- 1Joint Laboratory of the Modern Agricultural Technology International Cooperation, Ministry of Education, Jilin Agricultural University, Changchun, Jilin, China
- 2Key Lab of the Animal Production, Product Quality, and Security, Ministry of Education, Jilin Agricultural University, Changchun, Jilin, China
Introduction: Flaxseed oil (FO) and vitamin E (VE) both have antioxidant effects on sperm. The present study investigated the effects of dietary supplementation with FO and/or VE on semen quality.
Methods: 16 fertile Simmental bulls were selected and randomly divided into 4 groups (n = 4): the control group (control diet), FO group (control diet containing 24 g/kg FO), VE group (control diet containing 150 mg/kg VE) and FOVE group (control diet containing 150 mg/kg VE and 24 g/kg FO), and the trial lasted 10 weeks.
Results: The results showed that the addition of FO independently can increase sperm motion parameters, the levels of catalase (CAT), glutathione peroxidase (GSH-Px), testosterone (T) and estradiol (E2), while reduce oxidative stress in seminal plasma (P < 0.05). Supplement of VE independently can increased the motility, motility parameters, CAT and superoxide dismutase (SOD) levels, and reduce oxidative stress in seminal plasma (P < 0.05). There was an interaction effect of FO × VE on motility and reactive oxygen species (ROS), while GSH-Px and ROS were affected by week × VE 2-way interaction, levels of T and E2 were also affected by the dietary FO × week interaction (P < 0.05). The triple interaction effects of FO, VE and week were significant for malondialdehyde (MDA) (P < 0.05). Compared with the control group, sperm from the FOVE group had a significantly higher in vitro fertilization (IVF) rate, and subsequent embryos had increased developmental ability with reduced ROS levels at the eight-cell stage, then increased adenosine triphosphate (ATP) content and gene expression levels of CAT, CDX2, Nanog, and SOD at the blastocyst stage (P < 0.05). Metabolomic and transcriptomic results indicated that dietary supplementation of FO and VE increased the expression of the metabolite aconitic acid, as well as the expression of ABAT and AHDHA genes.
Conclusion: With in-silico analysis, it can be concluded that the effects of dietary FO and VE on improving semen quality and embryo development may be related to increased aconitic acid via the ABAT and AHDHA genes involved in the propionic acid metabolism pathway.
1 Introduction
Cryopreservation is the most practical approach for long-term storage of bull sperm. However, most sperm are damaged by oxidative stress during cryopreservation. This damage causes a loss and decline in motility, viability and fertilization ability. Therefore, improving the antioxidant capacity of frozen-thawed sperm and reducing the level of oxidative stress is particularly important for maintaining semen quality (1).
ω-3 Polyunsaturated fatty acids (ω-3 PUFAs) play important roles in the regulation of reproductive processes such as testosterone synthesis, sperm acrosome integrity and membrane fluidity (2). Ruminants lack the relevant fatty acid desaturase enzymes and are not able to synthesize ω-3 PUFAs. Thus, these animals must obtain ω-3 PUFAs or their pre-cursors from dietary sources (3). Flaxseed oil (FO) contains up to 50% ω-3 PUFAs, of which α-linolenic acid is converted to eicosapentaenoic and docosahexaenoic acids in mammals (4). In addition, ω-3 PUFAs contain stearic, oleic, linoleic and palmitic acids, and phenolic compounds, all of which have important roles in spermatogenesis and antioxidant enzyme activity (5).
Vitamin E (VE), one of the major antioxidants against reactive oxygen species (ROS) and lipid peroxidation, has been shown to be a major component of the antioxidant system of sperm (6, 7). VE deficiency may lead to developmental arrest of reproductive organs, including testicular tissue damage, and reduction of spermatogonia and spermatocytes (8). Previous studies demonstrated that supplementation of diets with VE could improve semen quality by reducing mitochondrial protection from oxidative stress damage (9).
In the past decade, in vitro fertilization (IVF) technology has made significant progress in animal reproduction (10). Compared with artificial insemination, IVF is more cost-effective and less time-consuming (11). Seminal plasma metabolomics has been used to screen for potential biomarkers of fertility in bulls (12). To investigate male fertility, transcriptome sequencing technology has been used to assess mRNA expression in sperm (13, 14). Therefore, metabolomic and transcriptomic analyses are important tools for identifying semen fertility.
At present, there is no relevant report on the effect of the combination of FO and VE in the diet on the frozen-thawed semen of bulls. Therefore, we speculate that the combined addition of FO and VE in the diets could further reduce oxidative stress and improve motility and motion parameters of frozen-thawed sperm, compared with FO or VE alone. At the same time, the combined addition of FO and VE could further improve the ability of IVF and early embryonic development by enhancing the antioxidant capacity. The aim of this study was to evaluate the effects of combined FO and VE supplementation on bull semen quality and subsequent embryonic development in vitro, and to explore the mechanism of the effects of FO and VE on semen quality using transcriptome and metabolome analyses.
2 Materials and methods
2.1 Experimental design
The trial lasted for 10 weeks, and 16 fertile Simmental bulls (approximately 2–3 years of age and 550–650 kg each) were selected from Changchun Xinmu Sciences & Technologies Co., Ltd, and divided randomly into four groups (n = 4). They were all kept under similar management. Ingredients and chemical composition of the diets are shown in Table 1. The dietary treatments were as follows: 1) control group: control diet, 2) FO group: control diet containing 24 g/kg FO (15), 3) VE group: control diet containing 150 mg/kg VE (16), and 4) FOVE group: control diet containing 150 mg/kg VE and 24 g/kg FO (17). In the current study, FO was purchased from Inner Mongolia Jiuding Food Co., Ltd., China. The molar percentages of linolenic acid, linoleic acid, oleic acid and saturated acyl groups in FO was 55.7 ± 0.0%, 14.2 ± 0.3%, 20.5 ± 1.2% and 9.5 ± 0.9% respectively (18). VE was purchased from Zhejiang NHU Co., Ltd., China. This study showed that the combination of FO and VE improved the motion parameters of sperm and the antioxidant capacity of seminal plasma after frozen-thawed at week 10. Therefore, the control and FOVE groups of week 10 samples were selected for subsequent IVF, seminal plasma metabolome and sperm transcriptome studies.
2.2 Semen collection and assessment
Semen was collected by experienced technicians, twice a week per bull, into a heated (38°C) artificial vagina connected to a disposable tube, and quickly transferred to an adjacent laboratory where the physiological parameters were assessed after semen collection. Semen samples were expanded in a BioXcell® (IMV Technologies, L’agile, France) extender to a concentration of 1.28 × 108, then loaded into thin tubes (IMV Technologies), and the semen was equilibrated at 4°C for 2 h, and subsequently cooled at approximately 8 min from 4°C to − 140°C by a turbofreezer (Minitube, Germany) as previously described by Memon et al. (19). After that, samples were stored in a liquid nitrogen tank (−196°C). The sperm motility and motion parameters were assessed using the Hamilton Thorne IVOS II automatic sperm analyzer (Hamilton-Thorne, Beverly, MA, USA). For each bull, 2 straws were thawed by immersion in a water bath at 37°C for 30 s. 10 μL were collected from each straw, and eight fields were randomly examined.
2.3 Oxidative stress index and hormones
The oxidative stress index and hormones of embryo culture medium and seminal plasma included superoxide dismutase (SOD, MM-34758O1, 1.2U/mL-42 U/mL), catalase (CAT, MM-50463O1, 0.5 U/mL-18U/mL), glutathione peroxidase (GSH-Px, MM-2465O1, 20 IU/L-480 IU/L), ROS (MM-50460O1, 5 IU/mL-160 IU/mL), malondialdehyde (MDA, MM-34745O1, 0.5 nmol/mL-16 nmol/mL), testosterone (T, MM-2382O1, 0.3nmol/L-12nmol/L) and estradiol (E2, MM-0023O1, 4 pmol/L-120 pmol/L) were determined using specific enzyme-linked immunoassay kits (Jiangsu Meimian Industrial Co., Ltd, Yancheng, China), according to the manufacturer’s instructions. Briefly, semen was centrifuged at 3000 rpm for 15 min, and the seminal plasma was extracted before analysis. Results were measured at 450 nm on a spectrophotometer (Shanghai Spectrophotometer Co., Ltd, Shanghai, China).
2.4 In vitro maturation
Oocyte collection and IVM were performed as described previously (20). Briefly, a 10 mL syringe was used to aspirate cumulus–oocyte complexes from antral follicles with a diameter between 2 and 8 mm. Oocytes surrounded by at least three cumulus cell layers were selected for subsequent experiments, then washed in collection medium M199 supplemented with 2% fetal bovine serum (FBS, Hyclone) and transferred to maturation medium modified M199 medium supplemented with alanyl-glutamine (10 μL/mL), sodium pyruvate (50 μL/mL), epidermal growth factor (10 μL/mL), follicle-stimulating hormone (4 μL/mL), luteinizing hormone (4 μL/mL), E2 (10 μL/mL), cysteamine (10 μL/mL), and 10% FBS, incubated for 22 h at 38.5°C, 5% CO2 in air and high humidity.
2.5 In vitro fertilization
After 22 h, cumulus–oocyte complexes were added to SOF-IVF medium (6.29 mg/mL NaCl, 0.53 mg/mL KCl, 0.16 mg/mL NaH2PO4, 0.1 mg/mL MgCl2·6H2O, 0.25 mg/mL CaCl2·6H2O, 0.758 mg/mL sodium lactate, 2.1 mg/mL NaHCO3, 6.25 mg/mL BSA, 10.417 μL/mL MEM non-essential amino acids, 10 μL/mL fructose, 10 μL/mL heparin and 10 μL/mL sodium pyruvate). Frozen-thawed pooled semen was washed through 45% and 90% Percoll gradients (21). Semen, layered on the top, was centrifuged (700 ×g, 15 min). 5 mL of sperm TALP medium (5.84 mg/mL NaCl, 0.23 mg/mL KCl, 35 μg/mL NaH2PO4, 2.1 mg/mL NaHCO3, 2.38 mg/mL HEPES, 80 μg/mL MgCl2·6H2O, 310 μg/mL CaCl2·6H2O, 3.07 μL/mL sodium lactate, 6 mg/mL BSA and 0.11 mg/mL sodium pyruvate) were used to wash the pellet (300 ×g, 5 min). Sperm concentration was calculated with a hemocytometer and adjusted to 106/mL with SOF-IVF medium.
