- 1Department of Fundamental Medicine, Chengdu University of Traditional Chinese Medicine, Chengdu, China
- 2School of Medical and Life Sciences/Reproductive & Women-Children Hospital, Chengdu University of Traditional Chinese Medicine, Chengdu, China
Introduction: A systematic review analysis was used to assess the profile of mitochondrial involvement in adipose tissue regulation and potential reagents to intervene in obesity through the mitochondrial pathway.
Methods: Three databases, PubMed, Web of Science, and Embase, were searched online for literature associated with mitochondria, obesity, white adipose tissue, and brown adipose tissue published from the time of their creation until June 22, 2022, and each paper was screened.
Results: 568 papers were identified, of which 134 papers met the initial selection criteria, 76 were selected after full-text review, and 6 were identified after additional searches. A full-text review of the included 82 papers was performed.
Conclusion: Mitochondria play a key role in adipose tissue metabolism and energy homeostasis, including as potential therapeutic agents for obesity.
1 Introduction
Adipose tissue, as a major energy storage site, is involved in physiological regulation of the whole body, including energy maintenance, insulin sensitivity, and food intake. Adipose tissue includes white adipose tissue (WAT), brown adipose tissue (BAT), and beige adipose tissue. Distinct fat depots have very unique biochemical properties and association with metabolic diseases (1). WAT stores excess energy as triglycerides (TG) and secretes adipokines such as leptin, TNF-α, and lipocalin, which contribute to the regulation of energy homeostasis (2), white adipocytes consist of single-compartment lipid droplets. BAT and beige adipose tissue are characterized by multi-compartment lipid droplets, high mitochondrial density and expression of uncoupling protein 1 (UCP1), and BAT consumes energy through non-fibrillatory thermogenesis by mitochondria (3). Adipose tissue is highly plastic and its restriction can drive pathological consequences such as obesity and metabolic diseases. As a risk factor for several metabolic diseases, obesity contributes to inflammation, insulin resistance, type 2 diabetes, non-alcoholic fatty liver disease and cardiovascular disease (4).
Mitochondria are organelles found in eukaryotes that are essential for energy metabolism and cellular homeostasis. Mitochondria have unique enzymes and systems that contribute to the citric acid cycle, fatty acid oxidation and oxidative phosphorylation (5). The mitochondria are essential to the differentiation of adipocytes (adipogenesis) and to major adipocyte functions (6). White adipocyte mitochondria are elongated and thin, which are involved in the production of ATP. Mitochondria of brown adipocytes have more quantity and larger body size compared to white adipocytes, and UCP1 located in the inner mitochondrial membrane can cause proton leakage across the inner membrane of the mitochondria, thus transforming the electrochemical energy into heat (7). The pathological expansion of the adipose tissue expansion is accompanied by the downregulation of mitochondrial oxidative pathways and changes in mitochondrial shape and number, ultimately leading to cell death (8–10). Mitochondrial dysfunction has deleterious effects on important adipocyte biology processes (lipid metabolism, adipocyte differentiation, insulin sensitivity, and thermogenesis), leading to metabolic diseases such as obesity and type 2 diabetes (11). Recent studies have shown that mitochondrial function can be improved by adding some herbal extracts and natural compounds in the diet, thereby inducing browning of WAT and adaptive thermogenesis of BAT to maintain metabolic homeostasis.
In this review, we focus on the specific role of mitochondria in adipocytes, summarize the mechanisms of mitochondrial regulation of adipose tissue, and discuss potential therapeutic agents from the perspective of treating obesity, which provide new insights for clinical treatment.
2 Research methods
2.1 Search strategy
The reporting items were created in strict accordance with the systematic review statement. All articles were retrieved from the PubMed, Embase and Web of Science databases. We used search words such as ‘Mitochondria’, ‘Obesity’, ‘Adipose Tissue, White’, and ‘Adipose Tissue, Brown’ in conjunction with Boolean operators. Within each concept, we combined subject words and free words with the ‘OR’ Boolean operator, and the four concepts were combined with the ‘AND’ Boolean operator. The specific search process can be found in the supplementary materials.
2.2 Study selection
We screened the titles and abstracts of each paper, and articles that were repeated and irrelevant were removed. The inclusion criteria were the mechanism of mitochondrial regulation of adipose tissue and potential reagents for obesity intervention through the mitochondrial pathway. Articles were excluded according to the following criteria: review articles, editorials, commentaries, conference abstracts, case reports, articles written in other languages, letters to the editor and articles not relevant to the main topic. A ‘PRISMA’ flow chart was used to document the selection process (Figure 1) (12).
3 Results
3.1 Mitochondria regulate adipocyte thermogenesis
Adipose tissue is one of the significant features in maintaining systemic energy homeostasis and insulin sensitivity, not only as a storehouse of excess energy substrates but also as a metabolic health sensor and regulator of energy storage and expenditure. Thermogenesis in adipose tissue is activated in a state of metabolic overload to rapidly utilize excess nutrients. BAT disrupt electron transport in the respiratory chain by activating UCP1 on the mitochondria, thereby preventing the production of ATP and converting energy into heat (13). In addition, mitochondria provide energy for thermogenesis due to efficient proton gradient generation during catabolic processes and electron transport chain (ETC) function (14). It has been reported that individuals with obesity have reduced adipocyte UCP1 expression and that activation of UCP1 improves obesity and metabolic complications. Therefore, targeting mitochondria to activate UCP1 is a strategy to treat obesity (15).
Sirtuin 3 (SIRT3) is a mitochondrially localized deacetylase (16) that belongs to the sirtuin family. Sirtuins are evolutionarily conserved deacetylases whose activity is dependent on NAD+ and have a variety of physiological functions, including the regulation of cell proliferation, DNA repair, antioxidant activity and mitochondrial energy homeostasis. One study reported that caloric restriction activated SIRT3 expression in WAT and BAT, and in BAT from genetically obese mice, SIRT3 was downregulated along with genes related to mitochondrial function, suggesting that SIRT3 activates mitochondrial function and contributes to adaptive thermogenesis in BAT (17, 18). The peroxisome biogenesis factor Pex16 (19), the angiogenic factor VEGF-A (20), disulfide-bond-A oxidoreductase-like protein (DsbA-L) (21), and PR domain-containing 16 (PRDM16) (22) may also regulate thermogenesis by regulating mitochondrial function.
The overexpression of hypoxia-inducible factor-1α (HIF-1α) in adipose tissue inhibits thermogenesis and cellular respiration in BAT and promotes weight gain in mice, which is associated with a reduction in oxygen consumption in BAT, while the decrease in oxygen consumption may be mediated by a reduction in mitochondria (23). Carbohydrate response element-binding protein (ChREBP) is one of the major transcription factors regulating lipogenesis (24). One study showed that overexpression of ChREBP-β reduced the expression of genes involved in mitochondrial biogenesis, autophagy and respiration, leading to a bleached phenotype of BAT (25), which suggested that ChREBP-β is a negative regulator of thermogenesis in BAT. Bone morphogenetic protein 7 (BMP7) has also been shown to upregulate UCP1 and increase adipocyte thermogenesis (26). The absence of the membrane-associated estrogen receptor G protein-coupled receptor 30 (GPR30) may promote BAT mitochondrial uncoupling of respiration (27), suggesting that it is a negative regulator of thermogenesis and contributes to reduced obesity. Optic atrophy protein 1 (OPA1) (28), Brain-derived neurotrophic factor (BDNF) (29), also plays an important role in the adaptive thermogenesis of BAT.
