- 1Division of Endocrinology and Metabolism, Department of Internal Medicine, CHA Gangnam Medical Center, CHA University School of Medicine, Seoul, Republic of Korea
- 2Division of Endocrinology and Metabolism, Department of Internal Medicine, Catholic Kwandong University College of Medicine, International St. Mary’s Hospital, Incheon, Republic of Korea
- 3Department of diabetes mellitus, Biomedical Research Center, Severance Hospital, Yonsei University College of Medicine, Seoul, Republic of Korea
- 4Division of Endocrinology and Metabolism, Department of Internal Medicine, Yonsei University College of Medicine, Seoul, Republic of Korea
Aims: The ketogenic pathway is an effective mechanism by which the liver disposes of fatty acids (FAs) to the peripheral tissues. Impaired ketogenesis is presumed to be related to the pathogenesis of metabolic-associated fatty liver disease (MAFLD), but the results of previous studies have been controversial. Therefore, we investigated the association between ketogenic capacity and MAFLD in subjects with type 2 diabetes (T2D).
Methods: A total of 435 subjects with newly diagnosed T2D was recruited for the study. They were classified into two groups based on median serum β-hydroxybutyrate (β-HB) level: intact vs. impaired ketogenesis groups. The associations of baseline serum β-HB and MAFLD indices of hepatic steatosis index, NAFLD liver fat score (NLFS), Framingham Steatosis index (FSI), Zhejian University index, and Chinese NAFLD score were investigated.
Results: Compared to the impaired ketogenesis group, the intact ketogenesis group showed better insulin sensitivity, lower serum triglyceride level, and higher low-density lipoprotein-cholesterol and glycated hemoglobin levels. Serum levels of liver enzymes were not different between the two groups. Of the hepatic steatosis indices, NLFS (0.8 vs. 0.9, p=0.045) and FSI (39.4 vs. 47.0: p=0.041) were significantly lower in the intact ketogenesis group. Moreover, intact ketogenesis was significantly associated with lower risk of MAFLD as calculated by FSI after adjusting for potential confounders (adjusted odds ratio 0.48, 95% confidence interval 0.25-0.91, p=0.025).
Conclusions: Our study suggests that intact ketogenesis might be associated with decreased risk of MAFLD in T2D.
Introduction
Non-alcoholic fatty liver disease (NAFLD) is a liver condition ranging from simple steatosis to inflammation or fibrosis in the absence of excessive alcohol consumption (1). Hepatic triglycerides (TGs) accumulate in the liver mainly through de novo lipogenesis or delivery of fatty acids (FAs) from peripheral tissues in the NAFLD or insulin resistant settings (2, 3). Recently, metabolic-associated fatty liver disease (MAFLD) was suggested as a term that more accurately reflects the pathogenesis of this type of fatty liver disease (4).
In the normal liver, excess delivered FAs undergo β-oxidation, yielding acetyl-CoA (5). The majority of acetyl-CoA is condensed in the ketogenic pathway to form ketone bodies, mostly β-hydroxybutyrate (βHB) and acetoacetate, which are exported to the peripheral tissues and are utilized as efficient fuels. Through β-oxidation and the ketogenic pathway, the normal liver has considerable capacity to dispose of delivered FAs (6).
In this context, it can be expected that ketogenesis is relatively downregulated in patients with MAFLD. Studies have reported that ketogenesis is suppressed in patients with fatty liver disease (7–9). One previous study using isotope tracers reported that acetyl-CoA oxidation through the tricarboxyclic acid (TCA) cycle was upregulated in MAFLD patients, whereas the ketogenic pathway from acetyl-CoA was markedly reduced (7). This suggested that impaired ketogenesis from acetyl-CoA in the liver might be important in the development of MAFLD. However, clinical data on the association between ketogenesis and MAFLD are controversial, as there were also previous studies that showed increased ketone levels in patients with fatty liver disease (10, 11).
In the present study, we aimed to investigate whether impaired ketogenesis is related to MAFLD in patients with type 2 diabetes (T2D). To this end, we recruited newly diagnosed T2D patients and investigated the association between ketogenic capacity based on blood βHB level and MAFLD status using various indices of fatty liver disease.
Methods
Study design and population
For this retrospective cross-sectional study, we registered the study subject on the basis of our previous studies (12, 13). Since 2009, we have built a cohort, the diabetes registry of Severance Diabetes Center (a tertiary care hospital in Seoul, Korea) with the patients who underwent a standardized mixed-meal stimulation test on their first visit to our diabetes center. The electronic medical records of patients from April 2017 to March 2022 were reviewed. In the registry protocol, we routinely collected blood samples at 0 and 90 minutes (basal and stimulated, respectively) for glucose, insulin, and C-peptide analyses. Inclusion criteria were patients aged ≥19 years with newly diagnosed T2D based on the 2019 Korean Diabetes Association guidelines (14) and measured serum βHB, which has been available at our center since 2017 from the initial visit. We excluded patients who had undergone organ transplantation or chemotherapy, steroid users, patients who had taken antidiabetic drugs prior to initial blood sampling, and those who visited the emergency room due to hyperglycemia.
