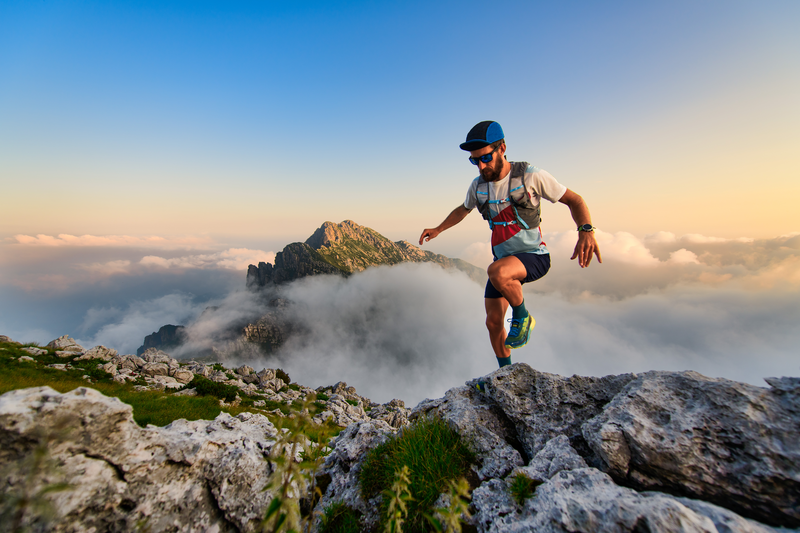
94% of researchers rate our articles as excellent or good
Learn more about the work of our research integrity team to safeguard the quality of each article we publish.
Find out more
REVIEW article
Front. Endocrinol. , 05 April 2023
Sec. Cancer Endocrinology
Volume 14 - 2023 | https://doi.org/10.3389/fendo.2023.1160267
This article is part of the Research Topic Lineage Plasticity and Epigenetic Rewiring in Hormone-Driven Cancers View all 6 articles
Prostate cancer is one of the most common malignancies in males wherein 1 in 8 men are diagnosed with this disease in their lifetime. The urgency to find novel therapeutic interventions is associated with high treatment resistance and mortality rates associated with castration-resistant prostate cancer. Anoikis is an apoptotic phenomenon for normal epithelial or endothelial cells that have lost their attachment to the extracellular matrix (ECM). Tumor cells that lose their connection to the ECM can die via apoptosis or survive via anoikis resistance and thus escaping to distant organs for metastatic progression. This review discusses the recent advances made in our understanding of the signaling effectors of anoikis in prostate cancer and the approaches to translate these mechanistic insights into therapeutic benefits for reducing lethal disease outcomes (by overcoming anoikis resistance). The prostate tumor microenvironment is a highly dynamic landscape wherein the balance between androgen signaling, cell lineage changes, epithelial-mesenchymal transition (EMT), extracellular matrix interactions, actin cytoskeleton remodeling as well as metabolic changes, confer anoikis resistance and metastatic spread. Thus, these mechanisms also offer unique molecular treatment signatures, exploitation of which can prime prostate tumors to anoikis induction with a high translational significance.
Prostate cancer (PCa) is a heterogeneous disease wherein prostatic intraepithelial neoplasia progresses into invasive metastatic carcinoma (1, 2). Treatment modalities effectively used in the clinic for localized disease include prostatectomy, radiation therapy and medical or surgical androgen deprivation (3). PCa is treatable if diagnosed early, however the high mortality rate associated with the disease is a result of therapeutic resistance to androgen-deprivation therapy (ADT), radiotherapy and chemotherapy. Targeted therapeutics that can overcome resistance are being developed by determining the underlying mechanisms of androgen receptor (AR) signaling in PCa. Moreover, distant metastasis associated with lethal disease holds high potential for the identification of new actionable sites during invasion and progression of PCa cells.
Eukaryotic cells die via mechanisms inducing apoptosis, anoikis and necrosis (4, 5). Normal tissue homeostasis is a result of a balance between cell proliferation and apoptosis (6). Apoptosis is the main pathway for tumor cell death in response to treatment modalities in prostate cancer patients and defects in apoptosis have been linked to not only tumor progression and metastasis but also therapeutic resistance (7, 8). The term “anoikis” (Greek for “homelessness”) was coined by Frisch to define apoptotic cell death induced by insufficient cell-extracellular matrix (ECM) interactions (1, 9). Anoikis prevents dissemination of cells to inappropriate distant sites during metastasis (1, 10). Resistance of cancer cells to anoikis confers them increased survival in the absence of ECM attachment, ability to travel through circulatory and lymphatic systems followed by dissemination and attachment at secondary sites leading to metastasis (11, 12). Understanding the molecular mechanisms contributing to anoikis resistance is thus crucial for development of targeted therapeutics (11).
Mechanistic regulation of anoikis proceeds by cell death receptor-mediated (extrinsic) and mitochondrial (intrinsic) apoptotic pathways (13). Death ligands (FAS, Tumor Necrosis Factor-a, TRAIL) bind to the extracellular domain of the death receptors to activate the death receptor pathway (14, 15). The death-inducing signaling complex (DISC) is formed when death effector domain (DED) of FAS-associated death domain (FADD) binds with caspase-8 (FLICE). FLICE when released into the cytoplasm cleaves caspase-3 and caspase-7 followed by cell death of cellular substrates (12, 16). FLICE-inhibitory protein (FLIP) inhibits caspase-8 binding and activation by having preferential affinity for DISC. This death receptor pathway wherein matrix attachment prevents FAS-induced apoptosis is mechanistically engaged in anoikis induction (12). Loss of ECM anchorage leads to increased FAS, decreased FLIP leading to anoikis (17, 18). X-linked inhibitor of apoptosis proteins (XIAP) binds and inhibits active Caspases 3/7. Anoikis resistant PCa cells have increased levels of XIAP compared to normal prostate epithelial cells (14, 19). In addition, increased level of XIAP in metastatic PCa cells is associated with anoikis resistance (14, 20). The mitochondrial intrinsic apoptotic signaling is regulated by proteins in the Bcl-2 family (15). Tumor cell survival and apoptosis is regulated by the balance between pro-apototic Bcl-2 proteins (Bax, Bad, and Bid) and anti-apoptotic proteins (Bcl-2 and Bcl-xL) (15). Initiation of death signals leads to the translocation of Bax or Bid from the cytosol to the outer mitochondrial membrane leading to cytochrome release and caspase 9 and 3 activation (12). This translocation of Bid after loss of adhesion has been observed during anoikis of mammary epithelial cells (12). Bcl-2 binds to Bax and Bad, maintains mitochondrial membrane integrity and prevents apoptosis. In Ras-transformed intestinal cells, decreased Bak and failure to downregulate Bcl-xL leads to anoikis resistance. Non-transformed intestinal cells undergo anoikis via release of mitochondrial Omi/HtrA2 and detachment-induced down-regulation of Bcl- xL (14). Moreover, there is evidence to suggest the presence of Bcl-2-independent anoikis mechanisms in PCa cells (21). The pro-apoptotic signal of Bim (Bax activator) is muted by its translocation to the mitochondria and interaction with Bcl-xL after cell detachment. Upregulation of Bim via inhibition of Src, Erk and Akt pathways “primes” breast cancer cells to anoikis (22).
Bit1 protein (Bcl2 inhibitor of transcription 1) mechanistically mediates anoikis via integrins, independent of caspases (23, 24). Bit1 apoptosis is blocked by integrin-mediated attachment thus making Bit1 a crucial player in anoikis. Bit1 suppression has been correlated with advanced metastasis via Erk pathway stimulation and anoikis resistance in breast cancer models (24). Bit1 expression is reduced in non-small cell lung carcinoma tumors and exogenous Bit1 overcomes anoikis resistance in human lung adenocarcinoma cells via caspase-independent pathways by prohibiting anchorage-independent growth (13). Bit1 overexpression/induction can be a critical path in overcoming anoikis resistance in PCa treatment.
Epithelial-mesenchymal transition (EMT) is a highly conserved cellular process that contributes to physiologic and pathologic conditions and allows a polarized epithelial cell to adopt a mesenchymal phenotype (25). The polarized epithelial cell thus loses its ability to maintain interactions with the basement membrane and converges into an apoptosis-resistant, migratory and invasive mesenchymal cell. Several studies have shown that the malignant transformation of cells is a result of disruption of homeostasis by increased EMT (25). Cellular markers for the morphological profiling of EMT include epithelial markers (E-cadherin, B-catenin, Cytokeratin) or mesenchymal markers (N-cadherin, Vimentin, Fibronectin, Snail, Slug, Twist or Matrix Metalloproteinases-2,3,9) (25) (Figure 1). The phenotypic landscape of EMT is represented by loss of epithelial markers (E-cadherin, B-catenin, occludin) and a gain of mesenchymal markers (e.g. N-Cadherin, Zeb-1/2, vimentin and E-cadherin repressors such as Snail, Twist) (4). Ras is also a critical signaling molecule which induces EMT via the PI3K or MAPK pathways (6, 26, 27). Loss of E-cadherin expression leads to loss of adherence to junctional proteins (B-catenin and actin), ultimately compromising epithelial cell plasticity and conferring anoikis resistance (4). Phenotypic EMT functionally contributes to tumor progression and recurrence via enhanced invasive and metastatic properties, increase in cancer stem cell populations and therapeutic resistance consequential to anoikis resistance (28).
Figure 1 The interplay of EMT-MET maintains the pivotal balance between normal cell anoikis and cancer progression/anoikis resistance. The mesenchymal phenotype contributes to anoikis resistance after ECM detachment via activation/dysregulation of pathways such as androgen signaling, Akt/PI3K, Src and Notch signaling. Anoikis resistance can be overcome by drugs (DZ-50), regulating ROS balance, targeting miRNA or cell adhesion molecules that inactivate these pathways, push the switch in favor of MET and/or make the cancer cells anoikis sensitive.
