation of the STING pathway in the intestine is associated with increased intestinal permeability (119). There is a possibility that dysbiosis in DR patients disrupts intestinal homeostasis and aggravates barrier dysfunction through the erroneous accumulation of STING in the gut. Subsequent translocation of microbial products into the blood allows access to the retina via the impaired BRB, resulting in chronic activation of the STING pathway in the retina, contributing to disease progression (119). In addition, the STING pathway has been linked to changes in the retina and retinal cells of patients with ocular diseases (120).
5.3 LPS
LPS, a gut microbial-derived product composed of lipid A-based glycolipid found on the outer membrane of gram-negative bacteria, is thought to be a pro-inflammatory mediator of insulin resistance. It is challenging to shed from the outer membrane of gram-negative bacteria in healthy populations, but it becomes detached and toxic when bacteria are lysed or damaged (121). LPS is released into the gut, and when it enters the blood, it causes LPS-related toxicity, known as endotoxemia (122). LPS can enter the circulatory system by direct diffusion due to increased intestinal permeability in DR or by absorption through enterocytes. LPS is transported in the blood by lipopolysaccharide-binding proteins, binding CD14 and Toll-Like Receptor 4(TLR4) in peripheral tissues such as skeletal muscle and adipose, causing macrophage aggregation in adipose tissue, promoting the release of inflammatory factors, inducing abnormal phosphorylation of IRS-1, and leading to IR (123). When it binds to TLR4, it activates NF-κB and increases oxidative stress, leading to systemic and retinal inflammation (123).
LPS causes endotoxemia and promotes inflammation, whereas other microorganisms produce protective effects. For example, Lactobacillus, Bacteroides, Faecalibacterium, Akkermansia muciniphila (A. muciniphila), and Roseburia are known to down-regulate the pro-inflammatory cytokines in the intestine. Bacteroides and A. muciniphila improve intestinal barrier function. Bacteroides reduce intestinal permeability, decrease LPS production, and improve endotoxemia by up-regulating the colonic tight junction gene expression (122). A. muciniphila reduces intestinal permeability by regulating extracellular vesicles, which improves intestinal tight junctions by activating AMP-activated protein kinase in intestinal epithelial cells, thereby enhancing intestinal defence (124). In addition, the outer membrane protein of A. muciniphila up-regulates tight junction protein expression and inhibits CB-1, improving intestinal integrity and reducing LPS levels (125).
5.4 The gut-retina axis regulates DR via angiotensin-converting enzyme 2 and peptidoglycan
Takkar et al. (126) reported that ACE2 and peptidogly can play an important role in regulating the pathogenesis of DR. In type 1 diabetic mice, ACE2 deficiency alters gut microbiome composition and gut integrity, as well as defects in the gut barrier repair process (127). Disruption of the intestinal vascular barrier and increased growth of Bifidobacterium animalis contributes to peptidoglycan synthesis. Consequently, bacterial peptidoglycan enters the bloodstream and promotes DR (128). ACE2 regulates bone marrow-derived myeloid angiogenesis, restoring intestinal epithelial and endothelial functions disrupted in DM (129, 130), and alters peptidoglycan biosynthesis by reducing microbiome-associated genes (128).
5.5 Affecting metabolites of gut microbiota
SCFAs, composed of acetate, propionate, and butyrate, are small organic metabolites produced by the fermentation of dietary fibers and resistant starch. They have numerous benefits in energy metabolism, intestinal homeostasis, and immune response regulation. It can inhibit the inflammatory response and oxidative stress and affect glycolipid metabolism as a signaling molecule between intestinal flora and the host (110). Glucolipid metabolism disorders and insulin resistance are characteristic manifestations of DR. SCFAs primarily influence glucose and lipid metabolism by regulating the endocrine system. It (especially acetate and butyrate) can specifically stimulate intestinal L-cells and promote the secretion of glucagon-like peptide-1 (GLP-1) and endocrine-regulated peptide (PYY). In obese mice, this improves insulin sensitivity and increases energy expenditure, preventing and treating diet-induced IR (131, 132). In addition, SCFAs reduce IR by inhibiting endotoxin-related inflammation and protecting the intestinal mucosal barrier (133).
6 Regulating gut microbiota as a therapeutic strategy for DR treatment
The external environment gradually shapes the diversity of the human intestinal microbiota. Before birth, the fetus is sterile in the intestine and progressively accumulates a specific intestinal flora through exposure to the surrounding environment (134). Childhood and adolescence are critical periods for forming intestinal flora, and individual-specific intestinal flora is formed in adulthood (135). Although the intestinal flora is relatively stable in adulthood, it is also modifiable, with its composition changing with age, diet, lifestyle, and environmental exposure (134). When the gut microbiota in patients with DM and DR is dysregulated, dietary modifications (e.g., probiotic/prebiotic supplementation and low-sugar diet) and fecal transplantation can maintain intestinal homeostasis and improve the condition (136). Moreover, studies on new technologies, such as gut flora editing and synthesis of the gut microbiome to regulate and synthesize gut flora, have been reported (137), providing ideas for using gut flora in treating DR.
Genetic factors have a limited impact on the composition of the host gut microbiota. For example, diets can influence gut microbes in healthy individuals. A high-fat diet is associated with an increased abundance of Bacteroides, whereas a high fiber intake is associated with an increased abundance of Prevotella (138). The diet also influences the production of intestinal flora metabolites, such as SCFAs, LPS, bile acids, and branched-chain amino acids (BCAA; valine, leucine, and isoleucine) (122). Beli et al. (42) reported that intermittent fasting (IF) can reduce retinal complications (DR) in diabetic mice. In particular, IF can reduce intestinal permeability and promote the production of tauroursodeoxycholic acid (TUDCA), a potent activator of Takeda-G-protein-receptor-5 (TGR5) in the retinal ganglion cell layer and can act as a neuroprotective agent. IF also improves intestinal vascular barrier function and lowers plasma peptidoglycan levels. Peptidogly can activates TLR2-mediated signaling cascades and exacerbates DR by interfering with the integrity of retinal endothelial cell junctions (42). In conclusion, these findings reveal that remodeling the intestinal microbiome has a protective effect on the retina, preventing the development of DR. In addition, it has been suggested that the potential mechanism by which DR does not occur in diabetic patients is closely related to intestinal microbiota imbalance, which varies between individuals (139).
