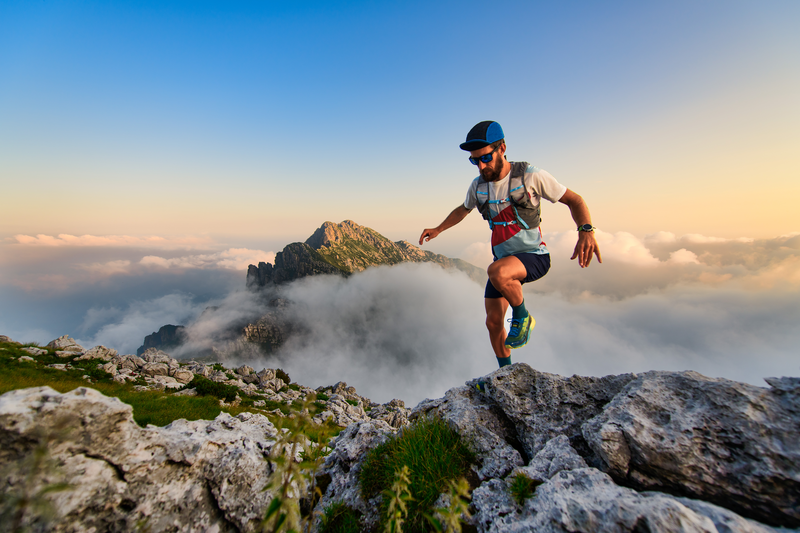
95% of researchers rate our articles as excellent or good
Learn more about the work of our research integrity team to safeguard the quality of each article we publish.
Find out more
REVIEW article
Front. Endocrinol. , 26 June 2023
Sec. Cancer Endocrinology
Volume 14 - 2023 | https://doi.org/10.3389/fendo.2023.1176731
This article is part of the Research Topic Developments in Diagnosis and Management of Thyroid Cancer View all 15 articles
The treatment of advanced, radioiodine refractory, differentiated thyroid cancers (RR-DTCs) has undergone major advancements in the last decade, causing a paradigm shift in the management and prognosis of these patients. Better understanding of the molecular drivers of tumorigenesis and access to next generation sequencing of tumors have led to the development and Food and Drug Administration (FDA)-approval of numerous targeted therapies for RR-DTCs, including antiangiogenic multikinase inhibitors, and more recently, fusion-specific kinase inhibitors such as RET inhibitors and NTRK inhibitors. BRAF + MEK inhibitors have also been approved for BRAF-mutated solid tumors and are routinely used in RR-DTCs in many centers. However, none of the currently available treatments are curative, and most patients will ultimately show progression. Current research efforts are therefore focused on identifying resistance mechanisms to tyrosine kinase inhibitors and ways to overcome them. Various novel treatment strategies are under investigation, including immunotherapy, redifferentiation therapy, and second-generation kinase inhibitors. In this review, we will discuss currently available drugs for advanced RR-DTCs, potential mechanisms of drug resistance and future therapeutic avenues.
Differentiated thyroid cancers (DTCs) have an excellent prognosis in most patients, with an overall 5-year relative survival rate of 98.4% according to the SEER database (1). However, a subtype of patients, representing 5-10% of all DTCs, will develop distant metastasis, most frequently in the lungs and bones (2). Prognosis remains favorable as long as metastatic disease is radioiodine-avid (3). Yet, 50% of metastatic DTCs are refractory to radioactive iodine (RAI), which is associated with poor outcomes and a 10-year survival rate of about 10% (4). On the other hand, many patients with advanced radioiodine refractory DTC (RR-DTC) can have an indolent or slowly progressive disease for many years. Thus, as multiple advances have been made in the treatment of advanced RR-DTCs in the last decade with multiple new therapeutic options, current challenges include identifying the appropriate timing for treatment initiation as well as choice of the right therapy.
The first step in treating advanced DTCs is to properly identify radioiodine refractory disease. In fact, until disease is considered unresponsive to RAI, 131I remains the gold standard in the treatment of metastatic advanced disease (3). However, taking into account the toxicity associated with high cumulative doses of RAI, it is crucial to properly identify when this therapy is no longer beneficial to the patient. The definition of RR-DTC can be challenging in clinical practice and remains somehow controversial. In most publications (2–6), RAI-refractory (RAI-R) disease is defined as either: (1) absence of RAI uptake outside the thyroid bed on the first posttherapy whole body scan, (2) loss of RAI concentration in a tumor tissue which was previously proved as RAI-avid, (3) concentration of RAI in some tumor lesions but not in others, and (4) progression of metastatic disease despite significant concentration of RAI, within a relevant time frame, usually considered as 6-12 months after 131I therapy. A fifth criterion which is highly debated is disease progression in a patient who has received ≥ 600 millicuries (mCi) of 131I. This is based on a single study which showed no further complete remissions after a cumulative dose of 22.2 GBq (600 mCi) (7). Therefore, factors such as response to previous therapies, duration of response, RAI uptake on diagnostic whole-body scan as well as previous treatment toxicity and patient preference should all be taken into account when considering if additional RAI therapy is indicated, rather than cumulative dose alone. Finally, 18F-FDG PET/CT could also be useful in identifying RAI-R disease. For instance, a study showed that a SUVmax greater than 4.0 in 18F-FDG avid metastases has a sensitivity of 75.3% and a specificity of 56.7 for predicting absence of 131I avidity (8).
Although associated with risk of progression and poorer prognosis, not all RAI-R disease needs immediate therapy. In fact, RAI-R metastatic DTCs can have an asymptomatic and indolent clinical course for several years. Such patients can be managed with active surveillance and TSH suppression alone as long as disease is asymptomatic, there is no or minimal progression, and tumor burden is low (2–4, 9). Active surveillance includes regular cross-sectional imaging of known sites of distant disease (every 3-12 months), serum thyroglobulin (Tg) and Tg antibody measurement, and as needed 18FDG-PET/CT whole body imaging, especially when Tg levels are increasing without explanation from cross-sectional imaging (2, 3, 9).
During this surveillance period, various scenarios can occur. First, disease can remain stable and asymptomatic, thus requiring no further intervention. Alternatively, there can be significant progression in one single lesion putting the patient at risk of complications or symptoms. This should be managed by locoregional therapy when feasible, including external beam radiation, stereotactic radiosurgery, thermal ablation, transarterial chemoembolization, and/or surgery (2, 3, 9). Finally, when local therapies are not feasible, or there is tumor progression despite local therapy, or there is significant disease progression in multiple lesions affecting more than one organ, then systemic therapy with kinase inhibitors becomes indicated (9).
Selecting the right kinase inhibitor to treat advanced progressive RR-DTC requires a comprehensive knowledge of the genetic alterations underlying these tumors. In fact, over the last decade, better understanding of the molecular mechanisms driving DTCs and RAI refractoriness has allowed the development of multiple targeted therapeutic agents (Figure 1).
Figure 1 Overview of mechanisms of tumorigenesis in differentiated thyroid cancer and targets of currently available drugs. Adapted from Cabanillas et al., Targeted Therapy for Advanced Thyroid Cancer: Kinase Inhibitors and Beyod. Endocr Rev. 2019;40(6):1573-604. By permission of Oxford University Press, License# 5497811051598.
The mitogen-activated protein kinase (MAPK) pathway is central to the pathogenesis of papillary thyroid carcinomas (PTCs). Mutually exclusive activating somatic alterations of genes encoding effectors in this pathway were found to represent over 80% of the known genetic alterations in these tumors in The Cancer Genome Atlas (TCGA) (10). BRAF V600E oncogenic mutations are the most frequent, encountered in about 60% of PTCs, followed by RAS point mutations and RET fusions (10). Rearrangements involving ALK and NTRK genes encoding tyrosine kinase receptors have also been described and are of particular interest since therapies targeting these mutations are now available (5, 11, 12). When no mutation in the MAPK pathway is identified, alterations in members of the phosphoinositide 3-kinase (PI3K) pathway are usually detected, including PTEN, PIK3CA and AKT1 mutations, although those are relatively rare (5, 10). EIF1AX has been described as a novel driver oncogene in approximate 1% of PTCs using the TCGA, and is mutually exclusive with MAPK mutations (10). Other mutations occasionally encountered in PTCs include fusions involving BRAF, THADA, MET, FGFR2 and ROS1 (10, 13).
Mutation profile can help predict tumor behavior and RAI refractoriness (14). In fact, it has been well described that tumors driven by BRAF V600E mutations exhibit high MAPK-signaling output and significant reduction in the expression of genes responsible for iodine uptake and metabolism, such as the sodium-iodide symporter (NIS) (10, 11). Tumors harboring BRAF V600E mutations had a significantly lower differentiation score in the TCGA cohort when compared to those with RAS mutations (10), explaining the decreased RAI uptake and responsiveness seen in BRAF-mutant tumors. A mouse model developed by Chakravarty and colleagues (15), in which oncogenic expression of BRAF V600E in thyroid follicular cells is inducible by doxycycline administration, supports this observation. Following induction of BRAF V600E expression, mice developed high-grade PTCs with increased MAPK transcriptional output and impairment of thyroid-specific gene expression, including near complete loss of NIS. Nevertheless, given the high frequency of BRAF mutations in PTC, and the indolent course of most cases, BRAF V600E mutation is likely insufficient on its own to explain the aggressive behavior of some tumors. Next generation sequencing (NGS) of advanced PTCs has shown that aggressive tumor behavior and recurrence are more likely when more than one oncogenic mutation is present, especially when TERT promoter, TP53, PIK3CA and/or AKT1 mutations co-exist with BRAF V600E mutations (3, 12, 16, 17). Moreover, these mutations may act in concert with BRAF V600E mutations to induce RAI refractoriness by leading to increased signaling in the MAPK and PI3K/AKT/mTOR pathways and further reducing NIS signaling (4, 18)
Follicular thyroid carcinomas (FTCs), which represent only 2-5% of all thyroid cancers, are most often associated with mutations involving the RAS oncogene, and rarely, PAX8-PPARγ rearrangements (11, 12). Mutations in genes encoding components of the PI3K/PTEN/AKT signaling pathway are also frequent: for instance, PTEN mutations are encountered in up to 10% of sporadic FTCs (19, 20). TERT promoter mutations can also be found in FTCs and are associated with more aggressive disease (16).
Oncocytic thyroid carcinomas (OTCs, previously Hürthle cell carcinomas), now considered as a separate subtype of thyroid cancer, harbor a mutational profile distinct from those of PTCs and FTCs (21, 22). In fact, OTCs are not associated with BRAF mutations, and rarely harbor RAS mutations or oncogenic fusions (5, 21, 22), justifying that it was inappropriate to consider them as a subtype of FTC. OTCs are rather characterized by near-haploid chromosomal content in most tumors, as well as mitochondrial DNA alterations (22). Furthermore, genes found to be more frequently altered in OTCs include DAXX, TP53, TERT promoter and EIF1AX, among others (5, 21, 22).
Agents targeting some of the mutations known to contribute to thyroid cancer pathogenesis have been developed in the last two decades, significantly changing the outcome of patients with advanced DTCs. Table 1 summarizes all currently FDA-approved kinase inhibitors for RR-DTCs.
The first two agents that were approved by the Food and Drug Administration (FDA) for the treatment of patients with locally recurrent or metastatic, progressive, RR-DTC are sorafenib (approved in 2013) and lenvatinib (approved in 2015), both multikinase inhibitors (MKI) with anti-angiogenic action through inhibition of the vascular endothelial growth factor receptors (VEGF-R) 1,2 and 3. In fact, DTCs were shown to exhibit disorganized vasculature and cancer-cell hypoxia, leading to an increased activation and expression of VEGF-R and a dependence on its signaling for tumor survival (11). VEGF and its receptor are therefore interesting therapeutic targets. Sorafenib and lenvatinib also have variable inhibitory actions on other kinases, including RET, fibroblast growth factor (FGF) and platelet-derived growth factor (PDGF) receptors.
Efficacity of sorafenib for the treatment of advanced DTC was demonstrated in the phase 3 randomized, double-blind, placebo-controlled trial DECISION (30). This study enrolled 416 patients with locally advanced or metastatic RR-DTC that had progressed in the previous 14 months and had not been previously treated with targeted therapy or chemotherapy. Median progression-free survival (PFS) was significantly longer in the sorafenib group (10.8 months) compared to the placebo group (5.8 months; hazard ratio [HR] 0.59, 95% CI 0.45-0.76; p < 0.0001). Objective response rate (ORR) and disease control rate (DCR) were also significantly higher in the sorafenib group, respectively 12.2% compared with 0.5%, and 54.1% compared with 33.8%. Overall survival (OS) did not differ significantly between the treatment groups (HR 0.80; 95% CI 0.54-1.19; p =0.14), but patients were allowed to cross over from the placebo to the treatment arm at disease progression.
