- 1Department of Neurosurgery, China Pituitary Disease Registry Center, Peking Union Medical College Hospital, Peking Union Medical College & Chinese Academy of Medical Sciences, Beijing, China
- 2Medical Research Center, Peking Union Medical College Hospital, Chinese Academy of Medical Sciences, Beijing, China
Exosomes are small extracellular vesicles that carry various bioactive molecules including various RNAs that modulate the activities of recipient cells. It has drawn considerable attention as means of cell communication and drug delivery. Exosome plays important role in various tumors, but it is rarely summarized in pituitary adenoma (PA). PA is the second most common primary central nervous system tumor, and its recurrence and persistent postoperative hormone hypersecretion lead to compromised quality of life. How exactly exosomes impact tumor development and hormone secretion is important for the development of this tumor diagnosis and treatment. In this review, we discuss how exosomal RNAs impact PAs and their potential as future clinical therapies. In our literature review, first, we found that exosomal microRNA hsa-miR-1180-3p is a potential early biomarker for NFPAs. Since NFPAs are typically difficult to diagnose, this is an especially important finding. Second, exosomal protein transcripts are potential invasive biomarker, such as MMP1, N-cadherin, CDK6, RHOU, INSM1, and RASSF10. Third, exosomal contents such as hsa-miR-21-5p promote distant bone formation of GHPA patients. Fourth, tumor suppressors in the exosome constitute novel therapeutic application of exosome, including long noncoding RNA (lncRNA) H19, miR-149-5p, miR-99a-3p, and miR-423-5p. This review discusses the possible mechanisms of exosome and their contents in PA and promotes the use of exosomes in both clinical diagnosis and treatment of this tumor.
Introduction
Pituitary adenoma (PA) is a common type of brain tumor that accounts for approximately 17.2% of intracranial tumors (1, 2). These tumors are classified according to the hormone they excessively secrete. Accordingly, they include prolactin-secreting pituitary adenoma (PRLPA), non-secreting pituitary adenoma (NFPA), growth hormone-secreting pituitary adenoma (GHPA), as well as adrenocorticotropic hormone-secreting pituitary adenoma (ACTHPA) and other types of PA. Treatments for PAs include surgery, radiotherapy, somatostatin analogues, growth hormone antagonists or dopamine agonist (3–5). However, these treatments are challenging due to development of drug resistance, lack of treatment targets, and limited in-depth understanding of the molecular mechanisms responsible for the development of these tumors (3, 5). Various molecules are secreted by cells via different types of extracellular vesicles, including microvesicles, apoptotic bodies, and exosomes. Recently, exosomes have drawn considerable attention as a means of intercellular communication because they carry bioactive molecules that can modulate the activities of recipient cells (6, 7). Additionally, exosomes are promising biomarkers and vehicles for drug delivery (Figure 1) (8, 9). Exosomes are small extracellular vesicles with average diameters of 40–150 nm. They are secreted by a wide variety of cells and have been detected in nearly all body fluids, including blood, urine, cerebrospinal fluid, and saliva (8, 9). The secretory quantity and content of exosomes can vary according to their biogenesis, cell of origin, and physiological or pathological cell status. Among these, noncoding RNAs (ncRNAs) are enriched and stable in exosomes and are important owing to their regulatory function in tumor initiation and progression, including in PA (10, 11).
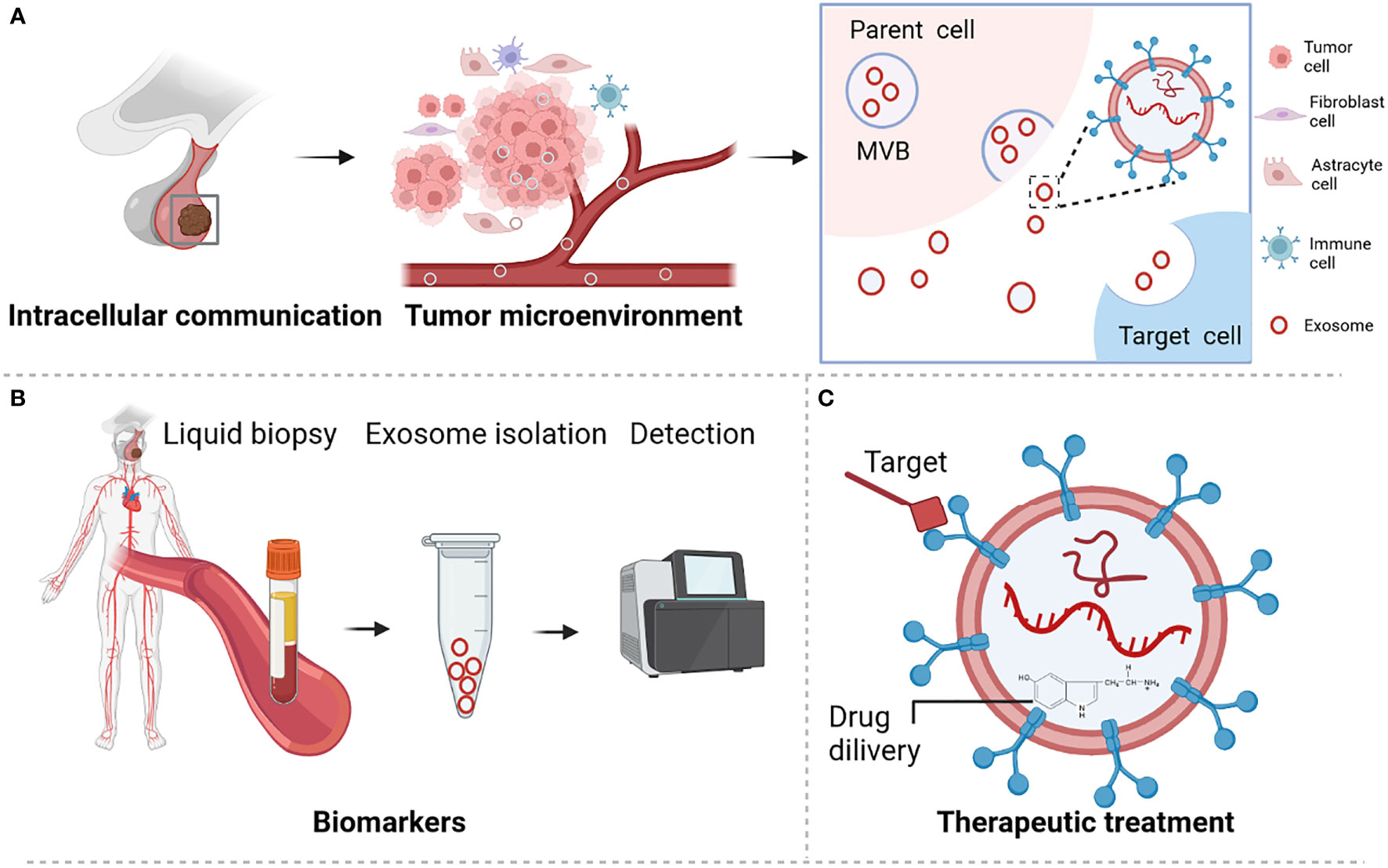
Figure 1 Role and functions of exosome in pituitary adenoma. (A) Exosome participates in microenvironment regulation and cell communication. (B) Exosome served as biomarker for PA disease. (C) Exosome is a promising novel treatment therapy. Figures are created in BioRender.com.
