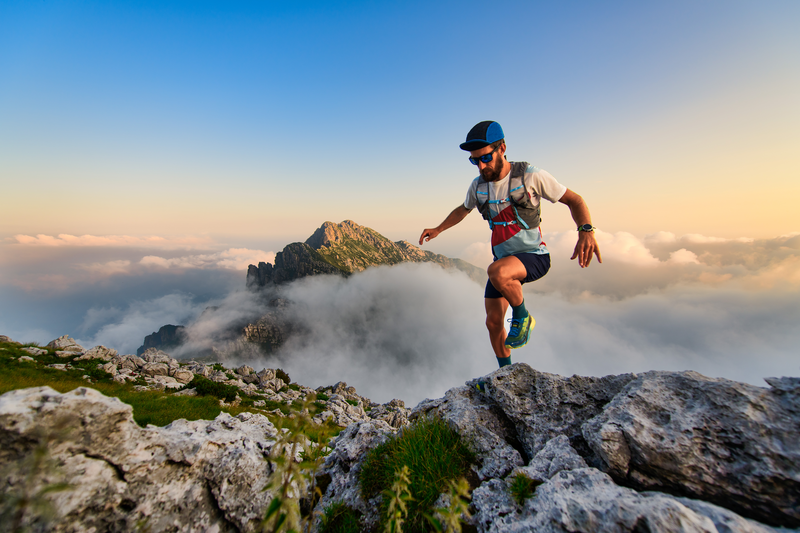
95% of researchers rate our articles as excellent or good
Learn more about the work of our research integrity team to safeguard the quality of each article we publish.
Find out more
SYSTEMATIC REVIEW article
Front. Endocrinol. , 16 August 2023
Sec. Reproduction
Volume 14 - 2023 | https://doi.org/10.3389/fendo.2023.1186068
This article is part of the Research Topic Exploring the Optimal Endometrial Preparation Protocol for Frozen-Thawed Embryo Transfer View all 12 articles
Objective: To investigate the effect of embryo stage at the time of transfer on obstetric and perinatal outcomes in programmed frozen-thawed embryo transfer (FET) versus natural FET cycles.
Design: Systematic review and meta-analysis.
Setting: Not applicable.
Patient(s): Women with programmed frozen-thawed embryo transfer (FET) and natural FET.
Intervention(s): The PubMed, MEDLINE, and EMBASE databases and the Cochrane Central Register of Controlled Trials (CCRT) were searched from 1983 to October 2022. Twenty-three observational studies were included.
Primary outcome measure: The primary outcomes were hypertensive disorders of pregnancy (HDPs), gestational hypertension and preeclampsia (PE). The secondary outcomes were gestational diabetes mellitus (GDM), placenta previa, postpartum haemorrhage (PPH), placental abruption, preterm premature rupture of membranes (PPROM), large for gestational age (LGA), small for gestational age (SGA), macrosomia, and preterm delivery (PTD).
Result(s): The risk of HDP (14 studies, odds ratio (OR) 2.17; 95% confidence interval (CI) 1.95-2.41; P<0.00001; I2 = 43%), gestational hypertension (11 studies, OR 1.38; 95% CI 1.15-1.66; P=0.0006; I2 = 19%), PE (12 studies, OR 2.09; 95% CI 1.88-2.32; P<0.00001; I2 = 0%), GDM (20 studies, OR 1.09; 95% CI 1.02-1.17; P=0.02; I2 = 8%), LGA (18 studies, OR 1.11; 95% CI 1.07-1.15; P<0.00001; I2 = 46%), macrosomia (12 studies, OR 1.15; 95% CI 1.07-1.24; P=0.0002; I2 = 31%), PTD (22 studies, OR 1.21; 95% CI 1.15-1.27; P<0.00001; I2 = 49%), placenta previa (17 studies, OR 1.2; 95% CI 1.02-1.41; P=0.03; I2 = 11%), PPROM (9 studies, OR 1.19; 95% CI 1.02-1.39; P=0.02; I2 = 40%), and PPH (12 studies, OR 2.27; 95% CI 2.02-2.55; P <0.00001; I2 = 55%) were increased in programmed FET cycles versus natural FET cycles with overall embryo transfer. Blastocyst transfer had a higher risk of HDP (6 studies, OR 2.48; 95% CI 2.12-2.91; P<0.00001; I2 = 39%), gestational hypertension (5 studies, OR 1.87; 95% CI 1.27-2.75; P=0.002; I2 = 25%), PE (6 studies, OR 2.23; 95% CI 1.93-2.56; P<0.00001; I2 = 0%), GDM (10 studies, OR 1.13; 95% CI 1.04-1.23; P=0.005; I2 = 39%), LGA (6 studies, OR 1.14; 95% CI 1.07-1.21; P<0.0001; I2 = 9%), macrosomia (4 studies, OR 1.15; 95% CI 1.05-1.26; P<0.002; I2 = 68%), PTD (9 studies, OR 1.43; 95% CI 1.31-1.57; P<0.00001; I2 = 22%), PPH (6 studies, OR 1.92; 95% CI 1.46-2.51; P<0.00001; I2 = 55%), and PPROM (4 studies, OR 1.45; 95% CI 1.14-1.83; P=0.002; I2 = 46%) in programmed FET cycles than in natural FET cycles. Cleavage-stage embryo transfers revealed no difference in HDPs (1 study, OR 0.81; 95% CI 0.32-2.02; P=0.65; I2 not applicable), gestational hypertension (2 studies, OR 0.85; 95% CI 0.48-1.51; P=0.59; I2 = 0%), PE (1 study, OR 1.19; 95% CI 0.58-2.42; P=0.64; I2not applicable), GDM (3 study, OR 0.79; 95% CI 0.52-1.20; P=0.27; I2 = 21%), LGA (1 study, OR 1.15; 95% CI 0.62-2.11; P=0.66; I2not applicable), macrosomia (1 study, OR 1.22; 95% CI 0.54-2.77; P=0.64; I2 not applicable), PTD (2 studies, OR 1.05; 95% CI 0.74-1.49; P=0.79; I2 = 0%), PPH (1 study, OR 1.49; 95% CI 0.85-2.62; P=0.17; I2not applicable), or PPROM (2 studies, OR 0.74; 95% CI 0.46-1.21; P=0.23; I2 = 0%) between programmed FET cycles and natural FET cycles.