The multilayered and complex relationship between the signaling effectors of EMT and anoikis in cancer progression and therapeutic resistance, remains the focus of intense investigative efforts. Studies in breast cancer using conditional knockout of E-cadherin have shown correlation of E-cadherin knockdown to a highly metastatic, angiogenesis- dependent anoikis resistant phenotype (29). Interestingly, anoikis sensitivity was restored in mammary epithelial cells when E-cadherin and B-catenin were knocked down together (4, 28, 30). Ankyrin-G is an actin cytoskeleton and cell membrane linker protein. The interaction of Ankyrin-G with E-cadherin also influences anoikis resistance. EMT downregulates ankyrin G leading to reduced p14ARF (tumor suppressor and anoikis promoter) (4, 31). Vimentin, a hallmark of mesenchymal cells is an intermediary filament that provides structural support to cells and is overexpressed in human cancers including prostate cancer. A significant association has been found between vimentin’s regulation and decreased prostate tumor cell invasiveness (32–36). Compelling evidence linking anoikis and EMT stems from studies on prostate apoptosis response-4 (Par-4), a tumor suppressor which selectively induces apoptosis via caspase-dependent mechanisms in cancer cells but not normal cells (37). At the mechanistic level, Arylquin 1 (a 3-arylquinoline derivative and potent Par-4 secretagogue) binds to vimentin, displaces Par-4 from vimentin and the secreted Par-4 induces paracrine tumor cell apoptosis in PCa cells (37, 38). Ginsenoside 20(R)-Rg3 can prevent EMT induction via p38 MAPK inhibition, vimentin and Snail suppression, E-cadherin increase and by overcoming anoikis resistance, migration and invasion in lung cancer cells (39). As the mesenchymal phenotype confers resistance to anoikis induction, the therapeutic response can be improved by priming them into being reprogrammed to the epithelial phenotype [reversing the EMT phenotype to mesenchymal-epithelial transition (MET)] (4). The plasticity of prostate cells in the tumor microenvironment (TME) allows for the interconversion between de-differentiated EMT and re-differentiated MET phenotypes during tumor progression (40). Transcriptional repressors of E-cadherin, such as Snail, activate the PI3K/Akt survival pathway, inhibiting capsase-3-mediated apoptosis (1, 4). Twist is another transcriptional regulator of EMT by inducing mesenchymal markers such as fibronectin and N-cadherin (1). Twist plays an anti-apoptotic role and its loss could be critical in conferring anoikis sensitivity in cancer cells (1, 41, 42). The zinc finger E-box-binding homeobox 1 (Zeb) family of transcription factors, also play a role in cancer progression wherein Zeb1 and Zeb2 suppress E-cadherin transcription (43). In studies using kidney cell line, the plasticity balance between epithelial to mesenchymal state interconversion is regulated by transforming growth factor-beta (TGF-β) related ZEB expression and miR-200 negative correlation (44). Knocking down Zeb1 as a mode of overcoming anoikis resistance and improving therapeutic response provides promise, wherein silencing Zeb1 in PCa cell lines leads to decreased cancer stem cell markers (CD44, CD133 and SOX2) and decreased ability to form protospheres and colonies (45). Of translational significance is the association of Zeb1 overexpression in highly aggressive tumors and higher Gleason grade in PCa, implicating a role for this EMT molecular regulator in prostate tumor progression to advanced disease (46).
TGF-β is a multifactorial regulator peptide that plays a dual role as an inhibitor of tumorigenesis in normal and early stage disease and a promoter of advanced PCa (32). In early tumor development, TGF-β induces cell cycle arrest and apoptosis to inhibit tumor development. This is then followed in the later stages by TGF-β induced tumor promotion through aberrant cell cycle regulation (47). In metastatic disease, increased production of TGF-β is associated with immunosuppression via T-cell regulation, ECM degradation, EMT propagation and angiogenesis – a combination that positively supports tumor cell invasion. TGF-β induces tumor progression and metastasis by promoting EMT in PCa cells (32, 48) via constitutively activating Akt and thus inhibiting SMAD3 translocation to the nucleus (49, 50). At the same time, there is a complex and fateful interaction between TGF-β and tumors making it imperative to target TGF-β before its involvement in the metastatic process, while it switches from a tumor suppressor to metastasis promoter (48). TGF-β induced EMT in PC3 PCa cells was inhibited via nuclear factor κB (NF-κB) signaling blockade resulting into decreased vimentin expression (32, 51). Snail regulates TGF-βI expression and TGF-β promotes post-translational modification of Snail (sumoylation). Sumoylated Snail leads to increased invasiveness and aggressiveness in PCa cells via Hes1 (transcriptional target in Notch signaling cascade) (52). Studies focused on understanding the effects of systemic TGF-β inhibition on early stages of prostate tumorigenesis have used trivalent TGF-β receptor trap (RER) comprised of domains from the TGF-β II and III receptors to completely block (a) TGF-β II binding and (b) TGF-β1 and TGF-β3 signaling in cultured PCa cells. Early inhibition of TGF-β using RER led to reduced prostate cell proliferation (reduced Ki67 positive cells) and invasion capacity of cells in prostate glands of Pten conditional null mice (53).
Prostate glandular epithelial cells depend on androgens for growth and survival. The AR is a steroid receptor that stays inactive while bound to chaperone proteins (heat shock protein 90) in the cytoplasm. Testosterone dissociates from sex hormone binding protein, diffuses across the prostatic plasma membrane and is converted into dihydrotestosterone (DHT) by cytochrome p450 enzyme 5α-reductase. AR after binding to DHT undergoes a conformational change and translocates into the nucleus to bind androgen response elements (ARE) leading to the stimulation of androgen-dependent proteins (54–58) (Figure 1). ADT achieved surgically or chemically (with luteinizing hormone-releasing hormone (LHRH) agonists, LHRH antagonists, or anti-androgens) is the mainstay therapeutic modality for the treatment of advanced PCa. AR is not only a driver of PCa progression but is also critical in clinical response to therapy (59). Significantly enough, androgen signaling has been implicated in EMT-MET phenotypic interconversions (60, 61). The evidence points to negative correlation between EMT effectors and AR activity, co-involved in metastatic PCa progression (62). AR deletion in the Transgenic Adenocarcinoma Mouse Prostate (TRAMP) tumors leads to TGF-β1 dependent decrease in MET, increased mesenchymal state and EMT also followed by higher cell invasion rate and anoikis resistance (63). AR expression is increased in triple negative breast cancer and this increase and anchorage-independent survival/anoikis resistance is also TGF-β dependent (64, 65). Interestingly, TGF-β mediated apoptosis was enhanced rather than prevented by androgens (DHT) in hormone-sensitive PCa cells thus opening avenues for priming PCa cells to apoptotic induction pathways by using physiologic levels of androgens (66). Bcl-2 (apoptotic suppressor) is able to block the apoptotic signaling of TGF-β and DHT. Thus, Bcl-2 has a role in the emergence of castration resistant PCa (CRPC) (67). Pre-clinical studies have shown that resistance to cabazitaxel chemotherapy can be overcome by anti-androgen induced EMT to MET conversion in androgen sensitive tumors (68). Interestingly enough a dual role for AR has been shown in the promotion of hepatocellular carcinoma (HCC) initiation; and loss of AR leads to HCC metastasis with increased incidence of undifferentiated tumors in preclinical models (69). Hepatic AR suppressed p38 phosphorylation and NF-κB/matrix metallopeptidase 9 pathway leading to anoikis induction and reduced migration of HCC cells (69). Thus AR plays a role in the phenotypic landscape of the TME in other cancers, besides prostate tumors.
The prostate epithelium consists of luminal, basal and neuroendocrine cells wherein the former two possess progenitor activity (70). Deletion of E-cadherin in prostate luminal cells results into anoikis followed by a tissue repair program of differentiation of basal epithelial cells to luminal cells (70). Basal cells express adhesion -associated membrane receptors and their substrates in the ECM and can establish cell-matrix interactions autonomously thus escaping anoikis (71). Transmembrane serine protease 4 (TMPRSS4) is responsible for invasion and proliferation of PCa cells and its elevated levels are associated with poor prognosis in several cancers including prostate, gastric, colorectal and non-small cell lung cancer. Stemness-related factors such as SOX2, BMI1 and CD133 can functionally promote anoikis resistance. The stemness and anoikis resistance promoting properties of TMPRSS4 were also associated with contributions by EMT-inducing transcription factors Slug and Twist (72). Metastatic human PCa cells when exposed to clinically-relevant radiation doses lead to generation of two radiation-surviving cell populations- adherent senescent-like cells and non-adherent anoikis-resistant stem cell-like cells (73). The anoikis-resistant cells also demonstrate radioresistance-associated EMT, via Snail over-expression and activation of the PI3K/Akt and Erk1/2 pathways (73).
Src is highly expressed in PCa and its activation has been associated with cancer progression and anoikis resistance (15). Dasatinib, a Src family kinase (SFK) inhibitor shows therapeutic promise in controlling tumor growth and lymph node metastases in PCa models in vivo (15, 74). Dasatinib blocks Focal adhesion kinase (FAK) in human PCa cells (15, 75). Persistent redox signals in PC3 cells result into chronic pro-survival signaling via Src oxidation in the absence of cell adhesion leading to epidermal growth factor phosphorylation (76). Notch activation induces anoikis resistance of prostate luminal progenitors by augmenting NF-kB activity and thus rescuing their capacity for renewal and unipotent differentiation (71). NF-κB also leads to anoikis resistance and tumor cell survival downstream of the PI3K/Akt pathway (11, 77).
The new stromal microenvironment, formed as a result of tumor cell invasion into basement membrane, is comprised of increased ECM remodeling, angiogenesis, growth factor bioavailability, protease activity and inflammatory influx (1). Anoikis resistance conferred by loss of apoptotic pathways and activation of survival mechanisms, is followed by phenotypic changes and increased neovascularization within the reactive stroma in the prostate TME (1). The myofibroblasts in the reactive stroma secrete molecules fibronectin, collagen I and III, glycoproteins and proteoglycans that directly influence ECM remodeling (1). Thus the reactive stroma is responsible for initiating EMT and prostate tumor neovascularization (1). Connective tissue growth factor (CTGF) is expressed by stromal cell types such as endothelial cells, fibroblasts, smooth muscle cells and myofibroblasts. CTGF plays a role in (a) stromal extracellular matrix synthesis, proliferation and migration in smooth muscle cells; (b) proliferation, cell adhesion, cell spreading in fibroblasts and also (c) endothelial cell adhesion, proliferation, migration, and angiogenesis. Thus, CTGF is a key component of the reactive stromal compartment of different cancers including tumor-promoting prostate stromal cells. TGF-β1 is a critical regulator of CTGF in the reactive prostate stroma (78). The expression levels of CTGF can influence therapeutic response (79). Over expression of CTGF is associated with poor clinical outcomes including advanced disease with larger tumor size, worse metastasis, decreased treatment response in many cancers such as but not limited to breast, gastric, esophageal, pancreatic and prostate cancer (79, 80). Interestingly, in lung cancer CTGF displays an anti-metastasis function wherein it binds to epidermal growth factor receptor (EGFR) leading to EGFR degradation by ubiquitination and also suppresses the phosphorylation of c-Src and promotes anoikis (81). This potential anoikis-promoting mechanism and metastasis suppressive impact of CTGF with anti-EGFR therapy serve as a therapeutic avenue for lung cancer (81).
