Corrigendum: Vascular endothelial growth factor levels in diabetic peripheral neuropathy: a systematic review and meta-analysis
- 1Department of Endocrinology, Hospital of Chengdu University of Traditional Chinese Medicine, Chengdu, China
- 2Department of Respiratory, Hospital of Chengdu University of Traditional Chinese Medicine, Chengdu, China
Objective: Vascular endothelial growth factors (VEGFs, including VEGF-A, VEGF-B, VEGF-C, VEGF-D and PLGF) have important roles in the development and function of the peripheral nervous system. Studies have confirmed that VEGFs, especially VEGF-A (so called VEGF) may be associated with the diabetic peripheral neuropathy (DPN) process. However, different studies have shown inconsistent levels of VEGFs in DPN patients. Therefore, we conducted this meta-analysis to evaluate the relationship between cycling levels of VEGFs and DPN.
Methods: This study searched 7 databases, including PubMed, Embase, Cochrane Library, China National Knowledge Infrastructure (CNKI), VIP Database, WanFang Database, and Chinese Biomedical Literature (CBM), to find the target researches. The random effects model was used to calculate the overall effect.
Results: 14 studies with 1983 participants were included, among which 13 studies were about VEGF and 1 was VEGF-B, so only the effects of VEGF were pooled. The result showed that there were obviously increased VEGF levels in DPN patients compared with diabetic patients without DPN (SMD:2.12[1.34, 2.90], p<0.00001) and healthy people (SMD:3.50[2.24, 4.75], p<0.00001). In addition, increased circulating VEGF levels were not associated with an increased risk of DPN (OR:1.02[0.99, 1.05], p<0.00001).
Conclusion: Compared with healthy people and diabetic patients without DPN, VEGF content in the peripheral blood of DPN patients is increased, but current evidence does not support the correlation between VEGF levels and the risk of DPN. This suggests that VEGF may play a role in the pathogenesis and repairment of DPN.
1 Introduction
Diabetic peripheral neuropathy (DPN) is a common complication of diabetes, more than 50% of diabetic patients suffer from it, and it is a leading cause of diabetic foot ulcers and non-traumatic lower limb amputations (1). The WHO estimates that 366 million people will be affected by this disease by 2030 (2). The main clinical manifestations of DPN are lower extremity pain, numbness, paresthesia, and weakness, patients are prone to falls, ulcers, and amputations in severe cases (3). The occurrence of DPN increases the suffering of patients, reduces the quality of life, all of which aggravate the burden on families and society.
Despite the great harm and large number of patients, the pathogenesis of DPN has not been clearly explained. The occurrence of DPN is related to many pathogenic factors, including microvascular dysfunction, accumulation of advanced glycation end products(AGEs), inflammation, oxidative stress and autoimmunity (4). DPN has long been considered as a microvascular complication of diabetes, just like nephropathy and retinopathy. Clinical studies and animal experiments have confirmed that microvascular dysfunction is closely related to DPN, and the degree of microvascular injury is related to the severity of clinical symptoms (5, 6). Cumulative evidence indicates that the DPN process is accompanied by significant nerve ischemia and hypoxia (4, 7). Nerve biopsies revealed capillary basement membrane thickening, loss of capillary pericyte coverage, and endothelial hyperplasia in endoneurial microvessels (8). The series of pathological changes led to a decrease in nerve stem perfusion, resulting in the simultaneous reduction of nerve conduction velocity and oxygen tension. Angiogenesis is an important compensatory step in the body’s response to hypoxia, it can induce neovascularization to reconstruct the vascular network and restore tissue blood supply (9). Intuitively, the researchers hope to improve nerve blood supply through angiogenesis caused by tissue hypoxia, but the role of angiogenesis in DPN has been somewhat contradictory, which is partly reflected in the unclear changes of vascular endothelial growth factor (VEGF), the most representative angiogenesis factor, in DPN (10).
An important family of growth factors is vascular endothelial growth factor (VEGF) family, whose expression is mainly regulated by hypoxia-inducible factor (HIF) during hypoxia and are important participants in the angiogenesis process (11). VEGF family proteins include VEGF-A, VEGF-B, VEGF-C, VEGF-D, and placental growth factor (PLGF). Given the dominant role that VEGF-A plays in regulating angiogenesis and disease, it is also directly called VEGF (this abbreviation is used below) and will largely be the focus of this review (12). The physiological effects of VEGF are far more than angiogenesis. In recent years, VEGF has been found to have neuroprotective and nutritive effects, and is an important signaling molecule for nerve repair and regeneration (13, 14).
As an important angiogenesis and neuroprotective factor, the changes in the VEGF family in diabetic complications have attracted wide attention. However, the changes of VEGF, the most famous molecule of the VEGF family, in the peripheral circulation of DPN patients are still contradictory, and different studies have shown different trends. It has been reported that VEGF levels in the peripheral blood of DPN patients are significantly higher than those of diabetic patients without DPN and healthy people, but some studies have reached different conclusions (15, 16). Due to the current inconclusive results, we conducted this meta-analysis to comprehensively evaluate the relationship between VEGF levels in blood and DPN. For the comprehensiveness of the study, other factors in the VEGF family, such as VEGF-B, VEGF-C, VEGF-D and PLGF were also retrieved for analysis.
2 Methods
This study was performed according to the Preferred Reporting Items for Systematic Reviews and Meta-Analyses (PRISMA) standard and the Meta-analysis of Observational Studies in Epidemiology (MOOSE) guidelines for systematic reviews of observational studies. This study was registered at the International Prospective Register of Systematic Reviews (PROSPERO), Number CRD42023389678.