Biogenesis and characteristics of exosomes
Exosome biogenesis begins at the cell membrane and is connected to endosomal uptake and form the early endosomes. Then, multivesicular bodies (MVBs) formation through inward budding of early endosomes and selected contents including RNAs, proteins or lipid. Finally, the MVB was fused with cell membrane leading to the release of exosome, or fuse with lysosome result in the degradation of exosome. During this process, MVBs are generated through two distinct mechanisms. One pathway involves a large multi-subunit protein complex called the endosomal sorting complex required for transport (ESCRT) mechanism, whereas the second pathway is an ESCRT-independent mechanism. ESCRT is an important mechanism of MVB synthesis as it guides the packaging of molecules within the exosomes of MVBs (12). Usually, MVBs form in an ESCRT-independent manner (13). However, the mechanism of ESCRT-independent exosome formation and its regulation in PA remain largely unknown.
Given their cell-specific uptake by extracellular receptors and their ubiquity in cell signaling, exosomes are suitable candidates as disease biomarkers and drug delivery vehicles (8). Next, we discuss the types of molecules identified in exosomes and their potential roles in PAs. These molecules include proteins, lipids, and nucleic acids, among which ncRNAs are more abundant in exosomes (14). In this review, we summarized the current understanding of the role of exosomal RNA and more specifically of ncRNAs, in relation to PA progression and its potential clinical applications.
Background of noncoding RNA in PAs
Although mRNAs were reported to play important role in tumor genesis, tumor development and treatment of PAs, ncRNAs which are enriched in exosomes have drawn more attention than mRNAs, because ncRNAs are usually much shorter and are easier to be contained in exosomes. Exosomes usually contain different types of ncRNAs, including microRNAs (miRNAs), long noncoding RNAs (lncRNAs), circular RNAs (circRNAs), and other ncRNAs. Different types of ncRNAs were all reported to be involved in tumor disorders (15, 16) (Figure 2).
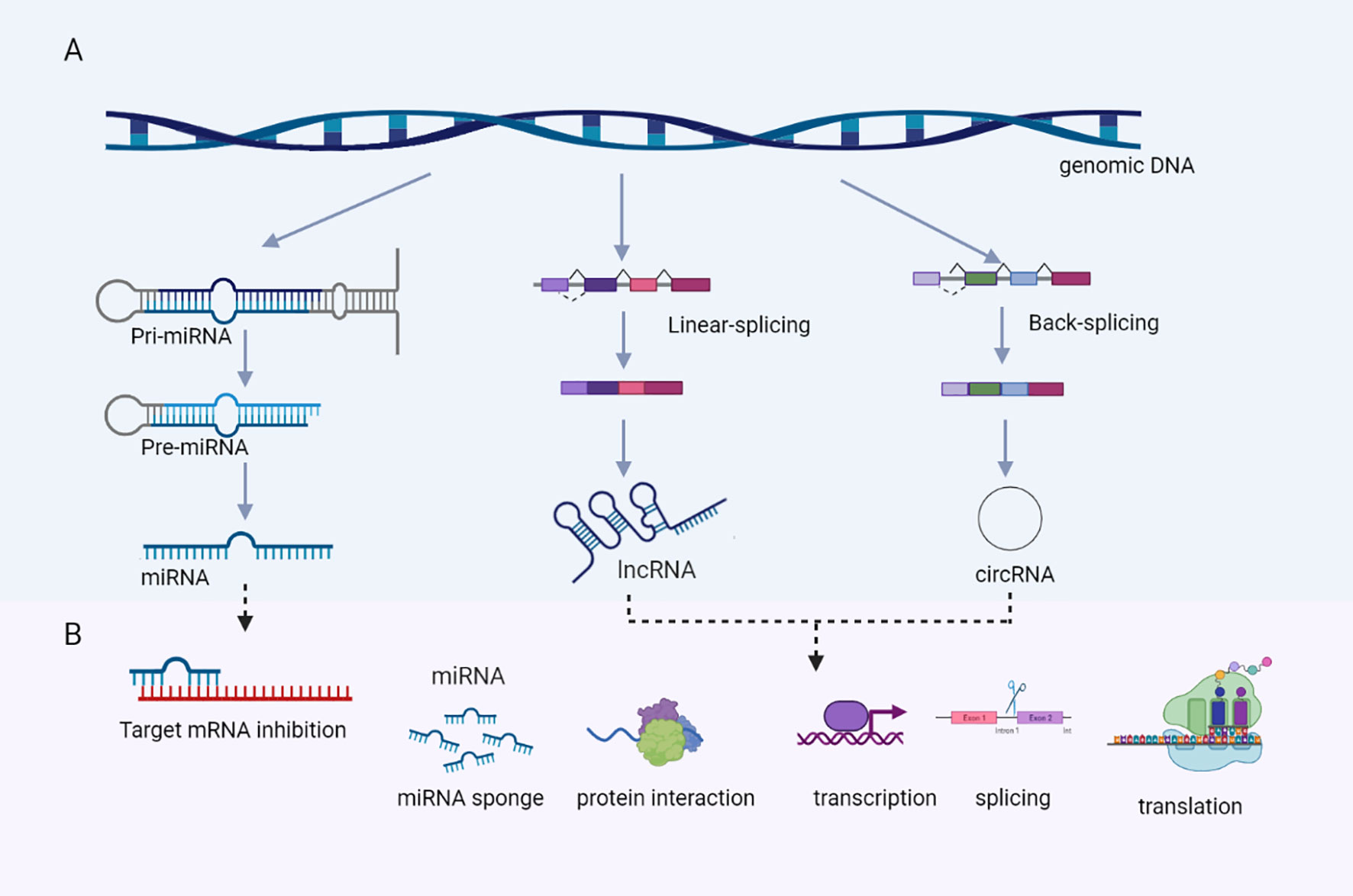
Figure 2 Genesis (A) and biological functions (B) of ncRNAs, including miRNA, lncRNA, and circRNA. Figures are created in BioRender.com.
MicroRNA in PAs
miRNAs are small ncRNA molecules that consist of approximately 21–25 nucleotides (17). The pri-miRNA is cleaved into precursor miRNA (called pre-miRNA) which is an 85-nucleotide stem-loop structure by a complex containing DGCR8 protein. Then Dicer processes hairpin structured precursor miRNA into mature miRNA (18). These mature miRNAs repress gene expression by binding to 3′ untranslated regions of target mRNAs, through which they impair mRNA stability and/or inhibit mRNA translation (17).
In PAs, miRNA expression is associated with PA tumorigenesis and treatment resistance. For example, AIP expression is negatively correlated with miR-34a in GHPAs, where elevated miR-34a levels promote the resistance to octreotide (19–22). Differentially expressed miRNAs have recently been discovered identified in exosomes of PA patients, suggesting that miRNAs are selectively sorted into exosomes participating in tumor progression, invasion, and non-hormonal action. Thus, these miRNAs represent potential markers for the diagnosis or treatment of PAs (23).
