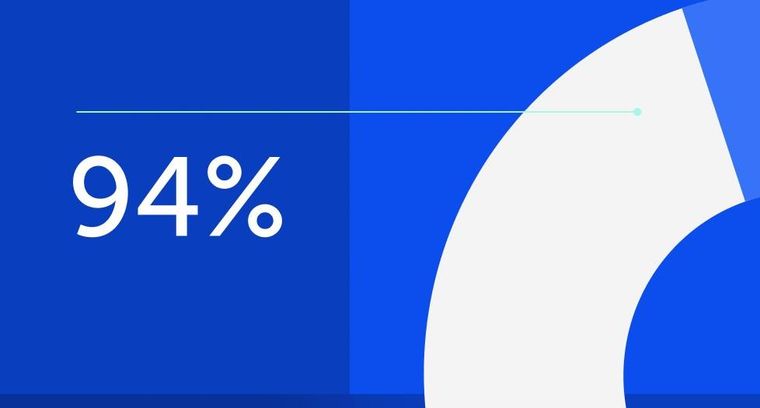
94% of researchers rate our articles as excellent or good
Learn more about the work of our research integrity team to safeguard the quality of each article we publish.
Find out more
ORIGINAL RESEARCH article
Front. Endocrinol., 12 April 2023
Sec. Cancer Endocrinology
Volume 14 - 2023 | https://doi.org/10.3389/fendo.2023.1138100
This article is part of the Research TopicNew molecular pathways in thyroid cancer and pathophysiology: Role of coding and noncoding genesView all 12 articles
Purpose: Genotyping is fundamental in papillary thyroid cancer (PTC) and helps to enhance diagnosis and prognosis and determine appropriate treatments. The phenotype-genotype association in PTC was previously studied, with BRAF V600E characterizing classic PTC and tall-cell PTC and RAS mutations characterizing follicular-variant PTC. In clinic, some non-classical histological subtypes of PTC were also identified, however, their genotype remains unclear. In this study, we collected samples of these non-classical PTC after the exclusion of classic phenotypes and examined their phenotypes, genotype and the relationship between phenotype and genotype.
Methods: We screened out non-classical PTC by excluding classical PTC from 1,059 different thyroid samples, and a total of 24 cases was obtained and described from the morphological features, which is rare in differentiated PTC. DNA/RNA sequencing was performed using 18 available samples to describe the genetic features.
Results: PTC with the non-classical phenotype were characterized cuboidal to low columnar tumor cells with subtle nuclear features of PTC and without discernible nuclear elongation, concurrently with dense microfollicles, delicate papillae or solid nodules with delicate fibrovascular cores. They were associated with lymphatic vessel invasion (P<0.001) but not with a worse prognosis (P=0.791). Gene fusions were identified in 14 of 18 (77.8%) cases, including eight fusions of NTRK and six fusions of RET. The high percentage of fusions in this papillary thyroid cancer subgroup suggested a correlation of gene fusions with the phenotype that does not belong to the BRAF V600E-mutant or RAS-mutant group.
Conclusions: Our study retrospectively screened a large cohort of different thyroid tissue samples, and presented the histopathological and genetic features of a non-classical phenotype of PTC from 24 patients. It may contribute to diagnose in PTC, and patients of these non-classical phenotype may benefit from targeted therapy, compared to a natural patient cohort without selection.
Globally, the incidence of thyroid cancer has markedly increased in the past 30 years (1, 2), and the prevalence of diverse histological and genetic characteristics has changed over time (3). With the exception of medullary thyroid cancer, all thyroid cancers are derived from follicular cells. About 80% to 85% of all thyroid cancers are papillary thyroid cancers (PTCs) (4–6), named for their papillary histological architecture. PTCs include several subtypes, such as classic PTC (CPTC), follicular-variant PTC (FVPTC), tall-cell PTC (TCPTC), and a few other rare variants (7, 8).
BRAF V600E and RAS mutations have been identified as the primary genetic drivers in thyroid carcinomas (9–11), followed by fusions involving RET (12) and other receptor tyrosine kinases (13). It is worthwhile pointing out that these mutations are almost always mutually exclusive (14). The BRAF V600E mutation is the most frequent genetic change in PTC, with a prevalence between 30 to 80% (15–17), and appears to be associated with a higher risk of cancer recurrence (18). The RAS oncogene family includes HRAS, NRAS, and KRAS. The prevalence of RAS mutations in thyroid cancer is approximately 20–40%, and the mutations are found in follicular lesions, including adenomas, carcinomas, and FVPTCs (19). Differentiated thyroid tumors harboring RAS mutations without co-alterations have an excellent prognosis (20). The Cancer Genome Atlas (TCGA) described a genomic landscape of 496 PTCs and confirmed the phenotype-genotype association in PTCs, with BRAF V600E characterizing CPTC and TCPTC and RAS mutations characterizing FVPTC (21).
RET fusion can result in oncogenic activation and occurs in about 5–35% of PTCs (22–24), whereas the rearrangements of other RTKs such as NTRK1/3, ALK, and ROS1 translocations have been reported in a minor subset of PTCs (25–31). The associations of gene fusions with the outcome of the disease are not yet well understood. Yuri E Nikiforov’s group described the morphological features of ETV6-NTRK3-positive PTC in adults without radiation exposure with the tumors exhibiting an admixture of papillary and follicular areas, clear cell or oncocytic foci with overt nuclear features of PTC, interspersed bland areas, glomeruloid follicles, reversed nuclear polarization and cytoplasmic vacuolization (32). However, this study did not propose a clear morphological standard for NTRK fusion-driven PTCs. At the same time, there are PTCs with histological characteristics that are non-classical from known pathological subtypes, and their genotypes are unclear. Thus, the relationship between morphological characteristics and genotypes still warrants further investigation.