2.1 Literature search
Two investigators (Rui Ding and Shicong Zhu) independently conducted a systematic search on the databases of PubMed, Embase, Cochrane Library, China National Knowledge Infrastructure (CNKI), VIP Database, WanFang Database, and Chinese Biomedical Literature (CBM) through December 30, 2022, with language restrictions to Chinese and English. MeSH and free-text words are used for searching, with appropriate modifications for different databases. The search terms were used as follows: (Diabetic Neuropathy OR Diabetic Autonomic Neuropathy OR Diabetic Neuralgias OR Painful Diabetic Neuropathy OR Diabetic Asymmetric Polyneuropathy OR Diabetic Mononeuropathy OR Diabetic Polyneuropathy OR Diabetic Peripheral Neuropathy) AND (Vascular Endothelial Growth Factors OR Vascular Endothelial Growth Factor A OR VEGF-A OR Vasculotropin OR VEGF OR Vascular Permeability Factor OR Glioma-Derived Vascular Endothelial Cell Growth Factor OR GD-VEGF OR Vascular Endothelial Growth Factor B OR VEGF-B OR Vascular Endothelial Growth Factor C OR VEGF-C OR Vascular Endothelial Growth Factor D OR VEGF-D OR placental growth factor OR PLGF). More detailed search terms and procedure are in Support Material.
2.2 Inclusion criteria
(1) cohort, cross-sectional, or case-control studies; (2) the controls were diabetic patients without DPN or healthy controls; (3) providing mean values of VEGFs levels, or odds ratio (OR) with 95% confidence interval (95% CI) or sufficient data to calculate these levels; (4) studies in Chinese or English were considered for inclusion; If two studies used the same study population during the same period, we included only the study with larger sample size and longer follow-up.
2.3 Exclusion criteria
(1) studies without available data for meta-analysis were excluded; (2) reported data were on only one group of subjects; (4) obviously irrelevant studies, articles without full text, conference abstracts, comments, reviews, case-only studies, and studies based on animal models or cell lines were excluded.
2.4 Data extraction and quality assessment
The risk of bias was evaluated using the Newcastle Ottawa scale (NOS) for case–control studies and an adapted form of the Newcastle Ottawa scale for cross-sectional studies (17, 18). The following data were extracted from each study by 2 independent reviewers (Shicong Zhu, Xiaoyan Zhao): first author’s name, year of publication, location of the study (country), study design, study index (VEGFs family member), sample size, participants’ characteristics, mean values of VEGFs levels, OR with 95%CI on the association of VEGFs with DPN, and adjusted confounding factors.
2.5 Statistical analyses
All meta-analyses were performed using RevMan software (version 5.3). Data will be analyzed using a random or fixed effects model (depending on which is appropriate). Continuous variables, presented as mean ± standard deviation, with SMD and 95%CI for effective measures. The pooled OR with 95% CI was calculated for the assessment of the association between VEGFs levels and DPN risk. The I2 index and Cochran’s Q statistics were used for heterogeneity assessment. If pQ-text< 0.1 and I2>50%, it indicates that there is statistical heterogeneity. A sensitivity analysis to test the robustness of pooled estimates by excluding every single study was also performed. In addition, we planned a priori subgroup analysis based on: study location, study design, sample size, sample type, disease type and ages. Publication bias was assessed using funnel plot, Egger’s test and Begg’s test performed by Stata 15.0 software. If publication bias exists, we would use the trim and fill method to add several possible missing studies and recalculate the pooled estimates. Statistical significance was considered as p < 0.05.
3 Result
3.1 Search result
Of 1488 records (346 from CBM, 95 from CNKI, 52 from Cochrane Library, 422 from Embase, 347 from Pubmed, 44 from VIP and 182 from Wanfang) were initially identified from the online databases. After removing 367 duplicates, studies that did not meet the inclusion criteria were excluded by reading the title and abstract. After an initial review, 35 studies will be read in full text to determine whether they meet the inclusion criteria. Finally, 14 studies (19–32) were included in our meta-analysis, including 7 studies (20, 23, 24, 26, 28, 31, 32) in Chinese and 7 studies (18, 20, 21, 24, 26, 28, 29) in English. The search process is shown in Figure 1.
Those 14 studies involved a total of 1983 participants, including 807 DPN patients, 808 diabetic patients without DPN and 368 healthy people. Among the 14 studies, 13 studies (19–23, 25–32) reported VEGF levels in peripheral blood and 1 study (24) examined VEGF-B levels in serum. 9 (20, 22, 23, 26, 27, 29–32) studies reported circulating VEGF levels in 3 groups (healthy group, diabetic patients without DPN, DPN patients), 5 (19, 21, 24, 25, 28) studies reported VEGF levels in DPN and diabetic patients without DPN. Studies were published in 2009~2020 and conducted in 7 different countries (China, Japan, Indonesia, Iraq, Greece, Poland and Egypt). 1 study (27) specifically targeted type 1 diabetes patients, 11 studies (19, 20, 23–26, 28–32)only included type 2 diabetes patients, and the remaining 2 studies (21, 22) included both type 1 and type 2 diabetes patients. 2 studies (21, 25) graded DPN patients for severity (0~3 level) and 1 study (22) divided DPN patients into painful and non-painful DPN. 3 studies (19, 25, 31) reported the OR value between blood VEGF levels and DPN risk. 12 studies (19–25, 27, 28, 30–32) were cross-sectional studies and 2 (26, 29) were case-control studies. As to the quality, the scores of included studies ranged from 5 to 7, and the average score for the quality assessment was 6.29 (Table 1).
3.2 VEGF levels in diabetic patients with DPN and without DPN
13 studies compared VEGF levels in the blood of DPN patients and diabetic patients without DPN (N-DPN), but 1 study did not specify the data type (e.g., median, quartile or maximum, minimum), so we pooled the analysis of VEGF levels in the 12 studies. 1 study divided DPN patients into three grades and separately reported the VEGF content in each grade, so we combined the data of the three levels. The random effects model was adopted to pool the data due to apparent heterogeneity. As shown in Figure 2, VEGF levels were significantly higher in DPN patients than in diabetic patients without DPN (SMD:2.12[1.34, 2.90], p<0.00001, Heterogeneity: Tau² = 1.77; Chi² =389.47, P < 0.00001; I² = 97%, Figure 2). Sensitivity analyses were performed by leaving each study separately. In this meta-analysis, sensitivity analysis did not change the high heterogeneity and the pooled estimates, which proves the stability of the present results. Draw the funnel plot of these 12 studies, and the Egger’s and Begg’s tests were conducted by Stata software to assess publication bias. The results indicated that the publication bias does exist (pEgger=0.004), the funnel plot is shown in Figure 3. After the trim and fill analysis imputed 4 potentially missed studies (Figure 4), the pooled effect (2.515[95%CI:1.089, 5.807]) is still consistent with the outcomes before the trim and fill analysis. This suggests that the result remains stable despite publication bias.
