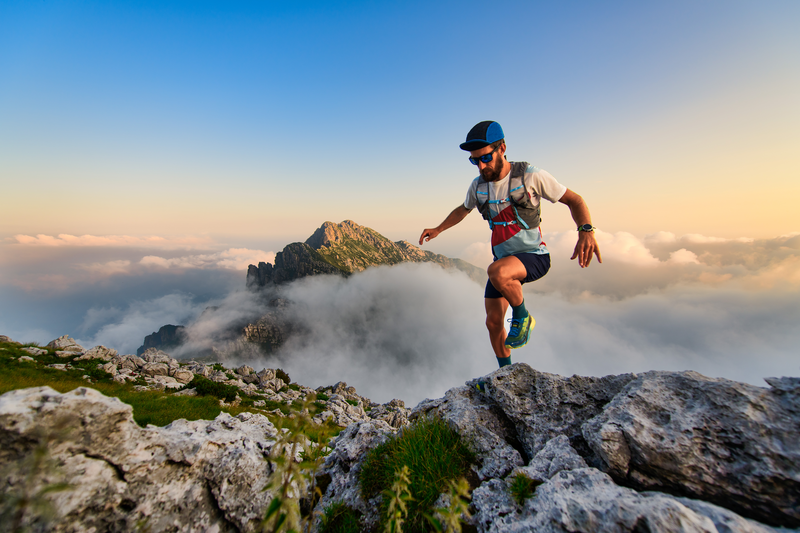
94% of researchers rate our articles as excellent or good
Learn more about the work of our research integrity team to safeguard the quality of each article we publish.
Find out more
ORIGINAL RESEARCH article
Front. Endocrinol. , 11 July 2023
Sec. Pediatric Endocrinology
Volume 14 - 2023 | https://doi.org/10.3389/fendo.2023.1164747
This article is part of the Research Topic Exploring Obesity Risk, Prevention, and Research Innovation in the First 2000 Days of Life, volume II View all 5 articles
Objective: This prospective cohort study was aimed at investigating the associations between cord blood metabolic factors and early-childhood growth, further elucidating the relationships between cord blood metabolites and overweight and obesity in early life.
Methods: A total of 2,267 pairs of mothers and offspring were recruited in our study. Cord blood plasma was assayed for triglycerides (TGs), total cholesterol (TC), low-density lipoprotein-cholesterol (LDL-C), high-density lipoprotein-cholesterol (HDL-C), C-peptide, insulin, and glycosylated hemoglobin type A1C (HbA1c) levels. Data of anthropometric measurements were collected from offspring at birth, 6 months, 12 months, and 18 months. Multiple linear regression models were used to evaluate the correlations between cord blood metabolic factors and weight Z-scores, body mass index (BMI) Z-scores, and weight gains at the early stage of life. Forward stepwise logistic regression analyses were applied to explore the associations between cord blood metabolic factors and early-childhood overweight and obesity. Receiver operating characteristic (ROC) curve analyses were applied to determine the optimal cutoff points for cord blood metabolic factors in predicting early-childhood overweight and obesity.
Results: After adjustments for covariates, cord blood TG concentrations and TG/TC ratios were negatively associated with weight Z-scores from birth to 18 months. Cord blood C-peptide and HbA1c levels were inversely associated with weight Z-scores at 6 months and 18 months. Cord blood TG concentrations and TG/TC ratios were negatively correlated with BMI Z-scores up to 18 months. Cord blood C-peptide levels and HbA1c levels were inversely correlated with BMI Z-scores at 18 months. Cord blood TG, TG/TC ratios, C-peptide, and HbA1c had negative correlations with weight gains from birth to 6 months, but the correlations attenuated as time went on. Increase in cord blood TG and HbA1c levels and TG/TC ratios were significantly associated with decreased risks of overweight and obesity at 6 months, 12 months, and 18 months.
Conclusions: Cord blood metabolic factors were significantly associated with early-childhood growth patterns.
Evidence has suggested that maternal nutrition and the intrauterine metabolic environment play an important role in placental-fetal growth and development. According to the Developmental Origins of Health and Disease (DOHaD) theory, individuals exposed to an undernourished environment in utero were more prone to developing type 2 diabetes, obesity, or cardiovascular diseases in adulthood life as a result of epigenetic modifications (1, 2). Recent studies have implied that maternal glucose and lipid metabolic dysfunctions, including gestational diabetes mellitus (GDM) and hyperlipidemia, were closely related with childhood overweight and adiposity (3–5).