A total of 435 patients was ultimately included for analysis in this study. Subjects were classified into two groups based on median initial serum βHB level: those with sufficient ketogenic capacity (intact ketogenesis group) and those without (impaired ketogenesis group).
This study was approved by the independent institutional review board of Severance Hospital (4-2022-1101).
Measurements and variables
Patient demographics and clinical and biochemical measurements were collected during the study period. The variables gathered were age, sex, body mass index (BMI), use of an antidiabetic drug, hypertension, and blood chemistry.
MAFLD was assessed using previous validated liver steatosis prediction models: hepatic steatosis index (HSI), NAFLD liver fat score (NLFS), Framingham Steatosis Index (FSI), Zhejian University (ZJU) index, and Chinese NAFLD score. The equations are described in Supplementary Table 1. HSI and NLFS are well-validated models to detect hepatic steatosis in the general population (15). FSI is a clinical model that includes metabolic parameters such as BMI, diabetes diagnosis, and good discrimination in the National Health and Nutrition Examination Survey III cohort (16). The diagnostic performance of the ZJU index and Chinese NAFLD score was verified in several studies, especially in an Asian cohort including Japanese and Chinese subjects (17, 18).
Serum βHB, the most abundant form of ketone body, was measured before initiating diabetes medication to determine the subject’s ketogenic capacity. Fasting serum βHB concentration was assessed by an enzymatic assay using a commercial reagent from Landox Laboratories Ltd. (County Antrim, UK) and an Atellica CH 930 analyzer (Siemens Healthcare Diagnostics, Marburg, Germany). βHB values measured below the lower limit of the assay were expressed as 0. Blood samples for measuring glucometabolic parameters including serum glucose, insulin, and cholesterol in the fasting state were obtained after overnight fasting. Low-density lipoprotein cholesterol (LDL-C) levels were calculated using Friedewald’s equation in cases of subjects without actual LDL-C measurements, if their blood TG levels were below 400 mg/dL (19). Postprandial insulin level was also measured 90 minutes after the mixed-meal test (Mediwell Diabetic Meal; Meail Dairies Co., Yeongdong-gun, Chungbuk, Korea). The estimated glomerular filtration rate (eGFR) was calculated based on the four-variable Modification of Diet in Renal Disease study equation. Insulin resistance and pancreatic β-cell function were assessed using the homeostasis model assessment of insulin resistance (HOMA-IR) index and HOMA- β.
Statistical analysis
Glucometabolic parameters and biomarker-based indices to assess hepatic steatosis were compared between the intact ketogenesis and impaired ketogenesis groups. Normally distributed continuous variables were presented as mean (standard deviation), and non-normal continuous variables were presented as median (interquartile range [IQR]). The normality of continuous variables was assessed by the Shapiro-Wilk test. Categorical variables were presented as number with percentage (%). The difference between groups was evaluated using Student’s t-test for continuous variables with normal distribution and Mann-Whitney U-test for continuous variables with non-normal distribution. The frequencies of categorical variables were compared using Pearson’s Chi-square test. The correlation between serum βHB level and each liver steatosis prediction model was assessed by Spearman’s correlation coefficient, which is a statistical method for non-normally distributed variables. In addition, we performed regression analysis to evaluate the clinical significance of initial βHB level for prediction of hepatic steatosis. Logistic regression analysis, a statistical technique used to predict the relationship between independent variables and a binary dependent variable was performed with MAFLD occurrence based on the cut-off values of each MAFLD indices as a dependent variable. The ketogenic capacity used as independent variable in the logistic regression analysis was defined as being divided into intact/impaired based on the median serum βHB level. In the adjusted model, age, sex, BMI, glycated hemoglobin (HbA1c), low-density lipoprotein cholesterol (LDL-C), HOMA-IR, and HOMA-β were adjusted. P-values <0.05 were considered statistically significant. Statistical analyses were performed using R software version 3.6.3 (R Project for Statistical Computing, Vienna, Austria).