Long noncoding RNA in PAs
LncRNA is noncoding RNAs that are longer than 200 nucleotides (24). LncRNA modulates gene expression through multiple mechanisms: (1) precursor of small RNAs such as miRNA (2) interaction with regulatory protein. (3) cis and/or in trans regulation of transcription (4) regulation of mRNA alternative splicing (5) lncRNAs can occasionally encode small peptides (25).
LncRNAs in PAs involves in hormone secretion (26), tumor suppress (27), tumor progress (28), recurrence prediction (29), as well as tumor invasion (30, 31). Furthermore, dysregulation of lncRNA expression has been observed in exosomes of bone-invading PAs. Hence, exosomal lncRNAs should be explored as biomarkers for both diagnosis and prognosis (32).
Circular RNA in PAs
Circular RNA (circRNA) owes its name to its closed-loop structure. CircRNAs were produced from corresponding pre-mRNAs via back-splicing (33),. These circRNAs regulate genes via two pathways: (1) by acting as a miRNA “sponges”, thereby affecting miRNA target genes; (2) by binding to specific RNA-binding proteins and impacting their functions (34, 35). A small part of circRNAs also could encode small peptides.
Interestingly, circRNAs are more abundant in the brain compared to other organs, thereby indicating that circRNA is important for brain function and brain diseases (36). Furthermore, many studies have shown that circRNAs are aberrantly expressed in PAs and are linked to proliferation (37), progression (38), invasion (39) and GH secretion in pituitary somatotroph adenoma (40). Several studies have identified circRNA in exosomes (41, 42). Notably, these studies showed that these circRNAs initiate their circulation process inside exosomes before reaching their respective recipient cells, where they carry out various biological functions (41, 43). Unlike linear RNAs, circRNAs are more suitable as biomarkers because their loop structures remain stable for a longer half-life (44, 45). However, the role of exosomal circRNA in PA is largely unknown. Therefore, investigation of exosomal circRNA in PA is a potential direction for PA diagnosis and treatment therapy.
Potential clinical applications of exosomal RNA in pituitary adenoma
Since there is a part of PAs exhibit increased cell proliferation, invasive propensities, excess secretion of various hormones, and even drug resistance. However, the mechanisms that underlie these behaviors remain unclear. Increasing evidence suggests that exosomes increase pituitary tumor progression via transfer of bioactive molecules between different cell populations (24, 46). As important exosomal components, RNAs, especially ncRNAs play key roles in multiple aspects of tumor biology, including proliferation, migration, and tumor microenvironment (10). In the following sections, we summarize the current understanding of the specific roles and mechanisms of exosomal RNAs in the progression of PAs (Table 1).