Umbilical cord blood metabolite levels were essential indicators reflecting intrauterine metabolic status of fetuses. It has been discovered that cord blood cardiometabolic factors were associated with fetal growth and birth weight status. Fetal weight was positively associated with insulin levels especially during the period of late gestation. Fetal weight was negatively correlated with triglyceride (TG) levels and positively correlated with high-density lipoprotein-cholesterol (HDL-C) levels during the third trimester of pregnancy (6). Results from prospective cohort studies showed that high cord serum TG levels and insulin levels were significantly associated with small for gestational age (SGA) and large for gestational age (LGA) neonates, respectively (7, 8). SGA newborns were more likely to have lower levels of total cholesterol (TC), low-density lipoprotein-cholesterol (LDL-C) and HDL-C in cord blood. Cord blood insulin levels were linearly associated with increased birth weights (8). Whereas cord blood TG levels were negatively associated with neonatal birth weights (9, 10). In addition, cord serum TG concentrations were found to have a strong negative association with insulin-like growth factor I (IGF-I), which assisted in predicting intrauterine growth restriction newborns (11). Umbilical cord leptin, known as a hormone regulating lipid metabolism through a negative feedback mechanism, was found to be positively correlated with neonatal birth weight and body mass index (BMI) (12).
Epidemiological studies further indicated that cord blood metabolic factors were associated with early-childhood growth and fat mass. A German research group followed up offspring’s postnatal growth trajectories (13, 14). Interestingly, they discovered that cord plasma insulin concentrations were positively correlated with neonatal birth weight and fat mass but inversely correlated with body weight gain up to 2 years old (13). Additionally, cord blood insulin was negatively associated with skinfold thicknesses and body fat percentage at 3 years old. The inverse relationship could also be observed between cord blood insulin and weight gain up to 5 years old in girls, but the effect size was small without clinical significance (14). In another prospective cohort study, cord blood insulin concentrations were found to be positively associated with skinfold thickness. Ghrelin concentrations were negatively associated with BMI and skinfold thickness at age 1 year (15). A meta-analysis retrieved 22 studies and concluded that umbilical cord blood leptin was positively associated with adiposity at birth but inversely related to adiposity up to 3 years of age. However, the association was not sustained up to 5 years old (16).
This multicenter prospective cohort study was aimed at investigating the associations between cord blood lipid and glucose metabolism and early-childhood growth, further elucidating the relationships between cord blood metabolic factors and overweight and obesity in early life.
Between 1 January 2010 and 30 June 2011, pregnant women who attended regular prenatal examinations and intended to give birth in Yongkang, Huzhou, Cixi, Ninghai, Shaoxing, Yiwu, Dongyang, and Wenling Maternal and Child Health Care Hospitals were invited to participate in this study. Before recruitment, every participant signed the written informed consent. At the same time, our study obtained approval from the Clinical Research Ethics Committee of the hospitals. Inclusion criteria of pregnant women were 28–37 weeks of gestation, singleton pregnancy, naturally conceived, having clear gestational age, and integrated medical records. Exclusion criteria of mothers were multiple pregnancies, having type 1 or type 2 diabetes mellitus, chromosomal abnormalities, inherited metabolic diseases or thyroid diseases, being conceived with assisted reproductive technology, and experiencing severe infections during early pregnancy. Information about maternal age, educational background, family income, parity, prepregnancy BMI, cigarette exposure, pregnancy complications [e.g., GDM, hypertensive disorders in pregnancy, intrahepatic cholestasis of pregnancy (ICP), etc.] was sourced from questionnaire and medical records. They were followed up until delivery, and information regarding delivery mode, gestational age, infant gender, birth weight, birth length, Apgar scores, and perinatal complications was recorded. Inclusion criteria of neonates were singleton and postpartum 5-min Apgar scores ≥7. Exclusion criteria for neonates were congenital abnormalities, inherited metabolic diseases, and postpartum 5-min Apgar scores <7. Finally, a total of 2,267 pairs of mothers and offspring were recruited in our study. Among the subjects, 1,250 participants were boys and 1,017 participants were girls.
Venous umbilical cord blood samples were collected at birth in anticoagulant ethylenediaminetetraacetic acid (EDTA) tubes. Serum samples were obtained through centrifugation (3,500 rpm at 4°C for 10 min) and stored at -80°C until analysis. Every cord blood plasma was assayed for TG, TC, LDL-C, HDL-C, C-peptide, insulin, and glycosylated hemoglobin type A1C (HbA1c) levels on an automatic biochemical analyzer (Roche Cobase C8000, Roche Diagnostics, Germany). All of the inter- and intra-assay coefficients of variation were <2.0%.
Offspring in our study were followed up and received regular physical examinations at birth, 6 months, 12 months, and 18 months. All of the data of anthropometric measurements were collected from standardized measuring devices. Weights were measured to the nearest 0.01 kg with an electronic scale (376, Hangzhou Seca Medical Measuring Apparatus Company, China). Lengths were measured to the nearest 0.1 cm with a length-measuring instrument (416, Hangzhou Seca Medical Measuring Apparatus Company, China). Both weight and length measurements were performed in light clothing. Z-scores of weight-for-age and BMI-for-age were calculated on the basis of WHO Child Growth Standards (17). Z-scores were calculated through the formula: Z-score = (x-μ)/σ.