2.6 In vitro culture
After 18 h of co-incubating cumulus–oocyte complexes and sperm at 38.5°C, 5% CO2 in air and high humidity, Zygotes were mechanically separated via pipetting and washed three times through IVC medium SOF-aa with 62.94 mg/mL NaCl, 5.33 mg/mL KCl, 1.62 mg/mL NaH2PO4, 0.46 mg/mL MgCl2, 4.7 mg/mL sodium lactate, 4.32 mg/mL glucose, 26.07 mg/mL NaHCO3, 2.52 mg/mL CaCl2·2H2O, 0.36 mg/mL sodium pyruvate, 20 μL/mL essential amino acids, 10 μL/mL non-essential amino acids, 4 mg/mL BSA and 0.15 mg/mL glutamine. The zygotes were cultured at 38.5°C, 5% CO2 in air and high humidity. The number of two-, four- and eight-cell embryos were recorded at 32, 42 and 72 h, respectively, and blastocyst yield was assessed at Day 7.
2.7 Measurement of intracellular ROS levels
Intracellular ROS levels in embryos were measured with a ROS detection kit (S0033S; Beyotime, China). Briefly, embryos were incubated with 10 mM DCFH-DA for 20 min at 37°C. After washing three times with 1% phosphate-buffered saline/PVP360 (PBS-PVP), the embryos were examined using a fluorescence microscope (Olympus, Tokyo, Japan) with a 460 nm excitation filter. Fluorescence images of all embryos were recorded using a digital camera (Nikon 990, Tokyo, Japan), and analyzed using the NIH ImageJ by Wayne Rasband from the National Institute of Health (Bethesda, MD, USA). Fluorescence intensities of the embryos were compared with that of the control after deducting the background value.
2.8 Measurement of intracellular ATP levels
Adenosine triphosphate (ATP) of embryos was detected using an ATP assay kit (S0063, Invitrogen). Briefly, embryos were collected, washed twice in PBS-PVP and then fixed in formaldehyde-PVP for 0.5 h at room temperature. Afterwards, the embryos were washed three times with PBS-PVP, 5 min each time, and then incubated with PBS-PVA containing 0.1% Triton X-100 for 1 h for membrane permeabilization. After thoroughly washing with PBS-PVP, the fluorescence signal was captured using an epifluorescence microscope, and the fluorescence intensity of embryos was analyzed using NIH ImageJ software.
2.9 RNA isolation, cDNA synthesis and q-PCR
Total RNA from bovine blastocysts was extracted using the RNeasy Mini kit (KIT0204, Qiagen, Hilden, Germany). A total of 18 blastocysts were used to extract RNA. The One-Step gDNA Removal and cDNA Synthesis SuperMix (AT311-03, TransGen Biotech, Beijing, China) was used to synthesize cDNA. The real-time PCR mix (25 μL) consisted of 2 μL of cDNA, 12.5 μL of SYBR green master mix, 9.5 μL of RNase-free water, and 0.5 μL each of primers (10 pmol). The primers used for the octamer-binding transcription factor 4 (OCT-4), caudal type homeobox 2 (CDX-2), Nanog, SOD, CAT, B-cell lymphoma 2 (Bcl2), BCL2 Associated X (Bax) genes and 18s rRNA are listed in Table 2. The program used for the amplification consisted of a denaturing cycle of 3 min at 95°C, 40 cycles of PCR (95°C for 10 s, 55°C for 45 s, and 95°C for 1 min), a melting curve analysis consisting of 95°C for 1 min followed by 55°C for 1 min, and a step cycle starting at 55°C for 10 s with a 0.5°C/s transition rate, and cooling at 4°C. Relative gene expression data were analyzed using q-PCR and the 2−△△CT method.
2.10 Metabolite extraction and analysis
Approximately 100 μL of each seminal plasma sample was transferred to a new 1.5 mL Eppendorf tube. Then an equal volume of each sample was sampled and mixed into 100 μL of a quality control (QC) sample. Then, 300 μL of cold methanol was added, shaken and mixed, and placed at −20°C for 2 h. After centrifugation at 25,000 ×g for 10 min at 4°C, 350 μL supernatant was placed in a new Eppendorf tube, and the centrifugation step was repeated. Then 25 μL supernatant was added to 225 μL 50% methanol, and 50 μL of supernatant from each sample was taken and mixed with a QC sample, and the remaining transferred to a new 1.5 mL Eppendorf tube for testing. An Acquity UPLC HSS T3 column (100 mm × 2.1 mm, 1.8 μm, Waters, UK) was used for reversed-phase separations. The column temperature was maintained at 40°C and the metabolites were eluted at a flow rate of 0.5 mL/min. The injection volume for each sample was 5 μL. A high-resolution tandem mass spectrometer Xevo G2 XS QTOF (Waters, UK) was used to detect small molecules eluted from the column. A quality control sample was acquired after every 10 samples to evaluate the stability of the LC-MS.
2.11 Data processing of metabolites
Peak extraction was performed primarily through the commercial software Progenesis QI (version 2.2). The resulting LC-MS data were inputted to the R software package for principal component analysis (PCA) and partial least squares discriminant analysis (PLS-DA) to evaluate differential ions, identify differentially expressed genes, and analyze metabolite and metabolic pathways.
2.12 Total RNA extraction of transcriptomics
Total RNA was extracted from sperm using the RNeasy Micro kit (KIT0204, Qiagen, Hilden, Germany) according to manufacturer’s instruction. Briefly, samples were transferred to tubes containing Buffer RL and one volume of 70% ethanol and then centrifuged. Buffer RW1, DNase I, Buffer RPE and 80% ethanol were added then samples centrifuged. High quality RNA was used for mRNA library construction.
2.13 mRNA library construction of transcriptomics
High-quality RNA was amplified (more than 200 pg) with oligo-dT and dNTPs to generate full-length cDNA by PCR. The Agilent 2100 bioanalyzer (Thermo Fisher Scientific, MA, USA) was used to determine the average molecular length of PCR products. The DNA products were purified by an Agencourt AMPure XP-Medium kit (Thermo Fisher Scientific, USA). Single-stranded circular DNA was formatted as the final library, which used phi29 (Thermo Fisher Scientific, MA, USA) to prepare a DNA nanosphere. The DNA nanosphere was loaded into a patterned nanoarray, with single end 50 base reads generated on a BGISEQ500 platform (BGI-Shenzhen, China).
2.14 Statistical analysis
All data are presented as least squares means ± SD. Each individual bull was considered an experimental unit in all statistical analysis. Data on oxidative stress index, T, E2 and the quality of semen were analyzed for the main effects of FO and VE. The FO, VE and time interactions used the General Linear Model procedure of the SPSS Institute (19.0) with a repeated measurement analysis. Differences among least square means were determined by the Tukey test and P < 0.05 was considered a significant difference. Differences in embryo development, CAT, SOD and ROS levels in embryo culture medium, and relative levels of gene expression, ROS and ATP in embryos, between the control and FOVE groups were compared by the T-test (SPSS 19.0), and P < 0.05 was considered a significant difference.
3 Results
3.1 Effect of dietary FO and VE on frozen-thawed sperm motility and motion parameters
The diet supplemented with VE significantly enhanced motility, straight distance (DSL), straight line velocity (VSL), curvilinear velocity (VCL) at week 8 and increased average path (DAP), DSL, curvilinear distance (DCL), velocity of the average path (VAP), VSL and VCL at week 10 compared with groups with no VE supplementation (P < 0.05) (Table 3). Sperm from bulls fed a diet containing FO had significantly higher values of DAP, DSL, DCL, VAP, VSL, VCL and amplitude of lateral head displacement (ALH) at week 10 compared with other groups (P < 0.05). There was an interaction effect of FO × VE on motility (P < 0.05).
3.2 Effect of dietary FO and VE on frozen-thawed semen plasma antioxidant status and hormones
As shown in Table 4, dietary FO significantly increased GSH-Px and decreased MDA at week 10. Supplementation with VE significantly increased SOD and decreased MDA at week 8 compared with groups with no VE supplementation (P < 0.05). The diet supplemented with FO or VE alone significantly increased CAT and decreased ROS at weeks 8 and week 10 compared with no FO or VE supplementation (P < 0.05). There was an effect of week × VE interaction on GSH-Px and ROS, and ROS was also affected by a dietary FO × VE 2-way interaction (P < 0.05). The triple interaction effects of FO, VE and week were significant for MDA (P < 0.05). Levels of T and E2 in frozen-thawed semen plasma are also shown in Table 4. Samples from bulls fed the diet with FO had increased levels of T and E2 at weeks 4, 8 and 10 compared with those from bulls fed the diet without FO. At week 8, elevated T content was observed with supplementation of VE in the diet (P < 0.05). Levels of T and E2 were also affected by the dietary FO × week interaction (P < 0.05).
3.3 Effect of dietary FO and VE on thawed sperm and subsequent embryo development outcomes
The above results show that adding FO and VE to the diet for 10 weeks can improve the motility and motion parameters of frozen-thawed sperm, and also improve the antioxidant capacity, and the combined addition of FO and VE has a better effect. The control group and FOVE group samples of week 10 were used for further experiments. IVF results (Figure 1) showed that the diet supplemented with FO and VE significantly increased the two-cell, four-cell, eight-cell and blastocyst rate compared with the control group (P < 0.05).
