- 1Priority Research Centre for Reproductive Science, School of Environmental and Life Sciences, The University of Newcastle, Callaghan, NSW, Australia
- 2Infertility and Reproduction Research Program, Hunter Medical Research Institute, New Lambton Heights, NSW, Australia
Male infertility is a commonly encountered pathology that is estimated to be a contributory factor in approximately 50% of couples seeking recourse to assisted reproductive technologies. Upon clinical presentation, such males are commonly subjected to conventional diagnostic andrological practices that rely on descriptive criteria to define their fertility based on the number of morphologically normal, motile spermatozoa encountered within their ejaculate. Despite the virtual ubiquitous adoption of such diagnostic practices, they are not without their limitations and accordingly, there is now increasing awareness of the importance of assessing sperm quality in order to more accurately predict a male’s fertility status. This realization raises the important question of which characteristics signify a high-quality, fertilization competent sperm cell. In this review, we reflect on recent advances in our mechanistic understanding of sperm biology and function, which are contributing to a growing armory of innovative approaches to diagnose and treat male infertility. In particular we review progress toward the implementation of precision medicine; the robust clinical adoption of which in the setting of fertility, currently lags well behind that of other fields of medicine. Despite this, research shows that the application of advanced technology platforms such as whole exome sequencing and proteomic analyses hold considerable promise in optimizing outcomes for the management of male infertility by uncovering and expanding our inventory of candidate infertility biomarkers, as well as those associated with recurrent pregnancy loss. Similarly, the development of advanced imaging technologies in tandem with machine learning artificial intelligence are poised to disrupt the fertility care paradigm by advancing our understanding of the molecular and biological causes of infertility to provide novel avenues for future diagnostics and treatments.
1 Introduction
Infertility is a relatively common condition afflicting upwards of 15% of couples of reproductive age. Male factors are uniquely responsible for an estimated 30% of couples experiencing difficulty conceiving and beyond this, are a recognized contributor in approximately 50% of all cases of infertility (1). In the four decades since the report of the first successful human in vitro fertilization (IVF) (2), considerable gains have been made toward improving the effectiveness of male subfertility treatments. In particular, the advent of gamete micromanipulation techniques, such as intracytoplasmic sperm injection (ICSI), have reframed the field of assisted reproduction by effectively mitigating the need for the fertilizing spermatozoon to exhibit progressive motility, be capable of recognizing an oocyte or completing acrosomal exocytosis (3). Indeed, so profound has the impact of ICSI been in permitting men with defective sperm parameters to reproduce, that it has become the favored choice for fertilization irrespective of the underlying etiology (4). As such, ICSI now features in approximately two thirds of all cycles of assisted reproduction undertaken in countries such as Australia; well beyond that of its initial indication (5). Despite this strategy, worldwide clinical pregnancy and live birth rates resulting from this procedure have remained stubbornly modest at only 26.8% and 20% per initiated cycle, respectively (3). Notwithstanding confounders, recent epidemiological data has also raised concern regarding the prospect of an increased risk of birth defects and the propagation of substandard semen profiles in men conceived using ICSI compared to that of their naturally conceived peers (6, 7). Such findings affirm the need for cautious utilization of ICSI beyond its intended application for men with severely compromised semen parameters and present a strong case for exploring new diagnostic approaches for sperm selection, patient stratification, and therapeutic treatment options for sub/infertile males (8). In this review, we give consideration to current and future directions in male infertility research that are helping address the long-standing question of how to personalize and improve the clinical management of infertile patients.
2 Diagnostic andrology
Traditional approaches to diagnostic andrology are grounded in the principle that a male’s fertility can be assessed using routine descriptive criteria of the semen profile, with emphasis being placed on sperm count, sperm morphology and sperm motility parameters (Figure 1). Unfortunately, this conventional clinical strategy sheds little light on the underlying infertility etiology or the functional integrity of a patient’s spermatozoa and has thus proven to be of limited utility in predicting fertilization success (9). Indeed, despite iterative improvements in the semen assessment guidelines curated by the WHO (10–12), a substantial proportion of infertile men (~15%) still present with ‘normal’ semen profiles according to WHO criteria (13). Such cases of unexplained, or idiopathic, infertility have led us to appreciate that there are many different pathologies that contribute to male sub/infertility, each of which impose different clinical implications for patient management and help account for the relatively weak prognostic value of routine andrological assessment pipelines (14). Similarly, although advances have been made toward the automation of sperm morphology assessment via the coupling of ultra-high magnification with machine learning [i.e., ‘motile sperm organelle morphology examination’ (MSOME)], with the goal of standardizing the detection of morphological anomalies, it remains uncertain which elements of sperm structure define the functionality of this highly specialized cell. Thus, despite the potential of improving reproductive outcomes associated with using MSOME in tandem with ICSI (i.e., intracytoplasmic morphologically selected sperm injection; IMSI) (15), there remains insufficient evidence to support a positive effect of IMSI on either clinical pregnancy, live birth or miscarriage rates (16). While such pitfalls could theoretically be addressed by the application of selective stains to discriminate key features of sperm structure and quality (e.g., fluorophores that differentially label spermatozoa according to their viability, acrosomal status, capacitation status, mitochondrial membrane potential, generation of reactive oxygen species (ROS), peroxidation of membrane lipids, apoptosis and the integrity of their DNA), the use of such probes is currently prohibited in clinical practice; and will likely remain so until such time as the development of alternative stains that can achieve the non-destructive labeling of sperm structures in a manner that is either reversible or biologically inert.
As a descriptive stalwart of conventional semen profiling, the assessment of sperm motility offers some promise, especially when this assay is performed with objective computer aided semen analysis (CASA) systems that accurately measure the kinematics of swimming spermatozoa (17). It follows that positive correlations have been reported between the concentration of progressively motile spermatozoa and the outcome of human sperm-cervical mucus interaction tests (18) as well as overall fertility (19). Unfortunately, since the sperm motility profile is not static, such correlations are often weak. Indeed, under the influence of the differing physiological environments that spermatozoa encounter during their passage to the site of fertilization, they variably display forward progressive motility, complete quiescence (permissive of the formation of a storage reservoir in the isthmus of the fallopian tubes), and a characteristic high‐frequency high‐amplitude, asymmetric flagellar beat known as hyperactivated motility (20, 21). Whilst CASA measurements can discriminate these alternate forms of sperm motility (17), their intermittent nature limits their application as robust diagnostic criteria in a clinical setting. This phenomenon also likely contributes to the situation whereby the application of ‘swim up’ techniques to select motile spermatozoa have failed to deliver improved pregnancy rates compared to that of the most widely utilized colloidal silica density gradient preparation methods (22–24). With the intention of providing a more complete appraisal of sperm motility characteristics, new acquisition methods are in development that enable high-resolution reconstruction of three-dimensional sperm trajectories (i.e., via real-time tracking of the position of the whole flagellum in three-dimensional space), with some also featuring simultaneous analysis of the morphological characteristics of individual free swimming sperm cells (25–28). One such recently described application exploited a high-speed off-axis holographic system to map the three-dimensional refractive-index profile of the sperm head, in tandem with the dynamic flagellum localization during free swim (29). The four-dimensional reconstructed profile so generated enabled the specimen’s natural movement to be tracked together with detailed volumetric data on internal organelle structures (such as the nucleus housing the paternal genome); all without an attendant need for cell staining (29). Notwithstanding the considerable promise afforded by these new developments, both in the context of biological assays and clinical use, it remains uncertain whether they will be able to resolve the limitations associated with the continuum of motility profiles displayed by the fertilizing spermatozoon.
3 Strategies to improve diagnostic andrology
It is clear that there is a pressing need for new modalities of fertility diagnosis and the selection of spermatozoa for use in ART. In terms of addressing the limitations and variability inherent in current methodologies, we have much to learn from studying both the molecular basis of sperm dysfunction and equally, the features of functionally competent spermatozoa that can successfully ascend the female reproductive tract to reach the site of fertilization and complete the sequence of cellular interactions that precede natural conception (Figures 1, 2). This latter, highly selected subset of spermatozoa not only possess the requisite motility to gain entry into the oviducts but also the competence to engage in capacitation; a complex suite of biochemical and biophysical changes that prime spermatozoa for interaction with the cumulus oophorus and zona pellucida (ZP) surrounding the ovulated oocyte (30). These interactions are orchestrated by specialized membrane domains adorning the anterior sperm head (31, 32), which are formed during spermatogenesis before being extensively modified coincident with sperm transit of both the male and female reproductive tracts (33, 34). It therefore stands to reason that the development of sperm selection protocols that mimic the stringency of the barriers that fertilizing spermatozoa naturally encounter have obvious appeal (Figure 2).
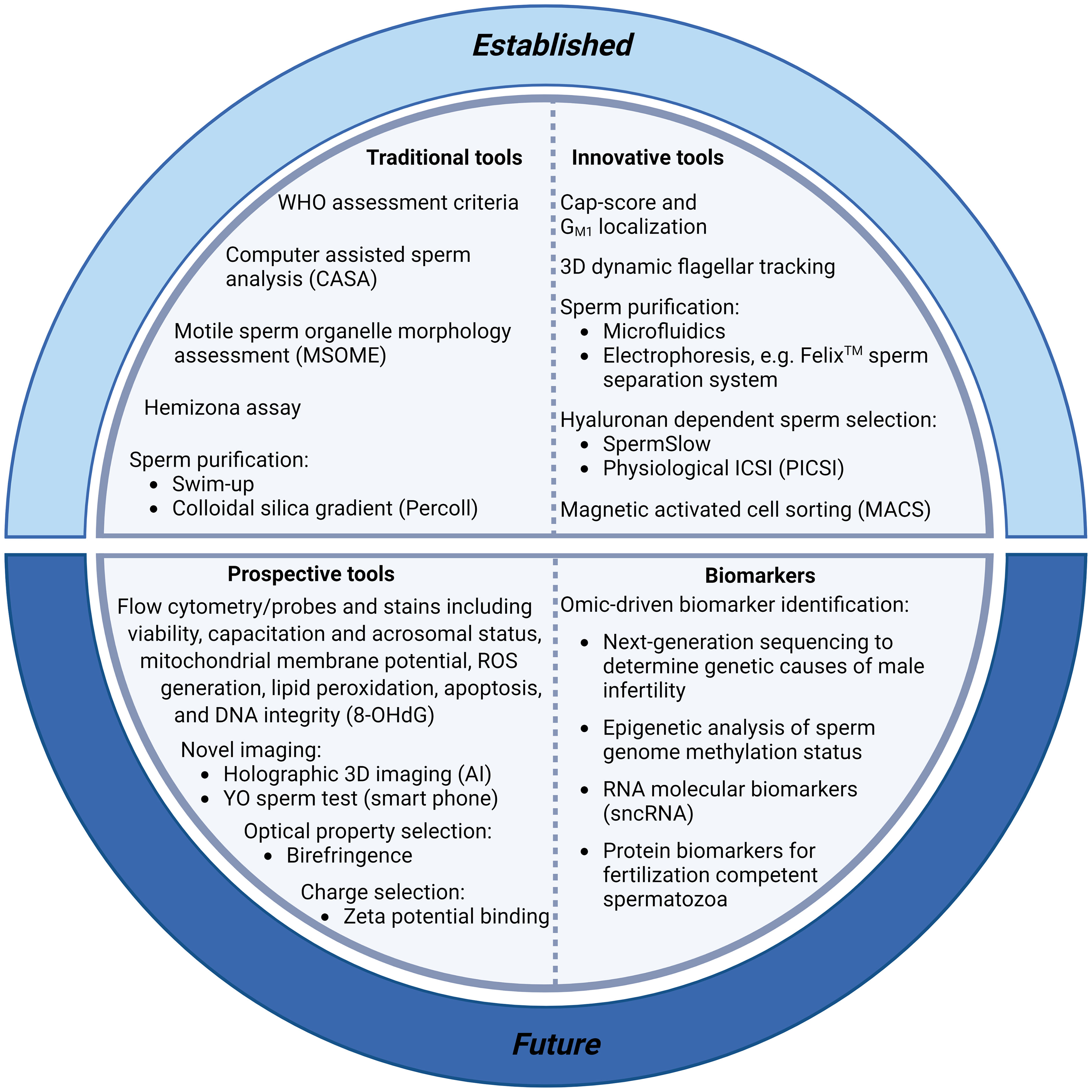
Figure 1 Summary of current and prospective tools for sperm selection and the clinical diagnosis of male infertility. Conventional protocols for the diagnosis of male infertility include assessment of sperm morphology, motility and concentration [including World Health Organisation (WHO) assessment criteria (35), computer assisted sperm analysis (CASA) (17), and motile sperm organelle morphology assessment (MSOME)] (16). These protocols are facilitated by the purification of sperm cells from seminal plasma with swim-up techniques or, more commonly, with density gradient centrifugation based on the use of colloidal silica suspensions. More innovative sperm selection strategies including microfluidics (36) and electrophoresis (Felix™; (37)) are also showing promise for use in clinical settings. Recent additions to the armoury of sperm selection tools include assays with the ability to determine DNA integrity and fertilization capacity [including the Cap-Score test (38), hyaluronan-based sperm immobilization methods (39), and high-throughput flow cytometry assays to detect DNA integrity [e.g., probes for the oxidized base 8-hydroxyl-2-deoxyguanosine (8-OHdG) (40)]. Further, smart phone technology is making semen analyses more accessible with at home YO sperm tests (41), and artificial intelligence (AI) advances have enabled new holographic 3D sperm imaging (28) and sperm quality assessment. Finally, technological advancements in omics platforms (34, 42) are permitting the identification of molecular biomarkers with which to stratify infertile patients, allowing enhanced evaluation of subfertility phenotypes, aiding our understanding of the genetic and epigenetic causes of male infertility, and potentially revolutionising fertility treatment with personalized therapeutic regimens.