BMI was calculated via the formula: BMI = weight/(height2). Maternal prepregnancy BMIs were categorized into underweight (<18.5 kg/m2), normal weight (18.5–24.9 kg/m2), overweight (25.0–29.9 kg/m2), and obesity (≥30.0 kg/m2) groups according to the WHO BMI classifications. Neonates were classified into appropriate/small/large for gestational age (AGA/SGA/LGA) based on Chinese Neonatal Birth Weight Curve for Different Gestational Age (18). Neonates were defined as AGA if their birth weights met or exceeded the 10th percentile and met or fell below the 90th percentile for gestational age. SGA/LGA were respectively defined as birth weights that fell below the 10th percentile/exceeded the 90th percentile for gestational age. Offspring overweight was defined as a BMI ≥85 percentile and <95 percentile among the same age and same gender children. Obesity was defined as a BMI ≥95 percentile among the same age and same gender children. Their reference values were based on the WHO Child Growth Standards (17).
In the present study, categorical variables were presented as N (%). Normally distributed and skewed distributed continuous variables were presented as mean (standard deviation, SD) and median (interquartile range, IQR), respectively. Multiple linear regression models were used to examine the correlations between cord serum lipid profile, glucose metabolism, and growth at early stage of life. Forward stepwise logistic regression analyses were applied to explore the associations between cord blood metabolic factors and offspring overweight and obesity. ROC curve analyses were applied to determine the optimal cutoff points for cord blood metabolic factors in predicting early-childhood overweight and obesity. Each optimal cutoff point was obtained through searching for the maximum value of sensitivity+specificity–1 (Youden index). All of the data analyses were performed through SPSS 25.0 version for Windows (SPSS Inc., Chicago, USA). P values <0.05 were regarded as statistically significant.
Table 1 presented the basic characteristics of our study population. Among the expectant mothers recruited in the study, the mean (SD) age at delivery was 26.56 (3.80) years old. Most (72.0%) of them had a normal prepregnancy BMI, and only 4.9% of them were stratified as overweight or obese before pregnancy. The prevalence of GDM, hypertensive disorders in pregnancy, and ICP was respectively 10.2%, 3.0%, and 0.5%. Most (87.2%) of them were nulliparous. Nearly half (41.5%) of them were exposed to cigarette during pregnancy. Among the newborns, approximately 55% were boys. The mean (SD) gestational age at birth was 39.06 (1.17) weeks. About 2.0% of the neonates were preterm birth. Their mean (SD) birth weight was 3,367.8 (398.4) g. In addition, 78.8% of them were AGA, 5.8% of them were SGA, and 15.4% of them were LGA.
Consistent with previous studies, we found that cord blood TG concentrations were strongly negatively associated with birth weights even when adjusted for maternal age, prepregnancy BMI, maternal education level, family income, parity, pregnancy complications, and child sex [β = -0.10; 95% confidence interval (CI) = -0.13, -0.07; P < 0.001]. Cord blood insulin concentrations had a positive association with birth weights when adjusted for confounding variables (β = 0.011; 95% CI = 0.008, 0.013; P < 0.001).
Table 2 displayed the associations between cord blood metabolic factor levels and early-childhood weight Z-scores in our participants. After adjustments for maternal age, prepregnancy BMI, maternal education level, family income, parity, GDM, hypertensive disorders in pregnancy, ICP, child sex and birth weight, cord blood TG concentrations were found to be negatively associated with weight Z-scores at 6 months (β = -0.22; 95% CI = -0.37, -0.07; P = 0.004), 12 months (β = -0.19; 95% CI = -0.34, -0.05; P = 0.009), and 18 months (β = -0.19; 95% CI = -0.32, -0.06; P = 0.005). Cord blood TG/TC ratios were significantly negatively associated with weight Z-scores at 6 months (β = -0.62; 95% CI = -1.05, -0.19; P = 0.005), 12 months (β = -0.76; 95% CI = -1.17, -0.35; P < 0.001), and 18 months (β = -0.55; 95% CI = -0.93, -0.17; P = 0.004). Cord plasma C-peptide levels were inversely associated with weight Z-scores at 6 months (β = -0.26; 95% CI = -0.46, -0.07; P = 0.008) and 18 months (β = -0.26; 95% CI = -0.43, -0.08; P = 0.004). Cord plasma HbA1c levels were inversely associated with weight Z-scores at 6 months (β = -0.10; 95% CI = -0.17, -0.03; P = 0.005) and 18 months (β = -0.07; 95% CI = -0.13, -0.01; P = 0.022). Additionally, insulin concentrations had a negative association with 18-month weight Z-scores, but the relationship was very small without clinical significance.