7 Conclusion and future perspectives
The gut-retina axis concept was developed in response to the dysregulation of gut microbiota observed in patients with retinal diseases such as AMD, DR, and glaucoma. Researchers used antibiotics, probiotics, and diet to reshape the gut microbiota, and the results improved eye disease, providing the link between the gut microbiota and the retina. Subsequent studies revealed further crosstalk between the eye and the gut.
The specificity of the abundance and function of microorganisms and their metabolites in retinal diseases is slowly being elucidated. Scientists have made several advances in the enumeration, characterization, and classification of the human microbiota since the advent of high-throughput sequencing and culture group technologies (134). The most commonly used method for determining microbiome composition was 16S rRNA gene sequencing, which had many limitations for strain-level identification and classification of microorganisms (140). The integrated application of multi-omics, such as macro-genomics, macro-proteomics, and macro-metabolomics, can provide a more accurate and direct interpretation of the functional properties of the intestinal flora for a more accurate understanding of the human micro-ecosystem (141). However, due to individual heterogeneity and the limitations of current diagnostic techniques, interventions on the gut microbiota for disease treatment still be carefully considered. Meanwhile, studies on gut microecology and DR have been reported infrequently compared to other disciplines, and more high-quality studies are required to support this in the future.
The link between microbiota and DR is now well established, and identifying pathogenic or protective microbes is an important step to follow in future. In conclusion, the concept of a gut-retina axis driven by various pathways is being actively investigated, and available data in animals and humans suggest possible therapeutic applications for disease through targeted manipulation of the microbiome.
Author contributions
HZ reviewed the literature and drafted this review. YM reviewed the literature, gave critical comments, and revised the manuscript. All authors contributed to the article and approved the submitted version.
Funding
The paper was supported by the National Natural Science Foundation of China(grant number 82274586); Sichuan Science and Technology Plan Project(grant number 2021YJ0252); Chengdu University of Traditional Chinese Medicine “Xinglin Scholars” Program(grant number XKTD2022005; grant number KPZX2022008).
Acknowledgments
The contributions of specific colleagues, institutions, or agencies that assisted the authors are gratefully acknowledged.
Conflict of interest
The authors declare that the research was conducted in the absence of any commercial or financial relationships that could be construed as a potential conflict of interest.
Publisher’s note
All claims expressed in this article are solely those of the authors and do not necessarily represent those of their affiliated organizations, or those of the publisher, the editors and the reviewers. Any product that may be evaluated in this article, or claim that may be made by its manufacturer, is not guaranteed or endorsed by the publisher.
References
1. Ipp E, Liljenquist D, Bode B, Shah VN, Silverstein S, Regillo CD, et al. Pivotal evaluation of an artificial intelligence system for autonomous detection of referrable and vision-threatening diabetic retinopathy. JAMA Netw Open (2021) 4:e2134254. doi: 10.1001/jamanetworkopen.2021.34254
2. Frudd K, Sivaprasad S, Raman R, Krishnakumar S, Revathy YR, Turowski P. Diagnostic circulating biomarkers to detect vision-threatening diabetic retinopathy: potential screening tool of the future? Acta Ophthalmol (2022) 100:e648–68. doi: 10.1111/aos.14954
3. Wong TY, Cheung CMG, Larsen M, Sharma S, Simó R. Diabetic retinopathy. Nat Rev Dis Primers (2016) 2:16012. doi: 10.1038/nrdp.2016.12
4. Sun H, Saeedi P, Karuranga S, Pinkepank M, Ogurtsova K, Duncan BB, et al. IDF diabetes atlas: global, regional and country-level diabetes prevalence estimates for 2021 and projections for 2045. Diabetes Res Clin Pract (2022) 183:109119. doi: 10.1016/j.diabres.2021.109119
5. Williams R, Karuranga S, Malanda B, Saeedi P, Basit A, Besançon S, et al. Global and regional estimates and projections of diabetes-related health expenditure: results from the international diabetes federation diabetes atlas, 9th edition. Diabetes Res Clin Pract (2020) 162:108072. doi: 10.1016/j.diabres.2020.108072
6. Tan GS, Cheung N, Simó R, Cheung GCM, Wong TY. Diabetic macular oedema. Lancet Diabetes Endocrinol (2017) 5:143–55. doi: 10.1016/S2213-8587(16)30052-3
7. Hammes HP. Diabetic retinopathy: hyperglycaemia, oxidative stress and beyond. Diabetologia (2018) 61:29–38. doi: 10.1007/s00125-017-4435-8
8. Rao H, Jalali JA, Johnston TP, Koulen P. Emerging roles of dyslipidemia and hyperglycemia in diabetic retinopathy: molecular mechanisms and clinical perspectives. Front Endocrinol (Lausanne) (2021) 12:620045. doi: 10.3389/fendo.2021.620045
9. Feng Y, Wang Y, Yang Z, Wu L, Hoffmann S, Wieland T, et al. Chronic hyperglycemia inhibits vasoregression in a transgenic model of retinal degeneration. Acta diabetologica (2014) 51(2):211–8. doi: 10.1007/s00592-013-0488-4
10. Soni D, Sagar P, Takkar B. Diabetic retinal neurodegeneration as a form of diabetic retinopathy. Int Ophthalmol (2021) 41:3223–48. doi: 10.1007/s10792-021-01864-4
11. Sachdeva MM. Retinal neurodegeneration in diabetes: an emerging concept in diabetic retinopathy. Curr Diabetes Rep (2021) 21:65. doi: 10.1007/s11892-021-01428-x
12. Simó-Servat O, Hernández C, Simó R. Diabetic retinopathy in the context of patients with diabetes. ORE (2019) 62:211–7. doi: 10.1159/000499541
13. Youngblood H, Robinson R, Sharma A, Sharma S. Proteomic biomarkers of retinal inflammation in diabetic retinopathy. Int J Mol Sci (2019) 20:4755. doi: 10.3390/ijms20194755
14. Tang L, Xu GT, Zhang JF. Inflammation in diabetic retinopathy: possible roles in pathogenesis and potential implications for therapy. Neural Regener Res (2023) 18:976–82. doi: 10.4103/1673-5374.355743