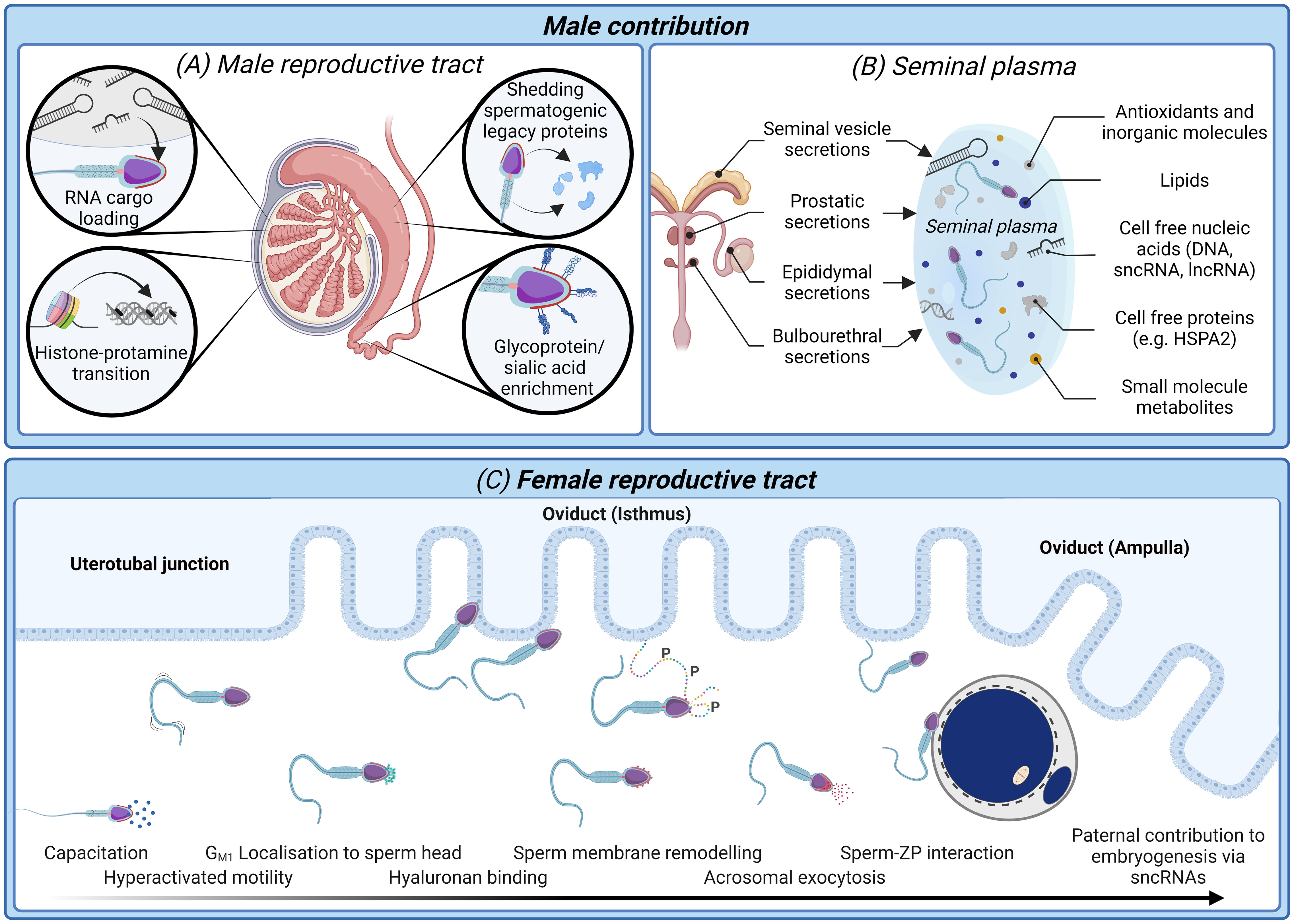
Figure 2 The biological processes and biomarkers of sperm production and functional maturation; an untapped resource for diagnostic tools of male infertility and sperm selection techniques. (A) In the male reproductive tract sperm nuclear compaction transitions from histone packaging to one characterized by predominantly protamines (43). Developing spermatozoa are simultaneously loaded with regulatory RNA cargo (42, 44) while also shedding excess cytoplasm and spermatogenic legacy proteins (34). Mature spermatozoa are identifiable upon completion of epididymal transit by an enrichment glycosylated surface proteins (34) and dynamic changes in their small non-coding RNAs (sncRNA) profile (45, 46). (B) Seminal plasma conveys not only spermatozoa to the female reproductive tract, but also several non-cellular components including antioxidants, lipids, and cell-free nucleic acids and proteins (47). Many of these non-cellular features offer high potential as biomarkers of a healthy, fertile human ejaculate. (C) After spermatozoa are deposited in the female reproductive tract they must successively negotiate the uterus and uterotubal junction before progressing through the oviduct isthmus and ampulla to contact the ovulated oocyte. Thereafter, spermatozoa must undergo a process termed capacitation, which is accompanied by dynamic protein phosphorylation events giving rise to altered patterns of motility (hyperactivated motility) and substantial membrane remodeling to facilitate the presentation/unmasking of the sperm surface receptors that orchestrate cumulus matrix (hyaluronan) and sperm-zona pellucida adhesion and penetration (30, 48). Finally, spermatozoa undergo an acrosome reaction leading to release of their acrosomal contents and remodeling of the sperm head architecture compatible with downstream gamete membrane fusion and fertilization (49). Beyond the delivery of the paternal genome, there is now compelling evidence that sperm convey epigenetic factors including sncRNAs to influence the trajectory of pre-implantation embryogenesis (50, 51).
3.1 Sperm-hyaluronic acid binding
Among an ever-expanding list of emerging sperm selection technologies designed to impose the physiological stringency of natural sperm selection barriers are protocols that exploit the hyaluronic acid (HA) binding properties of spermatozoa. These techniques harness the principle that functionally competent spermatozoa are endowed with HA binding sites that permit their adherence to, and digestion of, the HA-rich cumulus oophorus matrix (52). Two dominant protocols have been marketed under the umbrella of HA sperm selection, i.e., physiological intracytoplasmic sperm injection (PICSI; which features the use of HA immobilized on a solid support) and SpermSlow (which features HA suspended within a viscous medium). Such systems immobilize or retard sperm movement, theoretically enabling the positive selection of mature spermatozoa in preparation for ICSI (52). Notwithstanding the appeal and clinical interest in such interventions, current evidence appears insufficient to support their ability to improve either clinical pregnancy or live birth rates resulting from ART (53–55). Meta analyses have also concluded current evidence is inadequate to exclude adverse effects or differentiate the efficacy of SpermSlow versus PICSI HA binding systems (53). On the contrary, recent re-analysis of data from a parallel, two-group, randomized trial (i.e., HABSelect) has now reported that the use of PICSI is positively associated with a reduction in the rate of miscarriages, particularly in the subset of women aged over 35 (39, 55). Such an effect has been attributed to PICSI selection of spermatozoa with reduced levels of DNA damage.
In terms of offering a biological explanation for the lack of consensus regarding the clinical outcomes of sperm selection achieved using HA-based systems, there is now compelling evidence that the surface architecture of a human spermatozoon undergoes a substantial capacitation-associated remodeling in preparation of oocyte interaction (30, 33, 56–59). This event is characterized by a reduction in the proportion of sperm cells with surface exposed hyaluronidase enzymes [such as sperm adhesion molecule 1, (SPAM1)] (48, 60–62) and a reciprocal increase in those cells presenting ZP receptors on their surface (56). These receptors include arylsulfatase A (ARSA), an enzyme that is predominantly expressed on the surface of capacitated human spermatozoa where it displays affinity for the sulfated sugar ligands adorning the ZP (58, 62, 63). Importantly, recent work has demonstrated that sperm dysfunction may be due to the ubiquitination of ARSA on the sperm surface leading to an altered sperm surface glycosylation profile which, in turn, may negatively impact sperm-oocyte recognition (64). Thus, HA adhesion may favor the selection of the subset of spermatozoa that have yet to complete capacitation. However, whether this delay is reflective of underlying lesions in capacitation-associated signaling remains to be determined. Irrespective, the importance of capacitation as a predictive measure of fertility success is highlighted by recent developments such as the Cap-Score Sperm Function Test (65). As discussed below (please see Section 3.4.3: Sperm lipid biomarkers), this assay is formulated to assess sperm capacitation based on evaluation of the spatial distribution of the dominant sperm ganglioside, GM1.
3.2 Sperm-zona pellucida binding
After penetration of the HA-rich cumulus oophorus, spermatozoa encounter their final physiological barrier to fertilization in the form of the ZP (Figure 2); a glycoprotein matrix that envelops the ovulated oocyte (66). It is well established that an inability to bind and penetrate the ZP ranks among the most common defects recorded in the spermatozoa of infertile male patients (67). Thus, ~10% of men diagnosed with idiopathic infertility present with a failure of sperm-ZP adhesion (68). However, defects in sperm-ZP adhesion are commonly a matter of degree and upon quantification using techniques such as the hemizona assay (HZA), provide the highest discriminatory power for both in vitro and in vivo fertilization success of any sperm parameter (69). These findings have been taken as putative evidence that the ZP serves an important role in the selection of high quality spermatozoa (33, 66), a notion that resonates with multiple reports that the ZP preferentially binds spermatozoa that display superior motility, morphology, high levels of DNA integrity and lower global DNA methylation levels than that of their unbound counterparts (70–73). In accounting for this phenomenon, it is perhaps notable that each of these functional parameters are acutely sensitive to elevated levels of reactive oxygen species, the excessive production of which is a common etiology in the spermatozoa of infertile males (74). Indeed, work in our own laboratory has shown that molecular chaperone proteins (i.e., heat shock protein A2; HSPA2), which coordinate capacitation-associated membrane remodeling, are highly vulnerable to adduction by reactive lipid aldehydes [including 4-hydroxynonenal (4HNE)] formed as a consequence of oxidative-stress induced membrane lipid peroxidation (75–77). Such adductions disrupt HSPA2-client protein interactions and consequently limit the surface presentation of ZP receptors in capacitated spermatozoa (63).
As an extension of these findings, it has been shown that the use of ZP binding as a tool for selecting spermatozoa for downstream use in ICSI is associated with the production of higher quality embryos as well as improved implantation and clinical pregnancy rates compared to spermatozoa selected by conventional subjective criteria (71, 78, 79). Despite the biological importance of ZP binding, the advent of ICSI to bypass this physiological barrier has effectively diverted attention away from research into the molecular basis by which spermatozoa recognize and engage in productive interactions with the ZP (32). Nevertheless, the balance of clinical evidence as well as that compiled from animal studies indicates that this event likely involves contributions from both protein-protein and lectin-like interactions (80–84). Such observations raise the prospect of using ZP ligands to harvest superior quality, fertilization competent spermatozoa. Although the use of native ZP holds obvious appeal in this regard, ethical and resourcing limitations prohibit the use of this resource. Nevertheless, complex carbohydrate surrogates, such as fucoidan and neoglycoproteins decorated with the sialyl-Lewisx (sLex) sequence, have been identified that possess the ability to competitively inhibit human gamete interactions (81, 85). Similarly, agarose beads coated in recombinant human ZP2 peptides have shown promise in the selection of high-quality human spermatozoa (84). Taken together, these data support the tenant that specific carbohydrate and/or ZP peptide motifs can be harnessed as sperm selection strategies to enhance the success of downstream ART applications. Building on this principle, below we briefly discuss several other advanced sperm selection techniques that are in development based on exploiting the surface characteristics of functionally viable spermatozoa; including those that discriminate sperm on the basis of the degree of negative surface charge, exteriorization of apoptotic markers, and birefringence properties (Figures 1, 2). Notably, however, while several of these technologies have shown promise in a pre-clinical setting, additional high quality randomized evidence is required before advocating for their widespread clinical adoption (53, 54, 86).
3.3 Sperm surface characteristics
In common with their somatic cell counterparts, the surface of a human spermatozoon is furnished with a complex glycocalyx comprising a diversity of carbohydrates linked to membrane protein and lipid anchors (87, 88). Moreover, this acellular coat is substantially remodeled during the consecutive stages of sperm maturation, such that those spermatozoa that have successfully completed their epididymal maturation are characterized by an enrichment of glycoproteins with terminal sialic acid residues (89). This gradient of increasing sialylation is now a well-recognized hallmark of sperm maturity and one that has been associated with the protection of spermatozoa from immune surveillance within the female reproductive tract (89). However, beyond masking the allogeneic properties of the sperm cell, the accompanying increase in electronegativity brought about by sialyation has formed the basis of electrophoretic separation techniques designed to rapidly fractionate mature spermatozoa away from other deleterious ejaculate contaminates (i.e., immature germ cells, bacteria, and leucocytes) (90) (Figure 1). One such recent development, now marketed under the trademark of the Felix Sperm Separation System, has shown promise for the isolation of suspensions of viable, morphologically normal spermatozoa with high levels of total and progressive motility, and low levels of DNA damage (37, 90–93). Clinical compatibility has also been demonstrated with biopsied material, snap-frozen sperm suspensions and cryostored semen, and the first report of a human pregnancy and live birth using electrophoretically isolated spermatozoa (91). Alternate electrokinetic properties of mature spermatozoa including the ~ -16 to -20 mV charge differential across their plasma membrane (i.e. their zeta potential) have also been exploited as a means by which to capture these cells following their adherence to positively charged support matrices (94). Akin to electrophoretic separation methods, methods exploiting zeta potential have been reported to select mature sperm cells with enhanced levels of normal morphology, kinematic parameters and DNA integrity, and accordingly, have been associated with improved fertilization and pregnancy success following ART (95).