Progression of PCa is a dynamic integration of three primary events that critically impact the TME landscape: Phenotypic EMT, formation of a reactive stroma which involves structural rearrangement of the ECM, and cell death via anoikis (1). Diverse protein kinases and phosphatases are protagonists of anoikis regulation (6). The transmembrane proteins- integrins are primary regulators of cell-ECM interactions (15). FAK is an integrin signaling molecule that is recruited into focal adhesions upon cell-ECM contact, leading to phosphorylation and activation of FAK (11). Mechanistically, anoikis resistance is a result of constitutive activation of FAK (82, 83). PI3K is a FAK-activated protein that recruits Protein Kinase B (PKB/Akt) leading to cell survival (11). This complex chain of integrin-dependent events have been shown to be involved in PCa progression to metastatic disease (15, 84). Src belonging to the SFK is activated via β1-integrin through FAK in the focal adhesion complex upon cell-ECM adhesion (15). Anoikis resistance in PCa has been linked to the phosphorylation of FAK and Akt by β1-integrin (16, 85). Notably, loss of β1-integrins in PCa cells overcomes anoikis resistance and treatment with the β1-integrin neutralizing antibody mAB 33B6 reduces tumor metastases in vivo (85). Collagen XIII is an integrin-binding transmembrane protein responsible for cell-cell and cell-matrix interactions, that induces β1-integrin activation, cancer cell stemness, metastasis and anoikis resistance (86). Rad9, a DNA damage response and repair protein is upregulated in human PCa. Rad9 contributes to tumor survival, proliferation, migration and anoikis resistance in PCa cells, a phenomenon that can be overcome by silencing Rad9, towards increasing anoikis sensitivity (87). αvβ3 integrin can confer anoikis resistance and a migratory phenotype to PCa cells (11). Invasive AR-negative, androgen-independent PC3 cells express αvβ3 integrin whereas noninvasive androgen-sensitive LNCaP cells do not (88). Members of the arginine-glycine-aspartic acid (RGD)- binding integrin family that recognize the RGD sequence in the ECM are expressed differentially in primary prostate tumors compared to normal prostate tissue and are interesting therapeutic targets (89). Studies using Small-molecule integrin antagonists against RGD-integrins obstruct glioblastoma infiltration and malignancy via detachment-mediated anoikis induction in glioma cancer stem cells (90). The upregulation of adhesion complex protein-Talin is associated with anoikis resistance and increased invasion and metastatic properties in prostate tumor cells followed by downstream activation of FAK/Akt signaling. Human PCa specimens exhibit a higher expression of cytoplasmic talin1 in metastatic tissue compared to primary tumors (83, 91); and a significant inverse correlation between talin and E-cadherin in human prostate tumors and metastatic lesions has been reported (91). Talin thus serves as an anoikis effector which can be therapeutically targeted within the prostate TME and vascularity (83). The humanized monoclonal antibody Trastuzumab that targets human EGFR2 suppresses the angiogenic and invasive properties of anoikis-resistant endothelial cells. The reversal of tumor phenotype by Trastuzumab is via reduction in the expression of Heparan sulfate proteoglycans such as syndecan‐4 and perlecan that play a role in proliferative-migratory and angiogenic pathways respectively and are also overexpressed in anoikis-resistant endothelial cells (92, 93).
β-catenin is an adhesion molecule that functionally interacts with E-cadherin and regulates the dynamics of the actin cytoskeleton remodeling and phenotypic EMT dictating cell behavior (25). When invasive cells undergo EMT, B-catenin remains in the cytosol and nucleus in association with an activated Wnt pathway in comparison to non-invasive cells wherein it is on the membrane (25). B-catenin is a contributor to an invasive and malignant phenotype by binding to AR and translocating into the nucleus. This effect of B-catenin and AR interaction is further amplified in cells lacking E-cadherin (EMT phenotype) (25, 94, 95), pointing to B-catenin as a potential predictive biomarker for PCa progression and therapeutic response (25, 96). Tyrosine kinase inhibitor Imatinib mesylate, which is clinically used to treat leukemia and gastric cancers, might be considered for potentially treating PCa owing to its inhibition of the Wnt/B-catenin signaling pathway (35, 97, 98).
CTGF is a target gene for Yes-associated protein (YAP) which is an organ size regulator and a human oncogene. The Hippo tumor suppressor pathway is activated upon cytoskeletal reorganization and cell detachment and phosphorylates and inhibits YAP leading to anoikis in non-transformed cells. The Hippo pathway is deregulated in PCa cells and the expression of its kinases Lats1/2 is downregulated in metastatic PCa leading to anoikis resistance (99). YAP knockdown or inhibition by small molecule inhibitor (CA3) of YAP transcriptional activity overcomes anoikis resistance in melanoma cells (100). The β-adrenergic receptor antagonist, propranolol was able to overcome neuroendocrine signaling in cervical cancer cells by prohibiting norepinephrine-induced YAP-mediated anoikis resistance (101). Decreased α3-integrin expression is associated with cancer progression to metastasis under detached and low anchorage conditions via YAP upregulation (102). In the same PCa model, Abl kinase that plays a role in cytoskeleton remodeling in response to extracellular stimuli, cell motility and adhesion seemed to work together with α3-integrin to suppress RhoA activity and support Hippo function and thus restrain metastasis (102). Abl kinase inhibitor and Platelet-derived growth factor targeting imatinib shows cancer cell proliferation in PDGF independent tumors (102–104). Understanding the Hippo signaling pathway and the intricacies of its regulators in the context of anoikis are necessary for therapeutic development of Hippo and anoikis-targeted treatment in PCa.
Overcoming resistance in aggressive PCa needs to be a combination of (i) reversing anoikis resistance in tumor cells by increasing susceptibility to anoikis-inducing agents (ii) blocking key players or pathways involved in cancer cell seeding and survival at secondary locations (iii) making tumor cells less sensitive to chemotactic cues of the new target organ, thus decreasing the probability of metastatic spread (1).
α1-adrenoreceptor antagonists are clinically approved for the treatment of hypertension, and as first-line treatment for benign prostatic hyperplasia and show anti-tumor and pro-apoptotic activity (3, 105, 106). Clinically available quinazoline-based α1-adrenoceptor antagonists such as doxazosin and terazosin induce TGF-β dependent apoptosis in the epithelial, endothelial and smooth muscle cells of the prostate (15, 107). Doxazosin induces apoptosis by activating caspase-3 followed by FAK cleavage and ultimately anoikis thus operating via an α1-adrenoreceptor independent, anoikis dependent, anti-angiogenetic mechanism (108–111).
Structural optimization of quinazoline-based α1-adrenoceptor antagonist, Doxazosin, has led to the generation of a compound namely DZ-50 (16, 112, 113). DZ-50 induced anoikis and anti-angiogenesis in PCa and human PCa xenografts in nude mice (4). Critical effectors of EMT (N-cadherin) and tight junctions that are associated with poor clinical outcomes in PCa are downregulated during DZ-50 induced anoikis (114, 115). DZ-50 and its property to induce EMT to MET transition can thus be leveraged for metastatic CRPC treatment and potentially overcoming therapeutic resistance via an anoikis-driven response. Insulin growth factor binding protein 3 which is involved in stromal remodeling during PCa progression is downregulated in DZ-50 treated PCa cell lines (114). Genome-wide analysis in the DU-145 human PCa cell line has shown that DZ-50 down regulates target genes involved in focal adhesion integrity (fibronectin, integrin-a6 and talin), tight junction formation (claudin11) as well as angiogenesis modulator thrombospondin 1 (113). Targeting the TGF-β signaling pathway has also been addressed via these quinazoline based α1-adrenoceptor antagonists or anti-sense inhibition of TGF-β1 expression (107, 116).
Small molecule inhibitors for TGFβR kinases are under investigation in clinical trials for treatment of cancer patients via TGF-β signaling targeting. Galunisertib which selectively binds to TGFβR1 and inhibits its kinase activity has shown pre-clinical promise and Phase 1/2 clinical trials are underway for its use in metastatic disease in a variety of cancers including prostate, pancreatic cancer and glioblastoma (117). Studies conducted on Dominant Negative TGFβRII male mice using combination of galunisertib and FDA-approved antiandrogen enzalutamide, not only showed reduced prostate tumor growth by increased apoptosis and reduced tumor cell proliferation but also reduced cofilin and reversion of EMT to MET phenotype (118). Phase 2 clinical trials are underway for the use of Galunisertib and Enzalutamide in Metastatic CRPC (NCT02452008). Chemotherapy-induced transcriptome reveals a highly activated TGF-β signaling, involving a chemotherapeutic associated stimulation of Smad2/3 phosphorylation, cell migration, and upregulation of EMT and cancer stem cell markers. Herein, RER with cisplatin showed improved tumor inhibition in xenograft ovarian cancer models thus focusing on avenues of combining chemotherapeutics with TGF-β inhibition (119). Proteolysis-targeting chimeras (PROTACs) are bifunctional molecules that recruit a target protein and bind it to ubiquitin ligase leading to binding of the protein to ubiquitin and resulting into its degradation (43). PROTAC molecules were developed for targeting AR wherein ARV-110 (oral PROTAC drug for AR degradation) went into Phase 1 clinical trials for patients with metastatic CRPC (120). PROTAC technology shows promise in potentially overcoming anoikis resistance (43).
The death receptor pathway inhibitor- FLIP has been demonstrated to assist malignant cells in escaping anoikis (12, 14). FLIP inhibition with siRNA or small molecules in a mouse model of PCa metastases prevented the formation of distant metastatic tumors (14). In studies designed to use a novel high-throughput screen to identify molecules that can sensitize resistant cancer cells to anoikis, it was seen that anisomycin was able to sensitize anoikis-resistant PCa cells by decreasing levels of FLIP and thus activating the death receptor pathway (121). Polyphenylurea based inhibitors of XIAP have shown to sensitize resistant PCa cells to anoikis as well as prevent distant tumor formation in orthotopic PCa models (14, 20, 122, 123).
Reactive oxygen species (ROS) have a dual effect on anoikis resistance based upon the cell type and the mechanisms are not properly understood (124–126). Transient changes in levels of ROS are essential in cell cycle regulation, cell proliferation and adhesion (76). However, it was recently reported that ROS production is elevated in cancer cells (127), with evidence supporting a connection between elevated ROS in metastatic prostate carcinoma cells and resistance to anoikis via chronic pro-survival signaling (76). Tumor cells themselves also trigger anti-oxidant enzymes for survival in response to elevated ROS, by inducing transcription factor Nrf-2 (11). Snail is involved in increased ROS production in PCa cell lines via EMT (128). The differences in the apoptotic mechanisms in PCa cells and their variant antioxidant system has been implicated in cisplatin resistance (129). PC3 cells cope with stress conditions via decreased p53 and Bax to become resistant to apoptotic regulatory mechanisms, cell cycle arrest and cytostatics (129). The receptor tyrosine kinase- EGFR when phosphorylated by Src in high ROS conditions has been shown to induce anoikis inhibition in PCa cells (76, 130, 131). In lung cancer, anoikis resistance could be overcome by NOX4 knockdown followed by reduced activation of Src and EGFR (124). Further studies establishing a strong link between ROS and anoikis resistance show that when PC3 cells are detached, there is an increase in the expression of leukotriene B4 receptor-2 (BLT2), causing a cascade of events- NOX activation, ROS generation, NF-κB activation downstream of BLT2. This BLT2-NOX-ROS-NF-κB axis thus plays a role in incurring anoikis resistance in PCa cells and serves as a novel therapeutic target to overcome resistance (132). ROS generating agent plumbagin overcomes anoikis resistance via the inhibition of NF-kB in resistant breast cancer cells (133). In mammary epithelial cells, a role of anti-oxidation agents has been implicated, in cancer cell survival and anchorage-independent colony formation (134). Androgen-independent human prostate cancer PC3 cells have increased antioxidant capacity via increased metallothionein expression and decreased pro-apoptotic genes thus resulting into cisplatin resistance (129). In sharp contrast, plumbagin is lethal to prostate cancer cells compared to cisplatin, possibly through ROS generation that overcomes anoikis resistance (135). Certain antioxidants may also act as tumor promoters via anoikis inhibition (28, 134). Thus, understanding the antioxidant profile at different stages of PCa may elucidate new mechanisms for overcoming anoikis resistance and improving therapeutic vulnerability in advanced tumors.
