- 1Fujian Provincial Key Laboratory of Reproductive Health Research, School of Medicine, Xiamen University, Xiamen, China
- 2Fujian Provincial Key Laboratory for Developmental Biology and Neurosciences, College of Life Sciences, Fujian Normal University, Fuzhou, China
With the acceleration of life pace and the increase of work pressure, the problem of male infertility has become a social problem of general concern. Sphingolipids are important regulators of many cellular processes like cell differentiation and apoptosis, which are ubiquitously expressed in all mammalian cells. Various sphingolipid catabolic enzymes can generate multiple sphingolipids like sphingosine-1-phosphate and sphingomyelin. Present studies have already demonstrated the role of steroid hormones in the physiological processes of reproduction and development through hypothalamus-pituitary-gonad axis, while recent researches also found not only sphingolipids can modulate steroid hormone secretion, but also steroid hormones can control sphingolipid metabolites, indicating the role of sphingolipid metabolites in the homeostasis of steroid hormones. Furthermore, sphingolipid metabolites not only contribute to the regulation of gametogenesis, but also mediate damage-induced germ apoptosis, implying the role of sphingolipid metabolites in the maintenance of testicular functions. Together, sphingolipid metabolites are involved in impaired gonadal function and infertility in males, and further understanding of these bioactive sphingolipids will help us develop new therapeutics for male infertility in the future.
1 Introduction
Sphingolipids are a lipid family with a common sphingomyelin base skeleton, which participates in various physiopathological processes of cells, including steroid production, cell differentiation and apoptosis (1–12). In the mammalian reproductive system, many types of cells can produce sphingolipid metabolites, especially ceramide and sphingosine-1-phosphate, which are the second messengers (13–18). Therefore, sphingolipid metabolites in reproductive system have attracted much attention in recent years.
Steroid hormone is an important regulator of many physiological processes in mammalians (19–21). It is specifically synthesized in steroid-producing tissues through a series of multi-step reactions under the catalysis of monooxygenase and hydroxysteroid dehydrogenase superfamily members (20–23). It plays a very important regulatory role in maintaining testicular functions (19–27). Many sphingolipid metabolites can regulate the signaling pathways of steroid production, through acting as a second messenger in the signaling cascade to participate in the regulation of reproductive functions (5, 15, 17, 27–29).
Recent studies have shown that sphingolipid metabolites not only participate in the regulation of steroid biosynthesis (5, 16, 17, 21, 22, 24, 30), but also participate in the production of male sperm, and mediate the apoptosis of germ cells induced by stress or injury (9, 15, 27, 28), indicating that sphingolipids may be related to the impairment of gonadal function and infertility. In general, this research field provides an exciting direction for basic biology and clinical medicine, which can further study the possible role of abnormal sphingolipids in male infertility.
In the present review, we summarized the research progress on the role of sphingolipid metabolites in steroid hormone balance and testicular function maintenance during recent years, providing a theoretical basis to develop new therapies for male fertility.
2 Sphingolipid and its metabolites
Sphingolipids have always been considered as the structural inert component of the cell membrane with sphingomyelin base skeleton (31–33). Okazaki et al. found that in various types of cells, different stimuli can promote sphingomyelinase to split sphingomyelin, and then send signals (31, 34). Since sphingosine can inhibit PKC (35–37), people have generated great interest in sphingomyelin metabolites.
The biosynthesis of sphingolipids is from serine and palmitoyl CoA, and finally converted into ceramide, the precursor of all sphingolipids (33). The process of sphingolipid metabolism includes two stages, de novo synthesis (Figure 1A) and following salvage (Figure 1B). More and more evidences show that sphingolipids, as a structural component of cell membrane, have a signaling transduction function (11, 31, 32), and the special membrane micro-region rich in cholesterol and sphingolipid is considered as the center of signal molecular organization, participating in the signaling transduction of G protein-coupled receptor and growth factor receptor (9, 31, 32). Therefore, sphingolipids are widely considered to play an important role in cell signaling at present.
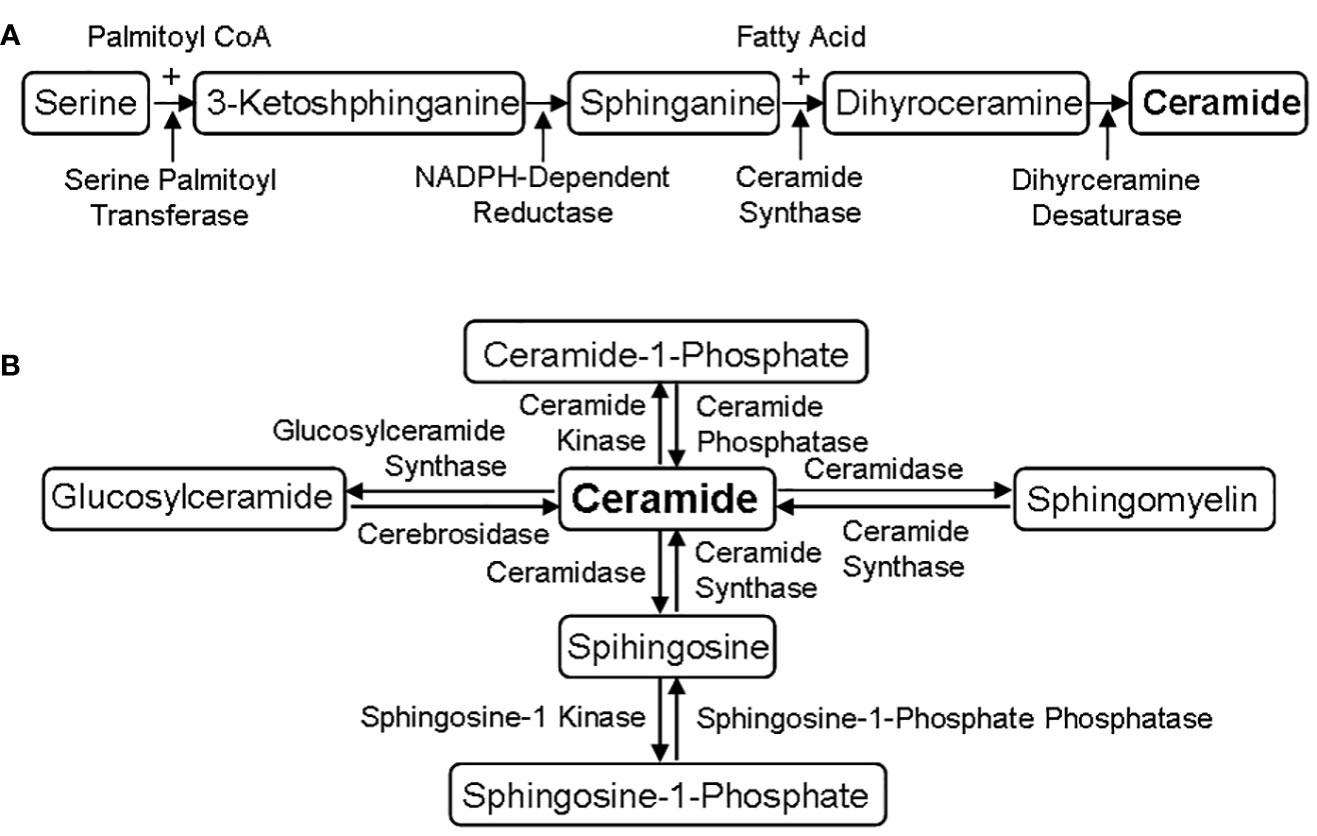
Figure 1 Sphingolipid metabolic pathways. (A) De novo synthesis of sphingolipids. The de novo biosynthesis of sphingolipids is from serine and palmitoyl CoA, and finally converted into ceramide through four-step reactions under the catalysis of serine palmitoyl transferase, NADPH-dependent reductase, ceramide synthase and dihyrceramine desaturase. (B) Different salvage of sphingolipids. Ceramide is the precursor of all sphingolipids, which can be converted into other phospholipids under the action of specific enzymes, such as ceramide synthase and ceramidase.
