- 1Department of Obstetrics and Gynecology, The Second Hospital of Jilin University, Changchun, China
- 2Department of Hepato-Biliary-Pancreatic Surgery, The Second Hospital of Jilin University, Changchun, China
Preeclampsia, gestational diabetes mellitus, and recurrent spontaneous abortion are common maternal pregnancy complications that seriously endanger women’s lives and health, and their occurrence is increasing year after year with a rejuvenation trend. In contrast to biomarkers found freely in tissues or body fluids, exosomes exist in a relatively independent environment and provide a higher level of stability. As backbone molecules, guidance molecules, and signaling molecules in the nucleus, lncRNAs can regulate gene expression. In the cytoplasm, lncRNAs can influence gene expression levels by modifying mRNA stability, acting as competitive endogenous RNAs to bind miRNAs, and so on. Exosomal lncRNAs can exist indefinitely and are important in intercellular communication and signal transduction. Changes in maternal serum exosome lncRNA expression can accurately and timely reflect the progression and regression of pregnancy-related diseases. The purpose of this paper is to provide a reference for clinical research on the pathogenesis, diagnosis, and treatment methods of pregnancy-related diseases by reviewing the role of exosome lncRNAs in female pathological pregnancy and related molecular mechanisms.
1 Introduction
Exosomes are membrane vesicles that are released into the extracellular fluid by various cells (1). The exosome membrane is high in cholesterol, sphingolipids, and other components, and it contains a variety of proteins, mRNA, and lncRNA, including mother cell-specific proteins and exosome-associated proteins. Exosomes are the best markers for determining the levels of various substances expressed within the mother cell (2). Scholars have paid close attention to the non-coding RNAs found in the non-coding region in recent years (3). lncRNAs are expressed in low amounts in cells or tissues and have a length of more than 200 nt. Their regulation primarily consists of epigenetic regulation, transcriptional regulation, and post-transcriptional regulation, all of which affect cell proliferation, apoptosis, and differentiation and play an essential role in the development of many diseases (4, 5). Research has found that lncRNA plays an important role in the homeostasis of cells or tissues during development. Although LncRNAs cannot directly regulate protein translation, they can exert regulatory power through miRNAs, which may be mediated as a mediator (6). Some lncRNAs are enriched in exosomes, while others are almost absent, implying that lncRNAs are selectively sorted into exosomes (7). Pathological pregnancy is becoming more common, and the resulting problem of reduced fertility cannot be ignored (8). Exosomal lncRNA, which is abundant and stable in plasma and has high ribonuclease activity, can serve as a reference for clinicians in the diagnosis of pregnancy-related disorders (9). As a result, it is clinically significant to investigate the exosome lncRNAs that affect women’s pathological pregnancy behavior, as this can help to further investigate the disease’s development mechanism and provide new ideas and strategies for disease treatment, thereby genuinely protecting women’s reproductive health (10).
2 Overview of exosomes
2.1 Biogenesis of exosomes
Exosomes are made up of a double-layered lipid membrane structure that ranges in size from 30nm to 150nm and contains DNA, mRNA, and lncRNA (11, 12). Exosomes are present in almost all eukaryotic body fluids (13), including uterine fluid, urine, amniotic fluid, breast milk, peritoneal fluid, and cell culture fluid, according to recent research (14). When exposed to extracellular stimuli, microbial attack, or other stress conditions, the cell membrane invaginates, allowing material from outside the cell membrane to enter the cell along with the cell membrane surface proteins, resulting in the formation of the early-sorting endosome (ESE) (15). By “budding,” the ESE membrane generates multivesicular bodies (MVBs) or late endosomes. Finally, MVBs are secreted extracellularly to form exosomes under the control of the endosomal sorting complex required for transport (ESCRT) and specific proteins (16). Exosomes and target cells interact in three ways: exosome surface membrane proteins directly bind to target cell receptors, activating intracellular signaling pathways; target cells take up exosomes via endocytosis; and exosomes can directly fuse with the plasma membrane of target cells, releasing the miRNAs and lncRNAs they carry into the target cells (17) (Figure 1).
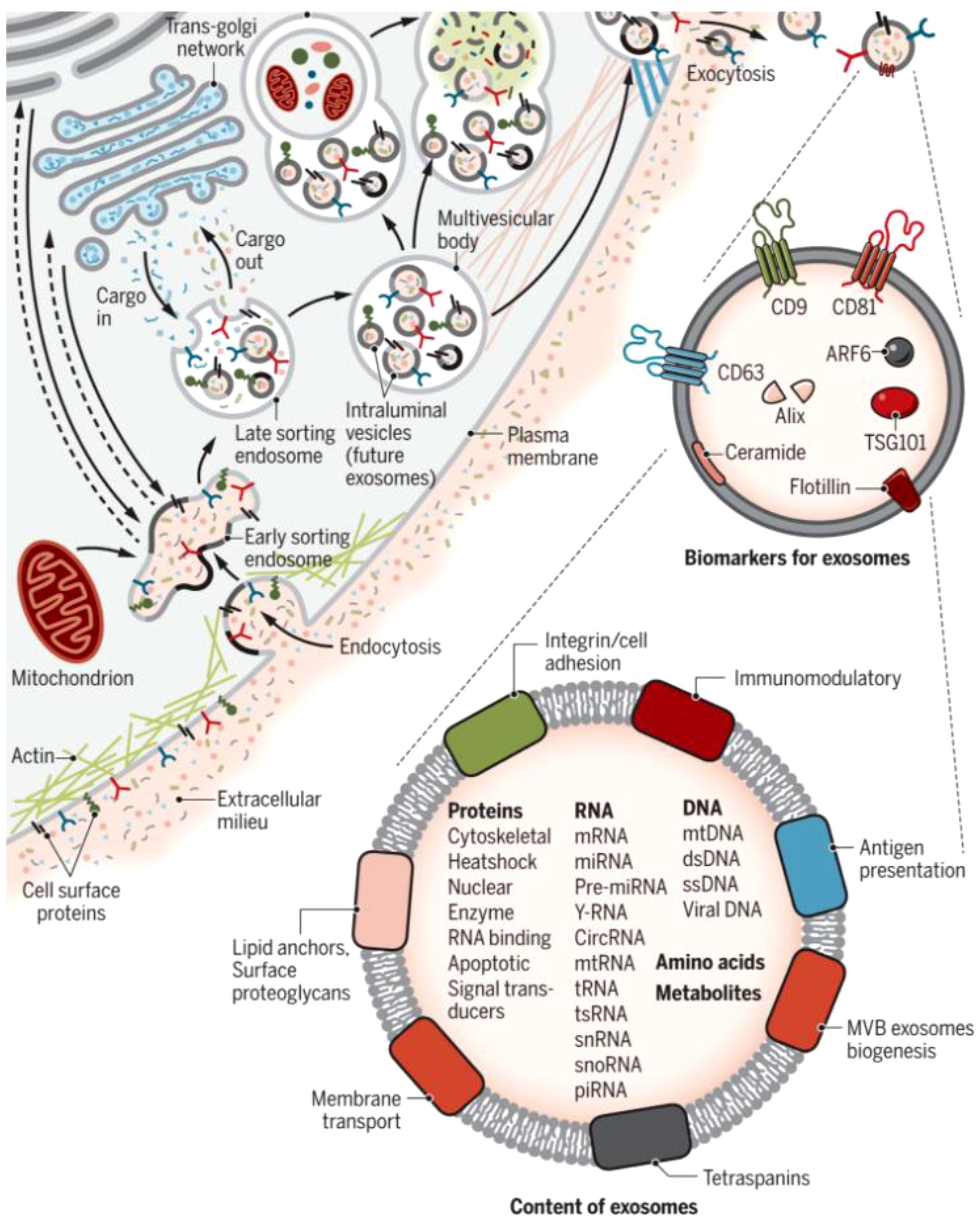
Figure 1 Biogenesis and identification of exosomes. Fluid and extracellular constituents such as proteins, lipids, metabolites, small molecules, and ions can enter cells, along with cell surface proteins, through endocytosis and plasma membrane invagination. The resulting plasma membrane bud formation in the luminal side of the cell presents with outside-in plasma membrane orientation. Several proteins are implicated in exosome biogenesis and include ESCRT proteins, as well as others that are also used as markers for exosomes (CD9, CD81, CD63, flotillin, ceramide, and Alix). Exosome surface proteins include tetraspanins, integrins, immunomodulatory proteins, and more. Exosomes can contain different types of cell surface proteins, intracellular proteins, RNA, DNA, amino acids, and metabolites (15).
2.2 Function of exosomes
Exosomes are critical intercellular messengers that regulate cellular physiological functions such as immune regulation, cell proliferation, antigen expression and presentation, and bioenergetic conversion (18, 19). Exosomes transport nucleic acids, which play an essential role in cellular communication (20). Exosomes contain at least ten different types of RNA, and actively secreted exosomes can package a large amount of intracellular information material for transmission from one cell to another, achieving cross-cellular regulation and participating in intercellular communication and microenvironment regulation (21). Because cell membrane transmembrane proteins are also expressed on the exosome membrane, it is critical for exosome identification (22). Glycoproteins and transmembrane proteins are enriched in intercellular communication and adhesion events, which can be utilized to determine their cellular or tissue origin, such as placental-derived exosomes that express placental-like alkaline phosphatase (PLAP) (23). Exosomes can control morphogenetic signaling, immune cell recruitment, and genetic material transport to carry out a range of cell biological tasks in the cellular microenvironment (24). The majority of methods for detecting exosomal nucleic acid information rely on the presence of mRNA and microRNA in exosomes (25). In recent years, the importance of targeting exosomal lncRNAs has gained more attention. LncRNAs protected by the exosomal tegument exhibit higher expression and better stability than lncRNAs isolated directly from peripheral bodily fluids, and their results are trustworthy.