In the normal epithelium, cell detachment from the ECM is associated with impaired glucose transport, ATP deficiency followed by apoptosis. In metastatic cancer cells however, survival and anoikis resistance are consequences of high-energy generation through increased oncogene products (2, 124). Interestingly enough, there is evidence to suggest disruption of anoikis resistance and metabolic reprogramming as a therapeutic strategy for treatment of advanced PCa (76). Cell migration-inducing protein (CEMIP) plays a role in migration, invasion, pyruvate and lactate production, ATP level regulation as well as detachment-induced anoikis (2). CEMIP is overexpressed in PCa-AR cells and promotes tumor metastasis via metabolic reprograming (2). Furthermore, CEMIP causes anoikis resistance and increases metastatic potential of cells by inducing PKCa translocation via calcium leakage from the endoplasmic reticulum. The plasma membrane located PKCa induced protective autophagy through Bcl-2/Beclin complex dissociation causing survival of the ECM-detached cells (136). CEMIP is elevated in late stage PCa tissue as compared to precancerous tissue and its suppression can be used to impair EMT and metastasis by inducing anoikis (136).
In human mammary cells, upregulation of pyruvate dehydrogenase kinase 4 (PDK4) through estrogen-related receptor gamma causes reprogramming of glucose metabolism by reducing the conversion of glycolysis-derived pyruvate into acetyl CoA leading to anoikis resistance via decreased glucose oxidation (137, 138). Microarray analysis has revealed elevated PDK4 in human cancer cell lines including prostate (DU145), renal, ovarian and lung (137). PDK4 along with other metabolic markers such as PGK1 and glucose-6-phosphate dehydrogenase hold tremendous promise as glucose metabolic biomarkers in circulating tumor cells during PCa metastasis (139). Mitochondrial DNA alterations detected in PCa cells are linked to anoikis resistance via the PI3K/Akt signaling (140).
An increasing body of evidence has established the role of miRNAs in PCa tumorigenesis, anoikis/apoptosis avoidance and associated metastasis. miRNAs are interesting candidates not only as biomarkers of metastatic disease but also potential targets for therapeutic intervention. Anoikis resistance in PCa and bone metastasis has been shown to be a result of activation of the PI3K/Akt pathway and downregulation of miR-133a-3p (27, 141). Circular RNA (Circ_0004585) binds with miR-1248 and enhances PCa invasion, anoikis resistance and metastasis (142). Growth factor granulin is highly expressed in a variety of cancers and has pro-carcinogenic effects such as increased epithelial cell division and anoikis resistance (143–145). Granulin facilitates migration and anchorage-independent growth in androgen dependent and independent PCa cell lines (144, 146). miR107 has been shown to downregulate granulin expression in PCa cells, thus implicating this group of miRNA as therapeutic targets to overcome anoikis resistance (145). Elevated levels of miRNA (miR-16, miR-148a and miR-195) involved in the PI3K/Akt pathway, have been detected in the plasma of PCa patients (147). miR-4534, is overexpressed in PCa and functionally contributes to downregulation of Pten tumor suppressor (148). Furthermore, inhibition of MiR-186-5p also resulted into reduced anoikis resistance and survival in PC-3 and/or MDA-PCa-2b PCa cells via inhibition of PI3K/Akt signaling (149, 150).
Anoikis resistance contributes to progression of advanced metastatic PCa and emergence of therapeutic resistance. The ideal treatment modality for PCa would be to build a patient-specific profile that predicts the nature of individual tumors including metastatic potential using pathological grading along-with anoikis-related signatures to predict the treatment response of specific type of tumors. There is rapidly growing evidence on the mechanisms of anoikis and its translational significance during prostate cancer progression (as predictive biomarker anoikis-centered signatures, and therapeutic targeting platform for advanced metastatic tumors) and recurrent disease. However, there are significant gaps in our knowledge about exploiting the dynamics of EMT-MET within the TME. The phenotypic EMT and its interconversion to MET and consequential decreased resistance can be navigated by anoikis sensitizing agents to enable the development of new targeted therapeutics against lethal disease (Figure 1). Novel quinazoline compounds which disrupt FAK and their interactions with proteins such as Bit1 (only released after loss of integrin-ECM interactions) will help elucidate the complexities of anoikis resistance and identify novel therapeutic targets in caspase-independent apoptotic pathways (3, 13). While compounds such as DZ-50 are able to induce anoikis via EMT to MET cycling their potential use in combination therapy with taxanes to overcome chemotherapeutic resistance in PCa treatment remains a matter of further investigation. Bit1 expression in the TME versus in normal prostate cells and its regulation of anoikis in the prostate during normal cell physiology requires in depth assessment to harvest its therapeutic potential (151). The Bcl-2 family of proteins and their role in anoikis regulation/resistance for PCa treatment require further mechanistic insight to develop specific treatment strategies. Brain-derived neurotrophic factor (BDNF) is overexpressed in many human cancers including PCa. BDNF activation leads to the activation of cell survival pathways including PI3K/Akt, Jak/STAT, Erk and NF-kB (152). BDNF/tropomyosin receptor kinase B (TrkB) over expression in PCa is associated with increased EMT, cell migration, invasion and anoikis resistance (153). Downregulation of TrkB resulted into EMT reversal and anoikis induction (154). Antagonists of peroxisome proliferator-activated receptor-γ (belonging to the nuclear hormone receptor family), induce anoikis, disrupting the interaction of cancer cells with the ECM in squamous cell carcinoma and HCC (15, 155–157). The F-actin serving protein Cofilin that drives cell migration via cytoskeletal reorganization is involved in TGF-β mediated responses for progressing PCa metastasis. Functional loss of cofilin also decreases cancer cell adhesion and abolishes PCa cell invasion thus, evidence supporting (a) its role in anoikis and (b) targeting value in metastatic tumors (158).
There is a critically unmet need for a clinical tool to identify patients who can benefit from anoikis-based enhancement of therapeutic vulnerability and support clinical decision-making. Extracellular vesicles (EVs) (50-150 nm in diameter) serve as biological carriers for cargo including miRNA, proteins, lipids and anoikis effectors. Actinin-4, an exosomal protein is over-expressed in patients with CRPC or untreated metastatic prostate cancer. When the gene encoding this protein was knocked down, PCa cell growth and invasion were suppressed (159, 160). EVs isolated from the urine of prostate cancer patients have shown the presence of prostate cancer biomarkers, PCA-3 and TMPRSS2:ERG thus showing specific diagnostic and clinical value for the transcriptome within tumor EV (161). EVs carrying miRNA cargo in PCa contribute to anoikis induction via ECM degradation (162). Once prostate cancer specific, anoikis priming signatures are created, EVs with anoikis cargo that are present in body fluids such as blood, urine and saliva can be defined as predictive markers for PCa (163).
Conceptualization: PN, NK. Investigation: PN. Original draft preparation: PN. Writing and editing: PN, NK. Supervision: NK. All authors contributed to the article and approved the submitted version.
This work was supported by National Institutes of Health/NCI Grant number R01 CA232574 (NK).
The authors declare that the research was conducted in the absence of any commercial or financial relationships that could be construed as a potential conflict of interest.
All claims expressed in this article are solely those of the authors and do not necessarily represent those of their affiliated organizations, or those of the publisher, the editors and the reviewers. Any product that may be evaluated in this article, or claim that may be made by its manufacturer, is not guaranteed or endorsed by the publisher.
PCa, Prostate cancer; CRPC, Castration resistant prostate cancer; TME, Tumor microenvironment; ECM, Extracellular matrix; EMT, Epithelial to mesenchymal transition; MET, Mesenchymal to epithelial transition; TGF-β, Transforming growth factor-beta; RER, TGF-β receptor trap; ADT, Androgen-deprivation therapy; AR, Androgen receptor; DHT, Dihydrotestosterone; ARE, Androgen response elements; DISC, Death-inducing signaling complex; DED, Death effector domain; FADD, FAS-associated death domain; FLIP, FLICE-inhibitory protein; XIAP, X-linked inhibitor of apoptosis proteins; Zeb, Zinc finger E-box-binding homeobox 1; NF-kB, Nuclear factor kB; SFK, Src family kinase; FAK, Focal adhesion kinase; PROTACs, Proteolysis-targeting chimeras; TRAMP, Transgenic Adenocarcinoma Mouse Prostate; TMPRSS4, Transmembrane serine protease 4; CTGF, Connective tissue growth factor; EGFR, Epidermal growth factor receptor; YAP, Yes-associated protein; PDGF, Platelet derived growth factor; ROS, Reactive oxygen species; BLT2, leukotriene B4 receptor-2; CEMIP, Cell migration-inducing protein; PDK4, Pyruvate dehydrogenase kinase 4; BDNF, Brain-derived neurotrophic factor; EVs, Extracellular vesicles; LHRH, Luteinizing hormone-releasing hormone; HCC, Hepatocellular carcinoma.
1. Rennebeck G, Martelli M, Kyprianou N. Anoikis and survival connections in the tumor microenvironment: Is there a role in prostate cancer metastasis? Cancer Res (2005) 65(24):11230–5. doi: 10.1158/0008-5472.CAN-05-2763
2. Zhang P, Song Y, Sun Y, Li X, Chen L, Yang L, et al. AMPK/GSK3β/β-catenin cascade-triggered overexpression of CEMIP promotes migration and invasion in anoikis-resistant prostate cancer cells by enhancing metabolic reprogramming. FASEB J (2018) 32(7):3924–35. doi: 10.1096/fj.201701078R
3. Bilbro J, Mart M, Kyprianou N. Therapeutic value of quinazoline-based compounds in prostate cancer. Anticancer Res (2013) 33(11):4695–700.