Table 3 showed the associations between cord blood metabolic factors levels and early-childhood BMI Z-scores in the population. After adjustments for the above covariates, cord blood TG concentrations were negatively correlated with BMI Z-scores at 6 months (β = -0.28; 95% CI = -0.45, -0.11; P = 0.001), 12 months (β = -0.28; 95% CI = -0.42, -0.13; P < 0.001), and 18 months (β = -0.23; 95% CI = -0.37, -0.08; P = 0.002). Cord blood TG/TC ratios were significantly negatively associated with BMI Z-scores at 6 months (β = -0.79; 95% CI = -1.27, -0.30; P = 0.001), 12 months (β = -1.03; 95% CI = -1.44, -0.62; P < 0.001), and 18 months (β = -0.57; 95% CI = -0.98, -0.16; P = 0.006). Cord plasma C-peptide levels (β = -0.19; 95% CI = -0.38, -0.01; P = 0.043) and HbA1c levels (β = -0.09; 95% CI = -0.15, -0.02; P = 0.009) were inversely correlated with BMI Z-scores at 18 months. In addition, cord plasma TC (β = -0.08; 95% CI = -0.16, 0.00; P = 0.047) and LDL-C (β = -0.14; 95% CI = -0.27, 0.00; P = 0.045) concentrations were inversely correlated with BMI Z-scores at 6 months, but the correlations attenuated at 12 months and 18 months.
Table 3 Associations between cord blood metabolic factors and early-childhood body mass index Z-scores.
Table 4 exhibited the associations between cord blood metabolic factor levels and early-childhood weight gains in our participants. After adjustments for the covariates, cord serum metabolic factors were negatively correlated with weight gains from birth to 6 months, but the correlations attenuated as time went on. Cord serum TG (β = -0.20; 95% CI = -0.33, -0.07; P = 0.004), TG/TC ratios (β = -0.56; 95% CI = -0.94, -0.17; P = 0.005), C-peptide (β = -0.24; 95% CI = -0.41, -0.06; P = 0.008), and HbA1c (β = -0.09; 95% CI = -0.15, -0.03; P = 0.005) were negatively correlated with weight gains from birth to 6 months. Cord serum C-peptide had a weak inverse correlation with weight gains from 12 months to 18 months (β = -0.14; 95% CI = -0.28, 0.00; P = 0.044). However, cord serum TC concentrations (β = 0.07; 95% CI = 0.02, 0.12; P = 0.008) and LDL-C concentrations (β = 0.14; 95% CI = 0.05, 0.23; P = 0.002) were found to be positively associated with weight gains from 6 months to 12 months.
Mean (SD) weight of our study population was 8.35 (0.93) kg at 6 months, 10.08 (1.07) kg at 12 months, and 11.34 (1.15) kg at 18 months. Mean (SD) BMI of our participants was 17.97 (1.50) kg/m2 at 6 months, 17.43 (1.28) kg/m2 at 12 months, and 16.67 (1.19) kg/m2 at 18 months.
Table 5 demonstrated the associations between cord blood metabolic factor levels and early-childhood overweight and obesity. After adjustments for maternal age, prepregnancy BMI, maternal education level, family income, parity, GDM, hypertensive disorders in pregnancy, ICP, child sex and birth weight, every mmol/L increase in cord blood TG concentration was significantly associated with decreased risks of overweight and obesity at 6 months [adjusted odds ratio (AOR) = 0.49; 95% CI = 0.29, 0.85; P = 0.011], 12 months (AOR = 0.58; 95% CI = 0.35, 0.96; P = 0.034), and 18 months (AOR = 0.46; 95% CI = 0.26, 0.83; P = 0.010). Increases in cord plasma TG/TC ratios were remarkably associated with decreased risks of overweight and obesity at 6 months (AOR = 0.10; 95% CI = 0.03, 0.35; P < 0.001), 12 months (AOR = 0.08; 95% CI = 0.02, 0.29; P < 0.001), and 18 months (AOR = 0.21; 95% CI = 0.06, 0.72; P = 0.013). Furthermore, every unit elevation in HbA1c significantly reduced the risks for overweight and obesity at 6 months (AOR = 0.81; 95% CI = 0.67, 0.98; P = 0.033), 12 months (AOR = 0.81; 95% CI = 0.67, 0.99; P = 0.037), and 18 months (AOR = 0.74; 95% CI = 0.60, 0.91; P = 0.005). However, there were no remarkable associations between other cord blood metabolic factors and early-childhood overweight and obesity outcomes.
Table 5 Associations between cord blood metabolic factors and early-childhood overweight and obesity.
The optimal cutoff point proposed by ROC curve analysis for cord blood TG in predicting 18-month overweight and obesity was <0.29 mmol/L (sensitivity = 56.1%, specificity = 50.9%, AUC = 0.54). The optimal cutoff point for cord blood TG/TC in predicting 18-month overweight and obesity was <0.20 (sensitivity = 39.0%, specificity = 69.3%, AUC = 0.54). The optimal cutoff point for cord blood HbA1c in predicting 18-month overweight and obesity was <3.25% (sensitivity = 20.4%, specificity = 86.9%, AUC = 0.53).