3 Overview of lncRNAs
3.1 lncRNAs play a role in the regulation of pre-transcription
By controlling the regulation of target genes by the epimodification complex before transcription takes place through chromatin modification, genomic imprinting, and dosage compensation effects, lncRNAs in the nucleus play an epigenetic function in gene expression (26, 27). The chromatin state and the way proteins attach to chromatin are both altered by the different ways that lncRNAs can modify histones, including methylation, acetylation, and ubiquitination. In order to interact with the histone modification complex Polycomb Repressive Complex 2 (PRC2) and mediate histone methylation and demethylation, LncRNA HOX antisense intergenic RNA (HOTAIR) may serve as a molecular scaffold. The ATP-dependent chromatin remodeling complex plays a major role in controlling chromatin remodeling, an enzymatic co-process that enables nucleosomal DNA acquisition by altering the structure, composition, and placement of nucleosomes. BRG1, the central component of the chromatin remodeling complex SWI/SNF, can interact with lncRNA MANTIS, stabilizing it. An open chromatin conformation is induced by the interaction of BRG1 with BAFl55, another chromatin remodeling factor, and this increases the transcription of genes involved in angiogenesis. MANTIS is a lncRNA that is thought to have pro-endothelial angiogenic potential (Figure 2).
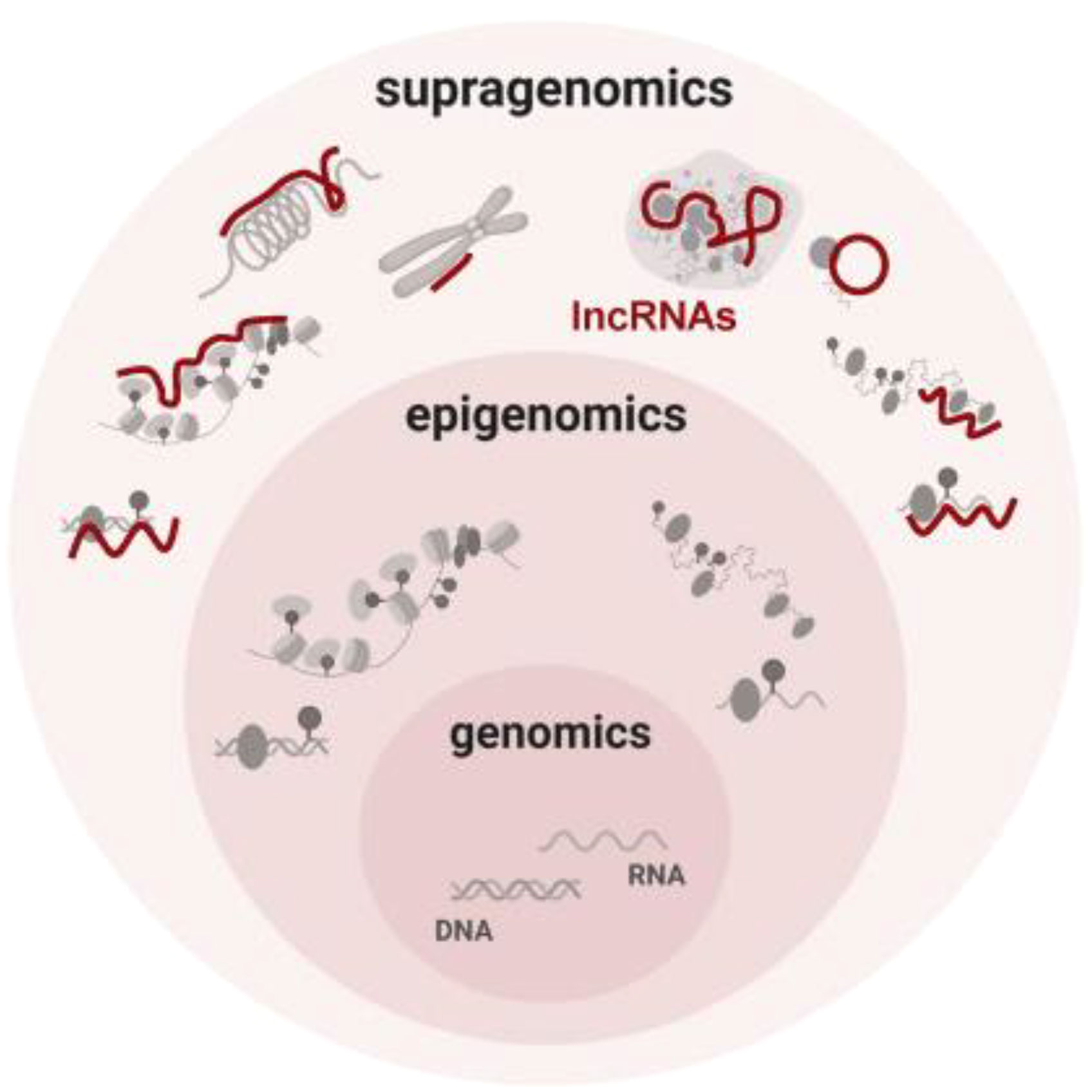
Figure 2 Conceptual summary of supragenomic regulation by lncRNAs Vast and heterogeneous lncRNAs interact broadly with gene regulatory machineries. By providing a supragenomic layer of control built upon genomic and epigenomic processes, lncRNAs modulate many levels of gene regulation, from transcription to protein modification (26).
3.2 LncRNAs play a role in controlling gene transcription
By preventing the RNA Pol II complex from attaching to the promoter, LncRNA can interfere with transcription (28). According to Latos et al., the lncRNA Aim transcript covers the lgf2r promoter region and prevents the recruitment of RNA Pol II, preventing the transcription of lgf2r. LncRNA can directly activate the downstream genes by bridging the gap between the enhancer and promoter regions (29). It is crucial for the transcriptional activation of Snail that activating lncRNAs interact with enhancers and transcription factors, be present inside the enhancer, and have enhancer activity. Studies demonstrate that the binding of activating lncRNAs to the Snail promoter region is mediated by the transcriptional co-activation complex Mediator, acting in concert with CDK8-catalyzed histone modifications to promote transcriptional activation of Snail, underscoring the significance of activating lncRNAs in human disease. lncRNA LNMAT2 is loaded into exosomes through direct interaction with heterogeneous ribonucleoprotein A2B1 (hnRNPA2B1) by direct interaction to exosomes and delivery to human lymphatic vessel endothelial cells (HLEC); subsequently, LncRNALNMAT2 forms a triple complex with the PROX1 promoter by inducing hnRNPA2B1-mediated H3K4me3 and enhances PROX1 transcription, thereby promoting lymphangiogenesis and lymph node metastasis.
3.3 LncRNAs participate in the selective shear regulation process
Genetic information from DNA is translated into mature mRNA by biological processes, including shearing and splicing; this process does not function directly (30). More than 95% of gene transcripts go through a process called selective splicing, which makes biological gene expression more complex and plays a regulatory function in the growth and development of living things (31). LncRNA participates in gene expression regulation by constructing different variable splicing forms through splicing factors, regulating miRNA precursor transcripts and upstream differentially methylated regions (DMR) (32). In order to control the phosphorylation level of the serine/arginine-rich (SR) protein family, which controls mRNA splicing, lncRNAs have been shown to function as regulatory factors (33). It has been demonstrated that the lncRNA metastasis-associated lung adenocarcinoma transcript 1 (MALAT1) associates with numerous proteins to form a nuclear speckle that takes part in varied pre-mRNA shearing (34). Vidisha et al. discovered that the lncRNA MALAT1 selectively binds to the nuclear protein TDP-43, resulting in the recruitment of the SR protein family to the nucleus. The nuclear speckle was recruited by splicing factors like TDP-43, which improved the SR protein family’s capacity to splice and thus raised its level of phosphorylation.
4 Exosomal lncRNA offers excellent clinical use potential
Specificity, as exosomes include particular indicators of tissue or cellular origin, is one of the properties of exosome-derived lncRNAs as biomarkers. Notably, the RNase activity is high in the extracellular environment, but extracellular ncRNAs remain relatively stable in plasma, suggesting that circulating ncRNAs may be protected and circumvented from harsh conditions (35). Exosome stability: Exosomes remain in a stable state in bodily fluids, and RNA is not significantly exposed because of the protection of lipid bilayer membranes, where enzymes cannot easily digest the contents of exosomes (36). Although the lncRNA expression is low in some cells, it is highly expressed in their secreted exosomes and correlates with the development of disease. Exosomes are widely distributed in different body fluids. The primary techniques for isolating exosomes are gradient density centrifugation, differential ultracentrifugation, polymer immunoprecipitation, gel exclusion separation, and membrane affinity kits (37, 38). Exosomal lncRNAs combine the benefits of exosomes and lncRNAs in a way that enhances the effectiveness of treatment and the prognosis of patients (39). Exosomes can be employed as specialized targets for treating disease. As a result, the non-invasive detection of lncRNA produced from exosomes has the potential to be exploited as a biomarker for future diagnosis and therapy (40).
5 Correlation of exosomal lncRNA with pregnancy
5.1 Endometrial tolerance
Fertilization, implantation, endometrial metaphase, placental development, and birth are significant, complex, and irreversible aspects of pregnancy in humans and other mammals (41). Abnormalities or the failure of any one of these processes can have an impact on the pregnancy’s outcome (9) (Figure 3). By directly influencing embryonic development and regulating the expression of important adhesion molecules, the endometrium can leak exosomes into the uterine fluid and govern implantation (42). h19 is a naturally occurring lncRNA that is widely produced, developmentally controlled, and affects Let-7 target genes (43). Reduced expression of the H19 gene and the ITG-3 protein was found in the recurrent implantation failure (RIF) group, proving that the expression of the lncRNAH19 is positively associated with that of the ITG-3 protein, reducing endometrial tolerance and ultimately causing implantation failure.lncRNATUNAR was initially expressed in the human endometrium and is thought to play a role in embryo implantation by controlling the attachment of blastocysts to the endometrial epithelium as well as the proliferation and ecdysis of embryonic stem cells. In healthy females, the expression of lncRNATUNAR was increased in LH+2 and downregulated in LH+7. Due to the cyclic expression of the endometrium and its abnormal expression in RIF patients, lncRNATUNAR may have a role in controlling the embryonic implantation process. lncRNATUNAR was found to be increased in LH+7 endometrium from RIF patients.