Conclusion(s): The risks of HDPs, gestational hypertension, PE, GDM, LGA, macrosomia, SGA, PTD, placenta previa, PPROM, and PPH were increased in programmed FET cycles versus natural FET cycles with overall embryo transfer and blastocyst transfer, but the risks were not clear for cleavage-stage embryo transfer.
Frozen-thawed embryo transfer (FET) has increased dramatically since the first successful human pregnancy in 1983. This strategy enables the use of preimplantation genetic diagnosis/screening, facilitates fertility preservation, and reduces ovarian hyperstimulation syndrome in clinical practice (1). Compelling data have shown that FET results in a higher live birth rate than fresh embryo transfer. However, it was coupled with an increased risk for obstetric and perinatal complications (2). A meta-analysis performed by Roque et al. (2019) with 11 studies reported an increased risk of preeclampsia (PE) in pregnant women following FET compared with fresh ET (3). A pivotal multicentre RCT revealed a 3.13-fold increased risk of PE and a 1.6-fold increased risk of large for gestational age (LGA) in FET compared with fresh ET (4). To date, the reason FET leads to elevated obstetric and perinatal complications is unknown and possibly multifactorial. Recently, researchers proposed that the absence of the corpus luteum (CL) in the programmed endometrial preparation protocol, which is commonly used for FET, may be one potential contributor. Indeed, von Versen-Höynck et al. showed for the first time that the programmed FET cycle (0 CL) was associated with higher rates of preeclampsia and preeclampsia with severe features than the natural FET cycle (1 CL) (5). A meta-analysis including 9 studies reported a significant increase in hypertensive disorders of pregnancy (HDPs), PE, postpartum haemorrhage (PPH), placenta previa and preterm premature rupture of membranes (PPROM) following programmed FET cycles versus natural FET cycles (6). However, the abovementioned studies did not specify the embryo stage at the time of transfer, which may influence the pregnancy outcomes. Indeed, increased risks of preterm birth, LGA and perinatal mortality (7), as well as placenta-related diseases, including placenta previa, placental abruption and pregnancy-induced hypertension (8, 9), have been observed following blastocyst transfers versus cleavage-stage embryo transfers. However, another study reported that the embryo stage at transfer has a neutral effect on obstetric and perinatal outcomes (10). Therefore, we conducted a review and meta-analysis to compare the obstetric and perinatal outcomes of programmed FET cycles and natural FETs according to the type of embryo stage at the time of transfer (cleavage stage or blastocyst stage).
An electronic search of literature was performed from 1983 to November 2022 in the database of PubMed, MEDLINE, EMBASE and Cochrane Central Register of Controlled Trials (CCRT), with the following search terms: (‘frozen embryo transfer’ OR ‘frozen-thawed embryo transfer’ OR ‘FET’ OR ‘vitrified-warmed embryo transfer’ OR ‘frozen blastocyst transfer’ OR ‘frozen cleavage-stage transfer’ OR ‘D5-6 frozen embryo transfer’ OR ‘D2-3 frozen embryo transfer’ OR ‘programmed frozen embryo transfer’ OR ‘natural frozen embryo transfer cycle’ OR ‘endometrial preparation protocols’ OR ‘hormone replacement therapy’ OR ‘artificial frozen-thawed embryo’ OR ‘corpus luteum’) AND (‘obstetric complication’ OR ‘pregnancy complication’ OR ‘perinatal complication’ OR ‘neonatal complication’ OR ‘preterm birth’ OR ‘gestational hypertension’ OR ‘preeclampsia’ OR ‘hypertensive disorders of pregnancy’ OR ‘Pregnancy induced hypertension’ OR ‘post-partum hemorrhage’ OR ‘placenta previa’ OR ‘placental abruption’ OR ‘post-term birth’ OR ‘gestational diabetes mellitus’ OR ‘premature rupture of membranes’ OR ‘macrosomia’ OR ‘large for gestational age’ OR ‘small for gestational age’). Articles in the reference lists that met the inclusion criteria were also searched manually.Twenty-three observational studies that comparing obstetric and/or perinatal outcomes between programmed FET cycles and natural FET cycles were included (Flow chart of studies).
Studies were included if they met the following criteria: (i) the study had at least two cohorts including programmed cycle FET versus natural FET cycle, and (ii) the study reported the obstetric and/or perinatal outcomes following programmed cycle FET versus natural FET cycle.
Three authors independently screened each the title and abstract of each. Full-text articles were read if the study met the inclusion criteria. Then, they extracted data with a standard extraction form. Details for the data extraction are shown in Table 1. Three o other authors independently assessed the risk of bias of the included studies using the Newcastle-Ottawa Scale (NOS) in three domains: selection of study groups, comparability of groups and ascertainment of exposure. Detailed scores are shown in Table 2. A discussion was conducted with a third author if there was any disagreement.
The primary outcomes were hypertensive disorders of pregnancy (HDPs), gestational hypertension and preeclampsia. The secondary outcomes were as follows: gestational diabetes mellitus (GDM), placenta previa, postpartum haemorrhage (PPH), placental abruption, preterm premature rupture of membranes (PPROM), large for gestational age (LGA), small for gestational age (SGA), macrosomia, and preterm delivery.
Statistical analyses were performed using Review Manager 5.3 (Nordic Cochrane Centre, Cochrane Collaboration). Dichotomous outcomes are presented as odds ratios (ORs) with 95% confidence intervals (CIs). We used the Mantel-Haenszel method and fixed-effects model to estimate the pooled effect of variables. Heterogeneity was assessed by the I-squared statistic (I2). When I2 > 50%, sensitivity analysis was applied to identify the sources of heterogeneity by excluding studies one by one. Differences were considered significant at P < 0.05.