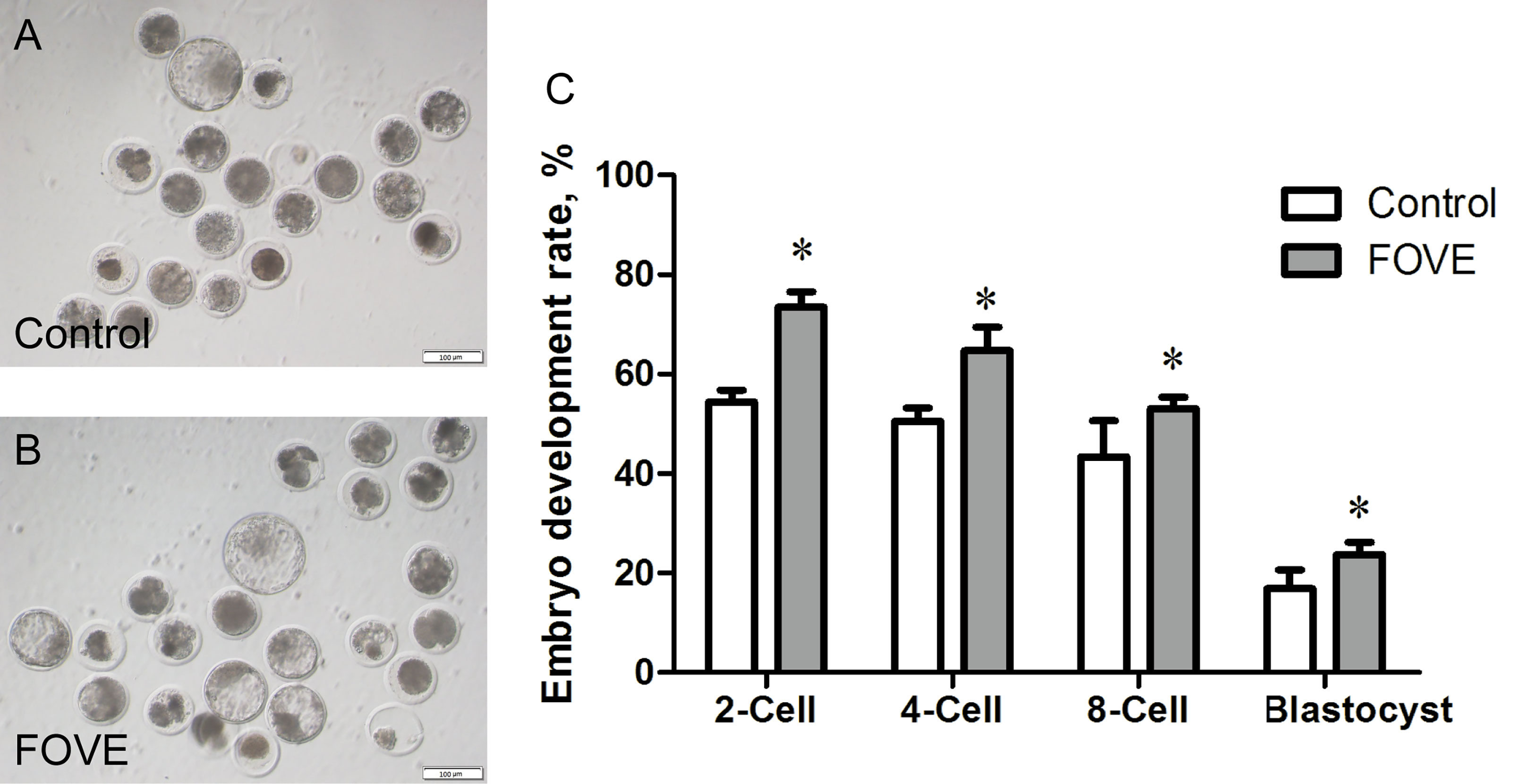
Figure 1 The above results show that adding FO and VE to the diet for 10 weeks can improve the motility and motion parameters of frozenthawed sperm, and also improve the antioxidant capacity, and the combined addition of FO and VE has a better effect. The control group and FOVE group samples of week 10 were used for further experiments. IVF results (A–C) showed that the diet supplemented with FO and VE significantly increased the twocell, four-cell, eight-cell and blastocyst rate compared with the control group (P < 0.05).
3.4 Effects of dietary FO and VE on the ROS and ATP of bovine embryos
Intracellular ROS levels were measured by assessing DCFH fluorescence (Figures 2A–F). Quantitative analysis showed that the ROS levels of four- and eight-cell embryos were significantly decreased (P < 0.05) in the FOVE group compared with the control group (Figures 2C–F). As shown in Figures 2K, L, the ATP levels of eight-cell embryos were significantly higher in the FOVE group than in the control group (P < 0.05).
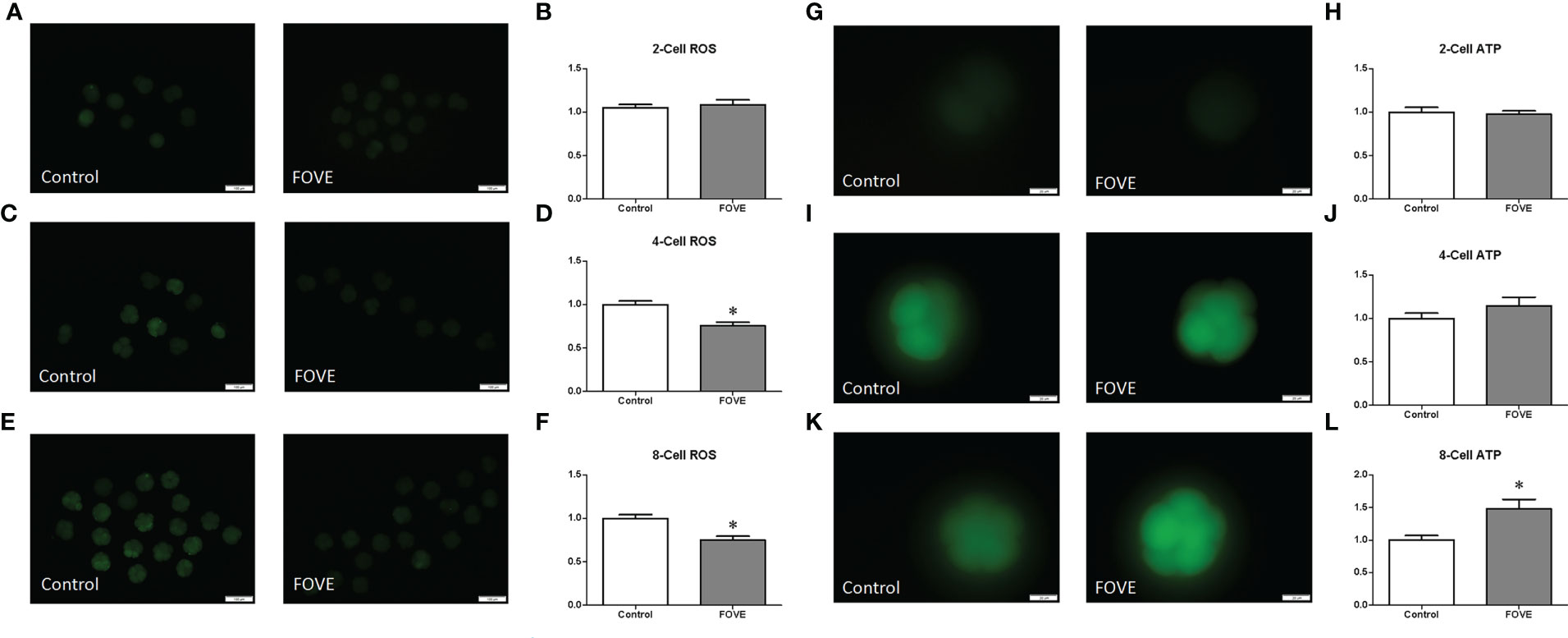
Figure 2 Intracellular ROS levels were measured by assessing DCFH fluorescence (A–F). Quantitative analysis showed that the ROS levels of four- and eight-cell embryos were significantly decreased (P < 0.05) in the FOVE group compared with the control group (C–F). There were no significant difference in ATP content between two- and four-cell shown in (G–J) (P > 0.05). As shown in Figures 2K, L, the ATP levels of eight-cell embryos were significantly higher in the FOVE group than in the control group (P < 0.05).
3.5 The effect of dietary FO and VE on the antioxidant status of embryo culture medium
Figure 3 shows the effect of dietary FO and VE on the antioxidant status of embryo culture medium. The activity of CAT was increased when the diet was supplemented with FO and VE (P < 0.05). In contrast, FO and VE supplementation resulted in decreased MDA levels in embryo culture medium, but the activity of SOD did not differ among the groups (P > 0.05).
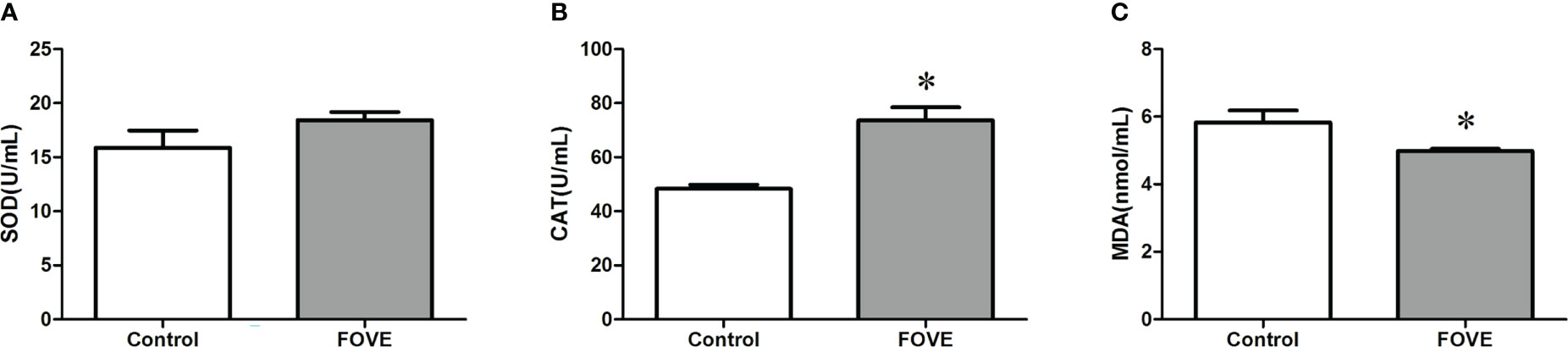
Figure 3 Antioxidant status of embryo culture medium. Values are shown as mean ± SD. *Significant difference compared with the control group (P < 0.05). (A–C) shows the effect of dietary FO and VE on the antioxidant status of embryo culture medium. The activity of CAT was increased when the diet was supplemented with FO and VE (P < 0.05). In contrast, FO and VE supplementation resulted in decreased MDA levels in embryo culture medium, but the activity of SOD did not differ among the groups (P > 0.05)
3.6 The effect of dietary FO and VE on the relative levels of gene expression in blastocysts
The effects of dietary FO and VE combined on blastocyst gene expression were examined by q-PCR. The relative abundance of gene expression in blastocysts is presented in Figure 4. The results showed that blastocysts from the sperm of bulls consuming a diet supplemented with FO and VE had significantly increased expression of CDX2, Nanog, SOD and CAT compared with control group (P < 0.05).