Fat burning relying on adaptive thermogenesis has emerged as a viable strategy to reduce obesity, and the activation of mitochondrial-localized UCP1 and related molecules targeting mitochondria becomes a potential driving force to execute this strategy. Therefore, mitochondria are an essential organelle for maintaining adipocyte metabolic homeostasis.
3.2 Activation of WAT browning and BAT whitening
Some WATs exhibit a BAT phenotype when exposed to certain stimuli, which is called “the browning of WAT”. WAT browning produces beige adipocytes, which exhibit UCP1-dependent thermogenesis, and fibroblast growth factor 21 (FGF21) was shown to play an important role in this thermogenesis (30). WAT browning has been found to suppress diet-induced obesity and improve systemic energy metabolism in many animal models (31, 32). Therefore, WAT browning has been investigated as an alternative therapy to BAT thermogenesis. Exercise is a major driver of fat browning. In this sense, the impact of myokines, which are factors secreted by the contracting muscle, on fat browning has provided a molecular mechanism to explain the benefits of exercise on weight loss and metabolic disease prevention. In this sense, several myokines act as positive (FNDC5/irisin, FNDC4, BAIBA and meteorin-like) and negative (myostatin) regulators of fat browning (33–37).
The role of mitochondria in WAT browning cannot be ignored. As an important factor of mitochondrial function, the expression of peroxisome prolilerators-activated receptor γ coactivator l α (PGC1α) was significantly increased in WAT of protein kinase Cβ (PKCβ) deficiency on profound obesity, double knockout (DBKO) mice (38). OPA1, a key protein for mitochondrial fusion, promotes browning of white adipocytes (39).Human white adipocyte mitochondrial activity is regulated by the ubiquitin carrier protein 9/microRNA-30a axis, which is involved in controlling white adipocyte browning (40). MiR-337-3p in adipocytes inhibits Twist1, a negative feedback regulator of BAT metabolism, and enhances adipocyte browning (41). It has also been found that the small molecule compounds RepSox (42), Endonuclease G (EndoG) (43), milk fat globule membrane (MFGM) and its components phosphatidylcholine (PC) (44), bone morphogenetic protein-4 (MBP4) (45), cannabinoid receptor type 1 (CB1R) (46), and E2F transcription factor 1(E2F1) (47) can be involved in the induction of WAT browning. Electroacupuncture (EA) has also been shown to remodel WAT to BAT by deacetylating SIRT-1-dependent peroxisome proliferator‐activated receptor gamma (PPARγ) and regulating the PGC1α-TFAM-UCP1 pathway in order to induce mitochondrial biogenesis (48). These appears to be potential strategies for the treatment of obesity through WAT browning.
The “whitening” of BAT is closely related to obesity-related BAT dysfunction. It is worth mentioning that the process of BAT whitening associated with obesity is reversible by cold exposure and bariatric surgery (49, 50). It is possible that inflammation in whitened BAT contributes to the typical inflammatory state found in obesity (49). Shimizu, I et al. (51) showed that vascular rarefaction results in mitochondrial dysfunction and loss in BAT, which is a noteworthy causal factor in the whitening of BAT in mice models and could affect obesity and obesity-linked diseases. Activation of mTOR signaling downregulated PGC1α, a key activator of mitochondrial biosynthesis, and nuclear respiratory factor 1 (NRF1), an important transcriptional regulator, and downregulated Mitofusin 2 (Mfn2) and OPA1, genes involved in mitochondrial dynamics (52), which may have led to the “whitening” of BAT, suggesting that inhibition of the mTOR signaling pathway is a potential therapeutic avenue for obesity.
Obesity is associated with reduced WAT browning and BAT thermogenesis, and some transcriptional regulators and signaling pathways can regulate WAT browning and BAT thermogenesis by improving mitochondrial quality control, suggesting that targeting mitochondrial regulation is an important entry point for obesity prevention and treatment (Figure 2).
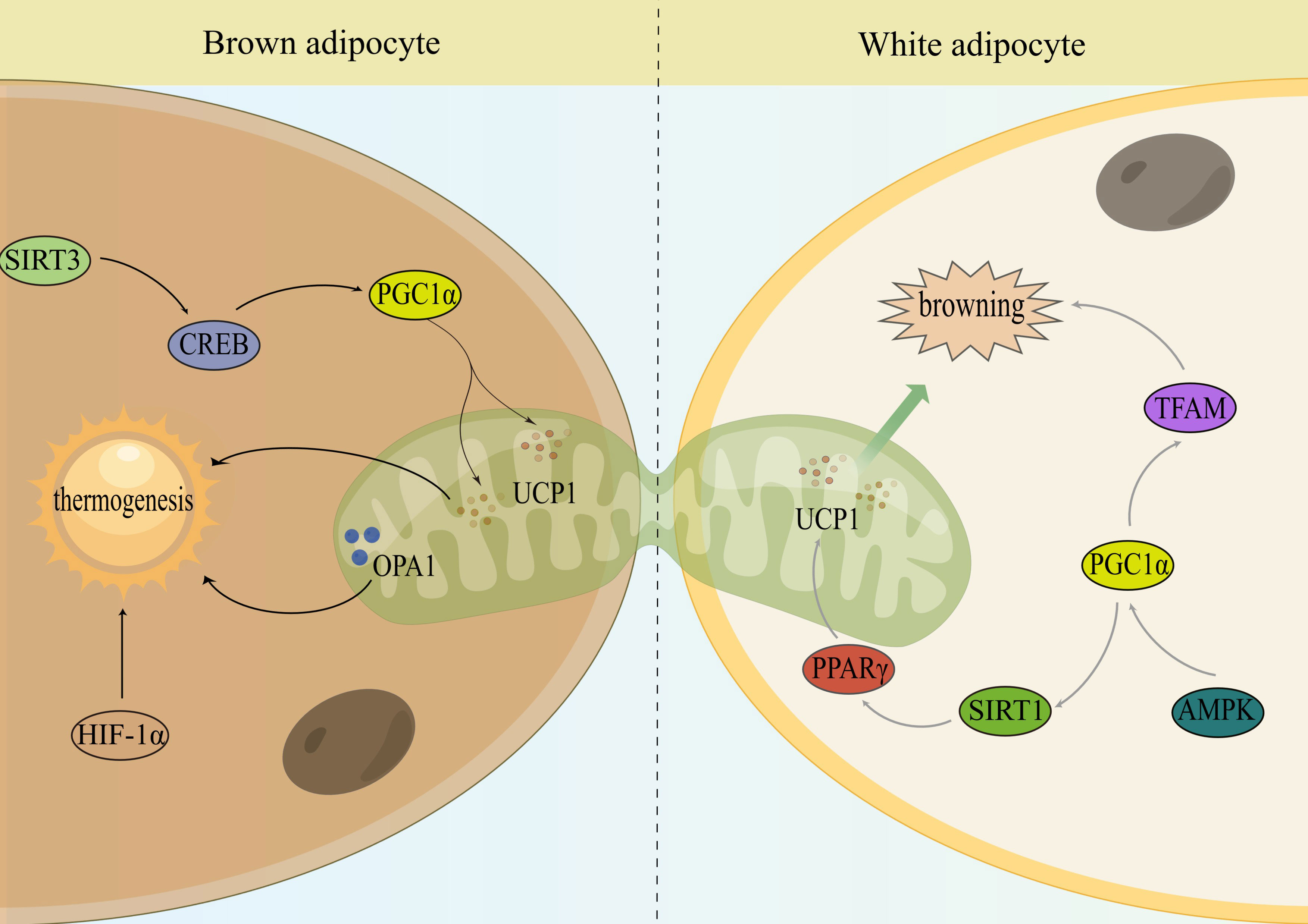
Figure 2 Mitochondria monitor the thermogenesis of brown and activate the browning of WAT. Sirtuin 3 (SIRT3); cAMP response element-binding protein (CREB); peroxisome proliferator-activated receptor-γ cofactor 1α (PGC1α); uncoupling protein-1 (UCP1); optic atrophy 1 (OPA1); hypoxia-inducible factor-1α (HIF-1α); peroxisome proliferator-activated receptor gamma (PPARγ); sirtuin 1 (SIRT1); AMP-activated protein kinase (AMPK); mitochondrial transcription factor A (TFAM).