Herein, 1,059 patients with thyroid cancer, who underwent surgery from 2008 to 2015, were retrospectively analyzed. A total of 24 PTC cases were identified according to morphological features that are different from the known subtypes. Molecular analyses were performed and revealed a high prevalence of gene fusion, while no mutations in BRAF, RAS, or TERT genes were detected. Identification of the relationship between these cytological morphologies and genomic fusions or clinical features will facilitate the rationalization of clinical treatment strategies.
The study was conducted according to the guidelines of the Declaration of Helsinki, and approved by the Ethics Committee of the First Affiliated Hospital College of Medicine, Zhejiang University (NO. IIT20210692A). As a retrospective research, the data are anonymous, the requirement for informed consent was therefore waived.
A total of 1,059 thyroid cancer tissues were collected from patients who underwent thyroid surgery at The First Affiliated Hospital, Zhejiang University School of Medicine (Hangzhou, China) between 2008 and 2015. All available samples were fresh frozen thyroid tissues. Detailed clinical and pathological data were collected from patients harboring primary dominant PTCs with dimensions ≥1 cm.
The genomic DNA was isolated using a commercial kit (QIAamp DNA Mini Kit; QIAGEN, Hilden, Germany). Exon 15 of the BRAF gene was amplified and then directly sequenced for BRAF V600E mutation as described previously (33).
The DNA and RNA extracted from PTC samples with the non-classical phenotype were analyzed with the Onco-Thyroid™ panel (Genetron Health, Beijing, China, gene list is in Table S1) using one-step multiplex PCR targeted amplicons, as previously described (34).
Fusioncapture™ (GenetronHealth), which is a 395-gene RNA panel (gene list is in Table S2), was conducted to identify gene fusions in the PTC samples with the non-classical phenotype. The specific method is stated in the previous paper (35).
Genomic DNA from PTC samples with the non-classical phenotype was fragmented for constructing a library using KAPA Hyper Prep kits (KAPA, KK8504) and captured using the Agilent SureSelect XT Human All Exon v5 kit (Agilent, Santa Clara, CA, USA). High-throughput sequencing was performed as previously described (36).
Comparisons of categorical variables were performed by Pearson’s chi-squared test. The analyses and data presentation were carried out using GraphPad Prism (8.0.1).
Figure 1 illustrates the study sample selection workflow. Of the 1,059 patients who underwent thyroid surgery at The First Affiliated Hospital, Zhejiang University School of Medicine (Hangzhou, China) between 2008 and 2015, 25 patients whose tumors were not primary differentiated PTC were first excluded, then 223 patients with papillary thyroid microcarcinoma were excluded. Finally, 787 PTC patients with common histopathologies, such as CPTC, FVPTC, TCPTC, oncocytic variant of PTC, the Warthin-like variant of PTC, and the diffuse sclerosing variant of PTC, were excluded. The final study sample consisted of 24 adult participants with no prior history of radiation exposure with a phenotype non-classical from common PTCs. The tumors were characterized as the following criteria: 1. Cuboidal to low-columnar tumor cells with subtle nuclear features of PTC and without discernible nuclear elongation. 2. Composed of dense microfollicles, delicate papillae or solid nodules with delicate fibrovascular cores.
The clinical and pathologic features of PTC patients with the non-classical phenotype are summarized (Table 1, Table S3). The prevalence of PTC samples measuring at least 1 cm was 3.0% (24/811). There were 19 (79.2%) females and 5 (20.8%) males. The age at initial diagnosis ranged from 19 to 70 years (mean 42.1 years). The tumor size ranged from 1.0 cm to 7.0 cm (mean 1.8 cm). Moreover, the tumors were often described as solitary nodules (87.5%). Additionally, 416 PTC patients with normal phenotypes and clinical information were also included in the analysis (Table 1, Table S4). Overall, the non-classical phenotype was significantly associated with lymphatic vessel invasion, a strong prognostic factor for poor clinical outcomes in lymph node-negative patients with breast carcinoma, bladder cancer, non-small cell lung cancer, colorectal cancer and differentiated thyroid carcinoma (37–42). While tumor recurrence was 6.3% (26 of 416) in patients with a normal phenotype, it was 0% (0 of 24) in patients with the non-classical phenotype (P = 0.791).
Microscopic examination revealed that all 24 tumors were lack of normal follicular structure of thyroid tissue and normal cell with small round-flat nucleus (Figure 2A). They were uniformly composed of small cuboidal to low columnar epithelial cells. The nucleus had subtle characteristics nuclear features of PTC and without significant elongation, in contrast, CPTC composed of columnar epithelial cells and the nucleus had classic characteristics nuclear features of PTC and with significant elongation (Figure 2B). Some nuclei were pushed by the cytoplasm and formed variable shapes such as rings and triangles. Furthermore, the cytoplasm was transparent or pale, and the borders of tumor cells were ill-defined. The tumors with non-classical phenotype showed three main patterns of growth. Firstly, it is the microfollicular pattern, which characterized by dense microfollicles (Figure 2C). The second one is papillary pattern, which characterized by monotonous delicate papillae with a fine central fibrovascular stalk (Figure 2D), of which the delicate papillae are quite different from those in CPTC (Figure 2B). Occasionally, tumors presented glomeruloid bodies resembling abortive papillae (Figure 2E). The last pattern is solid growth, characterized by delicate fibrovascular cores within the solid nodules or islands (Figure 2F). Tumors with the non-classical phenotype were mostly poorly circumscribed and arranged in pushing, smooth-bordered nodules or islands of variable sizes, as separated by normal thyroid tissue (Figure 3A) or broad fibrous septa (jigsaw-like pattern) (Figure 3B). Often, there were small satellite nodules near or away from the dominant tumor (Figure 3C). Conventional aggressive features of PTC, including extrathyroidal extension, lymphatic vessel invasion, and lymph node metastases, were present in 6/24, 14/24, and 14/24 cases, respectively (Table 1, Table S3).