The initial literature search identified a total of 3788 potentially relevant publications. After the titles and abstracts were thoroughly screened by two investigators independently, the full-text articles of 126 potential studies were selected for further review. Finally, 23 retrospective studies that met the inclusion criteria were included in this meta-analysis. The flow diagram of the selection procedure is presented in the flow chart of studies. The study characteristics are detailed in Table 1. The overall outcomes in the current study are presented in Table 3.
Table 3 Results of the overall outcomes comparing natural and programmed cycle FET according to embryo stage at the time of transfer.
Fourteen studies reported HDPs, including 21769 natural cycles and 13666 programmed cycles. The risk of HDP was significantly higher in programmed FET cycles (OR=2.17, 95% confidence interval (CI): 1.95-2.41, P<0.00001, I2 =43%). A subgroup analysis was performed to evaluate the effect of embryo stage at the time of transfer. With the use of blastocysts, there was an increased risk of HDP (OR 2.48, 95% CI 2.12-2.91, P <0.00001, I2 =39%) in programmed FET cycles compared with natural FET cycles. Only one study evaluated HDP for cleavage-stage embryo transfer and reported a non-significant increase in HDP in programmed FET cycles compared with natural FET cycles (OR=1.81, 95% CI: 0.32-2.02, P =0.65, I2 not applicable) (Figure 1; Supplementary Figure S1).
Nine studies including 9783 natural FET cycles and 7241 programmed FET cycles provided information on gestational hypertension. Compared with natural FET cycles, programmed FET cycles had a higher risk of gestational hypertension (OR 1.40, 95% CI 1.15-1.70, P =0.0006, I2 =23%). The subgroup analysis for blastocyst transfer revealed a higher risk of gestational hypertension in programmed cycles versus natural cycles (OR 1.87, 95% CI 1.27-2.75, P =0.002, I2 =25%). The subgroup analysis for cleavage-stage embryo transfer revealed no difference in gestational hypertension between programmed cycles and natural cycles (OR 0.85, 95% CI 0.48-1.51, P =0.59, I2 =0%) (Figure 2; Supplementary Figure S2).
Eleven studies with a total of 20608 natural FET cycles and 10368 programmed FET cycles were pooled. We observed a higher risk of preeclampsia in programmed cycles than in natural cycles (OR 2.15, 95% CI 1.94-2.39, P <0.00001, I2 =0%). The subgroup analysis revealed a higher risk of preeclampsia in programmed cycles than in natural cycles after blastocyst transfer (OR 2.23, 95% CI 1.93-2.56, P <0.00001, I2 =0%). Only one study evaluated preeclampsia for cleavage-stage embryo transfer, showing no difference between programmed cycles and natural cycles (OR=1.19, 95% CI: 0.58-2.42, P =0.64, I2 not applicable) (Figure 3; Supplementary Figure S3).
Eighteen studies reported GDM with 27349 natural FET cycles and 20768 programmed FET cycles. The incidence of GDM in programmed cycles was higher than that in natural cycles (OR 1.11, 95% CI 1.03-1.19, P =0.0006, I2 =7%). The subgroup analysis in patients with blastocyst transfer also revealed a higher risk of GDM in programmed cycles than natural cycles (OR 1.13, 95% CI 1.04-1.23, P =0.005, I2 =39%). However, the subgroup analysis for cleavage-stage embryo transfer revealed no difference in GDM between programmed cycles and natural cycles (OR 0.79, 95% CI 0.52-1.02, P =0.27, I2 =21%) (Figure 4; Supplementary Figure S4).
Eighteen studies including 52676 natural cycles and 46099 programmed cycles provided information on LGA. A higher risk of LGA was observed in programmed cycles when compared with natural cycles (OR 1.11, 95% CI 1.07-1.15, P <0.00001, I2 =46%). In the subgroup analysis for blastocyst transfer, there was an increased risk of LGA in programmed cycles versus natural cycles (OR 1.14, 95% CI 1.07-1.21, P <0.0001, I2 =9%). 1.19). However, for cleavage-stage embryo transfer, no difference in LGA was found between programmed cycles and natural cycles in one study (OR 1.15, 95% CI 0.62-2.11, P =0.66, I2 not applicable) (Figure 5; Supplementary Figure S5).
Thirteen studies that included 29097 natural FET cycles and 25658 programmed FET cycles evaluated macrosomia. The risk of macrosomia was significantly higher in programmed cycles than in natural cycles (OR 1.14, 95% CI 1.06-1.23, P =0.0004, I2 =30%). For blastocyst transfer, there was an increased risk of macrosomia in programmed cycles versus natural cycles (OR 1.15, 95% CI 1.05-1.26, P =0.002 I2 =68%). Only one study evaluated macrosomia following cleavage-stage embryo transfer and reported no difference in macrosomia between programmed cycles and natural cycles (OR=1.22, 95% CI: 0.54-2.77, P =0.64, I2 not applicable) (Figure 6; Supplementary Figure S6).
Two studies, including 54314 natural FET cycles and 48870 programmed FET cycles, reported preterm delivery. The pooled result showed that the overall risk of PTD was significantly higher in programmed cycles than in natural cycles (OR 1.21, 95% CI 1.15-1.27, P <0.00001, I2 =49%). The subgroup analysis for blastocyst transfer revealed an elevated risk of GDM in programmed cycles versus natural cycles (OR 1.43, 95% CI 1.31-1.57, P <0.00001, I2 =22%). However, the subgroup analysis for cleavage-stage embryo transfer revealed no difference in GDM between programmed cycles and natural cycles (OR 1.05, 95% CI 0.74-1.49, P =0.96, I2 =0%) (Figure 7; Supplementary Figure S7).