15. Pelikánová T. Diabetic retinopathy: pathogenesis and therapeutic implications. Vnitr Lek (2016) 62:620–8.
16. Kowluru RA, Mishra M. Regulation of matrix metalloproteinase in the pathogenesis of diabetic retinopathy. Prog Mol Biol Transl Sci (2017) 148:67–85. doi: 10.1016/bs.pmbts.2017.02.004
17. Domingo JJS, Sánchez SC. From the intestinal flora to the microbiome. Rev Esp Enferm Dig (2018) 110:51–6. doi: 10.17235/reed.2017.4947/2017
18. Wang Q, Hao C, Yao W, Zhu D, Lu H, Li L, et al. Intestinal flora imbalance affects bile acid metabolism and is associated with gallstone formation. BMC Gastroenterol (2020) 20:59. doi: 10.1186/s12876-020-01195-1
19. Ramírez-Pérez O, Cruz-Ramón V, Chinchilla-López P, Méndez-Sánchez N. The role of the gut microbiota in bile acid metabolism. Ann Hepatol (2017) 16:s15–20. doi: 10.5604/01.3001.0010.5494
20. Węgielska I, Suliburska J. The role of intestinal microbiota in the pathogenesis of metabolic diseases. Acta Sci Pol Technol Aliment (2016) 15:201–11. doi: 10.17306/J.AFS.2016.2.20
21. Li XY, He C, Zhu Y, Lu NH. Role of gut microbiota on intestinal barrier function in acute pancreatitis. World J Gastroenterol (2020) 26:2187–93. doi: 10.3748/wjg.v26.i18.2187
22. Zhou B, Yuan Y, Zhang S, Guo C, Li X, Li G, et al. Intestinal flora and disease mutually shape the regional immune system in the intestinal tract. Front Immunol (2020) 11:575. doi: 10.3389/fimmu.2020.00575
23. Wang C, Li Q, Ren J. Microbiota-immune interaction in the pathogenesis of gut-derived infection. Front Immunol (2019) 10:1873. doi: 10.3389/fimmu.2019.01873
24. Ding Y, Zhao J, Liu G, Li Y, Jiang J, Meng Y, et al. Total bilirubin predicts severe progression of diabetic retinopathy and the possible causal mechanism. J Diabetes Res (2020) 2020:7219852. doi: 10.1155/2020/7219852
25. Xue L, Huang L, Tian Y, Cao X, Song Y. Trimethylamine-N-Oxide promotes high-Glucose-Induced dysfunction and NLRP3 inflammasome activation in retinal microvascular endothelial cells. J Ophthalmol (2023) 2023:8224752. doi: 10.1155/2023/8224752
26. Matijašić M, Meštrović T, Paljetak HČ, Perić M, Barešić A, Verbanac D. Gut microbiota beyond bacteria-mycobiome, virome, archaeome, and eukaryotic parasites in IBD. Int J Mol Sci (2020) 21:2668. doi: 10.3390/ijms21082668
27. Baroja LM, Kirjavainen PV, Hekmat S, Reid G. Anti-inflammatory effects of probiotic yogurt in inflammatory bowel disease patients. Clin Exp Immunol (2007) 149:470–9. doi: 10.1111/j.1365-2249.2007.03434.x
28. Keshteli AH, Valcheva R, Nickurak C, Park H, Mandal R, van Diepen K, et al. Anti-inflammatory diet prevents subclinical colonic inflammation and alters metabolomic profile of ulcerative colitis patients in clinical remission. Nutrients (2022) 14:3294. doi: 10.3390/nu14163294
29. Li KL, Wang BZ, Li ZP, Li YL, Liang JJ. Alterations of intestinal flora and the effects of probiotics in children with recurrent respiratory tract infection. World J Pediatr (2019) 15:255–61. doi: 10.1007/s12519-019-00248-0
30. Quaglio AEV, Grillo TG, De-Oliveira ECS, Di-Stasi LC, Sassaki LY. Gut microbiota, inflammatory bowel disease and colorectal cancer. World J Gastroenterol (2022) 28:4053–60. doi: 10.3748/wjg.v28.i30.4053
31. Nishida A, Inoue R, Inatomi O, Bamba S, Naito Y, Andoh A. Gut microbiota in the pathogenesis of inflammatory bowel disease. Clin J Gastroenterol (2018) 11:1–10. doi: 10.1007/s12328-017-0813-5
32. Verhaar BJH, Prodan A, Nieuwdorp M, Muller M. Gut microbiota in hypertension and atherosclerosis: a review. Nutrients (2020) 12:2982. doi: 10.3390/nu12102982
33. Boyajian JL, Ghebretatios M, Schaly S, Islam P, Prakash S. Microbiome and human aging: probiotic and prebiotic potentials in longevity, skin health and cellular senescence. Nutrients (2021) 13:4550. doi: 10.3390/nu13124550
34. Myers B, Brownstone N, Reddy V, Chan S, Thibodeaux Q, Truong A, et al. The gut microbiome in psoriasis and psoriatic arthritis. Best Pract Res Clin Rheumatol (2019) 33:101494. doi: 10.1016/j.berh.2020.101494
35. He J, Chu Y, Li J, Meng Q, Liu Y, Jin J, et al. Intestinal butyrate-metabolizing species contribute to autoantibody production and bone erosion in rheumatoid arthritis. Sci Adv (2022) 8:eabm1511. doi: 10.1126/sciadv.abm1511
36. Rowan S, Jiang S, Korem T, Szymanski J, Chang ML, Szelog J, et al. Involvement of a gut-retina axis in protection against dietary glycemia-induced age-related macular degeneration. Proc Natl Acad Sci U.S.A. (2017) 114:E4472–81. doi: 10.1073/pnas.1702302114