Figure 2 Growth patterns of Papillary Thyroid Carcinomas tumors with non-classical phenotype and controls in 100X and 400X magnification. (A) Normal thyroid. (B) Classic PTC: low magnification shows the papillae found in CPTC usually vary in size and shape and some have broader central stalks. The arrow shows the papillae with broader central stalks. High magnification shows that the papillae composed of columnar epithelial cells. The nucleus has classic characteristics nuclear features of PTC and with significant elongation. (C) Dense microfollicles are composed of small cuboidal epithelia with round nuclei intermixed with some variable shaped nuclei. The tumor cells have transparent or pale cytoplasm with ill-defined borders. Arrows show the microfollicles. (D) Tumor is dominantly composed of delicate papillae (arrow). (E) Occasionally tumors present glomeruloid bodies resembling abortive papillae. (F) Solid growth pattern, characterized by delicate fibrovascular cores within the solid nodules. The tumor cells have nucleus without significant elongation.
Figure 3 Histopathological features of Papillary Thyroid Carcinomas tumors with non-classical phenotype. (A) In 40X and 100X magnification, tumor tissue arranged in smooth-bordered nodules (arrow), as separated by normal thyroid tissue. (B) In 40X and 100X magnification, tumor tissue arranged in a geographic, jigsaw-like pattern within a broad fibrous background (arrow). (C) 40X and 400X magnification shows small satellite nodules (arrow) near or away from the dominant tumor.
BRAF V600E was analyzed using ARMS-PCR (AmoyDx, China) in all the tumors with the non-classical phenotype (n = 24), and molecular analyses revealed non-BRAF V600E. Meanwhile, the percentage of BRAF V600E positive patients in our control cohort was 72.6% (302/416). For the cases with remaining tumor samples (n = 18), Onco-thyroid panel (18/18), RNA panel (13/18), and whole-exome (15/18) sequencing were performed (Figures 4A–D, Tables S5–S7) to acquire additional genetic information. Fourteen tumors (77.8%, 14/18) harboring gene fusions were recognized by the Onco-thyroid panel and/or Fusioncapture sequencing, seven of which were ETV6-NTRK3, one was TPR-NTRK1, and the others were fusions of the RET gene (Figures S1, S2, Table S3). RET fusions were most frequent (33/484, 6.8%) in the TCGA study, and the most common partner gene was CCDC6 (21/33, 63.6%) (21). Meanwhile, NCOA4-RET was recognized in four of cases (4/6, 66.7%) with the non-classical phenotype in this study and no CCDC6-RET was identified. More gene mutations, copy number variation (CNV), and tumor mutation burden (TMB) were detected and calculated by whole-exome sequencing, and the results showed that there were no other well-known driver mutations of PTC (Table S6). Notably, driver variations were not detected in 4 of the 13 patients, which indicates that the molecular mechanism of thyroid cancer still warrants further investigation. Extensive fibrosis was commonly encountered in our series, and cases with RET fusion (6/6) were more prone to severe fibrosis than those with the NTRK fusion (1/8). Besides, more patients with NTRK fusions (4/8) in the group appeared to have a high level of thyroid peroxidase antibody (TPO) compared to patients with RET fusions (1/6) (Table S3). However, the result of Fisher’s exact test suggested no statistically difference in TPO levels (P=0.3007), which may due to small sample size.
Figure 4 Landscape of genomic alterations and Kaplan-Meier analyses in Papillary Thyroid Carcinomas with non-classical phenotype. (A-D) Landscape of genomic alterations (n=24). (A) Tumor recurrence, lymph node metastasis, border, extrathyroidal extension, lymphatic vessel invasion, multifocality, tumor size, patient gender and age. (B) Fusion events detected by Onco-Thyroid Panel and Fusioncapture Panel sequencing. (C) Mutations of driver genes detected by WES. (D) Copy number changes detected by WES. (E) Kaplan-Meier analyses of the impacts of phenotype on recurrence-free survival of PTC patients (n=18).
Follow-up data were available for 18 patients (median 86 months, range 18-127 months): all patients were alive and without recurrence (Table S3). The effects of non-classical and normal phenotypes on PTC recurrence were compared. Although non-classical phenotypic tumors were more likely to have lymphatic vessel invasion (Table 1), they did not show an elevated risk for recrudescence (Figure 4E).
Key genetic alterations of PTC, co-occurring or mutually exclusive, are the basis of estimated prognosis. The phenotype-genotype association in PTCs was described as BRAF V600E characterizing CPTC, TCPTC and RAS mutations characterizing FVPTC (21). Numerous studies have demonstrated that co-occurrence of BRAF V600E and TERT promoter mutations defines an aggressive subgroup of PTCs (43–49). On the one hand, differentiated thyroid cancers harboring RAS mutations alone have an excellent prognosis (20). On the other hand, PTCs with a novel phenotype that do not belong to the BRAF V600E-mutant or RAS-mutant tumors should be better characterized, given that some of these tumors harbor gene fusions that can benefit from targeted therapy. As FDA approves larotrectinib and entrectinib for solid tumors with NTRK gene fusions, and pralsetinib and selpercatinib for thyroid cancers with RET gene mutations or fusions.