Eighteen studies, which included 52676 natural FET cycles and 46099 programmed FET cycles, were meta-analysed. There was no difference in the incidence of SGA between natural cycles and programmed cycles (OR 1.05, 95% CI 0.99-1.11, P =0.13, I2 =36%). The results of the subgroup analyses revealed that the incidence of SGA in blastocyst transfer (OR 0.96, 95% CI 0.85-1.07, P =0.07, I2 =36%) and cleavage-stage embryo transfer (OR 0.85, 95% CI 0.49-1.47, P =0.56, I2not applicable) were consistent with the overall results (Figure 8; Supplementary Figure S8)
Fifteen studies were included in the analysis (programmed cycles= 17288; natural cycles =30659). The overall risk of placenta previa was significantly higher in pregnancies resulting from programmed cycles (OR 1.30, 95% CI 1.10-1.55, P =0.003, I2 =0%). The subgroup analyses revealed no difference in placenta previa between programmed cycles and natural cycles in either blastocyst transfer (OR 1.23, 95% CI 0.98-1.54, P =0.60, I2 =0%) or cleavage-stage embryo transfer (OR 0.59, 95% CI 0.31-1.14, P =0.12, I2 not applicable) (Figure 9; Supplementary Figure S9).
Fifteen studies were included in the analysis (programmed cycles= 9205; natural cycles =18654). The overall risk of placental abruption was similar between programmed FET cycles and natural FET cycles (OR 1.23, 95% CI 0.81-1.89, P =0.33, I2 =0%). The results of the subgroup analyses for blastocyst transfer (OR 1.28, 95% CI 0.72-2.28, P=0.4, I2 =0%) and cleavage-stage embryo transfer (OR 0.86, 95% CI 0.10-7.42, P =0.89, I2 not applicable) were consistent with the overall results (Figure 10; Supplementary Figure S10).
Seven studies reported PPROM, including 6880 natural cycles and 6803 programmed cycles. The overall risk of PPROM was higher among the pregnancies resulting from the programmed FET cycles (OR 1.22, 95% CI 1.02-1.46, P =0.03, I2 =44%). The subgroup analysis for blastocyst transfer was consistent with the overall results (OR 1.45, 95% CI 1.14-1.83, P =0.002, I2 =46%). However, cleavage-stage embryo transfer showed no difference in PPROM between programmed cycles and natural cycles (OR=0.74, 95% CI: 0.46-1.21, P =0.23, I2 =0%) (Figure 11; Supplementary Figure S11).
Ten studies reported PPH, including 11338 natural cycles and 19794 programmed cycles. The overall risk of PPH was higher in the pregnancies resulting from the programmed FET cycles than in those resulting from natural FET cycles (OR 2.40, 95% CI 2.12-2.72, P <0.00001, I2 =53%). After a study suspected of being the source of heterogeneity was excluded (14), sensitivity analysis revealed that programmed cycles still had a significantly higher risk of PPH (OR 2.00, 95% CI 1.66-2.42, P <0.00001, I2 =34%). The subgroup analysis for blastocyst transfer was consistent with the overall results (OR 1.92, 95% CI 1.46-2.51, P <0.00001, I2 =55%). However, the subgroup analysis for cleavage-stage embryo transfer showed no difference in PPH between programmed cycles and natural cycles (OR 1.49, 95% CI: 0.85-2.62, P =0.17, I2 not applicable) (Figure 12; Supplementary Figure S12).
In our analysis of obstetric and perinatal outcomes between natural FET cycles and programmed FET cycles, we showed that programmed cycles had a higher risk of HDPs, GH, PE, GDM, LGA, macrosomia, PD, PP, PPROM and PPH than natural cycles, which is consistent with the meta-analysis (6). Similar results were also achieved in another meta-analysis (33). Via subgroup analysis, our present meta-analysis further evaluated the effect of embryo stage at the time of transfer on perinatal outcomes between programmed FET cycles and natural FET cycles and showed that the perinatal outcomes of blastocyst transfer were consistent with the overall results in programmed FET cycles; that is, the incidences of HDP, GH, PE, PPROM and PPH were elevated in programmed FET cycles compared with natural FET cycles following blastocyst transfer. However, the perinatal outcomes of cleavage-stage embryo transfers in programmed FET cycles are similar to those in natural FET cycles.
To date, studies evaluating the effect of cleavage-stage embryo and blastocyst transfers on pregnancy outcomes have shown conflicting results. Ginström Ernstad et al. reported that women with blastocyst transfer had a 2.08-fold increased risk of placenta previa and a 1.62-fold increased risk of placental abruption versus cleavage-stage embryo transferss (34). In contrast, a meta-analysis performed by Rosalik et al. including 15 studies reported that both blastocyst transfers and cleavage-stage transfers could lead to a higher risk of LGA in programmed FET cycles versus natural FET cycles (35). Our present study finds a neutral effect for cleavage-stage transfers on obstetric and perinatal outcomes in programmed FET cycles versus natural FET cycles. Of note, the number of studies included here for cleavage-stage embryo transfer were too small to obtain reliable results, so these findings should be considered with great caution.