Results
Clinical and laboratory characteristics of patients
The baseline characteristics of 435 newly diagnosed T2D patients categorized according to level of βHB are shown in Table 1. Subjects were divided into intact (n = 226) and impaired (n = 209) ketogenesis group. The median age of the study subjects was 54 years (IQR, 44 to 63) and 62.8% were men. The median serum βHB level was 0.11 (0.0-0.2) mmol/L. Compared to the impaired ketogenesis group, patients with intact ketogenesis were significantly younger and showed non-significantly lower BMI. There were no significant differences in aspartate aminotransferase, alanine aminotransferase, and eGFR between the two groups. Fasting blood glucose level and HbA1c level was significantly higher in the intact group than the impaired group. Moreover, the intact ketogenesis group showed markedly lower fasting and postprandial insulin levels as well as lower HOMA-β and HOMA-IR, suggesting decreased insulin secretory function, better insulin sensitivity, and poor glycemic control. The blood level of TG was lower and LDL-C was higher in the intact ketogenesis group.
The values of the five hepatic steatosis indices of the two groups are described in Figure 1. Overall, the intact ketogenesis group showed a tendency to lower hepatic steatosis indices compared to the impaired ketogenesis group. However, significant differences between the groups were found only for NLFS and FSI. In NLFS, the index values of the intact ketogenesis group and impaired ketogenesis group were 0.8 vs. 0.9 (p=0.045), and the index value of FSI in groups was 39.4 vs. 47.0 (p=0.041). Additionally, the incidence of MAFLD, based on the cut-off values, was lower in the intact ketogenesis group than in the impaired ketogenesis group. Based on assessment by FSI, 165 (73.0%) subjects fulfilled the definition of MAFLD in the intact ketogenesis group whereas 171 (81.8%) subjects in the impaired ketogenesis group (p=0.038). According to the Chinese NAFLD score, 133 (58.8%) in the intact group vs. 144 (68.9%) in the impaired group, indicating a significant difference between the two groups (p=0.038).
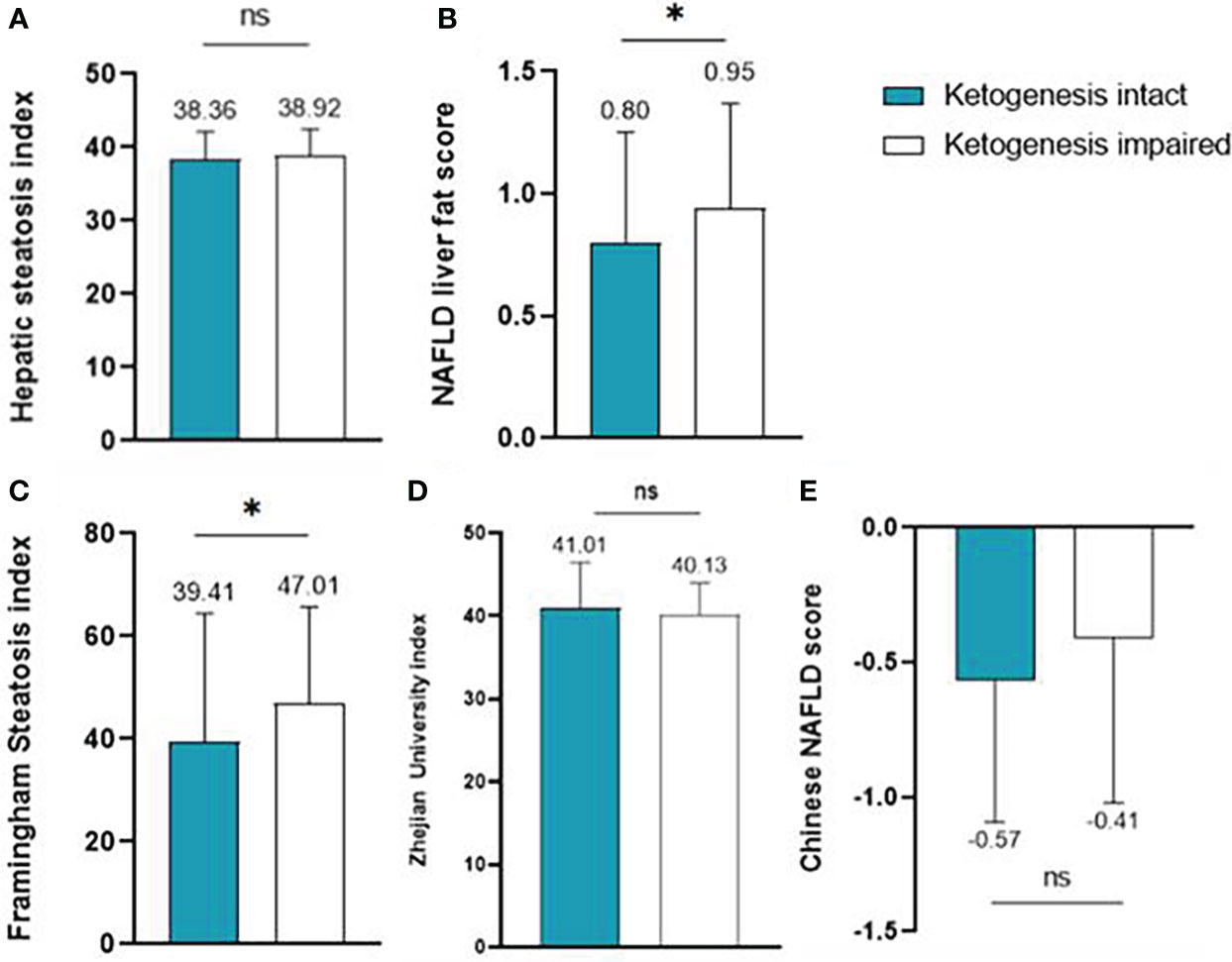
Figure 1 Liver steatosis indices of the intact ketogenesis group and the impaired ketogenesis group: (A) Hepatic steatosis index, (B) NAFLD liver fat score, (C) Framingham Steatosis Index, (D) Zhejian University index, and (E) Chinese NAFLD score. ns, statistically not significant (P-value ≥ 0.05)*, statistically significant (P-value < 0.05).