Among the leading non-genetic etiologies causally linked to male infertility is oxidative stress, a pathology that arises as a consequence of elevated levels of reactive oxygen species (ROS) within the male germline (74). Sperm cells are highly vulnerable to oxidative stress owing to an abundance of oxidizable substrates (including high levels of polyunsaturated fatty acids) combined with the fact that they possess limited capacity to protect themselves against oxidative attack or to enact repair once they sustain damage (74). Instead, spermatozoa revert to an apoptotic cascade upon the induction of oxidative stress, a situation that eliminates their fertilization potential and presumably promotes their phagocytosis without the propagation of an attendant inflammatory response within the female reproductive tract (96). One of the late hallmarks of the apoptotic sperm membrane is the externalization of phosphatidylserine resides, the detection of which forms the basis of negative selection protocols, including glass wool separation and magnetic activated cell sorting (MACS)-annexin V technologies, designed to remove senescent spermatozoa from within an ejaculate prior to ART (53, 54, 97–100). One potential flaw in this otherwise laudable approach is that the superficial exposure of phosphatidylserine has recently been reported in the head region of viable and motile mouse spermatozoa (101). Indeed, the surface presentation of phosphatidylserine is reported to progressively increase during sperm transit through the epididymis and again upon the capacitation of these cells. Moreover, the masking of sperm surface phosphatidylserine residues potently inhibited fertilization, thus identifying this ligand as a potential key player in sperm-oocyte fusion (101). Such findings raise the prospect that the loss of phospholipid asymmetry within the sperm plasma membrane is not simply a signature of apoptotic cells but rather one associated with the level of sperm functional maturity. As an important caveat however, it remains to be determined whether this model also holds true in the case of human spermatozoa. It is thus premature to conclude whether this is an extenuating factor that has limited the clinical utility of MACS-annexin V selection protocols (53, 54).
Apart from measurable differences in the macromolecular composition of the sperm surface that herald changes in their functional status, maturing sperm cells are also characterized by changes in their birefringence optical properties. Birefringence is a phenomenon exhibited by certain materials in which an incoming (incident) ray of light is split by polarization into two rays each assuming slightly different paths and travelling at different velocities. The extent of this so called ‘bi’refraction (i.e., birefringence) is determined by the non-uniform spatial (i.e., anisotropic) properties of the material; which in the case of human spermatozoa, originate from a combination of longitudinally orientated protein filaments residing within the subacrosomal, nuclear, and axonemal domains, each of which refract light differently (102–104). Accordingly, the use of polarized light microscopy has drawn attention as a diagnostic tool with which to evaluate the structural integrity of spermatozoa with applications extending to the selection of viable cells with normal morphology and low levels of DNA damage in preparation for ICSI (105, 106). However, the clinical utility of this technology has yet to be investigated in randomized trials (53).
3.4 Sperm biomarkers
As discussed above, there are numerous inherent limitations associated with existing strategies for sperm selection and defining male fertility. These limitations have, in turn, fueled renewed interest in the identification of non-invasive biomarkers that accurately predict fertilization outcome and provide molecular insight into underlying infertility pathophysiology (107). Such approaches have been aided by rapid technological advances in analytical ‘omic’ platforms leading to a recent proliferation in studies seeking to define the macromolecular signatures (e.g. lipidomic, metabolomic, proteomic, and epigenomic) of semen and spermatozoa from fertile males versus those produced by subfertile/infertile men (57, 108–112).
3.4.1 Sperm protein biomarkers
Due to their inability to engage in de novo protein synthesis, the functional maturation of human spermatozoa is reliant on changes associated with two phases of post-testicular maturation, namely: (i) the incorporation of new proteins encountered during their descent through the male reproductive tract (epididymis), and (ii) the processing and/or post-translational modification of their intrinsic proteome that occurs during post-ejaculatory capacitation (33, 34, 57) (Figure 2). These characteristics highlight the importance of proteomic tools for studying the molecular changes that drive the acquisition of sperm functionality (57, 110, 111). It follows that considerable research effort has been directed to cataloging the sperm proteome in addition to the seminal fluid and innumerable extracellular vesicles in which they are bathed (108, 109, 111, 113–116). In the context of spermatozoa, this growing proteomic resource has been assembled into a comprehensive reference library of 6,198 proteins; an inventory that accounts for ~80% of the estimated 7,500 proteins that are represented in human spermatozoa (117). Notably, recent work from our own laboratory has demonstrated that alongside proteins that are either gained and/or modified during post-testicular sperm maturation, the shedding of proteins also constitutes a major part of the proteomic changes that contribute to producing a fertilization competent sperm cell (34). Such proteins, which are underrepresented in the mature spermatozoon, have been shown to map to several infertility phenotypes and could thus represent negative selection markers to potentially identify poor quality spermatozoa. Amid the remaining challenges to realizing the transformative impact of this information are the investigation of protein interaction networks, characterization of those proteins whose function is influenced by post-translational modifications (e.g. phosphorylation, proteolytic cleavage, acetylation, glycosylation), and defining anomalies in protein abundance that signify specific defects in sperm function (57, 109).
Advances are being made toward these goals through the application of advanced mass spectrometry technology platforms to deliver new insight into the proteomic features of spermatozoa in different functional states (i.e., fertile vs. infertile, immature vs. mature, and non-capacitated vs. capacitated) (34, 61, 118–122). Such strategies are helping to shed light on specific proteomic elements that are of functional significance and facilitating improved interpretation of the spectrum of post-translational modifications necessary for generating a fertilization competent spermatozoon (57). Illustrative of this potential, mass spectrometry based proteomic analyses have been used to identify specific defects in human sperm cells associated with their failure of ZP recognition (61). Among the subset of proteins significantly under-represented in the spermatozoa of infertile patients, this study identified the molecular chaperone, HSPA2 (61). Such unbiased findings align with independent evidence that the overall abundance of HSPA2 in human spermatozoa provides a robust discriminative index of their fertilizing potential (52). By way of a biological explanation, HSPA2 appears to be a critical component of the machinery that orchestrates sperm plasma membrane remodeling events during spermiogenesis and capacitation (56, 59, 62, 123–125). Thus, an under-representation of HSPA2 likely has functional consequences in terms of the functional priming of ZP binding domains on the mature sperm surface. Moreover, the under-representation of HSPA2 within the sperm proteome has been causally linked to oxidative stress (63), thus reinforcing the concept that the fidelity of sperm-ZP interactions can be used to sensitively monitor the legacy of this pathophysiological challenge.
Aside from HSPA2, a subset of other sperm proteins have been identified as targets for damage brought about by the formation of oxidative 4HNE adducts (126–128), including A-kinase anchor protein 4 (AKAP4); a sperm-specific protein that localizes to the fibrous sheath of the flagellum, where it fulfils indispensable roles in spermatogenesis and subsequently in the support of sperm motility, capacitation-associated signaling and chemotaxis (129, 130). Data from our own laboratory have shown that both the AKAP4 protein and its precursor (proAKAP4), are targeted for 4HNE adduction in primary cultures of round spermatids and in mature mouse and human spermatozoa (127). We further demonstrated that exogenous 4HNE challenge of round spermatids and spermatozoa leads to a significant reduction in the detectable levels of both proAKAP4 and AKAP4 and a concomitant compromise of capacitation-associated phosphotyrosine expression in human spermatozoa; the latter putatively being caused dysregulation of the signaling network assembled around the AKAP4 scaffold. Such data affirm the utility of proAKAP4/AKAP4 as markers of sperm function and identify the measurement of proAKAP4/AKAP4 abundance as a promising approach to evaluate semen quality in male infertility disorders (131–133).
3.4.2 Sperm nucleic acid biomarkers
In addition to sperm proteins, alternative macromolecular features such as the sperm RNA signature and the integrity of the paternal genome have also attracted attention as prospective markers of fertilization success (134, 135). With regard to the latter, it has long been of interest to understand the principles, and biological consequences, associated with the packaging of approximately two meters of DNA into the sperm nucleus to create a structure far more compact than that of its somatic cell counterparts. It is now understood that this remarkable feat is accomplished by substantial remodeling of the chromatin during spermiogenesis linked to replacement of the majority of DNA packaging histones with protamines; effectively reducing the paternal DNA to a quasi-crystalline state approaching the physical limits of compaction (136) (Figure 2). In addition to streamlining the morphological profile of the spermatozoon, this strategy has attendant consequences in terms of reducing the exposure of the paternal genome to damaging agents yet effectively silencing transcription such that human spermatozoa possess limited capacity to enact DNA repair if they sustain damage to the paternal genome (137, 138). When attacks to the paternal genome do occur, they are commonly oxidatively induced as evidenced by the formation of the 8-hydroxy-2’-deoxyguanosine (8OHdG) DNA base adduct (139–141). Notably, such lesions are not randomly dispersed across the genome in mature spermatozoa but rather are targeted, with chromosome 15 being particularly susceptible to oxidative stress (142); a phenomenon potentially linked to its position within the nuclear matrix (143). Moreover, areas of vulnerability to oxidative attack are commonly associated with inter-linker regions (142); short (<1000 bp) non-protein bound segments interspersed between protamine-bound DNA toroids (43, 144, 145) and retained histone-bound DNA units that affix DNA to the nuclear matrix (146–148). Notably, these regions of vulnerability are of clinical relevance owing to the fact that they have been shown to harbor oxidative damage in the spermatozoa of infertile male patients (142). It follows that such defects (in addition to those associated with genetic mutations, exposure to environmental agents and disruption of chromatin proteins) have been causally linked to natural reproductive failures including reductions in fertilization rates, pregnancy rates, embryo quality, and increased rates of spontaneous abortion (149–151). However, it remains uncertain to what extent the evasion of natural conception barriers by directly injecting spermatozoa with damaged DNA into an oocyte, accounts for the increased risk of birth defects associated with assisted conception technologies such as ICSI (7, 152–154). Moreover, the retention of histones in approximately 4-15% of the sperm nuclear genome, contributes to a scenario in which epigenetic marks can endure reprogramming events (138, 155) thereby enabling transcriptional memory with potential implications extending across generations (156).
Accordingly, a battery of diagnostic assays have been brought to market that evaluate sperm DNA fragmentation such as the sperm chromatin structure assay (SCSA) (157), the single cell gel electrophoresis (Comet) assay (158), the terminal deoxynucleotidyl transferase mediated deoxyuridine triphosphate nick end labeling (TUNEL) assay (159), the chromomycin A3 test (160), in situ nick translation (161), the sperm chromatin dispersion (SCD) test (162, 163), and protamine ratio analysis (164). Regrettably, none of these report on the epigenetic state of the genome, and similarly, current evidence linking sperm DNA damage with outcomes of ART interventions is controversial. Thus, although some literature reports a strong negative association between sperm DNA fragmentation and pregnancy outcomes to justify the incorporation of sperm DNA testing into routine clinical tests (165, 166), other systematic reviews and meta-analyses suggest that our current armory of DNA damage assays have limited ability to predict pregnancy outcomes in the context of ART (167, 168). Thus, despite the potential benefits, there remains insufficient impetus to foster the routine application of sperm DNA damage testing in the management of couples seeking recourse to ART. We do, however, advocate for the continuation of high-quality research into the predictive value of sperm DNA fragmentation assays for both the success of pregnancy and for the choice of ART treatment.
Beyond the implications of sperm DNA damage and histone modifications, several classes of regulatory RNA species have also been implicated in epigenetic inheritance, including multiple sub-types of sperm-borne small non-coding RNAs (sncRNAs). Indeed, despite their transcriptionally and translationally inert state, it is well documented that mature spermatozoa convey a heterogenous cargo of RNA transcripts, including, mRNA, long non-coding RNA (lncRNA), and sncRNA (169). Moreover, a subset of the latter have been linked with regulation of the trajectory of pre-implantation embryo development and consequently, influencing the lifetime health of offspring (170). Thus, rather than being insignificant vestiges of spermatogenesis, sperm-borne sncRNAs are gaining attention for their potential prognostic value in evaluating sperm quality linked to male infertility (169). Growing interest has also focused on sperm-borne sncRNAs as diagnostic markers of a male’s exposure to environmental stressors and the fidelity of sperm production (134, 169). In this regard, mounting evidence has drawn links between an array of environmental stressors (e.g. dietary challenges, stress hormone administration, imposition of psychological stress, and exposure to environmental pollutants such as cigarette smoke) and pronounced alterations in the sperm sncRNA profile of exposed males (51, 171, 172). Of concern is that each of these changes can potentially contribute to sperm dysfunction and may account, at least in part, for the observation that the sperm sncRNA signature of idiopathic infertile males is evidently different from that of fertile individuals (169, 173–176). Notwithstanding the interest these studies have generated, the distinctive features of sperm biology pose several hurdles to utilizing RNA cargo for diagnostic purposes. Key among these are the low yield and the highly degraded nature of sperm RNA, yet it is hoped that these limitations will, in time, be circumvented by continued optimization of RNA isolation protocols (177). Such advances are necessary to realize the translational potential of data collected from pre-clinical animal studies and ensure the reproducibility of clinical assessments of male fertility using RNA biomarkers. Ultimately, the cataloging of human sperm RNA, similar to that achieved for the sperm proteome, may herald new opportunities to assess the effects of environmental, physical and chemical factors on semen quality with a level of unparalleled sensitivity.