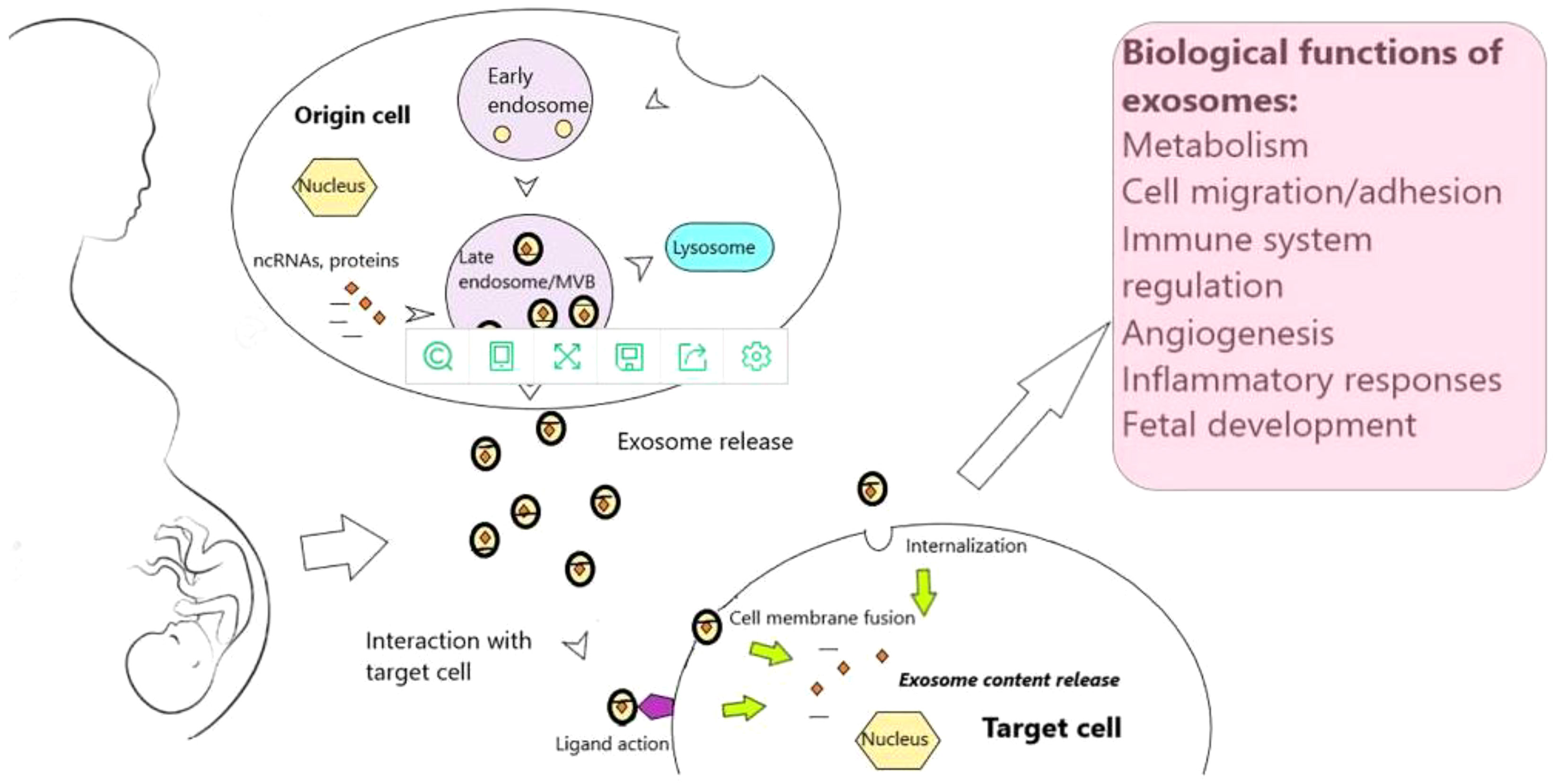
Figure 3 Exosomes and exosomal noncoding RNAs throughout human gestation (9).
5.2 Promote the establishment of immune tolerance at the maternal-fetal interface
The maternal-fetal interface, which is made up of extra-embryonic tissues and the meconium, is a crucial component that helps the mother’s immune system adapt to the fetus during pregnancy (44). By triggering the JNK and p38 signaling pathways in meconium macrophages via the exosomal lncRNA Zinc finger E-box-binding homeobox 2 antisense RNA 1 (ZEB2-AS1), trophoblast cells can create and maintain the maternal-fetal immune tolerance microenvironment. This promotes the polarization of macrophages toward the inhibitory M2 phenotype. In order to support the orderly development of a typical pregnancy, the induced M2 can also operate on trophoblast cells and encourage their proliferation and differentiation at the same time. The decline in recurrent spontaneous abortion patients’ metaphase macrophage M2 may be brought on by low expression of ZEB2-AS 1 in their trophoblast exosomes. The preservation of a stable pregnancy may be made possible by apoptosis, which may allow the human placenta and fetal allografts to avoid a detrimental maternal immune attack while pregnant and enjoy immunological privileges in the uterine cavity (45). The results point to functional FasL and TRAIL being secreted by human early and term placentas, which help deliver apoptosis and shield the fetal placenta from activated maternal immune cells. This suggests placental exosome-mediated fetal immunological privilege. Exosomes play a key role in preserving homeostasis at the maternal-fetal interface throughout pregnancy and are a cutting-edge instrument for intercellular communication (46).
5.3 Promote successful embryo implantation
Embryo implantation, a crucial stage in pregnancy, is the process by which the blastocyst interacts with the uterus in a receptive condition while in an activated state before making close contact with the endometrium (47). Mammalian reproduction depends on the embryo’s successful implantation into the mother (48). Placenta-derived exosome (PEXO) can be ingested by epithelial and stromal cells in the meconium, changing the regional immunological milieu, according to in vitro research. In order to start and maintain pregnancy, cell-to-cell communication at the maternal-fetal interface is crucial. Exosomes, which the embryo secretes to help with implantation, enhance the embryo’s natural adaptability and support successful implantation and the start of pregnancy, allowing the embryo to control its growth. Before embryo implantation, extracellular vesicles of decidual epithelial cells can activate the expression of Bcl2, Bax, Casp3, and Tp53 genes in endometrial epithelial cell apoptosis pathways. Exosomes primarily increase the expression of endometrial epithelial cell adhesion-related proteins following embryo implantation to aid subsequent adherence (49). Exosomes from human ectodermal stromal cells can upregulate the production of trophoblast calmodulin and so boost invasive activity in addition to that of epithelial cells. They can also promote the creation of endothelial cell tubes and may be crucial for angiogenesis. In mouse trials, embryos treated with embryonic-derived exosomes were able to increase implantation rates and improve implantation ability. They could also improve blastocyst formation rate, embryo quality, and future growth and development.
6 Exosomal lncRNA and pathological pregnancy
Exosomes contain various proteins and nucleic acids, serving as diagnostic markers for obstetric diseases with high specificity. The study of exosome lncRNA can explore the pathogenesis of various diseases in pathological pregnancy, screen biomarkers, and provide a new basic basis for the diagnosis and treatment of diseases. Many lncRNAs associated with tumor cell function may also play significant regulatory roles for trophoblasts because placental trophoblasts share characteristics with tumor cells during proliferation, migration, and invasion (50). This is especially true for pathways involved in angiogenesis, cell cycle regulation, cell migration, and invasion (51). Through interacting with miR-216a-5p and controlling recombinant hexokinase 2(HK2), LncRNA MALAT1 prevents placental trophoblast growth, migration, and invasion, as well as angiogenesis, cell cycle arrest, and apoptosis. In addition to the syncytial trophoblast-specific protein PLAP and the trophoblast-specific protein human leukocyte antigen G(HLAG), PEXO is abundant in the exosomal marker proteins CD9, CD63, and CD81 (52–54). These two proteins can be separated to form PEXO in maternal peripheral blood, and the quantity of PEXO can be used to forecast fetal growth and ascertain the success of a pregnancy. Exosomes carry a variety of payloads in maternal peripheral blood, and histological study of these exosomes in various disorders has shown that changes in their type and amount may negatively impact the function of target cells (55).
6.1 Exosomal lncRNA and Preeclampsia
6.1.1 Exosomes participate in the occurrence of PE
A key contributor to increased maternal and neonatal mortality, Preeclampsia is a pregnancy problem that manifests beyond 20 weeks of gestation with proteinuria, hypertension, or other systemic damage (56–60). To lower maternal and neonatal mortality and enhance mother and baby health, early Preeclampsia diagnosis is crucial (61). The number of placental exosomes, changes in their composition, and their impact on the maternal immune system are thought to be the key ways that PEXO contributes to Preeclampsia pathogenesis (62).
Preeclampsia patients exhibit decreased expression of functional proteins such as matrix metalloproteinase (MMP) 2 and MMP9, as well as increased levels of phosphatidylserine (PS) and lower levels of phosphatidic acid and phosphatidylglycerol in exosomes when compared to the normal pregnancy group (63, 64). The remodeling of spiral arteries, fetal growth, superficial placentation, reduced blood flow, and ultimately the development of Preeclampsia can all be impacted by decreased expression of any one of these proteins. Human umbilical cord mesenchymal stem cells (HUCMSCs)- exosomes can increase IL-10, TNF-, IFN-, and the local recruitment of NK cells and macrophages in utero, modulating the immunological balance at the maternal-fetal plane and indirectly affecting pregnancy outcomes. Compared to women who had normal pregnancies, the placentas of Preeclampsia patients had a significant number of differently expressed lncRNAs, according to research using lncRNA microarray technology. They were implicated in the development of Preeclampsia by interfering with trophoblast cell activity, among other things (65).
6.1.2 Decreased expression of exosomal lncRNA in Preeclampsia
A lncRNA called MALAT1 is linked to placental implantation and penetration (66). When compared to healthy pregnant women, Preeclampsia sufferers’ placental tissues express less of the lncRNA MALAT1 (67). When lncRNAMALAT1 levels are low, EMT is induced with less trophoblast invasion, migration, and angiogenesis, which can result in a higher uterine spiral artery remodeling injury (68). According to research, lncRNA MALAT1 levels in plasma exosomes from pregnant women with Preeclampsia were positively correlated with vascular endothelial growth factor (VEGF) expression levels. This suggests that downregulating lncRNA MALAT1 levels in plasma exosomes may speed up the progression of Preeclampsia by controlling VEGF expression, which in turn suppresses angiogenesis (69). Wu et al. discovered that lncRNAMALAT1 could bind to miR-206, prevent the latter from degrading IGF-1 mRNA, boost IGF-1 expression, and activate the PI3K/AKT signaling pathway, which in turn encouraged trophoblast migration and invasion (70).