The present prospective cohort study comprehensively displayed cord blood lipid and glucose metabolism in Chinese neonates and explored the associations between cord blood metabolic factors and growth indicators and overweight and obesity at the early stage of life. Previous research mostly investigated the associations between cord serum lipid profile, glucose metabolism and fetal growths and birth weights (6–10, 12). However, few studies followed up postnatal growth indicators and explored the associations between cord blood metabolic factors and early-childhood overweight and adiposity. Therefore, our study provided more epidemiological data of cord blood metabolic factors, improved insights into the associations between intrauterine metabolic environment and early-childhood growth, and indicated the underlying cord blood biomarkers for preventing childhood overweight and adiposity.
One outstanding finding from this study was that cord blood TG levels and TG/TC ratios were negatively associated with early-childhood growth. It has been reported that umbilical cord blood TG levels were negatively associated with fetal weights and birth weights (6–9). Our results further discovered inverse correlations between cord serum TG concentrations, TG/TC ratios and weight Z-scores and BMI Z-scores up to 18 months. These outcomes were in accordance with research work from Ye et al. (19) and Cao et al. (20). It has been shown that hypertriglyceridemia was closely related to islet β-cell dysfunctions, including interfered β-cell maturation, suppressed β-cell proliferation, inhibited insulin secretion, and induced insulin resistance. These disturbances hindered the activation of amino acid transport system, inhibited protein and fat synthesis, promoted lipolysis, and finally affected fetal growth and development, resulting in intrauterine growth restriction (21–24). At the same time, fetuses redistributed their nutrients for crucial organs (e.g., brain) to adapt to the adverse intrauterine environment, which further led to decreased fetal growth rate and birth weight (25). On the basis of the DOHaD theory, we speculated that fetal programming continued to play an important role in the growth patterns during the period of early childhood. Population-based cohort studies confirmed that fetal growth at different gestational periods was significantly associated with risks of impaired childhood growth, stunting, and obesity (26, 27). Increased fetal growth at each period was negatively associated with stunting and underweight at the age of 3 years (26). Conversely, intrauterine growth restriction infants were more prone to developing stunting when they were followed up to 18 months (27). These research findings supported metabolic disturbances and fetal growth restriction led to a long-term adverse effect on childhood growth. Thus, our findings provided convincing evidence for previous studies and figured out subsequent linear correlations sustained to early life.
Another interesting discovery from our research was that cord blood C-peptide and HbA1c levels had inverse correlations with early-childhood growth. This was a novel contribution rarely referred in previous studies. Once, a prior study discovered that cord serum C-peptide concentrations were positively associated with the incidence of neonatal macrosomia (28). An offspring follow-up research found that cord blood insulin concentrations were negatively associated with weight gain, skinfold thickness, and body fat percentage at early childhood (13, 14). Nevertheless, in another prospective cohort study, cord blood insulin concentrations were positively associated with skinfold thickness at age 1 year (15). Our study found weak associations between cord blood insulin concentrations and weight Z-scores, BMI Z-scores, and weight gains in infancy without clinical significance. These conflicting consequences suggested there still existed controversies, which deserved further explorations. It has been reported that umbilical blood insulin, C-peptide, and HbA1c were essential indicators reflecting glucose metabolic status of fetuses. Increased insulin, C-peptide, and HbA1c usually implied islet β-cell dysfunctions and insulin resistance, which was closely linked with dyslipidemia and amino acid transportation disorders (22, 29, 30). Fetal growth restriction and failure to thrive in early-childhood might occur as a result of impaired protein and lipid synthesis, transportation, and degradation (21–25). Furthermore, evidence has shown that oxidative stress and overproduction of reactive oxygen species (ROS) were involved in the process of metabolic dysfunction and obesity (31, 32). Thus, we speculated that multiple complicated factors contributed to the inverse associations between cord plasma C-peptide and HbA1c levels and weight Z-scores and BMI Z-scores at early childhood.
Compared with existing findings, there was an inconsistent result in our study. It has been demonstrated that cord plasma TG levels were positively associated with adiposity indicators among SGA infants but tended to be negatively associated with adiposity indicators among LGA infants in the research of Ye et al. (19). However, our outcomes did not find such opposite associations between TG levels and weight Z-scores or BMI Z-scores stratified by weight for gestational age, even after adjusting for confounding variables. From our perspective, the contradictory results might be explained by several factors affecting early-life growth including feeding pattern, dietary nutrition intake, physical activity, and other interference factors (especially catch-up growth in SGA infants). Future similar prospective studies were required to further explore the association and clarify the discrepancy.
Our study advanced insights regarding the relationship between cord blood metabolic factors and early-childhood overweight and obesity. Our consequences indicated that elevated cord plasma TG, HbA1c, and TG/TC ratios were associated with decreased risks for overweight and obesity. The results were consistent with findings from Cao et al. (20), which discovered that 22 triacylglycerols and diacylglycerols were negatively associated with early-onset overweight and obesity. A meta-analysis reviewed 22 studies and concluded that umbilical cord blood leptin was inversely associated with adiposity up to 3 years of age (16). In addition, two recent studies provided evidence convincing the involvement of cord blood metabolites (including cholestenone, branched-chain amino acid, and other metabolites) in the tendency to rapid postnatal growth and of rapid postnatal growth in the tendency to childhood overweight (33, 34). So far, explorations concerning the relationships between cord blood metabolites and childhood overweight and adiposity were very limited in past research. We contributed to finding the underlying cord blood biomarkers for predicting early-childhood overweight and obesity.