4. Begemann D, Anastos H, Kyprianou N. Cell death under epithelial-mesenchymal transition control in prostate cancer therapeutic response. Int J Urol (2018) 25(4):318–26. doi: 10.1111/iju.13505
5. Meredith JE, Fazeli B, Schwartz MA. The extracellular matrix as a cell survival factor. Mol Biol Cell (1993) 4(9):953–61. doi: 10.1091/mbc.4.9.953
6. Frisch SM, Screaton RA. Anoikis mechanisms. Curr Opin Cell Biol (2001) 13(5):555–62. doi: 10.1016/S0955-0674(00)00251-9
7. McKenzie S, Kyprianou N. Apoptosis evasion: The role of survival pathways in prostate cancer progression and therapeutic resistance. J Cell Biochem (2006) 97(1):18–32. doi: 10.1002/jcb.20634
8. Johnstone RW, Ruefli AA, Lowe SW. Apoptosis: A link between cancer genetics and chemotherapy. Cell (2002) 108(2):153–64. doi: 10.1016/S0092-8674(02)00625-6
9. Frisch SM, Francis H. Disruption of epithelial cell-matrix interactions induces apoptosis. J Cell Biol (1994) 124(4):619–26. doi: 10.1083/jcb.124.4.619
10. Reddig PJ, Juliano RL. Clinging to life: Cell to matrix adhesion and cell survival. Cancer Metastasis Rev (2005) 24(3):425–39. doi: 10.1007/s10555-005-5134-3
11. Paoli P, Giannoni E, Chiarugi P. Anoikis molecular pathways and its role in cancer progression. Biochim Biophys Acta (BBA) - Mol Cell Res (2013) 1833(12):3481–98. doi: 10.1016/j.bbamcr.2013.06.026
12. Kim Y-N, Koo KH, Sung JY, Yun U-J, Kim H. Anoikis resistance: An essential prerequisite for tumor metastasis. Int J Cell Biol (2012) 2012:306879. doi: 10.1155/2012/306879
13. Yao X, Jennings S, Ireland SK, Pham T, Temple B, Davis M, et al. The anoikis effector Bit1 displays tumor suppressive function in lung cancer cells. PloS One (2014) 9(7):e101564. doi: 10.1371/journal.pone.0101564
14. Simpson CD, Anyiwe K, Schimmer AD. Anoikis resistance and tumor metastasis. Cancer Lett (2008) 272(2):177–85. doi: 10.1016/j.canlet.2008.05.029
15. Sakamoto S, Kyprianou N. Targeting anoikis resistance in prostate cancer metastasis. Mol Aspects Med (2010) 31(2):205–14. doi: 10.1016/j.mam.2010.02.001
16. Cao Z, Livas T, Kyprianou N. Anoikis and EMT: Lethal "Liaisons" during cancer progression. Crit Rev Oncog (2016) 21(3-4):155–68. doi: 10.1615/CritRevOncog.2016016955
17. Aoudjit F, Vuori K. Matrix attachment regulates fas-induced apoptosis in endothelial cells: a role for c-flip and implications for anoikis. J Cell Biol (2001) 152(3):633–43. doi: 10.1083/jcb.152.3.633
18. Taddei ML, Giannoni E, Fiaschi T, Chiarugi P. Anoikis: An emerging hallmark in health and diseases. J Pathol (2012) 226(2):380–93. doi: 10.1002/path.3000
19. Krajewska M, Krajewski S, Banares S, Huang X, Turner B, Bubendorf L, et al. Elevated expression of inhibitor of apoptosis proteins in prostate cancer. Clin Cancer Res (2003) 9(13):4914–25.
20. Berezovskaya O, Schimmer AD, Glinskii AB, Pinilla C, Hoffman RM, Reed JC, Glinsky GV. Increased expression of apoptosis inhibitor protein XIAP contributes to anoikis resistance of circulating human prostate cancer metastasis precursor cells. Cancer Res (2005) 65(6):2378–86. doi: 10.1158/0008-5472.CAN-04-2649
21. Bondar VM, McConkey DJ. Anoikis is regulated by BCL-2-independent pathways in human prostate carcinoma cells. Prostate (2002) 51(1):42–9. doi: 10.1002/pros.10070
22. Woods NT, Yamaguchi H, Lee FY, Bhalla KN, Wang HG. Anoikis, initiated by mcl-1 degradation and bim induction, is deregulated during oncogenesis. Cancer Res (2007) 67(22):10744–52. doi: 10.1158/0008-5472.CAN-07-3148
23. Jan Y, Matter M, Pai JT, Chen YL, Pilch J, Komatsu M, et al. A mitochondrial protein, Bit1, mediates apoptosis regulated by integrins and Groucho/TLE corepressors. Cell (2004) 116(5):751–62. doi: 10.1016/S0092-8674(04)00204-1
24. Karmali PP, Brunquell C, Tram H, Ireland SK, Ruoslahti E, Biliran H. Metastasis of tumor cells is enhanced by downregulation of Bit1. PloS One (2011) 6(8):e23840. doi: 10.1371/journal.pone.0023840
25. Grant CM, Kyprianou N. Epithelial mesenchymal transition (EMT) in prostate growth and tumor progression. Trans Andrology Urol (2013) 2(3):202–11. doi: 10.3978/j.issn.2223-4683.2013.09.04
26. Thiery JP. Epithelial–mesenchymal transitions in development and pathologies. Curr Opin Cell Biol (2003) 15(6):740–6. doi: 10.1016/j.ceb.2003.10.006
27. Adeshakin FO, Adeshakin AO, Afolabi LO, Yan D, Zhang G, Wan X. Mechanisms for modulating anoikis resistance in cancer and the relevance of metabolic reprogramming. Front Oncol (2021) 11. doi: 10.3389/fonc.2021.626577
28. Frisch SM, Schaller M, Cieply B. Mechanisms that link the oncogenic epithelial–mesenchymal transition to suppression of anoikis. J Cell Sci (2013) 126(1):21–9. doi: 10.1242/jcs.120907
29. Derksen PWB, Liu X, Saridin F, van der Gulden H, Zevenhoven J, Evers B, et al. Somatic inactivation of e-cadherin and p53 in mice leads to metastatic lobular mammary carcinoma through induction of anoikis resistance and angiogenesis. Cancer Cell (2006) 10(5):437–49. doi: 10.1016/j.ccr.2006.09.013
30. Onder TT, Gupta PB, Mani SA, Yang J, Lander ES, Weinberg RA. Loss of e-cadherin promotes metastasis via multiple downstream transcriptional pathways. Cancer Res (2008) 68(10):3645–54. doi: 10.1158/0008-5472.CAN-07-2938
31. Kumar S, Park SH, Cieply B, Schupp J, Killiam E, Zhang F, et al. A pathway for the control of anoikis sensitivity by e-cadherin and epithelial-to-Mesenchymal transition. Mol Cell Biol (2011) 31(19):4036–51. doi: 10.1128/MCB.01342-10
32. Cao Z, Kyprianou N. Mechanisms navigating the TGF-β pathway in prostate cancer. Asian J Urol (2015) 2(1):11–8. doi: 10.1016/j.ajur.2015.04.011
33. Zhang X, Fournier MV, Ware JL, Bissell MJ, Yacoub A, Zehner ZE. Inhibition of vimentin or beta1 integrin reverts morphology of prostate tumor cells grown in laminin-rich extracellular matrix gels and reduces tumor growth in vivo. Mol Cancer Ther (2009) 8(3):499–508. doi: 10.1158/1535-7163.MCT-08-0544
34. Usman S, Waseem NH, Nguyen TKN, et al. Vimentin is at the heart of epithelial mesenchymal transition (EMT) mediated metastasis. Cancers (Basel) (2021) 13(19):4985. doi: 10.3390/cancers13194985
35. Livas L, Hasani S, Kyprianou N. Integrated therapeutic targeting of the prostate tumor microenvironment. In: Birbrair A, editor. Tumor microenvironments in organs: From the brain to the skin – part b. Cham: Springer International Publishing (2020). p. 183–98.
36. Wei J, Xu G, Wu M, Zhang Y, Li Q, Liu P, et al. Overexpression of vimentin contributes to prostate cancer invasion and metastasis via src regulation. Anticancer Res (2008) 28(1A):327–34.
37. Burikhanov R, Sviripa VM, Hebbar N, Zhang W, Layton WJ, Hamza A, et al. Arylquins target vimentin to trigger par-4 secretion for tumor cell apoptosis. Nat Chem Biol (2014) 10(11):924–6. doi: 10.1038/nchembio.1631
38. Strouhalova K, Přechová M, Gandalovičová A, Brábek J, Gregor M, Rosel D. Vimentin intermediate filaments as potential target for cancer treatment. Cancers (Basel) (2020) 12(1):184. doi: 10.3390/cancers12010184
39. Kim Y-J, Choi W-I, Jeon B-N, Choi K-C, Kim K, Kim T-J, et al. Stereospecific effects of ginsenoside 20-Rg3 inhibits TGF-β1-induced epithelial–mesenchymal transition and suppresses lung cancer migration, invasion and anoikis resistance. Toxicology (2014) 322:23–33. doi: 10.1016/j.tox.2014.04.002
40. Wade CA, Kyprianou N. Profiling prostate cancer therapeutic resistance. Int J Mol Sci (2018) 19(3):904. doi: 10.3390/ijms19030904
41. Dupont J, Fernandez AM, Glackin CA, Helman L, LeRoith D. Insulin-like growth factor 1 (IGF-1)-induced twist expression is involved in the anti-apoptotic effects of the IGF-1 receptor*. J Biol Chem (2001) 276(28):26699–707. doi: 10.1074/jbc.M102664200
42. Šošić D, Richardson JA, Yu K, Ornitz DM, Olson EN. Twist regulates cytokine gene expression through a negative feedback loop that represses NF-kappaB activity. Cell (2003) 112(2):169–80. doi: 10.1016/s0092-8674(03)00002-3
43. Huynh LK, Hipolito CJ, Ten Dijke P. A perspective on the development of TGF-β inhibitors for cancer treatment. Biomolecules (2019) 9(11):743. doi: 10.3390/biom9110743
44. Gregory PA, Bracken CP, Smith E, Bert AG, Wright JA, Roslan MSM, et al. An autocrine TGF-beta/ZEB/miR-200 signaling network regulates establishment and maintenance of epithelial-mesenchymal transition. Mol Biol Cell (2011) 22(10):1686–98. doi: 10.1091/mbc.e11-02-0103
45. Pérez G, López‑Moncada F, Indo S, Torres MJ, Castellón EA, Contreras HR. Knockdown of ZEB1 reverses cancer stem cell properties in prostate cancer cells. Oncol Rep (2021) 45(5):58. doi: 10.3892/or.2021.8009
46. Orellana-Serradell O, Herrera D, Castellon EA, Contreras HR. The transcription factor ZEB1 promotes an aggressive phenotype in prostate cancer cell lines. Asian J Andrology (2018) 20(3):294–9. doi: 10.4103/aja.aja_61_17
47. Jakowlew SB. Transforming growth factor-beta in cancer and metastasis. Cancer Metastasis Rev (2006) 25(3):435–57. doi: 10.1007/s10555-006-9006-2
48. Jones E, Pu H, Kyprianou N. Targeting TGF-β in prostate cancer: Therapeutic possibilities during tumor progression. Expert Opin Ther Targets (2009) 13(2):227–34. doi: 10.1517/14728220802705696
49. Ao M, Williams K, Bhowmick NA, Hayward SW. Transforming growth factor-β promotes invasion in tumorigenic but not in nontumorigenic human prostatic epithelial cells. Cancer Res (2006) 66(16):8007–16. doi: 10.1158/0008-5472.CAN-05-4451
50. Roberts AB, Tian F, Byfield SD, Stuelten C, Ooshima A, Saika S, et al. Smad3 is key to TGF-β-mediated epithelial-to-mesenchymal transition, fibrosis, tumor suppression and metastasis. Cytokine Growth factor Rev (2006) 17(1-2):19–27. doi: 10.1016/j.cytogfr.2005.09.008
51. Zhang Q, Helfand BT, Jang TL, Zhu LJ, Chen L, Yang XJ, et al. Nuclear factor-κB-Mediated transforming growth factor-β-Induced expression of vimentin is an independent predictor of biochemical recurrence after radical prostatectomy. Clin Cancer Res (2009) 15(10):3557–67. doi: 10.1158/1078-0432.CCR-08-1656
52. Gudey SK, Sundar R, Heldin CH, Bergh A, Landström M. Pro-invasive properties of Snail1 are regulated by sumoylation in response to TGFβ stimulation in cancer. Oncotarget (2017) 8(58):97703–26. doi: 10.18632/oncotarget.20097
53. Qin T, Barron L, Xia L, Huang H, Villarreal MM, Zwaagstra J, et al. A novel highly potent trivalent TGF-β receptor trap inhibits early-stage tumorigenesis and tumor cell invasion in murine pten-deficient prostate glands. Oncotarget (2016) 7(52):86087–102. doi: 10.18632/oncotarget.13343
54. Kahn B, Collazo J, Kyprianou N. Androgen receptor as a driver of therapeutic resistance in advanced prostate cancer. Int J Biol Sci (2014) 10(6):588–95. doi: 10.7150/ijbs.8671
55. Siiteri PK, Wilson JD. Testosterone formation and metabolism during male sexual differentiation in the human embryo. J Clin Endocrinol Metab (1974) 38(1):113–25. doi: 10.1210/jcem-38-1-113
56. Heinlein CA, Chang C. The roles of androgen receptors and androgen-binding proteins in nongenomic androgen actions. Mol Endocrinol (2002) 16(10):2181–7. doi: 10.1210/me.2002-0070
57. Isaacs JT, Lundmo PI, Berges R, Martikainen P, Kyprianou N, English HF. Androgen regulation of programmed death of normal and malignant prostatic cells. J Andrology (1992) 13(6):457–64.