Herein, we retrospectively analyzed a large cohort of thyroid tissue samples, screened out well-differentiated PTCs, and excluding PTMC, CPTC, FVPTC, TCPTC, oncocytic variants of PTC, the Warthin-like variant of PTC, and the diffuse sclerosing variant of PTC. A non-classical phenotype of PTC was summarized from 24 patients. The microfollicular architecture consisted of closely-packed small follicles with scanty colloids, different from the common FVPTC. The papillary structure could be predominant or even exclusive, but it was always monotonous, delicate. In comparison, the papillae found in CPTC usually vary in size and shape and generally have broader central stalks. We presumed that these delicate papillae were formed in the following ways: (1) originating from a solid structure with delicate fibrovascular cores by cellular dehiscence (Figure S3); or (2) originating from packed microfollicles by follicular-septum cracking, which may be different from the formation of the common papillae present in CPTC (Figure S4). Meanwhile, considering that the solid growth pattern was predominant (> 50%) in our cases, a diagnosis of a solid variant of PTC (SVPTC) may also be established (50, 51), which is most commonly seen in children after the Chernobyl nuclear accident (52, 53) or in few adults without a history of radiation exposure (51), accounting for 1-3% of all PTCs (54). Different from the most common encapsulated type (65%) (54) in reported SVPTC, our general solid growth cases were almost non-encapsulated and invasive (87.5%, 7/8), and showed a lower proportion (0.76%, 8/1059) in Chinese, suggest it may be clinically rare. Furthermore, extensive fibrosis was quite common in our series. Although sometimes seen in CPTC, the tumor nests were more irregular and infiltrative. Other features, including clear or pale cytoplasm, no elongated nuclei, and smaller and shorter cells, are also of tremendous significance in these subtypes of PTC.
We selected 416 patients with a common PTC phenotype who underwent surgery in the hospital from 2008 to 2015 as a real-world data control to explore whether PTC patients with a non-classical phenotype have significant differences in clinical characteristics. The median age of PTC patients with the non-classical phenotype (42 years old) was similar to that of PTC patients with a common phenotype (44 years old) (P = 0.388). The female to male ratios were 2.5:1 and 3.8:1 in the common and non-classical phenotype of PTC groups (P = 0.424), respectively. For aggressive features such as invasive border, extrathyroidal extension, lymphatic vessel invasion, and lymph node metastases, PTCs with the non-classical phenotype showed a significant difference in lymphatic vessel invasion (P < 0.001), whereas there was no difference in tumor recurrence (P = 0.414). The results suggest that although there are differences in histopathological features between common and non-classical phenotypes of PTC, clinical characteristics and recurrence are relatively similar. Among the 24 cases of PTC with a non-classical phenotype, 18 had fresh frozen tissues stored. After Onco-Thyroid and/or RNA panel sequencing, 14 gene fusions were recognized, including 8 fusions in NTRK and 6 fusions in RET. In the thyroid, NTRK-driven malignancies are rare, and their frequency ranges from 2 to 3% in adult patients without radiation exposure (21, 55–57). However, the frequency of NTRK fusion-positive PTCs was 44.4% (8/18) in this group. RET fusions occur in 5%–10% of sporadic PTCs (58, 59). In the TCGA study, 6.8% of cases harbored a RET fusion (21). In contrast, RET fusions were observed in 33.3% (6/18) of PTCs with the non-classical phenotype in this study. The results indicate that the fusions of NTRK and RET are associated with the non-classical phenotype of PTC. Meanwhile, in this phenotype of PTC, cases with RET fusion are more prone to fibrosis than those with NTRK fusion. Various RET and NTRK fusions have been reported in PTC. The two most prevalent RET fusions are CCDC6-RET and NCOA4-RET, which account for more than 90% of all RET rearrangements (60–62). NTRK fusions discovered in thyroid tumors so far include EML4-NTRK3 (57), ETV6-NTRK3 (26, 27, 63), SQSTM1-NTRK3 (57), IRF2BP2-NTRK1 (57), TPR-NTRK1 (26, 27), TPM3-NTRK1 (25, 64), TFG-NTRK1 (64, 65), and TRIM33-NTRK1 (65). Interestingly, the common fusions of RET/NTRK3 discovered in this study are NCOA4-RET (4/6) and ETV6-NTRK3 (7/7), showing selectivity of fusion partners. Meanwhile, except for one mutation in TP53, no mutations leading to the occurrence of PTC were found in other common genes (such as AKT1, BRAF, CTNNB1, EIF1AX, EZH1, GNAS, HRAS, KRAS, NRAS, PIK3CA, RET, SPOP, TERT, TSHR, ZNF148, and so on), signaling the pivotal role of gene fusion in this group of PTC. Recently, there has been a growing interest in testing and characterizing NTRK and RET fusion genes because they are therapeutically targetable. For instance, NTRK and RET fusion-positive tumors are sensitive to inhibitors, such as larotrectinib and selpercatinib, which appear to be well tolerated and effective (58, 66, 67). Due to the limited sample size in current research, it is not yet possible to have an impact on clinical treatment options for those patients. However, our study provides a possibility that this type of non-classical PTC may be classified together to spare these patients unnecessary aggressive therapy, as they shared similar molecular and clinical features and had a relatively good prognosis.
In conclusion, our study retrospectively screened a large cohort of different thyroid tissue samples, and a non-classical phenotype of PTC was summarized from 24 patients. Herein, the histopathological and genetic features of this subgroup of PTC were presented. Our findings may facilitate diagnostic approaches in PTC, and patients of this peculiar subgroup may benefit from targeted therapy compared to a natural patient cohort without selection. However, this study was based on a small population, and genetic testing were not performed on all tissue samples. In the future, further analyses are warranted to corroborate the results.
The raw datasets presented in this study can be found in the National Omics Data Encyclopedia (NODE) database (https://www.biosino.org/node/index), accession number OEP003392.
The studies involving human participants were reviewed and approved by the Ethics Committee of the First Affiliated Hospital College of Medicine, Zhejiang University (NO. IIT20210692A). Written informed consent for participation was not required for this study in accordance with the national legislation and the institutional requirements.