There were some limitations that could not be ignored in our study. Firstly, our study population was mainly enrolled from hospitals in Zhejiang Province. Given the huge population gross of China, a multicenter birth cohort study with a larger sample size and multiple nationalities would be more representative. Secondly, anthropometric measurements were obtained from different instruments by different clinicians and nurses. We could not rule out the possibility of measuring error. Thirdly, some maternal data (e.g., prepregnancy weight, height, and family income) were collected through self-reported questionnaires, which might be subject to recall bias. Fourthly, our participants were followed up to 18 months with weights and lengths measured at different time points. The study could be more ideal if other adiposity indicators such as subscapular and triceps skinfold thickness were included and longer periods were followed up. Finally, as mentioned before, childhood growth was impacted by several complicated factors (e.g., feeding pattern, dietary nutrition intake, physical activity, vitamin D supplement, etc.). Additional studies with confounding variables well-controlled are needed to elucidate the relationship between cord blood metabolic factors and growth and development in the future.
Cord blood TG concentrations and TG/TC ratios were negatively associated with weight Z-scores and BMI Z-scores from birth to 18 months. Cord blood C-peptide and HbA1c levels had inverse correlations with early-childhood weight Z-scores and BMI Z-scores. Cord blood TG, TG/TC ratios, C-peptide, and HbA1c had negative correlations with weight gains from birth to 6 months, but the correlations attenuated as time went on. Increased cord plasma TG, HbA1c levels, and TG/TC ratios were associated with decreased risks for overweight and obesity at 6 months, 12 months, and 18 months. Therefore, cord blood metabolic factors were significantly associated with early-childhood growth patterns.
The raw data supporting the conclusions of this article will be made available by the authors, without undue reservation.
The studies involving human participants were reviewed and approved by Clinical Research Ethics Committee of Yongkang, Huzhou, Cixi, Ninghai, Shaoxing, Yiwu, Dongyang and Wenling Maternal and Child Health Care Hospitals. Written informed consent to participate in this study was provided by the participants’ legal guardian/next of kin.
W-YJ participated in study design, data acquisition, literature review, statistical analysis and drafted the manuscript. X-YC, TH, YJ, T-TC and Z-HW contributed to data acquisition, literature review and performed statistical analysis. Z-WZ and Z-YZ conceived the study, participated in its design and were the corresponding authors of this manuscript. All the authors read and approved the final submitted version.
This study was funded by the “11th Five-Year Plan” (2009BAI80B03) and the “12th Five-Year Plan” (2012BAI02B03) from the National Science and Technology Issues Research, China and the Innovation Program for Early Screening and Intervention of Birth Defects, Zhejiang Province (2010R50045).
We are grateful for all the researchers from Yongkang, Huzhou, Cixi, Ninghai, Shaoxing, Yiwu, Dongyang and Wenling Maternal and Child Health Care Hospitals in the follow up and data collection and the participation of all the subjects in our study. We also thank Professor Colin Binns and Professor Andy Lee from School of Public Health, Curtin University, Australia for their guidance in statistical analysis.
The authors declare that the research was conducted in the absence of any commercial or financial relationships that could be construed as a potential conflict of interest.
All claims expressed in this article are solely those of the authors and do not necessarily represent those of their affiliated organizations, or those of the publisher, the editors and the reviewers. Any product that may be evaluated in this article, or claim that may be made by its manufacturer, is not guaranteed or endorsed by the publisher.