58. Sharifi N, Auchus RJ. Steroid biosynthesis and prostate cancer. Steroids (2012) 77(7):719–26. doi: 10.1016/j.steroids.2012.03.015
59. Dicken H, Hensley PJ, Kyprianou N. Prostate tumor neuroendocrine differentiation via EMT: The road less traveled. Asian J Urol (2019) 6(1):82–90. doi: 10.1016/j.ajur.2018.11.001
60. Zhu ML, Kyprianou N. Role of androgens and the androgen receptor in epithelial-mesenchymal transition and invasion of prostate cancer cells. FASEB J (2010) 24(3):769–77. doi: 10.1096/fj.09-136994
61. Sun Y, Wang BE, Leong KG, Yue P, Li L, Jhunjhunwala S, et al. Androgen deprivation causes epithelial-mesenchymal transition in the prostate: Implications for androgen-deprivation therapy. Cancer Res (2012) 72(2):527–36. doi: 10.1158/0008-5472.CAN-11-3004
62. Jennbacken K, Gustavsson H, Welén K, Vallbo C, Damber JE. Prostate cancer progression into androgen independency is associated with alterations in cell adhesion and invasivity. Prostate (2006) 66(15):1631–40. doi: 10.1002/pros.20469
63. Cai Q, Chen Y, Zhang D, Pan J, Xie Z, Ma S, et al. Loss of epithelial AR increase castration resistant stem-like prostate cancer cells and promotes cancer metastasis via TGF-β1/EMT pathway. Transl Androl Urol (2020) 9(3):1013–27. doi: 10.21037/tau.2020.03.02
64. Rosas E, Roberts J. T, O'Neill K. I, Christenson J. L, Williams M. M, Hanamura T, et al. A positive feedback loop between TGFβ and androgen receptor supports triple-negative breast cancer anoikis resistance. Endocrinology (2020) 162(2):bqaa226. doi: 10.1210/endocr/bqaa226
65. Rosas E, Richer J. The TGFβ and androgen receptor signaling pathways converge to support anoikis resistance in triple negative breast cancer. In: Frisch SM, editor. Anoikis: How the extracellular matrix regulates life-or-Death decisions. Cham: Springer International Publishing (2021). p. 173–92.
66. Bruckheimer EM, Kyprianou N. Dihydrotestosterone enhances transforming growth factor-β-Induced apoptosis in hormone-sensitive prostate cancer cells*. Endocrinology (2001) 142(6):2419–26. doi: 10.1210/endo.142.6.8218
67. Bruckheimer EM, Kyprianou N. Bcl-2 antagonizes the combined apoptotic effect of transforming growth factor-beta and dihydrotestosterone in prostate cancer cells. Prostate (2002) 53(2):133–42. doi: 10.1002/pros.10143
68. Martin SK, Pu H, Penticuff JC, Cao Z, Horbinski C, Kyprianou N. Multinucleation and mesenchymal-to-Epithelial transition alleviate resistance to combined cabazitaxel and antiandrogen therapy in advanced prostate cancer. Cancer Res (2016) 76(4):912–26. doi: 10.1158/0008-5472.CAN-15-2078
69. Ma W-L, Hsu C-L, Yeh C-C, Wu M-H, Huang C-K, Jeng L-B, et al. Hepatic androgen receptor suppresses hepatocellular carcinoma metastasis through modulation of cell migration and anoikis. Hepatology (2012) 56(1):176–85. doi: 10.1002/hep.25644
70. Toivanen R, Mohan A, Shen MM. Basal progenitors contribute to repair of the prostate epithelium following induced luminal anoikis. Stem Cell Rep (2016) 6(5):660–7. doi: 10.1016/j.stemcr.2016.03.007
71. Kwon O-J, Valdez JM, Zhang L, Zhang B, Wei X, Su Q, et al. Increased notch signalling inhibits anoikis and stimulates proliferation of prostate luminal epithelial cells. Nat Commun (2014) 5(1):4416. doi: 10.1038/ncomms5416
72. Lee Y, Yoon J, Ko D, Yu M, Lee S, Kim S. TMPRSS4 promotes cancer stem–like properties in prostate cancer cells through upregulation of SOX2 by SLUG and TWIST1. J Exp Clin Cancer Res (2021) 40(1):372. doi: 10.1186/s13046-021-02147-7
73. Kyjacova L, Hubackova S, Krejcikova K, Strauss R, Hanzlikova H, Dzijak R, et al. Radiotherapy-induced plasticity of prostate cancer mobilizes stem-like non-adherent, erk signaling-dependent cells. Cell Death Differentiation (2015) 22(6):898–911. doi: 10.1038/cdd.2014.97
74. Park SI, Zhang J, Phillips KA, Araujo JC, Najjar AM, Volgin AY, et al. Targeting SRC family kinases inhibits growth and lymph node metastases of prostate cancer in an orthotopic nude mouse model. Cancer Res (2008) 68(9):3323–33. doi: 10.1158/0008-5472.CAN-07-2997
75. Nam S, Kim D, Cheng JQ, Zhang S, Lee JH, Buettner R, et al. Action of the src family kinase inhibitor, dasatinib (BMS-354825), on human prostate cancer cells. Cancer Res (2005) 65(20):9185–9. doi: 10.1158/0008-5472.CAN-05-1731
76. Giannoni E, Fiaschi T, Ramponi G, Chiarugi P. Redox regulation of anoikis resistance of metastatic prostate cancer cells: Key role for src and EGFR-mediated pro-survival signals. Oncogene (2009) 28(20):2074–86. doi: 10.1038/onc.2009.77
77. Archer M, Dogra N, Kyprianou N. Inflammation as a driver of prostate cancer metastasis and therapeutic resistance. Cancers (2020) 12(10):2984. doi: 10.3390/cancers12102984
78. Yang F, Tuxhorn JA, Ressler SJ, McAlhany SJ, Dang TD, Rowley DR. Stromal expression of connective tissue growth factor promotes angiogenesis and prostate cancer tumorigenesis. Cancer Res (2005) 65(19):8887–95. doi: 10.1158/0008-5472.CAN-05-1702
79. Wells JE, Howlett M, Cole CH, Kees UR. Deregulated expression of connective tissue growth factor (CTGF/CCN2) is linked to poor outcome in human cancer. Int J Cancer (2015) 137(3):504–11. doi: 10.1002/ijc.28972
80. Gorlov IP, Sircar K, Zhao H, Maity SN, Navone NM, Gorlova OY, et al. Prioritizing genes associated with prostate cancer development. BMC Cancer (2010) 10(1):599. doi: 10.1186/1471-2407-10-599
81. Chang CC, Yang MH, Lin BR, Chen ST, Pan SH, Hsiao M, et al. CCN2 inhibits lung cancer metastasis through promoting DAPK-dependent anoikis and inducing EGFR degradation. Cell Death Differentiation (2013) 20(3):443–55. doi: 10.1038/cdd.2012.136
82. Frisch SM, Vuori K, Ruoslahti E, Chan-Hui PY. Control of adhesion-dependent cell survival by focal adhesion kinase. J Cell Biol (1996) 134(3):793–9. doi: 10.1083/jcb.134.3.793
83. Desiniotis A, Kyprianou N. Significance of talin in cancer progression and metastasis. Int Rev Cell Mol Biol (2011) 289:117–47. doi: 10.1016/B978-0-12-386039-2.00004-3
84. Goel HL, Li J, Kogan S, Languino LR. Integrins in prostate cancer progression. Endocr Relat Cancer (2008) 15(3):657–64. doi: 10.1677/ERC-08-0019
85. Lee Y-C, Jin J-K, Cheng C-J, Huang C-F, Song JH, Huang M, et al. Targeting constitutively activated β1 integrins inhibits prostate cancer metastasis. Mol Cancer Res (2013) 11(4):405–17. doi: 10.1158/1541-7786.MCR-12-0551
86. Zhang H, Fredericks T, Xiong G, Qi Y, Rychahou PG, Li J-D, et al. Membrane associated collagen XIII promotes cancer metastasis and enhances anoikis resistance. Breast Cancer Res (2018) 20(1):116. doi: 10.1186/s13058-018-1030-y
87. Broustas CG, Zhu A, Lieberman HB. Rad9 protein contributes to prostate tumor progression by promoting cell migration and anoikis resistance. J Biol Chem (2012) 287(49):41324–33. doi: 10.1074/jbc.M112.402784
88. Zheng D-Q, Woodard AS, Fornaro M, Tallini G, Languino LR. Prostatic carcinoma cell migration via αvβ3Integrin is modulated by a focal adhesion kinase Pathway1. Cancer Res (1999) 59(7):1655–64.