37. Jayasudha R, Das T, Kalyana CS, Sai PG, Bhargava A, Tyagi M, et al. Gut mycobiomes are altered in people with type 2 diabetes mellitus and diabetic retinopathy. PloS One (2020) 15:e0243077. doi: 10.1371/journal.pone.0243077
38. Bringer MA, Gabrielle PH, Bron AM, Creuzot-Garcher C, Acar N. The gut microbiota in retinal diseases. Exp Eye Res (2022) 214:108867. doi: 10.1016/j.exer.2021.108867
39. Gourgari E, Dabelea D, Rother K. Modifiable risk factors for cardiovascular disease in children with type 1 diabetes: can early intervention prevent future cardiovascular events? Curr Diabetes Rep (2017) 17:134. doi: 10.1007/s11892-017-0968-y
40. Papatheodorou K, Papanas N, Banach M, Papazoglou D, Edmonds M. Complications of diabetes 2016. J Diabetes Res (2016) 2016:6989453. doi: 10.1155/2016/6989453
41. Cheung N, Mitchell P, Wong TY. Diabetic retinopathy. Lancet (2010) 376:124–36. doi: 10.1016/S0140-6736(09)62124-3
42. Dong L, Zhang Z, Liu X, Wang Q, Hong Y, Li X, et al. RNA Sequencing reveals BMP4 as a basis for the dual-target treatment of diabetic retinopathy. J Mol Med (Berl) (2021) 99:225–40. doi: 10.1007/s00109-020-01995-8
43. Beli E, Yan Y, Moldovan L, Vieira CP, Gao R, Duan Y, et al. Restructuring of the gut microbiome by intermittent fasting prevents retinopathy and prolongs survival in db/db mice. Diabetes (2018) 67:1867–79. doi: 10.2337/db18-0158
44. Yang G, Wei J, Liu P, Zhang Q, Tian Y, Hou G, et al. Role of the gut microbiota in type 2 diabetes and related diseases. Metabolism (2021) 117:154712. doi: 10.1016/j.metabol.2021.154712
45. Iatcu CO, Steen A, Covasa M. Gut microbiota and complications of type-2 diabetes. Nutrients (2021) 14:166. doi: 10.3390/nu14010166
46. Das T, Jayasudha R, Chakravarthy S, Prashanthi GS, Bhargava A, Tyagi M, et al. Alterations in the gut bacterial microbiome in people with type 2 diabetes mellitus and diabetic retinopathy. Sci Rep (2021) 11:2738. doi: 10.1038/s41598-021-82538-0
47. Caruso G, Fresta CG, Fidilio A, O’Donnell F, Musso N, Lazzarino G, et al. Carnosine decreases PMA-induced oxidative stress and inflammation in murine macrophages. Antioxidants (Basel) (2019) 8:281. doi: 10.3390/antiox8080281
48. Qin J, Li R, Raes J, Arumugam M, Burgdorf KS, Manichanh C, et al. A human gut microbial gene catalogue established by metagenomic sequencing. Nature (2010) 464:59–65. doi: 10.1038/nature08821
49. de-Oliveira GLV, Leite AZ, Higuchi BS, Gonzaga MI, Mariano VS. Intestinal dysbiosis and probiotic applications in autoimmune diseases. Immunology (2017) 152:1–12. doi: 10.1111/imm.12765
50. Li J, Jia H, Cai X, Zhong H, Feng Q, Sunagawa S, et al. An integrated catalog of reference genes in the human gut microbiome. Nat Biotechnol (2014) 32:834–41. doi: 10.1038/nbt.2942
51. Zhu J, Ren H, Zhong H, Li X, Zou Y, Han M, et al. An expanded gene catalog of mouse gut metagenomes. mSphere (2021) 6:e01119–20. doi: 10.1128/mSphere.01119-20
52. Magne F, Gotteland M, Gauthier L, Zazueta A, Pesoa S, Navarrete P, et al. The Firmicutes/Bacteroidetes ratio: a relevant marker of gut dysbiosis in obese patients? Nutrients (2020) 12:1474. doi: 10.3390/nu12051474
53. Clemente JC, Ursell LK, Parfrey LW, Knight R. The impact of the gut microbiota on human health: an integrative view. Cell (2012) 148:1258–70. doi: 10.1016/j.cell.2012.01.035
54. Ley RE, Peterson DA, Gordon JI. Ecological and evolutionary forces shaping microbial diversity in the human intestine. Cell (2006) 124:837–48. doi: 10.1016/j.cell.2006.02.017
55. De-Luca F, Shoenfeld Y. The microbiome in autoimmune diseases. Clin Exp Immunol (2019) 195:74–85. doi: 10.1111/cei.13158
56. Shi N, Li N, Duan X, Niu H. Interaction between the gut microbiome and mucosal immune system. Mil Med Res (2017) 4:14. doi: 10.1186/s40779-017-0122-9
57. Kalyana CS, Jayasudha R, Sai PG, Ali MH, Sharma S, Tyagi M, et al. Dysbiosis in the gut bacterial microbiome of patients with uveitis, an inflammatory disease of the eye. Indian J Microbiol (2018) 58:457–69. doi: 10.1007/s12088-018-0746-9
58. Goodman CF, Doan T, Mehra D, Betz J, Locatelli E, Mangwani-Mordani S, et al. Case-control study examining the composition of the gut microbiome in individuals with and without immune-mediated dry eye. Cornea (2022) 13:10. doi: 10.1097/ICO.0000000000003195
59. Zinkernagel MS, Zysset-Burri DC, Keller I, Berger LE, Leichtle AB, Largiadèr CR, et al. Association of the intestinal microbiome with the development of neovascular age-related macular degeneration. Sci Rep (2017) 7:40826. doi: 10.1038/srep40826