Exosomal biomarkers of NFPAs
Early diagnosis for NFPAs
Exosome is assumed to be a novel biomarker of NFPA for early diagnosis (Figure 3A). NFPA is one of the most common pituitary adenomas. Critically, complete resection has shown to be particularly difficult for larger and more aggressive tumors. Hence, early diagnosis is key to prevent recurrence. However, the lack of reliable screening approach for NFPAs is an urgent problem to be solved. To screen for NFPA and make prognostic predictions, Lyu et al. developed a method based on serum exosomal miRNA profiling (47). In that study, hsa-miR-486-5p, hsa-miR-151a-5p, hsa-miR-652-3p_R+1, and hsa-miR-1180-3p were identified as promising biomarkers for NFPA. Of those miRNAs, miR-486-5p is assumed to be the most accurate as an efficient biomarker for the prediction of progression and relapse among NFPA patients. Notably, this miRNA relates to tumor progression by epigenetically regulating MAPK signaling pathways (47).
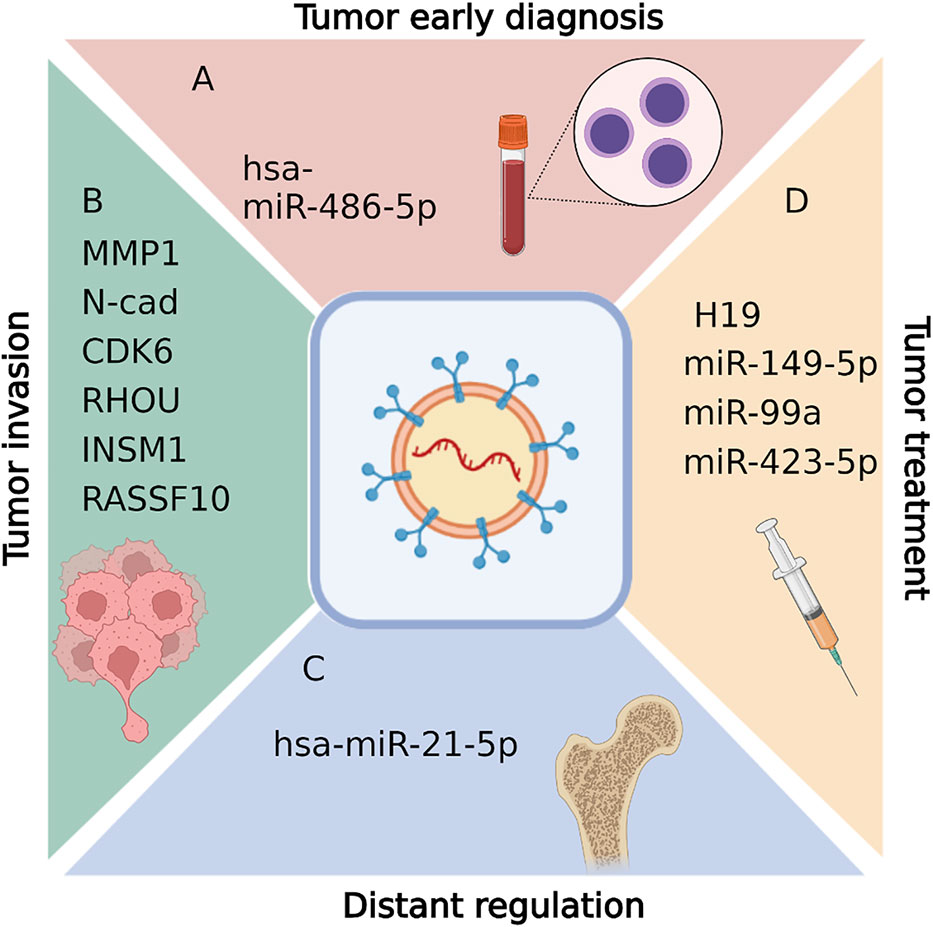
Figure 3 Biological functions of exosomal RNAs in pituitary adenomas, including tumor early diagnosis (A), tumor invasion diagnosis (B),distant regulation (C), and tumor treatment (D). Figures are created in BioRender.com.
Diagnosis for tumor invasion in NFPAs
Exosome also act as biomarkers in invasive PAs (Figure 3B). Tumor invasion into adjacent tissue is the main reason for PA recurrence, as invasion makes complete resection during surgery incredibly challenging. Thus, it is vital to understand tumor aggressive mechanisms and identify treatments that reduce invasion and improve PA surgical remission rates.
Several studies have identified different transcripts in exosomes as biomarkers for invasive PAs. It was reported that matrix metalloproteinase-1 (MMP1) (55), N-cadherin (48), E-cadherin (48), Epcam (48), CDK6 (49), RHOU (49), INSM1 (50) and RASSF10 (51) within the serum exosomes are elevated in invasive NFPA patients compared with noninvasive NFPA patients. Those disordered RNAs from exosome are potential diagnostic biomarkers for invasive PAs. In addition, the underlying regulation mechanisms are involved in cell migration (55), angiogenesis (55), epithelial-mesenchymal transition (48), and tumor microenvironment regulations (51). For example, enrichment of MMP1 regulates the protease-activated receptor-1 signaling pathway, stimulating tumor migration, growth, and angiogenesis in PAs (55). Reduced expression of RASSF10 can promote MDM2 expression, impacting exosome secretion and therefore regulating the tumor microenvironment (51).
Together, those findings support the predictive value of MMP1, INSM1, CDK6, RHOU, RASSF10, and EMT-related marker mRNA expression in exosomes as in situ or circulating biomarkers for patients with invasive NFPA.
Exosomal ncRNAs in disease regulation of hormone-secreting PA
Distant regulations caused by GHPAs
GHPAs usually result in disorders of organs distant from pituitary, which lead to clinical symptoms such as overgrowth of tissue, bone and multiple organs. Here we call it distant regulation of GHPA. In traditional concept, GH and insulin-like growth factor-1 (IGF-1) overproduction is the only reason for the distant disorders of GHPA patients. However, recently exosome was also indicated to exhibit distant regulations in GHPA disease progress (Figure 3C). Hyperostosis is one of the most common manifestations of acromegaly, which results from GH and insulin-like growth factor-1 (IGF-1) overproduction. However, current therapies focusing on the GH/IGF-1 axis are only effective in approximately 28%-50% of patients with acromegaly, while the remaining 50%-72% continue to experience excessive bone growth and/or abnormal bone metabolism, even after GHPA reduction or GH/IGF-1 level normalization (56–59).
