- 1Shunyi Hospital, Beijing Hospital of Traditional Chinese Medicine, Beijing, China
- 2Renal Division, Department of Medicine, Heilongjiang Academy of Chinese Medicine Sciences, Harbin, China
- 3Beijing Key Lab for Immune-Mediated Inflammatory Diseases, China-Japan Friendship Hospital, Beijing, China
Diabetic kidney disease (DKD) is the main cause of end-stage renal disease worldwide, and there is a lack of effective treatment strategies. Autophagy is a highly conserved lysosomal degradation process that maintains homeostasis and energy balance by removing protein aggregates and damaged organelles. Increasing evidence suggests that dysregulated autophagy may contribute to glomerular and tubulointerstitial lesions in the kidney under diabetic conditions. Emerging studies have shown that Chinese herbal medicine and its active compounds may ameliorate diabetic kidney injury by regulating autophagy. In this review, we summarize that dysregulation or insufficiency of autophagy in renal cells, including podocytes, glomerular mesangial cells, and proximal tubular epithelial cells, is a key mechanism for the development of DKD, and focus on the protective effects of Chinese herbal medicine and its active compounds. Moreover, we systematically reviewed the mechanism of autophagy in DKD regulated by Chinese herb compound preparations, single herb and active compounds, so as to provide new drug candidates for clinical treatment of DKD. Finally, we also reviewed the candidate targets of Chinese herbal medicine regulating autophagy for DKD. Therefore, further research on Chinese herbal medicine with autophagy regulation and their targets is of great significance for the realization of new targeted therapies for DKD.
1 Introduction
Diabetic nephropathy (DKD), one of the most prevalent complications of diabetes mellitus, is the leading cause of end-stage kidney disease (1, 2). DKD accounts for 30% to 50% of all chronic kidney disease (CKD) cases, covering 285 million people worldwide (3). Because of its high prevalence, poor prognosis, and healthcare cost burden, DKD is a major public health problem. The development of DKD is quite complicated and involves chronic low-grade inflammation, accumulation of advanced glycosylation end products and oxidative stress. Despite some progress in the treatment of DKD, such as controlling blood glucose, lipids, blood pressure and lifestyle modification, there is still no specific treatment (4, 5).
To date, a growing number of studies have been conducted to determine the association of autophagy with the progression of DKD (6, 7). In this review, we outline recent advances in the study of autophagy in DKD, with a particular focus on the relationship between autophagy and podocytes, glomerular mesangial cells, and proximal renal tubular epithelial cells in Figures 1, 2. Here, we present the role and mechanism of herbal medicines to improve the effect on DKD by regulating autophagy in Tables 1–3 and Figure 3. In addition, herbal medicines with autophagy-modulating ability are highlighted as a promising therapeutic strategy for the treatment of DKD.
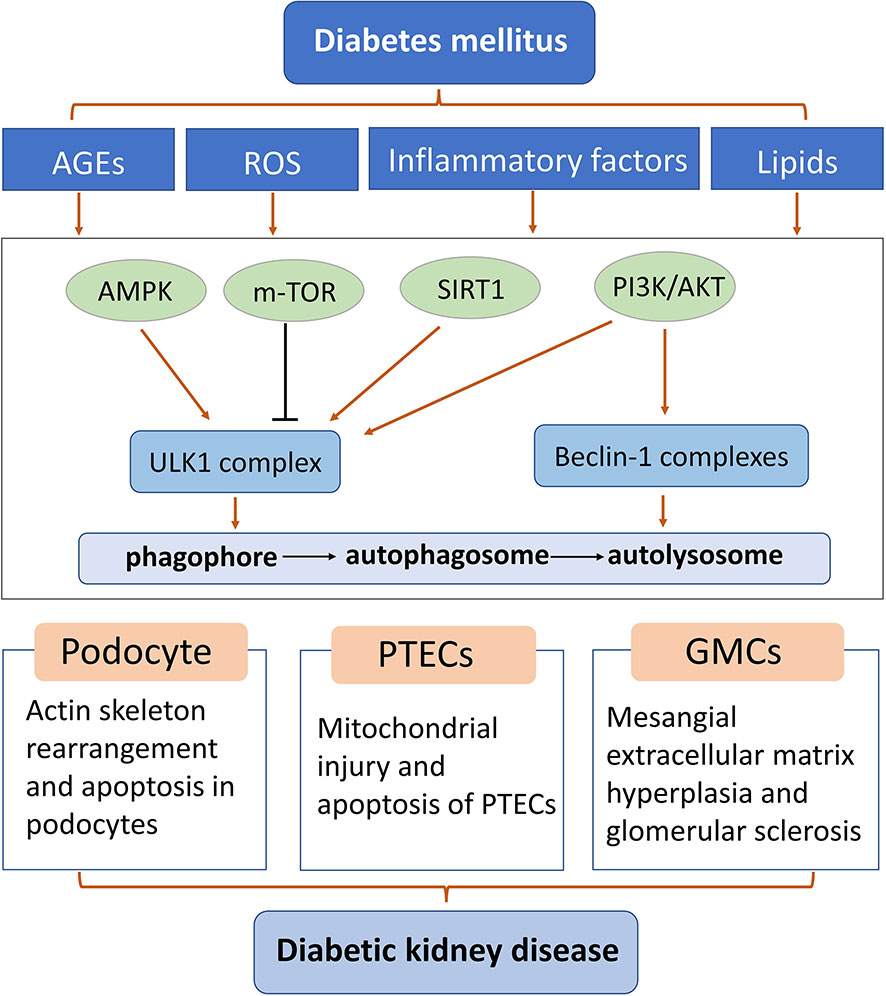
Figure 2 Diagram of some mechanisms of autophagy in podocytes, glomerular mesangial cells, and proximal renal tubular epithelial cells of diabetic kidney disease. AGEs: advanced glycation end products; GMCs: glomerular mesangial cells; PTEC: proximal renal tubular epithelial cells; ROS: reactive oxygen species.
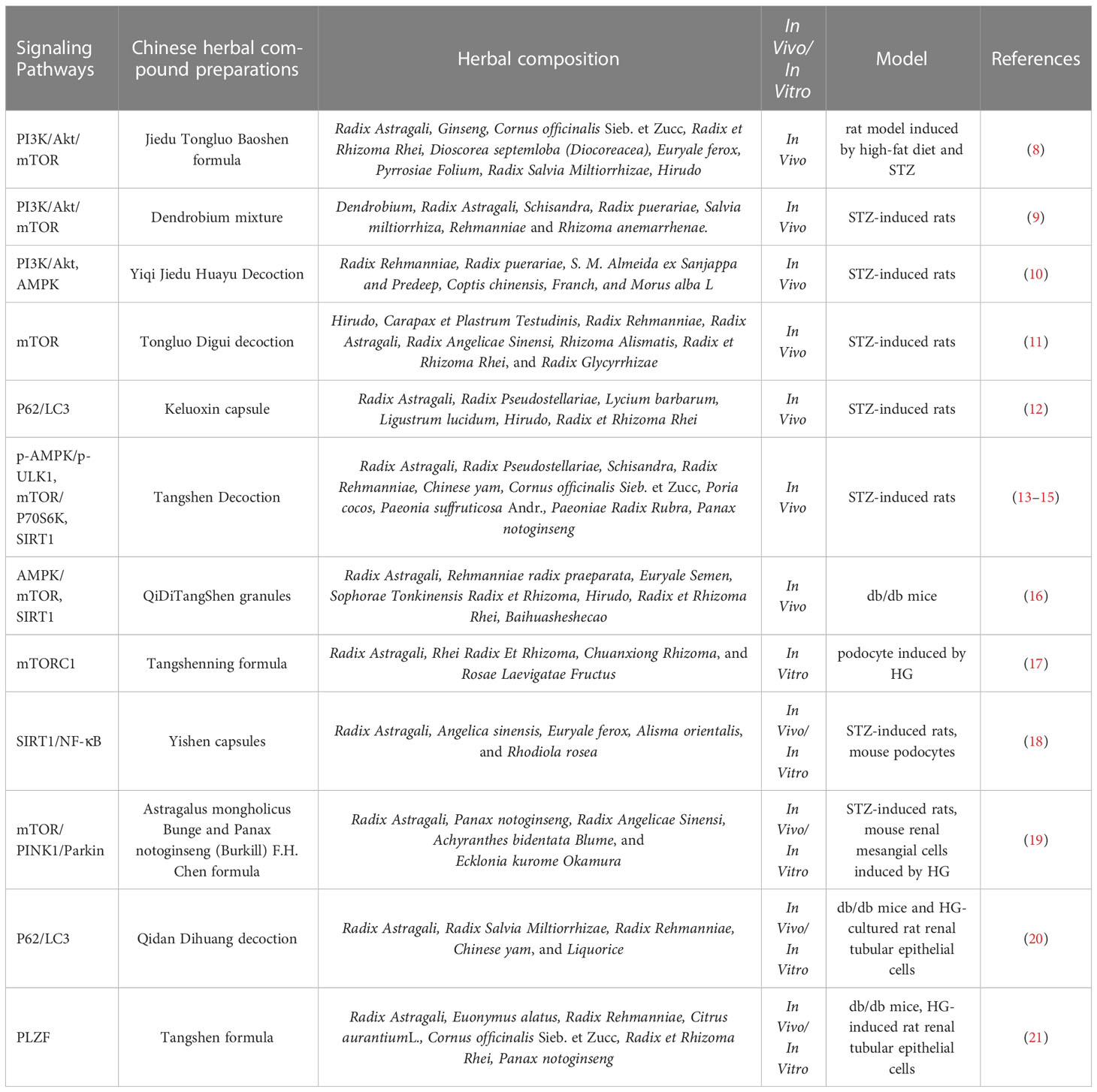
Table 1 The role of Chinese herbal compound preparations against renal injuries via regulating autophagy in DKD.
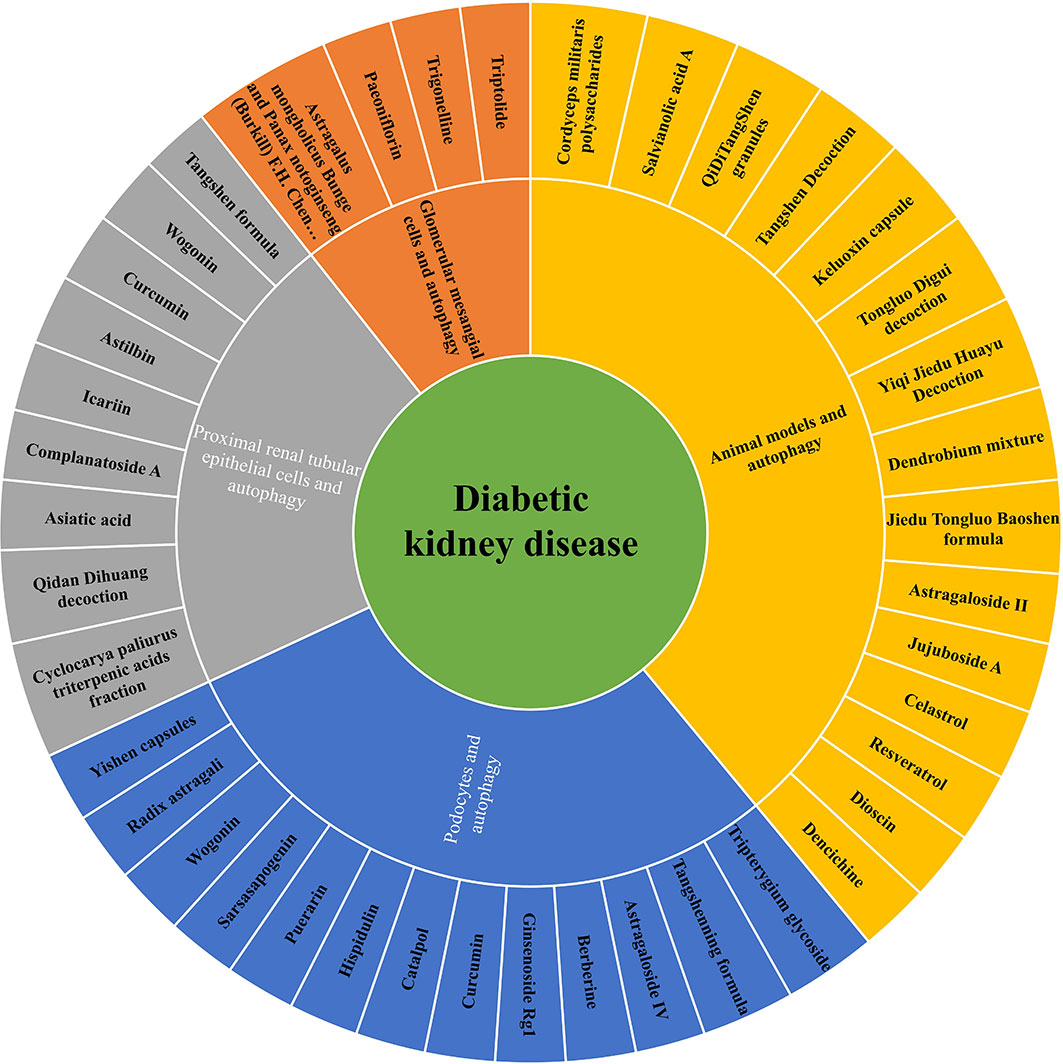
Figure 3 The role of Chinese herbal compound preparations, single herbs and active compounds against renal injuries via regulating autophagy in DKD.