Preeclampsia patients had a lower placental expression of the short nucleolar RNA host gene 22 (SNHG22) than healthy pregnant women did. By interacting with miR-128-3p to encourage PCDH11X expression and open up downstream pathways, lncRNASNHG22 can have a role in Preeclampsia. In comparison to normal pregnant placental tissues, the expression of lncRNA XIST was found to be considerably reduced in the placentas of Preeclampsia patients. lncRNA XIST is involved in the development of Preeclampsia by regulating the proliferation and invasive ability of trophoblast HTR-8/SVneo through miR-135b. The lncRNA TUG1 was downregulated in the placental tissue of Preeclampsia patients compared to healthy pregnant women, and this downregulation decreased cell proliferation, migration, and invasion while promoting trophoblast death. While TUG1 downregulation boosted the expression of the enhancer of zeste homolog 2 (EZH2) and decreased the levels of the Rho family GTPase 3 (RND3) in Preeclampsia, it prevented remodeling of the uterine spiral artery. Studies have shown that downregulating lnc-dendritic cell (DC), a lncRNA expressed in DC, prevents monocytes from differentiating into DC, diminishing the inhibitory effect of DC on Treg, encouraging the proliferation of Th1 cells in the meconium of Preeclampsia patients, and ultimately promoting Preeclampsia (71, 72).
6.1.3 Increased expression of exosomal lncRNA in Preeclampsia
Compared to normal pregnancies, the placenta of Preeclampsi patients has higher levels of lncRNAH19 expression (73). In the human choriocarcinoma cell line JEG-3 and the human choriocapillaris trophoblast cell line HTR-8/SVneo, lncRNAH19 regulates the PI3K/Akt/mTOR pathway and boosts autophagy and invasiveness (74). Moreover, the lncRNAH19 gene encodes miR-675, which can suppress cell growth by lowering the expression of the nodal modulator 1 (NOMO1) in JEG-3 cells (75). In the placental tissues of Preeclampsia patients, lncRNA GAS5 expression is elevated, and its level rises as the severity of the disease does as well (76). The development of atherosclerosis can be aided by the lncRNA GAS5, which can encourage the death of vascular endothelial cells as well as aberrant proliferation and migration of vascular smooth muscle cells. The degree to which lncRNA GAS5 was expressed in Preeclampsia patients was inversely correlated with spiral artery lumen area and positively correlated with spiral artery wall thickness, suggesting that lncRNA GAS5 may be connected to the process of placental spiral artery recasting. Many lncRNAs have an impact on trophoblast cells’ physiological processes, which are intimately associated with the development of Preeclampsia and include migration, invasion, proliferation, and apoptosis (77). Determining the regulatory roles that Preeclampsia-related lncRNAs play in various pathways can therefore assist in clarifying the interactions that contribute to Preeclampsia pathophysiology, identify essential molecules for diagnosis and therapy, and provide potential targets for Preeclampsia prevention and treatment (78).
6.2 Exosomal lncRNA and gestational diabetes mellitus
6.2.1 Exosomal lncRNA’s role in the emergence of gestational diabetes mellitus
The condition known as gestational diabetes mellitus is characterized by aberrant glucose metabolism in the body, which is brought on by insulin insufficiency and hormonal changes during pregnancy (79). In extreme cases, gestational diabetes mellitus can result in maternal and neonatal death. The incidence of gestational diabetes mellitus is increasing as people’s lifestyles and diets change (80). Research has revealed that gestational diabetes mellitus is a risk factor for cardiovascular disease and type 2 diabetes (T2DM), which can raise the risk of immediate or long-term issues in expecting mothers and children and gravely jeopardize the physical and mental health of women and neonates (81, 82). Insulin resistance (IR), one of the primary causes of gestational diabetes mellitus, has a complex etiology and unknown pathophysiology (83, 84). Hence, a contemporary topic that requires attention is the quest for biomarkers with high sensitivity and specificity for the early diagnosis and treatment of gestational diabetes mellitus as well as the maternal postpartum state (85).
Exosome levels and biological activity were shown to vary with gestational stage in pregnant women with gestational diabetes mellitus and normal glucose tolerance (NGT) (86). When matched for gestational weeks, the concentration of placental exosomes in the plasma of gestational diabetes mellitus patients is significantly higher than that of healthy pregnant women and may positively correlate with baby weight. Between 22 and 28 weeks of gestation, the plasma exosomes mostly displayed altered expression of proteins related to insulin sensitivity, including CAMK2b and pregnancy-as-sociated plasma protein A (PAPPA). As a result, gestational diabetes mellitus patients’ plasma exosomes play a significant role in controlling glucose homeostasis during pregnancy (87).
6.2.2 Decreased expression of exosomal lncRNA in gestational diabetes mellitus
An endogenous lncRNA called SNHG17 can bind and inhibit the transcription of miRNAs, which control the transcription and expression of target genes and contribute to the onset and progression of gestational diabetes mellitus (88). Research suggests that lncRNASNHG17, which is connected to vascular endothelial cell survival and angiogenesis, is abnormally underexpressed in the peripheral blood of T2DM patients (89). Serum lncRNASNHG17 levels are significantly lower in pregnant women with gestational diabetes mellitus than in healthy pregnant women, and they are correlated with fasting blood glucose (FBG), glycosylated hemoglobin, type A1c (HbA1c), and Homeostasis model assessment(HOMA)-IR negatively and HOMA-β positively. This suggests that lncRNASNHG17 may be involved in the pathological lesion process of gestational diabetes mellitus by influencing these variables (90). In gestational diabetes mellitus patients, the expression level of lncRNAMALAT1 was discovered to be correlated with the disease severity and to have a strong negative relationship with the maternal BMI and FBG at delivery (91). Compared to healthy pregnant women, gestational diabetes mellitus patients had significantly lower serum levels of the lncRNAMALAT1 gene (92). By increasing miR-155-5p expression, suppressing IGF2 expression, enhancing trophoblast cell survival, migration, and invasion, and reviving the biological activity of high glucose-induced trophoblast cells, in vitro cellular assays demonstrated that silencing lncRNAMALAT1 plays a role in the development of gestational diabetes mellitus (93).
6.2.3 Increased expression of exosomal lncRNA in gestational diabetes mellitus
Maternally expressed gene 3 (MEG3) has been linked to abnormal placental expression, trophoblast migration, and apoptosis. It also has an impact on the expression of the NF-B, caspase-3, and Bax proteins in the placenta. Human umbilical vein endothelial cells (HUVEC) from gestational diabetes mellitus have elevated MEG3 expression, which affects fetal endothelial function through the PI3K signaling pathway (94). MEG3 overexpression, meanwhile, was able to prevent human villous trophoblast HTR-8/SVneo from proliferating, migrating, and invading while inducing apoptosis, indicating that MEG3 may be implicated in the development of gestational diabetes mellitus and playing a significant role (95). The conserved family SNX member sortingnexin17 (SNX17) is crucial for the endocytic, intracellular transport of cell surface proteins. It is crucial for endocytosis and the intracellular activities that involve cell surface proteins. It was discovered that lncRNA-SNX17 was elevated and miR-517a was downregulated in the blood of gestational diabetes mellitus patients and that the two together were more useful for the diagnosis of gestational diabetes mellitus than the single index test (96). Both the lncRNA P21 and the lncRNA H19 were shown to be elevated in the serum and placental tissues of gestational diabetes mellitus patients. These two lncRNAs may cooperate to promote the development of gestational diabetes mellitus and correlate with newborns’ birth weights. The incidence of gigantic newborns in gestational diabetes mellitus patients was connected with serum lncRNA HOXA transcript expression at the distal tip (HOTTIP), which was considerably increased in gestational diabetes mellitus patients. Both miR-21 and lncRNA HOTTIP were discovered to be abnormally expressed in gestational diabetes mellitus and connected with a poor pregnancy outcome, which could be used as a prediction for early identification of gestational diabetes mellitus (97). In order to identify other potential targets for the therapy of gestational diabetes mellitus, we will keep screening exosomal lncRNAs strongly associated with IR and glucose metabolism and investigate their potential participation in regulatory networks (98, 99).
6.3 Exosomal lncRNA and recurrent spontaneous abortion
6.3.1 The emergence of recurrent spontaneous abortion is intimately related to exosomal lncRNA
Two or more consecutive spontaneous abortions constitute the incidence of recurrent spontaneous abortion (100). Early superficial placental implantation, poor trophoblast migration and invasion, and defective placental microvascular formation are three significant pathophysiological causes for the development of recurrent spontaneous abortion, all of which are becoming more common (101, 102). Exosomal lncRNAs participate in the regulation of trophoblast invasive capacity, the expression of cyclin-dependent kinases (CDKs), and various physiological processes like lipid metabolism and protein synthesis. These actions have an impact on early embryonic implantation. Exosomes produced by mesenchymal stem cells can operate on trophoblast cells to cause them to secrete MMP, which in turn makes trophoblasts more invasive. By being endocytosed by trophoblast cells, exosomes from metaphase macrophages can carry out the corresponding biological action (103). When we co-cultured exosomes from patients with unexplained recurrent spontaneous abortion (URSA) and patients with normal early pregnancy abortion with trophoblast HTR-8/SVneo cells, we discovered that the number of cells migrating in URSA patients was significantly lower than that in patients with normal early pregnancy abortion. Both the number of cells migrating and the viability of the cells were much lower in URSA patients. This shows that meconium macrophages can control trophoblast cells’ biological behavior by secreting exosomes, leading to embryonic arrest and playing a role in the emergence of URSA (104).
The regulation of embryonic development, endometrial tolerance, trophoblast function, stimulation of inflammation, placental vascular development, and the regulation of embryonic stem cells are the key ways that lncRNAs contribute to miscarriage (105, 106). It was discovered that the p53-regulated lncRNA lncPRESS1 safeguards embryonic stem cells by inhibiting the function of the silent information regulator (SIRT) 6 (107). Meanwhile, lncKdm2b stimulates the production of transcription factor zinc finger and BTB structural domain protein 3, promoting early embryonic development and embryonic stem cell self-renewal (108). Small interfering RNA-silenced mouse embryonic stem cells may suffer harm or even miscarry if appropriate lncRNAs are administered (109). LncRNA screening before embryo implantation can lower the chance of a failed transfer and miscarriage since lncRNAs play a significant role in controlling embryonic stem cell development (110, 111).