Similarly, the phase 3 SELECT trial led to the FDA approval of lenvatinib (27). This randomized, double-blind, placebo-controlled trial included 261 patients with RR-DTC that had progression within the previous 13 months. Patients treated with up to one prior tyrosine kinase inhibitor (TKI) were included. Median progression-free survival was 14.7 months longer in the lenvatinib group (PFS 18.3 versus 3.6 months; HR 0.21, 99% CI 0.14-0.31; p < 0.001). This PFS benefit was independent of previous TKI therapy. ORR was 64.8% in the lenvatinib group as opposed to 1.5% in the placebo group (Odds ratio [OR] 28.87; 95% CI 12.46 – 66.86; p < 0.001), with four complete responses (CR). Although no significant difference in OS was observed between the two groups (HR for death 0.73, 95% CI 0.50-1.07; p=0.10), there was a significant survival benefit with the use of lenvatinib in patients over the age of 65 despite crossover from the placebo to the treatment arm at disease progression (OS not reached VS 18.4 months; HR 0.53; 95% CI 0.31-0.91; p = 0.02).
Although they led to significant prolongation of PFS, these agents were associated with adverse events (AEs) in virtually all patients, including grade ≥ 3 adverse events in 75.9% of patients on lenvatinib and 37.2% of patients on sorafenib. AEs led to discontinuation of lenvatinib in 14.2% of patients and sorafenib in 18.8%, while treatment interruptions and dose reductions due to toxicity occurred in well over 50% of patient with both agents (27, 30). Most common AEs include hypertension, palmar-plantar erythrodysaesthesia syndrome, fatigue, weight loss, diarrhea, and stomatitis.
In September 2021, a third MKI, cabozantinib, was approved by the FDA as a second line therapy for patients with locally advanced or metastatic RR-DTC that has progressed following prior VEGF-R targeted therapy. Cabozantinib inhibits multiple tyrosine kinases involved in tumor growth and angiogenesis including VEGF-R2, AXL, c-MET and RET (5, 23). Notably, upregulation of c-MET and AXL signaling has been shown to play a role in resistance to antiangiogenic agents (31, 32), which serves as a premise for the use of cabozantinib in patients who have progressed on VEGF-R TKIs. Cabozantinib has been approved and widely used since 2012 for the treatment of advanced medullary thyroid carcinoma (MTC). More recent approval of this drug in DTC was based on results from COSMIC-311 (23), a double-blind, phase 3 placebo-controlled trial in which 258 patients with RR-DTC that had progressed on or following prior VEGF-R TKI treatment were randomized 2:1 to cabozantinib or placebo. Patients had to have received previous treatment with at least lenvatinib or sorafenib, and no more than two previous VEGF-R TKIs were allowed. Patients who progressed on placebo could crossover to open label cabozantinib. PFS was significantly prolonged in the group treated with cabozantinib (11.0 versus 1.9 months; HR 0.22, 96% CI 0.15-0.32; p < 0.0001) despite a short median follow-up of 10.1 months. PFS improvement was observed irrespective of previous treatment. ORR also favored cabozantinib (11% [95% CI 6.9%-16.9%] versus 0% [95% CI 0.0%-4.1%]; p=0.0003), with 18 confirmed partial responses (PR) in the treatment group as opposed to none in the placebo group. Overall, results of the COSMIC-311 trial were encouraging in a population of patients with aggressive disease that would have otherwise progressed rapidly, as illustrated by the very short median PFS in the placebo group, and in whom treatment options are limited.
Similar to sorafenib and lenvatinib, AEs are very frequent with cabozantinib, occurring in 94% of patients. Grade 3 or 4 AEs were observed in 57% of patients on cabozantinib, most frequently palmar-plantar erythrodysaesthesia syndrome, fatigue, hypertension, and diarrhea. These AEs were comparable to those reported in other studies and were manageable.
Although MKIs can significantly improve PFS in patients with advanced RR-DTCs, these therapies have multiple drawbacks. Their toxicity profile can have a major impact on patients’ quality of life and may limit their long-term effective use in clinical practice. For instance, real-life studies with lenvatinib describe treatment interruption and dose reduction rates as high as 79.5% (33). Moreover, as we will discuss below, many patients will eventually develop resistance to treatment and progress. For these reasons, the quest for treatments that do not target the angiogenic pathway and provide more personalized therapeutic options for patients with advanced DTCs has continued, culminating in the FDA-approval of various selective kinase inhibitors. These agents target more specifically one or a few kinases involved in tumorigenesis, which allows for better efficacy and most importantly less toxicity.
As mentioned earlier, the BRAF V600E mutation is the most frequent oncogenic driver in PTCs, present in 60% of cases, which makes it an attractive therapeutic target. BRAF + MEK inhibitors have been used for many years in other BRAF-mutated solid tumors, mainly melanoma and non-small cell lung carcinoma (NSCLC).
The combination of dabrafenib and trametinib, a selective BRAF and MEK 1/2 inhibitor respectively, was FDA approved in 2018 for the treatment of locally advanced or metastatic BRAF V600E-mutant anaplastic thyroid cancer (ATC) and has significantly changed the treatment paradigm of these tumors which were previously viewed as a death sentence (34–37). Most recently, based on data from the ROAR (NCT02034110) (38) and NCI-MATCH (39) basket trials, the FDA granted in June 2022 an accelerated approval of dabrafenib in combination with trametinib for the treatment of patients with BRAF V600E-mutated metastatic or unresectable solid tumors who have progressed on prior treatment and have no other satisfactory treatment options, including thyroid cancers.
Dabrafenib was first shown to be promising in patients with DTC in a phase 1 basket trial (40). This led to a randomized, multicenter, open-label phase 2 trial in patients with BRAF mutated PTC (24). This study included 53 patients with progressive disease within 13 months before enrollment. Patients could have received up to three priors oral MKIs, excluding other selective BRAF or MEK inhibitors. Patients were randomized to dabrafenib monotherapy or dabrafenib in combination with trametinib, and primary endpoint was ORR in each group within the first 24 weeks of therapy. It was hypothesized that combination therapy would have superior clinical efficacy due to dual inhibition of the MAPK pathway as well as mitigation of potential mechanisms of resistance to dabrafenib through MEK kinase inhibition. Patients on dabrafenib alone were allowed to crossover to the combination group on disease progression. ORR, which included minor responses (defined as a 20 to 29% decrease in the sum of target lesions), was 42% (95% CI 23–63%) with dabrafenib and 48% (95% CI 29–68%) with dabrafenib + trametinib (p = 0.67). Median PFS was also not statistically different between the two groups (10.7 [CI 3.8-34.7] versus 15.1 [CI 12.3-37.3] months; p=0.65). The median OS was 37.9 months [CI 23.4–NR] with single-agent dabrafenib and 47.5 months [CI 27.9–57.8] with combination therapy (p = 0.99). Notably, of the 14 patients who crossed over at progression, 4 (29%) had an objective response, including 3 PRs and one minor response, and 8 had stable disease (SD). Grade 3 AEs occurred in about 50% of patients in both groups. Most frequent AEs associated with dabrafenib alone were skin disorders, fever, and hyperglycemia, while fever, hypophosphatemia and fatigue were most common with combination therapy. Skin disorders were strikingly less frequent with the combination compared to dabrafenib alone (33% VS 65% respectively). This trial, although not showing any superiority of combined BRAF and MEK inhibition over BRAF inhibitor therapy alone, did show prolonged PFS and OS with both treatment strategies, making dabrafenib +/- trametinib a therapeutic option for patients with advanced BRAF-mutated PTCs, especially when anti-angiogenic agents are contraindicated or associated with significant risk. This being said, there has been no direct comparisons between dabrafenib and MKIs such as lenvatinib in BRAF-mutated advanced DTCs to justify favoring one treatment over the other.
RET is a transmembrane glycoprotein receptor-tyrosine kinase (RTK). Ligand binding leads to RET homodimerization followed by trans-phosphorylation of tyrosine residues within the intracellular domains and activation of several signal transduction cascades involved in cellular proliferation, including the MAPK and PI3K pathways (41). Oncogenic activation of RET can occur through three main mechanisms: mutations leading to activation of the kinase domain by ligand-independent dimerization, mutations causing direct activation of the RET kinase domain, and chromosomal rearrangements producing chimeric proteins with constitutively active RET kinase domain (41, 42). Germline activating RET mutations are associated with multiple endocrine neoplasia type 2 (MEN2) syndromes, while somatic RET mutations are found in ~ 65% of all sporadic MTCs (41). RET rearrangements, on the other hand, have been identified in various solid tumors, including about 5 to 10% of PTCs, most frequently in children and in patients with prior exposure to radiation (41). CCDC6-RET and NCOA4-RET are the most frequently identified RET fusions in PTCs.
Involvement of RET alterations in tumorigenesis makes this RTK a potentially actionable therapeutic target. Moreover, tissue-specific RET knockout studies in mice, targeting the hematopoietic, neuronal, and lymphoid tissues, suggested that RET inhibition would most likely result in very little clinically significant AEs (43–45). This led to efforts aiming to identify selective RET inhibitors that would be used to treat RET mutated tumors in patients.
Selpercatinib (LOXO-292) and pralsetinib (BLU-667) are two potent and highly selective RET kinase inhibitors that have been recently FDA approved in 2020 for patients with RET fusion-positive DTCs and RET-mutant MTCs who require systemic therapy.
Efficacy of selpercatinib in RET-altered thyroid cancers was demonstrated in the LIBRETTO-001 trial (29). This phase 1/2 study included 19 patients with RET fusion-positive thyroid cancers, mainly PTCs (13/19). Most patients (79%) had had previous therapy with at least one MKI. In the RET fusion-positive DTC cohort, ORR was 79% (95% CI 54-94), including one CR and 14 PRs. Interestingly, 2/3 patients with poorly differentiated thyroid cancers (PDTC), 1/1 patient with OTC and 1/2 patients with ATC had PRs to therapy. Median PFS was not reached, but 64% of patients were progression-free at 1 year. Among all the patients with RET-altered thyroid cancers treated with selpercatinib in the trial (n=162), grade 3 or grade 4 treatment related AEs occurred in 28% and 2% respectively, most frequently hypertension (in 21% of patients) and increased cytolytic liver enzymes (increased alanine aminotransferase in 11% and asparte aminotransferase in 9%).
ARROW is a phase 1/2 trial evaluating the efficacy of pralsetinib in patients with RET-altered locally advanced or metastatic solid tumors, including thyroid carcinomas (46). Updated data presented at the 2022 American Society of Clinical Oncology (ASCO) meeting in 21 patients with previously treated RET fusion-positive thyroid cancers showed an ORR of 86% (95% CI 64-97), including 15 PRs. Duration of response was 17.5 months (95% CI 16.0 – NR) and PFS was 19.4 months (95% CI 13.0 – NR) (28). Similar to selpercatinib, pralsetinib was well tolerated, with a manageable safety profile. Most frequent grade 3 AEs were hypertension (17% of all trial patients) and cytopenia (neutropenia in 13%, lymphopenia in 11% and anaemia in 10%). One case of grade 5 pneumonia also occurred (46).
In both the LIBRETTO-001 and ARROW trials, AE-related dose reductions and treatment discontinuations were relatively low with only 2 and 4% of discontinuations of selpercatinib and pralsetinib respectively (28, 46).
The tropomyosin-receptor kinase (TRK) family of RTKs includes TRKA, TRKB and TRKC which are encoded respectively by the neurotrophic receptor tyrosine kinase genes NTRK1, NTRK2 and NTRK3 (47–49). Once activated, TRK RTKs signal through several downstream pathways involved in cellular proliferation, among which MAPK and PI3K/AKT. TRK receptors play an important role in the nervous system development (47). Oncogenic fusions leading to constitutive activation of the kinase domain have been described in all three NTRK genes, and these alterations have been identified in multiple solid tumors including colorectal cancer, lung cancer, and melanoma. In the thyroid, NTRK-driven malignancies are rare, found in 2-3% of thyroid cancers in adults, including PTCs, OTC, ATCs, and PDTCs (10, 48, 49). Like RET-fusions, NTRK fusions are more frequent in pediatric patients with PTCs (up to 25% of cases) as well as in patients with previous radiation exposure. Despite their rarity, NTRK fusion-positive thyroid cancers are important to identify as we have now two FDA-approved targeted TRK inhibitors which have demonstrated clinical safety and efficacy in patients with metastatic or unresectable solid tumors with NTRK gene fusion.
Larotrectinib is a highly selective and potent TRK inhibitor with central nervous system activity (CNS). A pooled analysis (26) of 28 patients with NTRK fusion-positive thyroid cancers treated with larotrectinib from three basket trials (50–52) showed an ORR of 71% (95% CI 51-87), including 2 CRs, 18 PRs and 4 SDs. All patients with CNS metastases at baseline had a PR. 24-month PFS and OS were respectively 69 and 76%. When excluding the 7 patients with ATC, ORR increased to 86% (95% CI 64-97) and 24-months PFS to 84%. Response to therapy was irrespective of previous systemic therapy: 13 patients with DTC who had one or more prior lines of systemic therapy had an ORR of 92%. Notably, AEs were mainly grade 1 and 2, with only two patients who experienced grade 3 treatment-related AEs (anaemia and lymphopenia). No patients required treatment discontinuation due to AEs and only 2 patients experienced AEs leading to dose reduction.