2 Autophagy
Autophagy is a highly conserved lysosomal degradation pathway that ensures homeostasis by breaking down misfolded proteins, damaged organelles, and invasive pathogens for clearance and reuse (54). The three classical types of autophagy include macroautophagy, microautophagy, or chaperone mediated autophagy (55). Macroautophagy refers to the formation of an autophagosome with bilayer vesicles that envelops intracellular substances and eventually fuses with lysosomes to form an autophagosome, resulting in the degradation of the contents (56). In general, autophagy refers to macroautophagy. Microautophagy is a process in which lysosome or vacuole membrane directly invaginates cytoplasmic substances or organelles for degradation without the formation of autophagosomes (57). The molecular chaperone protein Hsc70 specifically recognizes the substrate protein molecule with the KFERQ group, binds to it, and then transports to the lysosome via the receptor LAMP2A on the lysosome membrane, which is finally degraded by lysosome hydrolase (58). According to the selectivity of its degradation substrates, autophagy can be divided into non-selective autophagy and selective autophagy (59). Non-selective autophagy degradation substrates are relatively non-selective, such as rapamycin induced autophagy, nitrogen source starvation induced autophagy, serum starvation induced autophagy, etc (60). Selective autophagy refers to the direct binding of selective autophagy receptors with LC3 (Atg8 in yeast and plant cells) to deliver specific degradation substrates to autophagosomes for degradation (61). The selective autophagy types found so far include mitochondrial autophagy, ribosomal autophagy, endoplasmic reticulum autophagy, peroxisome autophagy, etc (62).
Autophagy occurs through a series of successive stages, including autophagic initiation, nucleation, elongation, fusion and degradation, which are highly regulated by ATG proteins and other cofactors (63, 64). In the initiation stage of autophagy, the single or double layer membrane structure is formed in the cytoplasm and continuously expanded, which is phagophore (65). In the mature stage, phagophore continues to extend and completely envelops autophagy substances to form autophagosomes (66). In the fusion stage, the autophagosome and lysosome fuse to form autophagolysosome. The inner membrane of the autophagosome is degraded by lysosome enzyme, and the cargos in the autophagosome are degraded by lysosome enzyme. Small molecular substances such as amino acids and fatty acids produced are returned to the cytoplasm for reuse to maintain cell homeostasis (67). Thus, during autophagy, the formation, maturation, and subcellular reorientation of autophagosomes eventually lead to their fusion with lysosomes. The activity of the autophagy pathway can be demonstrated by the relative rate of autophagy substrate turnover, known as autophagy flux (68).
Researchers have identified molecular mechanisms involved in autophagy in yeast. Autophagy, as a common physiological regulatory mechanism in mammalian cells, has received extensive attention in recent years. The mammalian target protein of rapamycin and AMP kinase are two key molecules that regulate autophagy activity (69, 70). mTOR, a serine/threonine protein kinase, is a major component of the mTOR pathway and can form mTORC1 and mTORC2 complexes with other proteins (71). mTORC1 complex is a key regulator that controls growth and metabolism by integrating growth factors with nutrient signals such as amino acids, glucose and energy status (72). As a nutrient and energy sensor, AMPK can sense the level of ATP in cells and regulate cell metabolism and energy homeostasis (73). mTOR generally inhibits initiation of autophagy, which is regulated by the upstream regulator AMPK. In the presence of nutrient deficiency, AMPK is activated by high AMP levels, thereby increasing the function of tuberous sclerosis Complex 1(TSC1) and TSC2 and inhibiting the activity of GTP-binding proteins Rheb and mTOR (74). The inactivation of mTOR activates the UNC-51 like autophagy activating kinase 1 (ULK1) by dephosphorylation, the ULK1 complex (ULK1/2, ATGl3, ATG101, and FIP200) is then activated to initiate autophagy (75).
Autophagy extension is the continuous extension process of autophagy membrane with the participation of autophagy proteins (76). In this process, two ubiquitin-like binding systems of autophagy play an important role: the ATG5-ATG12-ATG16L ubiquitin-like binding system and the microtubule-associated protein 1 light chain 3(LC3) ubiquitin-like binding system. Ubiquitin E1-like ligase ATG7 non-covalently binds to ATG12 to form the ATG12-ATG7 complex, which, under the action of the E2-like enzyme ATG10, transfers ATG12 to ATG10 and then ATG12 to ATG5 (77). The two are covalently combined to form a complex, which can be combined with ATG16L to form ATG12-ATG5-ATG16L ternary complex. LC3 is also a ubiquitin-like protein with two forms, LC3-I and LC3-II (78). The lipoification of LC3-I to LC3-II is catalyzed by enzymes like E1, E2 and E3 (79). Under the catalysis of E1-like enzyme ATG7, the ATG7-LC3 complex is formed, and LC3 is transferred to E2-like enzyme ATG3 (80). Under the action of E3-like enzyme ATG12-ATG5-ATG16L, the phosphatidyl ethanolamine is added to the glycine residues at the carboxyl terminal of LC3-I to form LC3-II. LC3-PE can be stably bonded to the bilayer membrane of the autophagic vesicle. The two connective systems cooperate to promote the extension of autophagosome membrane (81). LC3-I is usually free in the cytoplasm, but once transformed into LC3-II, it will bind specifically to the membrane of autophagy and act as a marker protein for detecting autophagy. Western blot detection of LC3-II and LC3-I and observation of green fluorescence after transfection of GFP-LC3-II plasmid is a classical way to determine whether autophagy occurs in cells (82). In the fusion stage of autophagosome and lysosome, LC3-II in mature autophagosome is degraded by lysosome activity or converted into LC3-I by Atg4B in the outer membrane (83).
3 Autophagy in diabetic kidney disease
Autophagy homeostasis is essential for normal kidney function, and abnormal renal autophagy is closely related to the occurrence of DKD (84, 85). Abnormal autophagy has been reported to play a role in renal fibrosis and inflammation in DKD (38, 86). Autophagy dysfunction leads to the accumulation of damaged organelles, such as mitochondria, which play a major role in the formation of reactive oxygen species (ROS) (87, 88). This leads to functional impairment of various renal intrinsic cells, such as tubular epithelial cells, podocytes, and endothelial cells. In short, multiple diabetes risk factors lead to damage to every parenchymal cell type that makes up the kidney. Restoration of autophagy can improve renal function, inhibit renal inflammation and fibrosis, delay the progression of DKD, and become an important target for the treatment of DKD.
3.1 Podocytes and autophagy
The basic level of autophagy of podocytes is significantly higher than that of other innate cells of kidney, and such high level of autophagy is necessary to maintain the normal physiological function of podocytes (89). It was found that the abnormal autophagy pathway of podocyte is closely related to the pathogenesis of DKD. Animal studies showed that autophagy substrate protein p62 accumulated in DKD model podocytes, indicating that autophagy activity of kidney podocytes was inhibited under DKD condition (90, 91). Podocytes are the intrinsic cells of the kidney. The podocytes issued by the adjacent podocytes are wrapped around the basement membrane of the glomeruli, and form a hiatus diaphragm complex through interlacing adhesion molecules and proteoglycan molecules to maintain the morphology and structure of the basement membrane of the glomeruli, regulate the charge barrier to prevent the passage of large molecular proteins, stabilize the structure of the capillaries of the glomeruli, balance the static pressure of the capillaries, and maintain the function of the glomerular endothelial cells It can synthesize and secrete vascular endothelial growth factor.
Compared with diabetic renal biopsy samples with mild urinary protein excretion, renal samples from diabetic patients had less pathological damage and significantly decreased podocyte autophagy level. Clinical and animal experiments showed that autophagy was impaired in type 2 diabetic patients and OLETF rats with abundant albuminuria (92). Renal mesangial dilatation and glomerulosclerosis were more severe after specific ATG5 knockout in STZ-induced diabetic mouse podocytes, suggesting a critical role of podocyte autophagy in glomerulopathy (93). In addition, when podocyte-specific ATG5 knockout mice were given a high-fat diet, they developed abundant albuminuria, accompanied by severe tubulointerstitial lesions and podocytic damage, resulting in abnormal lysosome accumulation, compared with wild-type mice (93). Interestingly, autophagy levels of podocytes in DKD varied with exposure time. In vitro experiments showed that autophagy level increased in mouse podocytes lines induced by short-term high glucose. Long-term exposure to high sugar levels inhibited autophagy (94). In streptozotocin (STZ) -induced diabetic mice, podocyte autophagy was induced at 4 weeks and inhibited at 8 weeks (95).
3.2 Glomerular mesangial cells and autophagy
Glomerular mesangial cells (GMCs) are one of the most important intrinsic cells in glomeruli and play a crucial role in the occurrence and development of glomerular diseases (96, 97). Any intervention that maintains the balance between mesangial cell proliferation, hypertrophy, and apoptosis, and improves mesangial stroma metabolism, is key to alleviating or reversing glomerular disease. The renal pathological changes of DKD are mainly characterized by proliferation of mesangial cells and increased mesangial matrix. No matter mesangial cell proliferation or atrophy, mesangial extracellular proteins are associated accumulation, resulting in sclerosis of the glomeruli.
Studies have shown that mesangial autophagy is induced by transforming growth factor β1(TGF-β1) through TAK11 and PI3K-Akt dependent pathways in serum-free conditions, which prevents mesangial cell apoptosis and increases mesangial cell survival (98). Autophagy can negatively regulate mesangial cells to produce extracellular matrix through intracellular degradation of type I collagen (99). This suggests a new intracellular mechanism, mesangial cells reduce the level of collagen fibers through autophagy, thus inhibiting renal fibrosis, which is the main mechanism of the occurrence and development of CKD and DKD (100).
In vitro experiments also showed changes in autophagy of GMCs at DKD stage. The expression of metalloproteinase inhibitor 3 gene (Timp3) was down-regulated in the kidneys of diabetic patients, in the kidneys of STZ-induced diabetic mice, and in GMCs treated with high glucose (101). Studies using Timp3−/− mice and high-sugar-induced GMCs have shown that Timp3 gene deletion inhibits autophagy and exacerbates diabetic kidney injury in mice by reducing the expression of FOXO1a and its downstream autophagy related genes. After high expression of Timp3 by adenovirus, the autophagy disorder of GMCs restored by Timp3 gene was reversed and the kidney injury was improved. Mitochondrial autophagy levels are decreased in age-induced GMCs, and the recovery of autophagy significantly reverses mitochondrial dysfunction and apoptosis (102). Despite the above proof of the protective effect of autophagy on GMCs damage, its specific mechanism still needs to be further studied and verified.
3.3 Proximal renal tubular epithelial cells and autophagy
Proximal renal tubular epithelial cells (PTEC) are the most abundant cell type in the kidney, which is essential for normal kidney function and organ regeneration after injury. Proximal renal tubules are a key site of reabsorption activity in the kidney, making it a highly metabolically active organ, second only to the brain in terms of oxygen consumption. Evidence from recent studies suggests that PTECs play a leading role in nephron damage and renal dysfunction during diabetes, which is referred to as “diabetic tubular disease” (103). Due to their position in the nephron and major reabsorption, PTECs are often exposed to a variety of toxic factors in glomerular filtrate, such as high glucose, albumin, and advanced glycation end products (AGEs), which are associated with progressive interstitial inflammation and fibrosis (104, 105).
In physiological state, renal PTEC is in autophagic homeostasis. However, active transport of tubular epithelial cells consumes a lot of energy, making these cells more susceptible to hypoxia or energy deprivation (106). In addition, the renal medulla provides a lower oxygen environment than the renal cortex, which is more likely to lead to tubular epithelial cell damage (107). Autophagy ensures the normal function of cells in harsh environments, which is particularly important for tubular epithelial cell. When autophagy disorders occur in PTEC, structural changes will occur in the kidney, such as interstitial fibrosis (107, 108). In particular, PTEC autophagy disorder can cause mitochondrial damage and lead to severe renal homeostasis abnormalities (109, 110). Although the autophagy level of renal PTECs is relatively low under physiological conditions. However, selective Atg5 or Atg7 deletion in the proximal tubules in mice resulted in progressive renal injury and senescence, characterized by mitochondrial damage, accumulation of ubiquitinated aggregates, increased apoptosis, and tubulointerstitial fibrosis (111, 112). In contrast, the kidneys of mice with ATG5-deficient distal tubules or collecting tubules showed no significant histopathologic changes (113, 114). These results suggest that, under normal circumstances, low levels of basal autophagy are essential for maintaining PTEC function and that proximal tubules are more dependent on basal autophagy than other tubule segments.