3.3 VEGF levels in healthy people and DPN patients
There were a total of 368 healthy people from 9 studies assessing the VEGF levels compared with DPN patients. Meta-analysis of the random effect model showed that there were obviously higher VEGF levels in DPN patients compared with healthy subjects (SMD:3.50[2.24, 4.75], p<0.00001, Heterogeneity: Tau² = 3.51; Chi² =304.85, P < 0.00001; I² = 97%, Figure 5). Sensitivity analysis found no significant change in heterogeneity after excluding each study, implying that our results were stable and reliable. Considering that the source of high heterogeneity has not yet been resolved, we will then conduct a subgroup analysis to discuss the possible sources of heterogeneity according to the study location, study design, sample type, diabetes type, age, and sample size. The funnel plot of 9 studies is obviously asymmetric, and Egger’s test shows that pEgger=0.000, which indicates the existence of publication bias (Figure 6). Then, the trim and fill method was used to fill the possible missing studies. After adding 4 studies (Figure 7), the meta-analysis pooled results still strongly suggest that the VEGF levels are elevated in DPN patients compared with healthy individuals (5.083[95%CI:1.369, 18.878]).
3.4 Increased levels of VEGF and DPN risk
3 studies evaluated the association between elevated VEGF levels and DPN risk, while another study evaluated the association between VEGF-B and DPN. The OR values with 95%CI of 3 studies targeting VEGF were pooled for analysis. Random effects models were used to summarize the effect sizes due to high heterogeneity, and found no association between increased VEGF and DPN risk (OR:1.02[0.99, 1.05], p=0.13, Heterogeneity: Tau² = 0.00; Chi² =33.54, P < 0.00001; I² = 94%, Figure 8A). The sensitive analysis demonstrated that the article of ‘Barus 2018’ explained 37% of heterogeneity (OR:1.06[0.97, 1.17], p=0.20, Heterogeneity: Tau² = 0.00; Chi² =2.31, P=0.20; I² = 57%, Figure 8B). It can be seen that although all 3 studies independent reported that increased VEGF levels were associated with DPN risk, this association was no longer significant after our meta-analysis pooled the effect size. The article ‘hou 2018’ reported the relationship between VEGF-B level and the risk of DPN by logistic regression, which showed that VEGF-B was an independent risk factor for DPN in T2DM patients (OR: 1.441[1.154,1.797]).
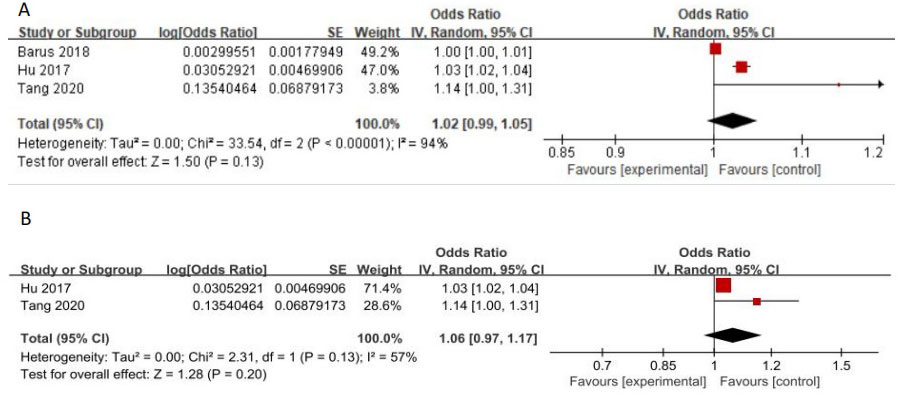
Figure 8 (A) Increased VEGF levels and the risk of DPN (B) Forest plot after the sensitivity analysis.
3.5 Subgroup analysis
Since sensitivity analysis has not fully addressed the issue of high heterogeneity, we performed subgroup analysis according to different study characteristics. Subgroup analysis will be performed based on study type, study location, sample type, sample size, disease type, and age of DPN patients. The specific subgroup analysis results are shown in Table 2.
In those studies comparing VEGF levels in diabetic patients with DPN and without DPN, the subgroup analysis yielded the following results. In these studies, whether conducted in China or other countries, VEGF level was increased in DPN patients compared with non-DPN patients with diabetes, also this increase was independent of study location, patient age and blood sample type. However, when the subjects were only type 1 diabetes or included type 1 diabetes, the increase in VEGF became less significant (SMD: 0.19[-0.15, 0.53]). At the same time, subgroup analysis also found that different study designs and sample sizes may also affect the level of VEGF. When the number of participants ≥200, VEGF in the peripheral blood of DPN patients did not significantly increase (SMD: 0.92[-0.49, 2.33]), and the same result was also found in the pooled effect of two case-control studies (SMD: 8.00[-2.80, 18.79]). Unfortunately, the above subgroup analysis fails to account for the high heterogeneity of the study. Some possible causes of heterogeneity such as disease course, diagnostic criteria, gender, and detection methods could not be further discussed in subgroup analysis due to the incomplete and inconsistent research reports.
Our subgroup analysis of 9 studies with healthy people as controls found that different types of diabetes also affected the results of the pooled analysis, when the subjects included or only included type 1 diabetes patients, the increase of VEGF level became less significant (SMD: 0.77[-0.19, 1.72]). Simultaneously, subgroup analysis showed that there was no significant difference in subgroup according to location (P = 0.39), sample type (p=0.05), age(p=0.26) and study design (P = 0.66).