3.3 Mitochondrial regulation of glucolipid metabolism in subjects with obesity
Mitochondrial dynamics in adipocytes may play a key role in initiating systemic metabolic dysregulation. Nutrient overload promotes hypoxia in BAT, which leads to whitening through mitochondrial dysfunction and loss, subsequently leading to impaired systemic glucose metabolism (53). Bean and coworkers (54) identified that the OPA1 gene regulates insulin sensitivity and adipose tissue functions, and controlled OPA1 overexpression in mice could reduce body weight, improve glucose metabolism and insulin sensitivity, reduce fat accumulation and promote browning of white adipocytes. Mfn2 is a gene that promotes mitochondrial fusion and mitochondrial-endoplasmic reticulum interactions. When Mfn2 is knocked down in adipocytes, adult mice consume more food, gain more fat, and have impaired glucose metabolism in standard diets (55). Mfn2 also plays a key role in the regulation of brown adipose tissue thermogenesis favouring mitochondria to lipid droplet interactions (56).
A dysfunctional mitochondrial system in WAT is implicated in obesity-related insulin resistance. OPA1 deletion completely prevented the increase in adiposity and improved insulin fate sensitivity in mice fed a high-fat diet (57). MLX interacting protein-like (MLXIPL) is a transcriptional regulator. MLXIPL-deficient mice are resistant to excessive lipid accumulation and heat-induced mitochondrial degradation in brown adipocytes (58). This result suggested that knockout of MLXPIL may be a potential therapeutic target for obesity-related metabolic diseases. Takaya et al. (59) found that the expression of UCP1 was increased in BAT-derived cultured preadipocytes and their local transplantation reduced inguinal fat pad weight. This finding suggests that local transplantation of BAT-derived preadipocytes may increase energy expenditure and thus reduce obesity. In the tricarboxylic acid cycle, pyruvate is transported to mitochondria by pyruvate carrier 1 (MPC1) to be oxidized to acetyl coenzyme A. Inhibition of MPC1 inhibits pyruvate transport and thus activates fatty acid oxidation (60), this suggests that MPC1 may be an important regulator of mitochondrial energy metabolism.
Mitochondria play a key role in the regulation of glucose utilization and lipid metabolism in adipocytes. By regulating mitochondrial biogenesis and mitochondrial dynamics in adipocytes, the development of obesity and metabolic diseases can be improved.
3.4 Other mitochondrial regulation approaches in obesity
Mitophagy is a process that selectively removes damaged mitochondria through a specialized form of autophagy and is essential for mitochondrial quality control (mitochondrial QC) and metabolic homeostasis. The mitochondrial autophagy receptor Fundc1 is a newly defined mitophagy receptor, and mice lacking Fundc1 develop more severe obesity and insulin resistance when fed a high-fat diet (HFD), and disruption of Fundc1 leads to impaired mitophagy and mitochondrial quality in WAT (61). PGC1α is essential for maintaining energy homeostasis. Overexpression of PGC1α in epicardial adipose tissue induces mitosis and improves mitochondrial function, resulting in improved adipose tissue quality (62). The deletion of mitochondrial transcription factor A (TFAM) in adipose tissue results in reduced mtDNA copy number, mitochondrial dysfunction, increased mitochondrial oxidation and positive metabolic effects (63, 64), suggesting that adipose tissue mitochondrial biosynthesis regulation may be a potential therapeutic target for the treatment of obesity.
The expression of PTEN-induced kinase 1 (PINK1), a protein involved in mitochondrial autophagic clearance, was upregulated in the WAT of HFD mice (65). Deletion of PINK1 induced BAT dysfunction, suggesting that the regulation of mitochondrial autophagy contributes to the “whitening” of adipose tissue during the development of obesity (66). The transition from beige to white adipocytes was associated with decreased mitochondria and increased autophagy (67), uncovering a mechanism by which autophagy-mediated mitochondrial clearance controls the maintenance of beige adipocytes, thus providing an opportunity to combat obesity.
Intercellular mitochondrial transfer can support the survival of cells with impaired metabolism. Rohatgi et al. (68) found that adipocytes and macrophages employ intercellular mitochondrial transfer as an immunometabolic crosstalk mechanism to regulate metabolic homeostasis, which is impaired in obesity. Borcherding et al. (69) showed the existence of a potential direct dietary mechanism on the basis of the above studies that could largely ameliorate mitochondrial translocation.
3.5 Mitochondrial targeting as a strategy to treat obesity
3.5.1 Herbal extracts and natural compounds
Increasing energy expenditure is a common approach to obesity prevention, and activating BAT may be a potential strategy against obesity. Beta vulgaris has been shown to have an anti-obesity effect by increasing UCP1 during nonshivering thermogenesis in brown adipocytes (70). Liu Z et al. (71) studied the effects of sesamol on disorders of adiposity and fat-related metabolism in mice fed a Western diet. They found that sesamol reduced WAT and BAT mass and adipocyte size by improving the expression of mitochondria-related genes, including PGC1α and UCP1. Jung Y et al. (72) investigated the anti-obesity effects of vanillic acid (VA) in vivo and in vitro and found that VA increased mitochondrial and thermogenesis-related factors such as UCP1 and PPARγ-1 in BAT and primary cultured brown adipocytes from mice, suggesting the potential of VA as a thermogenesis-activated anti-obesity agent.
Stimulating browning of white adipose cells helps to limit obesity and related metabolic disorders. Lactobacillus plantarum dy-1 (LFBE) can suppress obesity by enhancing thermogenic processes in BAT and browning of adipose tissue in the epithelium using a UCP1-dependent mechanism of activation (73). Averrhoa bilimbi can also induce adipocyte browning and enhance mitochondrial activity due to upregulation of UCP1 (74). Kang J et al. (75) studied the effects of secoisolariciresinol diglucoside (SDG) on WAT browning and found that SDG increased UCP1, PGC1α and PRDM16 in WAT and BAT in mice, as well as mitochondrial biogenesis and activation, suggesting that SDG is a potential candidate for ameliorating obesity and other metabolic disorders. Lycopene (LYC), one of the major carotenoids in tomatoes, has been used preclinically and clinically in the treatment of obesity and type 2 diabetes. LYC can induce browning and enhance mitochondrial respiration in white adipocytes and improve glucose and lipid metabolism by upregulating PPARγ (76).
The whitening of BAT during obesity and aging promotes metabolic disorders and related diseases. Gao P et al. (77) determined that the inhibitory effect of capsaicin on HFD-induced obesity and BAT whitening was dependent on the involvement of SIRT3, which could mediate the beneficial effects of capsaicin on attenuating reactive oxygen species production, increasing mitochondrial activity and limiting HFD-induced mitochondrial calcium overload.