Previous studies have shown autophagy disorders in kidney biopsy samples from DKD patients, diabetic animal models, and high-glucose treated PTECs. Mechanism studies have demonstrated that p53 is activated in DKD, thereby inducing the expression of miR-214 in renal tubules (115, 116). miR-214 directly targets ULK1, impedes initiation of autophagy, and leads to impaired autophagy of PTECs. More and more studies have shown mTOR activity dependent autophagy disorders in diabetic animal models and PTEC cells induced by high glucose or albumin overload (111, 117). Long-term or excessive albumin in glomerular filtrate of diabetic nephropathy patients can eventually impair autophagy of PTECs through mTOR dependent mechanism, leading to tubular injury. Studies have shown that obesity-induced autophagy inhibition in PTECs in type 2 diabetes patients and mice is associated with mTOR activation, and that the mTOR inhibitor rapamycin significantly improves renal autophagy deficiency in obese mice induced by a high fat diet (118). Long-term albumin overload resulted in decreased expression of BNIP3L, and then mitochondrial autophagy disorder occurred in PTECs (119, 120). mTOR activity in PTECs and SIRT1 pathway can be inhibited and autophagy disorder can be significantly reversed in diabetic Wistar fat rats after low protein diet and 40% daily food intake restriction, thus improving diabetic renal tubular injury (121, 122).
4 Chinese herbal medicine and its active compounds in attenuating renal injury via regulating autophagy in diabetic nephropathy
4.1 Chinese herb compound preparations in attenuating renal injury via regulating autophagy
Lately, Chinese herbal medicine and its active compounds are increasingly considered as alternative sources for modulating renal autophagy in DKD. Jiedu Tongluo Baoshen formula (JTBF), a mixture of nine crude drugs, reduced early clinical proteinuria in the treatment of DKD patients (123). In high-fat diet and streptozocin (STZ) induced rats, Jin et al. found that JTBF activated the expression of podocyte autophagy-related proteins (beclin-1, LC3 and P62) via suppressing PI3K/Akt/mTOR (8). Furthermore, they found that the extracts of JTBF included 77 active compounds by high performance liquid chromatography (HPLC) (8). Dendrobium mixture is a Chinese herb compound preparation for treating diabetes and its complications, which lowers glucose and lipid levels, and alleviates insulin resistance (124). In STZ-induced rats, Dendrobium mixture has protective effects on the kidney through suppressing the PI3K/Akt/mTOR signaling pathway and promoting renal autophagy (9). In addition, Yiqi Jiedu Huayu decoction also regulated the PI3K/Akt and AMPK pathways, thus promoted autophagy in STZ-induced rats (10). The changes in these key molecules suggest that PI3K/Akt/mTOR signaling pathway is the prototypical signaling pathway that regulates autophagy, and mTOR is a key target of autophagy regulation and plays a negative role in regulating autophagy.
Keluoxin capsule is a Chinese herb compound preparation for treatment of qi and yin deficiency and blood stasis in DKD (125). A meta-analysis for DKD found that Keluoxin capsule improved the levels of serum creatinine and lipid (126). A STZ-induced rat model was used by some researchers to demonstrate the protective effects of Keluoxin capsule
in vivo, they found Keluoxin capsule regulated autophagy in podocytes via increasing the expression of LC3-II and p62 proteins (12).
Tangshen Decoction, so-called Tangshenjian, is a Chinese herb compound preparation for DKD that effectively improve glucose and lipid metabolism and reduce urine protein (127, 128). Unc-51-like kinase 1 (ULK1) is an autophagy-related protein, which regulates by AMPK (129). A study proved that Tangshen Decoction significantly enhanced podocyte autophagy through modulation of p-AMPK/p-ULK1 signaling in STZ-induced rats (13). Ribosomal protein S6 kinase beta-1 (P70S6K) is one of mTOR downstream factors. Meanwhile, these researchers also confirmed that Tangshen Decoction regulated podocyte autophagy by mTOR/P70S6K pathway (14) and up-regulated renal tubule autophagy by regulating SIRT1 (15).
QiDiTangShen granules, a Chinese herb compound preparation, has been proven to effectively reduce proteinuria of DKD (130). Studies have reported that QiDiTangShen granules alleviated renal injuries via modulating the gut microbiome composition and improving bile acid profiles in DKD (131). Recent studies have reported that QiDiTangShen granules also activated autophagy through the regulation of nutrient-sensing signal pathways in db/db mice (16).
Tongluo Digui decoction is a Chinese herb compound preparation that has been used for decades in the treatment of DKD, which improved the clinical symptoms and renal function of patients with DKD in stage IV, reduced proteinuria, relieved inflammatory state and improved renal tubular injury (132, 133). In vivo experiments have demonstrated that Tongluo Digui decoction protected podocytes and reduced proteinuria through increasing autophagy by inhibiting mTOR phosphorylation in STZ-induced rats (11). Furthermore, another study reported that it alleviated renal inflammation in diabetic mice by reduce the expression of NLRP3 (134).
4.1.1 Chinese herb compound preparations in regulating autophagy of podocyte
Tangshenning formula (TSN) is a Chinese herb compound preparation to reduce proteinuria and improve renal function in patients with DKD (135). Some scholars have confirmed through in vivo and in vitro experiments that TSN ameliorated podocyte epithelial-mesenchymal transformation via inhibiting the Wnt/β-catenin pathway in KK-Ay mice (136), and restored podocyte autophagy via inhibiting mTORC1 pathway in HG-induced podocyte (17).
Yishen capsule is a Chinese herb compound preparation that has been used for decades in the First Affiliated Hospital of Shanxi Medical University (137, 138). In previous studies, it has been found that Yishen capsule restored podocyte foot process effacement (137) and alleviated renal inflammation by regulating NOD-like receptor signaling pathway in DKD mice (139). A recent study has found that, Yishen capsule improved renal injury by promoting podocyte autophagy via the SIRT1/NF-κB pathway in STZ-induced DKD rats (18). Network pharmacology and molecular docking found that Yishen capsule included in 12 key components (beta-sitosterol, beta-carotene, stigmasterol, alisol B, mairin, quercetin, caffeic acid, 1-monolinolein, kaempferol, jaranol, formononetin, and calycosin) (140), and reduced renal inflammation and fibrosis damage via upregulating the expression HIF-1α and inhibiting JAK/STAT signaling pathways (141).
4.1.2 Chinese herb compound preparations in regulating autophagy of glomerular mesangial cells and proximal renal tubular epithelial cells
Astragalus mongholicus Bunge and Panax notoginseng (Burkill) F.H. Chen formula (APF) is a Chinese herb compound preparation to significantly alleviate renal inflammation (142). Phosphatase and tensin homolog-induced kinase 1 (PINK1)/Parkin pathway is an important link in the regulation of mitophagy (143). A study demonstrated that APF also alleviated renal inflammation by upregulating autophagy of glomerular mesangial cells via suppressing mTOR and activating PINK1/Parkin signaling (19).
Qidan Dihuang decoction (QDD) is a Chinese herb compound preparation developed according to the etiology and pathogenesis of DKD in TCM, namely “deficiency of Qi and Yin, blood stasis”. QDD is composed of Radix Astragali, Radix Salvia Miltiorrhizae, Radix Rehmanniae, Chinese yam, and Liquorice, which inhibits renal fibrosis via modulating RAS system in STZ-induced rats (144). In db/db mice and HG-cultured rat renal tubular epithelial cells (NRK-52E), Liang et al. further found that QDD promoted renal autophagy by downregulation of the ratio of LC3-II/LC3-I and upregulation of P62 (20).
Tangshen Formula (TSF), a Chinese herb compound preparation, significantly extenuated proteinuria and improved the estimated glomerular filtration rate (eGFR) among DKD patients in a multicenter double-blind randomized placebo-controlled trial (145). TSF had six components in rat plasma, including astragaloside IV, notoginsenoside R1, ginsenoside Re, ginsenoside Rb1, loganin, and morroniside (146). Promyelocytic Leukemia Zinc Finger (PLZF) was dispensable for ILC3 development (147). Some researchers have confirmed through in vivo and in vitro experiments that TSF attenuated diabetic renal injuries by upregulating autophagy via inhibition of PLZF expression (21).
4.2 Single herbs in attenuating renal injury via regulating autophagy
Radix astragali, a medicinal material for tonifying Chinese Qi that is widely used in China to treat kidney diseases. Recent study found that Radix astragali enhanced podocytes autophagy and delays DKD probably by inhibiting the PI3K/Akt/mTOR pathway (23). In addition, Cassia auriculata leaf extract ameliorates renal injury by attenuating autophagic necroptosis via receptor-interacting protein kinase (RIP)-1/RIP-3-p-p38MAPK signaling in STZ-induced rats and rat glomerular endothelial cells induced by HG (22).
4.3 The active compounds in attenuating renal injury via regulating autophagy
It is known that the active compounds can attenuate renal injury by regulating autophagy. Dencichine is a non-protein amino acid from Panax notoginseng (148) with a wide range of biological activities. Recent studies found that Dencichine ameliorated renal injury by increasing autophagy through regulating AMPK/mTOR pathway (24). Dioscin is a glycoside of steroidal saponin, which is found in the rhizome of Dioscorea nipponica Makino, D. zingiberensis C. H. Wright, D. futschauensis Uline and other plants (149). Previous research studies reported that Dioscin has a potent effect against hyperuricemic (150, 151), ischemia/reperfusion injury (IRI) (152), inflammation (153–157), oxidative stress (154, 156, 158), and fibrosis (156). Zhong et al. found Dioscin enhanced autophagy via an AMPK/mTOR pathway in STZ-induced DKD rats (25).
Resveratrol is a natural non-flavonoid phenol, mainly found in Polygonum cuspidatum, mulberries and raspberries (159). Emerging evidence suggests that Resveratrol decreased mTOR/ULK1-mediated autophagy in nephrons in STZ-induced rats (26). Celastrol is a triterpene of Tripterygium wilfordii Hook F, which contributes to renal protection (160). Studies have proved that Celastrol also achieved podocyte homeostasis by regulating autophagy via PI3K/Akt pathway (27).
Salvianolic acid A is a water-soluble phenolic acid extracted from Salvia miltiorrhiza, their various pharmacological effects such as anti-inflammation and anti-diabetic (161, 162) have been the focus of research for many years. A study reported that Salvianolic acid A restored the disturbed autophagy in glomerular endothelial cell via AGE-RAGE-Nox4 axis (28). Jujuboside A, a triterpene saponin from Semen Ziziphi Spinosae, has several biological activities including anti-oxidant, anti-inflammation and anti-apoptosis (163). Another study also found that Jujuboside A enhanced autophagy via regulating calcium/calmodulin-dependent protein kinase kinase 2 (CaMKK2) -AMPK-mTOR and PINK1/Parkin pathways in DKD rats (29). Radix Astragali is one of the most widely used ‘‘benefiting Qi” herbs, which has renal protective effects on DKD (164). As one of the active constituents of Radix Astragali, Astragaloside II improved podocyte autophagy by regulating nuclear factor erythroid 2-related factor 2 (Nrf2) and PINK1 pathway (30).
Cordyceps militaris is an entomogenous fungus belonging to Cordyceps genus of Cordyceps family for the treatment of chronic kidney diseases (165). Cordyceps militaris polysaccharides is one of the most abundant constituents of Cordyceps militaris. Studies have found that Cordyceps militaris exhibits a variety of various pharmacological effects, such as antioxidant, anti-inflammatory, immunomodulatory, and antihyperlipidemic (166). Furthermore, another study found that Cordyceps militaris polysaccharides significantly increased the rate of renal autophagy through regulating the expression of p62 and LC3 (31).
4.3.1 The active compounds in attenuating renal injury via regulating autophagy of podocyte
Wogonin, a flavonoid derived from the root of Scutellaria baicalensis, which has the pharmacological effects of antivirus, antitumor, anti-inflammation (167). In STZ-induced diabetic mice and HG induced mouse podocyte clone 5 (MPC5) cells, Wogonin can regulate the expression of Bcl-2 to promote autophagy and inhibit apoptosis in a dose-dependent manner (32). In addition, Wogonin mitigated NF-κB p65-mediated renal inflammatory response in STZ-induced diabetic mice (32). Sarsasapogenin, the main active ingredient in Anemarrhena asphodeloides Bunge, restored podocyte autophagy through targeting glycogen synthase kinase-3 β (GSK-3β) signaling pathway (33).
Radix puerariae is a major herbal medicine to treat patients with DKD in China. Puerarin, an active compound of Radix puerariae exerted renoprotective effects in STZ-induced mice with endothelial nitric oxide synthase (eNOS) deficiency (168). The evidence from a study suggests that Puerarin also protected podocytes through heme oxygenase 1 (HMOX1) and Sirt1-mediated upregulation of autophagy in DKD (34). Hispidulin is a natural flavonoid in a wide range of herbs, such as Grindelia argentina, Arrabidaea chica, Saussurea involucrate, Salvia involucrata, Crossostephium Chinese, and Arrabidaea chica (169, 170). Hispidulin displays a wide range of pharmacological activities, including antifungal, anti-inflammatory, and antioxidant (171). At the same time, Hispidulin induced podocyte autophagy via the regulation of RCC1-related protein (Pim1)-p21-mTOR signaling axis (35).