At present, a variety of sphingolipid metabolites have been found and their physiological effects have also been clarified, such as glycosphingolipids (31, 33, 38). These molecules are similar in structure and can be transformed into each other, but their biological functions are different (31, 38). Given the low concentration of these sphingolipid metabolites, they may function as an important signaling molecules participating in various cell events, like cell cycle arrest, apoptosis and intercellular interaction, such as S1P and C1P (31, 39). C1P is the main metabolite of ceramide, which can inhibit cell apoptosis and induce cell survival (18, 35, 39), besides prostaglandin synthesis and arachidonic acid release (5, 19, 27). Like ceramide, SPH participates in apoptosis (5, 15) and also inhibits PKC (37, 40) and PLD (41). Noteworthy, sphingolipid metabolites are being extensively studied as potential anticancer targets, since many intracellular and extracellular cytokine regulates the dynamic balance between sphingolipid metabolites through ceramidase, SK and S1P phosphatase (3, 18, 30, 31, 34).
3 Testicular synthesis of steroid hormones
The biosynthesis of steroid hormones is from cholesterol through the sequence activity of CYP and HSD, mainly occurring in tissues such as testis, ovary, adrenal gland and placenta (Figure 2) (5, 15, 21, 27, 30, 38)
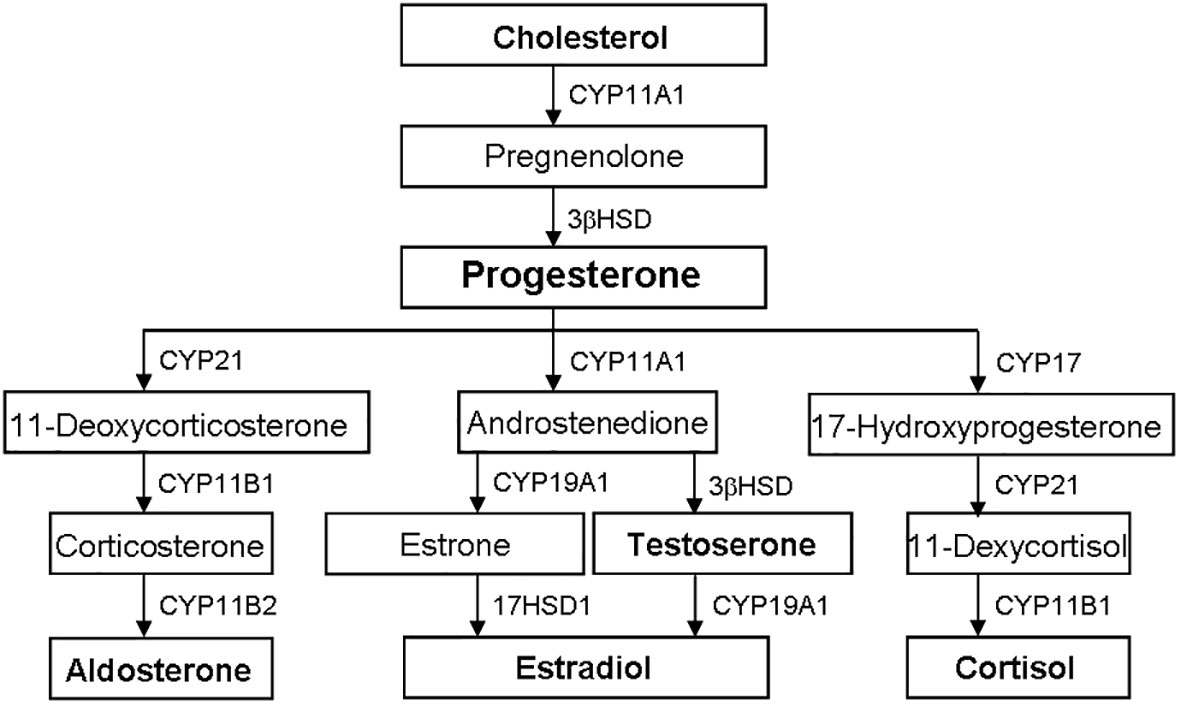
Figure 2 Biosynthetic pathways of steroid hormones. The biosynthesis of steroid hormones is from cholesterol through the sequence activity of CYP and HSD. Progesterone is the precursor of other steroid hormones, such as aldosterone, testosterone, estradiol and cortisol. CYP, cytochrome P450; HSD, hydroxysteroid dehydrogenase.
The main site of androgen biosynthesis is testis, and the expression of tissue-specific steroid gene is the reason for the difference of sex hormones (5, 17, 21). When the peptide hormone is combined with the homologous GPCR in the target tissue, the steroid biosynthesis is started, and then the steroid hormone is synthesized through the two different time stages of acute and chronic response stages, so as to ensure its secretion in an appropriate and controlled manner (5, 21, 30).
The acute response stage of steroid biosynthesis involves StAR activation. StAR can promote the rapid mobilization of cholesterol from the outer to the inner layer of mitochondrial membrane (42–44). Besides rate-limiting enzyme StAR, other proteins like PBR, HSL and SR-BI are also involved (42, 44–46).
The chronic response stage of steroid biosynthesis involves the transcription activation of steroid hormone genes. This process is mainly through activating various signaling like cAMP/PKA by adenylate cyclase for transcriptional activation (5, 21). SF-1 is one of the main transcription factors during this process, whose activity is regulated by phosphorylation and acetylation (38, 47, 48).
Many peptide hormones are involved in the initiation of steroidogenesis, such as FSH, LH and ACTH (16, 21). The combination of these peptide hormones to their homologous receptors leads to downstream signaling activation, mainly cAMP/PKA signaling, thus activating many target transcriptions (Figure 3) (5, 17). Besides cAMP/PKA, other signaling of cytokines and sphingolipids are also involved in regulating the synthesis of steroid hormones (5). The biosynthesis of testicular hormones is closely related to the specific expression profiles of steroid hormone synthases, such as CYP11A1 and 3βHSD, which are expressed in testis (21).