Entrectinib is another potent TRK inhibitor which was specifically designed to have systemic activity and cross the blood-brain barrier (25, 53, 54). Entrectinib also exhibits inhibitory action against ALK and ROS1 tyrosine kinases, which have been involved in resistance to TKIs (5, 25, 53, 54). In April 2022, updated pooled data (25) from two phase 1 studies (ALKA-372-001 and STARTRK-1) (53) in patients with NTRK, ROS1 or ALK alterations and one phase 2 basket study (STARTRK-2) (54) focusing on patients with NTRK fusion-positive solid tumors, were published. 13/121 patients had thyroid cancer, including 7 with CNS metastases. ORR was 53.8% (95% CI 25.1-80.8), median PFS was 19.9 months (95% CI 6.5-33.8), and OS was 19.9 months (14.5 – non evaluable [NE]). In the overall population, 11 patients had measurable CNS metastases at baseline, among which intracranial ORR was 63.6% (95%CI 30.8 – 89.1). Like larotrectinib, treatment related AEs were mostly grade 1/2, with AE-related treatment discontinuations in 8.3% of patients.
Therefore, larotrectinib and entrectinib appear as reliable and durable treatment options in NTRK fusion-positive thyroid cancers, including those with CNS metastases.
We are frequently faced in clinical practice with patients that progress or do not tolerate the previously described FDA-approved treatments. In these situations, we resort to the off-label use of other anti-neoplastic agents that have been or are currently being studied in advanced DTCs and have shown some efficacy.
Vemurafenib, a selective BRAF inhibitor approved for treatment of BRAF-mutated melanoma, was in fact the first BRAF-inhibitor studied in DTC. Its efficacy was initially demonstrated in a small case series of 3 patients with metastatic BRAF V600E-mutated PTC (55). This was later confirmed by a phase 2 non-randomized, open-label, multicenter trial in which patients with recurrent or metastatic RAI-refractory BRAF V600E-mutated PTC, who were either TKI-naïve (cohort 1, n=26) or had progressed on VEGF-R TKI (cohort 2, n=22), received single-agent vemurafenib (56). In cohort 1, DCR with vemurafenib was 73% (95% CI 52-88) with 10 (38.5%) patients who had PR and 9 (35%) who had SD as best overall response. In cohort 2, response rates were lower, with 6 (27.3%) patients who had a PR as best overall response and 6 who had SD, leading to a DCR of 55% (95% CI 32-76). Median PFS was 18.2 months (95% CI 15.5-29.3) and 8.9 months (95% CI 5.5 -NE) in cohorts 1 and 2 respectively. Median OS was not yet reached in cohort 1, while it was 14.4 months (95% CI 8.2-29.5) in cohort 2. AEs were mostly grade 1-2, including rash, fatigue, alopecia, dysgeusia, creatinine increase and weight loss. Vemurafenib seems therefore to be a valid therapeutic option for BRAF-mutated PTCs, although it yet has to be studied in a phase 3 trial.
Encorafenib is another BRAF inhibitor, currently approved in combination with the MEK-inhibitor binimetinib for BRAF-mutated metastatic melanoma and colorectal carcinoma. It has a more than 10-times longer dissociation half-life than dabrafenib or vemurafenib, allowing more sustained target inhibition and potentially a more potent antitumor activity (57). Moreover, it is associated with low rates of pyrexia and photosensitivity which are the two main dose-limiting AEs with the dabrafenib/trametinib and vemurafenib/cobimetinib combinations, respectively (57, 58). Although no clinical data is currently available for its use in thyroid cancer, there is an ongoing phase 2 trial examining encorafenib combined with binimetinib, with or without immunotherapy (nivolumab), in patients with metastatic BRAF V600E mutant RR-DTC (NCT04061980). In practice, this drug can be considered as an alternative when dabrafenib is not tolerated, especially due to intractable fevers.
Everolimus, an inhibitor of mammalian target of rapamycin (mTOR), has been studied in several trials for treatment of advanced RR-DTCs. In fact, as previously discussed, activation of the PI3K/PTEN/AKT signaling pathway is frequent in advanced thyroid cancers. This is often due to a mutation of the PTEN protein, a PI3K inhibitor. Parallel activation of this pathway has also been suggested as an escape mechanism to TKIs. mTOR, a serine-threonine kinase, is a downstream effector of the PI3K/AKT pathway and serves as a potential therapeutic target. The first reported trial of everolimus in thyroid cancers was a multicenter, open-label, phase 2 study in South Korea that enrolled patients with all thyroid cancer histologies, including 6 patients with ATC and 9 with MTC (59). Among the 38 patients that were evaluable for response, DCR was 81%, including 2 PRs (both in DTC patients). 45% of patients showed durable SD for 24 weeks or longer. Median PFS in patients with DTC was 43 weeks. Treatment was overall well tolerated with mostly grade 1 AEs. This study was followed by a second phase 2 trial in the Netherlands, which enrolled 28 patients with advanced DTC, 54% of whom had previous treatment with a TKI, namely sorafenib (60). Sixty five percent of patients showed SD as their best response, with 58% having SD lasting more than 24 weeks. However, there were no PRs or CRs. Median PFS was 9 months (95% CI, 4-14), and median OS was 18 months (95% CI 7-29). Hanna and colleagues further expanded on the topic with another phase 2 trial, once again in all thyroid cancer histologies (61). In the DTC cohort (n=33), in which 51% of patients had previously been treated with a TKI, best response to therapy was SD in 82% and PR in 3%. Median PFS was 12.9 months (7.3-18.6), and median OS was not reached. Interestingly, in this trial, DTC patients with only a BRAF mutation had the longest PFS on everolimus, while patients with alterations in the PI3K/mTOR/AKT pathway did not show better response to therapy. Thus, these three phase 2 trials demonstrate that mTOR inhibition is a viable second-line option in patients who progress on TKI therapy.
The combination of everolimus plus sorafenib showed improvement of PFS in comparison with sorafenib alone in a randomized phase 2 trial in patients with RAI-R oncocytic thyroid carcinoma that included 34 evaluable patients (62). PFS was significantly improved in the sorafenib plus everolimus arm (24.7 months (95% CI 6.1-no upper) compared to the sorafenib arm (10.9 months (95% CI 5.5-no upper). Response rates were similar between groups.
Pazopanib is an antiangiogenic MKI that inhibits VEGF, FGF, PDGF, KIT and RET receptors. It is currently FDA approved for other solid tumors including renal cell carcinoma. Pazopanib was evaluated in two phase 2 trials looking at its efficacy in patients with RR-DTC (63, 64). In 2010, Bible and colleagues conducted a first trial in 37 patients, 18 of which had confirmed PR to therapy (response rate 49%; 95%CI 35-68). Responses were seen in 8/11 (73%) patients with follicular tumors, 5/11 (45%) patients with oncocytic tumors, and 5/15 (33%) patients with papillary tumors. Therapy was well tolerated with 46% of patients taking pazopanib for 12 months or longer. The most frequent AEs were fatigue, skin and hair hypopigmentation, diarrhea, and nausea. In 2020, the same group published a larger phase 2 international study in 60 patients with advanced or progressive RR-DTC treated with pazopanib. In this second trial, response rate was slightly lower, with 36.7% of patients having a PR (CI 24.6-50.1). This is probably explained by the fact that patients were more heavily pretreated than in the prior study (91.7% VS 27%). Median PFS was 11.4 months and median OS 2.6 years. Both studies did not show any differences in response to therapy between histological subtypes of DTC, nor according to mutation profile. There is therefore substantiating evidence to support the efficacy of pazopanib in RR-DTC, and it should be considered as a therapeutic option in patients who progress or do not tolerate other FDA-approved therapies.
Other kinase inhibitors, such as sunitinib (65–67), vandetanib (68), axitinib (69–71) and dovitinib (72) have been tested in thyroid cancer, all showing modest efficacy.
Despite promising initial results, all kinase inhibitors seem to become eventually ineffective, leading to inevitable disease progression. Current research efforts are therefore focused on identifying resistance mechanisms to kinase inhibitors and ways to overcome them. To date, a few potential mechanisms of tumor resistance to kinase inhibitors have been described (73) (Figure 2).
First, acquired resistance to tyrosine kinase inhibitors can involve escape mechanisms that activate parallel signaling pathways. For instance, upregulation of alternative angiogenic signaling factors such as FGF2, PDGF or epidermal growth factor receptor (EGFR) has been observed in tumors resistant to anti-VEGF TKIs (74–76). One possible factor underlying this phenomenon is hypoxia secondary to VEGF-R inhibition (74–76). In fact, hypoxia induces gene expression of proangiogenic factors primarily through the HIF-1α (hypoxia inducible factor-1α) transcription factor. Moreover, activation of the PI3K/AKT pathway or reactivation of the JAK-STAT pathway were also shown to be involved in acquired resistance to sorafenib (76). Similarly, several studies have demonstrated that cancer cells develop resistance to BRAF inhibitors by overexpressing growth factor receptors at their surface, including KIT, c-MET, EGFR and PDGF-receptor-β (PDFGR-β), leading to MAPK pathway reactivation despite BRAF inhibition (73, 77, 78). The treatment strategy to counteract this activation of alternate pathways is to either add a second TKI or to switch to another targeted systemic therapy. For example, in a multicenter phase 2 International Thyroid Oncology Group (ITOG) trial, cabozantinib conferred significant additional PFS and OS benefits (12.7 and 34.7 months respectively) in advanced DTC patients who had progressed on prior VEGF-R targeted therapy (79).
Factors associated to the tumor microenvironement have also been involved in resistance to kinase inhibitors. Pericytes are stromal cells that play a key role in the angiogenic microenvironement of thyroid cancers, in part by facilitating vessel maturation. PDGF growth factor-BB (PDGF-BB), which promotes pericyte proliferation through interaction with PDGFR-β, has been found to be increased in BRAF V600E-mutated PTCs, and pericytes have been shown to support the growth and survival of PTC cells (80, 81). Furthermore, in vitro studies suggest that pericytes might play a role in resistance to sorafenib and vemurafenib through secretion of thrombospondin-1 (TSP-1) and TGFβ1, which trigger rebound elevation in ERK1/2 and AKT levels allowing tumor cells to overcome inhibitory effects of these targeted therapies (82). Cancer-associated fibroblasts (CAF) have also been shown to promote cancer growth and to play a role in drug resistance (83).
Epithelial-mesenchymal transition (EMT) of tumor cells, induced by secondary mutations, hypoxia and other stimulating factors from the tumor microenvironment, was also shown to be involved in resistance to sorafenib (76) and lenvatinib (84). In fact, studies identified changes in treatment-resistant cells towards a mesenchymal morphology (76, 84, 85). Tumor cells undergoing EMT loose cell adhesion molecules such as E-cadherin and gain mesenchymal cell markers such as vimentin and N-cadherin, resulting in loss of cell-to-cell contacts and increased motility, which favor their dissemination to distant sites (84, 85). In addition, EMT makes tumor cells resistant to apoptosis and anti-tumor drugs (84, 85). Nonetheless, the exact interaction between EMT and anti-VEGFR TKIs resistance remains unknown.
Acquired wild-type copy number amplifications has also been identified as a resistance mechanism to BRAF inhibitors. For example, MCL1 copy number gain has been associated with resistance to vemurafenib treatment in PTC (86). MCL1 is an anti-apoptotic member of the BCL2 family, which might regulate parallel signaling pathways activating BRAF in PTCs resistant to anti-BRAF agents. Similarly, in another case report of a PTC which underwent ATC transformation while on dabrafenib (87), acquired triploidy of chromosome 7, which harbors the EGFR, RAC1, MET, and BRAF genes, was demonstrated in the progressive metastatic lesion. Copy number amplifications of these protooncogenes were consequently present in the dedifferentiated sample, probably contributing to tumor progression.
Finally, acquisition of secondary point mutations has also been proposed as a resistance mechanism to TKIs. For instance, a study exposing BRAF V600E mutated KTC1 thyroid cancer cells to long term vemurafenib showed development of secondary KRAS point mutations, allowing these cells to bypass BRAF inhibition (88). In addition to RAS point mutations, other acquired mutations that possibly confer drug resistance were found in the RAC1, PTEN, NF1, NF2, TP53, and CDKN2A genes (73, 87, 89). Moreover, it is now well recognized that acquired mutations in the RET kinase domain cause resistance to selective RET-inhibitors by interfering with drug binding. These include RET G810 solvent-front mutations as well as non-gatekeeper mutations at hinge (Y806C/N) and β2 strand (V738A) sites within the RET kinase domain (90–93).
Identification and better understanding of these resistance mechanisms pave the way for future novel therapies including combination of kinase inhibitors, potentiation of TKIs by adding immunotherapy, and redifferentiation therapy.
In the past decade, immune checkpoint inhibitors (ICIs) have revolutionized cancer therapy. These monoclonal antibodies reactivate T-cell response against cancer cells, by blocking either the lymphocyte inhibitory receptor CTLA4 or the interaction between T-cell receptor PD-1 with its ligands PDL-1 and PDL-2 at the surface of cancer cells. To date, seven ICIs have received FDA approval for the treatment of various neoplasms including melanoma, NSCLC, renal cell carcinoma, and many others.
Just like in other neoplasia, thyroid cancer cells also escape immune surveillance, making ICIs an interesting therapeutic avenue. Immune escape in DTCs occurs through various mechanisms. First, deficient antigen presentation and reduced T-cell activation has been shown to play a role. This can occur either by downregulation of major histocompatibility complex (MHC) class I, mutations within the T-cell receptor binding domain of MHC-I, or loss of function of β2-microglobulin which results in disruption of MHC-I folding and transport to the cell surface (94, 95). Notably, MHC-I and β2-microglobulin expression were shown to be reduced or absent in 76% of PTCs (96).