Catalpol is an iridoid glycoside compound extracted from Radix Rehmanniae, which has been shown to have antioxidant and anti-inflammatory effects (172, 173). Similarly, as reported, Catalpol improved podocyte autophagy by inhibiting mTOR activity and promoting transcription factor EB (TFEB) nuclear translocation in STZ-induced mice and mouse podocyte induced by HG (36). Tripterygium glycoside is a fat-soluble mixture extracted from Tripterygium wilfordii Hook F, which composed of diterpene lactone, alkaloid and triterpenoid (174). Tripterygium glycoside has excellent anti-inflammatory and antioxidant damage properties and is widely used in China to treat proteinuria in patients with DKD (175, 176). Similarly, Tripterygium glycoside also alleviated podocyte apoptosis by upregulating autophagy through the mTOR/Twist-related protein 1 (TWIST1) signaling pathway (176) and downregulation of β-arrestin-1 (38).
Curcumin, a yellow phytochemical produced by Curcuma longa, has anti-inflammatory and antioxidant properties (38, 177).A finding of a study suggests that Curcumin induces podocyte autophagy through the PI3k/Akt/mTOR pathway (39). Curcumin as reported also activated autophagy in advanced glycation or glycoxidation end-products (AGEs)-cultured human renal tubular epithelial by the activation of PI3K/Akt pathway (48). Ginsenoside Rg1 is an active component of ginseng, which has multiple effects, including anti-inflammation, antioxidation and anti-apoptosis (178). Ginsenoside Rg1 also alleviated podocyte injury by regulating Akt/GSK3 β/β-Catenin pathway by restoring autophagic activity (40). Berberine is a quaternary ammonium salt originating from isoquinoline alkaloids discovered in Berberis vulgaris, Coptis Chinensis, and Berberis aristate (179). In HG-cultured mouse podocytes, Berberine activated podocyte autophagy by inhibiting the mTOR/P70S6K/4EBP1 signaling pathway (41). Further, several lines of evidence suggest that Astragaloside IV promoted podocyte autophagy via the SIRT-NF-κB p65 axis (42) and upregulating the expression of AMPK (43).
4.3.2 The active compounds in attenuating renal injury via regulating autophagy of glomerular mesangial cells and proximal renal tubular epithelial cells
Triptolide is a diterpene of Tripterygium wilfordii Hook F, which exerts immune suppression, anti-inflammatory and anti-cancer activities (180). Similarly, Triptolide restored autophagy to alleviate diabetic renal fibrosis through the miR-141-3p/PTEN/Akt/mTOR pathway (44). Trigonelline is a natural alkaloid from Trigonella foenum-graecum (181). In HG-cultured human mesangial cell, Trigonelline activated autophagy by up-regulating miR-5189-5p expression and activating the AMPK pathway (45). Paeoniflorin is the main bioactive component of Paeonia lactiflora Pall., which has commendable immune regulation and anti-inflammatory effects (182). In addition, Paeoniflorin alleviated AGEs-induced mesangial cell dysfunction by inhibiting autophagy through inhibiting RAGE and upregulating the level of p-mTOR (46).
Wogonin also alleviated renal tubular epithelial injury by inhibiting PI3K/Akt/NF-κB signaling pathways (47). Astilbin is a flavonoid compound from Smilax glabra rhizomes, Hypericum perforatum, which possesses strong anti-inflammation, immunosuppression and antioxidation activities (183). It is important to note that Astilbin inhibited autophagy and apoptosis through the PI3K/Akt pathway in HG-induced human proximal tubular epithelial cells (49).
Icariin is a flavonoid glycoside extracted from the medicinal plant Epimedium (184). Icariin exerts renal protection through multiple bioactivities such as anti-fibrosis (50, 185), anti-inflammatory (186, 187), and anti-oxidative stress (186). In STZ-induced DKD rats and in HG-incubated human renal tubular epithelial cells and rat renal fibroblasts, Icariin restored autophagy through the miR-192-5p/Glucagon-like peptide-1 receptor (GLP-1R) pathway (50). Complanatoside A, an active component from Semen Astragali Complanati, might be a potential therapeutic agent for DKD by suppressing autophagy via targeting NOX4 inhibition in a high-fat diet/streptozotocin-induced diabetic model and TGF-β1-induced HK-2 cells (51).
Cyclocarya paliurus is a common edible and medicinal plant in China, also known as “sweet tea tree” (188). Cyclocarya paliurus triterpenic acids fraction ameliorated renal injury through AMPK-mTOR-regulated autophagy pathway (52). In addition, a triterpenic-acid extract of Cyclocarya paliurus had been shown to reduce renal fibrosis in DKD (189). In STZ-induced rats and HG+TGF-β1-induced HK-2 cells, asiatic acid, a triterpenic-acid extract of Cyclocarya paliurus suppressed tubulointerstitial fibrosis by activating the autophagy-lysosome system via inhibiting TGF-β type I receptor (TGF-βRI) (53).
To sum up, the above Chinese herbal medicine research review is shown in Tables 1–3. These active compounds are classified into six categories based on their chemical structure-polyphenols, alkaloids, terpenes and terpenoids, flavonoid, glycosides and others in Table 3.
5 Conclusions and perspectives
With the development of modern society, there are more and more patients with DKD. Due to the complex etiology of DKD, a single treatment method targeting autophagy often does not work ideally, and Chinese herbal medicine has unique advantages in the treatment of DKD. First of all, since the mechanism of autophagy involves multiple pathways, it is sometimes difficult for drugs with a single target to take into account multiple pathways. Chinese herbal medicine can take into account multiple targets of autophagy in DKD at the same time through multi-target action. Secondly, Chinese herbal medicine and its active compounds have been proven to be safe. Many Chinese herbal compound preparations, including Yiqi Jiedu Huayu Decoction, Keluoxin capsule, and many others mentioned above, have been used in China for thousands of years. In consideration of objective factors such as the mixing of ingredients in Chinese herbal compound preparations, researchers in recent years have attempted to elucidate the mechanism of action of Chinese herbal compound preparations by isolating the active ingredients and conducting cellular or animal experiments (190, 191).
At this moment, there are many shortcomings in the research on regulation of DKD autophagy in Chinese herbal medicine. The research on the mechanism of regulating DKD autophagy in Chinese herbal medicine is still at the stage of observational studies and lacks in-depth mechanism exploration. This is partly due to the use of natural products in Chinese herbal medicine, which is characterized by confounding (192). Therefore, more standardized and larger scale clinical studies are necessary to facilitate wider acceptance of Chinese herbal medicine treatment in DKD patients.
Author contributions
PLiu, HZ, and PLi: conceptualization. PLiu, WZ, YW, and GM: writing - original draft preparation. HZ and PLi: editing, and revising. PLiu, HZ, and PLi: supervision. All authors contributed to the article and approved the submitted version.
Funding
This study was supported by the National Natural Science Foundation of China (No. 82274489, 82174144, 82174296) and National High Level Hospital Clinical Research Funding, Elite Medical Professionals Project of China-Japan Friendship Hospital (NO. ZRJY2021-QM15).
Conflict of interest
The authors declare that the research was conducted in the absence of any commercial or financial relationships that could be construed as a potential conflict of interest.
Publisher’s note
All claims expressed in this article are solely those of the authors and do not necessarily represent those of their affiliated organizations, or those of the publisher, the editors and the reviewers. Any product that may be evaluated in this article, or claim that may be made by its manufacturer, is not guaranteed or endorsed by the publisher.
References
1. Shang J, Cui W, Guo R, Zhang Y, Wang P, Yu W, et al. Zhao: The harmful intestinal microbial community accumulates during DKD exacerbation and microbiome-metabolome combined validation in a mouse model. Front Endocrinol (Lausanne) (2022) 13:964389. doi: 10.3389/fendo.2022.964389
2. Lu K, Wang L, Fu Y, Li G, X. Zhang and M. Cao: Bioinformatics analysis identifies immune-related gene signatures and subtypes in diabetic nephropathy. Front Endocrinol (Lausanne) (2022) 13:1048139. doi: 10.3389/fendo.2022.1048139
3. Webster AC, Nagler EV, Morton RL, Masson P. Chronic kidney disease. Lancet (2017) 389(10075):1238–52. doi: 10.1016/S0140-6736(16)32064-5
4. Gnudi L, Coward R, Long DA. Diabetic nephropathy: Perspective on novel molecular mechanisms. Trends Endocrinol Metab (2016) 27(11):820–30. doi: 10.1016/j.tem.2016.07.002
5. Wang P, Guo R, Bai X, Cui W, Zhang Y, Li H, et al. Sacubitril/Valsartan contributes to improving the diabetic kidney disease and regulating the gut microbiota in mice. Front Endocrinol (Lausanne) (2022) 13:1034818. doi: 10.3389/fendo.2022.1034818
6. Chen X, Wang J, Lin Y, Y. Liu, T. Z. Signaling pathways of podocyte injury in diabetic kidney disease and the effect of sodium-glucose cotransporter 2 inhibitors. Cells (2022) 11(23). doi: 10.3390/cells11233913
7. Hu Q, Jiang L, Yan Q, Zeng J, X. Ma, Zhao Y. A natural products solution to diabetic nephropathy therapy. Pharmacol Ther (2022) 241:108314. doi: 10.1016/j.pharmthera.2022.108314
8. Jin D, Liu F, Yu M, Zhao Y, Yan G, Xue J, et al. Jiedu tongluo baoshen formula enhances podocyte autophagy and reduces proteinuria in diabetic kidney disease by inhibiting PI3K/Akt/mTOR signaling pathway. J Ethnopharmacol (2022) 293:115246. doi: 10.1016/j.jep.2022.115246
9. Chen Y, Zheng YF, Lin XH, Zhang JP, Lin F, Shi H. Dendrobium mixture attenuates renal damage in rats with diabetic nephropathy by inhibiting the PI3K/Akt/mTOR pathway. Mol Med Rep (2021) 24(2). doi: 10.3892/mmr.2021.12229
10. Xuan C, Xi YM, Zhang YD, Tao CH, Zhang LY, Cao WF. Yiqi jiedu huayu decoction alleviates renal injury in rats with diabetic nephropathy by promoting autophagy. Front Pharmacol (2021) 12:624404. doi: 10.3389/fphar.2021.624404
11. Han J, Zhang Y, Shi X, Peng Z, Y. Xing and X. Pang: Tongluo digui decoction treats renal injury in diabetic rats by promoting autophagy of podocytes. J Tradit. Chin Med (2021) 41(1):125–32. doi: 10.19852/j.cnki.jtcm.2021.01.014
12. Yang X, Han X, Wen Q, Qiu X, Deng H, Chen Q. Protective effect of keluoxin against diabetic nephropathy in type 2 diabetic mellitus models. Evid Based Complement Alternat Med (2021) 2021:8455709. doi: 10.1155/2021/8455709