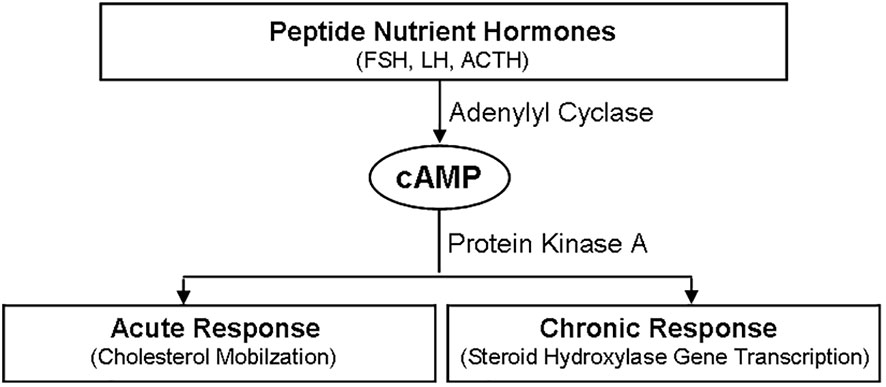
Figure 3 Regulatory Effects of peptide hormones on steroidogenesis. Peptide hormones, such FSH, LH and ACTH, initiate the synthesis of steroid hormones through cAMP/PKA signaling pathway, which includes two different time stages. One is the acute response stage, which is characterized by cholesterol mobilization. And the other is the chronic response stage, which is characterized by steroid hydroxylase gene transcription. FSH, follicle stimulating hormone; LH, luteinizing hormone; ACTH, adreno-cortico-tropic-hormone; PKA, protein kinase A; cAMP, cyclic adenosine monophosphate.
4 The role of sphingolipid metabolites in the synthesis of steroid hormones
Although cAMP/PKA is main signaling, studies have found that sphingolipids can be used as the second regulator of steroid biosynthesis (Figure 4) (17, 21). For example, ACTH/cAMP can reduce the number of sphingomyelin, ceramide and SPH in the cells, and increase S1P production through SK activation (16). Bioactive sphingolipids can not only regulate the expression of CYP (5), but also act as the ligand of SF-1 (17, 47). Therefore, sphingolipids have been identified as one important synthesis regulator.
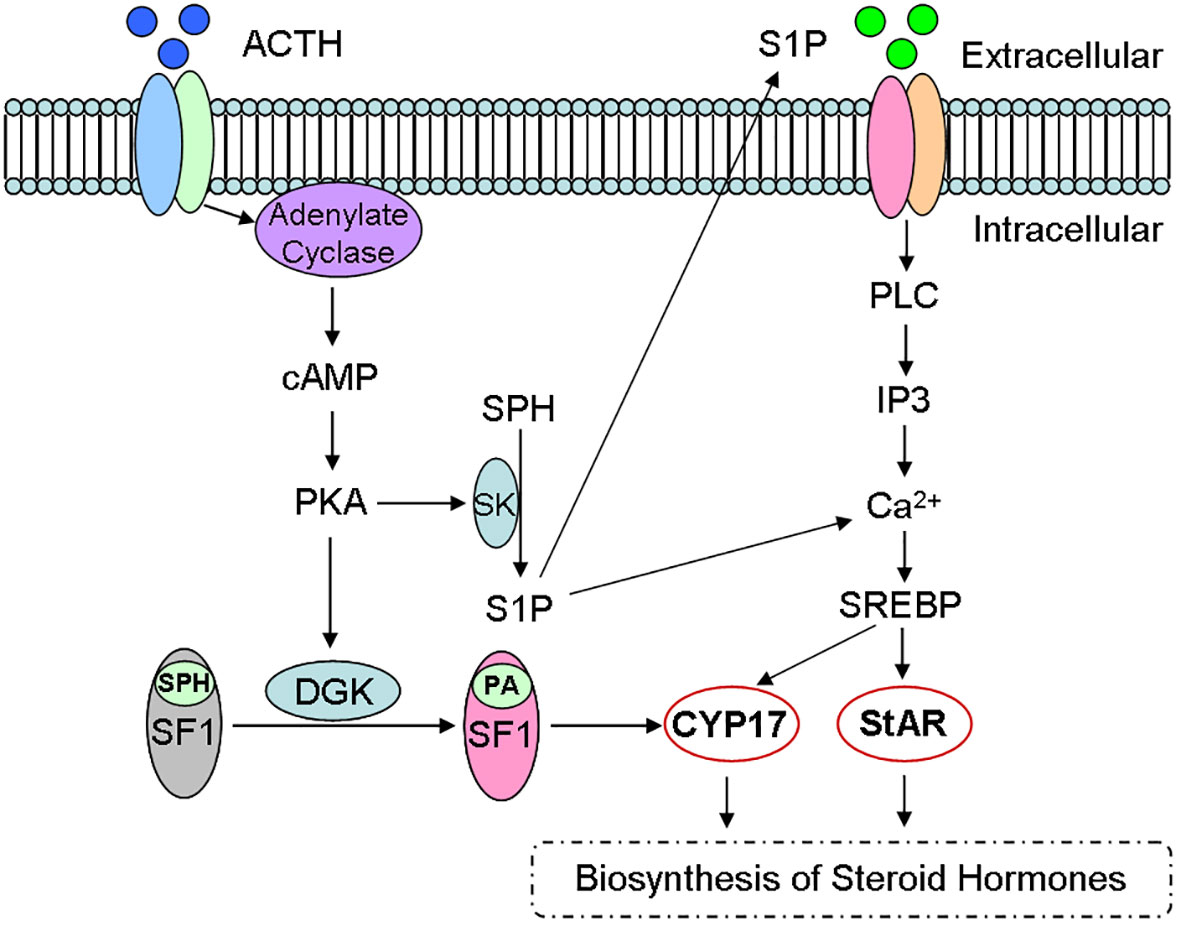
Figure 4 Sphingolipid metabolites and steroid biosynthesis. Steroid hormones are synthesized mainly via cAMP/PKA signaling pathway and sphingolipids act as the second regulator during the steroidogenesis. For example, ACTH activates cAMP/PKA signaling, reduces sphingomyelin number and increases S1P production, which participates in the biosynthesis of Steroid hormones through CYP and StAR activity. ACTH, adreno corticotropic hormone; cAMP, cyclic adenosine monophosphate; PKA, protein kinase A; DGK, diacylglycerol kinase; SPH, sphingosine; SF-1, steroid forming factor-1; PA, phosphatidic acid; SK, sphingosine kinase; S1P, sphingomyelin-1-phosphate; CYP, cytochrome P450; StAR, steroidogenic acute regulatory protein.
4.1 Ceramide and steroid biosynthesis
Ceramide can regulate cytokine-mediated signaling, like TNF-α, FasL, INF-γ and IL-1β, leading to the changes in steroid biosynthesis (17, 30, 49). They regulates ceramide concentration through activating intracellular sphingomyelinases (9, 38), but the mechanism of ceramide functions still remains to be further studied.
In Leydig cells, TNF-α and IL-1β can not only activate sphingomyelinases and generate ceramide (49), but also reduce StAR expression and testosterone secretion (50), while ceramide can inhibit testosterone synthesis induced by hCG (49).
Ceramide can also regulate the expression and activity of steroid synthases. For example, ceramide can regulate P450c17α expression and inhibit cAMP production (49). Ceramide can also induce C/EBPβ recruitment to 11β-HSD1 promoter, thus activating gene expression (51).