Regulation of mitochondrial biogenesis is also a potential avenue for the treatment of obesity. The ethanolic extracts of both rutin and Platycodon grandiflorum (PG) can provide benefits for obesity by increasing the expression of genes involved in mitochondrial biogenesis (78, 79). α-Lipoic acid (α-LIP) is a naturally occurring antioxidant that promotes mitochondrial biogenesis and brown-like remodeling in cultured white subcutaneous adipocytes from donors with obesity (80). Marqués and colleagues (81) found that resveratrol has potential therapeutic effects in improving mitochondrial biogenesis (Table 1).
Some compounds can also activate mitochondrial biogenesis in skeletal muscle. Resveratrol was shown in 2006 to affect skeletal muscle mitochondrial biogenesis and thus metabolic homeostasis by decreasing PGC1α acetylation and increasing PGC1α activity (88). As a thermogenic tissue, skeletal muscle is also capable of regulating energy expenditure. When UCP1 is absent or non-shivering thermogenesis is affected, skeletal muscle generates heat through shivering and is supplied with energy by carbohydrates and lipids (89). Thermogenesis in skeletal muscle is dependent on the homologue of UCP1, uncoupling protein 3 (UCP3). 5,3’-Triiodo-L-thyronine (T3) was reported to induce UCP3 expression to regulate skeletal muscle thermogenesis (90). It is interesting to note that these compounds also work through similar pathways in BAT. Recruitment of BAT and skeletal muscle bear very high degree of similarities (91). Both organs are highly vascularized, neuralized, and contain abundant mitochondria (92). Studies by Bal et al. (93) have shown that skeletal muscle thermogenesis can be activated to compensate for the absence of BAT, suggesting that the two thermogenic systems can be functionally complementary. It has been shown that PRDM16 can control the bidirectional transformation of brown adipocytes and skeletal muscle cells (94), which may represent two different potential therapeutic targets to expand the thermogenic capacity of adipose tissue.
3.5.2 Traditional Chinese medicine and other alternative medicines
Zhang et al. (95) found that the natural antioxidant Lycium could enhance UCP1 expression, upregulate PGC1α and induce browning in white adipocytes, as well as enhance glucose uptake and oxidative utilization, lipolysis and fatty acid oxidation in 3T3-L1 adipocytes, and the application of Lycium is a promising strategy to combat obesity and obesity-related metabolic disorders. Huangqi San (HQS) is a traditional Chinese medicine formula, and Hao M et al. (96) found that it could significantly increase the number of mitochondria, increase the expression of UCP1 and PGC1α in BAT, and improve metabolic disorders and lipid deposition in hyperlipidemia in obese rats after 13 W intravenous injection of HQS, suggesting that HSQ is an effective drug for the treatment of hyperlipidemia with obesity. Tanshinone IIA (TAN2A) is a major active ingredient of the traditional Chinese medicine tanshinone, and tanshinone 20 (TAN20) is a derivative of TAN2A. Ma L et al. (97) showed that both TAN2A and TAN20 were able to increase mitochondrial content in adipose tissue, increase energy expenditure and reduce body weight, thereby improving insulin sensitivity and metabolic homeostasis in mouse models of obesity and diabetes. It has also been shown (98) that administration of tanshinone 1 (TAN1) prevents HFD-induced obesity in mice, which was associated with enhanced expression of brown adipocyte-related genes in WAT and BAT, and that TAN1 also led to increased mtDNA content and lipolysis.
Drugs with therapeutic potential can combat obesity by affecting the differentiation of white adipocytes. Ravaud C et al. (99) showed that HIV protease inhibitors (PIs) can reduce the expression of UCP1, improve mitochondrial function in brown adipocytes and regulate thermogenesis and are promising potential drugs against obesity. Empagliflozin can increase mitochondrial biogenesis and fusion, improve their function and promote browning of 3T3-L1 adipocytes (100), FAM134B improves adipocyte differentiation by enhancing mitophagy (101), and melatonin drives WAT browning (102), which are also promising anti-obesity treatments.
Targeting mitochondria as a therapeutic strategy for metabolic diseases is being extensively investigated as a new potential approach. In conclusion, the possibility of reducing obesity and metabolic diseases by mediating mitochondrial biogenesis or mitochondrial dynamics to increase thermogenesis, activate WAT brownout, and promote lipid metabolism and fatty acid oxidation is being progressively demonstrated.
3.6 Conclusion
The importance of mitochondria in energy metabolism and cellular homeostasis has been extensively noted and studied. Since the rediscovery of active brown and beige adipocytes in humans a decade ago, more and more research has focused on the regulation of adipocyte function by mitochondria. We summarized the searched articles and showed that mitochondria are involved in regulating the thermogenesis of BAT and increasing energy expenditure; in lipid homeostasis of adipose tissue and regulating glucose metabolism; and are also able to participate in inducing browning of WAT while promoting the whitening of BAT. These are key therapeutic targets for obesity-related metabolic diseases. The regulation of mitochondrial biogenesis, mitochondrial autophagy and mitochondrial translocation are also potential therapeutic opportunities for obesity. In fact, a series of studies are working on the interpretation of drugs or potential drugs for the treatment of obesity through these mitochondrial pathways.
In conclusion, mitochondria-targeted drugs for adipocytes are very promising. Therefore, understanding the molecular mechanisms underlying adipocyte mitochondrial dysfunction and the pathogenesis of obesity-related metabolic diseases is crucial for the development of new therapeutic approaches. However, future studies need to elucidate the mechanisms by which mitochondria undergo metabolic disorders to better account for their pathogenic role in metabolic diseases.
Data availability statement
The original contributions presented in the study are included in the article/Supplementary Material. further inquiries can be directed to the corresponding authors.
Author contributions
YZ and HongyaY conceived the research question. YZ wrote the first draft of the manuscript with the support of WD, YP and YG. HongY and NY discussed the results. All authors contributed to the article and approved the submitted version.
Funding
This research received the Natural Science Foundation of China (No. 82074151).
Acknowledgments
Ying Ning, Yan He, Yingxiu Mei and Yue Jin are acknowledged for their assistance in the writing of this article.
Conflict of interest
The authors declare that the research was conducted in the absence of any commercial or financial relationships that could be construed as a potential conflict of interest.
Publisher’s note
All claims expressed in this article are solely those of the authors and do not necessarily represent those of their affiliated organizations, or those of the publisher, the editors and the reviewers. Any product that may be evaluated in this article, or claim that may be made by its manufacturer, is not guaranteed or endorsed by the publisher.