13. Yan L, Xu X, Fan Y, Zhang L, Niu X, Hu A. Tangshen decoction enhances podocytes autophagy to relieve diabetic nephropathy through modulation of p-AMPK/p-ULK1 signaling. Evid Based Complement Alternat Med (2022) 2022:3110854. doi: 10.1155/2022/3110854
14. Niu XJ, Zhang LF, Zhao J, Ye HL, Yan L. Study on the regulatory effect of tangshenjian decoction on podocyte autophagy and mTOR/P70S6K pathway in rats with diabetic nephropathy. J Guangxi Med Univ (2021) 38(11):2064–70. doi: 10.16190/j.cnki.45-1211/r.2021.11.008
15. Yan L, Niu XJ, Zhang LF. Promotive effect of tangshenjian on SIRT1-mediated autophagy in diabetic nephropathy rats. J Shanxi Med Univ (2022) 53(2):209–14. doi: 10.13753/j.issn.1007-6611.2022.02.013
16. Wang X, Zhao L, Ajay AK, Jiao B, Zhang X, Wang C, et al. QiDiTangShen granules activate renal nutrient-sensing associated autophagy in db/db mice. Front Physiol (2019) 10:1224. doi: 10.3389/fphys.2019.01224
17. Xu J, Shan X, Chen C, Gao Y, Zou D, Wang X, et al. Tangshenning attenuates high glucose-induced podocyte injury via restoring autophagy activity through inhibiting mTORC1 activation. J Diabetes Res (2022) 2022:1610416. doi: 10.1155/2022/1610416
18. Liu Y, Liu W, Zhang Z, Hu Y, Zhang X, Sun Y, et al. Yishen capsule promotes podocyte autophagy through regulating SIRT1/NF-κB signaling pathway to improve diabetic nephropathy. Ren Fail (2021) 43(1):128–40. doi: 10.1080/0886022X.2020.1869043
19. Wen D, Tan RZ, Zhao CY, Li JC, Zhong X, Diao H, et al. Astragalus mongholicus bunge and panax notoginseng (Burkill) F.H. Chen formula for renal injury in diabetic nephropathy-In vivo and In vitro evidence for autophagy regulation. Front Pharmacol (2020) 11:732. doi: 10.3389/fphar.2020.00732
20. Liang Q, Bai Z, Xie T, Lu H, Xiang L, Ma K, et al. Deciphering the pharmacological mechanisms of qidan dihuang decoction in ameliorating renal fibrosis in diabetic nephropathy through experimental validation In vitro and in vivo. Evid Based Complement Alternat Med (2022) 2022:4137578. doi: 10.1155/2022/4137578
21. Zhao H, Li X, Zhao T, Zhang H, Yan M, Dong X, et al. Tangshen formula attenuates diabetic renal injuries by upregulating autophagy via inhibition of PLZF expression. PloS One (2017) 12(2):e0171475. doi: 10.1371/journal.pone.0171475
22. Al SM, Chandramoorthy HC, Alshahrani M, Abohassan M, Eid RA, Ravichandran K, et al. Cassia auriculata leaf extract ameliorates diabetic nephropathy by attenuating autophagic necroptosis via RIP-1/RIP-3-p-p38MAPK signaling. J Food Biochem (2021) 45(7):e13810. doi: 10.1111/jfbc.13810
23. Yang F, Qu Q, Zhao C, Liu X, Yang P, Li Z, et al. Paecilomyces cicadae-fermented radix astragali activates podocyte autophagy by attenuating PI3K/AKT/mTOR pathways to protect against diabetic nephropathy in mice. Biomed Pharmacother (2020) 129:110479. doi: 10.1016/j.biopha.2020.110479
24. Huang C, Cheng L, Feng X, Li X, Wang L. Dencichine ameliorates renal injury by improving oxidative stress, apoptosis and fibrosis in diabetic rats. Life Sci (2020) 258:118146. doi: 10.1016/j.lfs.2020.118146
25. Zhong Y, Liu J, Sun D, Guo T, Yao Y, Xia X, et al. Dioscin relieves diabetic nephropathy via suppressing oxidative stress and apoptosis, and improving mitochondrial quality and quantity control. Food Funct (2022) 13(6):3660–73. doi: 10.1039/d1fo02733f
26. Zhu H, Zhong S, Yan H, Wang K, Chen L, Zhou M, et al. Resveratrol reverts streptozotocin-induced diabetic nephropathy. Front Biosci (Landmark Ed) (2020) 25(4):699–709. doi: 10.2741/4829
27. Nie Y, Fu C, Zhang H, Zhang M, Xie H, Tong X, et al. Celastrol slows the progression of early diabetic nephropathy in rats via the PI3K/AKT pathway. BMC Complement Med Ther (2020) 20(1):321. doi: 10.1186/s12906-020-03050-y
28. Hou B, Qiang G, Zhao Y, Yang X, Chen X, Yan Y, et al. And Du G: Salvianolic acid a protects against diabetic nephropathy through ameliorating glomerular endothelial dysfunction via inhibiting AGE-RAGE signaling. Cell Physiol Biochem (2017) 44(6):2378–94. doi: 10.1159/000486154
29. Zhong Y, Luo R, Liu Q, Zhu J, Lei M, Liang X, et al. Jujuboside a ameliorates high fat diet and streptozotocin induced diabetic nephropathy via suppressing oxidative stress, apoptosis, and enhancing autophagy. Food Chem Toxicol (2022) 159:112697. doi: 10.1016/j.fct.2021.112697
30. Su J, Gao C, Xie L, Fan Y, Shen Y, Huang Q, et al. Astragaloside II ameliorated podocyte injury and mitochondrial dysfunction in streptozotocin-induced diabetic rats. Front Pharmacol (2021) 12:638422. doi: 10.3389/fphar.2021.638422
31. Chen DD, Xu R, Zhou JY, Chen JQ, Wang L, Liu XS, et al. Cordyceps militaris polysaccharides exerted protective effects on diabetic nephropathy in mice via regulation of autophagy. Food Funct (2019) 10(8):5102–14. doi: 10.1039/c9fo00957d
32. Liu XQ, Jiang L, Li YY, Huang YB, Hu XR, Zhu W, et al. Wogonin protects glomerular podocytes by targeting bcl-2-mediated autophagy and apoptosis in diabetic kidney disease. Acta Pharmacol Sin (2022) 43(1):96–110. doi: 10.1038/s41401-021-00721-5
33. Li XZ, Jiang H, Xu L, Liu YQ, Tang JW, Shi JS, et al. Sarsasapogenin restores podocyte autophagy in diabetic nephropathy by targeting GSK3β signaling pathway. Biochem Pharmacol (2021) 192:114675. doi: 10.1016/j.bcp.2021.114675
34. Li X, Zhu Q, Zheng R, Yan J, Wei M, Fan Y, et al. Puerarin attenuates diabetic nephropathy by promoting autophagy in podocytes. Front Physiol (2020) 11:73. doi: 10.3389/fphys.2020.00073
35. Wu F, Li S, Zhang N, Huang W, Li X, Wang M, et al. Hispidulin alleviates high-glucose-induced podocyte injury by regulating protective autophagy. Biomed Pharmacother (2018) 104:307–14. doi: 10.1016/j.biopha.2018.05.017
36. Chen Y, Liu Q, Shan Z, Mi W, Zhao Y, Li M, et al. Catalpol ameliorates podocyte injury by stabilizing cytoskeleton and enhancing autophagy in diabetic nephropathy. Front Pharmacol (2019) 10:1477. doi: 10.3389/fphar.2019.01477
37. Tao M, Zheng D, Liang X, Wu D, Hu K, Jin J, et al. Tripterygium glycoside suppresses epithelial−to−mesenchymal transition of diabetic kidney disease podocytes by targeting autophagy through the mTOR/Twist1 pathway. Mol Med Rep (2021) 24(2). doi: 10.3892/mmr.2021.12231
38. Zhan H, Jin J, Liang S, Zhao L, Gong J, He Q. Tripterygium glycoside protects diabetic kidney disease mouse serum-induced podocyte injury by upregulating autophagy and downregulating β-arrestin-1. Histol. Histopathol (2019) 34(8):943–52. doi: 10.14670/HH-18-097
39. Tu Q, Li Y, Jin J, Jiang X, Ren Y, He Q. Curcumin alleviates diabetic nephropathy via inhibiting podocyte mesenchymal transdifferentiation and inducing autophagy in rats and MPC5 cells. Pharm Biol (2019) 57(1):778–86. doi: 10.1080/13880209.2019.1688843
40. Shi Y, Gao Y, Wang T, Wang X, He J, Xu J, et al. Ginsenoside Rg1 alleviates podocyte EMT passage by regulating AKT/GSK3 β/β-catenin pathway by restoring autophagic activity. Evid Based Complement Alternat Med (2020) 2020:1903627. doi: 10.1155/2020/1903627
41. Li C, Guan XM, Wang RY, Xie YS, Zhou H, Ni WJ, et al. Berberine mitigates high glucose-induced podocyte apoptosis by modulating autophagy via the mTOR/P70S6K/4EBP1 pathway. Life Sci (2020) 243:117277. doi: 10.1016/j.lfs.2020.117277
42. Wang X, Gao Y, Tian N, Wang T, Shi Y, Xu J, et al. Astragaloside IV inhibits glucose-induced epithelial-mesenchymal transition of podocytes through autophagy enhancement via the SIRT-NF-κB p65 axis. Sci Rep (2019) 9(1):323. doi: 10.1038/s41598-018-36911-1
43. Guo H, Wang Y, Zhang X, Zang Y, Zhang Y, Wang L, et al. Astragaloside IV protects against podocyte injury via SERCA2-dependent ER stress reduction and AMPKα-regulated autophagy induction in streptozotocin-induced diabetic nephropathy. Sci Rep (2017) 7(1):6852. doi: 10.1038/s41598-017-07061-7
44. Li XY, Wang SS, Han Z, Han F, Chang YP, Yang Y, et al. Triptolide restores autophagy to alleviate diabetic renal fibrosis through the miR-141-3p/PTEN/Akt/mTOR pathway. Mol Ther Nucleic Acids (2017) 9:48–56. doi: 10.1016/j.omtn.2017.08.011
45. Chen C, Ma J, Miao CS, Zhang H, Zhang M, Cao X, et al. Trigonelline induces autophagy to protect mesangial cells in response to high glucose via activating the miR-5189-5p-AMPK pathway. Phytomedicine (2021) 92:153614. doi: 10.1016/j.phymed.2021.153614
46. Chen J, Zhao D, Zhu M, Zhang M, Hou X, Ding W, et al. Paeoniflorin ameliorates AGEs-induced mesangial cell injury through inhibiting RAGE/mTOR/autophagy pathway. Biomed Pharmacother (2017) 89:1362–9. doi: 10.1016/j.biopha.2017.03.016
47. Lei L, Zhao J, Liu XQ, Chen J, Qi XM, Xia LL, et al. Wogonin alleviates kidney tubular epithelial injury in diabetic nephropathy by inhibiting PI3K/Akt/NF-κB signaling pathways. Drug Des Devel Ther (2021) 15:3131–50. doi: 10.2147/DDDT.S310882
48. Wei Y, Gao J, Qin L, Xu Y, Shi H, Qu L, et al. Curcumin suppresses AGEs induced apoptosis in tubular epithelial cells via protective autophagy. Exp Ther Med (2017) 14(6):6052–8. doi: 10.3892/etm.2017.5314
49. Chen F, Sun Z, Zhu X, Ma Y. Astilbin inhibits high glucose-induced autophagy and apoptosis through the PI3K/Akt pathway in human proximal tubular epithelial cells. Biomed Pharmacother (2018) 106:1175–81. doi: 10.1016/j.biopha.2018.07.072
50. Jia Z, Wang K, Zhang Y, Duan Y, Xiao K, Liu S, et al. Icariin ameliorates diabetic renal tubulointerstitial fibrosis by restoring autophagy via regulation of the miR-192-5p/GLP-1R pathway. Front Pharmacol (2021) 12:720387. doi: 10.3389/fphar.2021.720387
51. Ren C, Bao X, Lu X, Du W, Wang X, Wei J, et al. Complanatoside a targeting NOX4 blocks renal fibrosis in diabetic mice by suppressing NLRP3 inflammasome activation and autophagy. Phytomedicine (2022) 104:154310. doi: 10.1016/j.phymed.2022.154310
52. Zhang XX, Jiang CH, Liu Y, Lou DX, Huang YP, Gao M, et al. Cyclocarya paliurus triterpenic acids fraction attenuates kidney injury via AMPK-mTOR-regulated autophagy pathway in diabetic rats. Phytomedicine (2019) 64:153060. doi: 10.1016/j.phymed.2019.153060
53. Zhang XX, Liu Y, Xu SS, Yang R, Jiang CH, Zhu LP, et al. Asiatic Acid from cyclocarya paliurus regulates the autophagy-lysosome system via directly inhibiting TGF-β type I receptor and ameliorates diabetic nephropathy fibrosis. Food Funct (2022) 13(10):5536–46. doi: 10.1039/d1fo02445k
54. Locatelli AG, Cenci S. Autophagy and longevity: Evolutionary hints from hyper-longevous mammals. Front Endocrinol (Lausanne) (2022) 13:1085522. doi: 10.3389/fendo.2022.1085522
55. Habshi T, Shelke V, Kale A, Anders HJ, Gaikwad AB. Role of endoplasmic reticulum stress and autophagy in the transition from acute kidney injury to chronic kidney disease. J Cell Physiol (2022). doi: 10.1002/jcp.30918
56. Murley A, Dillin A. Macroautophagy in quiescent and senescent cells: a pathway to longevity? Trends Cell Biol (2022). doi: 10.1016/j.tcb.2022.10.004