3.4.3 Sperm lipid biomarkers
As integral components of the sperm surface, the composition, orientation and distribution of membrane lipids have also attracted attention as diagnostic biomarkers of male fertility. Illustrative of this potential are the MACs sperm selection technologies described previously, which exploit the externalization of phosphatidylserine in moribund cells (discussed above). However, recent developments have also seen a renewed focus on alternative lipids such as monosialotetrahexosylganglioside (GM1), which features as the target of assays marketed under the trademark of the Cap-Score Sperm Function Test (178, 179). In principle, Cap-Scores provide an indication of the percentage of capacitated spermatozoa within a given sample as determined by the localization of GM1; an integral component of sperm membrane rafts and one that becomes progressively concentrated within the vicinity of the anterior domain of the sperm head as capacitation proceeds (178, 180, 181). It follows that the re-localization of GM1 differs in the spermatozoa of cohorts of fertile and potentially infertile men, giving credence to the utility of Cap-Score. Indeed, Cap-Score has shown promise in predicting the success, or failure, of intrauterine insemination (IUI) in both retrospective (178) and prospective contexts (38, 65). Notably, Cap-Scores appear highly reproducible among ejaculates from a single individual and reliably identify those cells competent of completing an acrosome reaction induced by either an ionophore (179) or progesterone challenge (182); yet they have limited relationship with conventional semen analysis criteria (178). These data identify the utility of the Cap-Score as a predictive measure of male fertility and, if borne out across larger cohort studies, a strategy with important clinical applications in patient stratification. For a comprehensive summary of lipids that may be further developed as biomarkers of male infertility see (112, 183).
3.5 Seminal plasma biomarkers
Beyond the sperm component of the ejaculate, the seminal plasma in which they are bathed is also recognized as comprising a rich variety of biomolecules; many of which could potentially serve as non-invasive biomarkers of a male’s fertility status [e.g. proteins (125, 184, 185), small-molecule metabolites (186), lipids (187), cell-free nucleic acid (DNA, sncRNA and lncRNA) (188–191), as well as antioxidant agents and inorganic chemicals (ions) (192)]. Indeed, seminal plasma is an elaborate nutrient-rich fluid generated by the accessory glands of the male reproductive tract, which constitutes as much as 95% of human semen (193). Contrary to the long-held belief that seminal plasma acts solely as a transport medium for spermatozoa, a compelling body of data now supports key physiological roles in the promotion of early pregnancy success (47, 194). Such functions are attributed to direct communication with the female reproductive tract at insemination, effectively modulating cellular, molecular and immunological adaptions of the uterine environment to accommodate the semi-allogeneic conceptus and optimize pregnancy outcomes (47, 194). While it is apparent that human conception can proceed in the absence of seminal plasma, emerging clinical evidence (195–197) in tandem with data arising from pre-clinical animal models (198–201) suggests that an absence of seminal plasma exposure at conception results in suboptimal embryonic development and placentation; changes that can, in turn, manifest in pathological consequences for offspring. Such findings have prompted mounting interest in the active signaling factors present within seminal fluid (47) as well as the susceptibility of these factors to paternal stressors (202–204). Moreover, beyond those molecules with putative roles in modulating the female response, it is emerging that the components of seminal plasma may bear witness to the legacy of defective sperm production. Illustrative of this potential is HSPA2, a molecular chaperone protein previously discussed in relation to its role in the capacitation associated remodeling of the sperm surface, and one that is intimately associated with spermatogenesis. Recent work has also documented the presence of sperm-free HSPA2 in seminal plasma wherein its relative abundance is positively correlated with spermatogenic status (125). It follows that sub-classes of infertile males, such as cryptozoospermic patients, have low to non-detectable seminal plasma HSPA2; likely reflective of an almost total meiosis arrest. Additional work toward characterizing the molecular composition of seminal plasma and its relationship with a male’s fertility status is certainly warranted to help realize the potential of this easily accessible suspension as a source of biomarkers capable of predicting the potential success or failure of ART procedures.
4 Implications for therapeutic interventions
With ongoing limitations associated with the accurate diagnosis of male infertility, it is not surprising that a majority of the therapeutic interventions that have been clinically successful have been targeted to the treatment of pathologies with an obvious phenotype. Examples of these successful strategies lie in the treatment of varicocele through surgical means (205), the use of aromatase inhibitors in patients with abnormal testosterone-to-estradiol ratios (206), and extensive developments in ART procedures, such as ICSI (3), that have assisted patients with poor semen parameters, or those with an absence of sperm in the ejaculate through a combination of testicular sperm extraction (TESE) and ICSI.
In the case of varicocele, although the prevalence of the condition remains high at up to 20% of the male population, clinical varicocele now ranks as the most common correctable cause of male infertility (205). Varicocele repair is aimed at the dilation of the pampiniform plexus, a venous network located in the spermatic cord, to relieve the causative retrograde blood flow through the internal spermatic vein. In current practice, surgical approaches to repair varicocele, including ligation and resection of the dilated vessels by open surgery or microsurgery, are preferred. Further, the ability to tailor interventions to the grade and condition of each patient has led to a large array of treatment options (205). These innovations have had a significant impact on the recovery of fertility in patients that have undergone varicocelectomy. However, complications due to the inflammation and testicular heating paradigms associated with this condition have led to the suggestion that varicocele may be a progressive pathological condition, with implications extending to both structural and functional damage within the testis (207). Particularly concerning is that even in varicocele patients with normal semen parameters, or those with improved fertility post-surgery, the condition is commonly accompanied by an increased prevalence of sperm DNA fragmentation (208). Thus, issues associated with reduced paternal DNA integrity may persist in embryos generated through either natural conception or through ART interventions after varicocelectomy.
Although the nature of the sperm DNA damage experienced in varicocele patients is not entirely understood, one hypothesis is that excessive production of ROS in varicocele testes may contribute oxidative DNA lesions in spermatozoa and/or damaged chromatin in developing germ cells (205). One option for the management of these patients, pre- and post-surgery, is the administration of oral antioxidants to reduce the presence of ROS. While there is obvious theoretical appeal to this strategy and sound evidence from recent animal models that report the efficacy of targeted antioxidant strategies to reduce DNA damage (209), the use of oral antioxidants in a clinical setting has been met with mixed success. Recent reviews have highlighted just five oral antioxidant supplements that have resulted in increases in clinical pregnancy rates and live birth rates (76, 210), namely: astaxanthin, L-carnitine in combination with L-acetyl carnitine, zinc sulphate, vitamin E, and Menevit. Despite the initial promise of these therapeutic candidates, there have been few reports regarding the longevity of this success or follow up studies on the consistency of improved pregnancy outcomes following administration of these antioxidants. Moreover, throughout the analysis of 29 clinical studies of antioxidants targeted to men experiencing fertility problems, extensive variation in outcomes was observed with some studies reporting profound improvement in several semen parameters and some reporting no effect using the same oral antioxidant (210).
Certainly, some of this variability can be attributed to disparities in the intrinsic design of clinical trials, with potential confounders including variations in dose regimens, methodology and the duration of treatment. However, recent reports have also highlighted a lack of selectivity in the patient populations that are recruited for each trial (210, 211). Regrettably, very few antioxidant trials have been performed with cohorts of patients specifically selected based on the presentation of oxidative defects in their spermatozoa or high levels of ROS in their ejaculate. Moreover, the measures of success for these trials, while encompassing important outcomes such as improved semen parameters and time-to-conception, do not commonly include assessments to ensure a complete resolution of ROS levels or DNA damage in the patient’s spermatozoa (210). This has led to a concerning inability to account for why individual antioxidant trials have not been successful and also compromises our future ability to improve on formulations that may be beneficial in stratified patient cohorts.
The reasons for omitting crucial patient selection procedures are undoubtedly complex, though difficulties in the diagnosis of ROS-driven fertility issues and the accurate quantification of oxidative DNA damage remain major clinical roadblocks. Here, despite extensive validation of a number of reliable biomarkers for lipid peroxidation products, ROS, and oxidative DNA damage (211), there are still major challenges associated with the cost-efficiency and accuracy of these tools. Consideration should be given to the development of these common laboratory tools to form clinically applicable markers that can be employed for the high throughput analysis of ROS and oxidative DNA damage in human spermatozoa. Working toward this goal, validation has now been performed across several protocols to assess human DNA oxidation levels using 8-hydroxy-2’-deoxyguanosine (8-OHdG) antibodies in tandem with flow cytometry to discriminate patients with poor semen quality (40). Indeed, this study has helped to establish a consensus for a clinically applicable protocol that allows for the stratification of patients based on 8-OHdG fluorescence. Moreover, great care has been taken to provide evidence of the repeatability and accuracy of the assay, and a rationale for its use as part of routine diagnostics in ART clinics (40). It remains to be seen whether the clinical application of this technique will aid in the selection of patients for oral antioxidant trials. However, this approach is a step towards the development of better management strategies for patients with oxidative-stress derived infertility.
The examples provided here serve to highlight the necessity of a continuum between accurate diagnostics to stratify male reproductive pathologies and the successful development of appropriate treatment and management strategies. While there are many exceptions to this rule, such as the management of patients with non-obstructive azoospermia for which there are no current treatment options despite the clarity of the condition, gaining an advanced mechanistic understanding of male reproductive pathologies is essential to improve diagnostic and therapeutic strategies. In this context, the field of reproductive science stands to benefit from diagnostic innovations and personalized treatment strategies that are currently disrupting the standard of care for other clinical pathologies. Indeed, the current key gap in activity regarding precision medicine approaches to optimize ART commits couples to a standard trial-and-error treatment paradigm that is both inefficient and costly. The case for improved treatment selection tools is only further justified by ongoing research and big data efforts, which continue to uncover potential new fertility biomarkers, but we remain some way from actualizing these into novel therapeutic strategies. While most activity is currently focused on female infertility, notable recent efforts discussed above to address male infertility include the introduction of the Cap-Score to assess sperm capacitation, curation of big data arising from the application of omic-based approaches, broader adoption of sperm DNA fragmentation analyses earlier in diagnostic workups, novel imaging tools (e.g. holographic 3D imaging) that leverage artificial intelligence (AI) to improve the accuracy and speed of gamete selection for ART, and at-home smartphone-based semen testing (e.g., the FDA-approved YO Sperm Test).
5 Conclusions
Despite males accounting for a substantial proportion of human infertility, we currently lack the tools to accurately diagnose and treat this distressing condition. Traditional diagnostic approaches often overlook the subtleties associated with day-to-day variations in sperm production and have increasingly been found to be inadequate predictors of fertilization outcome and live birth rates. These shortcomings underscore an urgent need to develop improved diagnostic tools to more accurately inform patient stratification, improve sperm selection and identify valid therapeutic treatment options for males afflicted with subfertility and infertility. In terms of realizing these ambitious goals, we have much to gain from continued research into the molecular mechanisms that govern normal sperm function and an improved understanding of how sperm cell biology becomes dysregulated in infertile males. In particular, dissection of the highly specialized sequence of changes that accompany sperm production and their functional maturation during their transit of the male and female reproductive tracts promises to yield novel insights into how these cells behave during in vitro interventions (Figures 1, 2). Moreover, with the growing realization that poorer-quality sperm may impact offspring health, we have an obligation to define those contributions of the fertilizing spermatozoon that limit the possibility of an adverse outcome after ART interventions. The development of specific sperm biomarkers for this purpose remains a significant goal as does defining the biological signatures indicative of the stress(ors) that the male may have experienced; information that may eventually provide additional clinical decision support to guide ART treatment strategies and maximize success rates.
Author contributions
BN, JS, NB, DS-B, HH, GD, JM, TL and EB contributed to conception and design of this article. BN and EB wrote the first draft of the manuscript. JS, NB, DS-B, HH, GDI, JM, and TL each wrote sections of the manuscript. All authors contributed to manuscript revision, read, and approved the submitted version.
Funding
BN is supported by a National Health and Medical Research Council of Australia (NHMRC) Senior Researcher Fellowship (Grant number: APP1154837). EB is supported by an Australian Research Council (ARC) Discovery Early Career Researcher Award (Grant number: DE210100103). TL is supported by an Australian Research Council Discovery Early Career Research Award (DE220100032).
Conflict of interest
The authors declare that this review article has been prepared in the absence of any commercial or financial relationships that could be construed as a potential conflict of interest.
Publisher’s note
All claims expressed in this article are solely those of the authors and do not necessarily represent those of their affiliated organizations, or those of the publisher, the editors and the reviewers. Any product that may be evaluated in this article, or claim that may be made by its manufacturer, is not guaranteed or endorsed by the publisher.
References
2. Niederberger C, Pellicer A. Introduction: IVF’s 40th world birthday. Fertil Steril (2018) 110:4. doi: 10.1016/j.fertnstert.2018.05.017
3. O’Neill CL, Chow S, Rosenwaks Z, Palermo GD. Development of ICSI. Reproduction (2018) 156:F51–8. doi: 10.1530/REP-18-0011
4. Esteves SC. Who cares about oligozoospermia when we have ICSI. Reprod BioMed Online (2022) 44:769–75. doi: 10.1016/j.rbmo.2021.11.026
5. Fitzgerald O, Harris K, Paul RC, Chambers CG. Assisted reproductive technology in Australia and new Zealand 2015. Sydney: National Perinatal Epidemiology & Statistics Unit, University of New Sotuth Wales (2017).