Conceptualization, JZ and X-DT. Methodology, JZ, W-RW, T-HM and X-YZ. Software, H-FZ, Q-QG, and X-YZ. Validation, JZ, W-RW, W-BW, J-HZ and Y-SH. Formal Analysis, JZ, W-RW, H-FZ, Q-QG, W-BW, Y-SH, T-HM, X-YZ and X-DT. Investigation, JZ and W-RW. Resources, X-DT. Data Curation, JZ and W-RW. Writing – Original Draft Preparation, JZ, W-RW. Writing – Review & Editing, JC, T-HM and XDT. Visualization, JZ and W-RW. Funding – T-HM. Supervision, X-DT. Project Administration, X-DT. All authors contributed to the article and approved the submitted version.
This work was supported by grants from the Zhejiang Province medical and health research projects (Grant No. 2016KYB027 and Grant No. 2017KY010).
We gratefully acknowledge the participation of all patients in this study.
W-RW, J-HZ, Y-SH, JC, T-HM, X-YZ are the employees of Genetron Health Beijing Technology, Co. Ltd., Beijing, China.
The remaining authors declare that the research was conducted in the absence of any commercial or financial relationships that could be constructed as a potential conflict of interest.
All claims expressed in this article are solely those of the authors and do not necessarily represent those of their affiliated organizations, or those of the publisher, the editors and the reviewers. Any product that may be evaluated in this article, or claim that may be made by its manufacturer, is not guaranteed or endorsed by the publisher.
The Supplementary Material for this article can be found online at: https://www.frontiersin.org/articles/10.3389/fendo.2023.1138100/full#supplementary-material
Supplementary Figure 1 | The Integrative Genomics Viewer (IGV) screenshots display the reads from next-generation sequencing by Onco-Thyroid panel and reveal harbouring of gene fusions. The reference is fusion gene sequences.
Supplementary Figure 2 | The IGV screenshots display the reads from next-generation sequencing by Fusioncapture panel and reveal harbouring of gene fusions. The reference is GRCh37/hg19.
Supplementary Figure 3 | The delicate papillae result from cellular dehiscence, with rugged surface and adhesion.
Supplementary Figure 4 | Putative scheme of papillae formations. (A) The delicate papillae originate from packed microfollicles. (B) The common papillae originate from irregular follicles.
1. Li M, Dal Maso L, Vaccarella S. Global trends in thyroid cancer incidence and the impact of overdiagnosis. Lancet Diabetes Endocrinol (2020) 8:468–70. doi: 10.1016/S2213-8587(20)30115-7
2. Chen AY, Jemal A, Ward EM. Increasing incidence of differentiated thyroid cancer in the united states, 1988-2005. Cancer (2009) 115:3801–7. doi: 10.1002/cncr.24416
3. Jung CK, Little MP, Lubin JH, Brenner AV, Wells SA Jr., Sigurdson AJ, et al. The increase in thyroid cancer incidence during the last four decades is accompanied by a high frequency of BRAF mutations and a sharp increase in RAS mutations. J Clin Endocrinol Metab (2014) 99:E276–285. doi: 10.1210/jc.2013-2503
4. Jemal A, Bray F, Center MM, Ferlay J, Ward E, Forman D. Global cancer statistics. CA Cancer J Clin (2011) 61:69–90. doi: 10.3322/caac.20107
5. Gansler T, Ganz PA, Grant M, Greene FL, Johnstone P, Mahoney M, et al. Sixty years of CA: a cancer journal for clinicians. CA Cancer J Clin (2010) 60:345–50. doi: 10.3322/caac.20088
6. Pacini F, Schlumberger M, Dralle H, Elisei R, Smit JW, Wiersinga W, et al. European Consensus for the management of patients with differentiated thyroid carcinoma of the follicular epithelium. Eur J Endocrinol (2006) 154:787–803. doi: 10.1530/eje.1.02158
7. Lam AK, Lo CY, Lam KS. Papillary carcinoma of thyroid: A 30-yr clinicopathological review of the histological variants. Endocr Pathol (2005) 16:323–30. doi: 10.1385/ep:16:4:323
8. Shi X, Liu R, Basolo F, Giannini R, Shen X, Teng D, et al. Differential clinicopathological risk and prognosis of major papillary thyroid cancer variants. J Clin Endocrinol Metab (2016) 101:264–74. doi: 10.1210/jc.2015-2917
9. Garcia-Rostan G, Zhao H, Camp RL, Pollan M, Herrero A, Pardo J, et al. Tallini, g. ras mutations are associated with aggressive tumor phenotypes and poor prognosis in thyroid cancer. J Clin Oncol (2003) 21:3226–35. doi: 10.1200/JCO.2003.10.130
10. Xing M. Molecular pathogenesis and mechanisms of thyroid cancer. Nat Rev Cancer (2013) 13:184–99. doi: 10.1038/nrc3431
11. Xing M, Alzahrani AS, Carson KA, Viola D, Elisei R, Bendlova B, et al. Association between BRAF V600E mutation and mortality in patients with papillary thyroid cancer. JAMA (2013) 309:1493–501. doi: 10.1001/jama.2013.3190
12. Grieco M, Santoro M, Berlingieri MT, Melillo RM, Donghi R, Bongarzone I, et al. PTC is a novel rearranged form of the ret proto-oncogene and is frequently detected in vivo in human thyroid papillary carcinomas. Cell (1990) 60:557–63. doi: 10.1016/0092-8674(90)90659-3