57. Wang L, Klionsky DJ, Shen HM. The emerging mechanisms and functions of microautophagy. Nat Rev Mol Cell Biol (2022). doi: 10.1038/s41580-022-00529-z
58. Hubert V, Weiss S, Rees AJ, Kain R. Modulating chaperone-mediated autophagy and its clinical applications in cancer. Cells (2022) 11(16). doi: 10.3390/cells11162562
59. Vargas J, Hamasaki M, Kawabata T, Youle RJ, Yoshimori T. The mechanisms and roles of selective autophagy in mammals. Nat Rev Mol Cell Biol (2022). doi: 10.1038/s41580-022-00542-2
60. Trelford CB, Guglielmo GMDi. Molecular mechanisms of mammalian autophagy. Biochem J (2021) 478(18):3395–421. doi: 10.1042/BCJ20210314
61. Lee MJ, Park JS, Jo SB, Joe YA. Enhancing anti-cancer therapy with selective autophagy inhibitors by targeting protective autophagy. Biomol Ther (Seoul) (2023) 31(1):1–15. doi: 10.4062/biomolther.2022.153
62. Adriaenssens E, Ferrari L, Martens S. Orchestration of selective autophagy by cargo receptors. Curr Biol (2022) 32(24):R1357–71. doi: 10.1016/j.cub.2022.11.002
63. Zwilling E, Reggiori F. Membrane contact sites in autophagy. Cells (2022) 11(23). doi: 10.3390/cells11233813
64. Durgan J, Florey O. Many roads lead to CASM: Diverse stimuli of noncanonical autophagy share a unifying molecular mechanism. Sci Adv (2022) 8(43):eabo1274. doi: 10.1126/sciadv.abo1274
65. Negi S, Chaudhuri A, Kumar DN, Dehari D, Singh S, Agrawal AK. Nanotherapeutics in autophagy: a paradigm shift in cancer treatment. Drug Delivery Transl Res (2022) 12(11):2589–612. doi: 10.1007/s13346-022-01125-6
66. Rai P, Kumar RJ. Endosomal recycling protein Rab11 in parkin and Pink1 signaling in drosophila model of parkinson's disease. Exp Cell Res (2022) 420(2):113357. doi: 10.1016/j.yexcr.2022.113357
67. Zhu H, Wang W, Li Y. Molecular mechanism and regulation of autophagy and its potential role in epilepsy. Cells (2022) 11(17). doi: 10.3390/cells11172621
68. Fan G, Li F, Wang P, Jin X, Liu R. Natural-Product-Mediated autophagy in the treatment of various liver diseases. Int J Mol Sci (2022) 23(23). doi: 10.3390/ijms232315109
69. Subramanian A, Tamilanban T, Alsayari A, Ramachawolran G, Wong LS, Sekar M, et al. Trilateral association of autophagy, mTOR and alzheimer's disease: Potential pathway in the development for alzheimer's disease therapy. Front Pharmacol (2022) 13:1094351. doi: 10.3389/fphar.2022.1094351
70. Wang S, Li H, Yuan M, H. Fan and Z. Cai: Role of AMPK in autophagy. Front Physiol (2022) 13:1015500. doi: 10.3389/fphys.2022.1015500
71. Han X, Goh KY, Lee WX, Choy SM, Tang HW. The importance of mTORC1-autophagy axis for skeletal muscle diseases. Int J Mol Sci (2022) 24(1). doi: 10.3390/ijms24010297
72. Su YC, Lee WC, Wang CC, Yeh SA, Chen WH, Chen PJ. Targeting PI3K/AKT/mTOR signaling pathway as a radiosensitization in head and neck squamous cell carcinomas. Int J Mol Sci (2022) 23(24). doi: 10.3390/ijms232415749
73. Beena TB, Jesil MA, Harikumar KB. Cross-talk between AMP-activated protein kinase and the sonic hedgehog pathway in the high-fat diet triggered colorectal cancer. Arch Biochem Biophys (2023) 735:109500. doi: 10.1016/j.abb.2022.109500
74. Yang T, Yang WX. The dynamics and regulation of microfilament during spermatogenesis. Gene (2020) 744:144635. doi: 10.1016/j.gene.2020.144635
75. Wang X, Cong P, Wang X, Wang Z, Liu B, Xue C, et al. Docosahexaenoic acid-acylated astaxanthin monoester ameliorates chronic high-fat diet-induced autophagy dysfunction via ULK1 pathway in the hypothalamus of mice. J Sci Food Agric (2023). doi: 10.1002/jsfa.12429
76. Park S, Park SK. Anti-oxidant and anti-aging effects of phlorizin are mediated by DAF-16-Induced stress response and autophagy in caenorhabditis elegans. Antioxidants (Basel) (2022) 11(10). doi: 10.3390/antiox11101996
77. Rahman MA, Engelsen A, Sarowar S, Bindesbøll C, Birkeland E, Goplen D, et al. Chekenya: Bortezomib abrogates temozolomide-induced autophagic flux through an ATG5 dependent pathway. Front Cell Dev Biol (2022) 10:1022191. doi: 10.3389/fcell.2022.1022191
78. Peña-Martinez C, Rickman AD, Heckmann BL. Beyond autophagy: LC3-associated phagocytosis and endocytosis. Sci Adv (2022) 8(43):eabn1702. doi: 10.1126/sciadv.abn1702
79. Magné J, Green DR. LC3-associated endocytosis and the functions of Rubicon and ATG16L1. Sci Adv (2022) 8(43):eabo5600. doi: 10.1126/sciadv.abo5600
80. Varga VB, Keresztes F, Sigmond T, Vellai T, Kovács T. The evolutionary and functional divergence of the Atg8 autophagy protein superfamily. Biol Futur (2022) 73(4):375–84. doi: 10.1007/s42977-022-00123-6
81. Lamark T, Johansen T. Mechanisms of selective autophagy. Annu Rev Cell Dev Biol (2021) 37:143–69. doi: 10.1146/annurev-cellbio-120219-035530
82. Ma LY, Liu SF, Guo YG, Ma ZQ, Li Y, Wang SJ, et al. Diabetes influences the fusion of autophagosomes with lysosomes in SH-SY5Y cells and induces aβ deposition and cognitive dysfunction in STZ-induced diabetic rats. Behav. Brain Res (2023) 114286. doi: 10.1016/j.bbr.2023.114286
83. Hiura F, Kawabata Y, Aoki T, A. Mizokami and E. Jimi: Inhibition of the ATG4-LC3 pathway suppressed osteoclast maturation. Biochem Biophys Res Commun (2022) 632:40–7. doi: 10.1016/j.bbrc.2022.09.065
84. Parmar UM, Jalgaonkar MP, Kulkarni YA, Oza MJ. Autophagy-nutrient sensing pathways in diabetic complications. Pharmacol Res (2022) 184:106408. doi: 10.1016/j.phrs.2022.106408
85. Koch E, Nakhoul R, Nakhoul F, Nakhoul N. Autophagy in diabetic nephropathy: a review. Int Urol. Nephrol (2020) 52(9):1705–12. doi: 10.1007/s11255-020-02545-4
86. Liu XJ, Hu XK, Yang H, Gui LM, Cai ZX, Qi MS, et al. A review of traditional Chinese medicine on treatment of diabetic nephropathy and the involved mechanisms. Am J Chin Med (2022) 50(7):1739–79. doi: 10.1142/S0192415X22500744
87. Salami M, Salami R, Mafi A, Aarabi MH, Vakili O, Asemi Z. Therapeutic potential of resveratrol in diabetic nephropathy according to molecular signaling. Curr Mol Pharmacol (2022) 15(5):716–35. doi: 10.2174/1874467215666211217122523
88. Kaushal GP, Chandrashekar K, Juncos LA. Molecular interactions between reactive oxygen species and autophagy in kidney disease. Int J Mol Sci (2019) 20(15). doi: 10.3390/ijms20153791
89. Guo R, Wang P, Zheng X, Cui W, Shang J, Zhao Z. Corrigendum: SGLT2 inhibitors suppress epithelial-mesenchymal transition in podocytes under diabetic conditions via downregulating the IGF1R/PI3K pathway. Front Pharmacol (2022) 13:1074294. doi: 10.3389/fphar.2022.1074294
90. Wang X, Zeng HX, Jiang L, Liu XQ, Huang YB, Wu YG. Clinical significance of glomerular autophagy in evaluation of diabetic kidney disease progression. Diabetes Metab Syndr Obes (2022) 15:1945–59. doi: 10.2147/DMSO.S366907
91. Zheng T, Wang HY, Chen Y, Chen X, Wu ZL, Hu QY, et al. Src activation aggravates podocyte injury in diabetic nephropathy via suppression of FUNDC1-mediated mitophagy. Front Pharmacol (2022) 13:897046. doi: 10.3389/fphar.2022.897046
92. Woo CY, Baek JY, Kim AR, Hong CH, Yoon JE, Kim HS, et al. Inhibition of ceramide accumulation in podocytes by myriocin prevents diabetic nephropathy. Diabetes Metab J (2020) 44(4):581–91. doi: 10.4093/dmj.2019.0063
93. Matsuda J, Namba T, Takabatake Y, Kimura T, Takahashi A, Yamamoto T, et al. Isaka: Antioxidant role of autophagy in maintaining the integrity of glomerular capillaries. Autophagy (2018) 14(1):53–65. doi: 10.1080/15548627.2017.1391428
94. Zhang Y, Zhao X, Li C, Yang Y, Li L, Chen Y, et al. Chen: Aberrant NAD synthetic flux in podocytes under diabetic conditions and effects of indoleamine 2,3-dioxygenase on promoting de novo NAD synthesis. Biochem Biophys Res Commun (2022) 643:61–8. doi: 10.1016/j.bbrc.2022.12.059
95. Lenoir O, Jasiek M, Hénique C, Guyonnet L, Hartleben B, Bork T, et al. Endothelial cell and podocyte autophagy synergistically protect from diabetes-induced glomerulosclerosis. Autophagy (2015) 11(7):1130–45. doi: 10.1080/15548627.2015.1049799
96. Chen X, Xie H, Liu Y, Ou Q, Deng S. Interference of ALOX5 alleviates inflammation and fibrosis in high glucose−induced renal mesangial cells. Exp Ther Med (2023) 25(1):34. doi: 10.3892/etm.2022.11733
97. Zhong W, Hong C, Dong Y, Li Y, Xiao C, Liu X. ASH2L aggravates fibrosis and inflammation through HIPK2 in high glucose-induced glomerular mesangial cells. Genes (Basel) (2022) 13(12). doi: 10.3390/genes13122244
98. Bian Y, Shi C, Song S, Mu L, Wu M, Qiu D, et al. Sestrin2 attenuates renal damage by regulating hippo pathway in diabetic nephropathy. Cell Tissue Res (2022) 390(1):93–112. doi: 10.1007/s00441-022-03668-z
99. Lu X, Fan Q, Xu L, Li L, Yue Y, Xu Y, et al. Ursolic acid attenuates diabetic mesangial cell injury through the up-regulation of autophagy via miRNA-21/PTEN/Akt/mTOR suppression. PloS One (2015) 10(2):e0117400. doi: 10.1371/journal.pone.0117400
100. Chen K, Yu B, Liao J. LncRNA SOX2OT alleviates mesangial cell proliferation and fibrosis in diabetic nephropathy via Akt/mTOR-mediated autophagy. Mol Med (2021) 27(1):71. doi: 10.1186/s10020-021-00310-6
101. Fiorentino L, Cavalera M, Menini S, Marchetti V, Mavilio M, Fabrizi M, et al. Federici: Loss of TIMP3 underlies diabetic nephropathy via FoxO1/STAT1 interplay. EMBO Mol Med (2013) 5(3):441–55. doi: 10.1002/emmm.201201475
102. Ding J, Jin J, Na LY, Cui S, Ying LH, Lan ZH, et al. Exogenous pancreatic kininogenase protects against tacrolimus-induced renal injury by inhibiting PI3K/AKT signaling: The role of bradykinin receptors. Int Immunopharmacol (2022) 105:108547. doi: 10.1016/j.intimp.2022.108547
103. Ye G, Hu ML, Xiao L. Forkhead box A2-mediated lncRNA SOX2OT up-regulation alleviates oxidative stress and apoptosis of renal tubular epithelial cells by promoting SIRT1 expression in diabetic nephropathy. Nephrol (Carlton) (2022). doi: 10.1111/nep.14139
104. Du L, Chen Y, Shi J, Yu X, Zhou J, Wang X, et al. Inhibition of S100A8/A9 ameliorates renal interstitial fibrosis in diabetic nephropathy. Metabolism (2022), 155376. doi: 10.1016/j.metabol.2022.155376
105. Chen J, Peng H, Chen C, Wang Y, Sang T, Cai Z, et al. NAG-1/GDF15 inhibits diabetic nephropathy via inhibiting AGE/RAGE-mediated inflammation signaling pathways in C57BL/6 mice and HK-2 cells. Life Sci (2022) 311(Pt A):121142. doi: 10.1016/j.lfs.2022.121142
106. Chen Y, Zhang C, Du Y, Yang X, Liu M, Yang W, et al. Exosomal transfer of microRNA-590-3p between renal tubular epithelial cells after renal ischemia-reperfusion injury regulates autophagy by targeting TRAF6. Chin Med J (Engl) (2022) 135(20):2467–77. doi: 10.1097/CM9.0000000000002377
107. Li H, Ge H, Song X, Tan X, Xiong Q, Gong Y, et al. Neferine mitigates cisplatin-induced acute kidney injury in mice by regulating autophagy and apoptosis. Clin Exp Nephrol (2022). doi: 10.1007/s10157-022-02292-z