Serum βHB level is correlated with lower hepatic steatosis indices
The correlations between serum βHB level and the hepatic steatosis indices were analyzed using Spearman’s correlation coefficient (Table 2). There was a significant negative correlation between serum βHB level and hepatic steatosis as assessed by NLFS (r= -0.103, p=0.032) and FSI (r= -0.106, p=0.028). A negative correlation was observed between βHB level and hepatic steatosis based on the other indices, but the relationship was not statistically significant.
Ketogenic capacity is associated with risk of MAFLD incidence
Multivariable logistic regression analysis of the relationship between MAFLD and ketogenic capacity is shown in Table 3. To determine the independence of ketogenic capacity as a risk factor for MAFLD, multivariable logistic regression analysis was performed adjusting for covariates of age, sex, BMI, HbA1c, LDL-C, HOMA-IR, and HOMA-β. The analysis showed ketogenic capacity to maintain a significant association with MAFLD as calculated by FSI ([Odds ratio, 0.48; 95% confidence interval 0.25 to 0.91; P=0.025]. With other hepatic steatosis indices, ketogenic capacity tended to be associated with MAFLD incidence, but the relationship was not statistically significant.
Discussion
In this cross-sectional study, we focused on ketogenic capacity and MAFLD with glucometabolic disorders in patients with T2D. We found that T2D patients with intact ketogenesis showed better hepatic steatosis indices, especially FSI and NLFS. After multivariable logistic regression analysis, ketogenic capacity showed significance in predicting the extent of MAFLD as calculated by FSI. Most of the hepatic steatosis indices other than FSI tended to be correlated with ketogenic capacity but did not show significance in regression analysis. This may be because our study included the large number of newly diagnosed and drug-naïve T2D patients with mild or early stage of MAFLD, as shown in the normal mean liver enzyme levels and slightly increased hepatic steatosis indices. In addition, since the variables used in each index are different, it is believed that differences in sensitivity may have occurred.
In the current study, subjects with intact ketogenesis showed significantly lower insulin resistance without a significant obesity difference compared to those with impaired ketogenesis. The association between insulin resistance and MAFLD has already been well established, and it is thought to have influenced on the pathogenesis of MAFLD in the impaired ketogenesis group. In addition, relative hyperinsulinemia in the impaired ketogenesis group might be due not only to insulin resistance but also to lower hepatic insulin clearance, which has been previously reported to be associated with MAFLD (20). With respect to dyslipidemia in intact ketogenic patients with T2D, higher level of LDL-C and lower TG level were observed in the intact ketogenesis group. The most common pattern of dyslipidemia in T2D is hypertriglyceridemia and reduced HDL cholesterol level. T2D itself does not significantly increase level of LDL-C, but the small dense LDL particles are increased in T2D (21). As a possible explanation for the lipid profile of the intact ketogenesis group, it might be assumed that the pathway in which acetyl-CoA is converted to acetoacetyl-CoA during ketogenesis to result in cholesterol synthesis is more dominant than the pathway in which acetyl-CoA is converted to malonyl-CoA to produce TG in the T2D patients with intact ketogenesis (5, 6).