Lastly, ceramide metabolism enzymes also participate in steroid biosynthesis. For example, cortisol can increase sphingosine concentration through inducing N-acylsphingosine amidohydrolase 1 expression, leading to the inhibition of SF-1 functions during the regulation of steroid biosynthesis (17).
4.2 Sphingosine and steroid biosynthesis
Sphingosine is also confirmed as a SF-1 ligand, since SF-1 binds to phospholipids (17). Therefore, sphingosine is an antagonist of SF-1. Further investigation indicates that the combination of sphingosine with SF-1 leads to a decrease of CYP17 expression (17). In addition, phosphatidic acid is the endogenous ligand of SF-1 (52), since cAMP can promote phosphatidic acid binding to SF-1 and reverse sphingosine antagonism (Figure 4).
As SF-1 ligand, sphingosine adds another regulatory level of sphingomyelins during the synthesis of steroid hormones. Notably, sphingosine may regulate the process of development and sex differentiation through antagonizing SF-1, since SF-1 also regulates many related gene expressions during these processes (17, 27, 47).
4.3 Sphingosine-1-phosphate and steroid biosynthesis
S1P signaling is activated in and out of cells by binding to specific S1P receptors (52). There are five S1P receptors, which combines with multiple heterotrimeric G proteins to activate specific intracellular targets, such as PI-3K, ERK and Rho (10–12).
The binding of S1P and its homologous receptor is the main signaling transduction, and PI-3K/Akt and ERK pathways are its downstream targets (35, 37, 52). The relationship between PI-3K/ERK activation and steroid biosynthesis further indicates the important role of S1P during the regulation of steroid biosynthesis (Figure 4) (5, 16).
4.4 Sphingomyelin and steroid biosynthesis
Sphingomyelin exists in cell membrane, which is the target of many external signals, involving in the regulation of steroid biosynthesis (5, 32, 41). Sphingomyelin can be hydrolyzed into ceramide by sphingomyelinases (41), which is mediated by many stimulators, including TNF-α, IL-1β and FasL (39, 50). For example, S1P and C1P inhibit acid sphingomyelinase activity through the negative feedback loop of sphingomyelin metabolism pathway (52).
In Leydig cells, sphingomyelin hydrolysis is associated with cholesterol migration (21). In addition, lysosomal sphingomyelin can bind to SF-1 and cAMP can promote the separation of sphingomyelin from SF-1 (17, 47). Given the dynamic balance of different sphingolipid metabolites in specific cells, sphingomyelin play an important role in the regulation of steroid biosynthesis as a precursor of ceramide, lysosomal sphingomyelin and S1P (34).
4.5 Glycosphingolipids and steroid biosynthesis
Bioactive sphingomyelins participate in steroid biosynthesis, however whether steroid hormones are involved in sphingolipid metabolism is another issue, which is exemplified in the neurosteroidogenesis between sphingomyelin and isoproterenone (53, 54). Mellon et al. found that isoprenol treatment can reduce sphingomyelin and improve neurodegeneration (30, 55). At the same time, sphingomyelin is critical for testosterone synthesis (17, 27), and testosterone can regulate the level of renal sphingomyelin (43, 52). Although the exact mechanism of molecular regulation is still unclear, these demonstrate the importance of sphingolipid metabolites and the complexity of steroid biosynthesis.
5 The role of sphingolipid metabolites in spermatogenesis
In mammals, testes are mainly composed of two functional regions, the seminiferous tubules containing germ cells and sertoli cells, and the stroma containing Leydig cells (23, 26, 56). Among them, spermatogenesis is a complex physiological process, which mainly occurs in the seminiferous tubules and is regulated by the interaction between multiple hormones (FSH and LH) and cells (24, 46, 57). The seminal vesicle contains many hydrolases, including sphingomyelinase, which is involved in sperm phospholipid hydrolysis, and closely related to sperm capacitation and fertilization (27, 29, 38).
Glycosphingolipids are widely expressed in cell membrane (58) and involved in differentiation, growth and transmembrane signaling (4, 38). For example, glycosphingolipids GM1 distributed in spermatozoa (59), and GM3 distributed in sertoli cells of seminiferous tubules (60). Analysis of sphingomyelin acyl composition of in ram sperm found that VLC PUFAs only bind with sphingomyelin, but not with other phospholipids (28).
The study of fertile and sterile mice that block the biosynthesis of glycosphingolipids found that the synthesis of acidic subgroups of fucosylated glycosphingolipids was blocked in fertile and sterile mice, but the neutral subgroup of fucosylated glycosphingolipids was only missing in sterile mice (61). Glycosphingolipids not only play a role in the secretion of testosterone (62), but also is critical to the prolonged polarization of sperm and the stability of the sperm-sertoli cell contact (29).
At the initial stage of spermatogenesis, not only fucosylated glycosphingolipids, but also ceramide and sphingomyelin (VLC-PUFA contents) firstly appear in the pachytene spermatocyte stage (63), and ceramide and sphingomyelin also appears in spermatocytes 25-27 days after birth (64), these compounds are the main components of mammalian sperm head (65).
During the last step of sperm maturation, ceramide and sphingomyelin also play a very important role in the sperm capacitation (29), After sperm was treated with the agents of sperm capacitation, such as calcium, bicarbonate and albumin, sperm sphingomyelin was degraded to ceramide, which reduced the sphingomyelin/ceramide ratio several times (64). Therefore, the hydrolysis of sphingomyelin in sperm head may be the early biochemical change of sperm before fertilization (64), but the mechanism of its action in spermatogenesis and capacitation is still unclear and needs further research.
6 The role of sphingolipid metabolites in testicular cell apoptosis
Sphingolipid metabolites play not only an important role in spermatogenesis, but a regulatory role in apoptosis of germ cells after pathological stress of testis (19, 22, 23, 26, 27, 30).
Firstly, sphingomyelinase can inhibit gonadotropin-induced testosterone synthesis in Leydig cells, reduce the binding of gonadotropin to receptors, then decrease cAMP (17, 23, 27). In mammalian testis, Leydig cells can maintain spermatogenesis and androgen synthesis (17, 23, 30, 57), indicating that sphingomyelinase not only reduces androgen synthesis, but also leads to excessive apoptosis of spermatogonia and spermatocytes.
Secondly, ceramide is involved in the process of testicular cell apoptosis mediated by various pathophysiological stresses. When male rats were treated with toxic compounds, their testicular cells were mostly Fas-positive and more sensitive to FasL (66). During the spermatogenesis, FasL/Fad is involved in the loss of germ cells (66, 67) and FasL can induce the increase of intracellular ceramide level (68). In testicular cells, Fas can activate cell apoptosis through ceramide signaling (69), indicating that Fas pathway is a sensor for toxic substances to induce testicular injury.