4 Discussion
To our knowledge, this is the first meta-analysis to evaluate the association between VEGF levels and DPN. VEGF is the most important and concerned component in the VEGF family, was also the absolute focus of the studies included in this meta-analysis. Therefore, our discussion will also focus on VEGF. Both quantitative and qualitative data of our study indicate that VEGF is overexpressed in DPN, whether compared with normal diabetic patients without DPN or healthy people. This finding is not surprising, as ischemia and hypoxia have been observed in DPN, which are the most effective stimuli to induce VEGF secretion. VEGF is known for its powerful angiogenesis effects, forming a network of blood vessels to improve tissue blood supply in response to hypoxia. Despite significant increases in VEGF levels in DPN, our study did not find an association between VEGF levels and DPN risk. Thus, it can be seen that with VEGF as the response mode, angiogenesis is involved in the pathogenesis of DPN, but VEGF as the biomarker of DPN should be treated with caution. Evidence for this conclusion was provided by a prospective study that included 315 patients with diabetes at baseline. After 5 years of follow-up, 163 patients developed DPN and 152 did not, when baseline data were compared based on DPN status, no significant differences were observed between the DPN group and non-DPN group in VEGF (33). This highlights that the effect of VEGF on DPN development is uncertain, and more prospective studies should be conducted to clarify this issue. Therefore, VEGF levels cannot be used as biomarkers for predicting DPN at the moment.
Although the subgroup analysis failed to explain the source of the heterogeneity, we were surprised to find that the increase in VEGF was no longer significant when the study population included type 1 diabetes regardless of the subjects with which DPN patients were compared (healthy people or diabetic people without DPN). This reminds us to pay attention to the role of diabetic types. Microvascular reactivity does not appear to be the same between type 1 diabetes and type 2 diabetes. VEGF has a strong ability to promote vascular permeability (34), but this permeability shows different responses in different types of diabetes. When sodium fluorescein was injected into both type 1 and type 2 diabetics, the fluorescence intensity in type 1 diabetic patients became significant 30 minutes after injection of dye, while in type 2 diabetic patients, the difference was significant only 1 minute after injection (35).The different vascular permeability and reactivity in type 1 and type 2 diabetes patients mean that different types of diabetes may have various vascular lesion characteristics and discrepant responses to VEGF. Since different types of diabetes have inconsistent responses to the same molecule, these may become potential confounding factors, leading to the emergence of heterogeneity.
Though our subgroup analysis does not find any difference this time, the content of VEGF in serum and plasma may indeed differ. Hanefeld et al. (36) found that when serum was used to detect the circulating VEGF, the serum VEGF content of T2DM patients was significantly higher than the healthy controls. However, when plasma was used, the increase became no longer significant, and the serum VEGF level was 7.3 times higher than the plasma VEGF level. VEGF is released by activated platelets, platelet activation during blood collection may also be an artificial source of VEGF, resulting in large variations in VEGF concentrations, but this is usually ignored (37). A cross-sectional study of 21 healthy subjects and 64 patients with type 1 diabetes determined VEGF levels in plasma collected in both citrate and PECT (a medium that inactivates platelets) found that higher levels of VEGF in citrate plasma samples of diabetic patients do not represent the real situation in vivo but mainly originate from higher artificial ex vivo release from platelets (38). A recent meta-analysis showed that out of the different plasma types, using EDTA as an anticoagulant obtained the highest concentration of VEGF, followed by heparin and citrate, while CTAD reported the lowest content of VEGF (39). In addition, kits from different manufacturers bring different methods for the measurement of VEGF, and other factors, including batch, sensitivity and detection range, all contribute to the study of heterogeneity. Unfortunately, due to the imperfect report, we cannot make a corresponding subgroup analysis. The above analysis reveals that the standardization problem exists in relevant measurements. Only by establishing standardization of measurement methods can future research be less heterogeneous and more comparable across studies (40). Last but not least, the pooled effect of two case-control studies suggests that VEGF levels are still higher in DPN patients than in healthy individuals, but are no longer significant when compared to diabetic patients without DPN. These 2 studies, one from China and the other from Iraq, were of low quality and did not have clear diagnostic criteria for DPN. Considering that different races, living environments, case selection bias and laboratory conditions may affect the experimental results, we should be cautious about the results of this subgroup analysis and include more high-quality studies in the future to further discuss.
DPN is the most common diabetic microvascular complication. Long-term chronic hyperglycemia leads to neuronal damage and reduced nerve blood flow, in the meantime, ischemia and hypoxia caused by neurovascular defects can also lead to structural and functional changes in nerve tissue (41, 42). VEGF enhances nerve blood flow and oxygen supply by inducing angiogenesis, in response to nerve ischemia and hypoxia caused by DPN (43). Therefore, the enhanced secretion of VEGF in DPN patients can be considered as a protective mechanism against further damage (44). VEGF levels were significantly associated with blood glucose control, as represented by HbA1c. The researchers found that plasma VEGF levels were positively correlated with FBG and HbA1c, the multiple regression analysis showed that the HbA1c level were independent predictors of VEGF levels in T2DM (45). A few studies found that functional alteration of peripheral nerves causes up-regulation of VEGF in diabetic rats, and VEGF decreased significantly under treatment (46, 47). It seems likely that VEGF levels increased as a neuroprotection strategy in response to various insults in DPN, and the decrease of VEGF may be due to the repair of the nerve after proper treatment. Indeed, some studies have found that by promoting the secretion of VEGF, the blood flow and nerve conduction velocity of the DPN sciatic nerve can be restored (48, 49). A randomized controlled trial conducted by Ropper et al. (50)found that intradermal injection of VEGF plasmid for DPN significantly reduced lower extremity symptoms in patients. Given that VEGF supplementation may have the risk of exacerbating diabetic retinopathy and inducing tumors, the study confirmed that there was no progression of diabetic retinopathy and no neoplastic disease after a year of follow-up, which preliminarily suggesting that local VEGF supplementation does not alter systemic angiogenesis status. However, several adverse events, including limb pain, edema, and muscle spasms, were reported in this study. Therefore, although VEGF supplements have been proven effective, there is still a long way to go in clinical practice due to their excessive negative effects, and more safe methods need to be found.