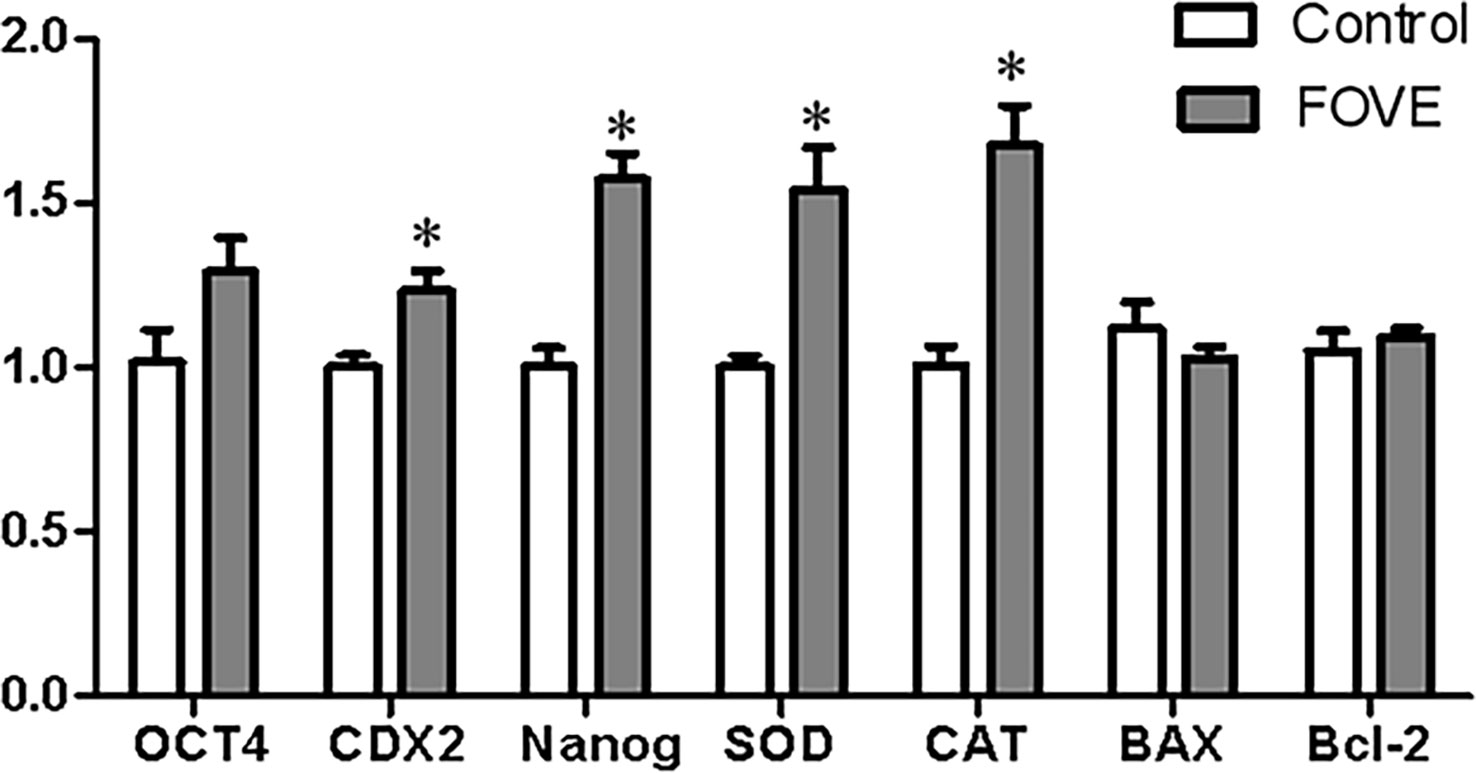
Figure 4 Relative levels of gene expression in blastocysts. Values are shown as mean ± SD. *Significant difference compared with the control group (P < 0.05).
3.7 Metabolomic changes and pathway enrichment analysis
The above experimental results suggest that the combined addition of FO and VE in the diet may improve the IVF rate of sperm and the developmental ability of early embryos by improving antioxidant capacity. Therefore, we used metabolomics and transcriptomics to deeply explore the mechanism of improving the motion parameters of frozen-thawed sperm motility and IVF ability. Significantly differences in metabolites were identified according to the PLS-DA VIP (variable importance in the projection) > 1, fold change ≥ 1.2 and P < 0.05 (Figures 5A, B). We identified a total of 12,252 positive ions (pos) and 10,101 negative ions (neg). As shown in Figures 5C, D, compared with the control group, 198 and 167 differentially expressed ions were identified in the pos and neg mode, respectively. Among them, 90 and 108 were up-regulated and down-regulated, respectively, in the pos mode, and 86 and 81 were up-regulated and down-regulated, respectively, in neg mode (Figures 5E, F). The main differences in metabolites are shown in Table 5. Pathway enrichment analysis was performed to study the effect of dietary FO and VE on sperm, as shown in Figures 5G, H. The results revealed that the metabolites with altered levels of expression were mainly associated with androgen and estrogen metabolism, riboflavin metabolism, fatty acid metabolism, steroid biosynthesis and bile acid biosynthesis in positive ion mode (Figure 5G), and mitochondrial electron transport chain, glycerolipid metabolism, citric acid cycle and tyrosine metabolism in negative ion mode (Figure 5H).
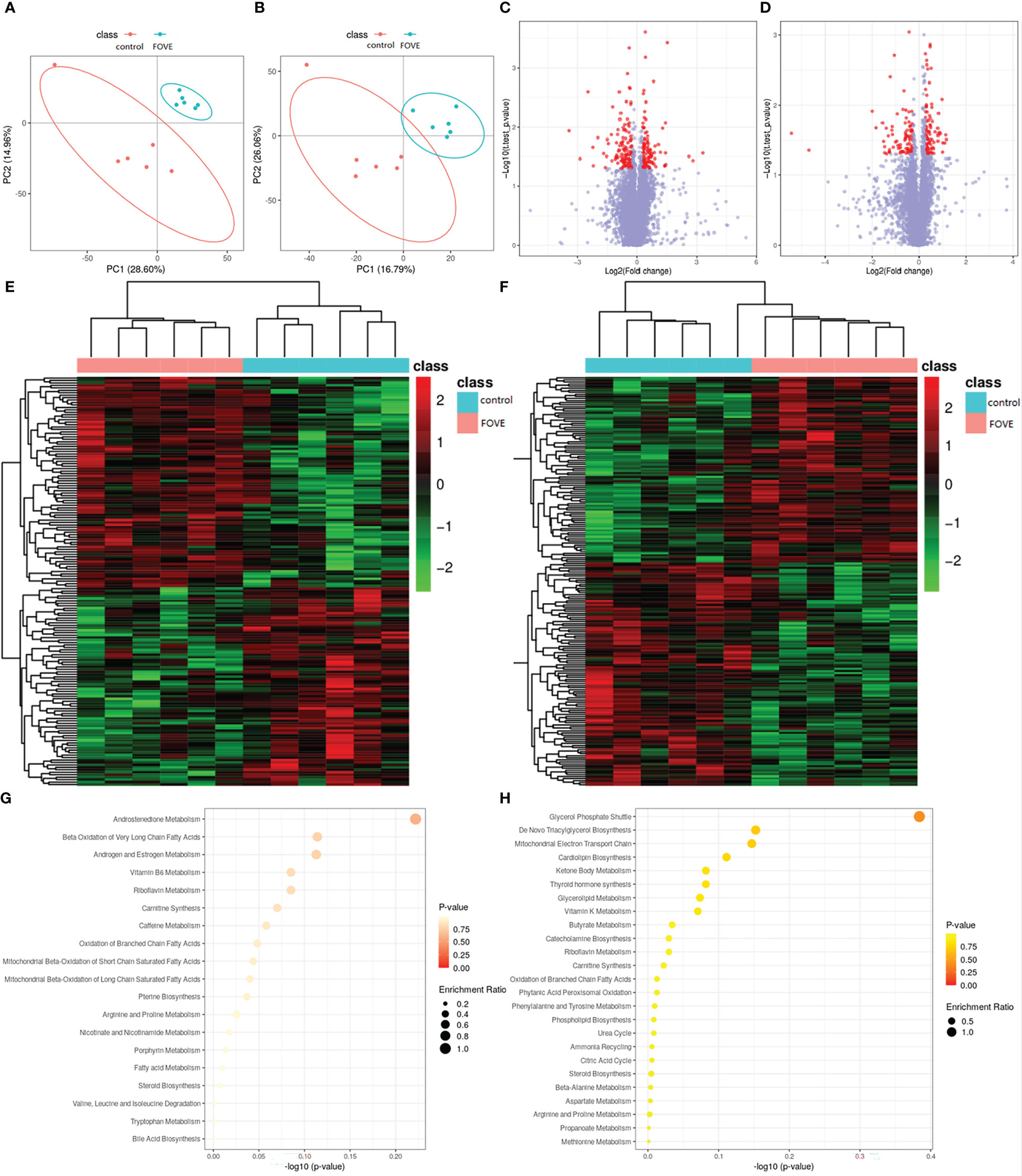
Figure 5 Seminal plasma metabolomic changes and pathway enrichment. Positive ion mode (A) and negative ion mode (B) for the partial least square-discriminate analysis (PLS-DA) score charts of metabolite profiling data. Analysis of the flaxseed oil + vitamin E (FOVE) group and control group based on metabolomics. Volcano figure under positive ion mode (C) and negative ion mode (D), with the red points representing different levels of metabolites. Hierarchical clustering analysis of positive ion mode (E) and negative ion mode (F), with red denoting increased expression and blue denoting decreased expression. The pathway analysis for positive ion mode (G) and negative ion mode (H). Colors (varying from yellow to red) show metabolites in the data with different levels of significance. The x-axis represents the pathway impact value computed from pathway topological analysis, and the y-axis is the-log of the P-value obtained from pathway enrichment analysis. The pathways that were most significantly changed are characterized by both a high-log (P) value and high impact value (top right region).
3.8 KEGG and Gene ontology analysis of differentially expressed genes
The overall distribution trend of all samples was investigated by PCA, and the PCA score plots of the two groups are shown in Figure 6A. Variations were identified in the PC1 and PC2 score plots. Differentially expressed genes were chosen with the DESeq package using the following criteria: |log2| (fold change) > 3 and adjusted P-value < 0.01. The results showed that 1336 genes and 987 genes were up-regulated and down-regulated, respectively, in the FOVE group compared with the control group (Figures 6B, C), and the top 5 significantly up-regulated genes in the two groups are listed in Table 6. Gene ontology (GO) analysis of DEGs was categorized functionally based on GO annotation terms. Figure 6D shows the top 20 enriched GO terms. To further characterize DEGs, we performed pathway analysis using the KEGG pathway database. As shown in Figure 6E, the major enrichment pathways included cell motility, lipid metabolism, amino acid metabolism, nucleotide metabolism, and energy metabolism.