Moreover, an immunosuppressive tumor microenvironment (TME) contributes to immune tolerance. Infiltration by regulatory T cells (Treg), which facilitate self-tolerance by suppressing effector T cells, has been observed in many tumor types (94). In PTC, increased Treg has been shown to correlate with lymph node metastasis and might be indicative of more aggressive disease (95, 97, 98). Other cells of the immune system including tumor-associated macrophages, plasmacytoid dendritic cells and tumor-associated mast cells are all overexpressed in the TME of DTCs and contribute to immune escape. Conversely, aberrant tumor vasculature that impairs the infiltration of immune cells can also occur (78). Finally, exhausted PD1+CD8+ T cells with defective cytokine production also play a role in the immunosuppressive milieu of DTCs (97, 98).
Several signaling pathways that are activated by oncogenic mutations associated with thyroid cancer can contribute to the immune escape. Among those, constitutive activation of the MAPK pathway impairs recruitment and function of tumor-infiltrating lymphocytes through increased expression of VEGF and multiple inhibitory cytokines (94, 95). Similarly, increased signaling in the PI3K pathway favors recruitment of inhibitory immune cells to the TME and reduces cytotoxic T-lymphocyte activity (94, 95).
Another major mechanism of immune escape in thyroid cancer as well as many other tumor types is up-regulation of inhibitory immune checkpoints, mainly PDL-1 but also PD-1, PDL-2 and CTLA4 (95). Notably, PDL-1 has been shown to be overexpressed in more advanced DTCs, with significant correlation between PDL-1 expression and lymph node metastasis, extrathyroidal invasion and disease-free survival (99). Interestingly, PDL-1 expression was higher in BRAF V600E mutant tumors, which are known to have the potential for more aggressive behavior.
Therefore, since pathogenesis of thyroid cancers includes escape of the immune system, reactivation of the anti-tumoral immune response may prove useful in the treatment of some thyroid neoplasia. This rationale led to various studies looking at ICIs in advanced DTCs.
KEYNOTE-028 is a phase Ib clinical trial of the PD-1 targeting antibody pembrolizumab in patients with PDL-1 positive, locally advanced or metastatic DTC (100). Of note, patients did not need to have radioiodine refractory disease or progression to be enrolled in the study. 22 patients were treated with pembrolizumab 10mg/kg every 2 weeks for 24 months or until confirmed progressive disease, unacceptable AEs, or investigator or patient decision to withdraw. 50% of patients had previously received an MKI. ORR was 9% (95% CI 1-29%) with only 2 PRs. Clinical benefit rate, defined as PR + SD for at least 6 months, was of 50% (95% CI 28-72%). Median PFS was 7 months (95% CI 2-14 months). At data cutoff, median OS was not reached (95% CI, 22 months to not reached), with 6- and 12-month OS rates of 100 and 90%, respectively. Treatment was overall well tolerated, the most frequent AEs being diarrhea (in 32% of patients) and fatigue (in 18%). Only one grade 3 AE occurred, namely colitis, and no grade 4 AEs or AE-related treatment discontinuations were described.
An ongoing phase 2 trial (NCT02973997) explores the combination of lenvatinib and pembrolizumab as a first line treatment of RR-DTCs with disease progression less than 14 month prior to enrollment (101).. In fact, VEGF has been associated with resistance to immune checkpoint blockade (94). VEGF axis promotes a hypoxic and immunosuppressive TME by decreasing T cell infiltration, impairing cytotoxic T cell activity, and promoting repressive immune cell infiltration. Thus, inhibition of VEGF signaling might represent an important strategy to enhance ICI efficacy. Inhibition of VEGF-R was correlated with improved response to ICIs in renal cell carcinoma, and the combination of lenvatinib + pembrolizumab has been approved for advanced endometrial carcinoma (102). Therefore, lenvatinib + pembrolizumab was also explored in DTC. Results reported in a poster at the 2020 ASCO meeting in 30 patients showed PR in 62% of patients and SD in 35%. Median time to tumor size nadir was 7.4 months (CI 1.6-17.8). Notably, 14/29 evaluable patients were still on therapy at data cutoff (7.6-18.9 months) and 6/19 (43%) patients had not yet reached tumor size nadir. Median PFS was not yet reached, but PFS at 12 months was 74%. Seventy percent of patients had grade 3 AEs and 10% had grade 4 AEs. The most common grade > 3 AEs were hypertension (in 47%), weight loss (in 13%) and maculopapular rash (in 13%). Therefore, the combination of lenvatinib + pembrolizumab seems promising, although it is unclear yet if addition of pembrolizumab brings any supplemental benefits to single agent lenvatinib as PR and SD rates with the combination are similar to those with lenvatinib alone (27). Updated data from the lenvatinib + pembrolizumab trial might help answer this question, especially if PFS or OS benefits are achieved.
Another ongoing multinational phase 1b trial, COSMIC-21 (NCT03170960), is evaluating cabozantinib in combination with the anti-PDL-1 antibody atezolizumab in advanced solid tumors, including DTCs. Similar to lenvatinib, cabozantinib has immunomodulatory properties that counteract tumor-induced immunosuppression and may enhance response to ICIs. Combination of cabozantinib and nivolumab, a PD-1 inhibitor, has already shown efficacy in a phase 3 randomized trial for advanced renal cell carcinoma (103). Efficacy and safety results of cabozantinib + atezolizumab as a first line therapy in 31 patients with locally advanced, metastatic and/or progressive RR-DTCs included in the COSMIC-021 trial were presented in a highlighted poster at the 2022 American Thyroid Association (ATA) meeting (104). Patients who had received any other systemic anticancer therapy were excluded. Fifty-eight percent of patients had PTC and 61% of the tumors were harboring a BRAF mutation. Patients were treated with cabozantinib 40mg daily and atezolizumab 1200mg every 3 weeks. At data cutoff, with a median follow-up of 24.9 months (95% CI 14.9-33.3), ORR was 42% (95% CI 25-61) including 13 PRs and 17 SDs. Impressively, DCR was 97% (30/31) with the remaining patient having no post-baseline assessment available. Duration of response to therapy was 22 months (95% CI 1.4 -28.0), median PFS was 15.2 months (95% CI 10.4-24.3), and 28/31 patients were still alive at data cutoff. Grade 3/4 AEs occurred in 55% of patients, mainly diarrhea (13%) and cytolytic transaminase increase (10%), with 4 patients having had to stop treatment due to AEs related to one or both drugs. Overall, AEs related to the combination therapy were consistent with those of the individual agents and were manageable. Therefore, first-line combination of cabozantinib + atezolizumab in advanced RR-DTC provided durable responses and a high rate of disease control across different subtypes of DTC, which makes it an interesting therapeutic option.
Combined CTLA4 and PD-1 blockade has also shown efficacy in multiple tumors including melanoma and renal cell carcinoma. In fact, combination therapy overcomes ICI resistance: CTLA4 inhibition increases T-cell priming and reduces Tregs in the TME, while PD-1 inhibition enhances T cell effector response (95). Preclinical data suggests that this combination could also be beneficial in aggressive RR-DTCs. Therefore, an ongoing phase 2 study (NCT03246958) is looking at the combination of nivolumab (an anti-PD-1) and ipilimumab (an anti-CTLA4) in RR-DTCs, including PDTCs (105). Results in 32 patients were presented in a poster at the 2020 ASCO meeting. Three (9%) patients achieved partial response, including one near-complete response, while 14/32 (44%) had stable disease. Median PFS at data cutoff was 4.9 months.
Ipilimumab/nivolumab combination has also been studied in association with cabozantinib in a multicenter phase 2 trial looking at locally advanced or metastatic RR-DTCs that have progressed on one previous anti-VEGFR therapy (NCT03914300). Interim results in 11 patients were presented at the 2022 ATA meeting (106). Interestingly, 45% (5/11) of patients included in the study had OTC and 18% (2/11) had PDTC. ORR within the first 6 months, which was the trial’s primary endpoint, was 9% (1/11), while ORR at data cutoff was 18% with 6 SDs and 2 PRs. Median PFS and OS were respectively 9 months (3.0-NR) and 19.2 months (4.6-NR). Only 3 patients were still on trial treatment at data cutoff. Therefore, although this triple combination therapy was overall well tolerated, efficacy was very limited and ipilimumab+ nivolumab did not seem to offer any additional advantage to cabozantinib monotherapy.
Multiple other clinical trials looking at various ICIs in advanced DTC are currently underway, including a phase 2 trial studying encorafenib + binimetinib with or without nivolumab in patients with metastatic BRAF V600E mutant RR-DTC (NCT04061980), and a phase 2 trial evaluating the combination of the anti-PDL-1 durvalumab with the anti-CTLA4 tremelimumab in advanced RR-DTC (NCT03753919). Table 2 summarizes ongoing and published trials of immunotherapy in DTC.
Loss of RAI sensitivity in DTCs is associated with more aggressive disease and a significantly poorer prognosis. RAI refractoriness is due to loss of thyroid differentiation features, among which the most important is Na/I symporter (NIS) function and expression. In fact, NIS allows active iodine transport into follicular cells and is responsible for RAI entry into thyroid cancer cells. Immunohistochemistry studies have shown that NIS protein expression is significantly decreased in differentiated thyroid cancer tissues (107). Decreased targeting of NIS to the plasma membrane through reduced vesicular trafficking (108) and impaired cell-cell adhesion secondary to loss of E-cadherin (109) might also play a role in loss of RAI uptake in advanced thyroid cancers.
It has now been well demonstrated that MAPK pathway activation is associated with dedifferentiation and a decreased NIS expression (110, 111). Moreover, studies have shown that the degree of tumor dedifferentiation correlates with the magnitude of activation of the MAPK pathway, and that BRAF V600E mutations lead to greater MAPK activation than RAS or RTK alterations (11, 110, 111). Conversely, suppressing the MAPK pathway with BRAF or MEK inhibitors in mice was shown to restore NIS expression and RAI uptake (15). These findings opened the floor to redifferentiation therapy, a treatment strategy in which we aim to restore RAI uptake, allowing subsequent treatment with RAI in a tumor which was previously considered as RAI-refractory.
In the first clinical study looking at redifferentiation therapy, 24 patients with RR-DTC were treated with a MEK inhibitor, selumetinib, for 4 weeks (112). Of the 20 patients who could be evaluated, 60% (12/20) had increased uptake on subsequent 124I PET-CT scan, 8 of which reached the dosimetry threshold for radioiodine therapy (i.e. if one or more lesions could be treated with a dose of ≥ 2000 cGy with an 131I administered activity ≤ 300 mCi) and were therefore treated with RAI. During the 6 months-follow-up period after radioiodine therapy, a reduction in the size of target lesions was observed in all patients, with confirmed PR in 5/8 patients and SD in 3/8 as the best overall response. In the study cohort, 9 patients had tumors harboring a BRAF V600E mutation, 5 a NRAS mutation, 3 a RET fusion and 3 had no identified mutation.
Interestingly, in the selumetinib redifferentiation trial, the 8 patients who reached the dosimetry threshold included all 5 patients with an NRAS mutation but only 1 patient with a BRAF mutation (112). This led to the hypothesis that MEK inhibitors possibly achieve an incomplete blockade of MAPK signaling in BRAF-mutant tumors which harbor a higher degree of pathway activation. Therefore, this was followed by four trials evaluating redifferentiation with BRAF inhibitors in BRAF-mutated RR-DTC.
First, Rothenberg and colleagues (113) enrolled ten patients with BRAF V600E mutant RAI-refractory PTCs. Each patient received dabrafenib for 25 days, followed by an 131I whole body scan (WBS). 6/10 patients whose scan showed new sites of radioiodine uptake remained on dabrafenib for a total of 42 days, after which they received an empiric dose of 150 mCi of RAI. At 3 months, 2/6 patients had PR and 4/6 patients had SD.
Similarly, Dunn and colleagues (114) studied redifferentiation therapy using vemurafenib in a cohort of 12 patients with BRAF mutant RR-DTC, excluding OTCs. Patients were treated with vemurafenib for 4 weeks. Pre-treatment 124I PET-CT lesional dosimetry was done before and 4 weeks after vemurafenib therapy. Patients in whom at least one index tumor (of ≥ 5mm in maximal diameter) was predicted to absorb ≥2000 cGy with a clinically administered 131I activity of ≤ 300 mCi, identified as 124I responders, were subsequently treated with RAI while still on vemurafenib. 10/12 completed the 4-week treatment course of vemurafenib, and 4 of them were 124I responders, qualifying for RAI therapy. At 6 months, 2/4 patients had SD and 2/4 had a PR. Of these four patients, two required subsequent thyroid cancer treatment at 9 and 18 months, and the other two patients have not required further therapy at 22 and 33 months, suggesting prolonged benefits.