Xiong et al. found that in vitro, GHPA exosomes promote bone formation, and that in vivo they increase the number of trabeculae and GHPA exosome-induced osteoblast proliferation via increased cell viability and DNA replication (54). Furthermore, they showed that exosomal hsa-miR-21-5p plays an important role in this process (which is distinct from that of the GH/IGF-1 axis), providing a novel mechanism for acromegaly development.
Potential treatment therapy for hormone-secreting PAs
Several groups have reported tumor-inhibiting ncRNAs and potential tumor treatment therapy in PAs (Table 1; Figure 3D).
PRLPAs
Drug treatment is the first-line treatment therapy for PRLPA patients. However, some patients suffer from drug resistance. Exosome carrying tumor suppressors could provide a potential therapy for those patients. Wu et al. reported that H19 acts as a tumor suppressor in various tumors including PRLPAs (24, 60, 61). Importantly, lncRNA H19 levels in exosomes were dramatically correlated with the prognostic outcome of PRLPA patients. Furthermore, exosomal H19 and cabergoline treatment was shown to exhibit synergistic therapeutic effects (52). Mechanistically, H19 was shown to inhibit 4E-BP1 phosphorylation (52). In addition, a recent study by Zhao et al. reported that both miR-149 and miR-99a-3p present in exosomes showed suppressive effects on in vitro cell viability, metastasis, tube formation ability, and on in vivo tumor growth and angiogenesis (46). Mechanistically, exosome-driven miR-99a-3p inhibited PA cell growth via regulating the expression of Nova1, Dtl, and Rab27b (46). Based on these findings, since exosome is a promising delivery, we propose that those exosomal ncRNAs constitute suitable candidates as tumor inhibitors to treat patients with PRLPAs.
GHPAs
Pituitary adenoma is a common benign tumor. Interestingly, Zhou et al. reported that exosomes of GHPA cell line treated colon cancer cell line (HCT116 cell line) showed decreased malignancy in vitro and decreased tumor metastasis in HCT116 xenografted nude mice (11). This decreased malignancy occurs proposed through altered gene expression in the p53 and MAPK pathways (11). In addition, Zhao et al. indicates that low miR-423-5p levels promote tumorigenesis in somatotropic adenomas (53). Hence, exosomes containing inhibitory lncRNA or miRNAs disrupt PA malignancy. Based on these findings, exosomal ncRNAs constitute suitable candidates as tumor inhibitors to treat patients with GHPAs or some malignancy tumors.
Conclusion
The studies of exosomal RNAs expand our understanding of the regulatory mechanisms in PAs. As exosome purification and detection technology developed in the past decade, it become easier to understand the precise contributions of exosomal RNAs in tumor genesis and development. In this review, we highlight the exosomal RNA functions as early diagnosis markers or invasive biomarkers in NFPAs. Since NFPAs are typically difficult to diagnose, this is an especially important finding. In addition, exosomal RNAs play distant regulation of bone formation in GHPAs. This propose novel insight which is different from traditional concept; Finally, tumor suppressor RNAs detected in exosome could be therapeutic applications. Since exosomes are promising vehicles for drug delivery, those RNAs detected in PA exosome propose important clinical application in the future. Although current investigations on exosome in PAs is limited, and it has not been fully translated into clinical applications. However, the current detections offer novel ideas and perspectives on the progress and treatment of PAs. The involved exosomal RNAs can also be exploited as reliable diagnostic biomarkers and therapeutic therapy in PA. Those investigations largely promote the use of exosome and their contents in both clinical diagnosis and treatment of this tumor.
Author contributions
XB, RW, MF, and PL conceived of the project. MC wrote the manuscript. SJ, XG, PL, MF and JG modified the manuscript. All authors have read and approved the final version of manuscript. All authors contributed to the article and approved the submitted version.
Funding
This work was supported by the National Key R&D Program of China (2021YFE0114300 to RW), National Natural Science Foundation of China (82171475 to RW, 82170799 to XB, 82103302 to MC, 82203527 to XG), China Postdoctoral Science Foundation (BX20190045 to MC, 2022M710451 to XG), and the Fundamental Research Funds for the Central Universities (3332022009 to XG).
Acknowledgments
We thank Dr. T. Juelich (University of the Chinese Academy of Sciences) for linguistic assistance during the preparation of this manuscript. The authors thank the Wang lab for the critical discussion of our manuscript.
Conflict of interest
The authors declare that the research was conducted in the absence of any commercial or financial relationships that could be construed as a potential conflict of interest.
Publisher’s note
All claims expressed in this article are solely those of the authors and do not necessarily represent those of their affiliated organizations, or those of the publisher, the editors and the reviewers. Any product that may be evaluated in this article, or claim that may be made by its manufacturer, is not guaranteed or endorsed by the publisher.