Ketogenesis or ketone body has been of interest since studies have shown that ketogenesis is associated with better metabolic outcomes. To apply this notion to lifestyle modification, ketogenic, low-carbohydrate diets have been reported to have a significant weight loss effect as well as a glucose-lowering effect (22–24). The ketogenic diet has also demonstrated its beneficial effects on MAFLD (25). In addition, some researchers have reported that subjects with intact ketogenesis, who can produce sufficient ketone bodies during fasting periods, have better metabolic features or clinical outcomes than those who cannot. A cross-sectional study using health check-up data showed that the presence of ketonuria after fasting was associated with metabolic superiority, such as lower body weight, waist circumstance, blood pressure, and blood glucose level (26). In a longitudinal prospective study, healthy individuals with spontaneous fasting ketonuria had a lower risk of incident diabetes (27). However, discrepant reports have been made on the association between ketogenesis and fatty liver disease. A longitudinal study using a cohort of 153,076 nondiabetic Korean subjects reported that fasting ketonuria was associated with reduced risk of incident hepatic steatosis (28). Similarly, a cross-sectional study reported that fasting ketonuria imparted reduced risk for advanced liver fibrosis in MAFLD patients (29). In contrast, Dutch researchers analyzed cohort data and reported that subjects with suspected fatty liver disease had higher blood levels of ketone bodies (11). Several previous studies showed that ketone levels were increased in patients with prediabetes or diabetes (30, 31), which was closely related to fatty liver disease. The result of our study in drug-naïve T2D patients is in line with previous studies that reported lower risk of steatosis or fibrosis in nondiabetic subjects with intact ketogenesis (28, 29).
The pathophysiology or mechanism by which intact ketogenesis in patients with T2D might be protective against MAFLD has not been elucidated, although candidate mechanisms have been proposed. First, in the liver, ketogenesis is an efficient pathway for disposal of FAs from peripheral lipolysis. FAs delivered to the liver are converted to acetyl-CoA through β-oxidation, and ketogenesis is the non-oxidative pathway that converts the acetyl-CoA into energy and disposes of it to the peripheral tissues (5, 6). When the ketogenic pathway is impaired, acetyl-CoA is oxidized to CO2 in the TCA cycle; researchers in the United States reported that the alternative TCA cycle was upregulated via fasting,while ketogenesis was impaired in patients with fatty liver disease (7). Unlike the ketogenic pathway, the TCA cycle promotes gluconeogenesis/de novo lipogenesis and increases hepatic oxygen consumption. This would cause steatosis and oxidative stress on the liver. As a result, when the ketogenic pathway is impaired, the effective pathway for disposal of FAs in the liver is impaired, and the materials for de novo lipogenesis increase along with oxidative stress. Second, the ketogenic process induces hepatic peroxisome proliferation-activated receptor α (PPARα) and fibroblast growth factor 21 (FGF21) action that is important for hepatic lipid metabolism (32–34). The PPARα-FGF21 axis plays a critical role in metabolism across a broad spectrum of organs as well as lipid metabolism in the liver (33, 35). Dysregulation of this axis is associated with pathogenesis of MAFLD (35, 36). Therefore, induction of the PPARα-FGF21 axis in a ketogenic state could decrease steatosis. FGF21 signaling also activates hepatic autophagy (35), in which reduction is a significant process in the pathogenesis of MAFLD (37).
Despite the supportive results of our study, several limitations should be considered. First, our study was a cross-sectional analysis with a relatively small sample size. Therefore, an association between ketogenesis and MAFLD could not be concluded. In addition, characteristics of the intact ketogenesis group, such as higher blood glucose levels and the reduced insulin secretory function, may have acted as prerequisites for the ketogenic ability. From that point of view, ketogenesis may act as a compensatory action in T2D patients with decreased insulin secretion. Due to the limitations of a cross-sectional study, this study cannot suggest the causal relationships and mechanisms clearly. Further studies with larger populations and longitudinal design are needed to collect more evidence on this issue. Second, the study did not use imaging modalities such as ultrasonography, computed tomography, or transient elastography to evaluate the presence or extent of MAFLD. Further studies using these modalities are needed. Third, we did not have data on acetoacetate or acetone because there is no measurable method for them in our center. Therefore, we evaluated ketogenic potency with serum βHB, which is the most abundant form of ketone bodies. In the pathogenesis of MAFLD, not only the metabolic component but also the genetic component are important, and the genetic aspect is mainly expressed in the form of hepatic redox state (38). The βHB/acetoacetate ratio is often used as a marker that reflects hepatic mitochondrial redox state but due to the lack of measurement of acetoacetate, we could not use this marker in our study. If this marker can be included in the analysis in the future, it is considered that the mechanistic explanation of our findings will be more clear. In addition, our study included only T2D patients. Therefore, care must be used when applying the results of this study to a nondiabetic population. However, since previous studies showing the association between ketogenesis and MAFLD were conducted on nondiabetic subjects, this limitation also shows the originality and clinical significance of this study. To the best of our knowledge, this study is the first to report the relationship of ketogenic capacity and hepatic steatogenic status in patients with T2D.