Weber and colleagues (115) performed a prospective phase II redifferentiation study in which 6 patients with BRAF-mutated RR-DTC were treated with dabrafenib + trametinib while 14 patients with BRAF wild-type tumors were treated with trametinib alone for 21 ± 3 days. Redifferentiation was achieved in 2/6 BRAF-mutated and 5/14 BRAF wild-type patients, all of which received a dosimetry-guided therapeutic dose of RAI. At one year, response to therapy per RECIST 1.1 was PR in 1/7 patient, SD in 5/7 patients and PD in 1/7 patient. Both BRAF-mutated patients had some decrease in tumor size following redifferentiation therapy (one PR and one SD).
Finally, Leboulleux et al. (116) recently published another prospective multicentric trial in which 21 patients with BRAF-mutated metastatic, progressive, RR-DTCs were treated with dabrafenib and trametinib for 42 days then received an empiric dose of RAI 150 mCi irrespective of uptake on diagnostic WBS. Only one patient had 131I uptake on baseline diagnostic WBS while 20 patients demonstrated uptake on the post-therapeutic WBS. Responses at six months were SD in 52% of patients, PR in 38% and PD in 10%, which corresponds to a tumor control rate of 90%. Eleven patients with PR at 6 or 12 months were re-treated with a second course of dabrafenib + trametinib followed by RAI. Nine of the 10 evaluable patients within this group had abnormal 131I uptake on the second post-treatment WBS. At 6 months, 6/10 patients had a PR and 1/10 a CR. The 12-month PFS rate was 82.0% (95% CI, 58.8-92.8). Notably, re-induction of 131I uptake and response rates following redifferentiation with dabrafenib and trametinib were higher in this study compared to what was reported by Weber et al. (115). Potential explanations for these differences include longer duration of drug therapy (42 vs 21 days), higher dose of dabrafenib (150 mg vs 75 mg twice daily), more limited tumor volume (no lesion larger than 3 cm) as well as empiric treatment of all patients regardless of restoration of uptake on diagnostic WBS in the trial by Leboulleux and colleagues.
Successful redifferentiation of RAS mutant tumors with MEK inhibition in the selumetinib pilot study (112) also led to a phase 2 trial looking at efficacy of the MEK 1/2 inhibitor trametinib for redifferentiation of RAS mutant and RAS wild-type RR-DTCs. 15/25 patients in the RAS-mutant cohort met the dosimetry threshold for radioiodine therapy on 124I PET, 14 of which received RAI. At 6 months, ORR was 32%, with 8 PRs (57%), 3 SDs (21%) and 2 PDs (21%). Six-month PFS in the RAS mutant patients was 44%. In the RAS wild-type cohort (n=9), 3/4 patients with BRAF Class II alterations and 1/4 patients with RET rearrangements qualified for RAI, with 3 SDs and 1 PR (in patient with a BRAF-altered tumor) (117).
Additional retrospective studies have confirmed that redifferentiation represents a promising new therapeutic approach in patients with advanced RR-DTCs. Jaber et al. (118) described 13 patients with RR-DTC in whom targeted therapy with either single-agent BRAF or MEK inhibitor, or combination of dabrafenib and trametinib (in one patient), led to increased 131I uptake. 9/13 patients were treated with RAI, all of whom had durable disease control (3 PRs, 6 SDs). Interestingly, RAS-mutated tumors seemed to have a better response to redifferentiation therapy compared to BRAF-mutated tumors in this study (118). On the other hand, Iravani et al (119) described 6 patients who received redifferentiation therapy with either trametinib in tumors harboring an NRAS mutation, or combined BRAF and MEK inhibition (with either dabrafenib+trametinib or vemurafenib + cobimetinib) in tumors with BRAF V600E mutations. Only 1/3 patients with an NRAS mutation but all 3 patients with a BRAF V600E mutation demonstrated restoration of RAI uptake and underwent subsequent RAI therapy. Of these 4 patients, 3 achieved PR and 1 had SD with a median follow-up of 16.6 months.
The concept of redifferentiation might also apply to tumors harboring other than BRAF or RAS mutations. For instance, Groussin and colleagues (120) described one case of successful redifferentiation therapy with Larotrectinib in a patient with metastatic PTC harboring an EML4-NTRK3 gene fusion. Similarly, restoration of radioiodine uptake in patients with RET-fusion positive RR-DTC has been reported following treatment with selective RET-inhibitors pralsetinib (121) and selpercatinib (122).
Thus, substantial data now shows that mutation-guided MAPK pathway inhibition seems to be an efficient strategy to redifferentiate RR-DTCs (Table 3). However, available trials are significantly heterogeneous with regard to multiple aspects, including definition of radioiodine-refractory disease, inclusion criteria, duration of TKI therapy prior to RAI administration, choice of imaging modality to determine restoration of RAI uptake (124I PET/CT versus 123I scintigraphy) and dose of RAI (dosimetry-guided versus empiric). It also remains unclear whether increase of uptake on diagnostic WBS performed after treatment with the kinase inhibitors should be used as a criterion to select candidates for RAI administration. Therefore, more studies are needed to identify the optimal choice and duration of TKI before RAI, to better determine the characteristics of patients who are most likely to benefit from redifferentiation therapy, and to clarify the long-term risks as well as the duration of response to this therapeutic approach.
When tolerated, TKIs can lead to a significant decrease in tumor size and could allow surgical resection of a previously inoperable tumor: this is referred to as neoadjuvant chemotherapy. Most MKIs used in advanced DTC are anti-angiogenic and thus may lead to poor wound healing and fistula formation. Therefore, these drugs need to be discontinued several weeks before surgery, which makes them unfit for use in the neoadjuvant setting. Nevertheless, case reports have been published in which MKIs, mostly lenvatinib (123) and sorafenib (124), have been successfully used to achieve shrinkage of locally aggressive tumors invading major cervical vessels, allowing subsequent complete surgical resection. More recently, a systematic review of neoadjuvant targeted therapy in locally advanced thyroid cancer (125) reported an R0/R1 resection rate of 78.1% among 27 patients, across all thyroid cancer subtype including ATC, MTC and PDTC. This review included 18 patients with DTC, all of whom were treated with non-selective TKIs with anti-VEGFR activity (anlotinib, lenvatinib, sorafenib). Despite this, no increased hemorrhagic risk during surgery was reported. To further explore this therapeutic avenue, there is currently a phase II multicenter clinical trial examining the efficacy of neoadjuvant lenvatinib in patients with locally advanced DTC (NCT04321954).
Selective kinase inhibitors, on the other hand, have little to no anti-angiogenic properties, which makes them potentially safer in the neoadjuvant setting. A clinical trial looking at the neoadjuvant use of the selective BRAF-inhibitor vemurafenib in 17 patients with unresectable BRAF-mutated PTC, has been reported (126). Eleven patients who completed the 56 days of treatment with vemurafenib underwent subsequent surgery: 8 had a complete resection (R0), and 3 had a resection leaving only microscopic residual disease (R1). 3/11 patients had an incomplete resection. One patient, whose tumor was involving the carotid, had a fatal hemorrhage two weeks after surgery.
Although neoadjuvant use of targeted therapy is not standard in the management of locally advanced DTCs, this approach is promising and is being increasingly used in clinical practice, especially with the growing availability of specific kinase inhibitors. Nevertheless, more data is required to confirm the efficacy, safety, and long-term benefits of this treatment strategy. An ongoing trial looking at the use of neoadjuvant selpercatinib for locally advanced RET-altered thyroid cancers might help answer some of these concerns (NCT04759911).
Another major therapeutic avenue that is being explored for advanced radioiodine refractory thyroid carcinomas, resistant to existing treatments, is chimeric antigen receptor T-cell (CAR-T) therapy. CAR-T cells are genetically engineered T-cells that express a chimeric antigen receptor, which contains a single-chain variable fragment (scFv) responsible for antigen recognition, and an intracellular signaling domain which initiates T cell activation. CAR molecules can reprogram T-cells to recognize and eliminate tumor cells expressing specific antigens (127, 128). CAR-Ts have demonstrated remarkable efficacy in hematological neoplasms and are currently being studied in various solid tumors. However, use of CAR-T therapy is more challenging in solid tumors, due to an immunosuppressive tumor microenvironment that impedes the access of CAR-T cells into the tumor. Moreover, antigen selection in solid tumors can also be challenging, because many tumor antigens also have some low-level expression in normal tissues, exposing the patient to a risk of “on-target, off-tumor” toxicity (128). The TSH-receptor, a well-known thyroid specific antigen, seems to be a promising target for CAR-Ts in advanced DTCs in in-vitro and mouse models (129). Moreover, a study assessing the safety and tolerability of autologous CAR-T cells targeting intracellular adhesion molecular-1 (ICAM-1) in advanced refractory poorly differentiated thyroid cancers is currently ongoing (NCT04420754).
Better understanding of the molecular mechanisms underlying thyroid cancer has revolutionized the treatment of advanced, radioiodine refractory disease. Over the past decade, we have seen an expansion in the use of kinase inhibitors for advanced thyroid cancers, with the most recent approval of six selective, less toxic, targeted agents. The increasing number of available drugs raises the question as to what is the optimal treatment sequence, which remains to be defined. Moreover, although these drugs offer a delay in disease progression and tumor size shrinkage, none have led to an improved length of survival. For many of these agents, drug related toxicity is non negligeable and can significantly alter quality of life. Furthermore, patients eventually develop resistance to these therapies and experience disease progression. Therefore, identification of the optimal timing for initiation of systemic therapy is crucial, taking into consideration disease burden and rate of progression, presence of symptoms, as well as patient comorbidities and toxicity profile of potential drugs. Given limitations of currently available therapies, the search for a curative treatment for RR-DTC, with long-term persistent efficacy, continues.
SH: Conceptualization; Literature review; Data curation and Writing original draft. MC, M-CH, PI, MH and NB: Review and editing of original draft. RD: Conceptualization; Review and editing of original draft. All authors contributed to the article and approved the submitted version.
RD has received grant funding from Eisai, Exelixis, AstraZeneca, and Merck and has participated in advisory boards for Exelixis and Bayer. MC has received grant funding from Genentech and Merck and has received consulting fees from Exelixis and Bayer. MH has received grant funding from Eli Lilly & Co. and has participated in a steering committee for Eli Lilly & Co. NB has received grant funding from GSK, has received consulting fees from Eisai and Loxo and has participated in advisory board for Exelixis.
The remaining authors declare that the research was conducted in the absence of any commercial or financial relationships that could be construed as a potential conflict of interest.
All claims expressed in this article are solely those of the authors and do not necessarily represent those of their affiliated organizations, or those of the publisher, the editors and the reviewers. Any product that may be evaluated in this article, or claim that may be made by its manufacturer, is not guaranteed or endorsed by the publisher.
1. Institute NC. Surveillance, epidemiology, and end results program. In: Cancer stat facts: thyroid cancer (2022). Available at: https://seer.cancer.gov/statfacts/html/thyro.html.