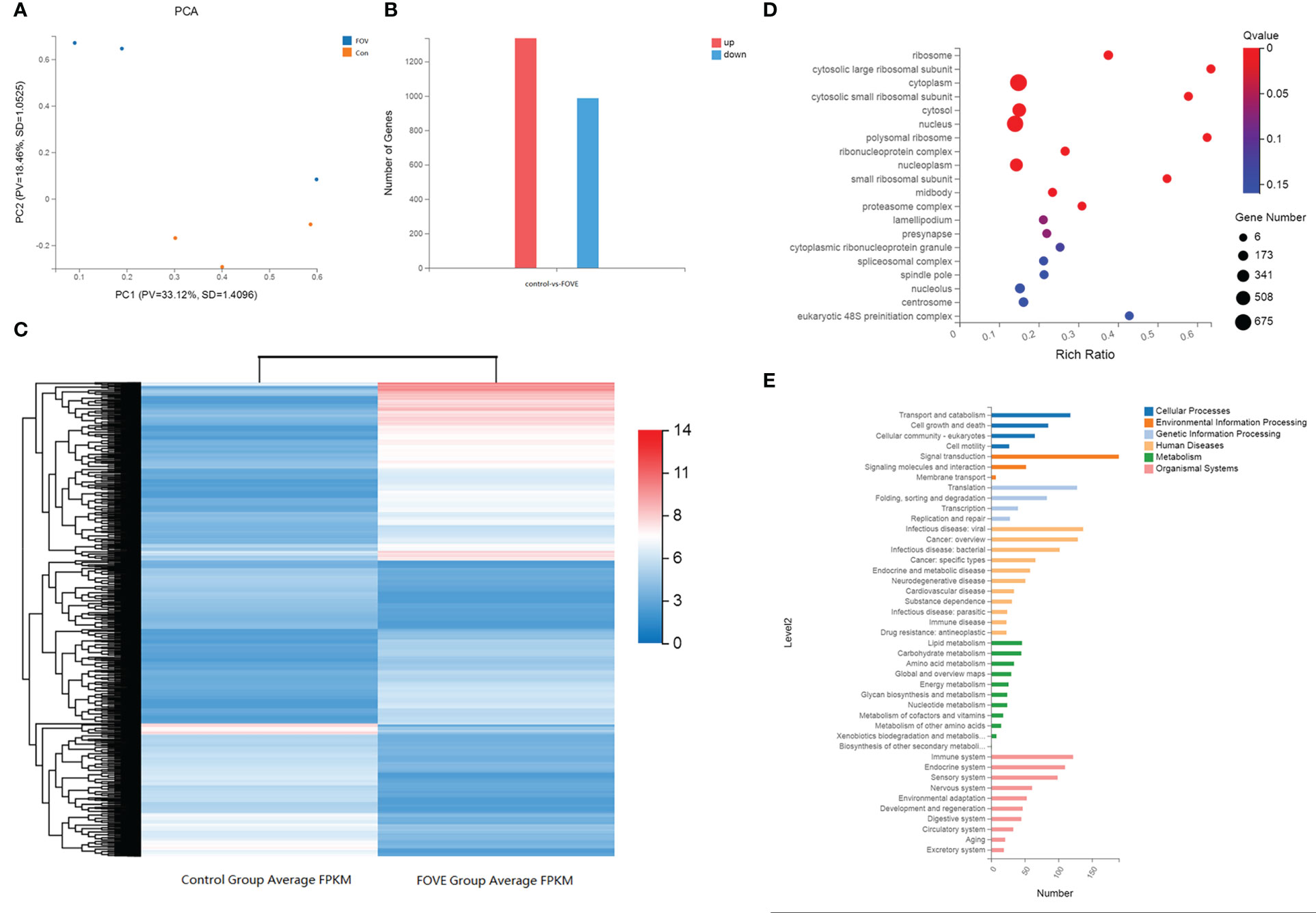
Figure 6 KEGG and GO analysis of differentially expressed genes. Principal component analysis (PCA) score plots of the two groups (A). The number of differentially expressed genes (DEGs), red column = increased expression (n=1336) and blue column = decreased expression (n=987) (B). Hierarchical clustering analysis of DEGs. Red denotes increased expression and blue denotes decreased expression (C). Function analysis of differentially expressed genes (DEGs) between two treatment groups based on Gene Ontology (D) and KEGG pathway (E) analysis. D, The size of the dot designates the number of DEGs in the pathway, and the color of the dot corresponds to a different Q value (for the most enriched top 20 pathway terms). E, The ordinate is the enriched KEGG pathway term, and the abscissa is the number of DEGs for the term. Different colors are used to differentiate cellular processes, environmental information processing, genetic information processing, human diseases, metabolism and organismal systems.
3.9 Integrated analysis of the metabolomic and transcriptomic data
To further explore the potential relationship between DEGs and metabolites, we integrated metabolomic and transcriptomic data at the pathway level. As shown in Figure 7, there were a total of 77 enriched pathways, including purine metabolism, nicotinate and nicotinamide metabolism, glycerolipid metabolism, glycerophospholipid metabolism, valine, leucine and isoleucine degradation, propanoate metabolism, fructose and mannose metabolism, tricarboxylic acid cycle (TCA cycle), phenylalanine, tyrosine and tryptophan biosynthesis and steroid biosynthesis.
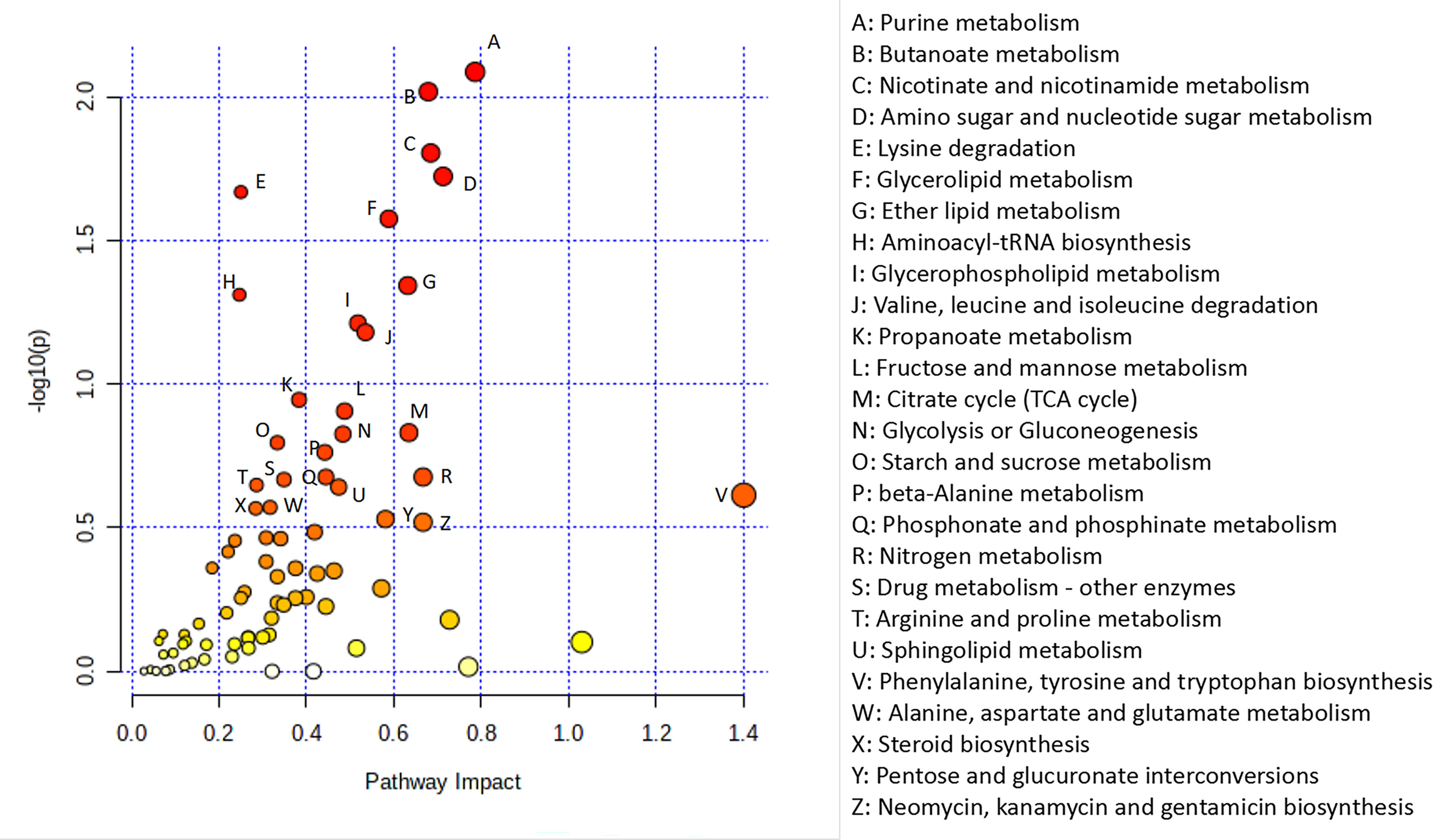
Figure 7 Integrated analysis of the metabolomic and transcriptomic data. Integrated analysis of the metabolomic and transcriptomic data performed with Metaboanalyst (https://www.metaboanalyst.ca/). Each point represents one metabolic pathway. The size of the dot represents a positive correlation with the metabolic pathway.
4 Discussion
In this study, metabolomics and transcriptomics were used to clarify the mechanism of dietary supplementation of FO and VE on semen quality and embryonic development. FO contains up to 50% ω-3 PUFAs, especially α-linolenic acid (22), which plays important roles in the regulation of membrane properties (23). Previous studies have shown that ω-3 PUFAs in diets improved sperm morphology in the boar (15). Dietary supplementation of VE could reduce the rate of sperm deformity in goats (9). Results of the present study indicated that dietary supplementation with 24 g/kg FO and 150 mg/kg VE had positive effects on bull sperm. The positive effects may be due to an increased level of aconitic acid via upregulated expression of 4-aminobutyrate aminotransferase (ABAT) and Hydroxyacyl-CoA dehydrogenase subunit alpha (HADHA) genes of the propionate metabolic pathway, providing energy for bull sperm and promoting the IVF rate and subsequent embryonic development.