60. Kalyana CS, Jayasudha R, Ranjith K, Dutta A, Pinna NK, Mande SS, et al. Alterations in the gut bacterial microbiome in fungal keratitis patients. PloS One (2018) 13:e0199640. doi: 10.1371/journal.pone.0199640
61. Jayasudha R, Chakravarthy SK, Prashanthi GS, Sharma S, Garg P, Murthy SI, et al. Alterations in gut bacterial and fungal microbiomes are associated with bacterial keratitis, an inflammatory disease of the human eye. J Biosci (2018) 43:835–56. doi: 10.1007/s12038-018-9798-6
62. Jabbehdari S, Sallam AB. Gut microbiome and diabetic retinopathy. Eur J Ophthalmol (2022) 32:2494–7. doi: 10.1177/11206721221083068
63. Rinninella E, Mele MC, Merendino N, Cintoni M, Anselmi G, Caporossi A, et al. The role of diet, micronutrients and the gut microbiota in age-related macular degeneration: new perspectives from the gut–Retina axis. Nutrients (2018) 10:1677. doi: 10.3390/nu10111677
64. Horai R, Caspi RR. Microbiome and autoimmune uveitis. Front Immunol (2019) 10:232. doi: 10.3389/fimmu.2019.00232
65. Rogler G, Singh A, Kavanaugh A, Rubin DT. Extraintestinal manifestations of inflammatory bowel disease: current concepts, treatment, and implications for disease management. Gastroenterology (2021) 161:1118–32. doi: 10.1053/j.gastro.2021.07.042
66. Willcox MD. Characterization of the normal microbiota of the ocular surface. Exp Eye Res (2013) 117:99–105. doi: 10.1016/j.exer.2013.06.003
67. Deng Y, Wen X, Hu X, Zou Y, Zhao C, Chen X, et al. Geographic difference shaped human ocular surface metagenome of young han Chinese from Beijing, wenzhou, and guangzhou cities. Invest Ophthalmol Vis Sci (2020) 61:47. doi: 10.1167/iovs.61.2.47
68. Suwajanakorn O, Puangsricharern V, Kittipibul T, Chatsuwan T. Ocular surface microbiome in diabetes mellitus. Sci Rep (2022) 12:21527. doi: 10.1038/s41598-022-25722-0
69. Prasad R, Asare-Bediko B, Harbour A, Floyd JL, Chakraborty D, Duan Y, et al. Microbial signatures in the rodent eyes with retinal dysfunction and diabetic retinopathy. Invest Ophthalmol Vis Sci (2022) 63:5. doi: 10.1167/iovs.63.1.5
70. Damms-Machado A, Mitra S, Schollenberger AE, Kramer KM, Meile T, Königsrainer A, et al. Effects of surgical and dietary weight loss therapy for obesity on gut microbiota composition and nutrient absorption. BioMed Res Int (2015) 2015:806248. doi: 10.1155/2015/806248
71. Huang Y, Yang B, Li W. Defining the normal core microbiome of conjunctival microbial communities. Clin Microbiol Infect (2016) 22:643.e7–643.e12. doi: 10.1016/j.cmi.2016.04.008
72. Ozkan J, Willcox M, Wemheuer B, Wilcsek G, Coroneo M, Thomas T. Biogeography of the human ocular microbiota. Ocul Surf (2019) 17:111–8. doi: 10.1016/j.jtos.2018.11.005
74. Caspi RR. In this issue: immunology of the eye-inside and out. Int Rev Immunol (2013) 32:1–3. doi: 10.3109/08830185.2012.750138
75. Hoang HT, Le DH, Le TTH, Nguyen TTN, Chu HH, Nguyen NT. Metagenomic 16S rDNA amplicon data of microbial diversity of guts in Vietnamese humans with type 2 diabetes and nondiabetic adults. Data Brief (2021) 34:106690. doi: 10.1016/j.dib.2020.106690
76. Qin J, Li Y, Cai Z, Li S, Zhu J, Zhang F, et al. A metagenome-wide association study of gut microbiota in type 2 diabetes. Nature (2012) 490:55–60. doi: 10.1038/nature11450
77. Karlsson FH, Tremaroli V, Nookaew I, Bergström G, Behre CJ, Fagerberg B, et al. Gut metagenome in European women with normal, impaired and diabetic glucose control. Nature (2013) 498:99–103. doi: 10.1038/nature12198
78. Aron-Wisnewsky J, Warmbrunn MV, Nieuwdorp M, Clément K. Metabolism and metabolic disorders and the microbiome: the intestinal microbiota associated with obesity, lipid metabolism, and metabolic health-pathophysiology and therapeutic strategies. Gastroenterology (2021) 160:573–99. doi: 10.1053/j.gastro.2020.10.057
79. Floyd JL, Grant MB. The gut-eye axis: lessons learned from murine models. Ophthalmol Ther (2020) 9:499–513. doi: 10.1007/s40123-020-00278-2
80. Moubayed NM, Bhat RS, Al Farraj D, Dihani NA, El Ansary A, Fahmy RM. Screening and identification of gut anaerobes (Bacteroidetes) from human diabetic stool samples with and without retinopathy in comparison to control subjects. Microb Pathog (2019) 129:88–92. doi: 10.1016/j.micpath.2019.01.025
81. Bai J, Wan Z, Zhang Y, Wang T, Xue Y, Peng Q. Composition and diversity of gut microbiota in diabetic retinopathy. Front Microbiol (2022) 13:926926. doi: 10.3389/fmicb.2022.926926