Thirdly, S1P may also participate in the apoptosis of testicular cells, since S1P can effectively inhibit chemotherapy-induced apoptosis of ovarian cells. Suomalainen et al. examined the changes of intracellular sphingolipids and cell apoptosis markers, and then found the elevated ceramide contents during the early apoptosis following with activated caspase 3 and appeared DNA laddering (70). Interestingly, reactive oxygen species inhibitor (n-acetyl-L-cysteine), not ceramide synthetase inhibitor (fumonisin B1) suppressed testicular apoptosis without ceramide changes, while S1P can inhibit testicular apoptosis by 30% (70). Additionally, ceramide-initiated SAPK/JNK signaling and protein phosphatase 2A activation is required during stress-induced apoptosis, such as TNF-a, FasL and X-rays (69–74).
Finally, ceramide is an important regulator of cell apoptosis (5, 9, 10, 18, 31, 35, 38, 61), which may play a role through the following three mechanisms (Figure 5). (i) Instantaneous acid sphingomyelinase activation increases the membrane ceramide, promotes receptor aggregation and subsequent apoptosis signaling transduction. (ii) Ceramide is continuously produced through the activation of neutral sphingomyelinase or ceramide activated protein phosphatase, resulting in cell apoptosis. And (iii) plasma membrane phospholipid disorder occurs, presenting sphingomyelin to neutral sphingomyelinase, producing ceramide, and then forming the morphological changes of membrane vesicles and apoptosis.
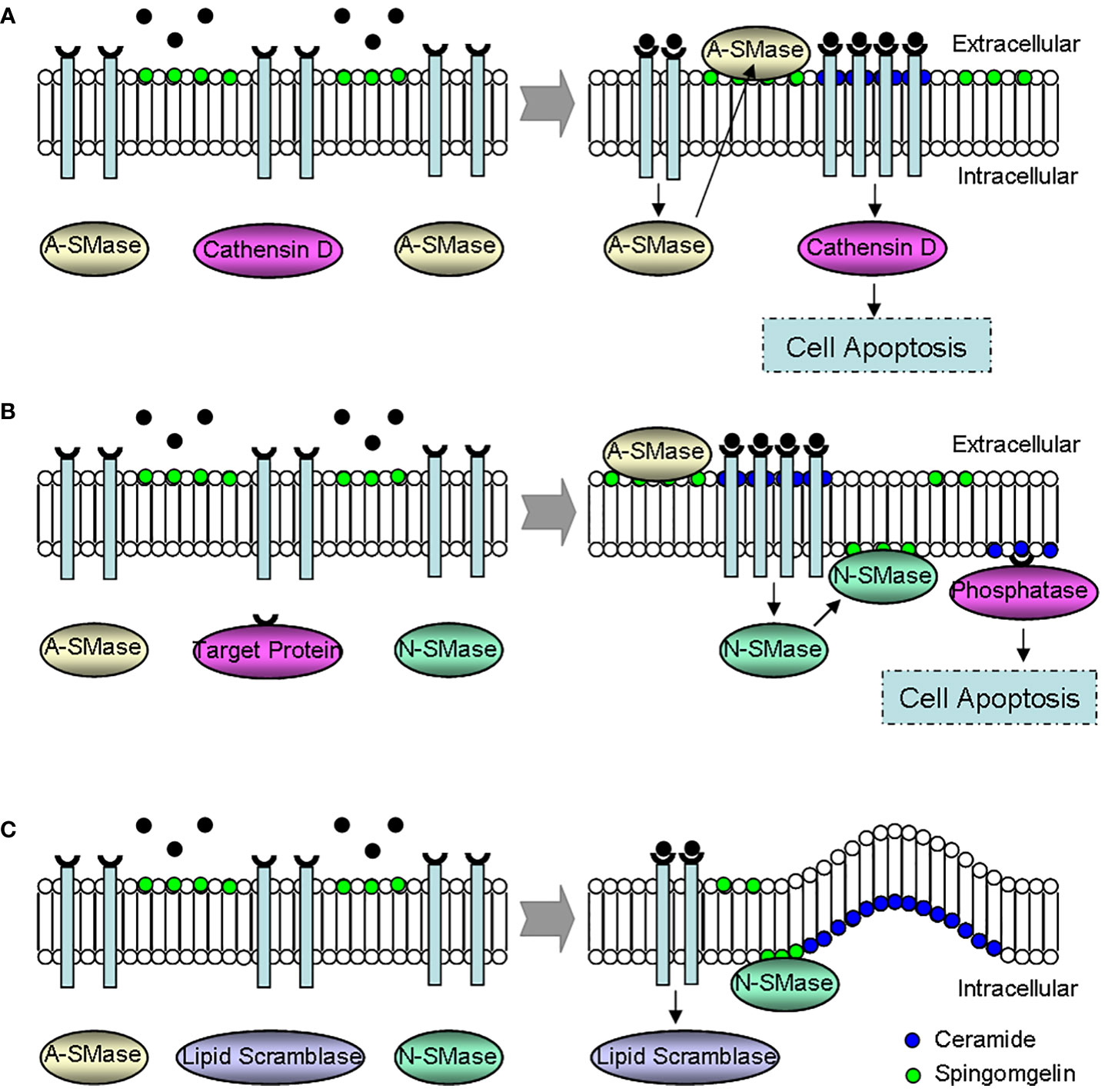
Figure 5 Effect mechanisms of ceramide during the regulation of cell apoptosis. (A) The instantaneous activation of A-SMase. A-SMase activation promotes ceramide-dependent receptor aggregation, leading to cell apoptosis via cathensin D. (B) The continuous activation of N-SMase. Ceramide induces cell apoptosis through the continuous activation of N-SMase and phosphatase. (C) The formation of membrane vesicles. The membrane morphology changes because of phospholipid disorder, and the membrane vesicle forms since N-SMase transforms sphingomyelin to excessive ceramide. N-SMase, neutral sphingomyelinase; A-SMase, acid sphingomyelinase.
At present, the role of ceramide in testicular cell apoptosis has made great progress, but the role of other sphingolipid metabolites during the apoptotic process of testicular cells remains to be further clarified.
7 Summary and prospect
Together, the contribution of sphingolipid metabolites to the homeostasis of steroid hormones and the maintenance of testicular functions is reviewed, but it is necessary to fully clarify their roles in male reproduction in the future. These bioactive sphingolipids, as key regulators, regulate steroid biosynthesis at different levels, including the expression and activity of steroid synthetases. In addition, sphingolipid metabolites participate in the regulation of male gametogenesis and testicular damage. However, the mechanism of these active molecules (such as ceramide and S1P) during male gonadal development and gametogenesis needs further study, and mass spectrometry, metabolomic analysis and proteomics are important tools to further study the physiological and pathological functions of sphingolipid metabolites. The present review about sphingolipid metabolites will help to formulate new strategies to improve gamete maturation and survival rate, thus optimizing various assisted reproductive technologies. In addition, the selective regulation of these active molecules in germ cells will help to protect the testis from chemotherapy damage, preserve the fertility of prognosis and improve the quality of life.
Author contributions
DW collected the literature and prepared the manuscript. YT collected the literature and made the illustrations. ZW conceptualized the article and revised the manuscript. All authors contributed to the article and approved the submitted version.
Funding
This work is supported by the Key Projects of Scientific and Technological Innovation in Fujian Province (2022G023), Fujian Provincial Natural Science Foundation (2020J01176 and 2022J01172), and the Innovation and Entrepreneurship Project of Fujian Normal University (I202102008).