2. Fugazzola L, Elisei R, Fuhrer D, Jarzab B, Leboulleux S, Newbold K, et al. European Thyroid association guidelines for the treatment and follow-up of advanced radioiodine-refractory thyroid cancer. Eur Thyroid J (2019) 8(5):227–45. doi: 10.1159/000502229
3. Haugen BR, Alexander EK, Bible KC, Doherty GM, Mandel SJ, Nikiforov YE, et al. 2015 American Thyroid association management guidelines for adult patients with thyroid nodules and differentiated thyroid cancer: the American thyroid association guidelines task force on thyroid nodules and differentiated thyroid cancer. Thyroid (2016) 26(1):1–133. doi: 10.1089/thy.2015.0020
4. Aashiq M, Silverman DA, Na’ara S, Takahashi H, Amit M. Radioiodine-refractory thyroid cancer: molecular basis of redifferentiation therapies, management, and novel therapies. Cancers (Basel). (2019) 11(9):1382. doi: 10.3390/cancers11091382
5. Cabanillas ME, Ryder M, Jimenez C. Targeted therapy for advanced thyroid cancer: kinase inhibitors and beyond. Endocr Rev (2019) 40(6):1573–604. doi: 10.1210/er.2019-00007
6. Boucher A, Ezzat S, Hotte S, Rachinsky I, Rajaraman M, Ruether D, et al. Canadian Consensus statement on the management of radioactive iodine-resistant differentiated thyroid cancer. Oral Oncol (2021) 121:105477. doi: 10.1016/j.oraloncology.2021.105477
7. Durante C, Haddy N, Baudin E, Leboulleux S, Hartl D, Travagli JP, et al. Long-term outcome of 444 patients with distant metastases from papillary and follicular thyroid carcinoma: benefits and limits of radioiodine therapy. J Clin Endocrinol Metab (2006) 91(8):2892–9. doi: 10.1210/jc.2005-2838
8. Liu M, Cheng L, Jin Y, Ruan M, Sheng S, Chen L. Predicting (131)I-avidity of metastases from differentiated thyroid cancer using (18)F-FDG PET/CT in postoperative patients with elevated thyroglobulin. Sci Rep (2018) 8(1):4352. doi: 10.1038/s41598-018-22656-4
9. Filetti S, Durante C, Hartl DM, Leboulleux S, Locati LD, Newbold K, et al. ESMO clinical practice guideline update on the use of systemic therapy in advanced thyroid cancer. Ann Oncol (2022) 33(7):674–84. doi: 10.1016/j.annonc.2022.04.009
10. Cancer Genome Atlas Research N. Integrated genomic characterization of papillary thyroid carcinoma. Cell (2014) 159(3):676–90. doi: 10.1016/j.cell.2014.09.050
11. Fagin JA, Wells SA Jr. Biologic and clinical perspectives on thyroid cancer. N Engl J Med (2016) 375(23):2307. doi: 10.1056/NEJMra1501993
12. Cabanillas ME, McFadden DG, Durante C. Thyroid cancer. Lancet (2016) 388(10061):2783–95. doi: 10.1016/S0140-6736(16)30172-6
13. Ritterhouse LL, Wirth LJ, Randolph GW, Sadow PM, Ross DS, Liddy W, et al. ROS1 rearrangement in thyroid cancer. Thyroid (2016) 26(6):794–7. doi: 10.1089/thy.2016.0101
14. Boucai L, Saqcena M, Kuo F, Grewal RK, Socci N, Knauf JA, et al. Genomic and transcriptomic characteristics of metastatic thyroid cancers with exceptional responses to radioactive iodine therapy. Clin Cancer Res (2023) 29(8):1620–30. doi: 10.1158/1078-0432.c.6533089.v2
15. Chakravarty D, Santos E, Ryder M, Knauf JA, Liao XH, West BL, et al. Small-molecule MAPK inhibitors restore radioiodine incorporation in mouse thyroid cancers with conditional BRAF activation. J Clin Invest. (2011) 121(12):4700–11. doi: 10.1172/JCI46382
16. Liu X, Qu S, Liu R, Sheng C, Shi X, Zhu G, et al. TERT promoter mutations and their association with BRAF V600E mutation and aggressive clinicopathological characteristics of thyroid cancer. J Clin Endocrinol Metab (2014) 99(6):E1130–6. doi: 10.1210/jc.2013-4048
17. Pappa T, Ahmadi S, Marqusee E, Johnson HL, Nehs MA, Cho NL, et al. Oncogenic mutations in PI3K/AKT/mTOR pathway effectors associate with worse prognosis in BRAF(V600E) -driven papillary thyroid cancer patients. Clin Cancer Res (2021) 27(15):4256–64. doi: 10.1158/1078-0432.CCR-21-0874
18. Liu J, Liu R, Shen X, Zhu G, Li B, Xing M. The genetic duet of BRAF V600E and TERT promoter mutations robustly predicts loss of radioiodine avidity in recurrent papillary thyroid cancer. J Nucl Med (2020) 61(2):177–82. doi: 10.2967/jnumed.119.227652
19. Xing M. Genetic alterations in the phosphatidylinositol-3 kinase/Akt pathway in thyroid cancer. Thyroid (2010) 20(7):697–706. doi: 10.1089/thy.2010.1646
20. Halachmi N, Halachmi S, Evron E, Cairns P, Okami K, Saji M, et al. Somatic mutations of the PTEN tumor suppressor gene in sporadic follicular thyroid tumors. Genes Chromosomes Cancer. (1998) 23(3):239–43. doi: 10.1002/(SICI)1098-2264(199811)23:3<239::AID-GCC5>3.0.CO;2-2
21. Ganly I, Ricarte Filho J, Eng S, Ghossein R, Morris LG, Liang Y, et al. Genomic dissection of hurthle cell carcinoma reveals a unique class of thyroid malignancy. J Clin Endocrinol Metab (2013) 98(5):E962–72. doi: 10.1210/jc.2012-3539
22. Gopal RK, Kubler K, Calvo SE, Polak P, Livitz D, Rosebrock D, et al. Widespread chromosomal losses and mitochondrial DNA alterations as genetic drivers in hurthle cell carcinoma. Cancer Cell (2018) 34(2):242–55 e5. doi: 10.1016/j.ccell.2018.06.013
23. Brose MS, Robinson BG, Sherman SI, Jarzab B, Lin CC, Vaisman F, et al. Cabozantinib for previously treated radioiodine-refractory differentiated thyroid cancer: updated results from the phase 3 COSMIC-311 trial. Cancer (2022) 128(24):4203–12. doi: 10.1002/cncr.34493
24. Busaidy NL, Konda B, Wei L, Wirth LJ, Devine C, Daniels GA, et al. Dabrafenib versus dabrafenib + trametinib in BRAF-mutated radioactive iodine refractory differentiated thyroid cancer: results of a randomized, phase 2, open-label multicenter trial. Thyroid (2022) 32(10):1184–92. doi: 10.1089/thy.2022.0115
25. Demetri GD, De Braud F, Drilon A, Siena S, Patel MR, Cho BC, et al. Updated integrated analysis of the efficacy and safety of entrectinib in patients with NTRK fusion-positive solid tumors. Clin Cancer Res (2022) 28(7):1302–12. doi: 10.1158/1078-0432.CCR-21-3597
26. Waguespack SG, Drilon A, Lin JJ, Brose MS, McDermott R, Almubarak M, et al. Efficacy and safety of larotrectinib in patients with TRK fusion-positive thyroid carcinoma. Eur J Endocrinol (2022) 186(6):631–43. doi: 10.1530/EJE-21-1259
27. Schlumberger M, Tahara M, Wirth LJ, Robinson B, Brose MS, Elisei R, et al. Lenvatinib versus placebo in radioiodine-refractory thyroid cancer. N Engl J Med (2015) 372(7):621–30. doi: 10.1056/NEJMoa1406470
28. Mansfield AS, Subbiah V, Schuler MH, Zhu VW, Hadoux J, Brose MS, et al. Pralsetinib in patients (pts) with advanced or metastatic RET-altered thyroid cancer (TC): updated data from the ARROW trial. J Clin Oncol (2022) 40(16_suppl):6080. doi: 10.1200/JCO.2022.40.16_suppl.6080
29. Wirth LJ, Sherman E, Robinson B, Solomon B, Kang H, Lorch J, et al. Efficacy of selpercatinib in RET-altered thyroid cancers. N Engl J Med (2020) 383(9):825–35. doi: 10.1056/NEJMoa2005651
30. Brose MS, Nutting CM, Jarzab B, Elisei R, Siena S, Bastholt L, et al. Sorafenib in radioactive iodine-refractory, locally advanced or metastatic differentiated thyroid cancer: a randomised, double-blind, phase 3 trial. Lancet (9940) 2014:319–28:384. doi: 10.1016/S0140-6736(14)60421-9
31. Zhou L, Liu XD, Sun M, Zhang X, German P, Bai S, et al. Targeting MET and AXL overcomes resistance to sunitinib therapy in renal cell carcinoma. Oncogene (2016) 35(21):2687–97. doi: 10.1038/onc.2015.343
32. Shojaei F, Lee JH, Simmons BH, Wong A, Esparza CO, Plumlee PA, et al. HGF/c-met acts as an alternative angiogenic pathway in sunitinib-resistant tumors. Cancer Res (2010) 70(24):10090–100. doi: 10.1158/0008-5472.CAN-10-0489
33. Giani C, Valerio L, Bongiovanni A, Durante C, Grani G, Ibrahim T, et al. Safety and quality-of-Life data from an Italian expanded access program of lenvatinib for treatment of thyroid cancer. Thyroid (2021) 31(2):224–32. doi: 10.1089/thy.2020.0276
34. Subbiah V, Kreitman RJ, Wainberg ZA, Cho JY, Schellens JHM, Soria JC, et al. Dabrafenib and trametinib treatment in patients with locally advanced or metastatic BRAF V600-mutant anaplastic thyroid cancer. J Clin Oncol (2018) 36(1):7–13. doi: 10.1200/JCO.2017.73.6785
35. Subbiah V, Kreitman RJ, Wainberg ZA, Cho JY, Schellens JHM, Soria JC, et al. Dabrafenib plus trametinib in patients with BRAF V600E-mutant anaplastic thyroid cancer: updated analysis from the phase II ROAR basket study. Ann Oncol (2022) 33(4):406–15. doi: 10.1016/j.annonc.2021.12.014
36. Wang JR, Zafereo ME, Dadu R, Ferrarotto R, Busaidy NL, Lu C, et al. Complete surgical resection following neoadjuvant dabrafenib plus trametinib in BRAF(V600E)-mutated anaplastic thyroid carcinoma. Thyroid (2019) 29(8):1036–43. doi: 10.1089/thy.2019.0133
37. Zhao X, Wang JR, Dadu R, Busaidy NL, Xu L, Learned KO, et al. Surgery after BRAF-directed therapy is associated with improved survival in BRAF(V600E) mutant anaplastic thyroid cancer: a single-center retrospective cohort study. Thyroid (2023) 33(4):484–91. doi: 10.1089/thy.2022.0504
38. Subbiah V, Bang Y-J, Lassen UN, Wainberg ZA, Soria J-C, Wen PY, et al. ROAR: a phase 2, open-label study in patients (pts) with BRAF V600E–mutated rare cancers to investigate the efficacy and safety of dabrafenib (D) and trametinib (T) combination therapy. J Clin Oncol (2016) 34(15_suppl):TPS2604–TPS. doi: 10.1200/JCO.2016.34.15_suppl.TPS2604
39. Salama AKS, Li S, Macrae ER, Park JI, Mitchell EP, Zwiebel JA, et al. Dabrafenib and trametinib in patients with tumors with BRAF(V600E) mutations: results of the NCI-MATCH trial subprotocol h. J Clin Oncol (2020) 38(33):3895–904. doi: 10.1200/JCO.20.00762
40. Falchook GS, Millward M, Hong D, Naing A, Piha-Paul S, Waguespack SG, et al. BRAF inhibitor dabrafenib in patients with metastatic BRAF-mutant thyroid cancer. Thyroid (2015) 25(1):71–7. doi: 10.1089/thy.2014.0123
41. Drilon A, Hu ZI, Lai GGY, Tan DSW. Targeting RET-driven cancers: lessons from evolving preclinical and clinical landscapes. Nat Rev Clin Oncol (2018) 15(3):151–67. doi: 10.1038/nrclinonc.2017.175
42. Subbiah V, Cote GJ. Advances in targeting RET-dependent cancers. Cancer Discovery (2020) 10(4):498–505. doi: 10.1158/2159-8290.CD-19-1116
43. Fonseca-Pereira D, Arroz-Madeira S, Rodrigues-Campos M, Barbosa IA, Domingues RG, Bento T, et al. The neurotrophic factor receptor RET drives haematopoietic stem cell survival and function. Nature (2014) 514(7520):98–101. doi: 10.1038/nature13498
44. Kramer ER, Aron L, Ramakers GM, Seitz S, Zhuang X, Beyer K, et al. Absence of ret signaling in mice causes progressive and late degeneration of the nigrostriatal system. PloS Biol (2007) 5(3):e39. doi: 10.1371/journal.pbio.0050039
45. Ibiza S, Garcia-Cassani B, Ribeiro H, Carvalho T, Almeida L, Marques R, et al. Glial-cell-derived neuroregulators control type 3 innate lymphoid cells and gut defence. Nature (2016) 535(7612):440–3. doi: 10.1038/nature18644
46. Subbiah V, Hu MI, Wirth LJ, Schuler M, Mansfield AS, Curigliano G, et al. Pralsetinib for patients with advanced or metastatic RET-altered thyroid cancer (ARROW): a multi-cohort, open-label, registrational, phase 1/2 study. Lancet Diabetes Endocrinol (2021) 9(8):491–501. doi: 10.1016/S2213-8587(21)00120-0
47. Vaishnavi A, Le AT, Doebele RC. TRKing down an old oncogene in a new era of targeted therapy. Cancer Discovery (2015) 5(1):25–34. doi: 10.1158/2159-8290.CD-14-0765
48. Chu YH, Dias-Santagata D, Farahani AA, Boyraz B, Faquin WC, Nose V, et al. Clinicopathologic and molecular characterization of NTRK-rearranged thyroid carcinoma (NRTC). Mod Pathol (2020) 33(11):2186–97. doi: 10.1038/s41379-020-0574-4
49. Pekova B, Sykorova V, Mastnikova K, Vaclavikova E, Moravcova J, Vlcek P, et al. NTRK fusion genes in thyroid carcinomas: clinicopathological characteristics and their impacts on prognosis. Cancers (Basel) (2021) 13(8):1932. doi: 10.3390/cancers13081932
50. Drilon A, Laetsch TW, Kummar S, DuBois SG, Lassen UN, Demetri GD, et al. Efficacy of larotrectinib in TRK fusion-positive cancers in adults and children. N Engl J Med (2018) 378(8):731–9. doi: 10.1056/NEJMoa1714448
51. Hong DS, DuBois SG, Kummar S, Farago AF, Albert CM, Rohrberg KS, et al. Larotrectinib in patients with TRK fusion-positive solid tumours: a pooled analysis of three phase 1/2 clinical trials. Lancet Oncol (2020) 21(4):531–40. doi: 10.1016/S1470-2045(19)30856-3
52. Laetsch TW, DuBois SG, Mascarenhas L, Turpin B, Federman N, Albert CM, et al. Larotrectinib for paediatric solid tumours harbouring NTRK gene fusions: phase 1 results from a multicentre, open-label, phase 1/2 study. Lancet Oncol (2018) 19(5):705–14. doi: 10.1016/S1470-2045(18)30119-0
53. Drilon A, Siena S, Ou SI, Patel M, Ahn MJ, Lee J, et al. Safety and antitumor activity of the multitargeted pan-TRK, ROS1, and ALK inhibitor entrectinib: combined results from two phase I trials (ALKA-372-001 and STARTRK-1). Cancer Discovery (2017) 7(4):400–9. doi: 10.1158/2159-8290.CD-16-1237
54. Doebele RC, Drilon A, Paz-Ares L, Siena S, Shaw AT, Farago AF, et al. Entrectinib in patients with advanced or metastatic NTRK fusion-positive solid tumours: integrated analysis of three phase 1-2 trials. Lancet Oncol (2020) 21(2):271–82. doi: 10.1016/S1470-2045(19)30691-6
55. Kim KB, Cabanillas ME, Lazar AJ, Williams MD, Sanders DL, Ilagan JL, et al. Clinical responses to vemurafenib in patients with metastatic papillary thyroid cancer harboring BRAF(V600E) mutation. Thyroid (2013) 23(10):1277–83. doi: 10.1089/thy.2013.0057
56. Brose MS, Cabanillas ME, Cohen EE, Wirth LJ, Riehl T, Yue H, et al. Vemurafenib in patients with BRAF(V600E)-positive metastatic or unresectable papillary thyroid cancer refractory to radioactive iodine: a non-randomised, multicentre, open-label, phase 2 trial. Lancet Oncol (2016) 17(9):1272–82. doi: 10.1016/S1470-2045(16)30166-8
57. Dummer R, Ascierto PA, Gogas HJ, Arance A, Mandala M, Liszkay G, et al. Encorafenib plus binimetinib versus vemurafenib or encorafenib in patients with BRAF-mutant melanoma (COLUMBUS): a multicentre, open-label, randomised phase 3 trial. Lancet Oncol (2018) 19(5):603–15. doi: 10.1016/S1470-2045(18)30142-6
58. Sullivan RJ, Weber J, Patel S, Dummer R, Carlino MS, Tan DSW, et al. A phase Ib/II study of the BRAF inhibitor encorafenib plus the MEK inhibitor binimetinib in patients with BRAF(V600E/K) -mutant solid tumors. Clin Cancer Res (2020) 26(19):5102–12. doi: 10.1158/1078-0432.CCR-19-3550
59. Lim SM, Chang H, Yoon MJ, Hong YK, Kim H, Chung WY, et al. A multicenter, phase II trial of everolimus in locally advanced or metastatic thyroid cancer of all histologic subtypes. Ann Oncol (2013) 24(12):3089–94. doi: 10.1093/annonc/mdt379
60. Schneider TC, de Wit D, Links TP, van Erp NP, van der Hoeven JJ, Gelderblom H, et al. Everolimus in patients with advanced follicular-derived thyroid cancer: results of a phase II clinical trial. J Clin Endocrinol Metab (2017) 102(2):698–707. doi: 10.1210/jc.2016-2525
61. Hanna GJ, Busaidy NL, Chau NG, Wirth LJ, Barletta JA, Calles A, et al. Genomic correlates of response to everolimus in aggressive radioiodine-refractory thyroid cancer: a phase II study. Clin Cancer Res (2018) 24(7):1546–53. doi: 10.1158/1078-0432.CCR-17-2297
62. Sherman EJ, Foster NR, Su YB, Shergill A, Ho AL, Konda B, et al. Randomized phase II study of sorafenib with or without everolimus in patients with radioactive iodine refractory hürthle cell thyroid cancer (HCC) (Alliance A091302/ITOG 1706). J Clin Oncol (2021) 39(15_suppl):6076.