In diabetes mellitus, excessive or defective angiogenesis occurs. Excessive angiogenesis is often seen in diabetic retinopathy, while insufficient angiogenesis often impedes healing of diabetic foot ulcers. Abnormal angiogenesis in DPN has been specifically observed in some studies. Compared with healthy animals, functional blood vessels of the sciatic nerve in DPN animals have smaller density and shorter diameter, the content of VEGF, Ang-1 and other pro-angiogenesis regulatory factors in the sciatic nerve is lower (5, 51). Thus, some angiogenic factors (VEGF, Ang-1) have been proposed to treat peripheral neuropathy (52, 53). However, it must be pointed out that some studies have found that VEGF levels in the sciatic nerve increased after STZ induction (54). These findings are not contradictory, as we must consider the possible effects of the disease course. Chavez et al. (55) confirmed that the expression of HIF-1α and its downstream factors (VEGF, EPO) in diabetic sciatic nerve showed a trend of first increasing and then decreasing in chronological order. Current human studies have found a negative association between endoneurial capillary density and disturbed nerve dysfunction, suggesting a compensatory increase in vascular density early in neuropathy (56). The 2 studies (21, 25) we included divided DPN into 4 stages, among which stage 0 was no evidence of DPN, stage 1~3 was divided according to severity, stage 1 was no symptoms, but abnormal signs of DPN, and stage 3 was disabling neuropathy. In both studies, stage 3 has the longest course of the disease, but stage 2 has the largest level of VEGF, which at least partially supports the above view, that is, the level of VEGF increases first and then decreases with the course or severity of the disease. This conclusion also implies that the proposed ‘angiogenic’ strategies to treat microangiopathy in nerves might not be suitable at every stage of DPN. As for why the compensatory effect of angiogenesis is weakened with the progression of the disease, studies have shown that high glucose can damage the HIF-1α signaling pathway under hypoxia conditions and reduce the levels of VEGF, but this damage may take time to accumulate. Hyperglycemia disrupts HIF-1α stability and this disruption may be mediated by PHD or VHL, PHD inhibition or VHL inactivation can largely rescue HIF-1α stability, but the underlying mechanism is not fully understood (57). What’s more, hyperglycemia also induces methylglyoxal (MGO) accumulation, which induces the degradation of HIF-1α and decreases the transcriptional activity of HIF-1. MGO modifies HIF-1α to increase its association with heat shock protein 40/70 (Hsp40/70), leading to CHIP (Carboxy terminus of Hsp70-Interacting Protein) recruitment, thereby promoting HIF-1α ubiquitination and degradation (58). MGO also modification of P300, which inhibits its recruitment of HIF-1α, and produces a decrease in VEGF expression (59). Although high glucose inhibits the HIF-1α response and the expression of VEGF, at the same time, the continuous existence of glucose metabolism disorder in diabetes means that the hypoxia factor cannot change and still stimulates the expression of HIF-1α and VEGF. Therefore, the blood VEGF content in DPN patients is still higher than that in healthy people and diabetic patients without DPN until decompensation occurs.
The limitations of our study should be acknowledged. First, no eligible prospective cohort studies were found in our search, thus no causal relationship could be explored. Second, only one study focused exclusively on type 1 diabetes, so a more detailed analysis of diabetes types was not possible. Third, the OR values involved in this study were all unadjusted, so more adjusted OR is needed to refine the conclusion. Fourth, most of the included studies were rated as medium in quality. In view of the fact that high-quality studies can provide more effective information for analysis, more excellent trials should be added in the future. Finally, high heterogeneity and publication bias reminds us to treat the results of this study with caution.
5 Conclusion
In conclusion, the circulating VEGF levels in DPN patients were significantly higher than that in diabetic patients without DPN and healthy controls. This meta-analysis did not find a connection between the level of VEGF in peripheral blood and the risk of DPN. Due to the limitations of this study, more high-quality studies, especially cohort studies, are needed in the future to further clarify the relationship between DPN and VEGF. In addition, although our studies have proved that VEGF plays an important role in the pathogenesis and treatment of DPN, it still needs careful consideration as a biomarker and therapeutic approach.
Author contributions
RD collected, analyzed, designed this study, and wrote the main manuscript as the first author. SZ and XZ extracted data and performed the statistical analysis. RD and SZ retrieved the literature and conducted a research quality assessment. RY supervised the work. All authors contributed to the article and approved the final manuscript.
Funding
This work was supported by the Sichuan Science and Technology Foundation (2021YFS0037).
Acknowledgments
We would like to thank Kunyuan Wang for the revision of our manuscript writing.
Conflict of interest
The authors declare that the research was conducted in the absence of any commercial or financial relationships that could be construed as a potential conflict of interest.
Publisher’s note
All claims expressed in this article are solely those of the authors and do not necessarily represent those of their affiliated organizations, or those of the publisher, the editors and the reviewers. Any product that may be evaluated in this article, or claim that may be made by its manufacturer, is not guaranteed or endorsed by the publisher.