Conclusions
In T2D patients, intact ketogenesis is associated with better MAFLD indices. Previous findings suggesting that ketogenesis is an efficient pathway for the liver to dispose of FAs, induces the PPARα-FGF21 axis, and reduces oxidative stress are considered to support the results. Large-scale prospective studies using imaging modalities are needed in the future.
Data availability statement
The raw data supporting the conclusions of this article will be made available by the authors, without undue reservation.
Ethics statement
The studies involving human participants were reviewed and approved by the Institutional review board of Severance Hospital. Written informed consent for participation was not required for this study in accordance with the national legislation and the institutional requirements.
Author contributions
SL, JB, and B-WL designed the research. DJ contributed to acquisition of data. SL, JB, and B-WL analyzed the data. SL and JB wrote the article. ML, Y-hL, EK, B-SC, and B-WL contributed to revision of the manuscript. All authors contributed to the article and approved the submitted version.
Conflict of interest
The authors declare that the research was conducted in the absence of any commercial or financial relationships that could be construed as a potential conflict of interest.
Publisher’s note
All claims expressed in this article are solely those of the authors and do not necessarily represent those of their affiliated organizations, or those of the publisher, the editors and the reviewers. Any product that may be evaluated in this article, or claim that may be made by its manufacturer, is not guaranteed or endorsed by the publisher.
Supplementary material
The Supplementary Material for this article can be found online at: https://www.frontiersin.org/articles/10.3389/fendo.2023.1124576/full#supplementary-material
References
1. Diehl AM, Day C. Cause, pathogenesis, and treatment of nonalcoholic steatohepatitis. N Engl J Med (2017) 377(21):2063–72. doi: 10.1056/NEJMra1503519
2. Lambert JE, Ramos–Roman MA, Browning JD, Parks EJ. Increased De novo lipogenesis is a distinct characteristic of individuals with nonalcoholic fatty liver disease. Gastroenterology (2014) 146(3):726–35. doi: 10.1053/j.gastro.2013.11.049
3. Sunny Nishanth E, Parks Elizabeth J, Browning Jeffrey D, Burgess Shawn C. Excessive hepatic mitochondrial tca cycle and gluconeogenesis in humans with nonalcoholic fatty liver disease. Cell Metab (2011) 14(6):804–10. doi: 10.1016/j.cmet.2011.11.004
4. Eslam M, Sanyal AJ, George J. Mafld: A consensus-driven proposed nomenclature for metabolic associated fatty liver disease. Gastroenterology (2020) 158(7):1999–2014.e1. doi: 10.1053/j.gastro.2019.11.312
5. McGarry JD, Foster DW. Regulation of ketogenesis and clinical aspects of the ketotic state. Metabolism: Clin Exp (1972) 21(5):471–89. doi: 10.1016/0026-0495(72)90059-5
6. Balasse EO. Kinetics of ketone body metabolism in fasting humans. Metabolism: Clin Exp (1979) 28(1):41–50. doi: 10.1016/0026-0495(79)90166-5
7. Fletcher JA, Deja S, Satapati S, Fu X, Burgess SC, Browning JD. Impaired ketogenesis and increased acetyl-coa oxidation promote hyperglycemia in human fatty liver. JCI Insight (2019) 5(11):e127737. doi: 10.1172/jci.insight.127737
8. Inokuchi T, Orita M, Imamura K, Takao T, Isogai S. Resistance to ketosis in moderately obese patients: Influence of fatty liver. Internal Med (Tokyo Japan) (1992) 31(8):978–83. doi: 10.2169/internalmedicine.31.978
9. Mey JT, Erickson ML, Axelrod CL, King WT, Flask CA, McCullough AJ, et al. B-hydroxybutyrate is reduced in humans with obesity-related nafld and displays a dose-dependent effect on skeletal muscle mitochondrial respiration in vitro. Am J Physiol Endocrinol Metab (2020) 319(1):E187–e95. doi: 10.1152/ajpendo.00058.2020