References
1. Ostrom QT, Gittleman H, Liao P, Rouse C, Chen Y, Dowling J, et al. CBTRUS statistical report: primary brain and central nervous system tumors diagnosed in the united states in 2007-2011. Neuro Oncol (2014) 16 Suppl 4:iv1–63. doi: 10.1093/neuonc/nou223
2. Ostrom QT, Price M, Neff C, Cioffi G, Waite KA, Kruchko C, et al. CBTRUS statistical report: Primary brain and other central nervous system tumors diagnosed in the united states in 2015-2019. Neuro Oncol (2022) 24:v1–v95. doi: 10.1093/neuonc/noac202
3. Melmed S. Medical progress: Acromegaly. N Engl J Med (2006) 355:2558–73. doi: 10.1056/NEJMra062453
4. Kopchick JJ, Parkinson C, Stevens EC, Trainer PJ. Growth hormone receptor antagonists: discovery, development, and use in patients with acromegaly. Endocr Rev (2002) 23:623–46. doi: 10.1210/er.2001-0022
5. Melmed S. Acromegaly pathogenesis and treatment. J Clin Invest (2009) 119:3189–202. doi: 10.1172/JCI39375
6. Raposo G, Stahl PD. Extracellular vesicles: a new communication paradigm? Nat Rev Mol Cell Biol (2019) 20:509–10. doi: 10.1038/s41580-019-0158-7
7. Tkach M, Théry C. Communication by extracellular vesicles: Where we are and where we need to go. Cell (2016) 164:1226–32. doi: 10.1016/j.cell.2016.01.043
8. Jiang L, Gu Y, Du Y, Liu J. Exosomes: Diagnostic biomarkers and therapeutic delivery vehicles for cancer. Mol Pharm (2019) 16:3333–49. doi: 10.1021/acs.molpharmaceut.9b00409
9. van Niel G, D'Angelo G, Raposo G. Shedding light on the cell biology of extracellular vesicles. Nat Rev Mol Cell Biol (2018) 19:213–28. doi: 10.1038/nrm.2017.125
10. Xie Y, Dang W, Zhang S, Yue W, Yang L, Zhai X, et al. The role of exosomal noncoding RNAs in cancer. Mol Cancer (2019) 18:37. doi: 10.1186/s12943-019-0984-4
11. Zhou C, Shen S, Moran R, Deng N, Marbán E, Melmed S. Pituitary somatotroph adenoma-derived exosomes: Characterization of nonhormonal actions. J Clin Endocrinol Metab (2022) 107:379–97. doi: 10.1210/clinem/dgab651
12. Wollert T, Hurley JH. Molecular mechanism of multivesicular body biogenesis by ESCRT complexes. Nature (2010) 464:864–9. doi: 10.1038/nature08849
13. Shao H, Im H, Castro CM, Breakefield X, Weissleder R, Lee H. New technologies for analysis of extracellular vesicles. Chem Rev (2018) 118:1917–50. doi: 10.1021/acs.chemrev.7b00534
14. Jeppesen DK, Fenix AM, Franklin JL, Higginbotham JN, Zhang Q, Zimmerman LJ, et al. Reassessment of exosome composition. Cell (2019) 177:428–445.e18. doi: 10.1016/j.cell.2019.02.029
15. Li B, Hong J, Hong M, Wang Y, Yu T, Zang S, et al. piRNA-823 delivered by multiple myeloma-derived extracellular vesicles promoted tumorigenesis through re-educating endothelial cells in the tumor environment. Oncogene (2019) 38:5227–38. doi: 10.1038/s41388-019-0788-4
16. Nie JH, Li TX, Zhang XQ, Liu J. Roles of non-coding RNAs in normal human brain development, brain tumor, and neuropsychiatric disorders. Noncoding RNA (2019) 5(2):36. doi: 10.3390/ncrna5020036
17. Lee RC, Feinbaum RL, Ambros V. The c. elegans heterochronic gene lin-4 encodes small RNAs with antisense complementarity to lin-14. Cell (1993) 75:843–54. doi: 10.1016/0092-8674(93)90529-Y
18. Peng Y, Croce CM. The role of MicroRNAs in human cancer. Signal Transduct Target Ther (2016) 1:15004. doi: 10.1038/sigtrans.2015.4
19. Lee YJ, Cho JM, Moon JH, Ku CR, Kim J, Kim SH, et al. Increased miR-338-3p expression correlates with invasiveness of GH-producing pituitary adenomas. Endocrine (2017) 58:184–9. doi: 10.1007/s12020-017-1390-6
20. Mao ZG, He DS, Zhou J, Yao B, Xiao WW, Chen CH, et al. Differential expression of microRNAs in GH-secreting pituitary adenomas. Diagn Pathol (2010) 5:79. doi: 10.1186/1746-1596-5-79
21. Palumbo T, Faucz FR, Azevedo M, Xekouki P, Iliopoulos D, Stratakis CA. Functional screen analysis reveals miR-26b and miR-128 as central regulators of pituitary somatomammotrophic tumor growth through activation of the PTEN-AKT pathway. Oncogene (2013) 32:1651–9. doi: 10.1038/onc.2012.190
22. Bogner EM, Daly AF, Gulde S, Karhu A, Irmler M, Beckers J, et al. miR-34a is upregulated in AIP-mutated somatotropinomas and promotes octreotide resistance. Int J Cancer (2020) 147:3523–38. doi: 10.1002/ijc.33268
23. Godlewski J, Krichevsky AM, Johnson MD, Chiocca EA, Bronisz A. Belonging to a network–microRNAs, extracellular vesicles, and the glioblastoma microenvironment. Neuro Oncol (2015) 17:652–62. doi: 10.1093/neuonc/nou292
24. Wu ZR, Yan L, Liu YT, Cao L, Guo YH, Zhang Y, et al. Inhibition of mTORC1 by lncRNA H19 via disrupting 4E-BP1/Raptor interaction in pituitary tumours. Nat Commun (2018) 9:4624. doi: 10.1038/s41467-018-06853-3