13. Pierotti MA, Bongarzone I, Borrello MG, Mariani C, Miranda C, Sozzi G, et al. Rearrangements of TRK proto-oncogene in papillary thyroid carcinomas. J Endocrinol Invest (1995) 18:130–3. doi: 10.1007/BF03349721
14. Soares P, Trovisco V, Rocha AS, Lima J, Castro P, Preto A, et al. BRAF mutations and RET/PTC rearrangements are alternative events in the etiopathogenesis of PTC. Oncogene (2003) 22:4578–80. doi: 10.1038/sj.onc.1206706
15. Xing M. BRAF mutation in thyroid cancer. Endocr Relat Cancer (2005) 12:245–62. doi: 10.1677/erc.1.0978
16. Tanaka A, Matsuse M, Saenko V, Nakao T, Yamanouchi K, Sakimura C, et al. TERT mRNA expression as a novel prognostic marker in papillary thyroid carcinomas. Thyroid (2019) 29:1105–14. doi: 10.1089/thy.2018.0695
17. Sun J, Zhang J, Lu J, Gao J, Ren X, Teng L, et al. BRAF V600E and TERT promoter mutations in papillary thyroid carcinoma in Chinese patients. PloS One (2016) 11:e0153319. doi: 10.1371/journal.pone.0153319
18. Enumah S, Fingeret A, Parangi S, Dias-Santagata D, Sadow PM, Lubitz CC. BRAF(V600E) mutation is associated with an increased risk of papillary thyroid cancer recurrence. World J Surg (2020) 44:2685–91. doi: 10.1007/s00268-020-05521-2
19. An JH, Song KH, Kim SK, Park KS, Yoo YB, Yang JH, et al. RAS mutations in indeterminate thyroid nodules are predictive of the follicular variant of papillary thyroid carcinoma. Clin Endocrinol (Oxf) (2015) 82:760–6. doi: 10.1111/cen.12579
20. Xing M. Clinical utility of RAS mutations in thyroid cancer: a blurred picture now emerging clearer. BMC Med (2016) 14:12. doi: 10.1186/s12916-016-0559-9
21. Cancer Genome Atlas Research, N. Integrated genomic characterization of papillary thyroid carcinoma. Cell (2014) 159:676–90. doi: 10.1016/j.cell.2014.09.050
22. Santoro M, Moccia M, Federico G, Carlomagno F. RET gene fusions in malignancies of the thyroid and other tissues. Genes (Basel) (2020) 11(4):424. doi: 10.3390/genes11040424
23. Kim P, Jia P, Zhao Z. Kinase impact assessment in the landscape of fusion genes that retain kinase domains: a pan-cancer study. Brief Bioinform (2018) 19:450–60. doi: 10.1093/bib/bbw127
24. Nikiforov YE. Molecular analysis of thyroid tumors. Mod Pathol (2011) 24 Suppl 2:S34–43. doi: 10.1038/modpathol.2010.167
25. Rabes HM, Demidchik EP, Sidorow JD, Lengfelder E, Beimfohr C, Hoelzel D, et al. Pattern of radiation-induced RET and NTRK1 rearrangements in 191 post-chernobyl papillary thyroid carcinomas: biological, phenotypic, and clinical implications. Clin Cancer Res (2000) 6:1093–103.
26. Prasad ML, Vyas M, Horne MJ, Virk RK, Morotti R, Liu Z, et al. NTRK fusion oncogenes in pediatric papillary thyroid carcinoma in northeast united states. Cancer (2016) 122:1097–107. doi: 10.1002/cncr.29887
27. Ricarte-Filho JC, Li S, Garcia-Rendueles ME, Montero-Conde C, Voza F, Knauf JA, et al. Identification of kinase fusion oncogenes in post-Chernobyl radiation-induced thyroid cancers. J Clin Invest (2013) 123:4935–44. doi: 10.1172/JCI69766
28. Chu YH, Dias-Santagata D, Farahani AA, Boyraz B, Faquin WC, Nose V, et al. Clinicopathologic and molecular characterization of NTRK-rearranged thyroid carcinoma (NRTC). Mod Pathol (2020) 33:2186–97. doi: 10.1038/s41379-020-0574-4
29. Hamatani K, Mukai M, Takahashi K, Hayashi Y, Nakachi K, Kusunoki Y. Rearranged anaplastic lymphoma kinase (ALK) gene in adult-onset papillary thyroid cancer amongst atomic bomb survivors. Thyroid (2012) 22:1153–9. doi: 10.1089/thy.2011.0511
30. Ritterhouse LL, Wirth LJ, Randolph GW, Sadow PM, Ross DS, Liddy W, et al. ROS1 rearrangement in thyroid cancer. Thyroid (2016) 26:794–7. doi: 10.1089/thy.2016.0101
31. Yakushina VD, Lerner LV, Lavrov AV. Gene fusions in thyroid cancer. Thyroid (2018) 28:158–67. doi: 10.1089/thy.2017.0318
32. Seethala RR, Chiosea SI, Liu CZ, Nikiforova M, Nikiforov YE. Clinical and morphologic features of ETV6-NTRK3 translocated papillary thyroid carcinoma in an adult population without radiation exposure. Am J Surg Pathol (2017) 41:446–57. doi: 10.1097/PAS.0000000000000814
33. Wang W, Zhao W, Wang H, Teng X, Wang H, Chen X, et al. 3rd; teng, l. poorer prognosis and higher prevalence of BRAF (V600E) mutation in synchronous bilateral papillary thyroid carcinoma. Ann Surg Oncol (2012) 19:31–6. doi: 10.1245/s10434-011-2096-2
34. Song Y, Xu G, Ma T, Zhu Y, Yu H, Yu W, et al. Utility of a multigene testing for preoperative evaluation of indeterminate thyroid nodules: A prospective blinded single center study in China. Cancer Med (2020) 9:8397–405. doi: 10.1002/cam4.3450
35. Shi M, Wang W, Zhang J, Li B, Lv D, Wang D, et al. Identification of RET fusions in a Chinese multicancer retrospective analysis by next-generation sequencing. Cancer Sci (2022) 113:308–18. doi: 10.1111/cas.15181