6.3.2 Exosomal lncRNA offers fresh approaches to identifying and treating recurrent spontaneous abortion
Defective gene expression and aberrant cell proliferation are brought on by the increased expression of LncRNA H19 in recurrent spontaneous abortion patients’ embryonic tissues (112). Through its binding to let-7, lncRNA H19 inhibits ITG3 expression. This has an impact on how cells adhere to the basement membrane and lowers endometrial tolerance. As a result, embryos are lost (113). Apoptosis and iron death are promoted by IncRNA H19 by downregulating the expression of Bax and upregulating the expression of Bcl2 and GPX4 in recurrent spontaneous abortion. Nuclear enriched transcript 1(NEAT1) and MALAT levels in recurrent spontaneous abortion patients are much lower than in healthy women, and trophoblast cell proliferation, migration, invasiveness, and apoptosis were all reduced when the MALAT1 gene was knocked down (114). The human plasmacytoma variant translocation 1 (PVT1) promoter is directly impacted by lncRNA regulation, which also lowers the ability of trophoblast cells to invade (115).
Patients with recurrent spontaneous abortion had increased villous tissue LINC01088 expression. ARG1 can be bound by LINC01088, which is mostly found in the nucleus of trophoblast cells (116). This increases ARG1’s protein stability and suppresses the expression of NOS. When LINC01088 is overexpressed, ARG1’s protein stability is improved, which in turn lowers the expression of NOS and lowers NO expression. The JNK/P38 MAPK signaling pathway is further activated by the decreased NO, which impairs trophoblast cell proliferation, invasion, and migration and contributes to the development of recurrent spontaneous abortion. The lnc-SLC4A1-1 gene was discovered to be significantly expressed in the villi of URSA patients and to be able to trigger an immunological response via the NF-B/CXCL8 axis (117). In peripheral blood mononuclear cells from pregnant women with URSA, the expression levels of the lncRNAs SNHG5 and KLF4 were aberrant, and both of these were risk factors for the development of URSA (118, 119). We discovered that the lncRNA types HOTAIR and SNHG7 are related to recurrent spontaneous abortion pathogenesis and control trophoblast proliferation, apoptosis, invasion, and chorionic villus angiogenesis (120, 121). These investigations revealed prospective biomarkers and therapeutic targets, offering fresh perspectives on the early detection and management of recurrent spontaneous abortion (122) (Table 1).
Conclusion
Exosomal lncRNAs have a wide range of potential for investigation (123). Exosomal lncRNAs have the power to control a wide range of cellular biological processes, including the recasting of the helical arteries, the inflammatory response, immunological control, cellular metabolism, and autophagy (124–126). Exosomal lncRNAs are more durable and less prone to degradation than serum-derived lncRNAs, allowing them to move unaltered from their “origin” to their “destination” and carry out their intended functions (127–130). Exosomal lncRNAs at the maternal-fetal interface have been shown in numerous studies to play an essential role in pregnancy-specific illnesses and to support embryo implantation and maintenance. Hence, it is necessary to address the issue of how to harvest exosomes that more accurately depict the cellular environment in vivo (126, 131, 132). Pregnancy-specific disorders have been linked to abnormal changes in placenta-derived exosomes seen in the peripheral blood of pregnant women, although larger sample sizes are still required to confirm their utility as biomarkers for clinical testing. Exosomes can also forecast embryonic developmental potential, and shortly, using exosomes as markers in clinical testing will be a promising and significant noninvasive test (133, 134).
Author contributions
MW, LZ, SM, RL, JL, and SY performed literature searches and selected the studies and reviews discussed in the manuscript. The first draft of the manuscript was prepared by MW. LZ, SM, RL, and JL made subsequent amendments. SY revised the manuscript. All authors read and approved the final manuscript and contributed to the conception of this review.
Funding
Funding for this work was provided by the Jilin Provincial Department of Finance Project and Jilin Provincial Development and Reform Commission Health Special Fund (No. 2020SCZT078 and No. 3D5204901429).
Conflict of interest
The authors declare that the research was conducted in the absence of any commercial or financial relationships that could be construed as a potential conflict of interest.
Publisher’s note
All claims expressed in this article are solely those of the authors and do not necessarily represent those of their affiliated organizations, or those of the publisher, the editors and the reviewers. Any product that may be evaluated in this article, or claim that may be made by its manufacturer, is not guaranteed or endorsed by the publisher.
Glossary
References
1. Han J, Zhang Y, Ge P, Dakal TC, Wen H, Tang S, et al. Exosome-derived CIRP: An amplifier of inflammatory diseases. Front Immunol (2023) 14. doi: 10.3389/fimmu.2023.1066721
2. Zhu L, Sun H-T, Wang S, Huang S-L, Zheng Y, Wang C-Q, et al. Isolation and characterization of exosomes for cancer research. J Hematol Oncol (2020) 13(1):152. doi: 10.1186/s13045-020-00987-y
3. Bridges MC, Daulagala AC, Kourtidis A. LNCcation: lncRNA localization and function. J Cell Biol (2021) 220(2):e202009045. doi: 10.1083/jcb.202009045
4. Chen A, Yu R, Jiang S, Xia Y, Chen Y. Recent advances of microRNAs, long non-coding RNAs, and circular RNAs in preeclampsia. Front Physiol (2021) 12. doi: 10.3389/fphys.2021.659638
5. Nojima T, Proudfoot NJ. Mechanisms of lncRNA biogenesis as revealed by nascent transcriptomics (vol 23, pg 389, 2022). Nat Rev Mol Cell Biol (2022) 23(12):853–. doi: 10.1038/s41580-022-00551-1
6. Yang J, Yang FJ, Wang YG, Su GF, Mao X. LncRNA MIR497HG inhibits proliferation and migration of retinal endothelial cells under high-level glucose treatment via miRNA-128-3p/SIRT1 axis. Eur Rev Med Pharmacol Sci (2020) 24(11):5871–7. doi: 10.26355/eurrev_202006_21479
7. Wen SW, Lima LG, Lobb RJ, Norris EL, Hastie ML, Krumeich S, et al. Breast cancer-derived exosomes reflect the cell-of-origin phenotype. Proteomics (2019) 19(8):e1800180. doi: 10.1002/pmic.201800180
8. Vatish M, Powys VR, Cerdeira AS. Novel therapeutic and diagnostic approaches for preeclampsia. Curr Opin Nephrol Hypertension. (2023) 32(2):124–33. doi: 10.1097/MNH.0000000000000870
9. Maligianni I, Yapijakis C, Nousia K, Bacopoulou F, Chrousos GP. Exosomes and exosomal non-coding RNAs throughout human gestation (Review). Exp Ther Med (2022) 24(3):582. doi: 10.3892/etm.2022.11518
10. Zhang T, Tang X, Zhu Y, Wang C, Jiang Z, Yang N, et al. IGF2BP2 enhances LincRNA01116 stability via m (6)A: A potential biomarker and therapeutic target for patients with pre-eclampsia. J Cell Biochem (2023) 124(2):239–53. doi: 10.1002/jcb.30358
11. Khazaei F, Rezakhani L, Alizadeh M, Mahdavian E, Khazaei M. Exosomes and exosome-loaded scaffolds: Characterization and application in modern regenerative medicine. Tissue Cell (2023) 80:102007. doi: 10.1016/j.tice.2022.102007
12. Wang Y, Liu Q, Wang F. Potential roles of exosome non-coding RNAs in cancer chemoresistance. Oncol Rep (2021) 45(2):439–47. doi: 10.3892/or.2020.7887
13. Théry C, Witwer KW, Aikawa E, Alcaraz MJ, Anderson JD, Andriantsitohaina R, et al. Minimal information for studies of extracellular vesicles 2018 (MISEV2018): a position statement of the International Society for Extracellular Vesicles and update of the MISEV2014 guidelines. J Extracell Vesicles. (2018) 7(1):1535750. doi: 10.1080/20013078.2018.1535750
14. Saheera S, Potnuri AG, Krishnamurthy P. Nano-vesicle (Mis)Communication in senescence-related pathologies. Cells (2020) 9(9):1974. doi: 10.3390/cells9091974
15. Kalluri R, LeBleu VS. The biology, function, and biomedical applications of exosomes. Science (2020) 367(6478):640–+. doi: 10.1126/science.aau6977
16. Nafar S, Nouri N, Alipour M, Fallahi J, Zare F, Tabei SMB. Exosome as a target for cancer treatment. J Invest Med (2022) 70(5):1212–8. doi: 10.1136/jim-2021-002194
17. Sun X, Ding T, Wang B, Chang Z, Fei H, Geng L, et al. Identification of lncRNA-miRNA-mRNA networks in circulating exosomes as potential biomarkers for systemic sclerosis. Front Med (2023) 10. doi: 10.3389/fmed.2023.1111812
18. Guo M, Hao Y, Feng Y, Li H, Mao Y, Dong Q, et al. Microglial exosomes in neurodegenerative disease. Front Mol Neurosci (2021) 14. doi: 10.3389/fnmol.2021.630808
19. Negahdaripour M, Vakili B, Nezafat N. Exosome-based vaccines and their position in next-generation vaccines. Int Immunopharmacology. (2022) 113(Pt A):109265. doi: 10.1016/j.intimp.2022.109265
20. Hade MD, Suire CN, Suo Z. Mesenchymal stem cell-derived exosomes: applications in regenerative medicine. Cells (2021) 10(8):1959. doi: 10.3390/cells10081959
21. Roy A, Girija As S, Sankar Ganesh P, Saravanan M, Sunny B. Exosome mediated cancer therapeutic approach: present status and future prospectives. Asian Pacific J Cancer prevention: APJCP. (2023) 24(2):363–73. doi: 10.31557/APJCP.2023.24.2.363
22. Gall AR, Amoah S, Kitase Y, Jantzie LL. Placental mediated mechanisms of perinatal brain injury: Evolving inflammation and exosomes. Exp Neurology. (2022) 347:113914. doi: 10.1016/j.expneurol.2021.113914