6. Belva F, Bonduelle M, Roelants M, Michielsen D, Van Steirteghem A, Verheyen G, et al. Semen quality of young adult ICSI offspring: the first results. Hum Reprod (2016) 31:2811–20. doi: 10.1093/humrep/dew245
7. Davies MJ, Rumbold AR, Moore VM. Assisted reproductive technologies: a hierarchy of risks for conception, pregnancy outcomes and treatment decisions. J Dev Orig Health Dis (2017) 8:443–7. doi: 10.1017/S2040174417000526
8. Simopoulou M, Gkoles L, Bakas P, Giannelou P, Kalampokas T, Pantos K, et al. Improving ICSI: A review from the spermatozoon perspective. Syst Biol Reprod Med (2016) 62:359–71. doi: 10.1080/19396368.2016.1229365
9. Barratt CLR, De Jonge CJ, Sharpe RM. ‘Man up’: the importance and strategy for placing male reproductive health centre stage in the political and research agenda. Hum Reprod (2018) 33:541–5. doi: 10.1093/humrep/dey020
10. Bjorndahl L. A paradigmatic shift in the care of male factor infertility: how can the recommendations for basic semen examination in the sixth edition of the WHO manual and the ISO 23162:2021 standard help? Reprod BioMed Online (2022) 45:731–6. doi: 10.1016/j.rbmo.2022.03.011
11. Bjorndahl L, Kirkman Brown J, other Editorial Board Members of the WHO Laboratory Manual for the Examination and Processing of Human Semen. The sixth edition of the WHO laboratory manual for the examination and processing of human semen: ensuring quality and standardization in basic examination of human ejaculates. Fertil Steril (2022) 117:246–51. doi: 10.1016/j.fertnstert.2021.12.012
12. Chung E, Arafa M, Boitrelle F, Kandil H, Henkel R, Saleh R, et al. The new 6th edition of the WHO laboratory manual for the examination and processing of human semen: is it a step toward better standard operating procedure? Asian J Androl (2022) 24:123–4. doi: 10.4103/aja2021118
13. Esteves SC. Evolution of the world health organization semen analysis manual: where are we? Nat Rev Urol (2022) 19:439–46. doi: 10.1038/s41585-022-00593-2
14. Pozzi E, Ramasamy R, Salonia A. Initial andrological evaluation of the infertile Male. Eur Urol Focus. (2022) 9:51–4. doi: 10.1016/j.euf.2022.09.012
15. Setti AS, Paes De Almeida Ferreira Braga D, Iaconelli A Jr., Aoki T, Borges E Jr. Twelve years of MSOME and IMSI: a review. Reprod BioMed Online (2013) 27:338–52. doi: 10.1016/j.rbmo.2013.06.011
16. Teixeira DM, Barbosa MA, Ferriani RA, Navarro PA, Raine-Fenning N, Nastri CO, et al. Regular (ICSI) versus ultra-high magnification (IMSI) sperm selection for assisted reproduction. Cochrane Database Syst Rev (2013) (7):CD010167. doi: 10.1002/14651858.CD010167.pub2
17. Mortimer D, Mortimer ST. Computer-aided sperm analysis (CASA) of sperm motility and hyperactivation. Methods Mol Biol (2013) 927:77–87. doi: 10.1007/978-1-62703-038-0_8
18. Mortimer D, Pandya IJ, Sawers RS. Relationship between human sperm motility characteristics and sperm penetration into human cervical mucus in vitro. J Reprod Fertil (1986) 78:93–102. doi: 10.1530/jrf.0.0780093
19. Hirano Y, Shibahara H, Obara H, Suzuki T, Takamizawa S, Yamaguchi C, et al. Relationships between sperm motility characteristics assessed by the computer-aided sperm analysis (CASA) and fertilization rates in vitro. J Assist Reprod Genet (2001) 18:213–8. doi: 10.1023/A:1009420432234
20. Tung CK, Suarez SS. Co-Adaptation of physical attributes of the mammalian female reproductive tract and sperm to facilitate fertilization. Cells (2021) 10:1297. doi: 10.3390/cells10061297
21. Zaferani M, Suarez SS, Abbaspourrad A. Mammalian sperm hyperactivation regulates navigation via physical boundaries and promotes pseudo-chemotaxis. Proc Natl Acad Sci U.S.A. (2021) 118:e2107500118. doi: 10.1073/pnas.2107500118
22. Allamaneni SS, Agarwal A, Rama S, Ranganathan P, Sharma RK. Comparative study on density gradients and swim-up preparation techniques utilizing neat and cryopreserved spermatozoa. Asian J Androl (2005) 7:86–92. doi: 10.1111/j.1745-7262.2005.00008.x
23. Karamahmutoglu H, Erdem A, Erdem M, Mutlu MF, Bozkurt N, Oktem M, et al. The gradient technique improves success rates in intrauterine insemination cycles of unexplained subfertile couples when compared to swim up technique; a prospective randomized study. J Assist Reprod Genet (2014) 31:1139–45. doi: 10.1007/s10815-014-0274-4
24. Baldini D, Ferri D, Baldini GM, Lot D, Catino A, Vizziello D, et al. Sperm selection for ICSI: Do we have a winner? Cells (2021) 10:3566. doi: 10.3390/cells10123566
25. Silva-Villalobos F, Pimentel JA, Darszon A, Corkidi G. Imaging of the 3D dynamics of flagellar beating in human sperm. Annu Int Conf IEEE Eng Med Biol Soc (2014) 2014:190–3. doi: 10.1109/EMBC.2014.6943561
26. Bukatin A, Kukhtevich I, Stoop N, Dunkel J, Kantsler V. Bimodal rheotactic behavior reflects flagellar beat asymmetry in human sperm cells. Proc Natl Acad Sci U.S.A. (2015) 112:15904–9. doi: 10.1073/pnas.1515159112
27. Daloglu MU, Luo W, Shabbir F, Lin F, Kim K, Lee I, et al. Label-free 3D computational imaging of spermatozoon locomotion, head spin and flagellum beating over a large volume. Light Sci Appl (2018) 7:17121. doi: 10.1038/lsa.2017.121
28. Gong A, Rode S, Gompper G, Kaupp UB, Elgeti J, Friedrich BM, et al. Reconstruction of the three-dimensional beat pattern underlying swimming behaviors of sperm. Eur Phys J E Soft Matter (2021) 44:87. doi: 10.1140/epje/s10189-021-00076-z
29. Dardikman-Yoffe G, Mirsky SK, Barnea I, Shaked NT. High-resolution 4-d acquisition of freely swimming human sperm cells without staining. Sci Adv (2020) 6:eaay7619. doi: 10.1126/sciadv.aay7619
30. Aitken RJ, Nixon B. Sperm capacitation: a distant landscape glimpsed but unexplored. Mol Hum Reprod (2013) 19:785–93. doi: 10.1093/molehr/gat067
31. Nixon B, Aitken RJ, Mclaughlin EA. New insights into the molecular mechanisms of sperm-egg interaction. Cell Mol Life Sci (2007) 64:1805–23. doi: 10.1007/s00018-007-6552-x
32. Dun MD, Mitchell LA, Aitken RJ, Nixon B. Sperm-zona pellucida interaction: molecular mechanisms and the potential for contraceptive intervention. Handb Exp Pharmacol (2010) 198:139–78. doi: 10.1007/978-3-642-02062-9_9
33. Nixon B, Cafe SL, Eamens AL, De Iuliis GN, Bromfield EG, Martin JH, et al. Molecular insights into the divergence and diversity of post-testicular maturation strategies. Mol Cell Endocrinol (2020) 517:110955. doi: 10.1016/j.mce.2020.110955
34. Skerrett-Byrne DA, Anderson AL, Bromfield EG, Bernstein IR, Mulhall JE, Schjenken JE, et al. Global profiling of the proteomic changes associated with the post-testicular maturation of mouse spermatozoa. Cell Rep (2022) 41:111655. doi: 10.1016/j.celrep.2022.111655
35. World Health Organization. WHO laboratory manual for the examination and processing of human semen, sixth edition. Geneva: World Health Organization (2021).
36. Heydari A, Zabetian Targhi M, Halvaei I, Nosrati R. A novel microfluidic device with parallel channels for sperm separation using spermatozoa intrinsic behaviors. Sci Rep (2023) 13:1185. doi: 10.1038/s41598-023-28315-7
37. Villeneuve P, Saez F, Hug E, Chorfa A, Guiton R, Schubert B, et al. Spermatozoa isolation with Felix TM outperforms conventional density gradient centrifugation preparation in selecting cells with low DNA damage. Andrology (2023). doi: 10.1111/andr.13384
38. Sharara F, Seaman E, Morris R, Schinfeld J, Nichols J, Sobel M, et al. Multicentric, prospective observational data show sperm capacitation predicts male fertility, and cohort comparison reveals a high prevalence of impaired capacitation in men questioning their fertility. Reprod BioMed Online (2020) 41:69–79. doi: 10.1016/j.rbmo.2020.03.011
39. West R, Coomarasamy A, Frew L, Hutton R, Kirkman-Brown J, Lawlor M, et al. Sperm selection with hyaluronic acid improved live birth outcomes among older couples and was connected to sperm DNA quality, potentially affecting all treatment outcomes. Hum Reprod (2022) 37:1106–25. doi: 10.1093/humrep/deac058
40. Vorilhon S, Brugnon F, Kocer A, Dollet S, Bourgne C, Berger M, et al. Accuracy of human sperm DNA oxidation quantification and threshold determination using an 8-OHdG immuno-detection assay. Hum Reprod (2018) 33:553–62. doi: 10.1093/humrep/dey038
41. Onofre J, Geenen L, Cox A, van der AuwerA I, Willendrup F, Andersen E, et al. Simplified sperm testing devices: a possible tool to overcome lack of accessibility and inconsistency in male factor infertility diagnosis. an opportunity for low- and middle- income countries. Facts Views Vis Obgyn (2021) 13:79–93. doi: 10.52054/FVVO.13.1.011
42. Nixon B, Stanger SJ, Mihalas BP, Reilly JN, Anderson AL, Tyagi S, et al. The microRNA signature of mouse spermatozoa is substantially modified during epididymal maturation. Biol Reprod (2015) 93:91. doi: 10.1095/biolreprod.115.132209
43. Wykes SM, Krawetz SA. The structural organization of sperm chromatin. J Biol Chem (2003) 278:29471–7. doi: 10.1074/jbc.M304545200
44. Hutcheon K, Mclaughlin EA, Stanger SJ, Bernstein IR, Dun MD, Eamens AL, et al. Analysis of the small non-protein-coding RNA profile of mouse spermatozoa reveals specific enrichment of piRNAs within mature spermatozoa. RNA Biol (2017) 14:1776–90. doi: 10.1080/15476286.2017.1356569
45. Conine CC, Sun F, Song L, Rivera-Perez JA, Rando OJ. Small RNAs gained during epididymal transit of sperm are essential for embryonic development in mice. Dev Cell (2018) 46:470–480 e3. doi: 10.1016/j.devcel.2018.06.024
46. Sharma U, Sun F, Conine CC, Reichholf B, Kukreja S, Herzog VA, et al. Small RNAs are trafficked from the epididymis to developing mammalian sperm. Dev Cell (2018) 46:481–494 e6. doi: 10.1016/j.devcel.2018.06.023
47. Schjenken JE, Robertson SA. The female response to seminal fluid. Physiol Rev (2020) 100:1077–117. doi: 10.1152/physrev.00013.2018
48. Bromfield EG, Nixon B. The function of chaperone proteins in the assemblage of protein complexes involved in gamete adhesion and fusion processes. Reproduction (2013) 145:R31–42. doi: 10.1530/REP-12-0316
49. Zhou W, Anderson AL, Turner AP, De Iuliis GN, Mccluskey A, Mclaughlin EA, et al. Characterization of a novel role for the dynamin mechanoenzymes in the regulation of human sperm acrosomal exocytosis. Mol Hum Reprod (2017) 23:657–73. doi: 10.1093/molehr/gax044
50. Trigg NA, Skerrett-Byrne DA, Xavier MJ, Zhou W, Anderson AL, Stanger SJ, et al. Acrylamide modulates the mouse epididymal proteome to drive alterations in the sperm small non-coding RNA profile and dysregulate embryo development. Cell Rep (2021) 37:109787. doi: 10.1016/j.celrep.2021.109787
51. Lee GS, Conine CC. The transmission of intergenerational epigenetic information by sperm microRNAs. Epigenomes (2022) 6:12. doi: 10.3390/epigenomes6020012
52. Huszar G, Jakab A, Sakkas D, Ozenci CC, Cayli S, Delpiano E, et al. Fertility testing and ICSI sperm selection by hyaluronic acid binding: clinical and genetic aspects. Reprod BioMed Online (2007) 14:650–63. doi: 10.1016/S1472-6483(10)61060-7
53. Mcdowell S, Kroon B, Ford E, Hook Y, Glujovsky D, Yazdani A. Advanced sperm selection techniques for assisted reproduction. Cochrane Database Syst Rev (2014) (10):CD010461. doi: 10.1002/14651858.CD010461.pub2
54. Lepine S, Mcdowell S, Searle LM, Kroon B, Glujovsky D, Yazdani A. Advanced sperm selection techniques for assisted reproduction. Cochrane Database Syst Rev (2019) 7:CD010461. doi: 10.1002/14651858.CD010461.pub3
55. Miller D, Pavitt S, Sharma V, Forbes G, Hooper R, Bhattacharya S, et al. Physiological, hyaluronan-selected intracytoplasmic sperm injection for infertility treatment (HABSelect): a parallel, two-group, randomised trial. Lancet (2019) 393:416–22. doi: 10.1016/S0140-6736(18)32989-1
56. Nixon B, Bromfield EG, Cui J, De Iuliis GN. Heat shock protein A2 (HSPA2): Regulatory roles in germ cell development and sperm function. Adv Anat Embryol Cell Biol (2017) 222:67–93. doi: 10.1007/978-3-319-51409-3_4
57. Nixon B, Dun MD, Aitken RJ. Proteomic analysis of human spermatozoa. In: Krause WKH, Naz RK, editors. Immune infertility: The impact of immune reactions on human infertility. Heidelberg, Germany: Springer Publishing Company (2017).