Supplementary material
The Supplementary Material for this article can be found online at: https://www.frontiersin.org/articles/10.3389/fendo.2023.1132342/full#supplementary-material
References
1. Sahu B, Tikoo O, Pati B, Senapati U, Bal NC. Role of distinct fat depots in metabolic regulation and pathological implications. Rev Physiol Biochem Pharmacol (2023) 186:135–76. doi: 10.1007/112_2022_73
2. Bartelt A, Heeren J. Adipose tissue browning and metabolic health. Nat Rev Endocrinol (2014) 10(1):24–36. doi: 10.1038/nrendo.2013.204
3. Betz MJ, Enerback S. Human brown adipose tissue: what we have learned so far. Diabetes (2015) 64(7):2352–60. doi: 10.2337/db15-0146
4. Ali Khan A, Hansson J, Weber P, Foehr S, Krijgsveld J, Herzig S, et al. Comparative secretome analyses of primary murine white and brown adipocytes reveal novel adipokines*. Mol Cell Proteomics (2018) 17(12):2358–70. doi: 10.1074/mcp.RA118.000704
5. Ernster L, Schatz G. Mitochondria: a historical review. J Cell Biol (1981) 91(3 Pt 2):227s–55s. doi: 10.1083/jcb.91.3.227s
6. Li Q, Gao Z, Chen Y, Guan MX. The role of mitochondria in osteogenic, adipogenic and chondrogenic differentiation of mesenchymal stem cells. Protein Cell (2017) 8(6):439–45. doi: 10.1007/s13238-017-0385-7
7. Shabalina IG, Petrovic N, de Jong JM, Kalinovich AV, Cannon B, Nedergaard J. UCP1 in brite/beige adipose tissue mitochondria is functionally thermogenic. Cell Rep (2013) 5(5):1196–203. doi: 10.1016/j.celrep.2013.10.044
8. Heinonen S, Saarinen L, Naukkarinen J, Rodriguez A, Fruhbeck G, Hakkarainen A, et al. Adipocyte morphology and implications for metabolic derangements in acquired obesity. Int J Obes (Lond) (2014) 38(11):1423–31. doi: 10.1038/ijo.2014.31
9. Heinonen S, Muniandy M, Buzkova J, Mardinoglu A, Rodriguez A, Fruhbeck G, et al. Mitochondria-related transcriptional signature is downregulated in adipocytes in obesity: a study of young healthy MZ twins. Diabetologia (2017) 60(1):169–81. doi: 10.1007/s00125-016-4121-2
10. Ejarque M, Ceperuelo-Mallafre V, Serena C, Maymo-Masip E, Duran X, Diaz-Ramos A, et al. Adipose tissue mitochondrial dysfunction in human obesity is linked to a specific DNA methylation signature in adipose-derived stem cells. Int J Obes (Lond) (2019) 43(6):1256–68. doi: 10.1038/s41366-018-0219-6
11. Boudina S, Graham TE. Mitochondrial function/dysfunction in white adipose tissue. Exp Physiol (2014) 99(9):1168–78. doi: 10.1113/expphysiol.2014.081414
12. Page MJ, McKenzie JE, Bossuyt PM, et al. The PRISMA 2020 statement: An updated guideline for reporting systematic reviews. J Clin Epidemiol. (2021) 134:178–89. doi: 10.1016/j.jclinepi.2021.03.001
13. Zafrir B. Brown adipose tissue: research milestones of a potential player in human energy balance and obesity. Horm Metab Res (2013) 45(11):774–85. doi: 10.1055/s-0033-1348264
14. Hankir MK, Klingenspor M. Brown adipocyte glucose metabolism: a heated subject. EMBO Rep (2018) 19(9):1–13. doi: 10.15252/embr.201846404
15. Cypess AM, Kahn CR. Brown fat as a therapy for obesity and diabetes. Curr Opin Endocrinol Diabetes Obes (2010) 17(2):143–9. doi: 10.1097/MED.0b013e328337a81f
16. Lahiri T, Brambilla L, Andrade J, Askenazi M, Ueberheide B, Levy DE. Mitochondrial STAT3 regulates antioxidant gene expression through complex I-derived NAD in triple negative breast cancer. Mol Oncol (2021) 15(5):1432–49. doi: 10.1002/1878-0261.12928
17. Shi T, Wang F, Stieren E, Tong Q. SIRT3, a mitochondrial sirtuin deacetylase, regulates mitochondrial function and thermogenesis in brown adipocytes. J Biol Chem (2005) 280(14):13560–7. doi: 10.1074/jbc.M414670200
18. Giralt A, Hondares E, Villena JA, Ribas F, Díaz-Delfin J, Giralt M, et al. Sirt3, a mitochondrial sirtuin controlling brown adipose thermogenic activation. Obes Facts (2012) 5:23. doi: 10.1159/000171026
19. Park H, He A, Tan M, Johnson JM, Dean JM, Pietka TA, et al. Peroxisome-derived lipids regulate adipose thermogenesis by mediating cold-induced mitochondrial fission. J Clin Invest (2019) 129(2):694–711. doi: 10.1172/JCI120606
20. Mahdaviani K, Chess D, Wu Y, Shirihai O, Aprahamian TR. Autocrine effect of vascular endothelial growth factor-a is essential for mitochondrial function in brown adipocytes. Metabolism: Clin Exp (2016) 65(1):26–35. doi: 10.1016/j.metabol.2015.09.012
21. Bai J, Su X, Chen H, Cervantes C, Zhang C, Hu D, et al. Identification of DSBA-l as a key regulator of mitochondrial function, thermogenesis, and energy homeostasis. Diabetes (2015) 64:A88. doi: 10.2337/db151385
22. Kissig M, Ishibashi J, Harms MJ, Lim HW, Stine RR, Won KJ, et al. PRDM16 represses the type I interferon response in adipocytes to promote mitochondrial and thermogenic programing. EMBO J (2017) 36(11):1528–42. doi: 10.15252/embj.201695588
23. Jun JC, Devera R, Unnikrishnan D, Shin MK, Bevans-Fonti S, Yao Q, et al. Adipose HIF-1alpha causes obesity by suppressing brown adipose tissue thermogenesis. J Mol Med (Berl) (2017) 95(3):287–97. doi: 10.1007/s00109-016-1480-6
24. Tsai PJ, Chang ML, Hsin CM, Chuang CC, Chuang LT, Wu WH. Antilipotoxicity activity of osmanthus fragrans and chrysanthemum morifolium flower extracts in hepatocytes and renal glomerular mesangial cells. Mediators Inflammation (2017) 2017:4856095. doi: 10.1155/2017/4856095
25. Wei C, Ma X, Su K, Qi S, Zhu Y, Lin J, et al. ChREBP-beta regulates thermogenesis in brown adipose tissue. J Endocrinol (2020) 245(3):343–56. doi: 10.1530/JOE-19-0498
26. Shaw A, Tóth BB, Arianti R, Csomós I, Póliska S, Vámos A, et al. Bmp7 increases ucp1-dependent and independent thermogenesis with a unique gene expression program in human neck area derived adipocytes. Pharmaceuticals (2021) 14(11). doi: 10.3390/ph14111078
27. Luo J, Wang Y, Gilbert E, Liu D. Deletion of GPR30 drives the activation of mitochondrial uncoupling respiration to induce adipose thermogenesis in female mice. Front Endocrinol (2022) 13:877152. doi: 10.3389/fendo.2022.877152
28. Jiménez B, García G, Marqués P, González Blanco C, Burillo J, Guillén C, et al. Essential role of OPA-1 in the functionality of thermogenesis in the brown adipose tissue. Obes Facts (2021) 14(SUPPL 1):158. doi: 10.1159/000516203
29. Colitti M, Montanari T. Brain-derived neurotrophic factor modulates mitochondrial dynamics and thermogenic phenotype on 3T3-L1 adipocytes. Tissue Cell (2020) 66. doi: 10.1016/j.tice.2020.101388