36. Xie L, Yang Y, Guo W, Che D, Xu J, Sun X, et al. The clinical implications of tumor mutational burden in osteosarcoma. Front Oncol (2020) 10:595527. doi: 10.3389/fonc.2020.595527
37. Lee AH, Pinder SE, Macmillan RD, Mitchell M, Ellis IO, Elston CW, et al. Prognostic value of lymphovascular invasion in women with lymph node negative invasive breast carcinoma. Eur J Cancer (2006) 42:357–62. doi: 10.1016/j.ejca.2005.10.021
38. Bolenz C, Herrmann E, Bastian PJ, Michel MS, Wulfing C, Tiemann A, et al. Lymphovascular invasion is an independent predictor of oncological outcomes in patients with lymph node-negative urothelial bladder cancer treated by radical cystectomy: a multicentre validation trial. BJU Int (2010) 106:493–9. doi: 10.1111/j.1464-410X.2009.09166.x
39. Naito Y, Goto K, Nagai K, Ishii G, Nishimura M, Yoshida J, et al. Vascular invasion is a strong prognostic factor after complete resection of node-negative non-small cell lung cancer. Chest (2010) 138:1411–7. doi: 10.1378/chest.10-0185
40. Zlobec I, Baker K, Minoo P, Jass JR, Terracciano L, Lugli A. Node-negative colorectal cancer at high risk of distant metastasis identified by combined analysis of lymph node status, vascular invasion, and raf-1 kinase inhibitor protein expression. Clin Cancer Res (2008) 14:143–8. doi: 10.1158/1078-0432.CCR-07-1380
41. Vuong HG, Kondo T, Duong UNP, Pham TQ, Oishi N, Mochizuki K, et al. Prognostic impact of vascular invasion in differentiated thyroid carcinoma: a systematic review and meta-analysis. Eur J Endocrinol (2017) 177:207–16. doi: 10.1530/EJE-17-0260
42. Wreesmann VB, Nixon IJ, Rivera M, Katabi N, Palmer F, Ganly I, et al. Prognostic value of vascular invasion in well-differentiated papillary thyroid carcinoma. Thyroid (2015) 25:503–8. doi: 10.1089/thy.2015.0052
43. Bae JS, Kim Y, Jeon S, Kim SH, Kim TJ, Lee S, et al. Clinical utility of TERT promoter mutations and ALK rearrangement in thyroid cancer patients with a high prevalence of the BRAF V600E mutation. Diagn Pathol (2016) 11:21. doi: 10.1186/s13000-016-0458-6
44. Insilla AC, Proietti A, Borrelli N, Macerola E, Niccoli C, Vitti P, et al. TERT promoter mutations and their correlation with BRAF and RAS mutations in a consecutive cohort of 145 thyroid cancer cases. Oncol Lett (2018) 15:2763–70. doi: 10.3892/ol.2017.7675
45. Liu R, Bishop J, Zhu G, Zhang T, Ladenson PW, Xing M. Mortality risk stratification by combining BRAF V600E and TERT promoter mutations in papillary thyroid cancer: Genetic duet of BRAF and TERT promoter mutations in thyroid cancer mortality. JAMA Oncol (2017) 3:202–8. doi: 10.1001/jamaoncol.2016.3288
46. Liu X, Qu S, Liu R, Sheng C, Shi X, Zhu G, et al. TERT promoter mutations and their association with BRAF V600E mutation and aggressive clinicopathological characteristics of thyroid cancer. J Clin Endocrinol Metab (2014) 99:E1130–1136. doi: 10.1210/jc.2013-4048
47. Song YS, Lim JA, Choi H, Won JK, Moon JH, Cho SW, et al. Prognostic effects of TERT promoter mutations are enhanced by coexistence with BRAF or RAS mutations and strengthen the risk prediction by the ATA or TNM staging system in differentiated thyroid cancer patients. Cancer (2016) 122:1370–9. doi: 10.1002/cncr.29934
48. Xing M, Liu R, Liu X, Murugan AK, Zhu G, Zeiger MA, et al. BRAF V600E and TERT promoter mutations cooperatively identify the most aggressive papillary thyroid cancer with highest recurrence. J Clin Oncol (2014) 32:2718–26. doi: 10.1200/JCO.2014.55.5094
49. Matsuse M, Yabuta T, Saenko V, Hirokawa M, Nishihara E, Suzuki K, et al. TERT promoter mutations and ki-67 labeling index as a prognostic marker of papillary thyroid carcinomas: combination of two independent factors. Sci Rep (2017) 7:41752. doi: 10.1038/srep41752
50. Carcangiu ML, Zampi G, Pupi A, Castagnoli A, Rosai J. Papillary carcinoma of the thyroid. a clinicopathologic study of 241 cases treated at the university of Florence, Italy. Cancer (1985) 55:805–28. doi: 10.1002/1097-0142(19850215)55:4<805::aid-cncr2820550419>3.0.co;2-z
51. Nikiforov YE, Erickson LA, Nikiforova MN, Caudill CM, Lloyd RV. Solid variant of papillary thyroid carcinoma: incidence, clinical-pathologic characteristics, molecular analysis, and biologic behavior. Am J Surg Pathol (2001) 25:1478–84. doi: 10.1097/00000478-200112000-00002
52. Furmanchuk AW, Averkin JI, Egloff B, Ruchti C, Abelin T, Schappi W, et al. Pathomorphological findings in thyroid cancers of children from the republic of Belarus: a study of 86 cases occurring between 1986 ('post-chernobyl') and 1991. Histopathology (1992) 21:401–8. doi: 10.1111/j.1365-2559.1992.tb00423.x
53. Nikiforov Y, Gnepp DR, Fagin JA. Thyroid lesions in children and adolescents after the Chernobyl disaster: implications for the study of radiation tumorigenesis. J Clin Endocrinol Metab (1996) 81:9–14. doi: 10.1210/jcem.81.1.8550800