108. Jin L, Yu B, Liu G, Nie W, Wang J, Chen J, et al. Mitophagy induced by UMI-77 preserves mitochondrial fitness in renal tubular epithelial cells and alleviates renal fibrosis. FASEB J (2022) 36(6):e22342. doi: 10.1096/fj.202200199RR
109. Hong S, Ghandriz R, Siddiqi S, Zhu XY, Saadiq IM, Jordan KL, et al. Effects of elamipretide on autophagy in renal cells of pigs with metabolic syndrome. Cells (2022) 11(18). doi: 10.3390/cells11182891
110. Ming S, Tian J, Ma K, Pei C, Li L, Wang Z, et al. Oxalate-induced apoptosis through ERS-ROS-NF-κB signalling pathway in renal tubular epithelial cell. Mol Med (2022) 28(1):88. doi: 10.1186/s10020-022-00494-5
111. Caza T, Wijewardena C, Al-Rabadi L, Perl A. Cell type-specific mechanistic target of rapamycin-dependent distortion of autophagy pathways in lupus nephritis. Transl Res (2022) 245:55–81. doi: 10.1016/j.trsl.2022.03.004
112. Chen Z, Wang J, He J, Fan H, Hou S, Lv Q. Myoglobin mediates autophagy of NRK-52E in rat renal tubular epithelial cells Via the Pink1/Parkin signaling pathway. Med Sci Monit (2020) 26:e923045. doi: 10.12659/MSM.923045
113. Peng X, Wang Y, Li H, Fan J, Shen J, Yu X, et al. ATG5-mediated autophagy suppresses NF-κB signaling to limit epithelial inflammatory response to kidney injury. Cell Death Dis (2019) 10(4):253. doi: 10.1038/s41419-019-1483-7
114. Li H, Peng X, Wang Y, Cao S, Xiong L, Fan J, et al. Atg5-mediated autophagy deficiency in proximal tubules promotes cell cycle G2/M arrest and renal fibrosis. Autophagy (2016) 12(9):1472–86. doi: 10.1080/15548627.2016.1190071
115. Ma Z, Li L, Livingston MJ, Zhang D, Mi Q, Zhang M, et al. p53/microRNA-214/ULK1 axis impairs renal tubular autophagy in diabetic kidney disease. J Clin Invest (2020) 130(9):5011–26. doi: 10.1172/JCI135536
116. Yildirim D, Bender O, Karagoz ZF, Helvacioglu F, Bilgic MA, Akcay A, et al. Role of autophagy and evaluation the effects of microRNAs 214, 132, 34c and prorenin receptor in a rat model of focal segmental glomerulosclerosis. Life Sci (2021) 280:119671. doi: 10.1016/j.lfs.2021.119671
117. Wang X, Su W, Ma M, Zhu L, Gao R, Qin C, et al. The KLF4-p62 axis prevents vascular endothelial cell injury via the mTOR/S6K pathway and autophagy in diabetic kidney disease. Endokrynol. Pol (2022) 73(5):837–45. doi: 10.5603/EP.a2022.0072
118. Promsan S, Thongnak L, Pengrattanachot N, Phengpol N, Sutthasupha P, Lungkaphin A. Agomelatine, a structural analog of melatonin, improves kidney dysfunction through regulating the AMPK/mTOR signaling pathway to promote autophagy in obese rats. Food Chem Toxicol (2022) 165:113190. doi: 10.1016/j.fct.2022.113190
119. Yu SH, Palanisamy K, Sun KT, Li X, Wang YM, Lin FY, et al. Human antigen r regulates hypoxia-induced mitophagy in renal tubular cells through PARKIN/BNIP3L expressions. J Cell Mol Med (2021) 25(5):2691–702. doi: 10.1111/jcmm.16301
120. Eleftheriadis T, Pissas G, Sounidaki M, Antoniadis N, Antoniadi G, Liakopoulos V, et al. Preconditioning of primary human renal proximal tubular epithelial cells without tryptophan increases survival under hypoxia by inducing autophagy. Int Urol. Nephrol (2017) 49(7):1297–307. doi: 10.1007/s11255-017-1596-9
121. Arab HH, Ashour AM, Eid AH, Arafa EA, Al KH, Abd ES. Targeting oxidative stress, apoptosis, and autophagy by galangin mitigates cadmium-induced renal damage: Role of SIRT1/Nrf2 and AMPK/mTOR pathways. Life Sci (2022) 291:120300. doi: 10.1016/j.lfs.2021.120300
122. Song FQ, Song M, Ma WX, Gao Z, Ti Y, Zhang X, et al. Overexpressing STAMP2 attenuates diabetic renal injuries via upregulating autophagy in diabetic rats. Biochem Biophys Res Commun (2021) 579:47–53. doi: 10.1016/j.bbrc.2021.09.026
123. Mi J, Piao XL, Wang XG, Nan Z. Professor nan zheng's experience in the treatment of thirsty kidney disease based on the theory of "complex disease. Forum Traditionally Chin Med (2016) 05:24–6. doi: 10.13913/j.cnki.41-1110/r.2016.05.013
124. Lin X, Shi H, Cui Y, Wang X, Zhang J, Yu W, et al. Dendrobium mixture regulates hepatic gluconeogenesis in diabetic rats via the phosphoinositide-3-kinase/protein kinase b signaling pathway. Exp Ther Med (2018) 16(1):204–12. doi: 10.3892/etm.2018.6194
125. Wu R, Wei F, Qu L, Bai L, Li J, Li F, et al. Effects of keluoxin capsule combined with losartan potassium on diabetic kidney disease: study protocol for a randomized double-blind placebo-controlled multicenter clinical trial. Trials (2020) 21(1):951. doi: 10.1186/s13063-020-04852-8
126. Bai LT, Li J, Zhao J, Li F, Wei F, Wu R, et al. Keluoxin capsule as adjuvant treatment for diabetic nephropathy: A meta-analysis of randomized controlled trials. Modernization Traditional Chin Med Materia Medica-World Sci Technol (2019) 21(01):65–73. doi: CNKI:SUN:SJKX.0.2019-01-016
127. Guan W, Hu AM. Clinical efficacy of tangshenjian combined with calcium dobesilate in the treatment of diabetic nephropathy. Res Integrated Traditional Chin Western Med (2013) 05(03):119–120+124. doi: 10.3969/j.issn.1674-4616.2013.03.003
128. Hu AM, Tao Y, Zhao J, Yan L, Niu XJ. Clinical Research of tangshenjian in the treatment of type 2 diabetic nephropathy. Chin J Basic Med Traditional Chin Med (2018) 24(01):80–2. doi: CNKI:SUN:ZYJC.0.2018-01-030
129. Song J, Liu J, Cui C, Hu H, Zang N, Yang M, et al. Mesenchymal stromal cells ameliorate diabetes-induced muscle atrophy through exosomes by enhancing AMPK/ULK1-mediated autophagy. J Cachexia Sarcopenia Muscle (2023). doi: 10.1002/jcsm.13177
130. Gao X, Liu H, An Z, He Q. QiDiTangShen granules reduced diabetic kidney injury by regulating the phosphorylation balance of the tyrosine and serine residues of insulin receptor substrate 1. Evid Based Complement Alternat Med (2018) 2018:2503849. doi: 10.1155/2018/2503849
131. Wei H, Wang L, An Z, Xie H, Liu W, Du Q, et al. Liu: QiDiTangShen granules modulated the gut microbiome composition and improved bile acid profiles in a mouse model of diabetic nephropathy. Biomed Pharmacother (2021) 133:111061. doi: 10.1016/j.biopha.2020.111061
132. Pang XX, Shi XJ, Zhang YG, Peng ZN, Xing YF, Han JR. Effect of tongluo digui decoction on clinical efficacy and renal tubular injury of type 2 diabetic nephropathy in stage IV. Lishizhen Med Materia Med Res (2020) 10:2335–8. doi: 10.3969/j.issn.1008-0805.2020.10.009
133. Pang XX, Shi XJ, Zhang YG, Peng ZN, Xing YF, Han JR. Clinical effect of tongluo digui decoction on patients with type 2 diabetic nephropathy in stage IV. Chin Gen Practice(30) 3846-3850 (2020). doi: 10.12114/j.issn.1007-9572.2020.00.267
134. Han JR, Zhang YG, Shi XJ, Pang XX. And sun XY: Effects of tongluo digui decoction on NLRP3 inflammasome and expressions of inflammatory factors in kidney of diabetic kidney disease mice. J Chin Medicinal Materials (2021) 04):943–8. doi: 10.13863/j.issn1001-4454.2021.04.031
135. Gao YB, Zhou H, Guan S, Zhao WJ, Li BM, Chen LY, et al. Multicenter randomizedand double-blind controlled clinical trial of tang shen ning granules in treating diabetic kidney disease. China J Traditional Chin Med Pharm (2017) 32(11):5212–5. doi: CNKI:SUN:BXYY.0.2017-11-113
136. Cui FQ, Gao YB, Wang YF, Meng Y, Cai Z, Shen C, et al. Effect of tang-Shen-Ning decoction on podocyte epithelial-esenchymal transformation via inhibiting wnt/β-catenin pathway in diabetic mice. Ann Palliat Med (2021) 10(12):12921–36. doi: 10.21037/apm-20-602
137. Fang J, Wei H, Sun Y, Zhang X, Liu W, Chang Q, et al. Regulation of podocalyxin expression in the kidney of streptozotocin-induced diabetic rats with Chinese herbs (Yishen capsule). BMC Complement Altern Med (2013) 13:76. doi: 10.1186/1472-6882-13-76
138. Fang JA, Deng AG, Liu JD. A clinical study of yishen capsule in treating early diabetic nephropathy. Chin J Integrated Traditional Western Nephrol (2005) 6(8):457–9. doi: 10.3969/j.issn.1009-587X.2005.08.007
139. Zhang Z, Hu Y, Liu W, Zhang X, Wang R, Li H, et al. Yishen capsule alleviated symptoms of diabetic nephropathy via NOD-like receptor signaling pathway. Diabetes Metab Syndr Obes (2022) 15:2183–95. doi: 10.2147/DMSO.S368867
140. Fang J, Wang C, Zheng J, Liu Y. Network pharmacology study of yishen capsules in the treatment of diabetic nephropathy. PloS One (2022) 17(9):e0273498. doi: 10.1371/journal.pone.0273498
141. Hu Y, Liu S, Liu W, Zhang Z, Liu Y, Li S, et al. Potential molecular mechanism of yishen capsule in the treatment of diabetic nephropathy based on network pharmacology and molecular docking. Diabetes Metab Syndr Obes (2022) 15:943–62. doi: 10.2147/DMSO.S350062
142. Lin X, Li JC, Tan RZ, Xie XS, Wang L. Astragalus propinquus schischkin and panax notoginseng compound improves kidney inflammatory reactions in mice with diabetic nephropathy via regulation of Arid2-IR/NF-κB signaling. Acta Laboratorium Animalis Scientia Sin (2020) 28(3):382–9. doi: 10.3969/j.issn.1005-4847.2020.03.014
143. Zhou X, Liu K, Li J, Cui L, Dong J, Li J, et al. PINK1/Parkin-mediated mitophagy enhances the survival of staphylococcus aureus in bovine macrophages. J Cell Mol Med (2023) 27(3):412–21. doi: 10.1111/jcmm.17664
144. Chen ZJ, Ma F, Sun XM, Zhao XS, Luo R. Renoprotective effect of a Chinese herbal formula, qidan dihuang decoction, on streptozotocin-induced diabetes in rat. Evid Based Complement Alternat Med (2018) 2018:7321086. doi: 10.1155/2018/7321086
145. Li P, Chen Y, Liu J, Hong J, Deng Y, Yang F, et al. Efficacy and safety of tangshen formula on patients with type 2 diabetic kidney disease: a multicenter double-blinded randomized placebo-controlled trial. PloS One (2015) 10(5):e0126027. doi: 10.1371/journal.pone.0126027
146. Yao Y, Yu YC, Cai MR, Zhang ZQ, Bai J, Wu HM, et al. UPLC-MS/MS method for the determination of the herb composition of tangshen formula and the in vivo pharmacokinetics of its metabolites in rat plasma. Phytochem Anal (2022) 33(3):402–26. doi: 10.1002/pca.3098
147. Xu Y, Zhang H, Wu S, Liu J, Liu H, Wang D, et al. PLZF restricts intestinal ILC3 function in gut defense. Cell Mol Immunol (2023). doi: 10.1038/s41423-023-00975-5
148. Ding S, Wang M, Fang S, Xu H, Fan H, Tian Y, et al. D-dencichine regulates thrombopoiesis by promoting megakaryocyte adhesion, migration and proplatelet formation. Front Pharmacol (2018) 9:297. doi: 10.3389/fphar.2018.00297
149. Zhang L, Ni R, Li J, Fan L, Song Y, Wang H, et al. Dioscin regulating bone marrow apoptosis in aplastic anemia. Drug Des Devel Ther (2022) 16:3041–53. doi: 10.2147/DDDT.S370506
150. Su J, Wei Y, Liu M, Liu T, Li J, Ji Y, et al. Anti-hyperuricemic and nephroprotective effects of rhizoma dioscoreae septemlobae extracts and its main component dioscin via regulation of mOAT1, mURAT1 and mOCT2 in hypertensive mice. Arch Pharm Res (2014) 37(10):1336–44. doi: 10.1007/s12272-014-0413-6