58. Gomez-Torres MJ, Huerta-Retamal N, Robles-Gomez L, Saez-Espinosa P, Aizpurua J, Aviles M, et al. Arylsulfatase a remodeling during human sperm In vitro capacitation using field emission scanning electron microscopy (FE-SEM). Cells (2021) 10:222. doi: 10.3390/cells10020222
59. Huerta-Retamal N, Saez-Espinosa P, Robles-Gomez L, Aviles M, Romero A, Aizpurua J, et al. Human sperm chaperone HSPA2 distribution during in vitro capacitation. J Reprod Immunol (2021) 143:103246. doi: 10.1016/j.jri.2020.103246
60. Aitken RJ, Nixon B, LIN M, Koppers AJ, Lee YH, Baker MA. Proteomic changes in mammalian spermatozoa during epididymal maturation. Asian J Androl (2007) 9:554–64. doi: 10.1111/j.1745-7262.2007.00280.x
61. Redgrove KA, Anderson AL, Dun MD, Mclaughlin EA, O’bryan MK, Aitken RJ, et al. Involvement of multimeric protein complexes in mediating the capacitation-dependent binding of human spermatozoa to homologous zonae pellucidae. Dev Biol (2011) 356:460–74. doi: 10.1016/j.ydbio.2011.05.674
62. Redgrove KA, Anderson AL, Mclaughlin EA, O’bryan MK, Aitken RJ, Nixon B. Investigation of the mechanisms by which the molecular chaperone HSPA2 regulates the expression of sperm surface receptors involved in human sperm-oocyte recognition. Mol Hum Reprod (2013) 19:120–35. doi: 10.1093/molehr/gas064
63. Bromfield EG, Aitken RJ, Anderson AL, Mclaughlin EA, Nixon B. The impact of oxidative stress on chaperone-mediated human sperm-egg interaction. Hum Reprod (2015) 30:2597–613. doi: 10.1093/humrep/dev214
64. Kelsey KM, Zigo M, Thompson WE, Kerns K, Manandhar G, Sutovsky M, et al. Reciprocal surface expression of arylsulfatase a and ubiquitin in normal and defective mammalian spermatozoa. Cell Tissue Res (2020) 379:561–76. doi: 10.1007/s00441-019-03144-1
65. Schinfeld J, Sharara F, Morris R, Palermo GD, Rosenwaks Z, Seaman E, et al. Cap-score prospectively predicts probability of pregnancy. Mol Reprod Dev (2018) 85:654–64. doi: 10.1002/mrd.23057
66. Bedford JM. Enigmas of mammalian gamete form and function. Biol Rev Camb Philos Soc (2004) 79:429–60. doi: 10.1017/S146479310300633X
67. Muller CH. Rationale, interpretation, validation, and uses of sperm function tests. J Androl (2000) 21:10–30. doi: 10.1002/j.1939-4640.2000.tb03270.x
68. Liu DY, Baker HW. Defective sperm-zona pellucida interaction: a major cause of failure of fertilization in clinical in-vitro fertilization. Hum Reprod (2000) 15:702–8. doi: 10.1093/humrep/15.3.702
69. Oehninger S, Franken DR, Ombelet W. Sperm functional tests. Fertil Steril (2014) 102:1528–33. doi: 10.1016/j.fertnstert.2014.09.044
70. Liu DY, Baker HW. Human sperm bound to the zona pellucida have normal nuclear chromatin as assessed by acridine orange fluorescence. Hum Reprod (2007) 22:1597–602. doi: 10.1093/humrep/dem044
71. Liu DY. Could using the zona pellucida bound sperm for intracytoplasmic sperm injection (ICSI) enhance the outcome of ICSI? Asian J Androl (2011) 13:197–8. doi: 10.1038/aja.2010.179
72. Ganeva R, Parvanov D, Velikova D, Vasileva M, Nikolova K, Stamenov G. Sperm morphology and DNA fragmentation after zona pellucida selection. Reprod Fertil (2021) 2:221–30. doi: 10.1530/RAF-21-0041
73. Wang L, Chen M, Yan G, Zhao S. DNA Methylation differences between zona pellucida-bound and manually selected spermatozoa are associated with autism susceptibility. Front Endocrinol (Lausanne) (2021) 12:774260. doi: 10.3389/fendo.2021.774260
74. Aitken RJ, Baker MA, De Iuliis GN, Nixon B. New insights into sperm physiology and pathology. Handb Exp Pharmacol (2010) 198:99–115. doi: 10.1007/978-3-642-02062-9_7
75. Walters JLH, De Iuliis GN, Dun MD, Aitken RJ, Mclaughlin EA, Nixon B, et al. Pharmacological inhibition of arachidonate 15-lipoxygenase protects human spermatozoa against oxidative stress. Biol Reprod (2018) 98:784–94. doi: 10.1093/biolre/ioy058
76. Walters JLH, De Iuliis GN, Nixon B, Bromfield EG. Oxidative stress in the male germline: A review of novel strategies to reduce 4-hydroxynonenal production. Antioxid (Basel) (2018) 7:132. doi: 10.3390/antiox7100132
77. Walters JLH, Anderson AL, Martins da Silva SJ, Aitken RJ, De Iuliis GN, Sutherland JM, et al. Mechanistic insight into the regulation of lipoxygenase-driven lipid peroxidation events in human spermatozoa and their impact on Male fertility. Antioxid (Basel) (2020) 10:43. doi: 10.3390/antiox10010043
78. Paes Almeida Ferreira De Braga D, Iaconelli A Jr., Cassia Savio De Figueira R, Madaschi C, Semiao-Francisco L, Borges E Jr.. Outcome of ICSI using zona pellucida-bound spermatozoa and conventionally selected spermatozoa. Reprod BioMed Online (2009) 19:802–7. doi: 10.1016/j.rbmo.2009.09.020
79. Jin R, Bao J, Tang D, Liu F, Wang G, Zhao Y, et al. Outcomes of intracytoplasmic sperm injection using the zona pellucida-bound sperm or manually selected sperm. J Assist Reprod Genet (2016) 33:597–601. doi: 10.1007/s10815-016-0676-6
80. Clark GF. The molecular basis of mouse sperm-zona pellucida binding: a still unresolved issue in developmental biology. Reproduction (2011) 142:377–81. doi: 10.1530/REP-11-0118
81. Pang PC, Chiu PC, Lee CL, Chang LY, Panico M, Morris HR, et al. Human sperm binding is mediated by the sialyl-lewis(x) oligosaccharide on the zona pellucida. Science (2011) 333:1761–4. doi: 10.1126/science.1207438
82. Avella MA, Xiong B, Dean J. The molecular basis of gamete recognition in mice and humans. Mol Hum Reprod (2013) 19:279–89. doi: 10.1093/molehr/gat004
83. Avella MA, Baibakov B, Dean J. A single domain of the ZP2 zona pellucida protein mediates gamete recognition in mice and humans. J Cell Biol (2014) 205:801–9. doi: 10.1083/jcb.201404025
84. Avella MA, Baibakov BA, Jimenez-Movilla M, Sadusky AB, Dean J. ZP2 peptide beads select human sperm in vitro, decoy mouse sperm in vivo, and provide reversible contraception. Sci Transl Med (2016) 8:336ra60.
85. Oehninger S, Clark GF, Fulgham D, Blackmore PF, Mahony MC, Acosta AA, et al. Effect of fucoidin on human sperm-zona pellucida interactions. J Androl (1992) 13:519–25.
86. Martin C, Woodland E. Sperm selection technology in ART. Semin Reprod Med (2021) 39:200–6. doi: 10.1055/s-0041-1732313
87. Schroter S, Osterhoff C, Mcardle W, Ivell R. The glycocalyx of the sperm surface. Hum Reprod Update (1999) 5:302–13. doi: 10.1093/humupd/5.4.302
88. Lan R, Xin M, Hao Z, You S, Xu Y, Wu J, et al. Biological functions and Large-scale profiling of protein glycosylation in human semen. J Proteome Res (2020) 19:3877–89. doi: 10.1021/acs.jproteome.9b00795
89. Tecle E, Gagneux P. Sugar-coated sperm: Unraveling the functions of the mammalian sperm glycocalyx. Mol Reprod Dev (2015) 82:635–50. doi: 10.1002/mrd.22500
90. Ainsworth C, Nixon B, Aitken RJ. Development of a novel electrophoretic system for the isolation of human spermatozoa. Hum Reprod (2005) 20:2261–70. doi: 10.1093/humrep/dei024
91. Ainsworth C, Nixon B, Jansen RP, Aitken RJ. First recorded pregnancy and normal birth after ICSI using electrophoretically isolated spermatozoa. Hum Reprod (2007) 22:197–200. doi: 10.1093/humrep/del351
92. Ainsworth CJ, Nixon B, Aitken RJ. The electrophoretic separation of spermatozoa: an analysis of genotype, surface carbohydrate composition and potential for capacitation. Int J Androl (2011) 34:e422–34. doi: 10.1111/j.1365-2605.2011.01164.x
93. Shapouri F, Mahendran T, Govindarajan M, Xie P, Kocur O, Palermo GD, et al. A comparison between the Felix electrophoretic system of sperm isolation and conventional density gradient centrifugation: a multicentre analysis. J Assist Reprod Genet (2022) 40:83-95. doi: 10.1007/s10815-022-02680-0
94. Said TM, Land JA. Effects of advanced selection methods on sperm quality and ART outcome: a systematic review. Hum Reprod Update (2011) 17:719–33. doi: 10.1093/humupd/dmr032
95. Simon L, Ge SQ, Carrell DT. Sperm selection based on electrostatic charge. Methods Mol Biol (2013) 927:269–78. doi: 10.1007/978-1-62703-038-0_25
96. Aitken RJ, De Iuliis GN, Gibb Z, Baker MA. The simmet lecture: new horizons on an old landscape–oxidative stress, DNA damage and apoptosis in the male germ line. Reprod Domest Anim (2012) 47 Suppl 4:7–14. doi: 10.1111/j.1439-0531.2012.02049.x
97. Pacheco A, Blanco A, Bronet F, Cruz M, Garcia-Fernandez J, Garcia-Velasco JA. Magnetic-activated cell sorting (MACS): A useful sperm-selection technique in cases of high levels of sperm DNA fragmentation. J Clin Med (2020) 9:3976. doi: 10.3390/jcm9123976
98. Pedrosa ML, Furtado MH, Ferreira MCF, Carneiro MM. Sperm selection in IVF: the long and winding road from bench to bedside. JBRA Assist Reprod (2020) 24:332–9. doi: 10.5935/1518-0557.20190081
99. Gil Julia M, Hervas I, Navarro-Gomez Lechon A, Quintana F, Amoros D, Pacheco A, et al. Sperm selection by magnetic-activated cell sorting before microinjection of autologous oocytes increases cumulative live birth rates with limited clinical impact: A retrospective study in unselected males. Biol (Basel) (2021) 10:430. doi: 10.3390/biology10050430
100. El Fekih S, Gueganic N, Tous C, Ali HB, Ajina M, Douet-Guilbert N, et al. MACS-annexin V cell sorting of semen samples with high TUNEL values decreases the concentration of cells with abnormal chromosomal content: a pilot study. Asian J Androl (2022) 24:445–50. doi: 10.4103/aja202197
101. Rival CM, Xu W, Shankman LS, Morioka S, Arandjelovic S, Lee CS, et al. Phosphatidylserine on viable sperm and phagocytic machinery in oocytes regulate mammalian fertilization. Nat Commun (2019) 10:4456. doi: 10.1038/s41467-019-12406-z
102. Koike-Tani M, Tani T, Mehta SB, Verma A, Oldenbourg R. Polarized light microscopy in reproductive and developmental biology. Mol Reprod Dev (2015) 82:548–62. doi: 10.1002/mrd.22221
103. Omidi M, Faramarzi A, Agharahimi A, Khalili MA. Noninvasive imaging systems for gametes and embryo selection in IVF programs: a review. J Microsc (2017) 267:253–64. doi: 10.1111/jmi.12573
104. Ribeiro MA, Broi MGD, Rose MB, Garolla A, Foresta C, Bragheto A, et al. Sperm selection by birefringence: a promising non-invasive tool to improve ICSI outcomes. JBRA Assist Reprod (2022). doi: 10.5935/1518-0557.20220055
105. Gianaroli L, Magli MC, Collodel G, Moretti E, Ferraretti AP, Baccetti B. Sperm head’s birefringence: a new criterion for sperm selection. Fertil Steril (2008) 90:104–12. doi: 10.1016/j.fertnstert.2007.05.078
106. Vermey BG, Chapman MG, Cooke S, Kilani S. The relationship between sperm head retardance using polarized light microscopy and clinical outcomes. Reprod BioMed Online (2015) 30:67–73. doi: 10.1016/j.rbmo.2014.09.011
107. Singh Jamwal VD. A systematic review identifying fertility biomarkers in semen: a clinical approach through omics to diagnose male infertility. Fertil Steril (2023) 119:158. doi: 10.1016/j.fertnstert.2022.10.031
108. Jodar M, Sendler E, Krawetz SA. The protein and transcript profiles of human semen. Cell Tissue Res (2016) 363:85–96. doi: 10.1007/s00441-015-2237-1