82. Khan R, Sharma A, Ravikumar R, Parekh A, Srinivasan R, George RJ, et al. Association between gut microbial abundance and sight-threatening diabetic retinopathy. Invest Ophthalmol Visual Sci (2021) 62(7):19. doi: 10.1167/iovs.62.7.19
83. Huang Y, Wang Z, Ma H, Ji S, Chen Z, Cui Z, et al. Dysbiosis and implication of the gut microbiota in diabetic retinopathy. Front Cell Infect Microbiol (2021) 11:646348. doi: 10.3389/fcimb.2021.646348
84. Socała K, Doboszewska U, Szopa A, Serefko A, Włodarczyk M, Zielińska A, et al. The role of microbiota-gut-brain axis in neuropsychiatric and neurological disorders. Pharmacol Res (2021) 172:105840. doi: 10.1016/j.phrs.2021.105840
85. Hillestad EMR, van der Meeren A, Nagaraja BH, Bjørsvik BR, Haleem N, Benitez-Paez A, et al. Gut bless you: the microbiota-gut-brain axis in irritable bowel syndrome. World J Gastroenterol (2022) 28:412–31. doi: 10.3748/wjg.v28.i4.412
86. Ancona A, Petito C, Iavarone I, Petito V, Galasso L, Leonetti A, et al. The gut-brain axis in irritable bowel syndrome and inflammatory bowel disease. Dig Liver Dis (2021) 53:298–305. doi: 10.1016/j.dld.2020.11.026
87. Hill JM, Lukiw WJ. Microbial-generated amyloids and alzheimer's disease (AD). Front Aging Neurosci (2015) 7:9. doi: 10.3389/fnagi.2015.00009
88. Zhao Y, Lukiw WJ. Microbiome-generated amyloid and potential impact on amyloidogenesis in alzheimer's disease (AD). J Nat Sci (2015) 1:e138.
89. Liu M, Geddis MS, Wen Y, Setlik W, Gershon MD. Expression and function of 5-HT4 receptors in the mouse enteric nervous system. Am J Physiol Gastrointest Liver Physiol (2005) 289:G1148–1163. doi: 10.1152/ajpgi.00245.2005
90. Young JB, Godara P, Williams V, Summerfelt P, Connor TB, Tarima S, et al. Assessing retinal structure in patients with parkinson's disease. J Neurol Neurophysiol (2019) 10:485. doi: 10.4172/2155-9562.1000485
91. Kashani AH, Asanad S, Chan JW, Singer MB, Zhang J, Sharifi M, et al. Past, present and future role of retinal imaging in neurodegenerative disease. Prog Retin Eye Res (2021) 83:100938. doi: 10.1016/j.preteyeres.2020.100938
92. Byun MS, Park SW, Lee JH, Yi D, Jeon SY, Choi HJ, et al. Association of retinal changes with Alzheimer disease neuroimaging biomarkers in cognitively normal individuals. JAMA Ophthalmol (2021) 139:548–56. doi: 10.1001/jamaophthalmol.2021.0320
93. Asanad S, Felix CM, Fantini M, Harrington MG, Sadun AA, Karanjia R. Retinal ganglion cell dysfunction in preclinical alzheimer's disease: an electrophysiologic biomarker signature. Sci Rep (2021) 11:6344. doi: 10.1038/s41598-021-85010-1
94. Marchesi N, Fahmideh F, Boschi F, Pascale A, Barbieri A. Ocular neurodegenerative diseases: interconnection between retina and cortical areas. Cells (2021) 10:2394. doi: 10.3390/cells10092394
95. Moon J, Yoon CH, Choi SH, Kim MK. Can gut microbiota affect dry eye syndrome? Int J Mol Sci (2020) 21:8443. doi: 10.3390/ijms21228443
96. Napolitano P, Filippelli M, Davinelli S, Bartollino S, dell'Omo R, Costagliola C. Influence of gut microbiota on eye diseases: an overview. Ann Med (2021) 53:750–61. doi: 10.1080/07853890.2021.1925150
97. Xue W, Li JJ, Zou Y, Zou B, Wei L. Microbiota and ocular diseases. Front Cell Infect Microbiol (2021) 11:759333. doi: 10.3389/fcimb.2021.759333
98. Jiao J, Yu H, Yao L, Li L, Yang X, Liu L. Recent insights into the role of gut microbiota in diabetic retinopathy. J Inflammation Res (2021) 14:6929–38. doi: 10.2147/JIR.S336148
99. Scuderi G, Troiani E, Minnella AM. Gut microbiome in retina health: the crucial role of the gut-retina axis. Front Microbiol (2021) 12:726792. doi: 10.3389/fmicb.2021.726792
100. Nakamura YK, Metea C, Karstens L, Asquith M, Gruner H, Moscibrocki C, et al. Gut microbial alterations associated with protection from autoimmune uveitis. Invest Ophthalmol Vis Sci (2016) 57:3747–58. doi: 10.1167/iovs.16-19733
101. Tang J, Tang Y, Yi I, Chen DF. The role of commensal microflora-induced T cell responses in glaucoma neurodegeneration. Prog Brain Res (2020) 256:79–97. doi: 10.1016/bs.pbr.2020.06.002
102. Masland RH. The fundamental plan of the retina. Nat Neurosci (2001) 4:877–86. doi: 10.1038/nn0901-877
103. Hoon M, Okawa H, Della Santina L, Wong ROL. Functional architecture of the retina: development and disease. Prog Retin Eye Res (2014) 42:44–84. doi: 10.1016/j.preteyeres.2014.06.003
104. Chen M, Luo C, Zhao J, Devarajan G, Xu H. Immune regulation in the aging retina. Prog Retin Eye Res (2019) 69:159–72. doi: 10.1016/j.preteyeres.2018.10.003
105. Eshaq RS, Aldalati AMZ, Alexander JS, Harris NR. Diabetic retinopathy: breaking the barrier. Pathophysiology (2017) 24:229–41. doi: 10.1016/j.pathophys.2017.07.001