10. Bugianesi E, Gastaldelli A, Vanni E, Gambino R, Cassader M, Baldi S, et al. Insulin resistance in non-diabetic patients with non-alcoholic fatty liver disease: Sites and mechanisms. Diabetologia (2005) 48(4):634–42. doi: 10.1007/s00125-005-1682-x
11. Post A, Garcia E, van den Berg EH, Flores-Guerrero JL, Gruppen EG, Groothof D, et al. Nonalcoholic fatty liver disease, circulating ketone bodies and all-cause mortality in a general population-based cohort. Eur J Clin Invest (2021) 51(12):e13627. doi: 10.1111/eci.13627
12. Lee EY, Hwang S, Lee SH, Lee YH, Choi AR, Lee Y, et al. Postprandial c-peptide to glucose ratio as a predictor of B-cell function and its usefulness for staged management of type 2 diabetes. J Diabetes Investig (2014) 5(5):517–24. doi: 10.1111/jdi.12187
13. Lee SH, Lee BW, Won HK, Moon JH, Kim KJ, Kang ES, et al. Postprandial triglyceride is associated with fasting triglyceride and homa-ir in Korean subjects with type 2 diabetes. Diabetes Metab J (2011) 35(4):404–10. doi: 10.4093/dmj.2011.35.4.404
14. Kim MK, Ko SH, Kim BY, Kang ES, Noh J, Kim SK, et al. 2019 Clinical practice guidelines for type 2 diabetes mellitus in Korea. Diabetes Metab J (2019) 43(4):398–406. doi: 10.4093/dmj.2019.0137
15. European Association for the Study of the Liver (EASL), European Association for the Study of Diabetes (EASD), European Association for the Study of Obesity (EASO). Easl-Easd-Easo clinical practice guidelines for the management of non-alcoholic fatty liver disease. J Hepatol (2016) 64(6):1388–402. doi: 10.1016/j.jhep.2015.11.004
16. Long MT, Pedley A, Colantonio LD, Massaro JM, Hoffmann U, Muntner P, et al. Development and validation of the framingham steatosis index to identify persons with hepatic steatosis. Clin Gastroenterol Hepatol (2016) 14(8):1172–80.e2. doi: 10.1016/j.cgh.2016.03.034
17. Wang J, Xu C, Xun Y, Lu Z, Shi J, Yu C, et al. Zju index: A novel model for predicting nonalcoholic fatty liver disease in a Chinese population. Sci Rep (2015) 5:16494. doi: 10.1038/srep16494
18. Xia MF, Yki-Järvinen H, Bian H, Lin HD, Yan HM, Chang XX, et al. Influence of ethnicity on the accuracy of non-invasive scores predicting non-alcoholic fatty liver disease. PloS One (2016) 11(8):e0160526. doi: 10.1371/journal.pone.0160526
19. Friedewald WT, Levy RI, Fredrickson DS. Estimation of the concentration of low-density lipoprotein cholesterol in plasma, without use of the preparative ultracentrifuge. Clin Chem (1972) 18(6):499–502. doi: 10.1093/clinchem/18.6.499
20. Najjar SM, Caprio S, Gastaldelli A. Insulin clearance in health and disease. Annu Rev Physiol (2022) 85:363–81. doi: 10.1146/annurev-physiol-031622-043133
21. Arca M, Pigna G, Favoccia C. Mechanisms of diabetic dyslipidemia: Relevance for atherogenesis. Curr Vasc Pharmacol (2012) 10(6):684–6. doi: 10.2174/157016112803520864
22. Hussain TA, Mathew TC, Dashti AA, Asfar S, Al-Zaid N, Dashti HM. Effect of low-calorie versus low-carbohydrate ketogenic diet in type 2 diabetes. Nutr (Burbank Los Angeles County Calif) (2012) 28(10):1016–21. doi: 10.1016/j.nut.2012.01.016
23. Saslow LR, Daubenmier JJ, Moskowitz JT, Kim S, Murphy EJ, Phinney SD, et al. Twelve-month outcomes of a randomized trial of a moderate-carbohydrate versus very low-carbohydrate diet in overweight adults with type 2 diabetes mellitus or prediabetes. Nutr Diabetes (2017) 7(12):304. doi: 10.1038/s41387-017-0006-9
24. Choi YJ, Jeon SM, Shin S. Impact of a ketogenic diet on metabolic parameters in patients with obesity or overweight and with or without type 2 diabetes: A meta-analysis of randomized controlled trials. Nutrients (2020) 12(7):2005. doi: 10.3390/nu12072005
25. Luukkonen PK, Dufour S, Lyu K, Zhang XM, Hakkarainen A, Lehtimäki TE, et al. Effect of a ketogenic diet on hepatic steatosis and hepatic mitochondrial metabolism in nonalcoholic fatty liver disease. Proc Natl Acad Sci USA (2020) 117(13):7347–54. doi: 10.1073/pnas.1922344117