Conflict of interest
The authors declare that the research was conducted in the absence of any commercial or financial relationships that could be construed as a potential conflict of interest.
Publisher’s note
All claims expressed in this article are solely those of the authors and do not necessarily represent those of their affiliated organizations, or those of the publisher, the editors and the reviewers. Any product that may be evaluated in this article, or claim that may be made by its manufacturer, is not guaranteed or endorsed by the publisher.
Abbreviation
SPH, sphingosine; S1P, sphingomyelin-1-phosphate; PKC, protein kinase C; PKA, protein kinase A; cAMP, cyclic adenosine monophosphate; PLD, phospholipase D; SK, sphingosine kinase; StAR, steroidogenic acute regulatory protein; CYP, cytochrome P450; HSD, hydroxysteroid dehydrogenase; GPCR, G protein-coupled receptor; PBR, peripheral benzodiazepine receptor; HSL, hormone-sensitive lipase; SR-BI, class B type I scavenger receptor; SF-1, steroid forming factor-1; FSH, follicle stimulating hormone; LH, luteinizing hormone; ACTH, adreno corticotropic hormone; SK, sphingosine kinase; TNF-α, tumor necrosis factor-αγ FasL, Fas ligand; INF-γ, interferon-γγ IL-1β, interleukin-1βγ hCG, human gonadotropin; C/EBPβ, CCAAT/enhancer-binding protein-β; PI-3K, phosphatidylinositol 3-kinase; ERK, extracellular regulated kinase; VLC PUFAs, very long chain polyunsaturated fatty acids.
References
1. Yang T, Zhao J, Liu F, Li Y. Lipid metabolism and endometrial receptivity. Hum Reprod Update (2022) 28:858–89. doi: 10.1093/humupd/dmac026
2. Hernandez-Coronado CG, Guzman A, Castillo-Juarez H, Zamora-Gutierrez D, Rosales-Torres AM. Sphingosine-1-phosphate (S1P) in ovarian physiology and disease. Ann Endocrinol (Paris) (2019) 80:263–72. doi: 10.1016/j.ando.2019.06.003
3. Hua G, Kolesnick R. Using ASMase knockout mice to model human diseases. Handb Exp Pharmacol (2013) 216:29–54. doi: 10.1007/978-3-7091-1511-4_2
4. Aerts JM, Boot RG, van Eijk M, Groener J, Bijl N, Lombardo E, et al. Glycosphingolipids and insulin resistance. Adv Exp Med Biol (2011) 721:99–119. doi: 10.1007/978-1-4614-0650-1_7
5. Lucki NC, Sewer MB. Multiple roles for sphingolipids in steroid hormone biosynthesis. Subcell Biochem (2008) 49:387–412. doi: 10.1007/978-1-4020-8831-5_15
6. Bhat OM, Yuan X, Li G, Lee R, Li PL. Sphingolipids and redox signaling in renal regulation and chronic kidney diseases. Antioxid Redox Signal (2018) 28:1008–26. doi: 10.1089/ars.2017.7129
7. Boini KM, Xia M, Koka S, Gehr TW, Li PL. Sphingolipids in obesity and related complications. Front Biosci (Landmark Ed) (2017) 22:96–116. doi: 10.2741/4474
8. Boini KM, Zhang C, Xia M, Poklis JL, Li PL. Role of sphingolipid mediator ceramide in obesity and renal injury in mice fed a high-fat diet. J Pharmacol Exp Ther (2010) 334:839–46. doi: 10.1124/jpet.110.168815
9. Jin S, Zhou F, Katirai F, Li PL. Lipid raft redox signaling: molecular mechanisms in health and disease. Antioxid Redox Signal (2011) 15:1043–83. doi: 10.1089/ars.2010.3619
10. Li G, Kidd J, Gehr TWB, Li PL. Podocyte sphingolipid signaling in nephrotic syndrome. Cell Physiol Biochem (2021) 55:13–34. doi: 10.33594/000000356
11. Li PL, Gulbins E. Lipid rafts and redox signaling. Antioxid Redox Signal (2007) 9:1411–5. doi: 10.1089/ars.2007.1736
12. Li PL, Gulbins E. Bioactive lipids and redox signaling: Molecular mechanism and disease pathogenesis. Antioxid Redox Signal (2018) 28:911–5. doi: 10.1089/ars.2017.7467
13. Tilly JL, Kolesnick RN. Sphingolipids, apoptosis, cancer treatments and the ovary: Investigating a crime against female fertility. Biochim Biophys Acta (2002) 1585:135–8. doi: 10.1016/s1388-1981(02)00333-5
14. Tilly JL. Commuting the death sentence: how oocytes strive to survive. Nat Rev Mol Cell Biol (2001) 2:838–48. doi: 10.1038/35099086
15. Morita Y, Tilly JL. Sphingolipid regulation of female gonadal cell apoptosis. Ann N Y Acad Sci (2000) 905:209–20. doi: 10.1111/j.1749-6632.2000.tb06551.x
16. Ozbay T, Merrill AH Jr., Sewer MB. ACTH regulates steroidogenic gene expression and cortisol biosynthesis in the human adrenal cortex via sphingolipid metabolism. Endocr Res (2004) 30:787–94. doi: 10.1081/erc-200044040
17. Urs AN, Dammer E, Sewer MB. Sphingosine regulates the transcription of CYP17 by binding to steroidogenic factor-1. Endocrinology (2006) 147:5249–58. doi: 10.1210/en.2006-0355
18. Zhang C, Li PL. Membrane raft redox signalosomes in endothelial cells. Free Radic Res (2010) 44:831–42. doi: 10.3109/10715762.2010.485994
19. Walker WH, Cooke PS. Functions of steroid hormones in the male reproductive tract as revealed by mouse models. Int J Mol Sci (2023) 24:2748. doi: 10.3390/ijms24032748
20. Detlefsen AJ, Paulukinas RD, Penning TM. Germline mutations in steroid metabolizing enzymes: a focus on steroid transforming aldo-keto reductases. Int J Mol Sci (2023) 24:1873. doi: 10.3390/ijms24031873
21. Naamneh Elzenaty R, du Toit T, Fluck CE. Basics of androgen synthesis and action. Best Pract Res Clin Endocrinol Metab (2022) 36:101665. doi: 10.1016/j.beem.2022.101665
22. Shah W, Khan R, Shah B, Khan A, Dil S, Liu W, et al. The molecular mechanism of sex hormones on sertoli cell development and proliferation. Front Endocrinol (Lausanne) (2021) 12:648141. doi: 10.3389/fendo.2021.648141
23. Xu R, Wang F, Zhang Z, Zhang Y, Tang Y, Bi J, et al. Diabetes-induced autophagy dysregulation engenders testicular impairment via oxidative stress. Oxid Med Cell Longev (2023) 2023:4365895. doi: 10.1155/2023/4365895
24. Liu W, Gong T, Shi F, Xu H, Chen X. Taste receptors affect male reproduction by influencing steroid synthesis. Front Cell Dev Biol (2022) 10:956981. doi: 10.3389/fcell.2022.956981
25. Jiang J, Liu S, Qi L, Wei Q, Shi F. Activation of ovarian taste receptors inhibits progesterone production potentially via NO/cGMP and apoptotic signaling. Endocrinology (2021) 162:bqaa240. doi: 10.1210/endocr/bqaa240