23. Ghafourian M, Mahdavi R, Akbari Jonoush Z, Sadeghi M, Ghadiri N, Farzaneh M, et al. The implications of exosomes in pregnancy: emerging as new diagnostic markers and therapeutics targets. Cell Communication Signaling (2022) 20(1):51. doi: 10.1186/s12964-022-00853-z
24. Shi Y, Du L, Lv D, Li Y, Zhang Z, Huang X, et al. Emerging role and therapeutic application of exosome in hepatitis virus infection and associated diseases. J Gastroenterology. (2021) 56(4):336–49. doi: 10.1007/s00535-021-01765-4
25. Ye Z, Wang S, Huang X, Chen P, Deng L, Li S, et al. Plasma exosomal miRNAs associated with metabolism as early predictor of gestational diabetes mellitus. Diabetes (2022) 71(11):2272–83. doi: 10.2337/db21-0909
26. Herman AB, Tsitsipatis D, Gorospe M. Integrated lncRNA function upon genomic and epigenomic regulation. Mol Cell (2022) 82(12):2252–66. doi: 10.1016/j.molcel.2022.05.027
27. Huang W, Li H, Yu Q, Xiao W, Wang DO. LncRNA-mediated DNA methylation: an emerging mechanism in cancer and beyond. J Exp Clin Cancer Res (2022) 41(1):100. doi: 10.1186/s13046-022-02319-z
28. Nunez-Martinez HN, Recillas-Targa F. Emerging Functions of lncRNA Loci beyond the Transcript Itself. Int J Mol Sci (2022) 23(11):6258. doi: 10.3390/ijms23116258
29. An B, Kameda T, Imamura T. The evolutionary acquisition and mode of functions of promoter-associated non-coding RNAs (pancRNAs) for mammalian development. In: Hon CC, editor. Essays Biochem 65(4):697–708. doi: 10.1042/EBC20200143
30. Sanbonmatsu K. Getting to the bottom of lncRNA mechanism: structure-function relationships. Mamm Genome. (2022) 33(2):343–53. doi: 10.1007/s00335-021-09924-x
31. Yang L, Li L-P, Yi H-C. DeepWalk based method to predict lncRNA-miRNA associations via lncRNA-miRNA-disease-protein-drug graph. BMC Bioinf (2022) 22(SUPPL 12):621. doi: 10.1186/s12859-022-04579-0
32. Tsagakis I, Douka K, Birds I, Aspden JL. Long non-coding RNAs in development and disease: conservation to mechanisms. J Pathology. (2020) 250(5):480–95. doi: 10.1002/path.5405
33. Jain N, Gupta P, Sahoo S, Mallick B. Non-coding RNAs and their cross-talks impacting reproductive health of women. Wiley Interdiscip Reviews-Rna. (2022) 13(3):e1695. doi: 10.1002/wrna.1695
34. Farzaneh M, Najafi S, Anbiyaee O, Azizidoost S, Khoshnam SE. LncRNA MALAT1-related signaling pathways in osteosarcoma. Clin Trans Oncol (2023) 25(1):21–32. doi: 10.1007/s12094-022-02876-x
35. Li C, Ni YQ, Xu H, Xiang QY, Zhao Y, Zhan JK, et al. Roles and mechanisms of exosomal non-coding RNAs in human health and diseases. Signal Transduct Target Ther (2021) 6(1):383. doi: 10.1038/s41392-021-00779-x
36. Yang H, Zhang H, Gu H, Wang J, Zhang J, Zen K, et al. Comparative analyses of human exosome proteomes. Protein J (2023) 42(4):365–73. doi: 10.1007/s10930-023-10100-0
37. Jia R, Rotenberg SA, Mirkin MV. Electrochemical resistive-pulse sensing of extracellular vesicles. Analytical Chem (2022) 94(37):12614–20. doi: 10.1021/acs.analchem.2c01216
38. Theodoraki M-N, Hong C-S, Donnenberg VS, Donnenberg AD, Whiteside TL. Evaluation of exosome proteins by on-bead flow cytometry. Cytometry Part A. (2021) 99(4):372–81. doi: 10.1002/cyto.a.24193
39. Kar R, Dhar R, Mukherjee S, Nag S, Gorai S, Mukerjee N, et al. Exosome-based smart drug delivery tool for cancer theranostics. ACS Biomaterials Sci Engineering. (2023) 9(2):577–94. doi: 10.1021/acsbiomaterials.2c01329
40. Peng W, Bai S, Zheng M, Chen W, Li Y, Yang Y, et al. An exosome-related lncRNA signature correlates with prognosis, immune microenvironment, and therapeutic responses in hepatocellular carcinoma. Trans Oncol (2023) 31:101651–. doi: 10.1016/j.tranon.2023.101651
41. Liu H, Huang X, Mor G, Liao A. Epigenetic modifications working in the decidualization and endometrial receptivity. Cell Mol Life Sci (2020) 77(11):2091–101. doi: 10.1007/s00018-019-03395-9
42. Liu C, Li L, Wang M, Shui S, Yao H, Sui C, et al. Endometrial extracellular vesicles of recurrent implantation failure patients inhibit the proliferation, migration, and invasion of HTR8/SVneo cells. J Assisted Reprod Genet (2021) 38(4):825–33. doi: 10.1007/s10815-021-02093-5
43. Hashemi M, Moosavi MS, Abed HM, Dehghani M, Aalipour M, Heydari EA, et al. Long non-coding RNA (lncRNA) H19 in human cancer: From proliferation and metastasis to therapy. Pharmacol Res (2022) 184:106418. doi: 10.1016/j.phrs.2022.106418
44. Kannampuzha S, Ravichandran M, Mukherjee AG, Wanjari UR, Renu K, Vellingiri B, et al. The mechanism of action of non-coding RNAs in placental disorders. Biomedicine Pharmacotherapy (2022) 156:113964. doi: 10.1016/j.biopha.2022.113964
45. Gurunathan S, Kang M-H, Song H, Kim NH, Kim J-H. The role of extracellular vesicles in animal reproduction and diseases. J Anim Sci Biotechnol (2022) 13(1):62. doi: 10.1186/s40104-022-00715-1
46. Chen F, Chen X, Cai W, Ruan H, Fang S. Mesenchymal Stem Cell-Derived Exosomal Long Noncoding RNA MALAT1-201 Regulated the Proliferation, Apoptosis and Migration of Trophoblast Cells via Targeting miR-141. Ann Clin Lab science. (2022) 52(5):741–52.
47. Gauster M, Moser G, Wernitznig S, Kupper N, Huppertz B. Early human trophoblast development: from morphology to function. Cell Mol Life Sci (2022) 79(6):345. doi: 10.1007/s00018-022-04377-0
48. Aljubran F, Nothnick WB. Long non-coding RNAs in endometrial physiology and pathophysiology. Mol Cell Endocrinol (2021) 525:111190. doi: 10.1016/j.mce.2021.111190
49. Monsivais D, Nagashima T, Prunskaite-Hyyrylainen R, Nozawa K, Shimada K, Tang S, et al. Endometrial receptivity and implantation require uterine BMP signaling through an ACVR2A-SMAD1/SMAD5 axis. Nat Commun (2021) 12(1):3386. doi: 10.1038/s41467-021-23571-5
50. Hu L, Ma J, Cao M, Lin Y, Long W, Shi Z, et al. Exosomal mRNA and lncRNA profiles in cord blood of preeclampsia patients. J Maternal-Fetal Neonatal Med (2022) 35(25):8199–209. doi: 10.1080/14767058.2021.1966413
51. Ren J, Jin H, Zhu Y. The role of placental non-coding RNAs in adverse pregnancy outcomes. Int J Mol Sci (2023) 24(5):5030. doi: 10.3390/ijms24055030
52. Giacomini E, Vago R, Sanchez AM, Podini P, Zarovni N, Murdica V, et al. Secretome of in vitro cultured human embryos contains extracellular vesicles that are uptaken by the maternal side. Sci Rep (2017) 7(1):5210. doi: 10.1038/s41598-017-05549-w
53. Jin J, Menon R. Placental exosomes: A proxy to understand pregnancy complications. Am J Reprod Immunol (2018) 79(5):e12788. doi: 10.1111/aji.12788
54. Barbaro G, Inversetti A, Cristodoro M, Ticconi C, Scambia G, Di Simone N. HLA-G and recurrent pregnancy loss. Int J Mol Sci (2023) 24(3):2557. doi: 10.3390/ijms24032557
55. Chen T, Liu D. The mystery of exosomes in gestational diabetes mellitus. Oxid Med Cell Longevity. (2022) 2022:2169259. doi: 10.1155/2022/2169259
56. Chappell LC, Cluver CA, Kingdom J, Tong S. Pre-eclampsia. Lancet (2021) 398(10297):341–54. doi: 10.1016/S0140-6736(20)32335-7
57. Gestational hypertension and preeclampsia: ACOG practice bulletin summary, number 222. Obstet Gynecol (2020) 135(6):1492–5. doi: 10.1097/AOG.0000000000003892
58. Mo H-Q, Tian F-J, Ma X-L, Zhang Y-C, Zhang C-X, Zeng W-H, et al. PDIA3 regulates trophoblast apoptosis and proliferation in preeclampsia via the MDM2/p53 pathway. Reproduction (2020) 160(2):293–305. doi: 10.1530/REP-20-0156
59. Raguema N, Moustadraf S, Bertagnolli M. Immune and apoptosis mechanisms regulating placental development and vascularization in preeclampsia. Front Physiol (2020) 11. doi: 10.3389/fphys.2020.00098
60. Farah O, Nguyen C, Tekkatte C, Parast MM. Trophoblast lineage-specific differentiation and associated alterations in preeclampsia and fetal growth restriction. Placenta (2020) 102:4–9. doi: 10.1016/j.placenta.2020.02.007
61. Peixoto-Filho FM, Costa F, Kobayashi S, Beitune PE, Garrido AG, Carmo AV, et al. Prediction and prevention of preeclampsia. Rev Bras ginecologia e obstetricia: Rev da Federacao Bras das Sociedades Ginecologia e Obstetricia. (2023) 45(1):49–54. doi: 10.1055/s-0043-1763495
62. Zhang T-N, Wang W, Huang X-M, Gao S-Y. Non-coding RNAs and extracellular vehicles: their role in the pathogenesis of gestational diabetes mellitus. Front Endocrinol (2021) 12. doi: 10.3389/fendo.2021.664287
63. Zhang Y, Chen X. lncRNA FOXD2-AS1 affects trophoblast cell proliferation, invasion and migration through targeting miRNA. Zygote (2020) 28(2):131–8. doi: 10.1017/S0967199419000807
64. Jing M, Chen X, Qiu H, He W, Zhou Y, Li D, et al. Insights into the immunomodulatory regulation of matrix metalloproteinase at the maternal-fetal interface during early pregnancy and pregnancy-related diseases. Front Immunol (2023) 13. doi: 10.3389/fimmu.2022.1067661
65. Mytareli C, Delivanis DA, Athanassouli F, Kalotychou V, Mantzourani M, Kassi E, et al. The diagnostic, prognostic and therapeutic role of miRNAs in adrenocortical carcinoma: A systematic review. Biomedicines (2021) 9(11):1501. doi: 10.3390/biomedicines9111501
66. Feng C, Cheng L, Jin J, Liu X, Wang F. Long non-coding RNA MALAT1 regulates trophoblast functions through VEGF/VEGFR1 signaling pathway. Arch Gynecology Obstetrics. (2021) 304(4):873–82. doi: 10.1007/s00404-021-05987-y
67. Chen H, Meng T, Liu X, Sun M, Tong C, Liu J, et al. Long non-coding RNA MALAT-1 is downregulated in preeclampsia and regulates proliferation, apoptosis, migration and invasion of JEG-3 trophoblast cells. Int J Clin Exp Pathology. (2015) 8(10):12718–27.