Supplementary material
The Supplementary Material for this article can be found online at: https://www.frontiersin.org/articles/10.3389/fendo.2023.1169405/full#supplementary-material
References
1. Selvarajah D, Kar D, Khunti K, Davies MJ, Scott AR, Walker J, et al. Diabetic peripheral neuropathy: advances in diagnosis and strategies for screening and early intervention. Lancet Diabetes Endocrinol (2019) 7(12):938–48. doi: 10.1016/S2213-8587(19)30081-6
2. Zochodne DW. The challenges of diabetic polyneuropathy: a brief update. Curr Opin Neurol (2019) 32(5):666–75. doi: 10.1097/WCO.0000000000000723
3. Feldman EL, Callaghan BC, Pop-Busui R, Zochodne DW, Wright DE, Bennett DL, et al. Diabetic neuropathy. Nat Rev Dis Primers (2019) 5(1):41. doi: 10.1038/s41572-019-0092-1
4. Kobayashi M, Zochodne DW. Diabetic neuropathy and the sensory neuron: new aspects of pathogenesis and their treatment implications. J Diabetes Investig (2018) 9(6):1239–54. doi: 10.1111/jdi.12833
5. Wang L, Chopp M, Szalad A, Lu X, Lu M, Zhang T, et al. Angiopoietin-1/Tie2 signaling pathway contributes to the therapeutic effect of thymosin β4 on diabetic peripheral neuropathy. Neurosci Res (2019) 147:1–8. doi: 10.1016/j.neures.2018.10.005
6. Borire AA, Issar T, Kwai NC, Visser LH, Simon NG, Poynten AM, et al. Sonographic assessment of nerve blood flow in diabetic neuropathy. Diabetes Med (2020) 37(2):343–9. doi: 10.1111/dme.14085
7. Horton WB, Barrett EJ. Microvascular dysfunction in diabetes mellitus and cardiometabolic disease. Endocr Rev (2021) 42(1):29–55. doi: 10.1210/endrev/bnaa025
8. Méndez-Morales ST, Pérez-De Marcos JC, Rodríguez-Cortés O, Flores-Mejía R, Martínez-Venegas M, Sánchez-Vera Y, et al. Diabetic neuropathy: molecular approach a treatment opportunity. Vascul Pharmacol (2022) 143:106954. doi: 10.1016/j.vph.2022.106954
9. Asadi-Yousefabad SL, Nammian P, Tabei SMB, Daneshi S, Fallahi J, Razban V, et al. Angiogenesis in diabetic mouse model with critical limb ischemia; cell and gene therapy. Microvasc Res (2022) 141:104339. doi: 10.1016/j.mvr.2022.104339
10. Fadini GP, Albiero M, Bonora BM, Avogaro A. Angiogenic abnormalities in diabetes mellitus: mechanistic and clinical aspects. J Clin Endocrinol Metab (2019) 104(11):5431–44. doi: 10.1210/jc.2019-00980
11. Zhang Y, Zhang YY, Pan ZW, Li QQ, Sun LH, Li X, et al. GDF11 promotes wound healing in diabetic mice via stimulating HIF-1α-VEGF/SDF-1α-mediated endothelial progenitor cell mobilization and neovascularization. Acta Pharmacol Sin (2023) 44(5):999–1013. doi: 10.1038/s41401-022-01013-2
12. Apte RS, Chen DS, Ferrara N. VEGF in signaling and disease: beyond discovery and development. Cell (2019) 176(6):1248–64. doi: 10.1016/j.cell.2019.01.021
13. Hecking I, Stegemann LN, Theis V, Vorgerd M, Matschke V, Stahlke S, et al. Neuroprotective effects of VEGF in the enteric nervous system. Int J Mol Sci (2022) 23(12):6756. doi: 10.3390/ijms23126756
14. Calvo PM, Hernández RG, de la Cruz RR, Pastor AM. VEGF is an essential retrograde trophic factor for motoneurons. Proc Natl Acad Sci USA (2022) 119(26):e2202912119. doi: 10.1073/pnas.2202912119
15. Kim HW, Ko GJ, Kang YS, Lee MH, Song HK, Kim HK, et al. Role of the VEGF 936 C/T polymorphism in diabetic microvascular complications in type 2 diabetic patients. Nephrol (Carlton) (2009) 14(7):681–8. doi: 10.1111/j.1440-1797.2009.01085.x
16. Eleftheriadou I, Dimitrakopoulou N, Kafasi N, Tentolouris A, Dimitrakopoulou A, Anastasiou IA, et al. Endothelial progenitor cells and peripheral neuropathy in subjects with type 2 diabetes mellitus. J Diabetes Complications (2020) 34(4):107517. doi: 10.1016/j.jdiacomp.2019.107517
17. Wells GA, Shea B, O’Connell D, et al. The Newcastle-Ottawa scale (NOS) for assessing the quality of nonrandomised studies in meta-analyses. Available at: http://www.ohri.ca/programs/clinicalepidemiology/oxford.asp.
18. Herzog R, Álvarez-Pasquin MJ, Díaz C, et al. Are healthcare workers' intentions to vaccinate related to their knowledge, beliefs and attitudes? a systematic review. BMC Public Health (2013) 13:154. doi: 10.1186/1471-2458-13-154
19. Barus J, Setyopranoto I, Sadewa AH, et al. Vascular endothelial growth factor 936 C/T gene polymorphism in Indonesian subjects with diabetic polyneuropathy. Open Access Maced J Med Sci (2018) 6(10):1784–9. doi: 10.3889/oamjms.2018.399
20. Cui L, Wu Y. Relationship between serum vascular endothelial growth factor and diabetic peripheral neuropathy. J Shantou Univ Med Coll (2012) 25(2):108–9.