The reason for the higher risk of HDPs in the programmed FET cycles is unknown. The role of the corpus luteum has recently been a focus of attention for investigators. Indeed, a prospective cohort study of singleton pregnancy reported that women with 0 CL had elevated rates of PE (12.8% versus 3.9%; P=0.02) and PE with severe features (9.6% versus 0.8%; P=0.002) compared to those who conceived with 1 CL. After adjusting for confounders, 0 CL was shown to be a positive predictor of PE and sPE (5). Similar results were also achieved in another prospective study (35). Recently, a hypothesis was proposed that the absence of the CL in programmed cycles may lead to impaired maternal haemodynamic and cardiovascular adaptation to pregnancy in the first trimester, which is associated with adverse pregnancy outcomes such as preeclampsia (36). Compelling findings support the hypothesis that the expected decline in carotid-femoral pulse wave velocity (cfPWV) and the expected rise in transit time (cfPWTT) in the first trimester are attenuated in women with 0 CL (programmed FET cycle) relative to normal women, which suggests that arterial compliance is impaired in pregnant women with no CL (37). Moreover, pregnant women with 0 CL also have a blunted reactive hyperaemia index, an index that reflects endothelial function in early pregnancy, when compared to women with 1 CL (37). All these observations have prompted investigators to explore which factors are secreted into the circulation of women by the CL that may be important for maternal cardiovascular adaptation to pregnancy. Relaxin, a 6-kDa peptide, is probably a potential mediator (38). It is predominantly secreted by CL granulosa lutein cells in the late luteal phase (50-100 pg/ml) and reaches a peak concentration of 1000-2500 pg/mL in the first trimester when pregnancy occurs (39). Circulating relaxin was undetectable in women lacking CL (40, 41). It binds to membrane-associated relaxin family peptide receptor 1 (RXFP1), which is a G protein-coupled receptor that is widely distributed in the uterus (myometrium and epithelial layer), ovary and placenta (42) and vascular smooth muscle (unpublished data). Relaxin is a potent vasodilator that mediates vasodilation through the activation of Gi/PI3K-induced cAMP and nitric oxide synthase (nNOS)-driven NO release (43). An experimental study showed that systemic and renal vasodilation and global arterial compliance in early pregnancy were decreased, which contributed to lower cardiac output and a lower glomerular filtration rate when a relaxin-neutralizing antibody was administered to gravid rats (44–46). In humans, the expected rise in 24-hour creatinine clearance was blunted in pregnant women with ovum donation (no circulating relaxin) compared with normal pregnant women, which suggests that the renal response is also impaired in human pregnancy (8, 41). Therefore, relaxin could play a similar role in the maternal circulatory changes that occur during the first trimester. Relaxin deficiency (0 CL) is a potential compromise of maternal cardiovascular adaptation to pregnancy, which is the basic circulatory pattern that occurs in preeclampsia. Indeed, Post Uiterweer et al. found that women with low relaxin concentrations (lowest centile: < p10) during the first trimester are at increased risk of developing late-onset preeclampsia (36). In addition, women with donor-fresh and donor-thawed treatment have significantly higher odds of HDPs relative to women undergoing autologous-fresh treatment. A common feature among donor oocyte cycles is the usage of programmed endometrial preparation, which has no functioning CL (47). Most strikingly, relaxin has been recommended as a potential therapeutic candidate for preeclampsia (38). On the other hand, relaxin is a potent stimulus of endometrial maturation (decidualization), which governs trophoblast invasion during pregnancy (48). Relaxin can enhance the effect of progestin on the induction of prolactin and insulin growth factor binding protein-1 (IGFBP-1) secretion as well as glycodelin expression from human endometrial stromal cells (hESCs), which are biomarkers of decidualization (42, 49). In an exogenous oestradiol- and progesterone-treated ovariectomized rhesus monkey model, giving relaxin can increase its resident endometrial lymphocyte number and promote endometrial angiogenesis compared to controls (50, 51). Therefore, deficient CL-derived relaxin in early pregnancy probably contributes to aberrant decidualization, which is an important contributor to downregulated cytotrophoblast invasion, impaired placentation and consequently the genesis of placenta-related diseases, such as HDPs, placenta previa, PPROM and PPH (33, 52). The conventional theory is that preeclampsia causes impaired trophoblast invasion and uterine spiral artery remodelling, which can lead to impaired placentation, including reduced placental perfusion and placental ischaemia and reperfusion injury. In addition to CL, suboptimal steroid hormone administration in the programmed FET cycle may also affect trophoblast invasion through immunomodulation in the first trimester (48) and, as a result, influence placenta formation and function. For example, oestrogen has different effects on immune modulation depending on its concentration. Natural killer cells, which have been considered prime regulators of trophoblast invasion, are inhibited by pregnancy levels of oestradiol (E2) but are stimulated at dioestrus to proestrus levels of E2. IL-1 is stimulated by E2 at low concentrations but is inhibited by E2 at pregnancy levels. In addition, IL-6 is inhibited by E2 at periovulatory to pregnancy levels with no effects at early follicular or postmenopausal levels (53). Interestingly, these interleukins have been reported to play a role in trophoblast invasion during early pregnancy, which is involved in the genesis of HDPs. Indeed, Albrecht et al. found that elevated oestrogen levels in baboons lead to markedly decreased invasion of uterine spiral arteries by placental extravillous cytotrophoblasts, an important cell type involved in maintaining placental function (54). To date, we know little about the specific mechanism underlying the association between endometrial preparation for ET and adverse obstetric and neonatal complications.
Our study has several limitations. The first is that the included studies are retrospective in design, and there are inherent biases across them that we cannot address. Another limitation is that we included preimplantation genetic testing cycles. In addition, we did not specify the true natural FET and modified natural cycles.
Our research showed that programmed FET cycles resulted in adverse obstetric and perinatal outcomes relative to natural FET cycles following mixed frozen embryo transfer (combined cleavage stage and blastocyst stage) or frozen blastocyst transfer, such as HDPs, gestational hypertension, PE, GDM,LGA, macrosomia, SGA, PTD, placenta previa, PPROM, and PPH. However, the obstetric and perinatal outcomes were similar after frozen cleavage-stage embryo transfers. Further investigations, including RCTs, should be conducted to elucidate the reason for obstetric and perinatal outcomes in programmed FET cycles.
The original contributions presented in the study are included in the article/Supplementary Material. Further inquiries can be directed to the corresponding author.
Designer of the study: ZZ. Data acquisition and analysis: ZZ, YC, HD, XS, DL, LH. Draft of the manuscript and interpretation: ZZ. Revision of the manuscript: LX. All authors contributed to the article and approved the submitted version.
This study was supported by the National Natural Science Foundation of China (no. 81971354).
The authors declare that the research was conducted in the absence of any commercial or financial relationships that could be construed as a potential conflict of interest.
All claims expressed in this article are solely those of the authors and do not necessarily represent those of their affiliated organizations, or those of the publisher, the editors and the reviewers. Any product that may be evaluated in this article, or claim that may be made by its manufacturer, is not guaranteed or endorsed by the publisher.