25. Kopp F, Mendell JT. Functional classification and experimental dissection of long noncoding RNAs. Cell (2018) 172:393–407. doi: 10.1016/j.cell.2018.01.011
26. Zhang W, Ren W, Han D, Zhao G, Wang H, Guo H, et al. LncRNA-m18as1 competitively binds with miR-18a-5p to regulate follicle-stimulating hormone secretion through the Smad2/3 pathway in rat primary pituitary cells. J Zhejiang Univ Sci B (2022) 23:502–14. doi: 10.1631/jzus.B2101052
27. Wang H, Wu B, Wang H, Jiang C, Liu Z. LncRNA growth arrest specific transcript 5 inhibits the growth of pituitary neuroendocrine tumors via miR-27a-5p/cylindromatosis axis. Bioengineered (2022) 13:10274–86. doi: 10.1080/21655979.2022.2062086
28. Wu H, Zhou S, Zheng Y, Pan Z, Chen Y, Wang X. LncRNA BBOX1-AS1 promotes pituitary adenoma progression via sponging miR-361-3p/E2F1 axis. Anticancer Drugs (2022) 33:652–62. doi: 10.1097/CAD.0000000000001309
29. Cheng S, Guo J, Wang D, Fang Q, Liu Y, Xie W, et al. A novel three-LncRNA signature predicting tumor recurrence in nonfunctioning pituitary adenomas. Front Genet (2021) 12:754503. doi: 10.3389/fgene.2021.754503
30. Li J, Qian Y, Zhang C, Wang W, Qiao Y, Song H, et al. LncRNA LINC00473 is involved in the progression of invasive pituitary adenoma by upregulating KMT5A via ceRNA-mediated miR-502-3p evasion. Cell Death Dis (2021) 12:580. doi: 10.1038/s41419-021-03861-y
31. Zhu HB, Li B, Guo J, Miao YZ, Shen YT, Zhang YZ, et al. LncRNA MEG8 promotes TNF-α expression by sponging miR-454-3p in bone-invasive pituitary adenomas. Aging (Albany NY) (2021) 13:14342–54. doi: 10.18632/aging.203048
32. Wang M, Zhou L, Yu F, Zhang Y, Li P, Wang K. The functional roles of exosomal long non-coding RNAs in cancer. Cell Mol Life Sci (2019) 76:2059–76. doi: 10.1007/s00018-019-03018-3
33. Chen LL. The biogenesis and emerging roles of circular RNAs. Nat Rev Mol Cell Biol (2016) 17:205–11. doi: 10.1038/nrm.2015.32
34. Ma S, Kong S, Wang F, Ju S. CircRNAs: biogenesis, functions, and role in drug-resistant tumours. Mol Cancer (2020) 19:119. doi: 10.1186/s12943-020-01231-4
35. Hansen TB, Jensen TI, Clausen BH, Bramsen JB, Finsen B, Damgaard CK, et al. Natural RNA circles function as efficient microRNA sponges. Nature (2013) 495:384–8. doi: 10.1038/nature11993
36. Rybak-Wolf A, Stottmeister C, Glažar P, Jens M, Pino N, Giusti S, et al. Circular RNAs in the mammalian brain are highly abundant, conserved, and dynamically expressed. Mol Cell (2015) 58:870–85. doi: 10.1016/j.molcel.2015.03.027
37. Zhang W, Chen S, Du Q, Bian P, Chen Y, Liu Z, et al. CircVPS13C promotes pituitary adenoma growth by decreasing the stability of IFITM1 mRNA via interacting with RRBP1. Oncogene (2022) 41:1550–62. doi: 10.1038/s41388-022-02186-0
38. Cheng J, Nie D, Li B, Gui S, Li C, Zhang Y, et al. CircNFIX promotes progression of pituitary adenoma via CCNB1 by sponging miR-34a -5p. Mol Cell Endocrinol (2021) 525:111140. doi: 10.1016/j.mce.2020.111140
39. Hu Y, Zhang N, Zhang S, Zhou P, Lv L, Richard SA, et al. Differential circular RNA expression profiles of invasive and non-invasive non-functioning pituitary adenomas: A microarray analysis. Med (Baltimore) (2019) 98:e16148. doi: 10.1097/MD.0000000000016148
40. Du Q, Zhang W, Feng Q, Hao B, Cheng C, Cheng Y, et al. Comprehensive circular RNA profiling reveals that hsa_circ_0001368 is involved in growth hormone-secreting pituitary adenoma development. Brain Res Bull (2020) 161:65–77. doi: 10.1016/j.brainresbull.2020.04.018
41. Wang Y, Liu J, Ma J, Sun T, Zhou Q, Wang W, et al. Exosomal circRNAs: biogenesis, effect and application in human diseases. Mol Cancer (2019) 18:116. doi: 10.1186/s12943-019-1041-z
42. Meng Q, Wang J, Cui J, Li B, Wu S, Yun J, et al. Prediction of COPD acute exacerbation in response to air pollution using exosomal circRNA profile and machine learning. Environ Int (2022) 168:107469. doi: 10.1016/j.envint.2022.107469
43. Lasda E, Parker R. Circular RNAs Co-precipitate with extracellular vesicles: A possible mechanism for circRNA clearance. PloS One (2016) 11:e0148407. doi: 10.1371/journal.pone.0148407
44. Li Y, Zheng Q, Bao C, Li S, Guo W, Zhao J, et al. Circular RNA is enriched and stable in exosomes: a promising biomarker for cancer diagnosis. Cell Res (2015) 25:981–4. doi: 10.1038/cr.2015.82
45. Tan S, Gou Q, Pu W, Guo C, Yang Y, Wu K, et al. Circular RNA f-circEA produced from EML4-ALK fusion gene as a novel liquid biopsy biomarker for non-small cell lung cancer. Cell Res (2018) 28:693–5. doi: 10.1038/s41422-018-0033-7
46. Zhao P, Cheng J, Li B, Nie D, Li C, Gui S, et al. Up-regulation of the expressions of MiR-149-5p and MiR-99a-3p in exosome inhibits the progress of pituitary adenomas. Cell Biol Toxicol (2021) 37:633–51. doi: 10.1007/s10565-020-09570-0