Our results demonstrated that the diet supplemented with FO and VE increased frozen-thawed sperm motion parameters. These results are consistent with previous studies that showed dietary supplementation with FO alone increased the motility of sperm, progressive motility, vitality, membrane integrity and quality of frozen-thawed sperm (16, 24). Similarly, it has been shown that 8% FO in goat diets increased the antioxidant capacity of testicular tissue and promoted testicular development and spermatogenesis (17). ω-3 PUFAs can affect the hypothalamic-pituitary-gonadal axis, thereby promoting the secretion of important hormones related to reproduction, such as T and E2 (25, 26). Similarly, FO promoted the secretion of T and E2 in our study. It can be speculated that FO may promote the production of steroid hormones and then stimulate the production of sperm.
Numerous studies have shown that high concentrations of VE in the diet improved semen quantity (27, 28). As the main antioxidant of sperm, vitamin E can scavenge oxygen free radicals in sperm membrane and avoid oxidative damage to sperm (29). It has been reported that diets supplemented with VE at 200 IU/sheep/d significantly reduced MDA levels and increased the activities of SOD and GSH-Px in semen (9). Consistent with these findings, our results showed that adding VE increased frozen-thawed sperm motility and increased SOD and CAT levels and reduced MDA and ROS levels in bull seminal plasma. Taken together, it was observed in this study that VE may enhance sperm fertility through antioxidant effects (30).
It was reported that the combined addition of ω-3 PUFAs and VE may have reduced oxidation of sperm plasma membranes and maintained semen quality during cryopreservation (31). In the current study, the addition of FO and VE in the diet may have prevented sperm lipid peroxidation caused by MDA and ROS. In addition, FO and VE in the diet improved sperm motility and motion parameters, which is consistent with the results of Kargar et al., suggesting that dietary supplementation with FO and VE improved sperm motility (17). Supporting these findings, supplementation of FO and VE in the diet improved rooster semen quality and fertility potential (32), and increased the motility and membrane integrity of stallion semen (31).
Accumulating evidence has revealed that sperm motility and motion parameters are positively correlated with early embryonic development (33). Low motility sperm may affect the acrosome reaction, and the rate of fertilization and blastocyst formation (34). Sperm with low motion parameters and motility can decrease embryo cleavage rates (35). The present results suggested that dietary FO and VE improves embryo development by improving sperm motility.
During embryonic development, mitochondria provide ATP to cleavage-stage embryos by uptake and oxidation of various substrates (36). ROS is a by-product of ATP generation through oxidative phosphorylation, and cause oxidative stress and embryo damage (37). The synthesis of ROS and ATP in the cytoplasm of embryonic cells may be affected by sperm quality (38). In this study, embryos from the FOVE group showed increased ATP levels at the eight-cell stage, and decreased ROS levels at the four- and eight-cell stage, suggesting that sperm from bulls fed a diet with FO and VE may be beneficial for subsequent embryonic development.
It is reported that concentrations of ROS in spent culture media may play a critical role in the success of IVF (39). Our research showed that sperm from bulls fed a diet containing FO and VE reduced the oxidative stress of embryo medium and up-regulated the expression CAT and SOD genes in blastocysts. In addition, this sperm led to significantly increased blastocyst expression of CDX2 and Nanog genes, which are important for normal embryonic development (40).
It is reported that lanosterol could affect oocyte maturation and subsequent embryonic development (41). Our results showed that a diet supplemented with FO and VE may promote the sperm fertilization rate through increased lanosterol levels, supporting previous findings that supplementation with 50 µM lanosterol during in vitro maturation improved preimplantation development of embryos by elevating lipid levels (42). A lack of riboflavin in the diet was reported to reduce murine sperm motility, morphology, and bioenergetic metabolism (43). Riboflavin has antioxidant properties while protecting cells from oxidative stress (44, 45). Similarly, protocatechuic acid can positively affect the reproductive function of male rats through anti-inflammatory and antioxidant effects (46–48). In the current study, we found that dietary FO and VE may enhance bull semen quality by increasing the levels of riboflavin and protocatechuic acid.
Lactate and palmitic acid provide an important energy source for sperm (49, 50). Thus, when high energy is required, sperm efficiently metabolize glycolysable substrates to yield ATP (51). The present study has shown that dietary FO and VE increased the content of lactate and palmitic acid, suggesting the sperm may utilize anaerobic glycolysis more efficiently (52). The current study also found that dietary FO and VE increased aconitase levels in sperm. Studies have shown that mitochondrial aconitase is an important regulator of the TCA cycle in asthenozoospermia, and aconitase was reduced in asthenozoospermia (53). Therefore, these results indicate that high expression of aconitase may promote the TCA cycle and improve sperm motility.
Our results showed that dietary FO and VE increased the expression of lipase A, lysosomal acid type (LIPA) and ABAT genes in sperm. It has been reported that LIPA and ABAT are involved in the TCA cycle and maintain mitochondrial membrane function (54, 55). As a key lipid metabolism enzyme, HADHA is associated with increased hepatic lipid accumulation when HADHA is inhibited, whereas lipid droplet formation is reduced when HADHA is overexpressed (56). Enoyl-CoA hydratase and 3-hydroxyacyl CoA dehydrogenase (EHHADH) is a part of the fatty acid β-oxidation pathway (57). Our results showed that a diet supplemented with FOVE increased EHHADH and HADHA gene expression, indicating that sperm lipid metabolism may be regulated through the fatty acid pathway. Aldehyde oxidase 1 (AOX1) induces antioxidant defense pathways and increases H2O2 levels, and subsequently promoting the production of antioxidant enzymes (58). The elevated expression of AOX1 genes in this study shows that the antioxidant capacity of sperm was increased.
Finally, we performed a combined analysis of metabolomic and transcriptomic data to identify the pathways by which dietary FO and VE affect sperm. We found that propanoate metabolism played an important role in improving sperm motility. Propionate metabolism is downstream of lipid metabolism and contributes to the tricarboxylic acid cycle and provides energy (59). A previous study showed that propanoate metabolic pathways play an important role in sperm cryopreservation (60). In addition, sperm must reserve sufficient energy to sustain motility, capacitation, and fertilization (61). Therefore, dietary FO and VE may affect sperm motility and subsequent embryonic developmental through the propionate pathway.
Based on transcriptome and metabolome analysis, as shown in Figure 8, we found that sperm from bulls fed a diet with FO and VE may regulate expression of the ABAT and HADHA genes in the propionic acid metabolism pathway to increase aconitate, thereby improving semen quality and subsequent embryo development. However, Due to the small number of bulls available for study under actual conditions of the experiment, as a large livestock animal, we cannot obtain a large number of experimental animals to carry out the experiment. In addition, an inherent limitation of this analysis was the in silico platform with results only based on bioinformation instead of direct investigation of biological mechanisms. Therefore, more method validation should be used to validate our hypothesis.
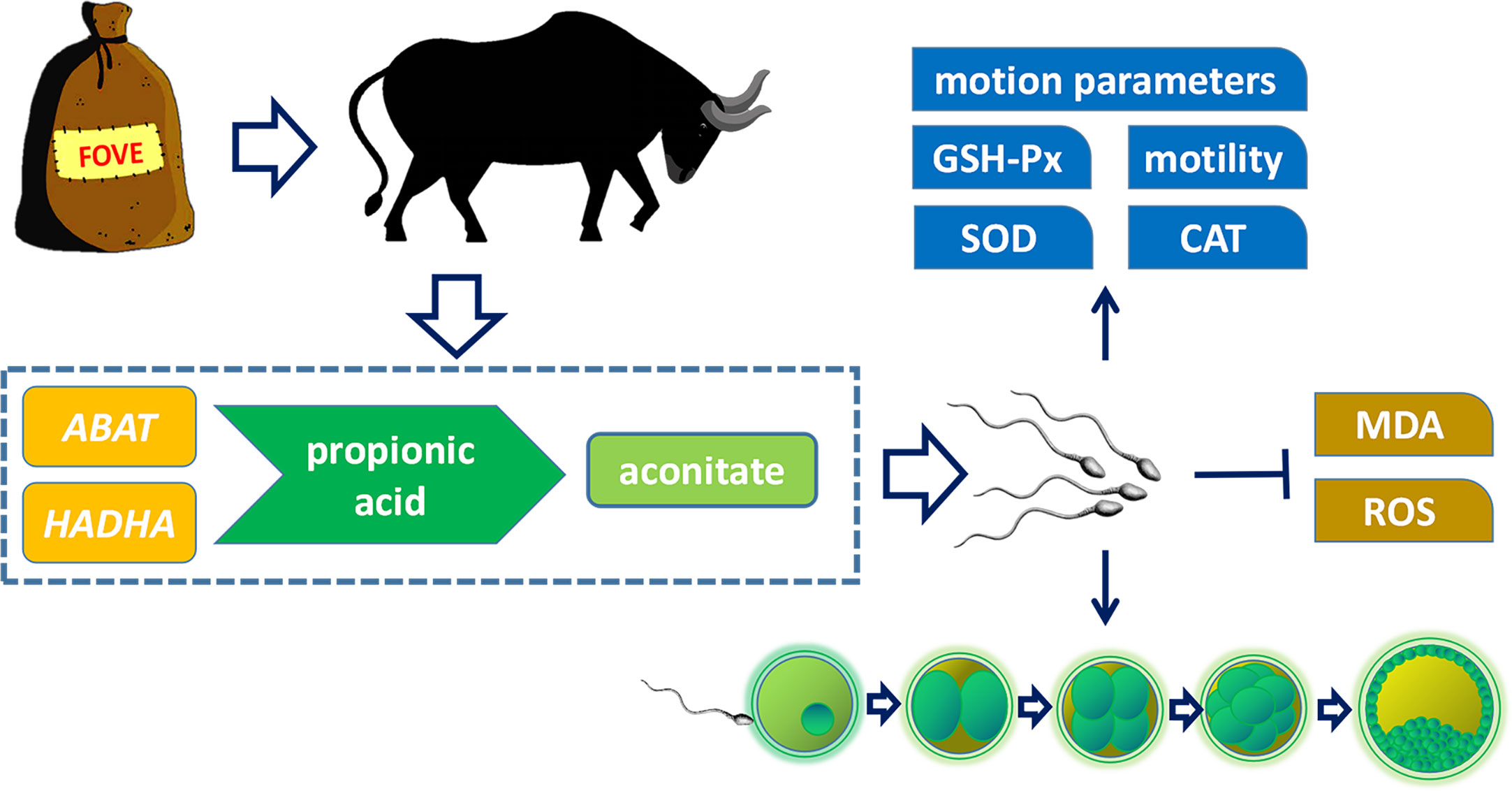
Figure 8 Effects of dietary supplementation of FO and VE on semen quality of bulls. The combination of FO and VE in diet can improve frozen-thawed sperm motility, motion parameters, antioxidant capacity and in vitro fertilization ability, which may be caused by the regulation of ABAT and HADHA genes and aconitate content in propionic acid metabolism pathway.