26. Shi Z, Enayatullah H, Lv Z, Dai H, Wei Q, Shen L, et al. Freeze-dried royal jelly proteins enhanced the testicular development and spermatogenesis in pubescent male mice. Anim (Basel) (2019) 9:977. doi: 10.3390/ani9110977
27. Tilly JL, Kolesnick RN. Sphingolipid signaling in gonadal development and function. Chem Phys Lipids (1999) 102:149–55. doi: 10.1016/s0009-3084(99)00083-3
28. Kwak DH, Seo BB, Chang KT, Choo YK. Roles of gangliosides in mouse embryogenesis and embryonic stem cell differentiation. Exp Mol Med (2011) 43:379–88. doi: 10.3858/emm.2011.43.7.048
29. Sandhoff R. Very long chain sphingolipids: tissue expression, function and synthesis. FEBS Lett (2010) 584:1907–13. doi: 10.1016/j.febslet.2009.12.032
30. Spaulding SC, Bollag WB. The role of lipid second messengers in aldosterone synthesis and secretion. J Lipid Res (2022) 63:100191. doi: 10.1016/j.jlr.2022.100191
31. Goni FM, Sot J, Alonso A. Biophysical properties of sphingosine, ceramides and other simple sphingolipids. Biochem Soc Trans (2014) 42:1401–8. doi: 10.1042/BST20140159
32. Goni FM, Alonso A. Biophysics of sphingolipids i. membrane properties of sphingosine, ceramides and other simple sphingolipids. Biochim Biophys Acta (2006) 1758:1902–21. doi: 10.1016/j.bbamem.2006.09.011
33. Merrill AH Jr., Jones DD. An update of the enzymology and regulation of sphingomyelin metabolism. Biochim Biophys Acta (1990) 1044:1–12. doi: 10.1016/0005-2760(90)90211-f
34. Qi T, Li L, Weidong T. The role of sphingolipid metabolism in bone remodeling. Front Cell Dev Biol (2021) 9:752540. doi: 10.3389/fcell.2021.752540
35. Liu Z, Yang H, Zhi L, Xue H, Lu Z, Zhao Y, et al. Sphingosine 1-phosphate stimulates insulin secretion and improves cell survival by blocking voltage-dependent k(+) channels in beta cells. Front Pharmacol (2021) 12:683674. doi: 10.3389/fphar.2021.683674
36. Bode C, Berlin M, Rostel F, Teichmann B, Graler MH. Evaluating sphingosine and its analogues as potential alternatives for aggressive lymphoma treatment. Cell Physiol Biochem (2014) 34:1686–700. doi: 10.1159/000366370
37. Davis W Jr. The ATP-binding cassette transporter-2 (ABCA2) overexpression modulates sphingosine levels and transcription of the amyloid precursor protein (APP) gene. Curr Alzheimer Res (2015) 12:847–59. doi: 10.2174/156720501209151019105834
38. Huwiler A, Kolter T, Pfeilschifter J, Sandhoff K. Physiology and pathophysiology of sphingolipid metabolism and signaling. Biochim Biophys Acta (2000) 1485:63–99. doi: 10.1016/s0009-3084(99)00071-7
39. Slotte JP. Sphingomyelin-cholesterol interactions in biological and model membranes. Chem Phys Lipids (1999) 102:13–27. doi: 10.1016/s0009-3084(99)00071-7
40. Hung WC, Chang HC, Chuang LY. Activation of caspase-3-like proteases in apoptosis induced by sphingosine and other long-chain bases in Hep3B hepatoma cells. Biochem J (1999) 338(Pt 1):161–6. doi: 10.1042/bj3380161
41. Natarajan V, Jayaram HN, Scribner WM, Garcia JG. Activation of endothelial cell phospholipase d by sphingosine and sphingosine-1-phosphate. Am J Respir Cell Mol Biol (1994) 11:221–9. doi: 10.1165/ajrcmb.11.2.8049083
42. Miller WL. Mechanism of StAR's regulation of mitochondrial cholesterol import. Mol Cell Endocrinol (2007) 265-266:46–50. doi: 10.1016/j.mce.2006.12.002
43. Sewer MB, Dammer EB, Jagarlapudi S. Transcriptional regulation of adrenocortical steroidogenic gene expression. Drug Metab Rev (2007) 39:371–88. doi: 10.1080/03602530701498828
44. Hauet T, Liu J, Li H, Gazouli M, Culty M, Papadopoulos V. PBR, StAR, and PKA: partners in cholesterol transport in steroidogenic cells. Endocr Res (2002) 28:395–401. doi: 10.1081/erc-120016814
45. Kraemer FB, Shen WJ, Harada K, Patel S, Osuga J, Ishibashi S, et al. Hormone-sensitive lipase is required for high-density lipoprotein cholesteryl ester-supported adrenal steroidogenesis. Mol Endocrinol (2004) 18:549–57. doi: 10.1210/me.2003-0179
46. Liu J, Rone MB, Papadopoulos V. Protein-protein interactions mediate mitochondrial cholesterol transport and steroid biosynthesis. J Biol Chem (2006) 281:38879–93. doi: 10.1074/jbc.M608820200
47. Parker KL, Rice DA, Lala DS, Ikeda Y, Luo X, Wong M, et al. Steroidogenic factor 1: an essential mediator of endocrine development. Recent Prog Horm Res (2002) 57:19–36. doi: 10.1210/rp.57.1.19
48. Jameson JL. Molecular mechanisms of end-organ resistance. Growth Horm IGF Res (2004) 14 Suppl A:S45–50. doi: 10.1016/j.ghir.2004.03.012
49. Meroni SB, Pellizzari EH, Canepa DF, Cigorraga SB. Possible involvement of ceramide in the regulation of rat leydig cell function. J Steroid Biochem Mol Biol (2000) 75:307–13. doi: 10.1016/s0960-0760(00)00188-6
50. Morales V, Santana P, Diaz R, Tabraue C, Gallardo G, Lopez Blanco F, et al. Intratesticular delivery of tumor necrosis factor-alpha and ceramide directly abrogates steroidogenic acute regulatory protein expression and leydig cell steroidogenesis in adult rats. Endocrinology (2003) 144:4763–72. doi: 10.1210/en.2003-0569
51. Arai N, Masuzaki H, Tanaka T, Ishii T, Yasue S, Kobayashi N, et al. Ceramide and adenosine 5'-monophosphate-activated protein kinase are two novel regulators of 11beta-hydroxysteroid dehydrogenase type 1 expression and activity in cultured preadipocytes. Endocrinology (2007) 148:5268–77. doi: 10.1210/en.2007-0349
52. Kihara A, Mitsutake S, Mizutani Y, Igarashi Y. Metabolism and biological functions of two phosphorylated sphingolipids, sphingosine 1-phosphate and ceramide 1-phosphate. Prog Lipid Res (2007) 46:126–44. doi: 10.1016/j.plipres.2007.03.001
53. Mellon S, Gong W, Griffin LD. Niemann pick type c disease as a model for defects in neurosteroidogenesis. Endocr Res (2004) 30:727–35. doi: 10.1081/erc-200044016