The Supplementary Material for this article can be found online at: https://www.frontiersin.org/articles/10.3389/fendo.2023.1186068/full#supplementary-material
1. Singh B, Reschke L, Segars J, Baker VL. Frozen-thawed embryo transfer: the potential importance of the corpus luteum in preventing obstetrical complications. Fertil Steril (2020) 113:252–7. doi: 10.1016/j.fertnstert.2019.12.007
2. Chen ZJ, Shi Y, Sun Y, Zhang B, Liang X, Cao Y, et al. Fresh versus frozen embryos for infertility in the polycystic ovary syndrome. N Engl J Med (2016) 375:523–33. doi: 10.1056/NEJMoa1513873
3. Roque M, Haahr T, Geber S, Esteves SC, Humaidan P. Fresh versus elective frozen embryo transfer in IVF/ICSI cycles: a systematic review and meta-analysis of reproductive outcomes. Hum Reprod Update (2019) 25:2–14. doi: 10.1093/humupd/dmy033
4. Wei D, Liu JY, Sun Y, Shi Y, Zhang B, Liu JQ, et al. Frozen versus fresh single blastocyst transfer in ovulatory women: a multicentre, randomised controlled trial. Lancet (2019) 393:1310–8. doi: 10.1016/S0140-6736(18)32843-5
5. von Versen-Höynck F, Schaub AM, Chi YY, Chiu KH, Liu J, Lingis M, et al. Increased preeclampsia risk and reduced aortic compliance with in vitro fertilization cycles in the absence of a corpus luteum. Hypertension (2019) 73:640–9. doi: 10.1161/HYPERTENSIONAHA.118.12043
6. Busnelli A, Schirripa I, Fedele F, Bulfoni A, Levi-Setti PE. Obstetric and perinatal outcomes following programmed compared to natural frozen-thawed embryo transfer cycles: a systematic review and meta-analysis. Hum Reprod (2022) 37:1619–41. doi: 10.1093/humrep/deac073
7. Martins WP, Nastri CO, Rienzi L, van der Poel SZ, Gracia CR, Racowsky C. Obstetrical and perinatal outcomes following blastocyst transfer compared to cleavage transfer: a systematic review and meta-analysis. Hum Reprod (2016) 31:2561–9. doi: 10.1093/humrep/dew244
8. Smith MC, Murdoch AP, Danielson LA, Conrad KP, Davison JM. Relaxin has a role in establishing a renal response in pregnancy. Fertil Steril (2006) 86:253–5. doi: 10.1016/j.fertnstert.2005.11.070
9. Ishihara O, Araki R, Kuwahara A, Itakura A, Saito H, Adamson GD. Impact of frozen-thawed single-blastocyst transfer on maternal and neonatal outcome: an analysis of 277,042 single-embryo transfer cycles from 2008 to 2010 in Japan. Fertil Steril (2014) 101:128–33. doi: 10.1016/j.fertnstert.2013.09.025
10. Fernando D, Halliday JL, Breheny S, Healy DL. Outcomes of singleton births after blastocyst versus nonblastocyst transfer in assisted reproductive technology. Fertil Steril (2012) 97:579–84. doi: 10.1016/j.fertnstert.2011.12.032
11. Asserhøj LL, Spangmose AL, Aaris Henningsen AK, Clausen TD, Ziebe S, Jensen RB, et al. Adverse obstetric and perinatal outcomes in 1,136 singleton pregnancies conceived after programmed frozen embryo transfer (FET) compared with natural cycle FET. Fertil Steril (2021) 115:947–56. doi: 10.1016/j.fertnstert.2020.10.039
12. Dallagiovanna C, Cappellari M, D’Ambrosi F, Reschini M, Kordas K, Piani LL,, et al. Endometrial preparation does not affect the risk of hypertensive disorders of pregnancy in low-risk women undergoing frozen embryo transfer. Gynecol Endocrinol (2021) 38:238–42. doi: 10.1080/09513590.2021.1955342
13. Fu Y, Chen D, Cai B, Xu Y, Zhu S, Ding C, et al. Comparison of two mainstream endometrial preparation regimens in vitrified-warmed embryo transfers after PGT. Reprod BioMed Online (2022) 44:239–46. doi: 10.1016/j.rbmo.2021.09.009
14. Ginström Ernstad E, Wennerholm UB, Khatibi A, Petzold M, Bergh C. Neonatal and maternal outcome after frozen embryo transfer: Increased risks in programmed cycles. Am J Obstet Gynecol (2019) 221:126. doi: 10.1016/j.ajog.2019.03.010
15. Guan Y, Fan H, Styer AK, Xiao Z, Li Z, Zhang J, et al. A modified natural cycle results in higher live birth rate in vitrified-thawed embryo transfer for women with regular menstruation. Syst Biol Reprod Med (2016) 62:335–42. doi: 10.1080/19396368.2016.1199064
16. Gu F, Wu Y, Tan M, Hu R, Chen Y, Li X, et al. Programmed frozen embryo transfer cycle increased risk of hypertensive disorders of pregnancy: a multicenter cohort study in ovulatory women. Am J Obstet Gynecol MFM (2023) 5:100752. doi: 10.1016/j.ajogmf.2022.100752
17. Hu KL, Zhang D, Li R. Endometrium preparation and perinatal outcomes in women undergoing single-blastocyst transfer in frozen cycles. Fertil Steril (2021) 115:1487–94. doi: 10.1016/j.fertnstert.2020.12.016
18. Jing S, Li XF, Zhang S, Gong F, Lu G, Lin G. Increased pregnancy complications following frozen-thawed embryo transfer during an artificial cycle. J Assist Reprod Genet (2019) 36:925–33. doi: 10.1007/s10815-019-01420-1
19. Li C, He YC, Xu JJ, Wang Y, Liu H, Duan CC, et al. Perinatal outcomes of neonates born from different endometrial preparation protocols after frozen embryo transfer: a retrospective cohort study. BMC Pregnancy Childbirth (2021) 21:341. doi: 10.1186/s12884-021-03791-9