21. Deguchi T, Hashiguchi T, Horinouchi S, Uto T, Oku H, Kimura K, et al. Serum VEGF increases in diabetic polyneuropathy, particularly in the neurologically active symptomatic stage. Diabetes Med (2009) 26(3):247–52. doi: 10.1111/j.1464-5491.2009.02680.x
22. Doupis J, Lyons TE, Wu S, Gnardellis C, Dinh T, Veves A. Microvascular reactivity and inflammatory cytokines in painful and painless peripheral diabetic neuropathy. J Clin Endocrinol Metab (2009) 94(6):2157–63. doi: 10.1210/jc.2008-2385
23. Ha H, Huang X, Zhao J, Pu H, Chen L. The relationship between IL-17, VEGF and the number of diabetic microangiopathy. Sci Tech Inf Gansu (2017) 46(10):94–96,62. doi: 10.3969/j.issn.1672-6375.2017.10.027
24. Hou L, Gu W, Zhou L, Zhang X. Study on the association between serum neopterin, VEGF-b and peripheral neuropa-thy in newly diagnosed patients with type 2 diabetes mellitus. Trans Med J (2022) 11(2):92–6. doi: 10.3969/j.issn.2095-3097.2022.02.007
25. Hu G, Zhai F, Mo F, He L, Shen W, Wang H. Effectiveness and feasibility of nailfold microcirculation test to screen for diabetic peripheral neuropathy. Diabetes Res Clin Pract (2017) 131:42–8. doi: 10.1016/j.diabres.2017.06.017
26. Huang D, Deng Y. Correlation between VEGF and DPN in type 2 diabetes mellitus. J Pract Traditional Chin Med (2015) 2015(7):677–677,678. doi: 10.3969/j.issn.1004-2814.2015.07.064
27. Kuryliszyn-Moskal A, Zarzycki W, Dubicki A, Moskal D, Kosztyła-Hojna B, Hryniewicz A. Clinical usefulness of videocapillaroscopy and selected endothelial cell activation markers in people with type 1 diabetes mellitus complicated by microangiopathy. Adv Med Sci (2017) 62(2):368–73. doi: 10.1016/j.advms.2016.11.007
28. Liu Y, Zhu Y, Shi L, Yu Y. The study of the expressions of vascular endothelial growth factor and solubility vascular endothelial growth factor receptors-2 in diabetic perineuropathy. Chin J Difficult Complicated cases (2009) 8(4):228–9. doi: 10.3969/j.issn.1671-6450.2009.04.019
29. Mohamed AH, Zaidan HK. Evaluation of vascular endothelial growth factor level of diabetic peripheral neuropathy patients in babylon province. J Pharm Sci Res (2019) 11(1):247–50.
30. Motawi TK, Rizk SM, Ibrahim IA, El-Emady YF. Alterations in circulating angiogenic and anti-angiogenic factors in type 2 diabetic patients with neuropathy. Cell Biochem Funct (2014) 32(2):155–63. doi: 10.1002/cbf.2987
31. Tang F, Zhong S, Li Q, Zhao B, Zhu W, Lu B, et al. Investigation of hypoxia-inducible factor-1α and vascular endothelial growth factor in type 2 diabetes with peripheral neuropathy. Beijing Med J (2020) 42(4):287–90. doi: 10.15932/j.0253-9713.2020.04.005
32. Wang X, Zhang Z, Shi Q. Correlation between homocysteine, vascular endothelial growth factor, serum cystatin c and microangiopathy in type 2 diabetes mellitus. World Chin Med (2016) 2016(B06):2231–2. doi: 10.3969/j.issn.1673-7202.2016.06.657
33. Zheng H, Sun W, Zhang Q, Zhang Y, Ji L, Liu X, et al. Proinflammatory cytokines predict the incidence of diabetic peripheral neuropathy over 5 years in Chinese type 2 diabetes patients: a prospective cohort study. EClinicalMedicine (2020) 31:100649. doi: 10.1016/j.eclinm.2020.100649
34. Phoenix KN, Yue Z, Yue L, Cronin CG, Liang BT, Hoeppner LH, et al. PLCβ2 promotes VEGF-induced vascular permeability. Arterioscler Thromb Vasc Biol (2022) 42(10):1229–41. doi: 10.1161/ATVBAHA.122.317645
35. Brausewetter F, Jehle PM, Jung MF, Boehm BO, Brueckel J, Hombach V, et al. Microvascular permeability is increased in both types of diabetes and correlates differentially with serum levels of insulin-like growth factor I (IGF-I) and vascular endothelial growth factor (VEGF). Horm Metab Res (2001) 33(12):713–20. doi: 10.1055/s-2001-19133
36. Hanefeld M, Appelt D, Engelmann K, Sandner D, Bornstein SR, Ganz X, et al. Serum and plasma levels of vascular endothelial growth factors in relation to quality of glucose control, biomarkers of inflammation, and diabetic nephropathy. Horm Metab Res (2016) 48(8):529–34. doi: 10.1055/s-0042-106295
37. Lopez Yomayuza CC, Preissner KT, Lorenz B, Stieger K. Optimizing measurement of vascular endothelial growth factor in small blood samples of premature infants. Sci Rep (2019) 9(1):6744. doi: 10.1038/s41598-019-43108-7
38. Schlingemann RO, Van Noorden CJ, Diekman MJ, Tiller A, Meijers JC, Koolwijk P, et al. VEGF levels in plasma in relation to platelet activation, glycemic control, and microvascular complications in type 1 diabetes. Diabetes Care (2013) 36(6):1629–34. doi: 10.2337/dc12-1951
39. Sjöbom U, Nilsson AK, Gyllensten H, Hellström A, Löfqvist C. A systematic review and meta-analysis of preanalytical factors and methodological differences influencing the measurement of circulating vascular endothelial growth factor. PloS One (2022) 17(7):e0270232. doi: 10.1371/journal.pone.0270232
40. Ng TKS, Ho CSH, Tam WWS, Kua EH, Ho RC. Decreased serum brain-derived neurotrophic factor (BDNF) levels in patients with alzheimer's disease (AD): a systematic review and meta-analysis. Int J Mol Sci (2019) 20(2):257. doi: 10.3390/ijms20020257
41. Crasto W, Patel V, Davies MJ, Khunti K. Prevention of microvascular complications of diabetes. Endocrinol Metab Clin North Am (2021) 50(3):431–55. doi: 10.1016/j.ecl.2021.05.005