5 Conclusions
In conclusion, combined supplementation of FO and VE in bull diets could improve frozen-thawed sperm motion parameters, IVF rate and subsequent embryonic development by enhancing antioxidant capacity, which may be related to increased aconitic acid via the ABAT and AHDHA genes involved in the propionic acid metabolism pathway.
Data availability statement
The original contributions presented in the study are included in the article/Supplementary Material. Further inquiries can be directed to the corresponding authors.
Ethics statement
The animal study was reviewed and approved by Jilin Agricultural University in P.R. China and approved by the Experimental Animal Welfare and Ethics Committee of Jilin Agricultural University. Written informed consent was obtained from the owners for the participation of their animals in this study.
Author contributions
CY wrote the paper. KZ and XM performed the experiments. ZW and JZ did the statistical analysis. HL collected the samples and data. WL and JW edited and reviewed the paper. All authors contributed to the article and approved the submitted version.
Funding
This work was supported by the 14th Five-Year Key Research and Development Special Sub-project of the Ministry of Science and Technology (2021YFF1000701); Young and middle-aged scientific and technological innovation leaders and teams in Jilin Province (20200301031RQ); and the China Agriculture Research System of MOF and MARA (CARS-37).
Acknowledgments
We thank Changchun Xinmu Technology Co., Ltd. for providing experimental animals and samples and Charles Allan, PhD, from Liwen Bianji (Edanz) (www.liwenbianji.cn/), for editing the English text of a draft of this manuscript.
Conflict of interest
The authors declare that the research was conducted in the absence of any commercial or financial relationships that could be construed as a potential conflict of interest.
Publisher’s note
All claims expressed in this article are solely those of the authors and do not necessarily represent those of their affiliated organizations, or those of the publisher, the editors and the reviewers. Any product that may be evaluated in this article, or claim that may be made by its manufacturer, is not guaranteed or endorsed by the publisher.
References
1. Gliozzi TM, Zaniboni L, Maldjian A, Luzi F, Maertens L, Cerolini S. Quality and lipid composition of spermatozoa in rabbits fed DHA and vitamin e rich diets. Theriogenology (2009) 71(6):910–9. doi: 10.1016/j.theriogenology.2008.10.022
2. Wathes DC, Abayasekara DR, Aitken RJ. Polyunsaturated fatty acids in male and female reproduction. Biol Reprod (2007) 77(2):190–201. doi: 10.1095/biolreprod.107.060558
3. Mattos R, Staples CR, Thatcher WW. Effects of dietary fatty acids on reproduction in ruminants. Rev Reprod (2000) 5(1):38–45. doi: 10.1530/ror.0.0050038
4. Sugasini D, Lokesh BR. Curcumin and linseed oil co-delivered in phospholipid nanoemulsions enhances the levels of docosahexaenoic acid in serum and tissue lipids of rats. Prostaglandins Leukot Essent Fatty Acids (2017) 119:45–52. doi: 10.1016/j.plefa.2017.03.007
5. Kakilashvili B, Zurabashvili DZ, Turabelidze DG, Shanidze LA, Parulava GK. [The fatty acid composition of ordinary flax seed oil (Linum usitatissimum l.) cultivated in Georgia and its byological activity]. Georgian Med News (2014) 227:86–8.
6. Surai P, Kostjuk I, Wishart G, Macpherson A, Speake B, Noble R, et al. Effect of vitamin e and selenium supplementation of cockerel diets on glutathione peroxidase activity and lipid peroxidation susceptibility in sperm, testes, and liver. Biol Trace Elem Res (1998) 64(1-3):119–32. doi: 10.1007/BF02783329
7. Akiyama M. [In vivo scavenging effect of ethylcysteine on reactive oxygen species in human semen]. Nihon Hinyokika Gakkai Zasshi (1999) 90(3):421–8. doi: 10.5980/jpnjurol1989.90.421
8. Wilson MJ, Kaye D, Smith WE, Quach HT, Sinha AA, Vatassery GT. Effect of vitamin e deficiency on the growth and secretory function of the rat prostatic complex. Exp Mol Pathol (2003) 74(3):267–75. doi: 10.1016/S0014-4800(03)00021-2
9. Yue D, Yan L, Luo H, Xu X, Jin X. Effect of vitamin e supplementation on semen quality and the testicular cell membranal and mitochondrial antioxidant abilities in aohan fine-wool sheep. Anim Reprod Sci (2010) 118(2-4):217–22. doi: 10.1016/j.anireprosci.2009.08.004
10. Li F, Cui L, Yu D, Hao H, Liu Y, Zhao X, et al. Exogenous glutathione improves intracellular glutathione synthesis via the gamma-glutamyl cycle in bovine zygotes and cleavage embryos. J Cell Physiol (2019) 234(5):7384–94. doi: 10.1002/jcp.27497
11. Rodriguez-Martinez H. State of the art in farm animal sperm evaluation. Reprod Fertil Dev (2007) 19(1):91–101. doi: 10.1071/RD06104
12. Kumar A, Kroetsch T, Blondin P, Anzar M. Fertility-associated metabolites in bull seminal plasma and blood serum: 1H nuclear magnetic resonance analysis. Mol Reprod Dev (2015) 82(2):123–31. doi: 10.1002/mrd.22450
13. Jodar M, Selvaraju S, Sendler E, Diamond MP, Krawetz SA. The presence, role and clinical use of spermatozoal RNAs. Hum reprod update. (2013) 19(6):604–24. doi: 10.1093/humupd/dmt031
14. Jodar M, Sendler E, Moskovtsev SI, Librach CL, Goodrich R, Swanson S, et al. Absence of sperm RNA elements correlates with idiopathic male infertility. Sci Transl Med (2015) 7(295):295re6. doi: 10.1126/scitranslmed.aab1287
15. Lin Y, Cheng X, Mao J, Wu D, Ren B, Xu SY, et al. Effects of different dietary n-6/n-3 polyunsaturated fatty acid ratios on boar reproduction. Lipids Health Dis (2016) 15(31):8–17. doi: 10.1186/s12944-016-0193-8
16. Hong Z, Hailing L, Hui M, Guijie Z. Effect of vitamin e supplementation on development of reproductive organs in Boer goat. Anim Reprod Sci (2009) 113(1-4):93–101. doi: 10.1016/j.anireprosci.2008.05.076
17. Kargar R, Forouzanfar M, Ghalamkari G, Nasr EM. Dietary flax seed oil and/or vitamin e improve sperm parameters of cloned goats following freezing-thawing. Cryobiology (2017) 74(2):110–114. doi: 10.1016/j.cryobiol.2016.11.007
18. Alberdi-Cedeño J, Ibargoitia ML, Guillén MD. A global study by 1H NMR spectroscopy and SPME-GC/MS of the in vitro digestion of virgin flaxseed oil enriched or not with mono-, di- or tri-phenolic derivatives. antioxidant efficiency of these compounds. Antioxidants (Basel Switzerland) (2020) 9(4):312. doi: 10.3390/antiox9040312
19. Memon AA, Wahid H, Rosnina Y, Goh YM, Ebrahimi M, Nadia FM. Effect of antioxidants on post thaw microscopic, oxidative stress parameter and fertility of Boer goat spermatozoa in tris egg yolk glycerol extender. Anim Reprod Sci (2012) 136(1-2):55–60. doi: 10.1016/j.anireprosci.2012.10.020
20. Ma X, Zhang S, Zhang M, Zhu Y, Ma P, Yang S, et al. TRIM28 down-regulation on methylation imprints in bovine preimplantation embryos - ERRATUM. Zygote (2019) 27(3):191. doi: 10.1017/S0967199419000108
21. Parrish JJ, Krogenaes A, Susko-Parrish JL. Effect of bovine sperm separation by either swim-up or percoll method on success of in vitro fertilization and early embryonic development. Theriogenology (1995) 44(6):859–69. doi: 10.1016/0093-691X(95)00271-9
22. Jain AP, Aggarwal KK, Zhang PY. Omega-3 fatty acids and cardiovascular disease. Eur Rev Med Pharmacol Sci (2015) 19(3):441–5.