109. Jodar M, Soler-Ventura A, Oliva R, Molecular Biology of R, Development Research G. Semen proteomics and male infertility. J Proteomics (2017) 162:125–34. doi: 10.1016/j.jprot.2016.08.018
110. Agarwal A, Baskaran S, Panner Selvam MK, Barbarosie C, Master K. Unraveling the footsteps of proteomics in male reproductive research: A scientometric approach. Antioxid Redox Signal (2020) 32:536–49. doi: 10.1089/ars.2019.7945
111. Agarwal A, Panner Selvam MK, Baskaran S. Proteomic analyses of human sperm cells: Understanding the role of proteins and molecular pathways affecting Male reproductive health. Int J Mol Sci (2020) 21:1621. doi: 10.3390/ijms21051621
112. Walters JLH, Gadella BM, Sutherland JM, Nixon B, Bromfield EG. Male Infertility: Shining a light on lipids and lipid-modulating enzymes in the Male germline. J Clin Med (2020) 9:327. doi: 10.3390/jcm9020327
113. Zhou W, De Iuliis GN, Dun MD, Nixon B. Characteristics of the epididymal luminal environment responsible for sperm maturation and storage. Front Endocrinol (Lausanne) (2018) 9:59. doi: 10.3389/fendo.2018.00059
114. Zhou W, Stanger SJ, Anderson AL, Bernstein IR, De Iuliis GN, Mccluskey A, et al. Mechanisms of tethering and cargo transfer during epididymosome-sperm interactions. BMC Biol (2019) 17:35. doi: 10.1186/s12915-019-0653-5
115. Nixon B, De Iuliis GN, Hart HM, Zhou W, Mathe A, Bernstein IR, et al. Proteomic profiling of mouse epididymosomes reveals their contributions to post-testicular sperm maturation. Mol Cell Proteomics (2019) 18:S91–S108. doi: 10.1074/mcp.RA118.000946
116. Tamessar CT, Trigg NA, Nixon B, Skerrett-Byrne DA, Sharkey DJ, Robertson SA, et al. Roles of male reproductive tract extracellular vesicles in reproduction. Am J Reprod Immunol (2021) 85:e13338. doi: 10.1111/aji.13338
117. Amaral A, Castillo J, Ramalho-Santos J, Oliva R. The combined human sperm proteome: cellular pathways and implications for basic and clinical science. Hum Reprod Update (2014) 20:40–62. doi: 10.1093/humupd/dmt046
118. Ayaz A, Agarwal A, Sharma R, Kothandaraman N, Cakar Z, Sikka S. Proteomic analysis of sperm proteins in infertile men with high levels of reactive oxygen species. Andrologia (2018) 50:e13015. doi: 10.1111/and.13015
119. Dias TR, Samanta L, Agarwal A, Pushparaj PN, Panner Selvam MK, Sharma R. Proteomic signatures reveal differences in stress response, antioxidant defense and proteasomal activity in fertile men with high seminal ROS levels. Int J Mol Sci (2019) 20:203. doi: 10.3390/ijms20010203
120. Panner Selvam MK, Agarwal A, Pushparaj PN, Baskaran S, Bendou H. Sperm proteome analysis and identification of fertility-associated biomarkers in unexplained Male infertility. Genes (Basel) (2019) 10:522. doi: 10.3390/genes10070522
121. Martins AD, Panner Selvam MK, Agarwal A, Alves MG, Baskaran S. Alterations in seminal plasma proteomic profile in men with primary and secondary infertility. Sci Rep (2020) 10:7539. doi: 10.1038/s41598-020-64434-1
122. Panner Selvam MK, Baskaran S, Agarwal A, Henkel R. Protein profiling in unlocking the basis of varicocele-associated infertility. Andrologia (2021) 53:e13645. doi: 10.1111/and.13645
123. Huszar G, Stone K, Dix D, Vigue L. Putative creatine kinase m-isoform in human sperm is identifiedas the 70-kilodalton heat shock protein HspA2. Biol Reprod (2000) 63:925–32. doi: 10.1095/biolreprod63.3.925
124. Bromfield E, Aitken RJ, Nixon B. Novel characterization of the HSPA2-stabilizing protein BAG6 in human spermatozoa. Mol Hum Reprod (2015) 21:755–69. doi: 10.1093/molehr/gav041
125. Nowicka-Bauer K, Malcher A, Wloczkowska O, Kamieniczna M, Olszewska M, Kurpisz MK. Evaluation of seminal plasma HSPA2 protein as a biomarker of human spermatogenesis status. Reprod Biol (2022) 22:100597. doi: 10.1016/j.repbio.2021.100597
126. Baker MA, Weinberg A, Hetherington L, Villaverde AI, Velkov T, Baell J, et al. Defining the mechanisms by which the reactive oxygen species by-product, 4-hydroxynonenal, affects human sperm cell function. Biol Reprod (2015) 92:108. doi: 10.1095/biolreprod.114.126680
127. Nixon B, Bernstein IR, Cafe SL, Delehedde M, Sergeant N, Anderson AL, et al. A kinase anchor protein 4 is vulnerable to oxidative adduction in Male germ cells. Front Cell Dev Biol (2019) 7:319. doi: 10.3389/fcell.2019.00319
128. Netherton JK, Hetherington L, Ogle RA, Gavgani MM, Velkov T, Villaverde AIB, et al. Mass spectrometry reveals new insights into the production of superoxide anions and 4-hydroxynonenal adducted proteins in human sperm. Proteomics (2020) 20:e1900205. doi: 10.1002/pmic.201900205
129. Miki K, Willis WD, Brown PR, Goulding EH, Fulcher KD, Eddy EM. Targeted disruption of the Akap4 gene causes defects in sperm flagellum and motility. Dev Biol (2002) 248:331–42. doi: 10.1006/dbio.2002.0728
130. Fang X, Huang LL, Xu J, Ma CQ, Chen ZH, Zhang Z, et al. Proteomics and single-cell RNA analysis of Akap4-knockout mice model confirm indispensable role of Akap4 in spermatogenesis. Dev Biol (2019) 454:118–27. doi: 10.1016/j.ydbio.2019.06.017
131. Luconi M, Cantini G, Baldi E, Forti G. Role of a-kinase anchoring proteins (AKAPs) in reproduction. Front Biosci (Landmark Ed) (2011) 16:1315–30. doi: 10.2741/3791
132. Jumeau F, Sigala J, Dossou-Gbete F, Frimat K, Barbotin AL, Buee L, et al. A-kinase anchor protein 4 precursor (pro-AKAP4) in human spermatozoa. Andrology (2018) 6:854–9. doi: 10.1111/andr.12524
133. Carracedo S, Briand-Amirat L, Dordas-Perpinya M, Ramos Escuredo Y, Delcombel R, Sergeant N, et al. ProAKAP4 protein marker: Towards a functional approach to male fertility. Anim Reprod Sci (2022) 247:107074. doi: 10.1016/j.anireprosci.2022.107074
134. Jenkins TG, Aston KI, James ER, Carrell DT. Sperm epigenetics in the study of male fertility, offspring health, and potential clinical applications. Syst Biol Reprod Med (2017) 63:69–76. doi: 10.1080/19396368.2016.1274791
135. Simon L, Emery BR, Carrell DT. Review: Diagnosis and impact of sperm DNA alterations in assisted reproduction. Best Pract Res Clin Obstet Gynaecol (2017) 44:38–56. doi: 10.1016/j.bpobgyn.2017.07.003
136. Johnson GD, Lalancette C, Linnemann AK, Leduc F, Boissonneault G, Krawetz SA. The sperm nucleus: chromatin, RNA, and the nuclear matrix. Reproduction (2011) 141:21–36. doi: 10.1530/REP-10-0322
137. Braun RE. Packaging paternal chromosomes with protamine. Nat Genet (2001) 28:10–2. doi: 10.1038/ng0501-10
138. Hammoud SS, Nix DA, Zhang H, Purwar J, Carrell DT, Cairns BR. Distinctive chromatin in human sperm packages genes for embryo development. Nature (2009) 460:473–8. doi: 10.1038/nature08162
139. Aitken RJ, De Iuliis GN. Origins and consequences of DNA damage in male germ cells. Reprod BioMed Online (2007) 14:727–33. doi: 10.1016/S1472-6483(10)60676-1
140. De Iuliis GN, Thomson LK, Mitchell LA, Finnie JM, Koppers AJ, Hedges A, et al. DNA Damage in human spermatozoa is highly correlated with the efficiency of chromatin remodeling and the formation of 8-hydroxy-2’-deoxyguanosine, a marker of oxidative stress. Biol Reprod (2009) 81:517–24. doi: 10.1095/biolreprod.109.076836
141. Aitken RJ, De Iuliis GN. On the possible origins of DNA damage in human spermatozoa. Mol Hum Reprod (2010) 16:3–13. doi: 10.1093/molehr/gap059
142. Xavier MJ, Nixon B, Roman SD, Scott RJ, Drevet JR, Aitken RJ. Paternal impacts on development: identification of genomic regions vulnerable to oxidative DNA damage in human spermatozoa. Hum Reprod (2019) 34:1876–90. doi: 10.1093/humrep/dez153
143. Noblanc A, Damon-Soubeyrand C, Karrich B, Henry-Berger J, Cadet R, Saez F, et al. DNA Oxidative damage in mammalian spermatozoa: where and why is the male nucleus affected? Free Radic Biol Med (2013) 65:719–23. doi: 10.1016/j.freeradbiomed.2013.07.044
144. Balhorn R, Brewer L, Corzett M. DNA Condensation by protamine and arginine-rich peptides: analysis of toroid stability using single DNA molecules. Mol Reprod Dev (2000) 56:230–4. doi: 10.1002/(SICI)1098-2795(200006)56:2+<230::AID-MRD3>3.0.CO;2-V
145. Conwell CC, Vilfan ID, Hud NV. Controlling the size of nanoscale toroidal DNA condensates with static curvature and ionic strength. Proc Natl Acad Sci U.S.A. (2003) 100:9296–301. doi: 10.1073/pnas.1533135100
146. Zalenskaya IA, Bradbury EM, Zalensky AO. Chromatin structure of telomere domain in human sperm. Biochem Biophys Res Commun (2000) 279:213–8. doi: 10.1006/bbrc.2000.3917
147. Van Der Heijden GW, Ramos L, Baart EB, Van Den Berg IM, Derijck AA, van der Vlag J, et al. Sperm-derived histones contribute to zygotic chromatin in humans. BMC Dev Biol (2008) 8:34. doi: 10.1186/1471-213X-8-34
148. Ward WS. Function of sperm chromatin structural elements in fertilization and development. Mol Hum Reprod (2010) 16:30–6. doi: 10.1093/molehr/gap080
149. Boe-Hansen GB, Fedder J, Ersboll AK, Christensen P. The sperm chromatin structure assay as a diagnostic tool in the human fertility clinic. Hum Reprod (2006) 21:1576–82. doi: 10.1093/humrep/del019
150. Cebesoy FB, Aydos K, Unlu C. Effect of sperm chromatin damage on fertilization ratio and embryo quality post-ICSI. Arch Androl (2006) 52:397–402. doi: 10.1080/01485010600666953
151. Bungum M, Humaidan P, Axmon A, Spano M, Bungum L, Erenpreiss J, et al. Sperm DNA integrity assessment in prediction of assisted reproduction technology outcome. Hum Reprod (2007) 22:174–9. doi: 10.1093/humrep/del326
152. Davies MJ, Moore VM, Willson KJ, Van Essen P, Priest K, Scott H, et al. Reproductive technologies and the risk of birth defects. N Engl J Med (2012) 366:1803–13. doi: 10.1056/NEJMoa1008095
153. Seggers J, De Walle HE, Bergman JE, Groen H, Hadders-Algra M, Bos ME, et al. Congenital anomalies in offspring of subfertile couples: a registry-based study in the northern Netherlands. Fertil Steril (2015) 103:1001–1010 e3. doi: 10.1016/j.fertnstert.2014.12.113
154. Wijers CH, Van Rooij IA, Rassouli R, Wijnen MH, Broens PM, Sloots CE, et al. Parental subfertility, fertility treatment, and the risk of congenital anorectal malformations. Epidemiology (2015) 26:169–76. doi: 10.1097/EDE.0000000000000226
155. Brykczynska U, Hisano M, Erkek S, Ramos L, Oakeley EJ, Roloff TC, et al. Repressive and active histone methylation mark distinct promoters in human and mouse spermatozoa. Nat Struct Mol Biol (2010) 17:679–87. doi: 10.1038/nsmb.1821
156. Lim JP, Brunet A. Bridging the transgenerational gap with epigenetic memory. Trends Genet (2013) 29:176–86. doi: 10.1016/j.tig.2012.12.008
157. Evenson DP, Darzynkiewicz Z, Melamed MR. Relation of mammalian sperm chromatin heterogeneity to fertility. Science (1980) 210:1131–3. doi: 10.1126/science.7444440
158. Singh NP, Mccoy MT, Tice RR, Schneider EL. A simple technique for quantitation of low levels of DNA damage in individual cells. Exp Cell Res (1988) 175:184–91. doi: 10.1016/0014-4827(88)90265-0
159. Gorczyca W, Gong J, Darzynkiewicz Z. Detection of DNA strand breaks in individual apoptotic cells by the in situ terminal deoxynucleotidyl transferase and nick translation assays. Cancer Res (1993) 53:1945–51.