68. Tao H, Liu X, Liu X, Liu W, Wu D, Wang R, et al. LncRNA MEG3 inhibits trophoblast invasion and trophoblast-mediated VSMC loss in uterine spiral artery remodeling. Mol Reprod Dev (2019) 86(6):686–95. doi: 10.1002/mrd.23147
69. Li X, Song Y, Liu F, Liu D, Miao H, Ren J, et al. Long non-coding RNA MALAT1 promotes proliferation, angiogenesis, and immunosuppressive properties of mesenchymal stem cells by inducing VEGF and IDO. J Cell Biochem (2017) 118(9):2780–91. doi: 10.1002/jcb.25927
70. Wu H-Y, Wang X-H, Liu K, Zhang J-L. LncRNA MALAT1 regulates trophoblast cells migration and invasion via miR-206/IGF-1 axis. Cell Cycle (2020) 19(1):39–52. doi: 10.1080/15384101.2019.1691787
71. Liu J, Zhang X, Cheng Y, Cao X. Dendritic cell migration in inflammation and immunity. Cell Mol Immunol (2021) 18(11):2461–71. doi: 10.1038/s41423-021-00726-4
72. Zhang W, Zhou Y, Ding Y. Lnc-DC mediates the over-maturation of decidual dendritic cells and induces the increase in Th1 cells in preeclampsia. Am J Reprod Immunol (2017) 77(6). doi: 10.1111/aji.12647
73. Yang J, Qi M, Fei X, Wang X, Wang K. LncRNA H19: A novel oncogene in multiple cancers. Int J Biol Sci (2021) 17(12):3188–208. doi: 10.7150/ijbs.62573
74. Xu J, Xia Y, Zhang H, Guo H, Feng K, Zhang C. Overexpression of long non-coding RNA H19 promotes invasion and autophagy via the PI3K/AKT/mTOR pathways in trophoblast cells. Biomedicine Pharmacotherapy. (2018) 101:691–7. doi: 10.1016/j.biopha.2018.02.134
75. Gao W-l, Liu M, Yang Y, Yang H, Liao Q, Bai Y, et al. The imprinted H19 gene regulates human placental trophoblast cell proliferation via encoding miR-675 that targets Nodal Modulator 1 (NOMO1). RNA Biol (2012) 9(7):1002–10. doi: 10.4161/rna.20807
76. Zheng D, Hou Y, Li Y, Bian Y, Khan M, Li F, et al. Long non-coding RNA Gas5 is associated with preeclampsia and regulates biological behaviors of trophoblast via microRNA-21 (vol 11, 188, 2020). Front Genet (2022) 12. doi: 10.3389/fgene.2020.00188
77. Jiang S, Chen Q, Liu H, Gao Y, Yang X, Ren Z, et al. Preeclampsia-associated lncRNA INHBA-AS1 regulates the proliferation, invasion, and migration of placental trophoblast cells. Mol Therapy-Nucleic Acids (2020) 22:684–95. doi: 10.1016/j.omtn.2020.09.033
78. Song X, Zhang X, Xia Q, Li C, Zhang Y, Huang Y, et al. LncRNA LINC00534 regulates cell proliferation and migration via the miR-494-3p/PTEN axis in HTR-8/SVneo cells. J Clin Lab Anal (2023) 37(1):e24802. doi: 10.1002/jcla.24802
79. American Diabetes Association. 2. Classification and diagnosis of diabetes: standards of medical care in diabetes-2021. Diabetes Care (2021) 44:S15–33. doi: 10.2337/dc21-S002
80. Tang L, Li P, Li L. Whole transcriptome expression profiles in placenta samples from women with gestational diabetes mellitus. J Diabetes Invest (2020) 11(5):1307–17. doi: 10.1111/jdi.13250
81. Ormazabal V, Nair S, Carrion F, McIntyre HD, Salomon C. The link between gestational diabetes and cardiovascular diseases: potential role of extracellular vesicles. Cardiovasc Diabetology. (2022) 21(1):174. doi: 10.1186/s12933-022-01597-3
82. Alejandro EU, Mamerto TP, Chung G, Villavieja A, Gaus NL, Morgan E, et al. Gestational diabetes mellitus: A harbinger of the vicious cycle of diabetes. Int J Mol Sci (2020) 21(14):5003. doi: 10.3390/ijms21145003
83. Reyes AB, Burger D. Small extracellular vesicles: a new player in GDM pathogenesis. Clin Science. (2022) 136(24):1873–5. doi: 10.1042/CS20220658
84. Filardi T, Catanzaro G, Mardente S, Zicari A, Santangelo C, Lenzi A, et al. Non-coding RNA: role in gestational diabetes pathophysiology and complications. Int J Mol Sci (2020) 21(11):4020. doi: 10.3390/ijms21114020
85. Yuan Y, Li Y, Hu L, Wen J. Exosomal RNA expression profiles and their prediction performance in patients with gestational diabetes mellitus and macrosomia. Front Endocrinol (2022) 13. doi: 10.3389/fendo.2022.864971
86. Mitra T, Gulati R, Uppal A, Kumari SR, Tripathy S, Ranjan P, et al. Prospecting of exosomal-miRNA signatures as prognostic marker for gestational diabetes mellitus and other adverse pregnancy outcomes. Front Endocrinol (2023) 14. doi: 10.3389/fendo.2023.1097337
87. Bernea EG, Suica VI, Uyy E, Cerveanu-Hogas A, Boteanu RM, Ivan L, et al. Exosome proteomics reveals the deregulation of coagulation, complement and lipid metabolism proteins in gestational diabetes mellitus. Molecules (2022) 27(17):5502. doi: 10.3390/molecules27175502
88. Ma L, Gao J, Zhang N, Wang J, Xu T, Lei T, et al. Long noncoding RNA SNHG17: a novel molecule in human cancers. Cancer Cell Int (2022) 22(1):104. doi: 10.1186/s12935-022-02529-7
89. Wang C, Qu Y, Wang D, Zhu Y. The proangiogenic roles of long nonCoding RNAs revealed by RNA-sequencing following oxygen-glucose deprivation/re-oxygenation. Cell Physiol biochemistry: Int J Exp Cell physiology biochemistry Pharmacol (2019) 52(4):708–27. doi: 10.33594/000000050
90. Li J, Du B, Geng X, Zhou L. lncRNA SNHG 17 is downregulated in gestational diabetes mellitus (GDM) and has predictive values. Diabetes Metab Syndrome Obesity-Targets Ther (2021) 14:831–8. doi: 10.2147/DMSO.S263942
91. Zhang Y, Wu H, Wang F, Ye M, Zhu H, Bu S. Long non-coding RNA MALAT1 expression in patients with gestational diabetes mellitus. Int J Gynecol Obstet. (2018) 140(2):164–9. doi: 10.1002/ijgo.12384
92. Liu P, Jia S-B, Shi J-M, Li W-J, Tang L-S, Zhu X-H, et al. LncRNA-MALAT1 promotes neovascularization in diabetic retinopathy through regulating miR-125b/VE-cadherin axis. Bioscience Rep (2019) 39(5):BSR20181469. doi: 10.1042/BSR20181469
93. Hocaoglu M, Demirer S, Loclar Karaalp I, Kaynak E, Attar E, Turgut A, et al. Identification of miR-16-5p and miR-155-5p microRNAs differentially expressed in circulating leukocytes of pregnant women with polycystic ovary syndrome and gestational diabetes. Gynecological Endocrinology. (2021) 37(3):216–20. doi: 10.1080/09513590.2020.1843620
94. Ye HH, Yang SH, Zhang Y. MEG3 damages fetal endothelial function induced by gestational diabetes mellitus via AKT pathway. Eur Rev Med Pharmacol Sci (2018) 22(24):8553–60. doi: 10.26355/eurrev_201812_16617
95. Zhang H. Mechanism associated with aberrant lncRNA MEG3 expression in gestational diabetes mellitus. Exp Ther Med (2019) 18(5):3699–706. doi: 10.3892/etm.2019.8062
96. Song G, Na Q, Wang R, Wang D, Chen B, Li Y, et al. LncRNA-SNX17 promotes HTR-8/SVneo proliferation and invasion through miR-517a/IGF-1 in the placenta of diabetic macrosomia. Reprod Sci (2022) 29(2):596–605. doi: 10.1007/s43032-021-00687-z
97. Al-Ghazali MJ, Ali HA, Al-Rufaie MM. Serum irisin levels as a potential marker for diagnosis of gestational diabetes mellitus. Acta bio-medica: Atenei Parmensis. (2020) 91(1):56–63. doi: 10.23750/abm.v91i1.7675
98. Fu X, Cong H, Zhao S, Li Y, Liu T, Sun Y, et al. Construction of Glycometabolism- and Hormone-Related lncRNA-Mediated Feedforward Loop Networks Reveals Global Patterns of lncRNAs and Drug Repurposing in Gestational Diabetes. Front Endocrinol (2020) 11. doi: 10.3389/fendo.2020.00093
99. Li Y, Li D, Cheng X. The association between expression of lncRNAs in patients with GDM. Endocrine Connections. (2021) 10(9):1080–90. doi: 10.1530/EC-21-0227
100. Youssef A, Vermeulen N, Lashley EELO, Goddijn M, van der Hoorn MLP. Comparison and appraisal of (inter)national recurrent pregnancy loss guidelines. Reprod Biomedicine Online. (2019) 39(3):497–503. doi: 10.1016/j.rbmo.2019.04.008
101. Andreescu M, Frincu F, Plotogea M, Mehedintu C. Recurrent abortion and the involvement of killer-cell immunoglobulin-like receptor (KIR) genes, activated T cells, NK abnorMalities, and cytokine profiles. J Clin Med (2023) 12(4):1355. doi: 10.3390/jcm12041355