42. Singh R, Farooq SA, Mannan A, Singh TG, Najda A, Grażyna Z, et al. Animal models of diabetic microvascular complications: relevance to clinical features. BioMed Pharmacother (2022) 145:112305. doi: 10.1016/j.biopha.2021.112305
43. Takeshita Y, Sato R, Kanda T. Blood-nerve barrier (BNB) pathology in diabetic peripheral neuropathy and In vitro human BNB model. Int J Mol Sci (2020) 22(1):62. doi: 10.3390/ijms22010062
44. Mahdy RA, Nada WM, Hadhoud KM, El-Tarhony SA. The role of vascular endothelial growth factor in the progression of diabetic vascular complications. Eye (Lond) (2010) 24(10):1576–84. doi: 10.1038/eye.2010.86
45. Zhang Q, Fang W, Ma L, Wang ZD, Yang YM, Lu YQ. VEGF levels in plasma in relation to metabolic control, inflammation, and microvascular complications in type-2 diabetes: a cohort study. Med (Baltimore) (2018) 97(15):e0415. doi: 10.1097/MD.0000000000010415
46. Samii A, Unger J, Lange W. Vascular endothelial growth factor expression in peripheral nerves and dorsal root ganglia in diabetic neuropathy in rats. Neurosci Lett (1999) 262(3):159–62. doi: 10.1016/s0304-3940(99)00064-6
47. Lei T, Jing D, Xie K, Jiang M, Li F, Cai J, et al. Therapeutic effects of 15 Hz pulsed electromagnetic field on diabetic peripheral neuropathy in streptozotocin-treated rats. PloS One (2013) 8(4):e61414. doi: 10.1371/journal.pone.0061414
48. Hata M, Omi M, Kobayashi Y, Nakamura N, Miyabe M, Ito M, et al. Sustainable effects of human dental pulp stem cell transplantation on diabetic polyneuropathy in streptozotocine-induced type 1 diabetes model mice. Cells (2021) 10(9):2473. doi: 10.3390/cells10092473
49. Saboory E, Gholizadeh-Ghaleh Aziz S, Samadi M, Biabanghard A, Chodari L. Exercise and insulin-like growth factor 1 supplementation improve angiogenesis and angiogenic cytokines in a rat model of diabetes-induced neuropathy. Exp Physiol (2020) 105(5):783–92. doi: 10.1113/EP088069
50. Ropper AH, Gorson KC, Gooch CL, Weinberg DH, Pieczek A, Ware JH, et al. Vascular endothelial growth factor gene transfer for diabetic polyneuropathy: a randomized, double-blinded trial. Ann Neurol (2009) 65(4):386–93. doi: 10.1002/ana.21675
51. Han JW, Choi D, Lee MY, Huh YH, Yoon YS.. Bone marrow-derived mesenchymal stem cells improve diabetic neuropathy by direct modulation of both angiogenesis and myelination in peripheral nerves. Cell Transplant (2016) 25(2):313–26. doi: 10.3727/096368915x688209
52. Schratzberger P, Walter DH, Rittig K, Bahlmann FH, Pola R, Curry C, et al. Reversal of experimental diabetic neuropathy by VEGF gene transfer. J Clin Invest (2001) 107(9):1083–92. doi: 10.1172/JCI12188
53. Kosacka J, Nowicki M, Klöting N, Kern M, Stumvoll M, Bechmann I, et al. COMP-angiopoietin-1 recovers molecular biomarkers of neuropathy and improves vascularisation in sciatic nerve of ob/ob mice. PloS One (2012) 7(3):e32881. doi: 10.1371/journal.pone.0032881
54. Atif F, Prunty MC, Turan N, Stein DG, Yousuf S. Progesterone modulates diabetes/hyperglycemia-induced changes in the central nervous system and sciatic nerve. Neuroscience (2017) 350:1–12. doi: 10.1016/j.neuroscience.2017.03.007
55. Chavez JC, Almhanna K, Berti-Mattera LN. Transient expression of hypoxia-inducible factor-1 alpha and target genes in peripheral nerves from diabetic rats. Neurosci Lett (2005) 374(3):179–82. doi: 10.1016/j.neulet.2004.10.050
56. Thrainsdottir S, Malik RA, Dahlin LB, Wiksell P, Eriksson KF, Rosén I, et al. Endoneurial capillary abnormalities presage deterioration of glucose tolerance and accompany peripheral neuropathy in man. Diabetes (2003) 52(10):2615–22. doi: 10.2337/diabetes.52.10.2615
57. Catrina SB, Zheng X. Hypoxia and hypoxia-inducible factors in diabetes and its complications. Diabetologia (2021) 64(4):709–16. doi: 10.1007/s00125-021-05380-z
58. Bento CF, Fernandes R, Ramalho J, Marques C, Shang F, Taylor A, et al. The chaperone-dependent ubiquitin ligase CHIP targets HIF-1α for degradation in the presence of methylglyoxal. PloS One (2010) 5(11):e15062. doi: 10.1371/journal.pone.0015062
Keywords: diabetic peripheral neuropathy, vascular endothelial growth factor, angiogenesis, microcirculation, meta analysis
Citation: Ding R, Zhu S, Zhao X and Yue R (2023) Vascular endothelial growth factor levels in diabetic peripheral neuropathy: a systematic review and meta-analysis. Front. Endocrinol. 14:1169405. doi: 10.3389/fendo.2023.1169405
Received: 19 February 2023; Accepted: 28 April 2023;
Published: 12 May 2023.
Edited by:
Marta Letizia Hribal, Magna Græcia University, ItalyReviewed by:
Liangjun Yang, Guangzhou University of Chinese Medicine, ChinaGeorgios Dimtsas, National and Kapodistrian University of Athens, Greece
Copyright © 2023 Ding, Zhu, Zhao and Yue. This is an open-access article distributed under the terms of the Creative Commons Attribution License (CC BY). The use, distribution or reproduction in other forums is permitted, provided the original author(s) and the copyright owner(s) are credited and that the original publication in this journal is cited, in accordance with accepted academic practice. No use, distribution or reproduction is permitted which does not comply with these terms.
*Correspondence: Rensong Yue, c29uZ3Jlbnl1ZUBjZHV0Y20uZWR1LmNu