106. Brockhaus K, Melkonyan H, Prokosch-Willing V, Liu H, Thanos S. Alterations in tight- and adherens-junction proteins related to glaucoma mimicked in the organotypically cultivated mouse retina under elevated pressure. Invest Ophthalmol Vis Sci (2020) 61:46. doi: 10.1167/iovs.61.3.46
107. Kayama H, Okumura R, Takeda K. Interaction between the microbiota, epithelia, and immune cells in the intestine. Annu Rev Immunol (2020) 38:23–48. doi: 10.1146/annurev-immunol-070119-115104
108. Sato J, Kanazawa A, Azuma K, Ikeda F, Goto H, Komiya K, et al. Probiotic reduces bacterial translocation in type 2 diabetes mellitus: a randomized controlled study. Sci Rep (2017) 7:12115. doi: 10.1038/s41598-017-12535-9
109. Deng Y, Ge X, Li Y, Zou B, Wen X, Chen W, et al. Identification of an intraocular microbiota. Cell Discovery (2021) 7:13. doi: 10.1038/s41421-021-00245-6
110. Chen N, Wu J, Wang J, Piri N, Chen F, Xiao T, et al. Short chain fatty acids inhibit endotoxin-induced uveitis and inflammatory responses of retinal astrocytes. Exp Eye Res (2021) 206:108520. doi: 10.1016/j.exer.2021.108520
111. Andriessen EM, Wilson AM, Mawambo G, Dejda A, Miloudi K, Sennlaub F, et al. Gut microbiota influences pathological angiogenesis in obesity-driven choroidal neovascularization. EMBO Mol Med (2016) 8:1366–79. doi: 10.15252/emmm.201606531
112. Dalile B, Van Oudenhove L, Vervliet B, Verbeke K. The role of short-chain fatty acids in microbiota-gut-brain communication. Nat Rev Gastroenterol Hepatol (2019) 16:461–78. doi: 10.1038/s41575-019-0157-3
113. Gyawali A, Kang YS. Blood-to-Retina transport of imperatorin involves the carrier-mediated transporter system at the inner blood-retinal barrier. J Pharm Sci (2019) 108:1619–26. doi: 10.1016/j.xphs.2018.11.040
114. Gurung M, Li Z, You H, Rodrigues R, Jump DB, Morgun A, et al. Role of gut microbiota in type 2 diabetes pathophysiology. EBioMedicine (2020) 51:102590. doi: 10.1016/j.ebiom.2019.11.051
115. Tilg H, Zmora N, Adolph TE, Elinav E. The intestinal microbiota fuelling metabolic inflammation. Nat Rev Immunol (2020) 20(1):40–54. doi: 10.1038/s41577-019-0198-4
116. Rizzetto L, Fava F, Tuohy KM, Selmi C. Connecting the immune system, systemic chronic inflammation and the gut microbiome: the role of sex. J Autoimmun (2018) 92:12–34. doi: 10.1016/j.jaut.2018.05.008
117. Motwani M, Pesiridis S, Fitzgerald KA. DNA Sensing by the cGAS-STING pathway in health and disease. Nat Rev Genet (2019) 20:657–74. doi: 10.1038/s41576-019-0151-1
118. Shmuel-Galia L, Humphries F, Lei X, Ceglia S, Wilson R, Jiang Z, et al. Dysbiosis exacerbates colitis by promoting ubiquitination and accumulation of the innate immune adaptor STING in myeloid cells. Immunity (2021) 54:1137–1153.e8. doi: 10.1016/j.immuni.2021.05.008
119. Qin X, Zou H, Niu C. The STING pathway: an uncharacterized angle beneath the gut–retina axis. Exp Eye Res (2022) 217:108970. doi: 10.1016/j.exer.2022.108970
120. Zou M, Gong L, Ke Q, Qi R, Zhu X, Liu W, et al. Heterochromatin inhibits cGAS and STING during oxidative stress-induced retinal pigment epithelium and retina degeneration. Free Radic Biol Med (2022) 178:147–60. doi: 10.1016/j.freeradbiomed.2021.11.040
121. Wang C, Xiao Y, Yu L, Tian F, Zhao J, Zhang H, et al. Protective effects of different bacteroides vulgatus strains against lipopolysaccharide-induced acute intestinal injury, and their underlying functional genes. J Adv Res (2022) 36:27–37. doi: 10.1016/j.jare.2021.06.012
122. Liu J, He Z, Ma N, Chen ZY. Beneficial effects of dietary polyphenols on high-fat diet-induced obesity linking with modulation of gut microbiota. J Agric Food Chem (2020) 68:33–47. doi: 10.1021/acs.jafc.9b06817
123. Lee CJ, Sears CL, Maruthur N. Gut microbiome and its role in obesity and insulin resistance. Ann N Y Acad Sci (2020) 1461:37–52. doi: 10.1111/nyas.14107
124. Chelakkot C, Choi Y, Kim DK, Park HT, Ghim J, Kwon Y, et al. Akkermansia muciniphila-derived extracellular vesicles influence gut permeability through the regulation of tight junctions. Exp Mol Med (2018) 50:e450. doi: 10.1038/emm.2017.282
125. Plovier H, Everard A, Druart C, Depommier C, Van Hul M, Geurts L, et al. A purified membrane protein from akkermansia muciniphila or the pasteurized bacterium improves metabolism in obese and diabetic mice. Nat Med (2017) 23:107–13. doi: 10.1038/nm.4236