26. Joo NS, Lee DJ, Kim KM, Kim BT, Kim CW, Kim KN, et al. Ketonuria after fasting may be related to the metabolic superiority. J Korean Med Sci (2010) 25(12):1771–6. doi: 10.3346/jkms.2010.25.12.1771
27. Kim G, Lee SG, Lee BW, Kang ES, Cha BS, Ferrannini E, et al. Spontaneous ketonuria and risk of incident diabetes: A 12 year prospective study. Diabetologia (2019) 62(5):779–88. doi: 10.1007/s00125-019-4829-x
28. Kim Y, Chang Y, Kwon MJ, Hong YS, Kim MK, Sohn W, et al. Fasting ketonuria and the risk of incident nonalcoholic fatty liver disease with and without liver fibrosis in nondiabetic adults. Am J Gastroenterol (2021) 116(11):2270–8. doi: 10.14309/ajg.0000000000001344
29. Lim K, Kang M, Park J. Association between fasting ketonuria and advanced liver fibrosis in non-alcoholic fatty liver disease patients without prediabetes and diabetes mellitus. Nutrients (2021) 13(10):3400. doi: 10.3390/nu13103400
30. Mahendran Y, Vangipurapu J, Cederberg H, Stancáková A, Pihlajamäki J, Soininen P, et al. Association of ketone body levels with hyperglycemia and type 2 diabetes in 9,398 Finnish men. Diabetes (2013) 62(10):3618–26. doi: 10.2337/db12-1363
31. Saasa V, Beukes M, Lemmer Y, Mwakikunga B. Blood ketone bodies and breath acetone analysis and their correlations in type 2 diabetes mellitus. Diagnostics (Basel Switzerland) (2019) 9(4):224. doi: 10.3390/diagnostics9040224
32. Badman MK, Pissios P, Kennedy AR, Koukos G, Flier JS, Maratos-Flier E. Hepatic fibroblast growth factor 21 is regulated by pparalpha and is a key mediator of hepatic lipid metabolism in ketotic states. Cell Metab (2007) 5(6):426–37. doi: 10.1016/j.cmet.2007.05.002
33. Piccinin E, Moschetta A. Hepatic-specific pparα-Fgf21 action in nafld. Gut (2016) 65(7):1075–6. doi: 10.1136/gutjnl-2016-311408
34. Zhang Y, Lei T, Huang JF, Wang SB, Zhou LL, Yang ZQ, et al. The link between fibroblast growth factor 21 and sterol regulatory element binding protein 1c during lipogenesis in hepatocytes. Mol Cell Endocrinol (2011) 342(1-2):41–7. doi: 10.1016/j.mce.2011.05.003
35. Byun S, Seok S, Kim YC, Zhang Y, Yau P, Iwamori N, et al. Fasting-induced Fgf21 signaling activates hepatic autophagy and lipid degradation Via Jmjd3 histone demethylase. Nat Commun (2020) 11(1):807. doi: 10.1038/s41467-020-14384-z
36. Li H, Fang Q, Gao F, Fan J, Zhou J, Wang X, et al. Fibroblast growth factor 21 levels are increased in nonalcoholic fatty liver disease patients and are correlated with hepatic triglyceride. J Hepatol (2010) 53(5):934–40. doi: 10.1016/j.jhep.2010.05.018
37. Czaja MJ. Function of autophagy in nonalcoholic fatty liver disease. Digestive Dis Sci (2016) 61(5):1304–13. doi: 10.1007/s10620-015-4025-x
Keywords: ketogenesis, β-hydroxybutyrate, steatosis, MAFLD, diabetes
Citation: Lee S, Bae J, Jo DR, Lee M, Lee Y-h, Kang ES, Cha B-S and Lee B-W (2023) Impaired ketogenesis is associated with metabolic-associated fatty liver disease in subjects with type 2 diabetes. Front. Endocrinol. 14:1124576. doi: 10.3389/fendo.2023.1124576
Received: 15 December 2022; Accepted: 09 February 2023;
Published: 22 February 2023.
Edited by:
Ping Wang, College of Human Medicine, Michigan State University, United StatesReviewed by:
Rick I. Meijer, Radboud University Medical Centre, NetherlandsJerry Raymond Colca, Metabolic Solutions Development Company, United States
Copyright © 2023 Lee, Bae, Jo, Lee, Lee, Kang, Cha and Lee. This is an open-access article distributed under the terms of the Creative Commons Attribution License (CC BY). The use, distribution or reproduction in other forums is permitted, provided the original author(s) and the copyright owner(s) are credited and that the original publication in this journal is cited, in accordance with accepted academic practice. No use, distribution or reproduction is permitted which does not comply with these terms.
*Correspondence: Byung-Wan Lee, YndhbmxlZUB5dWhzLmFj
†These authors have contributed equally to this work and share first authorship