30. Fisher FM, Kleiner S, Douris N, Fox EC, Mepani RJ, Verdeguer F, et al. FGF21 regulates PGC-1alpha and browning of white adipose tissues in adaptive thermogenesis. Genes Dev (2012) 26(3):271–81. doi: 10.1101/gad.177857.111
31. Vegiopoulos A, Muller-Decker K, Strzoda D, Schmitt I, Chichelnitskiy E, Ostertag A, et al. Cyclooxygenase-2 controls energy homeostasis in mice by de novo recruitment of brown adipocytes. Science (2010) 328(5982):1158–61. doi: 10.1126/science.1186034
32. Seale P, Conroe HM, Estall J, Kajimura S, Frontini A, Ishibashi J, et al. Prdm16 determines the thermogenic program of subcutaneous white adipose tissue in mice. J Clin Invest (2011) 121(1):96–105. doi: 10.1172/JCI44271
33. Bostrom P, Wu J, Jedrychowski MP, Korde A, Ye L, Lo JC, et al. A PGC1-alpha-dependent myokine that drives brown-fat-like development of white fat and thermogenesis. Nature (2012) 481(7382):463–8. doi: 10.1038/nature10777
34. Shan T, Liang X, Bi P, Kuang S. Myostatin knockout drives browning of white adipose tissue through activating the AMPK-PGC1alpha-Fndc5 pathway in muscle. FASEB J (2013) 27(5):1981–9. doi: 10.1096/fj.12-225755
35. Knudsen JG, Murholm M, Carey AL, Bienso RS, Basse AL, Allen TL, et al. Role of IL-6 in exercise training- and cold-induced UCP1 expression in subcutaneous white adipose tissue. PloS One (2014) 9(1):e84910. doi: 10.1371/journal.pone.0084910
36. Roberts LD, Bostrom P, O’Sullivan JF, Schinzel RT, Lewis GD, Dejam A, et al. Beta-aminoisobutyric acid induces browning of white fat and hepatic beta-oxidation and is inversely correlated with cardiometabolic risk factors. Cell Metab (2014) 19(1):96–108. doi: 10.1016/j.cmet.2013.12.003
37. Fruhbeck G, Fernandez-Quintana B, Paniagua M, Hernandez-Pardos AW, Valenti V, Moncada R, et al. FNDC4, a novel adipokine that reduces lipogenesis and promotes fat browning in human visceral adipocytes. Metabolism (2020) 108:154261. doi: 10.1016/j.metabol.2020.154261
38. Huang W, Bansode RR, Bal NC, Mehta M, Mehta KD. Protein kinase cbeta deficiency attenuates obesity syndrome of ob/ob mice by promoting white adipose tissue remodeling. J Lipid Res (2012) 53(3):368–78. doi: 10.1194/jlr.M019687
39. Bean C, Audano M, Varanita T, Favaretto F, Medaglia M, Gerdol M, et al. The mitochondrial protein Opa1 promotes adipocyte browning that is dependent on urea cycle metabolites. Nat Metab (2021) 3(12):1633–47. doi: 10.1038/s42255-021-00497-2
40. Koh EH, Chen Y, Bader DA, Hamilton MP, He B, York B, et al. Mitochondrial activity in human white adipocytes is regulated by the ubiquitin carrier protein 9/microRNA-30a axis. J Biol Chem (2016) 291(47):24747–55. doi: 10.1074/jbc.M116.749408
41. Vonhogen I, El AH, Olieslagers S, Vasilevich A, de Boer J, Tinahones FJ, et al. MiR-337-3p promotes adipocyte browning by inhibiting TWIST1. Cells (2020) 9(4):1–16. doi: 10.3390/cells9041056
42. Tu WZ, Fu YB, Xie X. RepSox, a small molecule inhibitor of the TGFbeta receptor, induces brown adipogenesis and browning of white adipocytes. Acta Pharmacol Sin (2019) 40(12):1523–31. doi: 10.1038/s41401-019-0264-2
43. Pardo R, Blasco N, Vila M, Beiroa D, Nogueiras R, Canas X, et al. EndoG knockout mice show increased brown adipocyte recruitment in white adipose tissue and improved glucose homeostasis. Endocrinology (2016) 157(10):3873–87. doi: 10.1210/en.2015-1334
44. Li T, Du M, Wang H, Mao X. Milk fat globule membrane and its component phosphatidylcholine induce adipose browning both in vivo and in vitro. J Nutr Biochem (2020) 81:108372. doi: 10.1016/j.jnutbio.2020.108372
45. Gustafson B, Hammarstedt A, Hedjazifar S, Hoffmann JM, Svensson PA, Grimsby J, et al. BMP4 and BMP antagonists regulate human white and beige adipogenesis. Diabetes (2015) 64(5):1670–81. doi: 10.2337/db14-1127
46. Perwitz N, Wenzel J, Wagner I, Büning J, Drenckhan M, Zarse K, et al. Cannabinoid type 1 receptor blockade induces transdifferentiation towards a brown fat phenotype in white adipocytes. Diabetes Obes Metab (2010) 12(2):158–66. doi: 10.1111/j.1463-1326.2009.01133.x
47. Xiong M, Hu W, Tan Y, Yu H, Zhang Q, Zhao C, et al. Transcription factor E2F1 knockout promotes mice white adipose tissue browning through autophagy inhibition. Front IN Physiol (2021) 12:748040. doi: 10.3389/fphys.2021.748040
48. Tang Q, Lu M, Xu B, Wang Y, Lu S, Yu Z, et al. Electroacupuncture regulates inguinal white adipose tissue browning by promoting sirtuin-1-Dependent PPARgamma deacetylation and mitochondrial biogenesis. Front Endocrinol (Lausanne) (2020) 11:607113. doi: 10.3389/fendo.2020.607113
49. Kotzbeck P, Giordano A, Mondini E, Murano I, Severi I, Venema W, et al. Brown adipose tissue whitening leads to brown adipocyte death and adipose tissue inflammation. J Lipid Res (2018) 59(5):784–94. doi: 10.1194/jlr.M079665
50. Fruhbeck G, Mendez-Gimenez L, Becerril S, Ramirez B, Hernandez-Pardos AW, Cienfuegos JA, et al. Increased aquaporin-7 expression is associated with changes in rat brown adipose tissue whitening in obesity: impact of cold exposure and bariatric surgery. Int J Mol Sci (2023) 24(4):1–17. doi: 10.3390/ijms24043412
51. Shimizu I, Walsh K. The whitening of brown fat and its implications for weight management in obesity. Curr Obes Rep (2015) 4(2):224–9. doi: 10.1007/s13679-015-0157-8
52. Huwatibieke B, Yin W, Liu L, Jin Y, Xiang X, Han J, et al. Mammalian target of rapamycin signaling pathway regulates mitochondrial quality control of brown adipocytes in mice. Front Physiol (2021) 12:638352. doi: 10.3389/fphys.2021.638352
53. Shimizu I, Aprahamian T, Kikuchi R, Shimizu A, Papanicolaou KN, MacLauchlan S, et al. Vascular rarefaction mediates whitening of brown fat in obesity. J Clin Invest (2014) 124(5):2099–112. doi: 10.1172/JCI71643
54. Bean C, Varanita T, Favaretto F, Vettor R. The mitochondria and cristae shaping protein Opa1 impinges on fat browning to control insulin sensitivity. Obes Rev (2016) 17:15. doi: 10.1111/obr.12398
55. Mancini G, Pirruccio K, Yang X, Bluher M, Rodeheffer M, Horvath TL. Mitofusin 2 in mature adipocytes controls adiposity and body weight. Cell Rep (2019) 26(11):2849–2858.e4. doi: 10.1016/j.celrep.2019.02.039
56. Boutant M, Kulkarni SS, Joffraud M, Ratajczak J, Valera-Alberni M, Combe R, et al. Mfn2 is critical for brown adipose tissue thermogenic function. EMBO J (2017) 36(11):1543–58. doi: 10.15252/embj.201694914
57. Pereira RO, White J, Fang S, Olvera A, Hewezi R, McGlauflin R, et al. Mice lacking OPA1 in adipose tissue are resistant to diet-induced obesity and insulin resistance. Circulation (2017) 136.