63. Bible KC, Suman VJ, Molina JR, Smallridge RC, Maples WJ, Menefee ME, et al. Efficacy of pazopanib in progressive, radioiodine-refractory, metastatic differentiated thyroid cancers: results of a phase 2 consortium study. Lancet Oncol (2010) 11(10):962–72. doi: 10.1016/S1470-2045(10)70203-5
64. Bible KC, Menefee ME, Lin CJ, Millward MJ, Maples WJ, Goh BC, et al. An international phase 2 study of pazopanib in progressive and metastatic thyroglobulin antibody negative radioactive iodine refractory differentiated thyroid cancer. Thyroid (2020) 30(9):1254–62. doi: 10.1089/thy.2019.0269
65. Bikas A, Kundra P, Desale S, Mete M, O’Keefe K, Clark BG, et al. Phase 2 clinical trial of sunitinib as adjunctive treatment in patients with advanced differentiated thyroid cancer. Eur J Endocrinol (2016) 174(3):373–80. doi: 10.1530/EJE-15-0930
66. Carr LL, Mankoff DA, Goulart BH, Eaton KD, Capell PT, Kell EM, et al. Phase II study of daily sunitinib in FDG-PET-positive, iodine-refractory differentiated thyroid cancer and metastatic medullary carcinoma of the thyroid with functional imaging correlation. Clin Cancer Res (2010) 16(21):5260–8. doi: 10.1158/1078-0432.CCR-10-0994
67. Ravaud A, de la Fouchardiere C, Caron P, Doussau A, Do Cao C, Asselineau J, et al. A multicenter phase II study of sunitinib in patients with locally advanced or metastatic differentiated, anaplastic or medullary thyroid carcinomas: mature data from the THYSU study. Eur J Cancer. (2017) 76:110–7. doi: 10.1016/j.ejca.2017.01.029
68. Leboulleux S, Bastholt L, Krause T, de la Fouchardiere C, Tennvall J, Awada A, et al. Vandetanib in locally advanced or metastatic differentiated thyroid cancer: a randomised, double-blind, phase 2 trial. Lancet Oncol (2012) 13(9):897–905. doi: 10.1016/S1470-2045(12)70335-2
69. Capdevila J, Trigo JM, Aller J, Manzano JL, Adrian SG, Llopis CZ, et al. Axitinib treatment in advanced RAI-resistant differentiated thyroid cancer (DTC) and refractory medullary thyroid cancer (MTC). Eur J Endocrinol (2017) 177(4):309–17. doi: 10.1530/EJE-17-0243
70. Cohen EE, Rosen LS, Vokes EE, Kies MS, Forastiere AA, Worden FP, et al. Axitinib is an active treatment for all histologic subtypes of advanced thyroid cancer: results from a phase II study. J Clin Oncol (2008) 26(29):4708–13. doi: 10.1200/JCO.2007.15.9566
71. Locati LD, Licitra L, Agate L, Ou SH, Boucher A, Jarzab B, et al. Treatment of advanced thyroid cancer with axitinib: phase 2 study with pharmacokinetic/pharmacodynamic and quality-of-life assessments. Cancer (2014) 120(17):2694–703. doi: 10.1002/cncr.28766
72. Lim SM, Chung WY, Nam KH, Kang SW, Lim JY, Kim HG, et al. An open label, multicenter, phase II study of dovitinib in advanced thyroid cancer. Eur J Cancer. (2015) 51(12):1588–95. doi: 10.1016/j.ejca.2015.05.020
73. Hofmann MC, Kunnimalaiyaan M, Wang JR, Busaidy NL, Sherman SI, Lai SY, et al. Molecular mechanisms of resistance to kinase inhibitors and redifferentiation in thyroid cancers. Endocr Relat Cancer. (2022) 29(11):R173–R90. doi: 10.1530/ERC-22-0129
74. Casanovas O, Hicklin DJ, Bergers G, Hanahan D. Drug resistance by evasion of antiangiogenic targeting of VEGF signaling in late-stage pancreatic islet tumors. Cancer Cell (2005) 8(4):299–309. doi: 10.1016/j.ccr.2005.09.005
75. Fischer C, Jonckx B, Mazzone M, Zacchigna S, Loges S, Pattarini L, et al. Anti-PlGF inhibits growth of VEGF(R)-inhibitor-resistant tumors without affecting healthy vessels. Cell (2007) 131(3):463–75. doi: 10.1016/j.cell.2007.08.038
76. Zhu YJ, Zheng B, Wang HY, Chen L. New knowledge of the mechanisms of sorafenib resistance in liver cancer. Acta Pharmacol Sin (2017) 38(5):614–22. doi: 10.1038/aps.2017.5
77. Corcoran RB, Ebi H, Turke AB, Coffee EM, Nishino M, Cogdill AP, et al. EGFR-mediated re-activation of MAPK signaling contributes to insensitivity of BRAF mutant colorectal cancers to RAF inhibition with vemurafenib. Cancer Discovery (2012) 2(3):227–35. doi: 10.1158/2159-8290.CD-11-0341
78. Nazarian R, Shi H, Wang Q, Kong X, Koya RC, Lee H, et al. Melanomas acquire resistance to b-RAF(V600E) inhibition by RTK or n-RAS upregulation. Nature (2010) 468(7326):973–7. doi: 10.1038/nature09626
79. Cabanillas ME, de Souza JA, Geyer S, Wirth LJ, Menefee ME, Liu SV, et al. Cabozantinib as salvage therapy for patients with tyrosine kinase inhibitor-refractory differentiated thyroid cancer: results of a multicenter phase II international thyroid oncology group trial. J Clin Oncol (2017) 35(29):3315–21. doi: 10.1200/JCO.2017.73.0226
80. Iesato A, Li S, Roti G, Hacker MR, Fischer AH, Nucera C. Lenvatinib targets PDGFR-beta pericytes and inhibits synergy with thyroid carcinoma cells: novel translational insights. J Clin Endocrinol Metab (2021) 106(12):3569–90. doi: 10.1210/clinem/dgab552
81. Iesato A, Nucera C. Tumor microenvironment-associated pericyte populations may impact therapeutic response in thyroid cancer. Adv Exp Med Biol (2021) 1329:253–69. doi: 10.1007/978-3-030-73119-9_14
82. Prete A, Lo AS, Sadow PM, Bhasin SS, Antonello ZA, Vodopivec DM, et al. Pericytes elicit resistance to vemurafenib and sorafenib therapy in thyroid carcinoma via the TSP-1/TGFbeta1 axis. Clin Cancer Res (2018) 24(23):6078–97. doi: 10.1158/1078-0432.CCR-18-0693
83. Zhu L, Zhang X, Zhang S, Zhang Q, Cao L, Zhang Y, et al. Cancer-associated fibroblasts in papillary thyroid carcinoma. Clin Exp Med (2023). doi: 10.1007/s10238-023-00998-2
84. Khan HY, Ge J, Nagasaka M, Aboukameel A, Mpilla G, Muqbil I, et al. Targeting XPO1 and PAK4 in 8505C anaplastic thyroid cancer cells: putative implications for overcoming lenvatinib therapy resistance. Int J Mol Sci (2019) 21(1):237. doi: 10.3390/ijms21010237
85. Marcucci F, Stassi G, De Maria R. Epithelial-mesenchymal transition: a new target in anticancer drug discovery. Nat Rev Drug Discovery (2016) 15(5):311–25. doi: 10.1038/nrd.2015.13
86. Duquette M, Sadow PM, Husain A, Sims JN, Antonello ZA, Fischer AH, et al. Metastasis-associated MCL1 and P16 copy number alterations dictate resistance to vemurafenib in a BRAFV600E patient-derived papillary thyroid carcinoma preclinical model. Oncotarget (2015) 6(40):42445–67. doi: 10.18632/oncotarget.6442
87. Bagheri-Yarmand R, Busaidy NL, McBeath E, Danysh BP, Evans KW, Moss TJ, et al. RAC1 alterations induce acquired dabrafenib resistance in association with anaplastic transformation in a papillary thyroid cancer patient. Cancers (Basel) (2021) 13(19). doi: 10.3390/cancers13194950
88. Danysh BP, Rieger EY, Sinha DK, Evers CV, Cote GJ, Cabanillas ME, et al. Long-term vemurafenib treatment drives inhibitor resistance through a spontaneous KRAS G12D mutation in a BRAF V600E papillary thyroid carcinoma model. Oncotarget (2016) 7(21):30907–23. doi: 10.18632/oncotarget.9023
89. Cabanillas ME, Dadu R, Iyer P, Wanland KB, Busaidy NL, Ying A, et al. Acquired secondary RAS mutation in BRAF(V600E)-mutated thyroid cancer patients treated with BRAF inhibitors. Thyroid (2020) 30(9):1288–96. doi: 10.1089/thy.2019.0514
90. Subbiah V, Shen T, Terzyan SS, Liu X, Hu X, Patel KP, et al. Structural basis of acquired resistance to selpercatinib and pralsetinib mediated by non-gatekeeper RET mutations. Ann Oncol (2021) 32(2):261–8. doi: 10.1016/j.annonc.2020.10.599
91. Fancelli S, Caliman E, Mazzoni F, Brugia M, Castiglione F, Voltolini L, et al. Chasing the target: new phenomena of resistance to novel selective RET inhibitors in lung cancer. updated evidence and future perspectives. Cancers (Basel) (2021) 13(5):1091. doi: 10.3390/cancers13051091
92. Lu C, Zhou Q. Diagnostics, therapeutics and RET inhibitor resistance for RET fusion-positive non-small cell lung cancers and future perspectives. Cancer Treat Rev (2021) 96:102153. doi: 10.1016/j.ctrv.2021.102153
93. Solomon BJ, Tan L, Lin JJ, Wong SQ, Hollizeck S, Ebata K, et al. RET solvent front mutations mediate acquired resistance to selective RET inhibition in RET-driven malignancies. J Thorac Oncol (2020) 15(4):541–9. doi: 10.1016/j.jtho.2020.01.006
94. Fares CM, Van Allen EM, Drake CG, Allison JP, Hu-Lieskovan S. Mechanisms of resistance to immune checkpoint blockade: why does checkpoint inhibitor immunotherapy not work for all patients? Am Soc Clin Oncol Educ Book (2019) 39:147–64. doi: 10.1200/EDBK_240837
95. Moretti S, Menicali E, Nucci N, Guzzetti M, Morelli S, Puxeddu E. THERAPY OF ENDOCRINE DISEASE immunotherapy of advanced thyroid cancer: from bench to bedside. Eur J Endocrinol (2020) 183(2):R41–55. doi: 10.1530/EJE-20-0283
96. Angell TE, Lechner MG, Jang JK, LoPresti JS, Epstein AL. MHC class I loss is a frequent mechanism of immune escape in papillary thyroid cancer that is reversed by interferon and selumetinib treatment in vitro. Clin Cancer Res (2014) 20(23):6034–44. doi: 10.1158/1078-0432.CCR-14-0879
97. French JD, Kotnis GR, Said S, Raeburn CD, McIntyre RC Jr., Klopper JP, et al. Programmed death-1+ T cells and regulatory T cells are enriched in tumor-involved lymph nodes and associated with aggressive features in papillary thyroid cancer. J Clin Endocrinol Metab (2012) 97(6):E934–43. doi: 10.1210/jc.2011-3428
98. Severson JJ, Serracino HS, Mateescu V, Raeburn CD, McIntyre RC Jr., Sams SB, et al. PD-1+Tim-3+ CD8+ T lymphocytes display varied degrees of functional exhaustion in patients with regionally metastatic differentiated thyroid cancer. Cancer Immunol Res (2015) 3(6):620–30. doi: 10.1158/2326-6066.CIR-14-0201
99. Ulisse S, Tuccilli C, Sorrenti S, Antonelli A, Fallahi P, D’Armiento E, et al. PD-1 ligand expression in epithelial thyroid cancers: potential clinical implications. Int J Mol Sci (2019) 20(6):1405. doi: 10.3390/ijms20061405
100. Mehnert JM, Varga A, Brose MS, Aggarwal RR, Lin CC, Prawira A, et al. Safety and antitumor activity of the anti-PD-1 antibody pembrolizumab in patients with advanced, PD-L1-positive papillary or follicular thyroid cancer. BMC Cancer. (2019) 19(1):196. doi: 10.1186/s12885-019-5380-3
101. Haugen B, French J, Worden FP, Konda B, Sherman EJ, Dadu R, et al. Lenvatinib plus pembrolizumab combination therapy in patients with radioiodine-refractory (RAIR), progressive differentiated thyroid cancer (DTC): results of a multicenter phase II international thyroid oncology group trial. J Clin Oncol (2020) 38(15_suppl):6512. doi: 10.1200/JCO.2020.38.15_suppl.6512
102. Makker V, Colombo N, Casado Herraez A, Santin AD, Colomba E, Miller DS, et al. Lenvatinib plus pembrolizumab for advanced endometrial cancer. N Engl J Med (2022) 386(5):437–48. doi: 10.1056/NEJMoa2108330
103. Choueiri TK, Powles T, Burotto M, Escudier B, Bourlon MT, Zurawski B, et al. Nivolumab plus cabozantinib versus sunitinib for advanced renal-cell carcinoma. N Engl J Med (2021) 384(9):829–41. doi: 10.1056/NEJMoa2026982
104. Taylor M DG, Thein K, Larlot Y, Khan S, Goldschmidt J, Lebellec L, et al. Cabozantinib in combination with atezolizumab as first line thrapy in patients with radioaiodine-refractory differentiated thyroid: results from cohort of 18 of the phase 1B COSMIC-21 study. Thyroid (2022) 32(S1).