54. Griffin LD, Gong W, Verot L, Mellon SH. Niemann-pick type c disease involves disrupted neurosteroidogenesis and responds to allopregnanolone. Nat Med (2004) 10:704–11. doi: 10.1038/nm1073
55. Mellon SH. Neurosteroid regulation of central nervous system development. Pharmacol Ther (2007) 116:107–24. doi: 10.1016/j.pharmthera.2007.04.011
56. Mruk DD, Cheng CY. Sertoli-sertoli and sertoli-germ cell interactions and their significance in germ cell movement in the seminiferous epithelium during spermatogenesis. Endocr Rev (2004) 25:747–806. doi: 10.1210/er.2003-0022
57. Akhtar MF, Ahmad E, Mustafa S, Chen Z, Shi Z, Shi F. Spermiogenesis, stages of seminiferous epithelium and variations in seminiferous tubules during active states of spermatogenesis in yangzhou goose ganders. Anim (Basel) (2020) 10:570. doi: 10.3390/ani10040570
58. Kim SM, Kwak DH, Kim SM, Jung JU, Lee DH, Lee S, et al. Differential expression of gangliosides in the ovary and uterus of streptozotocin-induced and db/db diabetic mice. Arch Pharm Res (2006) 29:666–76. doi: 10.1007/BF02968251
59. Trevino CL, Serrano CJ, Beltran C, Felix R, Darszon A. Identification of mouse trp homologs and lipid rafts from spermatogenic cells and sperm. FEBS Lett (2001) 509:119–25. doi: 10.1016/s0014-5793(01)03134-9
60. Jung KY, Kim BH, Hwang MR, Cho JR, Kim HM, Lee YC, et al. Differential distribution of ganglioside GM3 in seminiferous tubule and epididymis of adult rats. Arch Pharm Res (2001) 24:360–6. doi: 10.1007/BF02975107
61. Sandhoff R, Geyer R, Jennemann R, Paret C, Kiss E, Yamashita T, et al. Novel class of glycosphingolipids involved in male fertility. J Biol Chem (2005) 280:27310–8. doi: 10.1074/jbc.M502775200
62. Takamiya K, Yamamoto A, Furukawa K, Zhao J, Fukumoto S, Yamashiro S, et al. Complex gangliosides are essential in spermatogenesis of mice: Possible roles in the transport of testosterone. Proc Natl Acad Sci USA (1998) 95:12147–52. doi: 10.1073/pnas.95.21.12147
63. Rabionet M, van der Spoel AC, Chuang CC, von Tumpling-Radosta B, Litjens M, Bouwmeester D, et al. Male Germ cells require polyenoic sphingolipids with complex glycosylation for completion of meiosis: A link to ceramide synthase-3. J Biol Chem (2008) 283:13357–69. doi: 10.1074/jbc.M800870200
64. Furland NE, Zanetti SR, Oresti GM, Maldonado EN, Aveldano MI. Ceramides and sphingomyelins with high proportions of very long-chain polyunsaturated fatty acids in mammalian germ cells. J Biol Chem (2007) 282:18141–50. doi: 10.1074/jbc.M700708200
65. Furland NE, Oresti GM, Antollini SS, Venturino A, Maldonado EN, Aveldano MI. Very long-chain polyunsaturated fatty acids are the major acyl groups of sphingomyelins and ceramides in the head of mammalian spermatozoa. J Biol Chem (2007) 282:18151–61. doi: 10.1074/jbc.M700709200
66. Lee J, Richburg JH, Younkin SC, Boekelheide K. The fas system is a key regulator of germ cell apoptosis in the testis. Endocrinology (1997) 138:2081–8. doi: 10.1210/endo.138.5.5110
67. Lee DH, Koo DB, Ko K, Ko K, Kim SM, Jung JU, et al. Effects of daunorubicin on ganglioside expression and neuronal differentiation of mouse embryonic stem cells. Biochem Biophys Res Commun (2007) 362:313–8. doi: 10.1016/j.bbrc.2007.07.142
68. Sramek J, Nemcova-Furstova V, Kovar J. Molecular mechanisms of apoptosis induction and its regulation by fatty acids in pancreatic beta-cells. Int J Mol Sci (2021) 22:4285. doi: 10.3390/ijms22084285
69. Verheij M, Bose R, Lin XH, Yao B, Jarvis WD, Grant S, et al. Requirement for ceramide-initiated SAPK/JNK signalling in stress-induced apoptosis. Nature (1996) 380:75–9. doi: 10.1038/380075a0
70. Suomalainen L, Hakala JK, Pentikäinen V, Otala M, Erkkilä K, Pentikäinen MO, et al. Sphingosine-1-phosphate in inhibition of male germ cell apoptosis in the human testis. J Clin Endocrinol Metab (2003) 88:5572–9. doi: 10.1210/jc.2003-030776
71. Bharath LP, Ruan T, Li Y, Ravindran A, Wan X, Nhan JK, et al. Ceramide-initiated protein phosphatase 2A activation contributes to arterial dysfunction in vivo. Diabetes (2015) 64:3914–26. doi: 10.2337/db15-0244
72. Kolesnick R, Golde DW. The sphingomyelin pathway in tumor necrosis factor and interleukin-1 signaling. Cell (1994) 77(3):325–8. doi: 10.1016/0092-8674(94)90147-3
73. Nikolova-Karakashian MN, Rozenova KA. Ceramide in stress response. Adv Exp Med Bio (2010) 688:86–108. doi: 10.1007/978-1-4419-6741-1_6
74. Orsini M, Chateauvieux S, Rhim J, Gaigneaux A, Cheillan D, Christov C, et al. Sphingolipid-mediated inflammatory signaling leading to autophagy inhibition converts erythropoiesis to myelopoiesis in human hematopoietic stem/progenitor cells. Cell Death Differ (2019) 26(9):1796–812. doi: 10.1038/s41418-018-0245-x
Keywords: steroid hormone, gametogenesis, sphingosine-1-phosphate, sphingomyelin, testicular function
Citation: Wang D, Tang Y and Wang Z (2023) Role of sphingolipid metabolites in the homeostasis of steroid hormones and the maintenance of testicular functions. Front. Endocrinol. 14:1170023. doi: 10.3389/fendo.2023.1170023
Received: 20 February 2023; Accepted: 07 March 2023;
Published: 17 March 2023.
Edited by:
Guangbi Li, Virginia Commonwealth University, United StatesReviewed by:
Fangxiong Shi, Nanjing Agricultural University, ChinaGenbao Shao, Jiangsu University, China
Yanqing Wu, Wenzhou University, China
Copyright © 2023 Wang, Tang and Wang. This is an open-access article distributed under the terms of the Creative Commons Attribution License (CC BY). The use, distribution or reproduction in other forums is permitted, provided the original author(s) and the copyright owner(s) are credited and that the original publication in this journal is cited, in accordance with accepted academic practice. No use, distribution or reproduction is permitted which does not comply with these terms.
*Correspondence: Dr. Zhengchao Wang, emN3YW5nQGZqbnUuZWR1LmNu