20. Li X, Xie Q, Luan T, Su Y, Zhang J, Zhang J, et al. Maternal and child-health outcomes in different endometrial preparation methods for frozen-thawed embryo transfer: a retrospective study. Hum Fertil (Camb) (2022), 1–12. doi: 10.1080/14647273.2022.2053593
21. Lin J, Zhao J, Hao G, Tan J, Pan Y, Wang Z, et al. Maternal and neonatal complications after natural vs. hormone replacement therapy cycle regimen for frozen single blastocyst transfer. Front Med (Lausanne) (2020) 7:338. doi: 10.3389/fmed.2020.00338
22. Makhijani R, Bartels C, Godiwala P, Bartolucci A, Nulsen J, Grow D, et al. Maternal and perinatal outcomes in programmed versus natural vitrified-warmed blastocyst transfer cycles. Reprod BioMed Online (2020) 41:300–8. doi: 10.1016/j.rbmo.2020.03.009
23. Man Y, Bian Y, Zhao S, Zhao R, Xu X, Wei D, et al. The effect of different endometrial preparations on women with polycystic ovary syndrome undergoing initial frozen embryo transfer: A historical cohort analysis. Acta Obstet Gynecol Scand (2021) 100:1116–23. doi: 10.1111/aogs.14058
24. Pan Y, Li B, Wang Z, Wang Y, Gong X, Zhou W, et al. Hormone replacement versus natural cycle protocols of endometrial preparation for frozen embryo transfer. Front Endocrinol (Lausanne) (2020) 11:546532. doi: 10.3389/fendo.2020.546532
25. Saito K, Miyado K, Yamatoya K, Kuwahara A, Inoue E, Miyado M, et al. Increased incidence of post-term delivery and cesarean section after frozen-thawed embryo transfer during a hormone replacement cycle. J Assist Reprod Genet (2017) 34:465–70. doi: 10.1007/s10815-017-0869-7
26. Tatsumi T, Jwa SC, Kuwahara A, Irahara M, Kubota T, Saito H. Pregnancy and neonatal outcomes following letrozole use in frozen-thawed single embryo transfer cycles. Hum Reprod (2017) 32:1244–8. doi: 10.1093/humrep/dex066
27. Wang Z, Liu H, Song H, Li X, Jiang J, Sheng Y, et al. Increased risk of pre-eclampsia after frozen-thawed embryo transfer in programming cycles. Front Med (Lausanne) (2020a) 7:104. doi: 10.3389/fmed.2020.00104
28. Wang B, Zhang J, Zhu Q, Yang X, Wang Y. Effects of different cycle regimens for frozen embryo transfer on perinatal outcomes of singletons. Hum Reprod (2020b) 35:1612–22. doi: 10.1093/humrep/deaa093
29. Xu J, Zhou H, Zhou T, Guo Y, Liang S, Jia Y, et al. The impact of different endometrial preparation protocols on obstetric and neonatal complications in frozen-thawed embryo transfer: a retrospective cohort study of 3,458 singleton deliveries. Reprod Biol Endocrinol (2022) 20:141. doi: 10.1186/s12958-022-01009-x
30. Zaat TR, Brink AJ, de Bruin JP, Goddijn M, Broekmans F, Cohlen BJ, et al. Increased obstetric and neonatal risks in artificial cycles for frozen embryo transfers. Reprod BioMed Online (2021) 42:919–29. doi: 10.1016/j.rbmo.2021.01.015
31. Zhou R, Zhang X, Huang L, Wang S, Li L, Dong M, et al. The impact of different cycle regimens on birthweight of singletons in frozen-thawed embryo transfer cycles of ovulatory women. Fertil Steril (2022) 117:573–82. doi: 10.1016/j.fertnstert.2021.09.033
32. Zong L, Liu P, Zhou L, Wei D, Ding L, Qin Y. Increased risk of maternal and neonatal complications in hormone replacement therapy cycles in frozen embryo transfer. Reprod Biol Endocrinol (2020) 18:36. doi: 10.1186/s12958-020-00601-3
33. Moreno-Sepulveda J, Espinós JJ, Checa MA. Lower risk of adverse perinatal outcomes in natural versus artificial frozen-thawed embryo transfer cycles: a systematic review and meta-analysis. Reprod BioMed Online (2021) 42:1131–45. doi: 10.1016/j.rbmo.2021.03.002
34. Ginström Ernstad E, Bergh C, Khatibi A, Källén KB, Westlander G, Nilsson S, et al. Neonatal and maternal outcome after blastocyst transfer: a population-based registry study. Am J Obstet Gynecol (2016) 214:378.e1–378.e10. doi: 10.1016/j.ajog.2015.12.040
35. Rosalik K, Carson S, Pilgrim J, Luizzi J, Levy G, Heitmann R, et al. Effects of different frozen embryo transfer regimens on abnormalities of fetal weight: a systematic review and meta-analysis. Hum Reprod Update (2021) 28:1–14. doi: 10.1093/humupd/dmab037
36. Post Uiterweer ED, Koster M, Jeyabalan A, Kuc S, Siljee JE, Stewart DR, et al. Circulating pregnancy hormone relaxin as a first trimester biomarker for preeclampsia. Pregnancy Hypertens (2020) 22:47–53. doi: 10.1016/j.preghy.2020.07.008
37. von Versen-Höynck F, Narasimhan P, Selamet Tierney ES, Martinez N, Conrad KP, Baker VL, et al. Absent or excessive corpus luteum number is associated with altered maternal vascular health in early pregnancy. Hypertension (2019) 73:680–90. doi: 10.1161/HYPERTENSIONAHA.118.12046
38. Pereira MM, Mainigi M, Strauss JF. Secretory products of the corpus luteum and preeclampsia. Hum Reprod Update (2021) 27:651–72. doi: 10.1093/humupd/dmab003
39. Aragón-Herrera A, Feijóo-Bandín S, Anido-Varela L, Moraña-Fernández S, Roselló-Lletí E, Portolés M, et al. Relaxin-2 as a potential biomarker in cardiovascular diseases. J Pers Med (2022) 12. doi: 10.3390/jpm12071021