105. Lorch JH, Barletta JA, Nehs M, Uppaluri R, Alexander EK, Haddad RI, et al. A phase II study of nivolumab (N) plus ipilimumab (I) in radioidine refractory differentiated thyroid cancer (RAIR DTC) with exploratory cohorts in anaplastic (ATC) and medullary thyroid cancer (MTC). J Clin Oncol (2020) 38(15_suppl):6513. doi: 10.1200/JCO.2020.38.15_suppl.6513
106. Konda B SE, Masarelli E, Xia B, Muzaffar J, Morris J, Ryder m, et al. Cabozantinb in combination with nivolumab and ipilimumab in patients with radioactive iodine-refractory differentiated thyroid cancer whose cancer progressed after one prior VEGFR targeted therapy: interim results of a multicenter phase 2 NCI-ITOG trial (NCI#10240). Thyroid (2022) 32(S1).
107. Caillou B, Troalen F, Baudin E, Talbot M, Filetti S, Schlumberger M, et al. Na+/I- symporter distribution in human thyroid tissues: an immunohistochemical study. J Clin Endocrinol Metab (1998) 83(11):4102–6. doi: 10.1210/jc.83.11.4102
108. Fletcher A, Read ML, Thornton CEM, Larner DP, Poole VL, Brookes K, et al. Targeting novel sodium iodide symporter interactors ADP-ribosylation factor 4 and valosin-containing protein enhances radioiodine uptake. Cancer Res (2020) 80(1):102–15. doi: 10.1158/0008-5472.CAN-19-1957
109. Faria M, Vareda J, Miranda M, Bugalho MJ, Silva AL, Matos P. Adherens junction integrity is a critical determinant of sodium iodide symporter residency at the plasma membrane of thyroid cells. Cancers (Basel). (2022) 14(21):5362. doi: 10.3390/cancers14215362
110. Buffet C, Wassermann J, Hecht F, Leenhardt L, Dupuy C, Groussin L, et al. Redifferentiation of radioiodine-refractory thyroid cancers. Endocr Relat Cancer. (2020) 27(5):R113–R32. doi: 10.1530/ERC-19-0491
111. Lamartina L, Anizan N, Dupuy C, Leboulleux S, Schlumberger M. Redifferentiation-facilitated radioiodine therapy in thyroid cancer. Endocr Relat Cancer (2021) 28(10):T179–T91. doi: 10.1530/ERC-21-0024
112. Ho AL, Grewal RK, Leboeuf R, Sherman EJ, Pfister DG, Deandreis D, et al. Selumetinib-enhanced radioiodine uptake in advanced thyroid cancer. N Engl J Med (2013) 368(7):623–32. doi: 10.1056/NEJMoa1209288
113. Rothenberg SM, Daniels GH, Wirth LJ. Redifferentiation of iodine-refractory BRAF V600E-mutant metastatic papillary thyroid cancer with dabrafenib-response. Clin Cancer Res (2015) 21(24):5640–1. doi: 10.1158/1078-0432.CCR-15-2298
114. Dunn LA, Sherman EJ, Baxi SS, Tchekmedyian V, Grewal RK, Larson SM, et al. Vemurafenib redifferentiation of BRAF mutant, RAI-refractory thyroid cancers. J Clin Endocrinol Metab (2019) 104(5):1417–28. doi: 10.1210/jc.2018-01478
115. Weber M, Kersting D, Riemann B, Brandenburg T, Fuhrer-Sakel D, Grunwald F, et al. Enhancing radioiodine incorporation into radioiodine-refractory thyroid cancer with MAPK inhibition (ERRITI): a single-center prospective two-arm study. Clin Cancer Res (2022) 28(19):4194–202. doi: 10.1158/1078-0432.CCR-22-0437
116. Leboulleux S, Do Cao C, Zerdoud S, Attard M, Bournaud C, Lacroix L, et al. A phase II redifferentiation trial with dabrafenib-trametinib and 131I in metastatic radioactive iodine refractory BRAF p.V600E mutated differentiated thyroid cancer. Clin Cancer Res (2023) CCR-23-0046. doi: 10.1158/1078-0432.c.6641715.v1
117. Burman B, Tuttle RM, Grewal RK, Sherman EJ, Baxi SS, Boucai L, et al. Phase 2 of trametinib plus radioiodine in RAS-mutant and wild-type, radioiodine-refractory thyroid cancer (ETCTN9446). J Clin Oncol (2022) 40(16_suppl):6089. doi: 10.1200/JCO.2022.40.16_suppl.6089
118. Jaber T, Waguespack SG, Cabanillas ME, Elbanan M, Vu T, Dadu R, et al. Targeted therapy in advanced thyroid cancer to resensitize tumors to radioactive iodine. J Clin Endocrinol Metab (2018) 103(10):3698–705. doi: 10.1210/jc.2018-00612
119. Iravani A, Solomon B, Pattison DA, Jackson P, Ravi Kumar A, Kong G, et al. Mitogen-activated protein kinase pathway inhibition for redifferentiation of radioiodine refractory differentiated thyroid cancer: an evolving protocol. Thyroid (2019) 29(11):1634–45. doi: 10.1089/thy.2019.0143
120. Groussin L, Clerc J, Huillard O. Larotrectinib-enhanced radioactive iodine uptake in advanced thyroid cancer. N Engl J Med (2020) 383(17):1686–7. doi: 10.1056/NEJMc2023094
121. Chan HP, Chen IF, Tsai FR, Kao CH, Shen DH. Reversing “Flip-flop” phenomenon of 131 I and glucose avidity in RET-fusion positive radioiodine-refractory thyroid cancer lesions after treatment of pralsetinib. Clin Nucl Med (2023) 48(3):e147–e8. doi: 10.1097/RLU.0000000000004475
122. Lee YA, Lee H, Im SW, Song YS, Oh DY, Kang HJ, et al. NTRK and RET fusion-directed therapy in pediatric thyroid cancer yields a tumor response and radioiodine uptake. J Clin Invest (2021) 131(18):e144847. doi: 10.1172/JCI144847
123. Katoh H, Kajita S, Yokota M, Sengoku N, Sangai T. Neoadjuvant use of lenvatinib in locally advanced papillary thyroid carcinoma involving critical vessels. Int J Endocrine Oncol (2020) 7(3):IJE33. doi: 10.2217/ije-2020-0014
124. Nava CF, Scheffel RS, Cristo AP, Ferreira CV, Weber S, Zanella AB, et al. Neoadjuvant multikinase inhibitor in patients with locally advanced unresectable thyroid carcinoma. Front Endocrinol (Lausanne). (2019) 10:712. doi: 10.3389/fendo.2019.00712
125. Huang N-s, Wang Y, Wei W-j, Xiang J, Chen J-y, Guan Q, et al. A systematic review of neoadjuvant targeted therapy in locally advanced thyroid cancer. Holistic Integr Oncol (2022) 1(1):16. doi: 10.1007/s44178-022-00016-7
126. Cabanillas M, Busaidy N, Zafereo M, Waguespack S, Hu M, Hofmann M, et al. Neoadjuvant vemurafenib in patients with locally advanced papillary thyroid cancer (PTC). Eur Thyroid J (2017) 6(Suppl 1):38.
127. Ding J, Li D, Liu X, Hei H, Sun B, Zhou D, et al. Chimeric antigen receptor T-cell therapy for relapsed and refractory thyroid cancer. Exp Hematol Oncol (2022) 11(1):59. doi: 10.1186/s40164-022-00311-z
128. Edeline J, Houot R, Marabelle A, Alcantara M. CAR-T cells and BiTEs in solid tumors: challenges and perspectives. J Hematol Oncol (2021) 14(1):65. doi: 10.1186/s13045-021-01067-5
Keywords: drug resistance, immunotherapy, neoadjuvant, metastatic thyroid cancer, radioiodine refractoriness, tyrosine kinase inhibitor, novel treatments
Citation: Hamidi S, Hofmann M-C, Iyer PC, Cabanillas ME, Hu MI, Busaidy NL and Dadu R (2023) Review article: new treatments for advanced differentiated thyroid cancers and potential mechanisms of drug resistance. Front. Endocrinol. 14:1176731. doi: 10.3389/fendo.2023.1176731
Received: 28 February 2023; Accepted: 05 June 2023;
Published: 26 June 2023.
Edited by:
Joana Simões-Pereira, Instituto Português de Oncologia de Lisboa Francisco Gentil, PortugalReviewed by:
Alessandro Prete, University of Pisa, ItalyCopyright © 2023 Hamidi, Hofmann, Iyer, Cabanillas, Hu, Busaidy and Dadu. This is an open-access article distributed under the terms of the Creative Commons Attribution License (CC BY). The use, distribution or reproduction in other forums is permitted, provided the original author(s) and the copyright owner(s) are credited and that the original publication in this journal is cited, in accordance with accepted academic practice. No use, distribution or reproduction is permitted which does not comply with these terms.
*Correspondence: Ramona Dadu, cmRhZHVAbWRhbmRlcnNvbi5vcmc=
Disclaimer: All claims expressed in this article are solely those of the authors and do not necessarily represent those of their affiliated organizations, or those of the publisher, the editors and the reviewers. Any product that may be evaluated in this article or claim that may be made by its manufacturer is not guaranteed or endorsed by the publisher.
Research integrity at Frontiers
Learn more about the work of our research integrity team to safeguard the quality of each article we publish.