47. Lyu L, Li H, Chen C, Yu Y, Wang L, Yin S, et al. Exosomal miRNA profiling is a potential screening route for non-functional pituitary adenoma. Front Cell Dev Biol (2021) 9:771354. doi: 10.3389/fcell.2021.771354
48. Chen K, Li G, Kang X, Liu P, Qian L, Shi Y, et al. EMT-related markers in serum exosomes are potential diagnostic biomarkers for invasive pituitary adenomas. Neuropsychiatr Dis Treat (2021) 17:3769–80. doi: 10.2147/NDT.S339067
49. Yu S, Wang XS, Cao KC, Bao XJ, Yu J. Identification of CDK6 and RHOU in serum exosome as biomarkers for the invasiveness of non-functioning pituitary adenoma. Chin Med Sci J (2019) 34:168–76. doi: 10.24920/003585
50. Bao X, Wang G, Yu S, Sun J, He L, Zhao H, et al. Transcriptomic analysis identifies a tumor subtype mRNA classifier for invasive non-functioning pituitary neuroendocrine tumor diagnostics. Theranostics (2021) 11:132–46. doi: 10.7150/thno.47525
51. Wang T, Liu H, Ji Z, Cheng Y, Du Y, Liu H, et al. RASSF10 regulates bone invasion of growth hormone-secreting adenomas via exosomes. Biochem Biophys Res Commun (2020) 527:603–10. doi: 10.1016/j.bbrc.2020.04.131
52. Zhang Y, Liu YT, Tang H, Xie WQ, Yao H, Gu WT, et al. Exosome-transmitted lncRNA H19 inhibits the growth of pituitary adenoma. J Clin Endocrinol Metab (2019) 104:6345–56. doi: 10.1210/jc.2019-00536
53. Zhao S, Li J, Feng J, Li Z, Liu Q, Lv P, et al. Identification of serum miRNA-423-5p expression signature in somatotroph adenomas. Int J Endocrinol (2019) 2019:8516858. doi: 10.1155/2019/8516858
54. Xiong Y, Tang Y, Fan F, Zeng Y, Li C, Zhou G, et al. Exosomal hsa-miR-21-5p derived from growth hormone-secreting pituitary adenoma promotes abnormal bone formation in acromegaly. Transl Res (2020) 215:1–16. doi: 10.1016/j.trsl.2019.07.013
55. Ren Y, Wang Y, Bao X, Feng M, Xing B, Lian W, et al. Diagnosis of invasive non-functional pituitary adenomas using exosomal biomarkers. Clin Chim Acta (2022) 529:25–33. doi: 10.1016/j.cca.2022.01.014
56. Guo X, Wang K, Yu S, Gao L, Wang Z, Zhu H, et al. Quality of life and its determinants in patients with treated acromegaly: A cross-sectional nationwide study in China. J Clin Endocrinol Metab (2021) 106:211–25. doi: 10.1210/clinem/dgaa750
57. Claessen KM, Ramautar SR, Pereira AM, Smit JW, Roelfsema F, Romijn JA, et al. Progression of acromegalic arthropathy despite long-term biochemical control: a prospective, radiological study. Eur J Endocrinol (2012) 167:235–44. doi: 10.1530/EJE-12-0147
58. Claessen KM, Kroon HM, Pereira AM, Appelman-Dijkstra NM, Verstegen MJ, Kloppenburg M, et al. Progression of vertebral fractures despite long-term biochemical control of acromegaly: a prospective follow-up study. J Clin Endocrinol Metab (2013) 98:4808–15. doi: 10.1210/jc.2013-2695
59. Somm E, Bonnet N, Martinez A, Marks PM, Cadd VA, Elliott M, et al. A botulinum toxin-derived targeted secretion inhibitor downregulates the GH/IGF1 axis. J Clin Invest (2012) 122:3295–306. doi: 10.1172/JCI63232
60. Ren J, Ding L, Zhang D, Shi G, Xu Q, Shen S, et al. Carcinoma-associated fibroblasts promote the stemness and chemoresistance of colorectal cancer by transferring exosomal lncRNA H19. Theranostics (2018) 8:3932–48. doi: 10.7150/thno.25541
Keywords: pituitary adenoma, exosome, biomarker, noncoding RNA, treatment therapy
Citation: Chang M, Jiang S, Guo X, Gao J, Liu P, Bao X, Feng M and Wang R (2023) Exosomal RNAs in the development and treatment of pituitary adenomas. Front. Endocrinol. 14:1142494. doi: 10.3389/fendo.2023.1142494
Received: 11 January 2023; Accepted: 06 February 2023;
Published: 17 February 2023.
Edited by:
Murat Aydin Sav, Yeditepe University, TürkiyeReviewed by:
Qingfang Sun, Shanghai Jiao Tong University, ChinaShun Yao, Center for Pituitary Tumor Surgery, Sun Yat-sen University, China
Copyright © 2023 Chang, Jiang, Guo, Gao, Liu, Bao, Feng and Wang. This is an open-access article distributed under the terms of the Creative Commons Attribution License (CC BY). The use, distribution or reproduction in other forums is permitted, provided the original author(s) and the copyright owner(s) are credited and that the original publication in this journal is cited, in accordance with accepted academic practice. No use, distribution or reproduction is permitted which does not comply with these terms.
*Correspondence: Xinjie Bao, YmFveGluamllMUBwdW1jaC5jbg==; Ming Feng, amFja2lldHpAMTYzLmNvbQ==; Renzhi Wang, d2FuZ3J6QDEyNi5jb20=
†These authors have contributed equally to this work and share first authorship
‡ORCID: Xinjie Bao, orcid.org/0000-0003-2117-7692
Ming Feng, orcid.org/0000-0002-7159-9028
Renzhi Wang, orcid.org/0000-0003-2080-5474