40. Conrad KP, Graham GM, Chi YY, Zhai X, Li M, Williams RS, et al. Potential influence of the corpus luteum on circulating reproductive and volume regulatory hormones, angiogenic and immunoregulatory factors in pregnant women. Am J Physiol Endocrinol Metab (2019) 317:E677–85. doi: 10.1152/ajpendo.00225.2019
41. von Versen-Höynck F, Strauch NK, Liu J, Chi YY, Keller-Woods M, Conrad KP, et al. Effect of mode of conception on maternal serum relaxin, creatinine, and sodium concentrations in an infertile population. Reprod Sci (2019) 26:412–9. doi: 10.1177/1933719118776792
42. Hsu SY, Nakabayashi K, Nishi S, Kumagai J, Kudo M, Sherwood OD, et al. Activation of orphan receptors by the hormone relaxin. Science (2002) 295:671–4. doi: 10.1126/science.1065654
43. Devarakonda T, Salloum FN. Heart disease and relaxin: new actions for an old hormone. Trends Endocrinol Metab (2018) 29:338–48. doi: 10.1016/j.tem.2018.02.008
44. Novak J, Danielson LA, Kerchner LJ, Sherwood OD, Ramirez RJ, Moalli PA, et al. Relaxin is essential for renal vasodilation during pregnancy in conscious rats. J Clin Invest (2001) 107:1469–75. doi: 10.1172/JCI11975
45. Debrah DO, Novak J, Matthews JE, Ramirez RJ, Shroff SG, Conrad KP. Relaxin is essential for systemic vasodilation and increased global arterial compliance during early pregnancy in conscious rats. Endocrinology (2006) 147:5126–31. doi: 10.1210/en.2006-0567
46. Conrad KP. G-Protein-coupled receptors as potential drug candidates in preeclampsia: targeting the relaxin/insulin-like family peptide receptor 1 for treatment and prevention. Hum Reprod Update (2016) 22:647–64. doi: 10.1093/humupd/dmw021
47. Luke B, Brown MB, Eisenberg ML, Callan C, Botting BJ, Pacey A, et al. In vitro fertilization and risk for hypertensive disorders of pregnancy: associations with treatment parameters. Am J Obstet Gynecol (2020) 222:350.e1–350.e13. doi: 10.1016/j.ajog.2019.10.003
48. Sharma S, Godbole G, Modi D. Decidual control of trophoblast invasion. Am J Reprod Immunol (2016) 75:341–50. doi: 10.1111/aji.12466
49. Dimitriadis E, Stoikos C, Baca M, Fairlie WD, McCoubrie JE, Salamonsen LA. Relaxin and prostaglandin E(2) regulate interleukin 11 during human endometrial stromal cell decidualization. J Clin Endocrinol Metab (2005) 90:3458–65. doi: 10.1210/jc.2004-1014
50. Oron G, Sokal-Arnon T, Son WY, Demirtas E, Buckett W, Zeadna A, et al. Extended embryo culture is not associated with increased adverse obstetric or perinatal outcome. Am J Obstet Gynecol (2014) 211:165.e1–7. doi: 10.1016/j.ajog.2014.03.018
51. Garrido-Gomez T, Dominguez F, Quiñonero A, Diaz-Gimeno P, Kapidzic M, Gormley M, et al. Defective decidualization during and after severe preeclampsia reveals a possible maternal contribution to the etiology. Proc Natl Acad Sci U.S.A. (2017) 114:E8468–77. doi: 10.1073/pnas.1706546114
52. Conrad KP. Evidence for corpus luteal and endometrial origins of adverse pregnancy outcomes in women conceiving with or without assisted reproduction. Obstet Gynecol Clin North Am (2020) 47:163–81. doi: 10.1016/j.ogc.2019.10.011
53. Straub RH. The complex role of estrogens in inflammation. Endocr Rev (2007) 28:521–74. doi: 10.1210/er.2007-0001
Keywords: frozen–thawed embryo transfer, programmed cycle, natural cycle, embryo, embryo at time of transfer
Citation: Zhao Z, Chen Y, Deng H, Huang L, Lu D, Shen X and Xu L (2023) The influence of embryo stage on obstetric complications and perinatal outcomes following programmed compared to natural frozen-thawed embryo transfer cycles: a systematic review and meta-analysis. Front. Endocrinol. 14:1186068. doi: 10.3389/fendo.2023.1186068
Received: 14 March 2023; Accepted: 14 July 2023;
Published: 16 August 2023.
Edited by:
Zhen-Gao Sun, Affiliated Hospital of Shandong University of Traditional Chinese Medicine, ChinaReviewed by:
Aleksander Makatsariya, I.M. Sechenov First Moscow State Medical University, RussiaCopyright © 2023 Zhao, Chen, Deng, Huang, Lu, Shen and Xu. This is an open-access article distributed under the terms of the Creative Commons Attribution License (CC BY). The use, distribution or reproduction in other forums is permitted, provided the original author(s) and the copyright owner(s) are credited and that the original publication in this journal is cited, in accordance with accepted academic practice. No use, distribution or reproduction is permitted which does not comply with these terms.
*Correspondence: Liangzhi Xu, eHVsaWFuZ3poaXNjdUAxNjMuY29t
†These authors have contributed equally to this work and share first authorship
Disclaimer: All claims expressed in this article are solely those of the authors and do not necessarily represent those of their affiliated organizations, or those of the publisher, the editors and the reviewers. Any product that may be evaluated in this article or claim that may be made by its manufacturer is not guaranteed or endorsed by the publisher.
Research integrity at Frontiers
Learn more about the work of our research integrity team to safeguard the quality of each article we publish.