58. Schlein C, Fischer AW, Worthmann A, Schaltenberg N, John C, Lynes M, et al. Mlxipl controls brown adipose tissue whitening. Med Genet.-Berlin (2019) 31(1):106. doi: 10.1007/s11825-019-0234-6
59. Takaya K, Matsuda N, Asou T, Kishi K. Brown preadipocyte transplantation locally ameliorates obesity. Arch Plast Surg (2021) 48(4):440–7. doi: 10.5999/aps.2020.02257
60. Zou S, Zhu L, Huang K, Luo H, Xu W, He X. Adipose tissues of MPC1± mice display altered lipid metabolism-related enzyme expression levels. PeerJ (2021) 6:e5799. doi: 10.7717/peerj.5799
61. Wu H, Wang Y, Li W, Chen H, Du L D, Wang X, et al. Chen: Deficiency of mitophagy receptor FUNDC1 impairs mitochondrial quality and aggravates dietary-induced obesity and metabolic syndrome. Autophagy (2019) 15(11):1882–98. doi: 10.1080/15548627.2019.1596482
62. Waldman M, Alex R, Kornowski R, Hochhauser E, Arad M, Abraham NG. Adipocyte pgc1-α and nov/ccn3: novel targets in the management of obesity-linked cardiomyopathy. Circulation (2021) 144(SUPPL 1). doi: 10.1161/circ.144.suppl-1.13903
63. Vernochet C, Mourier A, Bezy O, MacOtela Y, Boucher J, Rardin MJ, et al. Adipose-specific deletion of TFAM increases mitochondrial oxidation and protects mice against obesity and insulin resistance. Cell Metab (2012) 16(6):765–76. doi: 10.1016/j.cmet.2012.10.016
64. Vernochet C, Bezy O, Macotela Y, Emanuelli B, Larson NG, Kahn CR. Deletion of the mitochondrial transcription factor a (Tfam) in adipose tissue protects mice from age-and diet-induced obesity and insulin resistance. Endocr Rev (2011) 32(3). doi: 10.1210/endo-meetings.2011.PART4.OR3.OR31-3
65. Hill BG, Cummins TD, Sansbury BE, Holden CR, Bhatnagar A. Metabolic remodeling of white adipose tissue in obesity. Free Radical Bio Med (2012) 53:S93. doi: 10.1016/j.freeradbiomed.2012.10.167
66. Ko MS, Yun JY, Baek IJ, Jang JE, Hwang JJ, Lee SE, et al. Mitophagy deficiency increases NLRP3 to induce brown fat dysfunction in mice. Autophagy (2021) 17(5):1205–21. doi: 10.1080/15548627.2020.1753002
67. Altshuler-Keylin S, Shinoda K, Hasegawa Y, Ikeda K, Hong H, Kang Q, et al. Beige adipocyte maintenance is regulated by autophagy-induced mitochondrial clearance. Cell Metab (2016) 24(3):402–19. doi: 10.1016/j.cmet.2016.08.002
68. Brestoff JR, Wilen CB, Moley JR, Li Y, Zou W, Malvin NP, et al. Intercellular mitochondria transfer to macrophages regulates white adipose tissue homeostasis and is impaired in obesity. Cell Metab (2021) 33(2):270–282.e8. doi: 10.1016/j.cmet.2020.11.008
69. Borcherding N, Moley J, Field R, Brestoff JR. Mapping cell-to-cell mitochondria transfer in obesity using high-dimensional spectral flow cytometry. Am J Clin Pathol (2021) 156(SUPPL 1):S3–4. doi: 10.1093/ajcp/aqab189.005
70. Otieno D, Lee SG, Kang HW. Beetroot extract increases the expression of uncoupling protein 1 in primary brown adipocytes in vitro. FASEB J (2016) 30. doi: 10.1096/fasebj.30.1_supplement.691.36
71. Lin C, Chen J, Hu M, Zheng W, Song Z, Qin H. Sesamol promotes browning of white adipocytes to ameliorate obesity by inducing mitochondrial biogenesis and inhibition mitophagy via beta 3-AR/PKA signaling pathway. Food Nutr Res (2021) 65:1–12. doi: 10.29219/fnr.v65.7577
72. Jung Y, Park J, Kim HL, Sim JE, Youn DH, Kang J, et al. Vanillic acid attenuates obesity via activation of the AMPK pathway and thermogenic factors in vivo and in vitro. FASEB J (2018) 32(3):1388–402. doi: 10.1096/fj.201700231RR
73. Xiao X, Bai J, Li MS, Zhang JY, Sun XJ, Dong Y. Supplementation of fermented barley extracts with lactobacillus plantarum dy-1 inhibits obesity via a UCP1-dependent mechanism. Biomed Environ sciences: BES (2019) 32(8):578–91. doi: 10.3967/bes2019.076
74. Lau WK, Noruddin NAA, Ariffin AH, Mahmud MZ, Noor MHM, Amanah A, et al. Novel discovery of averrhoa bilimbi ethanolic leaf extract in the stimulation of brown fat differentiation program in combating diet-induced obesity. BMC Complem Altern M (2019) 19(1):243. doi: 10.1186/s12906-019-2640-3
75. Kang J, Park J, Park WY, Jiao W, Lee S, Jung Y, et al. A phytoestrogen secoisolariciresinol diglucoside induces browning of white adipose tissue and activates non-shivering thermogenesis through AMPK pathway. Pharmacol Res (2020) 158:104852. doi: 10.1016/j.phrs.2020.104852
76. Zhu R, Wei J, Liu H, Liu C, Wang L, Chen B, et al. Lycopene attenuates body weight gain through induction of browning via regulation of peroxisome proliferator-activated receptor γ in high-fat diet-induced obese mice. J Nutr Biochem (2020) 78:1–13. doi: 10.1016/j.jnutbio.2019.108335
77. Gao P, Jiang Y, Wu H, Sun F, Li Y, He H, et al. Inhibition of mitochondrial calcium overload by SIRT3 prevents obesity-or age-related whitening of brown adipose tissue. Diabetes (2020) 69(2):165–80. doi: 10.2337/db19-0526
78. Seo S, Oh S, Shin Y, Jung S, Kim Y. Reduction of body weight by rutin is associated with an increase of brown adipose tissue mitochondrial biogenesis in high-fat diet induced obese rat. FASEB J (2014) 28(1). doi: 10.1096/fasebj.28.1_supplement.lb430
79. Kim H, Park J, Park H, Jung Y, Youn D, Kang J, et al. Platycodon grandiflorum a. de candolle ethanolic extract inhibits adipogenic regulators in 3T3-L1 cells and induces mitochondrial biogenesis in primary brown preadipocytes. J Agr Food Chem (2015) 63(35):7721–30. doi: 10.1021/acs.jafc.5b01908
80. Fernández-Galilea M, Pérez-Matute P, Prieto-Hontoria PL, Houssier M, Burrell MA, Langin D, et al. α-lipoic acid treatment increases mitochondrial biogenesis and promotes beige adipose features in subcutaneous adipocytes from overweight/obese subjects. Biochim Biophys Acta - Mol Cell Biol Lipids (2015) 1851(3):273–81. doi: 10.1016/j.bbalip.2014.12.013
81. Marqués P, Martínez H, Jiménez B, Burillo J, González-Blanco C, García G, et al. Resveratrol enhanced cold-induced thermogenesis through a differential browning effect on the adipose organ in a mouse model showing atrophied interscapular adipose tissue: a therapeutic approach. Obes Rev (2020) 21(SUPPL 1). doi: 10.1111/obr.13115
82. Overby H, Zu Y, Wang S, Zhao L. Nanoparticles encapsulated with resveratrol induce browning of white adipocytes. FASEB J (2017) 31(1).