160. Manicardi GC, Bianchi PG, Pantano S, Azzoni P, Bizzaro D, Bianchi U, et al. Presence of endogenous nicks in DNA of ejaculated human spermatozoa and its relationship to chromomycin A3 accessibility. Biol Reprod (1995) 52:864–7. doi: 10.1095/biolreprod52.4.864
161. Sakkas D, Urner F, Bianchi PG, Bizzaro D, Wagner I, Jaquenoud N, et al. Sperm chromatin anomalies can influence decondensation after intracytoplasmic sperm injection. Hum Reprod (1996) 11:837–43. doi: 10.1093/oxfordjournals.humrep.a019263
162. Fernandez JL, Muriel L, Rivero MT, Goyanes V, Vazquez R, Alvarez JG. The sperm chromatin dispersion test: a simple method for the determination of sperm DNA fragmentation. J Androl (2003) 24:59–66. doi: 10.1002/j.1939-4640.2003.tb02641.x
163. Fernandez JL, Muriel L, Goyanes V, Segrelles E, Gosalvez J, Enciso M, et al. Simple determination of human sperm DNA fragmentation with an improved sperm chromatin dispersion test. Fertil Steril (2005) 84:833–42. doi: 10.1016/j.fertnstert.2004.11.089
164. Aoki VW, Emery BR, Liu L, Carrell DT. Protamine levels vary between individual sperm cells of infertile human males and correlate with viability and DNA integrity. J Androl (2006) 27:890–8. doi: 10.2164/jandrol.106.000703
165. Simon L, Zini A, Dyachenko A, Ciampi A, Carrell DT. A systematic review and meta-analysis to determine the effect of sperm DNA damage on in vitro fertilization and intracytoplasmic sperm injection outcome. Asian J Androl (2017) 19:80–90. doi: 10.4103/1008-682X.182822
166. Ribas-Maynou J, Yeste M, Becerra-Tomas N, Aston KI, James ER, Salas-Huetos A. Clinical implications of sperm DNA damage in IVF and ICSI: updated systematic review and meta-analysis. Biol Rev Camb Philos Soc (2021) 96:1284–300. doi: 10.1111/brv.12700
167. Collins JA, Barnhart KT, Schlegel PN. Do sperm DNA integrity tests predict pregnancy with in vitro fertilization? Fertil Steril (2008) 89:823–31. doi: 10.1016/j.fertnstert.2007.04.055
168. Zini A, Sigman M. Are tests of sperm DNA damage clinically useful? pros and cons. J Androl (2009) 30:219–29. doi: 10.2164/jandrol.108.006908
169. Jodar M, Selvaraju S, Sendler E, Diamond MP, Krawetz SA, Reproductive Medicine N. The presence, role and clinical use of spermatozoal RNAs. Hum Reprod Update (2013) 19:604–24. doi: 10.1093/humupd/dmt031
170. Burl RB, Clough S, Sendler E, Estill M, Krawetz SA. Sperm RNA elements as markers of health. Syst Biol Reprod Med (2018) 64:25–38. doi: 10.1080/19396368.2017.1393583
171. Sharma U. Paternal contributions to offspring health: Role of sperm small RNAs in intergenerational transmission of epigenetic information. Front Cell Dev Biol (2019) 7:215. doi: 10.3389/fcell.2019.00215
172. Trigg NA, Eamens AL, Nixon B. The contribution of epididymosomes to the sperm small RNA profile. Reproduction (2019) 157:R209–23. doi: 10.1530/REP-18-0480
173. Kiani M, Salehi M, Mogheiseh A. MicroRNA expression in infertile men: its alterations and effects. Zygote (2019) 27:263–71. doi: 10.1017/S0967199419000340
174. Alves MBR, Celeghini ECC, Belleannee C. From sperm motility to sperm-borne microRNA signatures: New approaches to predict Male fertility potential. Front Cell Dev Biol (2020) 8:791. doi: 10.3389/fcell.2020.00791
175. Salas-Huetos A, James ER, Aston KI, Carrell DT, Jenkins TG, Yeste M. The role of miRNAs in male human reproduction: a systematic review. Andrology (2020) 8:7–26. doi: 10.1111/andr.12714
176. Barbu MG, Thompson DC, Suciu N, Voinea SC, Cretoiu D, Predescu DV. The roles of MicroRNAs in Male infertility. Int J Mol Sci (2021) 22:2910. doi: 10.3390/ijms22062910
177. Bianchi E, Stermer A, Boekelheide K, Sigman M, Hall SJ, Reyes G, et al. High-quality human and rat spermatozoal RNA isolation for functional genomic studies. Andrology (2018) 6:374–83. doi: 10.1111/andr.12471
178. Cardona C, Neri QV, Simpson AJ, Moody MA, Ostermeier GC, Seaman EK, et al. Localization patterns of the ganglioside G(M1) in human sperm are indicative of male fertility and independent of traditional semen measures. Mol Reprod Dev (2017) 84:423–35. doi: 10.1002/mrd.22803
179. Moody MA, Cardona C, Simpson AJ, Smith TT, Travis AJ, Ostermeier GC. Validation of a laboratory-developed test of human sperm capacitation. Mol Reprod Dev (2017) 84:408–22. doi: 10.1002/mrd.22801
180. Nixon B, Aitken RJ. The biological significance of detergent-resistant membranes in spermatozoa. J Reprod Immunol (2009) 83:8–13. doi: 10.1016/j.jri.2009.06.258
181. Nixon B, Mitchell LA, Anderson AL, Mclaughlin EA, O’Bryan M K, Aitken RJ. Proteomic and functional analysis of human sperm detergent resistant membranes. J Cell Physiol (2011) 226:2651–65. doi: 10.1002/jcp.22615
182. Ostermeier GC, Cardona C, Moody MA, Simpson AJ, Mendoza R, Seaman E, et al. Timing of sperm capacitation varies reproducibly among men. Mol Reprod Dev (2018) 85:387–96. doi: 10.1002/mrd.22972
183. Shan S, Xu F, Hirschfeld M, Brenig B. Sperm lipid markers of Male fertility in mammals. Int J Mol Sci (2021) 22:8767. doi: 10.3390/ijms22168767
184. Drabovich AP, Jarvi K, Diamandis EP. Verification of male infertility biomarkers in seminal plasma by multiplex selected reaction monitoring assay. Mol Cell Proteomics (2011) 10:M110 004127. doi: 10.1074/mcp.M110.004127
185. Korbakis D, Schiza C, Brinc D, Soosaipillai A, Karakosta TD, Legare C, et al. Preclinical evaluation of a TEX101 protein ELISA test for the differential diagnosis of male infertility. BMC Med (2017) 15:60. doi: 10.1186/s12916-017-0817-5
186. Lazzarino G, Listorti I, Muzii L, Amorini AM, Longo S, Di Stasio E, et al. Low-molecular weight compounds in human seminal plasma as potential biomarkers of male infertility. Hum Reprod (2018) 33:1817–28. doi: 10.1093/humrep/dey279
187. Gamidov SI, Shatylko TV, Tambiev AK, Tokareva AO, Chagovets VV, Bitsoev TB, et al. [Prognostic ability of seminal plasma lipidomic analysis in predicting the success of microTESE in men with azoospermia]. Urologiia (2022) 4:32–7. doi: 10.18565/urology.2022.4.32-37
188. Li HG, Huang SY, Zhou H, Liao AH, Xiong CL. Quick recovery and characterization of cell-free DNA in seminal plasma of normozoospermia and azoospermia: implications for non-invasive genetic utilities. Asian J Androl (2009) 11:703–9. doi: 10.1038/aja.2009.65
189. Hong Y, Wang C, Fu Z, Liang H, Zhang S, Lu M, et al. Systematic characterization of seminal plasma piRNAs as molecular biomarkers for male infertility. Sci Rep (2016) 6:24229. doi: 10.1038/srep24229
190. Zhi EL, Liang GQ, Li P, Chen HX, Tian RH, Xu P, et al. Seminal plasma miR-192a: a biomarker predicting successful resolution of nonobstructive azoospermia following varicocele repair. Asian J Androl (2018) 20:396–9. doi: 10.4103/aja.aja_8_18
191. Xu Y, Zhang Y, Yang Y, Liu X, Chen Y. Seminal plasma miR-210-3p is a biomarker for screening dyszoospermia caused by varicocele. Andrologia (2019) 51:e13244. doi: 10.1111/and.13244
192. Wang F, Yang W, Ouyang S, Yuan S. The vehicle determines the destination: The significance of seminal plasma factors for male fertility. Int J Mol Sci (2020) 21:8499. doi: 10.3390/ijms21228499
193. Bromfield JJ. Seminal fluid and reproduction: much more than previously thought. J Assist Reprod Genet (2014) 31:627–36. doi: 10.1007/s10815-014-0243-y
194. Robertson SA, Sharkey DJ. Seminal fluid and fertility in women. Fertil Steril (2016) 106:511–9. doi: 10.1016/j.fertnstert.2016.07.1101
195. Kho EM, Mccowan LM, North RA, Roberts CT, Chan E, Black MA, et al. Duration of sexual relationship and its effect on preeclampsia and small for gestational age perinatal outcome. J Reprod Immunol (2009) 82:66–73. doi: 10.1016/j.jri.2009.04.011
196. Crawford G, Ray A, Gudi A, Shah A, Homburg R. The role of seminal plasma for improved outcomes during in vitro fertilization treatment: review of the literature and meta-analysis. Hum Reprod Update (2015) 21:275–84. doi: 10.1093/humupd/dmu052
197. Saccone G, Di Spiezio Sardo A, Ciardulli A, Caissutti C, Spinelli M, Surbek D, et al. Effectiveness of seminal plasma in in vitro fertilisation treatment: a systematic review and meta-analysis. BJOG (2019) 126:220–5. doi: 10.1111/1471-0528.15004
198. Chan OC, Chow PH, O WS. Total ablation of paternal accessory sex glands curtails developmental potential in preimplantation embryos in the golden hamster. Anat Embryol (Berl) (2001) 204:117–22. doi: 10.1007/s004290100186
199. Wong CL, Lee KH, Lo KM, Chan OC, Goggins W, O WS, et al. Ablation of paternal accessory sex glands imparts physical and behavioural abnormalities to the progeny: an in vivo study in the golden hamster. Theriogenology (2007) 68:654–62. doi: 10.1016/j.theriogenology.2007.04.062
200. Wong CL, Chan OC, Lee KH, O WS, Chow PH. Absence of paternal accessory sex glands dysregulates preimplantation embryo cell cycle and causes early oviductal-uterine transit in the golden hamster in vivo. Fertil Steril (2008) 89:1021–4. doi: 10.1016/j.fertnstert.2007.04.038
201. Bromfield JJ, Schjenken JE, Chin PY, Care AS, Jasper MJ, Robertson SA. Maternal tract factors contribute to paternal seminal fluid impact on metabolic phenotype in offspring. Proc Natl Acad Sci U.S.A. (2014) 111:2200–5. doi: 10.1073/pnas.1305609111
202. Skerrett-Byrne DA, Nixon B, Bromfield EG, Breen J, Trigg NA, Stanger SJ, et al. Transcriptomic analysis of the seminal vesicle response to the reproductive toxicant acrylamide. BMC Genomics (2021) 22:728. doi: 10.1186/s12864-021-07951-1
203. Skerrett-Byrne DA, Trigg NA, Bromfield EG, Dun MD, Bernstein IR, Anderson AL, et al. Proteomic dissection of the impact of environmental exposures on mouse seminal vesicle function. Mol Cell Proteomics (2021) 20:100107. doi: 10.1016/j.mcpro.2021.100107
204. Smyth SP, Nixon B, Anderson AL, Murray HC, Martin JH, Macdougall LA, et al. Elucidation of the protein composition of mouse seminal vesicle fluid. Proteomics (2022) 22:e2100227. doi: 10.1002/pmic.202100227
205. Zavattaro M, Ceruti C, Motta G, Allasia S, Marinelli L, Di Bisceglie C, et al. Treating varicocele in 2018: current knowledge and treatment options. J Endocrinol Invest (2018) 41:1365–75. doi: 10.1007/s40618-018-0952-7
206. Khourdaji I, Lee H, Smith RP. Frontiers in hormone therapy for male infertility. Transl Androl Urol (2018) 7:S353–66. doi: 10.21037/tau.2018.04.03
207. Jung A, Schuppe HC. Influence of genital heat stress on semen quality in humans. Andrologia (2007) 39:203–15. doi: 10.1111/j.1439-0272.2007.00794.x
208. Moazzam A, Sharma R, Agarwal A. Relationship of spermatozoal DNA fragmentation with semen quality in varicocele-positive men. Andrologia (2015) 47:935–44. doi: 10.1111/and.12360
209. Gharagozloo P, Gutierrez-Adan A, Champroux A, Noblanc A, Kocer A, Calle A, et al. A novel antioxidant formulation designed to treat male infertility associated with oxidative stress: promising preclinical evidence from animal models. Hum Reprod (2016) 31:252–62. doi: 10.1093/humrep/dev302
210. Majzoub A, Agarwal A. Systematic review of antioxidant types and doses in male infertility: Benefits on semen parameters, advanced sperm function, assisted reproduction and live-birth rate. Arab J Urol (2018) 16:113–24. doi: 10.1016/j.aju.2017.11.013
Keywords: andrology diagnosis, biomarker, assisted reproductive technologies (ART), male infertility, sperm function assay, sperm
Citation: Nixon B, Schjenken JE, Burke ND, Skerrett-Byrne DA, Hart HM, De Iuliis GN, Martin JH, Lord T and Bromfield EG (2023) New horizons in human sperm selection for assisted reproduction. Front. Endocrinol. 14:1145533. doi: 10.3389/fendo.2023.1145533
Received: 16 January 2023; Accepted: 08 February 2023;
Published: 22 February 2023.
Edited by:
Kun Li, Hangzhou Medical College, ChinaReviewed by:
Stuart A. Meyers, University of California, Davis, United StatesEnrica Bianchi, University of York, United Kingdom
Copyright © 2023 Nixon, Schjenken, Burke, Skerrett-Byrne, Hart, De Iuliis, Martin, Lord and Bromfield. This is an open-access article distributed under the terms of the Creative Commons Attribution License (CC BY). The use, distribution or reproduction in other forums is permitted, provided the original author(s) and the copyright owner(s) are credited and that the original publication in this journal is cited, in accordance with accepted academic practice. No use, distribution or reproduction is permitted which does not comply with these terms.
*Correspondence: Brett Nixon, brett.nixon@newcastle.edu.au