89. Sutherland M, Gordon A, Shnyder SD, Patterson LH, Sheldrake HM. RGD-binding integrins in prostate cancer: Expression patterns and therapeutic prospects against bone metastasis. Cancers (Basel) (2012) 4(4):1106–45. doi: 10.3390/cancers4041106
90. Paolillo M, Galiazzo MC, Daga A, Ciusani E, Serra M, Colombo L, et al. An RGD small-molecule integrin antagonist induces detachment-mediated anoikis in glioma cancer stem cells. Int J Oncol (2018) 53(6):2683–94. doi: 10.3892/ijo.2018.4583
91. Sakamoto S, McCann RO, Dhir R, Kyprianou N. Talin1 promotes tumor invasion and metastasis via focal adhesion signaling and anoikis resistance. Cancer Res (2010) 70(5):1885–95. doi: 10.1158/0008-5472.CAN-09-2833
92. Onyeisi JOS, Castanho de Almeida Pernambuco Filho P, de Araujo Lopes S, Nader HB, Lopes CC. Heparan sulfate proteoglycans as trastuzumab targets in anoikis-resistant endothelial cells. J Cell Biochem (2019) 120(8):13826–40. doi: 10.1002/jcb.28656
93. Elgundi Z, Papanicolaou M, Major G, Cox TR, Melrose J, Whitelock JM, et al. Cancer metastasis: The role of the extracellular matrix and the heparan sulfate proteoglycan perlecan. Front Oncol (2020) 9. doi: 10.3389/fonc.2019.01482
94. Yang F, Li X, Sharma M, Sasaki CY, Longo DL, Lim B, et al. Linking beta-catenin to androgen-signaling pathway. J Biol Chem (2002) 277(13):11336–44. doi: 10.1074/jbc.M111962200
95. Truica CI, Byers S, Gelmann EP. Beta-catenin affects androgen receptor transcriptional activity and ligand specificity. Cancer Res (2000) 60(17):4709–13.
96. Whitaker HC, Girling J, Warren AY, Leung H, Mills IG, Neal DE. Alterations in beta-catenin expression and localization in prostate cancer. Prostate (2008) 68(11):1196–205. doi: 10.1002/pros.20780
97. Kantarjian H, O'Brien S, Garcia-Manero G, Faderl S, Ravandi F, Jabbour E, et al. Very long-term follow-up results of imatinib mesylate therapy in chronic phase chronic myeloid leukemia after failure of interferon alpha therapy. Cancer (2012) 118(12):3116–22. doi: 10.1002/cncr.26568
98. Rao AS, Kremenevskaja N, von Wasielewski R, Jakubcakova V, Kant S, Resch J, et al. Wnt/β-catenin signaling mediates antineoplastic effects of imatinib mesylate (Gleevec) in anaplastic thyroid cancer. J Clin Endocrinol Metab (2006) 91(1):159–68. doi: 10.1210/jc.2005-1381
99. Zhao B, Li L, Wang L, Wang CY, Yu J, Guan KL. Cell detachment activates the hippo pathway via cytoskeleton reorganization to induce anoikis. Genes Dev (2012) 26(1):54–68. doi: 10.1101/gad.173435.111
100. Zhao B, Xie J, Zhou X, Zhang L, Cheng X, Liang C. YAP activation in melanoma contributes to anoikis resistance and metastasis. Exp Biol Med (Maywood) (2021) 246(8):888–96. doi: 10.1177/1535370220977101
101. Li Y, Yang S, Sadaoui NC, Hu W, Dasari SK, Mangala LS, et al. Sustained adrenergic activation of YAP1 induces anoikis resistance in cervical cancer cells. iScience (2020) 23(7):101289. doi: 10.1016/j.isci.2020.101289
102. Varzavand A, Hacker W, Ma D, Gibson-Corley K, Hawayek M, Tayh OJ, et al. α3β1 integrin suppresses prostate cancer metastasis via regulation of the hippo pathway. Cancer Res (2016) 76(22):6577–87. doi: 10.1158/0008-5472.CAN-16-1483
103. Bajaj GK, Zhang Z, Garrett-Mayer E, Drew R, Sinibaldi V, Pili R, et al. Phase II study of imatinib mesylate in patients with prostate cancer with evidence of biochemical relapse after definitive radical retropubic prostatectomy or radiotherapy. Urology (2007) 69(3):526–31. doi: 10.1016/j.urology.2006.12.006
104. Lin AM, Rini BI, Weinberg V, Fong K, Ryan CJ, Rosenberg JE, et al. A phase II trial of imatinib mesylate in patients with biochemical relapse of prostate cancer after definitive local therapy. BJU Int (2006) 98(4):763–9. doi: 10.1111/j.1464-410X.2006.06396.x
105. Keledjian K, Borkowski A, Kim G, Isaacs JT, Jacobs SC, Kyprianou N. Reduction of human prostate tumor vascularity by the alpha1-adrenoceptor antagonist terazosin. Prostate (2001) 48(2):71–8. doi: 10.1002/pros.1083
106. KIRBY RS, POOL JL. Alpha adrenoceptor blockade in the treatment of benign prostatic hyperplasia: past, present and future. Br J Urol (1997) 80(4):521–32. doi: 10.1046/j.1464-410X.1997.00247.x
107. Partin JV, Anglin IE, Kyprianou N. Quinazoline-based alpha 1-adrenoceptor antagonists induce prostate cancer cell apoptosis via TGF-beta signalling and I kappa b alpha induction. Br J Cancer (2003) 88(10):1615–21. doi: 10.1038/sj.bjc.6600961
108. Walden PD, Globina Y, Nieder A. Induction of anoikis by doxazosin in prostate cancer cells is associated with activation of caspase-3 and a reduction of focal adhesion kinase. Urol Res (2004) 32(4):261–5. doi: 10.1007/s00240-003-0365-7
109. Keledjian K, Kyprianou N. Anoikis induction by quinazoline based alpha 1-adrenoceptor antagonists in prostate cancer cells: antagonistic effect of bcl-2. J Urol (2003) 169(3):1150–6. doi: 10.1097/01.ju.0000042453.12079.77
110. Keledjian K, Garrison JB, Kyprianou N. Doxazosin inhibits human vascular endothelial cell adhesion, migration, and invasion. J Cell Biochem (2005) 94(2):374–88. doi: 10.1002/jcb.20240
111. Benning CM, Kyprianou N. Quinazoline-derived α1-adrenoceptor antagonists induce prostate cancer cell apoptosis via an α1-adrenoceptor-independent Action1. Cancer Res (2002) 62(2):597–602.
112. Garrison JB, Shaw YJ, Chen CS, Kyprianou N. Novel quinazoline-based compounds impair prostate tumorigenesis by targeting tumor vascularity. Cancer Res (2007) 67(23):11344–52. doi: 10.1158/0008-5472.CAN-07-1662
113. Hensley PJ, Desiniotis A, Wang C, Stromberg A, Chen CS, Kyprianou N. Novel pharmacologic targeting of tight junctions and focal adhesions in prostate cancer cells. PloS One (2014) 9(1):e86238. doi: 10.1371/journal.pone.0086238
114. Cao Z, Koochekpour S, Strup SE, Kyprianou N. Reversion of epithelial-mesenchymal transition by a novel agent DZ-50 via IGF binding protein-3 in prostate cancer cells. Oncotarget (2017) 8(45):78507–19. doi: 10.18632/oncotarget.19659
115. Hensley PJ, Cao Z, Pu H, Dicken H, He D, Zhou Z, et al. Predictive and targeting value of IGFBP-3 in therapeutically resistant prostate cancer. Am J Clin Exp Urol (2019) 7(3):188–202.
116. Guo Y, Kyprianou N. Restoration of transforming growth factor beta signaling pathway in human prostate cancer cells suppresses tumorigenicity via induction of caspase-1-mediated apoptosis. Cancer Res (1999) 59(6):1366–71.
117. Liu S, Ren J, ten Dijke P. Targeting TGFβ signal transduction for cancer therapy. Signal Transduction Targeted Ther (2021) 6(1):8. doi: 10.1038/s41392-020-00436-9
118. Paller C, Pu H, Begemann DE, Wade CA, Hensley PJ, Kyprianou N. TGF-β receptor I inhibitor enhances response to enzalutamide in a pre-clinical model of advanced prostate cancer. Prostate (2019) 79(1):31–43. doi: 10.1002/pros.23708
119. Zhu H, Gu X, Xia L, Zhou Y, Bouamar H, Yang J, et al. A novel TGFβ trap blocks chemotherapeutics-induced TGFβ1 signaling and enhances their anticancer activity in gynecologic cancers. Clin Cancer Res (2018) 24(12):2780–93. doi: 10.1158/1078-0432.CCR-17-3112
120. Guo J, Liu J, Wei W. Degrading proteins in animals: “PROTAC”tion goes in vivo. Cell Res (2019) 29(3):179–80. doi: 10.1038/s41422-019-0144-9
121. Mawji IA, Simpson CD, Gronda M, Williams MA, Hurren R, Henderson CJ, et al. A chemical screen identifies anisomycin as an anoikis sensitizer that functions by decreasing FLIP protein synthesis. Cancer Res (2007) 67(17):8307–15. doi: 10.1158/0008-5472.CAN-07-1687
122. Schimmer AD, Welsh K, Pinilla C, Wang Z, Krajewska M, Bonneau M-J, et al. Small-molecule antagonists of apoptosis suppressor XIAP exhibit broad antitumor activity. Cancer Cell (2004) 5(1):25–35. doi: 10.1016/S1535-6108(03)00332-5
123. Wang Z, Cuddy M, Samuel T, Welsh K, Schimmer A, Hanaii F, et al. Cellular, biochemical, and genetic analysis of mechanism of small molecule IAP inhibitors *. J Biol Chem (2004) 279(46):48168–76. doi: 10.1074/jbc.M405022200
124. Kim H, Sung JY, Park E-K, Kho S, Koo KH, Park S-Y, et al. Regulation of anoikis resistance by NADPH oxidase 4 and epidermal growth factor receptor. Br J Cancer (2017) 116(3):370–81. doi: 10.1038/bjc.2016.440
125. Giannoni E, Chiarugi P. Redox circuitries driving src regulation. Antioxid Redox Signal (2014) 20(13):2011–25. doi: 10.1089/ars.2013.5525
126. Buchheit CL, Rayavarapu RR, Schafer ZT. The regulation of cancer cell death and metabolism by extracellular matrix attachment. Semin Cell Dev Biol (2012) 23(4):402–11. doi: 10.1016/j.semcdb.2012.04.007
127. Szatrowski TP, Nathan CF. Production of large amounts of hydrogen peroxide by human tumor cells. Cancer Res (1991) 51(3):794–8.