83. Liu Z, Qiao Q, Sun Y, Chen Y, Ren B, Liu X. Sesamol ameliorates diet-induced obesity in C57BL/6J mice and suppresses adipogenesis in 3T3-L1 cells via regulating mitochondria-lipid metabolism. Mol Nutr Food Res (2017) 61(8). doi: 10.1002/mnfr.201600717
84. Zhang F, Ai W, Hu X, Meng Y, Yuan C, Su H, et al. Phytol stimulates the browning of white adipocytes through the activation of AMP-activated protein kinase (AMPK) α in mice fed high-fat diet. Food Funct (2018) 9(4):2043–50. doi: 10.1039/C7FO01817G
85. Chen Y, Liu H, Lee T. Ursodeoxycholic acid regulates hepatic energy homeostasis and white adipose tissue macrophages polarization in leptin-deficiency obese mice. CELLS (2019) 8(3):1–24. doi: 10.3390/cells8030253
86. Nederveen JP, Manta K, Bujak AL, Simone AC, Fuda MR, Nilsson MI, et al. A novel multi-ingredient supplement activates a browning program in white adipose tissue and mitigates weight gain in high-fat diet-fed mice. Nutrients (2021) 13(11):1–22. doi: 10.3390/nu13113726
87. Yau WW, Singh BK, Lesmana R, Zhou J, Sinha RA, Wong KA, et al. Thyroid hormone (T3) stimulates brown adipose tissue activation via mitochondrial biogenesis and MTOR-mediated mitophagy. Autophagy (2019) 15(1):131–50. doi: 10.1080/15548627.2018.1511263
88. Lagouge M, Argmann C, Gerhart-Hines Z, Meziane H, Lerin C, Daussin F, et al. Resveratrol improves mitochondrial function and protects against metabolic disease by activating SIRT1 and PGC-1alpha. Cell (2006) 127(6):1109–22. doi: 10.1016/j.cell.2006.11.013
89. Rowland LA, Bal NC, Kozak LP, Periasamy M. Uncoupling protein 1 and sarcolipin are required to maintain optimal thermogenesis, and loss of both systems compromises survival of mice under cold stress. J Biol Chem (2015) 290(19):12282–9. doi: 10.1074/jbc.M115.637603
90. Lombardi A, Moreno M, de Lange P, Iossa S, Busiello RA, Goglia F. Regulation of skeletal muscle mitochondrial activity by thyroid hormones: focus on the “old” triiodothyronine and the “emerging” 3,5-diiodothyronine. Front Physiol (2015) 6:237. doi: 10.3389/fphys.2015.00237
91. Gorrell E, Shemery A, Kowalski J, Bodziony M, Mavundza N, Titus AR, et al. Skeletal muscle thermogenesis induction by exposure to predator odor. J Exp Biol (2020) 223(Pt 8). doi: 10.1242/jeb.218479
92. Pani S, Dey S, Pati B, Senapati U, Bal NC. Brown to white fat transition overlap with skeletal muscle during development of larger mammals: is it a coincidence? J Endocr Soc (2022) 6(12):bvac151. doi: 10.1210/jendso/bvac151
93. Bal NC, Singh S, Reis F, Maurya SK, Pani S, Rowland LA, et al. Both brown adipose tissue and skeletal muscle thermogenesis processes are activated during mild to severe cold adaptation in mice. J Biol Chem (2017) 292(40):16616–25. doi: 10.1074/jbc.M117.790451
94. Seale P, Bjork B, Yang W, Kajimura S, Chin S, Kuang S, et al. PRDM16 controls a brown fat/skeletal muscle switch. Nature (2008) 454(7207):961–7. doi: 10.1038/nature07182
95. Zhang HQ, Chen SY, Wang AS, Yao AJ, Fu JF, Zhao JS, et al. Sulforaphane induces adipocyte browning and promotes glucose and lipid utilization. Mol Nutr Food Res (2016) 60(10):2185–97. doi: 10.1002/mnfr.201500915
96. Hao M, Guan Z, Gao Y, Xing J, Zhou X, Wang C, et al. Huang-Qi San ameliorates hyperlipidemia with obesity rats via activating brown adipocytes and converting white adipocytes into brown-like adipocytes. Phytomedicine (2020) 78. doi: 10.1016/j.phymed.2020.153292
97. Ma L, Zhao Z, Guo X, Li J, Xu L, Mei W, et al. Tanshinone IIA and its derivative activate thermogenesis in adipocytes and induce “beiging” of white adipose tissue. Mol Cell Endocrinol (2022) 544. doi: 10.1016/j.mce.2022.111557
98. Jung DY, Suh N, Jung MH. Tanshinone 1 prevents high fat diet-induced obesity through activation of brown adipocytes and induction of browning in white adipocytes. Life Sci (2022) 298. doi: 10.1016/j.lfs.2022.120488
99. Ravaud C, Paré M, Yao X, Azoulay S, Mazure NM, Dani C, et al. Resveratrol and HIV-protease inhibitors control UCP1 expression through opposite effects on p38 MAPK phosphorylation in human adipocytes. J Cell Physiol (2020) 235(2):1184–96. doi: 10.1002/jcp.29032
100. Xu L, Xu C, Liu X, Li X, Li T, Yu X, et al. Empagliflozin induces white adipocyte browning and modulates mitochondrial dynamics in KK cg-Ay/J mice and mouse adipocytes. Front Physiol (2021) 12:745058. doi: 10.3389/fphys.2021.745058
101. Cai M, Zhao J, Liu Q, Wang X, Wang Y. FAM134B improves preadipocytes differentiation by enhancing mitophagy. Biochim Biophys Acta - Mol Cell Biol Lipids (2019) 1864(12). doi: 10.1016/j.bbalip.2019.08.004
Keywords: obesity, mitochondria, white adipose tissue (WAT), brown adipose tissue (BAT), UCP1
Citation: Zheng Y, Yang N, Pang Y, Gong Y, Yang H, Ding W and Yang H (2023) Mitochondria-associated regulation in adipose tissues and potential reagents for obesity intervention. Front. Endocrinol. 14:1132342. doi: 10.3389/fendo.2023.1132342
Received: 27 December 2022; Accepted: 24 May 2023;
Published: 16 June 2023.
Edited by:
Endre Károly Kristóf, University of Debrecen, HungaryCopyright © 2023 Zheng, Yang, Pang, Gong, Yang, Ding and Yang. This is an open-access article distributed under the terms of the Creative Commons Attribution License (CC BY). The use, distribution or reproduction in other forums is permitted, provided the original author(s) and the copyright owner(s) are credited and that the original publication in this journal is cited, in accordance with accepted academic practice. No use, distribution or reproduction is permitted which does not comply with these terms.
*Correspondence: Hongya Yang, eWh5NjAxOEAxNjMuY29t; Weijun Ding, ZGluZ3dlaWp1bkBjZHV0Y20uZWR1LmNu