102. Deng T, Liao X, Zhu S. Recent advances in treatment of recurrent spontaneous abortion. Obstetrical Gynecological Survey. (2022) 77(6):355–66. doi: 10.1097/OGX.0000000000001033
103. Menon R, Shahin H. Extracellular vesicles in spontaneous preterm birth. Am J Reprod Immunol (2021) 85(2):e13353. doi: 10.1111/aji.13353
104. Young SL. Introduction: Reproductive immunology: checkered past and bright future. Fertility Sterility. (2016) 106(3):497–8. doi: 10.1016/j.fertnstert.2016.07.1090
105. Gan J, Gu T, Yang H, Ao Z, Cai G, Hong L, et al. Non-coding RNAs regulate spontaneous abortion: A global network and system perspective. Int J Mol Sci (2022) 23(8):4214. doi: 10.3390/ijms23084214
106. Chen X, Guo D-Y, Yin T-L, Yang J. Non-coding RNAs regulate placental trophoblast function and participate in recurrent abortion. Front Pharmacol (2021) 12. doi: 10.3389/fphar.2021.646521
107. Jain AK, Xi Y, McCarthy R, Allton K, Akdemir KC, Patel LR, et al. LncPRESS1 is a p53-regulated lncRNA that safeguards pluripotency by disrupting SIRT6-mediated de-acetylation of histone H3K56. Mol Cell (2016) 64(5):967–81. doi: 10.1016/j.molcel.2016.10.039
108. Ye B, Liu B, Yang L, Zhu X, Zhang D, Wu W, et al. LncKdm2b controls self-renewal of embryonic stem cells via activating expression of transcription factor Zbtb3. EMBO J (2018) 37(8):e97174. doi: 10.15252/embj.201797174
109. Hamazaki N, Uesaka M, Nakashima K, Agata K, Imamura T. Gene activation-associated long noncoding RNAs function in mouse preimplantation development. Development (2015) 142(5):910–20. doi: 10.1242/dev.116996
110. Zhang Y, Wang S. The possible role of long non-coding RNAs in recurrent miscarriage. Mol Biol Rep (2022) 49(10):9687–97. doi: 10.1007/s11033-022-07427-9
111. Yang Y, Xiong Y, Pan Z. Role of ceRNAs in non-tumor female reproductive diseases(dagger). Biol Reprod (2022) 108(3):363–81. doi: 10.1093/biolre/ioac200
112. Fan L-j, Han H-j, Guan J, Zhang X-w, Cui Q-h, Shen H, et al. Aberrantly expressed long noncoding RNAs in recurrent implantation failure: A microarray related study. Syst Biol Reprod Med (2017) 63(4):269–78. doi: 10.1080/19396368.2017.1310329
113. He D, Zeng H, Chen J, Xiao L, Zhao Y, Liu N. H19 regulates trophoblastic spheroid adhesion by competitively binding to let-7. Reproduction (2019) 157(5):423–30. doi: 10.1530/REP-18-0339
114. Wang Y, Liu H-Z, Liu Y, Wang H-J, Pang W-W, Zhang J-J. Disordered p53-MALAT1 pathway is associated with recurrent miscarriage. Kaohsiung J Med Sci (2019) 35(2):87–94. doi: 10.1002/kjm2.12013
115. Wang Q, Lu X, Li C, Zhang W, Lv Y, Wang L, et al. Down-regulated long non-coding RNA PVT1 contributes to gestational diabetes mellitus and preeclampsia via regulation of human trophoblast cells. Biomedicine Pharmacotherapy. (2019) 120:109501. doi: 10.1016/j.biopha.2019.109501
116. Zhao H. The role of long-stranded non-coding RNA in maternal-fetal immune tolerance and recurrent miscarriage. Shandong University, Shandong, China (2021).
117. Huang Z, Du G, Huang X, Han L, Han X, Xu B, et al. The enhancer RNA lnc-SLC4A1-1 epigenetically regulates unexplained recurrent pregnancy loss (URPL) by activating CXCL8 and NF-kB pathways. Ebiomedicine (2018) 38:162–70. doi: 10.1016/j.ebiom.2018.11.015
118. Sawant L, Wijesekera N, Jones C. Pioneer transcription factors, progesterone receptor, and Kruppel like transcription factor 4, cooperatively stimulate the bovine herpesvirus 1 ICP0 early promoter and productive late protein expression. Virus Res (2020) 288:198115. doi: 10.1016/j.virusres.2020.198115
119. Huang Y, Hao J, Liao Y, Zhou L, Wang K, Zou H, et al. Transcriptome sequencing identified the ceRNA network associated with recurrent spontaneous abortion. BMC Med Genomics (2021) 14(1):278. doi: 10.1186/s12920-021-01125-4
120. Xiang H, Yan H, Sun B, Feng F, Chen P. Decreased expression of long non-coding RNA SNHG7 cause recurrent spontaneous abortion through suppression proliferation and invasion of trophoblast cells via miR-34a. Am J Trans Res (2019) 11(1):463–72.
121. Zhou H, Gao L, Yu Z-H, Hong S-J, Zhang Z-W, Qiu Z-Z. LncRNA HOTAIR promotes renal interstitial fibrosis by regulating Notch1 pathway via the modulation of miR-124. Nephrology (2019) 24(4):472–80. doi: 10.1111/nep.13394
122. Soltani N, Mirzaei F, Ayatollahi H. Cytogenetic studies of 608 couples with recurrent spontaneous abortions in northeastern Iran. Iranian J pathology. (2021) 16(4):418–25. doi: 10.30699/ijp.2021.521514.2554
123. Rezaie J, Feghhi M, Etemadi T. A review on exosomes application in clinical trials: perspective, questions, and challenges. Cell Commun Signal (2022) 20(1):145. doi: 10.1186/s12964-022-00959-4
124. Nikfarjam S, Rezaie J, Zolbanin NM, Jafari R. Mesenchymal stem cell derived-exosomes: a modern approach in translational medicine. J Transl Med (2020) 18(1):449. doi: 10.1186/s12967-020-02622-3
125. Nemati M, Singh B, Mir RA, Nemati M, Babaei A, Ahmadi M, et al. Plant-derived extracellular vesicles: a novel nanomedicine approach with advantages and challenges. Cell Commun Signal (2022) 20(1):69. doi: 10.1186/s12964-022-00889-1
126. Vahabi A, Rezaie J, Hassanpour M, Panahi Y, Nemati M, Rasmi Y, et al. Tumor Cells-derived exosomal CircRNAs: Novel cancer drivers, molecular mechanisms, and clinical opportunities. Biochem Pharmacol (2022) 200:115038. doi: 10.1016/j.bcp.2022.115038
127. Joo HS, Suh JH, Lee HJ, Bang ES, Lee JM. Current knowledge and future perspectives on mesenchymal stem cell-derived exosomes as a new therapeutic agent. Int J Mol Sci (2020) 21(3):727. doi: 10.3390/ijms21030727
128. Rezaie J, Ahmadi M, Ravanbakhsh R, Mojarad B, Mahbubfam S, Shaban SA, et al. Tumor-derived extracellular vesicles: The metastatic organotropism drivers. Life Sci (2022) 289:120216. doi: 10.1016/j.lfs.2021.120216
129. Rezaie J, Etemadi T, Feghhi M. The distinct roles of exosomes in innate immune responses and therapeutic applications in cancer. Eur J Pharmacol (2022) 933:175292. doi: 10.1016/j.ejphar.2022.175292
130. Almohammai A, Rahbarghazi R, Keyhanmanesh R, Rezaie J, Ahmadi M. Asthmatic condition induced the activity of exosome secretory pathway in rat pulmonary tissues. J Inflammation (Lond). (2021) 18(1):14. doi: 10.1186/s12950-021-00275-7
131. Rahbarghazi R, Jabbari N, Sani NA, Asghari R, Salimi L, Kalashani SA, et al. Tumor-derived extracellular vesicles: reliable tools for Cancer diagnosis and clinical applications. Cell Commun Signal (2019) 17(1):73. doi: 10.1186/s12964-019-0390-y
132. Pegtel DM, Gould SJ. Exosomes. Annu Rev Biochem. (2019) 88:487–514. doi: 10.1146/annurev-biochem-013118-111902
133. Shaban SA, Rezaie J, Nejati V. Exosomes derived from senescent endothelial cells contain distinct pro-angiogenic miRNAs and proteins. Cardiovasc Toxicol (2022) 22(6):592–601. doi: 10.1007/s12012-022-09740-y
Keywords: exosome lncRNA, pregnancy, pre-eclampsia, diabetes, gestational, abortion, biomarkers
Citation: Wang M, Zheng L, Ma S, Lin R, Li J and Yang S (2023) Biogenesis and function of exosome lncRNAs and their role in female pathological pregnancy. Front. Endocrinol. 14:1191721. doi: 10.3389/fendo.2023.1191721
Received: 22 March 2023; Accepted: 21 August 2023;
Published: 07 September 2023.
Edited by:
Fengxiang Wei, Shenzhen Longgang District Maternal and Child Health Care Hospital, ChinaCopyright © 2023 Wang, Zheng, Ma, Lin, Li and Yang. This is an open-access article distributed under the terms of the Creative Commons Attribution License (CC BY). The use, distribution or reproduction in other forums is permitted, provided the original author(s) and the copyright owner(s) are credited and that the original publication in this journal is cited, in accordance with accepted academic practice. No use, distribution or reproduction is permitted which does not comply with these terms.
*Correspondence: Shuli Yang, eWFuZ3NsQGpsdS5lZHUuY24=