The Supplementary Material for this article can be found online at: https://www.frontiersin.org/articles/10.3389/fendo.2023.1164747/full#supplementary-material
1. Gillman MW. Developmental origins of health and disease. N Engl J Med (2005) 353:1848–50. doi: 10.1056/NEJMe058187
2. Arima Y, Fukuoka H. Developmental origins of health and disease theory in cardiology. J Cardiol (2020) 76:14–7. doi: 10.1016/j.jjcc.2020.02.003
3. Patro Golab B, Santos S, Voerman E, Lawlor DA, Jaddoe VWV, Gaillard R, et al. Influence of maternal obesity on the association between common pregnancy complications and risk of childhood obesity: an individual participant data meta-analysis. Lancet Child Adolesc Health (2018) 2:812–21. doi: 10.1016/S2352-4642(18)30273-6
4. Gademan MG, Vermeulen M, Oostvogels AJ, Roseboom TJ, Visscher TL, van Eijsden M, et al. Maternal prepregancy BMI and lipid profile during early pregnancy are independently associated with offspring's body composition at age 5-6 years: the ABCD study. PloS One (2014) 9:e94594. doi: 10.1371/journal.pone.0094594
5. Daraki V, Georgiou V, Papavasiliou S, Chalkiadaki G, Karahaliou M, Koinaki S, et al. Metabolic profile in early pregnancy is associated with offspring adiposity at 4 years of age: the rhea pregnancy cohort Crete, Greece. PloS One (2015) 10:e0126327. doi: 10.1371/journal.pone.0126327
6. Huang JS, Chen QZ, Zheng SY, Ramakrishnan R, Zeng JY, Zhuo CP, et al. Associations of longitudinal fetal growth patterns with cardiometabolic factors at birth. Front Endocrinol (Lausanne) (2021) 12:771193. doi: 10.3389/fendo.2021.771193
7. Hou RL, Jin WY, Chen XY, Jin Y, Wang XM, Shao J, et al. Cord blood c-peptide, insulin, HbA1c, and lipids levels in small- and large-for-gestational-age newborns. Med Sci Monit (2014) 20:2097–105. doi: 10.12659/MSM.890929
8. Wang J, Shen S, Price MJ, Lu J, Sumilo D, Kuang Y, et al. Glucose, insulin, and lipids in cord blood of neonates and their association with birthweight: differential metabolic risk of Large for gestational age and small for gestational age babies. J Pediatr (2020) 220:64–72.e2. doi: 10.1016/j.jpeds.2020.01.013
9. Bhowmik B, Siddique T, Majumder A, Mdala I, Hossain IA, Hassan Z, et al. Maternal BMI and nutritional status in early pregnancy and its impact on neonatal outcomes at birth in Bangladesh. BMC Pregnancy Childbirth (2019) 19:413. doi: 10.1186/s12884-019-2571-5
10. Kadakia R, Talbot O, Kuang A, Bain JR, Muehlbauer MJ, Stevens RD, et al. Cord blood metabolomics: association with newborn anthropometrics and c-peptide across ancestries. J Clin Endocrinol Metab (2019) 104:4459–72. doi: 10.1210/jc.2019-00238
11. Sifianou P, Zisis D. Cord blood triglycerides are associated with IGF-I levels and contribute to the identification of growth-restricted neonates. Growth Horm IGF Res (2012) 22(6):219–23. doi: 10.1016/j.ghir.2012.08.004
12. Mellati AA, Mazloomzadeh S, Anjomshoaa A, Alipour M, Karimi F, Mazloomi S, et al. Multiple correlations between cord blood leptin concentration and indices of neonatal growth. Arch Med Res (2010) 41:26–32. doi: 10.1016/j.arcmed.2009.12.001
13. Brunner S, Schmid D, Hüttinger K, Much D, Heimberg E, Sedlmeier EM, et al. Maternal insulin resistance, triglycerides and cord blood insulin in relation to post-natal weight trajectories and body composition in the offspring up to 2 years. Diabetes Med (2013) 30:1500–7. doi: 10.1111/dme.12298
14. Meyer DM, Brei C, Stecher L, Brunner S, Hauner H. Maternal insulin resistance, triglycerides and cord blood insulin are not determinants of offspring growth and adiposity up to 5 years: a follow-up study. Diabetes Med (2018) 35:1399–403. doi: 10.1111/dme.13765
15. Zhang DL, Du Q, Djemli A, Julien P, Fraser WD, Luo ZC. Cord blood insulin, IGF-I, IGF-II, leptin, adiponectin and ghrelin, and their associations with insulin sensitivity, β-cell function and adiposity in infancy. Diabetes Med (2018) 35:1412–9. doi: 10.1111/dme.13671
16. Bagias C, Sukumar N, Weldeselassie Y, Oyebode O, Saravanan P. Cord blood adipocytokines and body composition in early childhood: a systematic review and meta-analysis. Int J Environ Res Public Health (2021) 18:1897. doi: 10.3390/ijerph18041897
17. World Health Organization. The WHO child growth standards. Switzerland: WHO (2006). Available at: http://www.who.int/childgrowth/standards/en/.