151. Zhang Y, Jin L, Liu J, Wang W, Yu H, Li J, et al. Effect and mechanism of dioscin from dioscorea spongiosa on uric acid excretion in animal model of hyperuricemia. J Ethnopharmacol (2018) 214:29–36. doi: 10.1016/j.jep.2017.12.004
152. Qi M, Zheng L, Qi Y, Han X, Xu Y, Xu L, et al. Dioscin attenuates renal ischemia/reperfusion injury by inhibiting the TLR4/MyD88 signaling pathway via up-regulation of HSP70. Pharmacol Res (2015) 100:341–52. doi: 10.1016/j.phrs.2015.08.025
153. Qi M, Yin L, Xu L, Tao X, Qi Y, Han X, et al. Dioscin alleviates lipopolysaccharide-induced inflammatory kidney injury via the microRNA let-7i/TLR4/MyD88 signaling pathway. Pharmacol Res (2016) 111:509–22. doi: 10.1016/j.phrs.2016.07.016
154. Zhang Y, Xu Y, Qi Y, Xu L, Song S, Yin L, et al. Protective effects of dioscin against doxorubicin-induced nephrotoxicity via adjusting FXR-mediated oxidative stress and inflammation. Toxicology (2017) 378:53–64. doi: 10.1016/j.tox.2017.01.007
155. Zhang Y, Tao X, Yin L, Xu L, Xu Y, Qi Y, et al. Protective effects of dioscin against cisplatin-induced nephrotoxicity via the microRNA-34a/sirtuin 1 signalling pathway. Br J Pharmacol (2017) 174(15):2512–27. doi: 10.1111/bph.13862
156. Qiao Y, Xu L, Tao X, Yin L, Qi Y, Xu Y, et al. Protective effects of dioscin against fructose-induced renal damage via adjusting Sirt3-mediated oxidative stress, fibrosis, lipid metabolism and inflammation. Toxicol Lett (2018) 284:37–45. doi: 10.1016/j.toxlet.2017.11.031
157. Cai S, Chen J, Li Y. Dioscin protects against diabetic nephropathy by inhibiting renal inflammation through TLR4/NF-κB pathway in mice. Immunobiology (2020) 225(3):151941. doi: 10.1016/j.imbio.2020.151941
158. Li Y, Gao M, Yin LH, Xu LN, Qi Y, Sun P, et al. Dioscin ameliorates methotrexate-induced liver and kidney damages via adjusting miRNA-145-5p-mediated oxidative stress. Free Radic Biol Med (2021) 169:99–109. doi: 10.1016/j.freeradbiomed.2021.03.035
159. Zhang S, Xu Y, Ye M, Ye W, Xiao J, Zhou H, et al. Resveratrol in liquor exacerbates alcoholic liver injury with a reduced therapeutic effect in mice: An unsupervised herbal wine habit is risky. Nutrients (2022) 14(22). doi: 10.3390/nu14224752
160. Tang YW, Yang RC, Wan F, Tang XL, Zhang HQ, Lin Y. Celastrol attenuates renal injury in 5/6 nephrectomized rats via inhibiting epithelial-mesenchymal transition and transforming growth factor-β1/Smad3 pathway. Exp Biol Med (Maywood) (2022) 247(21):1947–55. doi: 10.1177/15353702221118087
161. Wu Y, Xu S, Tian XY. The effect of salvianolic acid on vascular protection and possible mechanisms. Oxid Med Cell Longev (2020) 2020:5472096. doi: 10.1155/2020/5472096
162. Qian W, Wang Z, Xu T, Li D. Anti-apoptotic effects and mechanisms of salvianolic acid a on cardiomyocytes in ischemia-reperfusion injury. Histol. Histopathol (2019) 34(3):223–31. doi: 10.14670/HH-18-048
163. Zhang M, Qian C, Zheng ZG, Qian F, Wang Y, Thu PM, et al. Jujuboside a promotes aβ clearance and ameliorates cognitive deficiency in alzheimer's disease through activating Axl/HSP90/PPARγ pathway. Theranostics (2018) 8(15):4262–78. doi: 10.7150/thno.26164
164. Yang Y, Hong M, Lian WW, Chen Z. Review of the pharmacological effects of astragaloside IV and its autophagic mechanism in association with inflammation. World J Clin cases (2022) 10(28):10004–16. doi: 10.12998/wjcc.v10.i28.10004
165. Miao M, Yu WQ, Li Y, Sun YL, Guo SD. Structural elucidation and activities of cordyceps militaris-derived polysaccharides: A review. Front Nutr (2022) 9:898674. doi: 10.3389/fnut.2022.898674
166. Hu Z, Wang J, Jin L, Zong T, Duan Y, Sun J, et al. Preparation, characterization and anti-complementary activity of three novel polysaccharides from cordyceps militaris. Polymers (Basel) (2022) 14(21). doi: 10.3390/polym14214636
167. Liang M, Meng Y, Wang X, Wang L, Tang G, Wang W. The effectiveness of wogonin on treating cough mice with mycoplasma pneumoniae infection. Front Mol Biosci (2022) 9:803842. doi: 10.3389/fmolb.2022.803842
168. Li X, Cai W, Lee K, Liu B, Deng Y, Chen Y, et al. Puerarin attenuates diabetic kidney injury through the suppression of NOX4 expression in podocytes. Sci Rep (2017) 7(1):14603. doi: 10.1038/s41598-017-14906-8
169. Chen Z, Zhu G, Sheng C, Lei J, Song S, Zhu J. Hispidulin enhances temozolomide (TMZ)-induced cytotoxicity against malignant glioma cells In vitro by inhibiting autophagy. Comput Intell Neurosci (2022) 2022:5266770. doi: 10.1155/2022/5266770
170. Kim K, Leem J. Hispidulin ameliorates endotoxin-induced acute kidney injury in mice. Molecules (2022) 27(6). doi: 10.3390/molecules27062019
171. Ashaq A, Maqbool MF, Maryam A, Khan M, Shakir HA, Irfan M, et al. Hispidulin: A novel natural compound with therapeutic potential against human cancers. Phytother. Res (2021) 35(2):771–89. doi: 10.1002/ptr.6862
172. Chen H, Deng C, Meng Z, Meng S. Effects of catalpol on alzheimer's disease and its mechanisms. Evid Based Complement Alternat Med (2022) 2022:2794243. doi: 10.1155/2022/2794243
173. Bhattamisra SK, Koh HM, Lim SY, Choudhury H, Pandey M. Molecular and biochemical pathways of catalpol in alleviating diabetes mellitus and its complications. Biomolecules (2021) 11(2). doi: 10.3390/biom11020323
174. Liu P, Zhang J, Wang Y, Shen Z, Wang C, Chen DQ, et al. The active compounds and therapeutic target of tripterygium wilfordii hook. f. in attenuating proteinuria in diabetic nephropathy: A review. Front Med (Lausanne) (2021) 8:747922. doi: 10.3389/fmed.2021.747922
175. Shi H, Deng P, Dong C, Lu R, Si G, Yang T. Quality of evidence supporting the role of tripterygium glycosides for the treatment of diabetic kidney disease: An overview of systematic reviews and meta-analyses. Drug Des Devel Ther (2022) 16:1647–65. doi: 10.2147/DDDT.S367624
176. Wu X, Huang Y, Zhang Y, He C, Zhao Y, Wang L, et al. Efficacy of tripterygium glycosides combined with ARB on diabetic nephropathy: a meta-analysis. Biosci Rep (2020) 40(11). doi: 10.1042/BSR20202391
177. Emami E, Heidari-Soureshjani S, Sherwin CM. Anti-inflammatory response to curcumin supplementation in chronic kidney disease and hemodialysis patients: A systematic review and meta-analysis. Avicenna J Phytomed (2022) 12(6):576–88. doi: 10.22038/AJP.2022.20049
178. Shang D, Li Z, Tan X, Liu H, Tu Z. Inhibitory effects and molecular mechanisms of ginsenoside Rg1 on the senescence of hematopoietic stem cells. Fundam Clin Pharmacol (2022). doi: 10.1111/fcp.12863
179. Ataei S, Kesharwani P, Sahebkar A. Berberine: Ins and outs of a nature-made PCSK9 inhibitor. EXCLI J (2022) 21:1099–110. doi: 10.17179/excli2022-5234
180. Cui Y, Jiang X, Feng J. The therapeutic potential of triptolide and celastrol in neurological diseases. Front Pharmacol (2022) 13:1024955. doi: 10.3389/fphar.2022.1024955
181. Tanveer MA, Rashid H, Nazir LA, Archoo S, Shahid NH, Ragni G, et al. Trigonelline, a plant derived alkaloid prevents ultraviolet-b-induced oxidative DNA damage in primary human dermal fibroblasts and BALB/c mice via modulation of phosphoinositide 3-kinase-Akt-Nrf2 signalling axis. Exp Gerontol (2022), 112028. doi: 10.1016/j.exger.2022.112028
182. Zhang XX, Zuo JQ, Wang YT, Duan HY, Yuan JH, Hu YH. Paeoniflorin in paeoniaceae: Distribution, influencing factors, and biosynthesis. Front Plant Sci (2022) 13:980854. doi: 10.3389/fpls.2022.980854
183. Ding S, Lu G, Wang B, Xiang J, Hu C, Lin Z, et al. Astilbin activates the reactive oxidative Species/PPARγ pathway to suppress effector CD4(+) T cell activities via direct binding with cytochrome P450 1B1. Front Pharmacol (2022) 13:848957. doi: 10.3389/fphar.2022.848957
184. Wang M, Yang X, Zhou Q, Guo Y, Chen Y, Song L, et al. Neuroprotective mechanism of icariin on hypoxic ischemic brain damage in neonatal mice. Oxid Med Cell Longev (2022) 2022:1330928. doi: 10.1155/2022/1330928
185. Wang M, Wang L, Zhou Y, Feng X, Ye C, Wang C. Icariin attenuates renal fibrosis in chronic kidney disease by inhibiting interleukin-1β/transforming growth factor-β-mediated activation of renal fibroblasts. Phytother. Res (2021) 35(11):6204–15. doi: 10.1002/ptr.7256
186. Zhou YD, Hou JG, Yang G, Jiang S, Chen C, Wang Z, et al. Icariin ameliorates cisplatin-induced cytotoxicity in human embryonic kidney 293 cells by suppressing ROS-mediated PI3K/Akt pathway. Biomed Pharmacother (2019) 109:2309–17. doi: 10.1016/j.biopha.2018.11.108
187. Qi MY, He YH, Cheng Y, Fang Q, Ma RY, Zhou SJ, et al. Icariin ameliorates streptozocin-induced diabetic nephropathy through suppressing the TLR4/NF-κB signal pathway. Food Funct (2021) 12(3):1241–51. doi: 10.1039/d0fo02335c
188. Zhu Y, Li X, Da C, Liang P, Jin S, Tang C. Effects of cyclocarya paliurus (Batal.) extracts on oxidative stability and sensory quality in meat products (Frankfurters). Foods (2022) 11(22). doi: 10.3390/foods11223721
189. Liu Y, Zhang XX, Xu SS, Fang SY, Zhu LP, Song Z, et al. New triterpenoids from the cyclocarya paliurus (Batalin) iljinskaja and their anti-fibrotic activity. Phytochemistry (2022) 204:113434. doi: 10.1016/j.phytochem.2022.113434
190. Fu B, Shang Z, Song S, Xu Y, Wei L, Li G, et al. Adverse reactions of niaoduqing granules: A systematic review and meta-analysis. Phytomedicine (2022) 109:154535. doi: 10.1016/j.phymed.2022.154535
191. Gao Y, Guo Z, Liu Y. Analysis of the potential molecular biology of triptolide in the treatment of diabetic nephropathy: A narrative review. Med (Baltimore) (2022) 101(48):e31941. doi: 10.1097/MD.0000000000031941
Keywords: diabetic kidney disease (DKD), autophagy, Chinese medicine, active compounds, herbal extracts
Citation: Liu P, Zhu W, Wang Y, Ma G, Zhao H and Li P (2023) Chinese herbal medicine and its active compounds in attenuating renal injury via regulating autophagy in diabetic kidney disease. Front. Endocrinol. 14:1142805. doi: 10.3389/fendo.2023.1142805
Received: 12 January 2023; Accepted: 17 February 2023;
Published: 03 March 2023.
Edited by:
Xuefei Tian, Yale University, United StatesReviewed by:
Yue Tu, Nanjing University of Chinese Medicine, ChinaLi Wang, Southwest Medical University, China
De Jin, China Academy of Chinese Medical Sciences, China
Copyright © 2023 Liu, Zhu, Wang, Ma, Zhao and Li. This is an open-access article distributed under the terms of the Creative Commons Attribution License (CC BY). The use, distribution or reproduction in other forums is permitted, provided the original author(s) and the copyright owner(s) are credited and that the original publication in this journal is cited, in accordance with accepted academic practice. No use, distribution or reproduction is permitted which does not comply with these terms.
*Correspondence: Hailing Zhao, zhly0167@163.com; Ping Li, lp8675@163.com