54. Xu B, Viswanathan K, Zhang L, Edmund LN, Ganly O, Tuttle RM, et al. The solid variant of papillary thyroid carcinoma: a multi-institutional retrospective study. Histopathology (2022) 81(2):171–82. doi: 10.1111/his.14668
55. Solomon JP, Linkov I, Rosado A, Mullaney K, Rosen EY, Frosina D, et al. NTRK fusion detection across multiple assays and 33,997 cases: diagnostic implications and pitfalls. Mod Pathol (2020) 33:38–46. doi: 10.1038/s41379-019-0324-7
56. Okamura R, Boichard A, Kato S, Sicklick JK, Bazhenova L, Kurzrock R. Analysis of NTRK alterations in pan-cancer adult and pediatric malignancies: implications for NTRK-targeted therapeutics. JCO Precis Oncol (2018) 2018. doi: 10.1200/PO.18.00183
57. Liang J, Cai W, Feng D, Teng H, Mao F, Jiang Y, et al. Genetic landscape of papillary thyroid carcinoma in the Chinese population. J Pathol (2018) 244:215–26. doi: 10.1002/path.5005
58. Subbiah V, Yang D, Velcheti V, Drilon A, Meric-Bernstam F. State-of-the-Art strategies for targeting RET-dependent cancers. J Clin Oncol (2020) 38:1209–21. doi: 10.1200/JCO.19.02551
59. Kohno T, Tabata J, Nakaoku T. REToma: a cancer subtype with a shared driver oncogene. Carcinogenesis (2020) 41:123–9. doi: 10.1093/carcin/bgz184
60. Elisei R, Romei C, Vorontsova T, Cosci B, Veremeychik V, Kuchinskaya E, et al. RET/PTC rearrangements in thyroid nodules: studies in irradiated and not irradiated, malignant and benign thyroid lesions in children and adults. J Clin Endocrinol Metab (2001) 86:3211–6. doi: 10.1210/jcem.86.7.7678
61. Fenton CL, Lukes Y, Nicholson D, Dinauer CA, Francis GL, Tuttle RM. The ret/PTC mutations are common in sporadic papillary thyroid carcinoma of children and young adults. J Clin Endocrinol Metab (2000) 85:1170–5. doi: 10.1210/jcem.85.3.6472
62. Romei C, Fugazzola L, Puxeddu E, Frasca F, Viola D, Muzza M, et al. Modifications in the papillary thyroid cancer gene profile over the last 15 years. J Clin Endocrinol Metab (2012) 97:E1758–1765. doi: 10.1210/jc.2012-1269
63. Leeman-Neill RJ, Kelly LM, Liu P, Brenner AV, Little MP, Bogdanova TI, et al. ETV6-NTRK3 is a common chromosomal rearrangement in radiation-associated thyroid cancer. Cancer (2014) 120:799–807. doi: 10.1002/cncr.28484
64. Musholt TJ, Musholt PB, Khaladj N, Schulz D, Scheumann GF, Klempnauer J. Prognostic significance of RET and NTRK1 rearrangements in sporadic papillary thyroid carcinoma. Surgery (2000) 128:984–93. doi: 10.1067/msy.2000.110845
65. Pfeifer A, Rusinek D, Zebracka-Gala J, Czarniecka A, Chmielik E, Zembala-Nozynska E, et al. Novel TG-FGFR1 and TRIM33-NTRK1 transcript fusions in papillary thyroid carcinoma. Genes Chromosomes Cancer (2019) 58:558–66. doi: 10.1002/gcc.22737
66. Hong DS, DuBois SG, Kummar S, Farago AF, Albert CM, Rohrberg KS, et al. Larotrectinib in patients with TRK fusion-positive solid tumours: a pooled analysis of three phase 1/2 clinical trials. Lancet Oncol (2020) 21:531–40. doi: 10.1016/S1470-2045(19)30856-3
Keywords: papillary thyroid carcinoma, phenotype, gene fusion, ETV6-NTRK3, NCOA4-RET
Citation: Zhou J, Wang W-R, Zhang H-F, Gao Q-Q, Wang W-B, Zhu J-H, Han Y-S, Chen J, Ma T-H, Zhang X-Y and Teng X-D (2023) Molecular and clinical features of papillary thyroid cancer in adult patients with a non-classical phenotype. Front. Endocrinol. 14:1138100. doi: 10.3389/fendo.2023.1138100
Received: 05 January 2023; Accepted: 28 March 2023;
Published: 12 April 2023.
Edited by:
Francesca Mancuso, University of Perugia, ItalyReviewed by:
Vicki E. Smith, University of Birmingham, United KingdomCopyright © 2023 Zhou, Wang, Zhang, Gao, Wang, Zhu, Han, Chen, Ma, Zhang and Teng. This is an open-access article distributed under the terms of the Creative Commons Attribution License (CC BY). The use, distribution or reproduction in other forums is permitted, provided the original author(s) and the copyright owner(s) are credited and that the original publication in this journal is cited, in accordance with accepted academic practice. No use, distribution or reproduction is permitted which does not comply with these terms.
*Correspondence: Xiao-Dong Teng, dGVuZzExMDIwNjlAemp1LmVkdS5jbg==
†These authors have contributed equally to this work and share first authorship
Disclaimer: All claims expressed in this article are solely those of the authors and do not necessarily represent those of their affiliated organizations, or those of the publisher, the editors and the reviewers. Any product that may be evaluated in this article or claim that may be made by its manufacturer is not guaranteed or endorsed by the publisher.
Research integrity at Frontiers
Learn more about the work of our research integrity team to safeguard the quality of each article we publish.