128. Barnett P, Arnold RS, Mezencev R, Chung LWK, Zayzafoon M, Odero-Marah V. Snail-mediated regulation of reactive oxygen species in ARCaP human prostate cancer cells. Biochem Biophys Res Commun (2011) 404(1):34–9. doi: 10.1016/j.bbrc.2010.11.044
129. Gumulec J, Balvan J, Sztalmachova M, Raudenska M, Dvorakova V, Knopfova L, et al. Cisplatin-resistant prostate cancer model: Differences in antioxidant system, apoptosis and cell cycle. Int J Oncol (2014) 44(3):923–33. doi: 10.3892/ijo.2013.2223
130. Giannoni E, Buricchi F, Grimaldi G, Parri M, Cialdai F, Taddei ML, et al. Redox regulation of anoikis: Reactive oxygen species as essential mediators of cell survival. Cell Death Differ (2008) 15(5):867–78. doi: 10.1038/cdd.2008.3
131. Buchheit CL, Weigel KJ, Schafer ZT. Cancer cell survival during detachment from the ECM: Multiple barriers to tumour progression. Nat Rev Cancer (2014) 14(9):632–41. doi: 10.1038/nrc3789
132. Lee JW, Kim JH. Activation of the leukotriene B4 receptor 2-reactive oxygen species (BLT2-ROS) cascade following detachment confers anoikis resistance in prostate cancer cells. J Biol Chem (2013) 288(42):30054–63. doi: 10.1074/jbc.M113.481283
133. Howe EN, Cochrane DR, Cittelly DM, Richer JK. miR-200c targets a NF-κB up-regulated TrkB/NTF3 autocrine signaling loop to enhance anoikis sensitivity in triple negative breast cancer. PloS One (2012) 7(11):e49987. doi: 10.1371/journal.pone.0049987
134. Schafer ZT, Grassian AR, Song L, Jiang Z, Gerhart-Hines Z, Irie HY, et al. Antioxidant and oncogene rescue of metabolic defects caused by loss of matrix attachment. Nature (2009) 461(7260):109–13. doi: 10.1038/nature08268
135. Balvan J, Gumulec J, Raudenska M, Krizova A, Stepka P, Babula P, et al. Oxidative stress resistance in metastatic prostate cancer: Renewal by self-eating. PloS One (2015) 10(12):e0145016. doi: 10.1371/journal.pone.0145016
136. Yu Y, Liu B, Li X, Lu D, Yang L, Chen L, et al. ATF4/CEMIP/PKCα promotes anoikis resistance by enhancing protective autophagy in prostate cancer cells. Cell Death Dis (2022) 13(1):46. doi: 10.1038/s41419-021-04494-x
137. Kamarajugadda S, Stemboroski L, Cai Q, Simpson NE, Nayak S, Tan M, et al. Glucose oxidation modulates anoikis and tumor metastasis. Mol Cell Biol (2012) 32(10):1893–907. doi: 10.1128/MCB.06248-11
138. Bose S, Zhang C, Le A. Glucose metabolism in cancer: The warburg effect and beyond. In: Le A, editor. The heterogeneity of cancer metabolism. Cham: Springer International Publishing (2021). p. 3–15.
139. Chen J, Cao S, Situ B, Zhong J, Hu Y, Li S, et al. Metabolic reprogramming-based characterization of circulating tumor cells in prostate cancer. J Exp Clin Cancer Res (2018) 37(1):127. doi: 10.1186/s13046-018-0682-x
140. Moro L, Arbini AA, Yao JL, di Sant'Agnese PA, Marra E, Greco M. Mitochondrial DNA depletion in prostate epithelial cells promotes anoikis resistance and invasion through activation of PI3K/Akt2. Cell Death Differentiation (2009) 16(4):571–83. doi: 10.1038/cdd.2008.178
141. Tang Y, Pan J, Huang S, Peng X, Zou X, Luo Y, et al. Downregulation of miR-133a-3p promotes prostate cancer bone metastasis via activating PI3K/AKT signaling. J Exp Clin Cancer Res (2018) 37(1):160. doi: 10.1186/s13046-018-0813-4
142. Yu Y, Song Y, Cheng L, Chen L, Liu B, Lu D, et al. CircCEMIP promotes anoikis-resistance by enhancing protective autophagy in prostate cancer cells. J Exp Clin Cancer Res (2022) 41(1):188. doi: 10.1186/s13046-022-02381-7
143. He Z, Bateman A. Progranulin (granulin-epithelin precursor, PC-cell-derived growth factor, acrogranin) mediates tissue repair and tumorigenesis. J Mol Med (Berl) (2003) 81(10):600–12. doi: 10.1007/s00109-003-0474-3
144. Jackson BL, Grabowska A, Ratan HL. MicroRNA in prostate cancer: functional importance and potential as circulating biomarkers. BMC Cancer (2014) 14(1):930. doi: 10.1186/1471-2407-14-930
145. Wang W-X, Kyprianou N, Wang X, Nelson PT. Dysregulation of the mitogen granulin in human cancer through the miR-15/107 microRNA gene group. Cancer Res (2010) 70(22):9137–42. doi: 10.1158/0008-5472.CAN-10-1684
146. Monami G, Emiliozzi V, Bitto A, Lovat F, Xu SQ, Goldoni S, et al. Proepithelin regulates prostate cancer cell biology by promoting cell growth, migration, and anchorage-independent growth. Am J Pathol (2009) 174(3):1037–47. doi: 10.2353/ajpath.2009.080735
147. Al-Qatati A, Akrong C, Stevic I, Pantel K, Awe J, Saranchuk J, et al. Plasma microRNA signature is associated with risk stratification in prostate cancer patients. Int J Cancer (2017) 141(6):1231–9. doi: 10.1002/ijc.30815
148. Nip H, Dar AA, Saini S, Colden M, Varahram S, Chowdhary H, et al. Oncogenic microRNA-4534 regulates PTEN pathway in prostate cancer. Oncotarget (2016) 7(42):68371–84. doi: 10.18632/oncotarget.12031
149. Jones DZ, Schmidt ML, Suman S, Hobbing KR, Barve SS, Gobejishvili L, et al. Micro-RNA-186-5p inhibition attenuates proliferation, anchorage independent growth and invasion in metastatic prostate cancer cells. BMC Cancer (2018) 18(1):421. doi: 10.1186/s12885-018-4258-0
150. Lee HY, Son SW, Moeng S, Choi SY, Park JK. The role of noncoding RNAs in the regulation of anoikis and anchorage-independent growth in cancer. Int J Mol Sci (2021) 22(2):627. doi: 10.3390/ijms22020627
151. Jenning S, Pham T, Ireland SK, Ruoslahti E, Biliran H. Bit1 in anoikis resistance and tumor metastasis. Cancer Lett (2013) 333(2):147–51. doi: 10.1016/j.canlet.2013.01.043
152. Malekan M, Nezamabadi SS, Samami E, Mohebalizadeh M, Saghazadeh A, Rezaei N. BDNF and its signaling in cancer. J Cancer Res Clin Oncol (2022) 10.1007/s00432-022-04365-8. doi: 10.1007/s00432-022-04365-8
153. Li T, Yu Y, Song Y, Li X, Lan D, Zhang P, et al. Activation of BDNF/TrkB pathway promotes prostate cancer progression via induction of epithelial-mesenchymal transition and anoikis resistance. FASEB J (2020) 34(7):9087–101. doi: 10.1096/fj.201802159RRR
154. Tan X, Zhao L, Tang Y. The function of BDNF and its receptor in the Male genitourinary system and its potential clinical application. Curr Issues Mol Biol (2023) 45(1):110–21. doi: 10.3390/cimb45010008
155. Elrod HA, Sun SY. PPARgamma and apoptosis in cancer. PPAR Res 2008 (2008) p:704165. doi: 10.1155/2008/704165
156. Chi T, Wang M, Wang X, Yang K, Xie F, Liao Z, et al. PPAR-γ modulators as current and potential cancer treatments. Front Oncol (2021) 11:737776. doi: 10.3389/fonc.2021.737776
157. Schaefer KL, Wada K, Takahashi H, Matsuhashi N, Ohnishi S, Wolfe MM, et al. Peroxisome proliferator-activated receptor gamma inhibition prevents adhesion to the extracellular matrix and induces anoikis in hepatocellular carcinoma cells. Cancer Res (2005) 65(6):2251–9. doi: 10.1158/0008-5472.CAN-04-3037
158. Collazo J, Zhu B, Larkin S, Martin SK, Pu H, Horbinski C, et al. Cofilin drives cell-invasive and metastatic responses to TGF-β in prostate cancer. Cancer Res (2014) 74(8):2362–73. doi: 10.1158/0008-5472.CAN-13-3058
159. Gaglani S, Gonzalez-Kozlova E, Lundon DJ, Tewari AK, Dogra N, Kyprianou N. Exosomes as a next-generation diagnostic and therapeutic tool in prostate cancer. Int J Mol Sci (2021) 22(18):10131. doi: 10.3390/ijms221810131
160. Ishizuya Y, Uemura M, Narumi R, Tomiyama E, Koh Y, Matsushita M, et al. The role of actinin-4 (ACTN4) in exosomes as a potential novel therapeutic target in castration-resistant prostate cancer. Biochem Biophys Res Commun (2020) 523(3):588–94. doi: 10.1016/j.bbrc.2019.12.084
161. Nilsson J, Skog J, Nordstrand A, Baranov V, Mincheva-Nilsson L, Breakefield XO, et al. Prostate cancer-derived urine exosomes: A novel approach to biomarkers for prostate cancer. Br J Cancer (2009) 100(10):1603–7. doi: 10.1038/sj.bjc.6605058
162. Salimi L, Akbari A, Jabbari N, Mojarad B, Vahhabi A, Szafert S, et al. Synergies in exosomes and autophagy pathways for cellular homeostasis and metastasis of tumor cells. Cell Bioscience (2020) 10(1):64. doi: 10.1186/s13578-020-00426-y
Keywords: prostate cancer, anoikis, treatment resistance, EMT, metastasis, phenotypic reprogramming, extracellular matrix
Citation: Nepali PR and Kyprianou N (2023) Anoikis in phenotypic reprogramming of the prostate tumor microenvironment. Front. Endocrinol. 14:1160267. doi: 10.3389/fendo.2023.1160267
Received: 07 February 2023; Accepted: 09 March 2023;
Published: 05 April 2023.
Edited by:
Amina Zoubeidi, University of British Columbia, CanadaReviewed by:
Wenliang Li, University of Texas Health Science Center at Houston, United StatesCopyright © 2023 Nepali and Kyprianou. This is an open-access article distributed under the terms of the Creative Commons Attribution License (CC BY). The use, distribution or reproduction in other forums is permitted, provided the original author(s) and the copyright owner(s) are credited and that the original publication in this journal is cited, in accordance with accepted academic practice. No use, distribution or reproduction is permitted which does not comply with these terms.
*Correspondence: Natasha Kyprianou, TmF0YXNoYS5LeXByaWFub3VAbW91bnRzaW5haS5vcmc=
Disclaimer: All claims expressed in this article are solely those of the authors and do not necessarily represent those of their affiliated organizations, or those of the publisher, the editors and the reviewers. Any product that may be evaluated in this article or claim that may be made by its manufacturer is not guaranteed or endorsed by the publisher.
Research integrity at Frontiers
Learn more about the work of our research integrity team to safeguard the quality of each article we publish.