18. Capital Institute of Pediatrics, the Coordinating Study Group of Nine Cities on the Physical Growth and Development of Children. Growth standard curves of birth weight, length and head circumference of Chinese newborns of different gestation. Zhonghua Er Ke Za Zhi (2020) 58:738–46. doi: 10.3760/cma.j.cn112140-20200316-00242
19. Ye QQ, Kong SM, Yin X, Gao C, Lu MS, Ramakrishnan R, et al. Associations of cord blood lipids with childhood adiposity at the age of three years: a prospective birth cohort study. Metabolites (2022) 12:522. doi: 10.3390/metabo12060522
20. Cao T, Zhao J, Hong X, Wang G, Hu FB, Wang X, et al. Cord blood metabolome and BMI trajectory from birth to adolescence: a prospective birth cohort study on early life biomarkers of persistent obesity. Metabolites (2021) 11:739. doi: 10.3390/metabo11110739
21. Gatford KL, Simmons RA. Prenatal programming of insulin secretion in intrauterine growth restriction. Clin Obstet Gynecol (2013) 56:520–8. doi: 10.1097/GRF.0b013e31829e5b29
22. Ma M, Liu H, Yu J, He S, Li P, Ma C, et al. Triglyceride is independently correlated with insulin resistance and islet beta cell function: a study in population with different glucose and lipid metabolism states. Lipids Health Dis (2020) 19:121. doi: 10.1186/s12944-020-01303-w
23. Li Y, Feng D, Esangbedo IC, Zhao Y, Han L, Zhu Y, et al. Insulin resistance, beta-cell function, adipokine profiles and cardiometabolic risk factors among Chinese youth with isolated impaired fasting glucose versus impaired glucose tolerance: the BCAMS study. BMJ Open Diabetes Res Care (2020) 8:e000724. doi: 10.1136/bmjdrc-2019-000724
24. Javed K, Fairweather SJ. Amino acid transporters in the regulation of insulin secretion and signalling. Biochem Soc Trans (2019) 47:571–90. doi: 10.1042/BST20180250
25. Saltiel AR, Kahn CR. Insulin signalling and the regulation of glucose and lipid metabolism. Nature (2001) 414:799–806. doi: 10.1038/414799a
26. He JR, Ramakrishnan R, Wei XL, Lu JH, Lu MS, Xiao WQ, et al. Fetal growth at different gestational periods and risk of impaired childhood growth, low childhood weight and obesity: a prospective birth cohort study. BJOG (2021) 128:1615–24. doi: 10.1111/1471-0528.16698
27. Sania A, Spiegelman D, Rich-Edwards J, Hertzmark E, Mwiru RS, Kisenge R, et al. The contribution of preterm birth and intrauterine growth restriction to childhood undernutrition in Tanzania. Matern Child Nutr (2015) 11:618–30. doi: 10.1111/mcn.12123
28. Niknam A, Ramezani Tehrani F, Behboudi-Gandevani S, Rahmati M, Hedayati M, Abedini M, et al. Umbilical cord blood concentration of connecting peptide (C-peptide) and pregnancy outcomes. BMC Pregnancy Childbirth (2022) 22:764. doi: 10.1186/s12884-022-05081-4
29. Tong X, Liu S, Stein R, Imai Y. Lipid droplets' role in the regulation of β-cell function and β-cell demise in type 2 diabetes. Endocrinology (2022) 163:bqac007. doi: 10.1210/endocr/bqac007
30. Bröer S. Amino acid transporters as modulators of glucose homeostasis. Trends Endocrinol Metab (2022) 33:120–35. doi: 10.1016/j.tem.2021.11.004
31. Furukawa S, Fujita T, Shimabukuro M, Iwaki M, Yamada Y, Nakajima Y, et al. Increased oxidative stress in obesity and its impact on metabolic syndrome. J Clin Invest (2004) 114:1752–61. doi: 10.1172/JCI21625
32. Arogbokun O, Rosen E, Keil AP, Milne GL, Barrett E, Nguyen R, et al. Maternal oxidative stress biomarkers in pregnancy and child growth from birth to age 6. J Clin Endocrinol Metab (2021) 106:1427–36. doi: 10.1210/clinem/dgab018
33. Alfano R, Plusquin M, Robinson O, Brescianini S, Chatzi L, Keski-Rahkonen P, et al. Cord blood metabolites and rapid postnatal growth as multiple mediators in the prenatal propensity to childhood overweight. Int J Obes (Lond) (2022) 46:1384–93. doi: 10.1038/s41366-022-01108-0
Keywords: cord blood, metabolic factors, TG, HbA1c, C-peptide, growth, overweight and obesity
Citation: Jin W-Y, Chen X-Y, Han T, Jin Y, Chen T-T, Wang Z-H, Zhao Z-Y and Zhu Z-W (2023) Associations between cord blood metabolic factors and early-childhood growth and overweight and obesity. Front. Endocrinol. 14:1164747. doi: 10.3389/fendo.2023.1164747
Received: 13 February 2023; Accepted: 23 June 2023;
Published: 11 July 2023.
Edited by:
Li Ming Wen, The University of Sydney, AustraliaReviewed by:
Andrew Whatmore, The University of Manchester, United KingdomCopyright © 2023 Jin, Chen, Han, Jin, Chen, Wang, Zhao and Zhu. This is an open-access article distributed under the terms of the Creative Commons Attribution License (CC BY). The use, distribution or reproduction in other forums is permitted, provided the original author(s) and the copyright owner(s) are credited and that the original publication in this journal is cited, in accordance with accepted academic practice. No use, distribution or reproduction is permitted which does not comply with these terms.
*Correspondence: Zhi-Wei Zhu, emh1emhpd2VpQHpqdS5lZHUuY24=; Zheng-Yan Zhao, emhhb3p5QHpqdS5lZHUuY24=
Disclaimer: All claims expressed in this article are solely those of the authors and do not necessarily represent those of their affiliated organizations, or those of the publisher, the editors and the reviewers. Any product that may be evaluated in this article or claim that may be made by its manufacturer is not guaranteed or endorsed by the publisher.
Research integrity at Frontiers
Learn more about the work of our research integrity team to safeguard the quality of each article we publish.