126. Takkar B, Sheemar A, Jayasudha R, Soni D, Narayanan R, Venkatesh P, et al. Unconventional avenues to decelerate diabetic retinopathy. Surv Ophthalmol (2022) 67:1574–92. doi: 10.1016/j.survophthal.2022.06.004
127. Dean RG, Burrell LM. ACE2 and diabetic complications. Curr Pharm Des (2007) 13:2730–5. doi: 10.2174/138161207781662876
128. Duan Y, Prasad R, Feng D, Beli E, Li Calzi S, Longhini ALF, et al. Bone marrow-derived cells restore functional integrity of the gut epithelial and vascular barriers in a model of diabetes and ACE2 deficiency. Circ Res (2019) 125:969–88. doi: 10.1161/CIRCRESAHA.119.315743
129. Patel VB, Bodiga S, Basu R, Das SK, Wang W, Wang Z, et al. Loss of angiotensin-converting enzyme-2 exacerbates diabetic cardiovascular complications and leads to systolic and vascular dysfunction: a critical role of the angiotensin II/AT1 receptor axis. Circ Res (2012) 110:1322–35. doi: 10.1161/CIRCRESAHA.112.268029
130. Verma A, Shan Z, Lei B, Yuan L, Liu X, Nakagawa T, et al. ACE2 and ang-(1-7) confer protection against development of diabetic retinopathy. Mol Ther (2012) 20:28–36. doi: 10.1038/mt.2011.155
131. Covasa M, Stephens RW, Toderean R, Cobuz C. Intestinal sensing by gut microbiota: targeting gut peptides. Front Endocrinol (Lausanne) (2019) 10:82. doi: 10.3389/fendo.2019.00082
132. Grasset E, Puel A, Charpentier J, Collet X, Christensen JE, Tercé F, et al. A specific gut microbiota dysbiosis of type 2 diabetic mice induces GLP-1 resistance through an enteric NO-dependent and gut-brain axis mechanism. Cell Metab (2017) 25:1075–1090.e5. doi: 10.1016/j.cmet.2017.04.013
133. Zhang XY, Chen J, Yi K, Peng L, Xie J, Gou X, et al. Phlorizin ameliorates obesity-associated endotoxemia and insulin resistance in high-fat diet-fed mice by targeting the gut microbiota and intestinal barrier integrity. Gut Microbes (2020) 12:1–18. doi: 10.1080/19490976.2020.1842990
134. Milani C, Duranti S, Bottacini F, Casey E, Turroni F, Mahony J, et al. The first microbial colonizers of the human gut: composition, activities, and health implications of the infant gut microbiota. Microbiol Mol Biol Rev (2017) 81:e00036–17. doi: 10.1128/MMBR.00036-17
135. Parkin K, Christophersen CT, Verhasselt V, Cooper MN, Martino D. Risk factors for gut dysbiosis in early life. Microorganisms (2021) 9:2066. doi: 10.3390/microorganisms9102066
136. Rowan S, Taylor A. The role of microbiota in retinal disease. Adv Exp Med Biol (2018) 1074:429–35. doi: 10.1007/978-3-319-75402-4_53
137. Zhu W, Miyata N, Winter MG, Arenales A, Hughes ER, Spiga L, et al. Editing of the gut microbiota reduces carcinogenesis in mouse models of colitis-associated colorectal cancer. J Exp Med (2019) 216:2378–93. doi: 10.1084/jem.20181939
138. Ghosh TS, Rampelli S, Jeffery IB, Santoro A, Neto M, Capri M, et al. Mediterranean Diet intervention alters the gut microbiome in older people reducing frailty and improving health status: the NU-AGE 1-year dietary intervention across five European countries. Gut (2020) 69:1218–28. doi: 10.1136/gutjnl-2019-319654
139. Liu K, Zou J, Fan H, Hu H, You Z. Causal effects of gut microbiota on diabetic retinopathy: a mendelian randomization study. Front Immunol (2022) 13:930318. doi: 10.3389/fimmu.2022.930318
140. Hamady M, Knight R. Microbial community profiling for human microbiome projects: tools, techniques, and challenges. Genome Res (2009) 19:1141–52. doi: 10.1101/gr.085464.108
Keywords: diabetes mellitus (DM), diabetic retinopathy (DR), gut-retina axis, gut microbiota, retina, mechanics, treatment
Citation: Zhang H and Mo Y (2023) The gut-retina axis: a new perspective in the prevention and treatment of diabetic retinopathy. Front. Endocrinol. 14:1205846. doi: 10.3389/fendo.2023.1205846
Received: 14 April 2023; Accepted: 19 June 2023;
Published: 04 July 2023.
Edited by:
Shakilur Rahman, Jamia Hamdard University, IndiaReviewed by:
Juan Sanchez Naves, Opthalmedic and I.P.O., Balearic Island, SpainClaudio Bucolo, University of Catania, Italy
Copyright © 2023 Zhang and Mo. This is an open-access article distributed under the terms of the Creative Commons Attribution License (CC BY). The use, distribution or reproduction in other forums is permitted, provided the original author(s) and the copyright owner(s) are credited and that the original publication in this journal is cited, in accordance with accepted academic practice. No use, distribution or reproduction is permitted which does not comply with these terms.
*Correspondence: Ya Mo, bW95YTUyODZAY2R1dGNtLmVkdS5jbg==
†These authors share first authorship