23. Li W, Tang D, Li F, Tian H, Yue X, Li F, et al. Supplementation with dietary linseed oil during peri-puberty stimulates steroidogenesis and testis development in rams. Theriogenology (2017) 102(10):10–15. doi: 10.1016/j.theriogenology.2017.07.002
24. Mourvaki E, Cardinali R, Dal Bosco A, Corazzi L, Castellini C. Effects of flaxseed dietary supplementation on sperm quality and on lipid composition of sperm subfractions and prostatic granules in rabbit. Theriogenology (2010) 73(5):629–37. doi: 10.1016/j.theriogenology.2009.10.019
25. Gonzalez-Moran MG, Guerra-Araiza C, Campos MG, Camacho-Arroyo I. Histological and sex steroid hormone receptor changes in testes of immature, mature, and aged chickens. Domest Anim Endocrinol (2008) 35(4):371–9. doi: 10.1016/j.domaniend.2008.08.001
26. Feng Y, Ding Y, Liu J, Tian Y, Yang Y, Guan S, et al. Effects of dietary omega-3/omega-6 fatty acid ratios on reproduction in the young breeder rooster. BMC Vet Res (2015) 11:73–9. doi: 10.1186/s12917-015-0394-9
27. Sonmez M, Turk G, Yuce A. The effect of ascorbic acid supplementation on sperm quality, lipid peroxidation and testosterone levels of male wistar rats. Theriogenology (2005) 63(7):2063–72. doi: 10.1016/j.theriogenology.2004.10.003
28. Cerolini S, Zaniboni L, Maldjian A, Gliozzi T. Effect of docosahexaenoic acid and alpha-tocopherol enrichment in chicken sperm on semen quality, sperm lipid composition and susceptibility to peroxidation. Theriogenology (2006) 66(4):877–86. doi: 10.1016/j.theriogenology.2006.02.022
29. Surai PF, Noble RC, Sparks NH, Speake BK. Effect of long-term supplementation with arachidonic or docosahexaenoic acids on sperm production in the broiler chicken. J Reprod Fertil (2000) 120(2):257–64. doi: 10.1530/reprod/120.2.257
30. Sahoo DK, Roy A, Bhanja S, Chainy GB. Hypothyroidism impairs antioxidant defence system and testicular physiology during development and maturation. Gen Comp Endocrinol (2008) 156(1):63–70. doi: 10.1016/j.ygcen.2007.11.007
31. Schmid-Lausigk Y, Aurich C. Influences of a diet supplemented with linseed oil and antioxidants on quality of equine semen after cooling and cryopreservation during winter. Theriogenology (2014) 81(7):966–73. doi: 10.1016/j.theriogenology.2014.01.021
32. Zanussi HP, Shariatmadari F, Sharafi M, Ahmadi H. Dietary supplementation with flaxseed oil as source of omega-3 fatty acids improves seminal quality and reproductive performance in aged broiler breeder roosters. Theriogenology (2019) 130:41–48. doi: 10.1016/j.theriogenology.2019.02.030
33. Bartolacci A, Pagliardini L, Makieva S, Salonia A, Papaleo E, Vigano P. Abnormal sperm concentration and motility as well as advanced paternal age compromise early embryonic development but not pregnancy outcomes: a retrospective study of 1266 ICSI cycles. J Assist Reprod Genet (2018) 35(10):1897–903. doi: 10.1007/s10815-018-1256-8
34. Khatun A, Rahman MS, Ryu DY, Kwon WS, Pang MG. Elevated aminopeptidase n affects sperm motility and early embryo development. PloS One (2017) 12(8):e0184294. doi: 10.1371/journal.pone.0184294
35. Liao QY, Huang B, Zhang SJ, Chen J, Chen G, Li KZ, et al. Influence of different quality sperm on early embryo morphokinetic parameters and cleavage patterns: A retrospective time-lapse study. Curr Med Sci (2020) 40(5):960–7. doi: 10.1007/s11596-020-2272-3
36. Bradley J, Swann K. Mitochondria and lipid metabolism in mammalian oocytes and early embryos. Int J Dev Biol (2019) 63(3-4-5):93–103. doi: 10.1387/ijdb.180355ks
37. Guerin P, El MS, Menezo Y. Oxidative stress and protection against reactive oxygen species in the pre-implantation embryo and its surroundings. Hum Reprod Update (2001) 7(2):175–89. doi: 10.1093/humupd/7.2.175
38. Tesarik J, Mendoza C, Greco E. Paternal effects acting during the first cell cycle of human preimplantation development after ICSI. Hum Reprod (2002) 17(1):184–9. doi: 10.1093/humrep/17.1.184
39. Lan KC, Lin YC, Chang YC, Lin HJ, Tsai YR, Kang HY. Limited relationships between reactive oxygen species levels in culture media and zygote and embryo development. J Assist Reprod Genet (2019) 36(2):325–34. doi: 10.1007/s10815-018-1363-6
40. Xiao L, Hu J, Song L, Zhang Y, Dong W, Jiang Y, et al. Profile of melatonin and its receptors and synthesizing enzymes in cumulus-oocyte complexes of the developing sheep antral follicle-a potential estradiol-mediated mechanism. Reprod Biol Endocrinol (2019) 17(1):1. doi: 10.1186/s12958-018-0446-7
41. Marco-Jimenez F, Llobat L, Vicente JS. Effects of lanosterol on in vitro maturation of porcine oocytes. Anim Reprod Sci (2010) 117(3-4,):288–94. doi: 10.1016/j.anireprosci.2009.04.008
42. Lee S, Jin JX, Khoirinaya C, Kim GA, Lee BC. Lanosterol influences cytoplasmic maturation of pig oocytes in vitro and improves preimplantation development of cloned embryos. Theriogenology (2016) 85(4):575–84. doi: 10.1016/j.theriogenology.2015.09.041
43. Kuang W, Zhang J, Lan Z, Deepak R, Liu C, Ma Z, et al. SLC22A14 is a mitochondrial riboflavin transporter required for sperm oxidative phosphorylation and male fertility. Cell Rep (2021) 35(3):109025. doi: 10.1016/j.celrep.2021.109025
44. Barile M, Giancaspero TA, Leone P, Galluccio M, Indiveri C. Riboflavin transport and metabolism in humans. J Inherit Metab Dis (2016) 39(4):545–57. doi: 10.1007/s10545-016-9950-0
45. Suwannasom N, Kao I, Pruss A, Georgieva R, Baumler H. Riboflavin: The health benefits of a forgotten natural vitamin. Int J Mol Sci (2020) 21(3):950–71. doi: 10.3390/ijms21030950
46. Adedara IA, Omole O, Okpara ES, Fasina OB, Ayeni MF, Ajayi OM, et al. Impact of prepubertal exposure to dietary protocatechuic acid on the hypothalamic-pituitary-testicular axis in rats. Chem Biol Interact (2018) 290:99–109. doi: 10.1016/j.cbi.2018.05.013
47. Adedara IA, Okpara ES, Busari EO, Omole O, Owumi SE, Farombi EO. Dietary protocatechuic acid abrogates male reproductive dysfunction in streptozotocin-induced diabetic rats via suppression of oxidative damage, inflammation and caspase-3 activity. Eur J Pharmacol (2019) 849:30–42. doi: 10.1016/j.ejphar.2019.01.033
48. Owumi SE, Adedara IA, Farombi EO, Oyelere AK. Protocatechuic acid modulates reproductive dysfunction linked to furan exposure in rats. Toxicology (2020) 442:152556–66. doi: 10.1016/j.tox.2020.152556
49. Darr CR, Varner DD, Teague S, Cortopassi GA, Datta S, Meyers SA. Lactate and pyruvate are major sources of energy for stallion sperm with dose effects on mitochondrial function, motility, and ROS production. Biol Reprod (2016) 95(2):34. doi: 10.1095/biolreprod.116.140707
50. Menezes EB, Velho A, Santos F, Dinh T, Kaya A, Topper E, et al. Uncovering sperm metabolome to discover biomarkers for bull fertility. BMC Genomics (2019) 20(1):714. doi: 10.1186/s12864-019-6074-6
51. Paventi G, Lessard C, Bailey JL, Passarella S. In boar sperm capacitation l-lactate and succinate, but not pyruvate and citrate, contribute to the mitochondrial membrane potential increase as monitored via safranine O fluorescence. Biochem Biophys Res Commun (2015) 462(3):257–62. doi: 10.1016/j.bbrc.2015.04.128
52. Andersen JM, Ronning PO, Herning H, Bekken SD, Haugen TB, Witczak O. Fatty acid composition of spermatozoa is associated with BMI and with semen quality. Andrology (2016) 4(5):857–65. doi: 10.1111/andr.12227
53. Tang M, Liu BJ, Wang SQ, Xu Y, Han P, Li PC, et al. The role of mitochondrial aconitate (ACO2) in human sperm motility. Syst Biol Reprod Med (2014) 60(5):251–6. doi: 10.3109/19396368.2014.915360
54. Zhao G, Li N, Li S, Wu W, Wang X, Gu J. High methylation of the 4-aminobutyrate aminotransferase gene predicts a poor prognosis in patients with myelodysplastic syndrome. Int J Oncol (2019) 54(2):491–504. doi: 10.3892/ijo.2018.4652
55. Arnaboldi L, Ossoli A, Giorgio E, Pisciotta L, Lucchi T, Grigore L, et al. LIPA gene mutations affect the composition of lipoproteins: Enrichment in ACAT-derived cholesteryl esters. Atherosclerosis (2020) 297:8–15. doi: 10.1016/j.atherosclerosis.2020.01.026
56. Maeyashiki C, Oshima S, Otsubo K, Kobayashi M, Nibe Y, Matsuzawa Y, et al. HADHA, the alpha subunit of the mitochondrial trifunctional protein, is involved in long-chain fatty acid-induced autophagy in intestinal epithelial cells. Biochem Biophys Res Commun (2017) 484(3):636–41. doi: 10.1016/j.bbrc.2017.01.159
57. Houten SM, Denis S, Argmann CA, Jia Y, Ferdinandusse S, Reddy JK, et al. Peroxisomal l-bifunctional enzyme (Ehhadh) is essential for the production of medium-chain dicarboxylic acids. J Lipid Res (2012) 53(7):1296–303. doi: 10.1194/jlr.M024463
58. Schmeisser K, Mansfeld J, Kuhlow D, Weimer S, Priebe S, Heiland I, et al. Role of sirtuins in lifespan regulation is linked to methylation of nicotinamide. Nat Chem Biol (2013) 9(11):693–700. doi: 10.1038/nchembio.1352
59. Huang R, Li Z, Zhu X, Yan P, Song D, Yin H, et al. Collagen type III alpha 1 chain regulated by GATA-binding protein 6 affects type II IFN response and propanoate metabolism in the recurrence of lower grade glioma. J Cell Mol Med (2020) 24(18):10803–15. doi: 10.1111/jcmm.15705
60. Fu L, An Q, Zhang K, Liu Y, Tong Y, Xu J, et al. Quantitative proteomic characterization of human sperm cryopreservation: using data-independent acquisition mass spectrometry. BMC Urol (2019) 19(1):133. doi: 10.1186/s12894-019-0565-2
Keywords: cryopreservation, bull semen, flaxseed oil, vitamin E, metabolomics, transcriptomics
Citation: Yuan C, Zhang K, Wang Z, Ma X, Liu H, Zhao J, Lu W and Wang J (2023) Dietary flaxseed oil and vitamin E improve semen quality via propionic acid metabolism. Front. Endocrinol. 14:1139725. doi: 10.3389/fendo.2023.1139725
Received: 07 January 2023; Accepted: 15 March 2023;
Published: 14 April 2023.
Edited by:
Asim Ali, COMSATS University Islamabad, PakistanReviewed by:
Wuzi Dong, Northwest A&F University, ChinaSaleh Rahmati, Islamic Azad University System, Iran
Copyright © 2023 Yuan, Zhang, Wang, Ma, Liu, Zhao, Lu and Wang. This is an open-access article distributed under the terms of the Creative Commons Attribution License (CC BY). The use, distribution or reproduction in other forums is permitted, provided the original author(s) and the copyright owner(s) are credited and that the original publication in this journal is cited, in accordance with accepted academic practice. No use, distribution or reproduction is permitted which does not comply with these terms.
*Correspondence: Jing Zhao, emhhb2ppbmdAamxhdS5lZHUuY24=; Wenfa Lu, bHZ3ZW5mYUBqbGF1LmVkdS5jbg==; Jun Wang, anVud2FuZ0BqbGF1LmVkdS5jbg==